- 1CIMMYT-Borlaug Institute for South Asia (BISA), Samastipur, Bihar, India
- 2ICAR-Indian Agricultural Research Institute, Regional Station, Samastipur, Bihar, India
- 3Bihar Agricultural University, Bhagalpur, Bihar, India
- 4Dr. Rajendra Prasad Central Agricultural University, Samastipur, Bihar, India
- 5Krishi Vigyan Kendra, Tingachhiya, Katihar, Bihar, India
- 6Krishi Vigyan Kendra, Harnaut, Nalanda, Bihar, India
- 7Krishi Vigyan Kendra, Jalalgarh, Purnea, Bihar, India
- 8Krishi Vigyan Kendra, Bikramganj, Rohtas, Bihar, India
- 9Veer Kunwar Singh College of Agriculture, Buxar BAU, Buxar, Bihar, India
- 10Bhola Paswan Shastri Agricultural College, Purnea, BAU, Purnea, Bihar, India
- 11ICAR-Research Complex for Eastern Region, Patna, Bihar, India
- 12Department of Agriculture, Government of Bihar, Patna, Bihar, India
Maize is an economically important cereal crop, whose adaptability to a variety of agroecological zones and uses as a food, feed, and input in various industries has ensured its global importance. In this study, the implications of the raised bed planting (RBP) system on smallholder maize farming in Bihar, India, for four consecutive Rabi seasons were evaluated from 2020–2021 to 2023–2024. The research focuses on key parameters such as productivity, profitability, water use efficiency (WUE), and nutrient use efficiency (NUE) to present a sustainable alternative to traditional flatbed planting systems. Maize yield at RBP ranged from 9.28 to 10.53 t ha−1, significantly higher than the range of 5.70 to 8.29 t ha−1 for flatbed (FB). The raised bed planting (RBP) system was more profitable as well, where net return increased by 20% compared to the FB system. WUE was 35% better in the RBP system, and NUE (grain yield per unit of applied N) was 25% better than FB systems. RBP enhances water and nutrient (nitrogen, phosphorus, and potassium) use efficiency, boosting productivity and profitability.
1 Introduction
Maize (Zea mays L.) is a highly adaptable and resilient cereal crop that can thrive in a wide range of conditions, from tropical to temperate locations. People recognize it for its many uses and versatility. Maize is the second-most extensively grown crop globally, including different types such as field corn, sweet corn, popcorn, and baby corn. In fact, maize is very crucial because it serves as a partial source of food, animal feed, and raw materials in most industries in the world (Hartkamp et al., 2001; Amanullah Iqbal and Khalil, 2007). From its total cultivated area of 193.7 million hectares (Mha), India produces approximately 1147.7 million metric tons of agricultural produce, resulting in an average productivity of approximately 5.75 t ha−1 (FAOSTAT, 2022). Maize is becoming increasingly popular as a crop in rice-based systems in South Asia (Das et al., 2018). Maize is a crucial crop, with 61% used for feed, 17% for food, and 22% for industrial purposes. Ensuring sustainable maize farming, especially for smallholders, is vital for food and livelihood security (Herrero et al., 2014). Foley et al. (2011) and Tilman et al. (2011) reported that the estimated world population would be 9.5 billion by 2050. Shifting land and soil degradation affect the global efficiency, productivity, and profitability of farms (Montgomery, 2007; Pimentel et al., 2010). Agriculture in Bihar (an important part of the Eastern Indo-Gangetic Plains—EIGPs) uses more than 75% of the population, primarily consisting of small-scale farmers (Jat et al., 2025). Bihar is ranked low in SDG-2 (zero hunger); this shows that the state has the lowest development indices among all Indian states. Agriculture also represents a major source of agri-food insecurity among rural households, who depend on agricultural operations for their agri-food and income (Godfray et al., 2010). Sustainable farming is a combination of agriculture that is productive, profitable, efficient in its input use, and environmentally sound (Jat et al., 2011). To ensure that we derive the most from maize-based systems (Krupnik et al., 2017), there is a need to optimize their productivity and profitability with respect to resource provision (Singh et al., 2014). This is crucial for sustainable crop production worldwide (Yuan and Peng, 2017; Dar et al., 2020; Rockström et al., 2009). We also need to find ways to reduce the environmental impact, especially when it comes to water usage, so that maize-based systems can be sustainable and adaptable in the long run.
Bihar, located in the EIGPs and ranked third in maize production in India, primarily follows a rice–wheat rotation, with a rice–maize rotation in northeastern Bihar (Mandal et al., 2020). Maize is cultivated year-round in three seasons: Rabi (October–April), Kharif (July–September), and Spring (May–July). Bihar comprises approximately 0.65 Mha of maize growing areas; the annual production stands approximately at 2.22 Mt. with an average productivity of approximately 3.43 t ha−1 (Hoda et al., 2021). Maize farming in both the Kharif and Rabi seasons faces challenges such as waterlogging and declining yields, worsened by the conventional FB planting system with poor drainage and aeration. Despite India exporting 2.88 Mt. of maize in 2020–2021, historical evidence shows FB planting has reduced yields and limited sustainable maize production in Bihar.
Raised bed planting (RBP) is a sustainable and economically viable solution to mitigate these challenges. It offers multiple benefits, including effective weed management, improved input (water and nutrients) use efficiency, and reduced maize lodging and waterlogging (Sayre and Ramos, 1997; Fischer et al., 2019). In addition to that, it promotes direct seeding of cereals, thereby increasing crop productivity (Sahu et al., 2024). Studies indicated that RBP plays a role in both controlling crop growth and improving soil quality while also increasing the input use efficiency (Jat et al., 2020; Du et al., 2021a). There is always an insufficiency of data that is necessary for a proper comparison of different planting techniques from an environmental and ecological point of view, particularly in EIGPs. The results from different studies are contradictory to each other. More research is needed to highlight the advantages and disadvantages of RBP over FB methods. The purpose of this research is to improve the efficiency of maize farming systems in terms of production, profitability, and sustainability. The use of RBP techniques will support climate resilience, productivity, profitability, and resource use efficiency (WUE, NUE) compared to traditional FB methods.
We hypothesized that RBP significantly enhances climate resilience, productivity, profitability, and resource use efficiency (WUE, NUE) compared to FB methods in maize farming systems. This hypothesis led us to the following objectives: (i) to assess the impact of RBP on climate resilience in maize farming systems, (ii) to assess the productivity and profitability (economic returns) of maize farming, and (iii) to examine the impact of RBP techniques on resource use efficiency (WUE, NUE) of maize farming systems.
2 Methods
2.1 Project sites
The large comparative field demonstration (over an acre per farmer) was conducted in four successive growing rabi (winter) seasons from 2020–2021 to 2023–2024 (4 years) at demonstration sites of farmer fields in Purnea (250 77’ N, 870 47′ E), and Katihar (250 55’ N, 870 56′ E), districts of Bihar state (250 96’ N, 850 27′ E), India. These project sites (PSs) are situated within the Climate-Resilient Agriculture (CRA) program, strategically located in the EIGPs along the Ganga River region in India. A total of 10 PS /villages were selected for both Purnea and Katihar districts (five sites/villages of each) (Figure 1). A total of 60 farmers (6 for each PS) were chosen for the study.
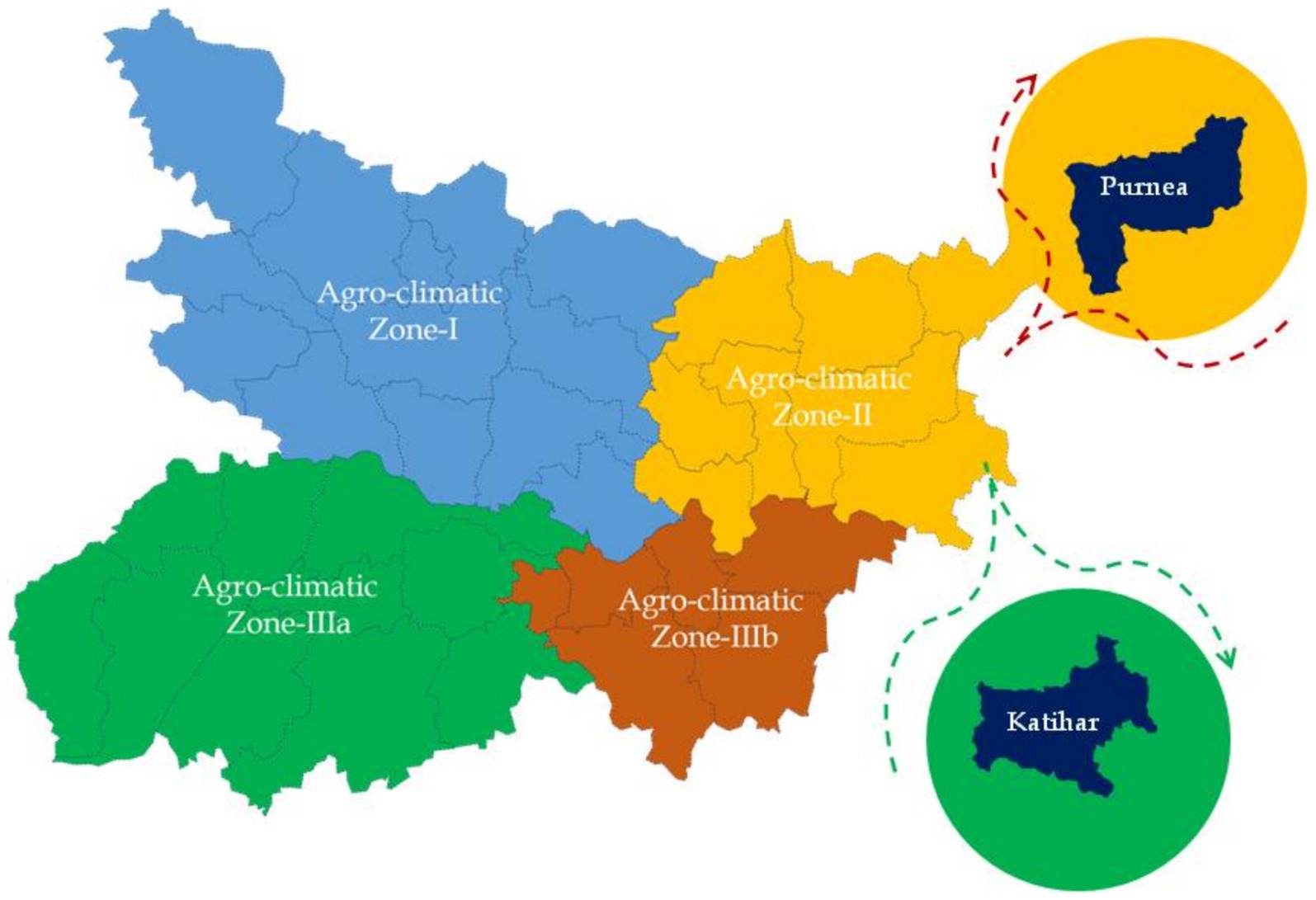
Figure 1. Pilot study area in Purnea (250 77’ N, 870 47′ E) and Katihar (250 55’ N, 870 56′ E) districts of Bihar state.
2.2 Site characteristic
The PSs comprise Dogachhi, Dholbazza, Basantpur, Kullakhas, and Kullasundar in Purnia, and Basgarha, Mahinathpur, Barakhal, Musapur, and Sisiya in Katihar (Supplementary Table S1). The project sites have a humid subtropical climate with well-defined monsoon and dry seasons. Annual rainfall during the study period (2019–2024) ranged from 1,380 to 1,510 mm in Purnia and from 1,305 to 1,450 mm in Katihar, mainly in the range of June to September. The mean annual temperature ranged between 26.2°C and 27.9°C with seasonal variations impacting crop growth phases. In comparison with historical trends (1991–2020), the study period revealed an increase in temperature (by 0.5–1.2°C) and increased variability in rainfall, suggesting potential climatic changes impacting soil moisture and crop yield (Supplementary Figure S1).
The soils in the study area were predominantly alluvial and sandy loam, with variations observed across different sites. Bulk density ranged from 1.12 to 1.19 g/cm3, field capacity from 39.89 to 45.8%, and wilting point from 9.65 to 12.05%. Soil organic carbon varied between 0.21 and 0.32%, and pH from 6.48 to 6.9. Available N, P, and K ranged from 198.65 to 225.45 kg/ha, 13.45 to 16.9 kg/ha, and 116.78 to 140.1 kg/ha, respectively, indicating variations in soil fertility and moisture retention across project sites (Supplementary Table S2).
2.3 Planting techniques
2.3.1 Raised bed planting (RBP) technology
Among the developed practices, the RBP was considered a superlative planting technique for maize farming, and seeds were sown by a raised bed planter (Figure 2).
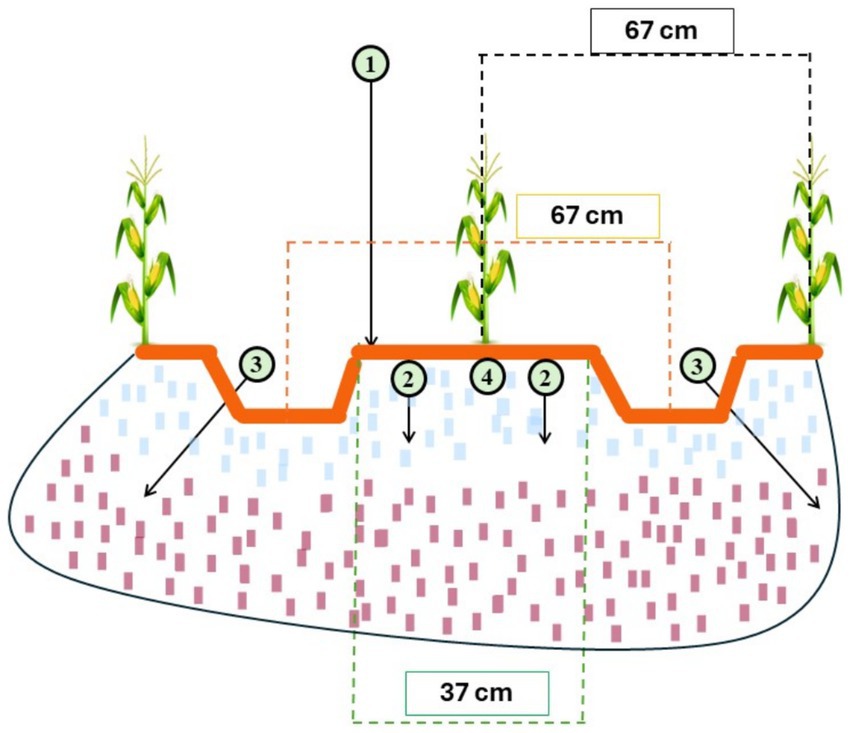
Figure 2. Cross-sectional diagram of a raised bed demonstrating enhanced water management and soil conditions: (i) increased capacity for infiltration, (ii) rapid internal drainage, (iii) surface water removal, and (iv) optimized aero-hydro thermal conditions.
2.3.2 Flatbed system
Traditional flat planting included three-pass plowing with a cultivator followed by one-pass plowing with a rotavator, and seeds were sown manually (Figures 3A–E).
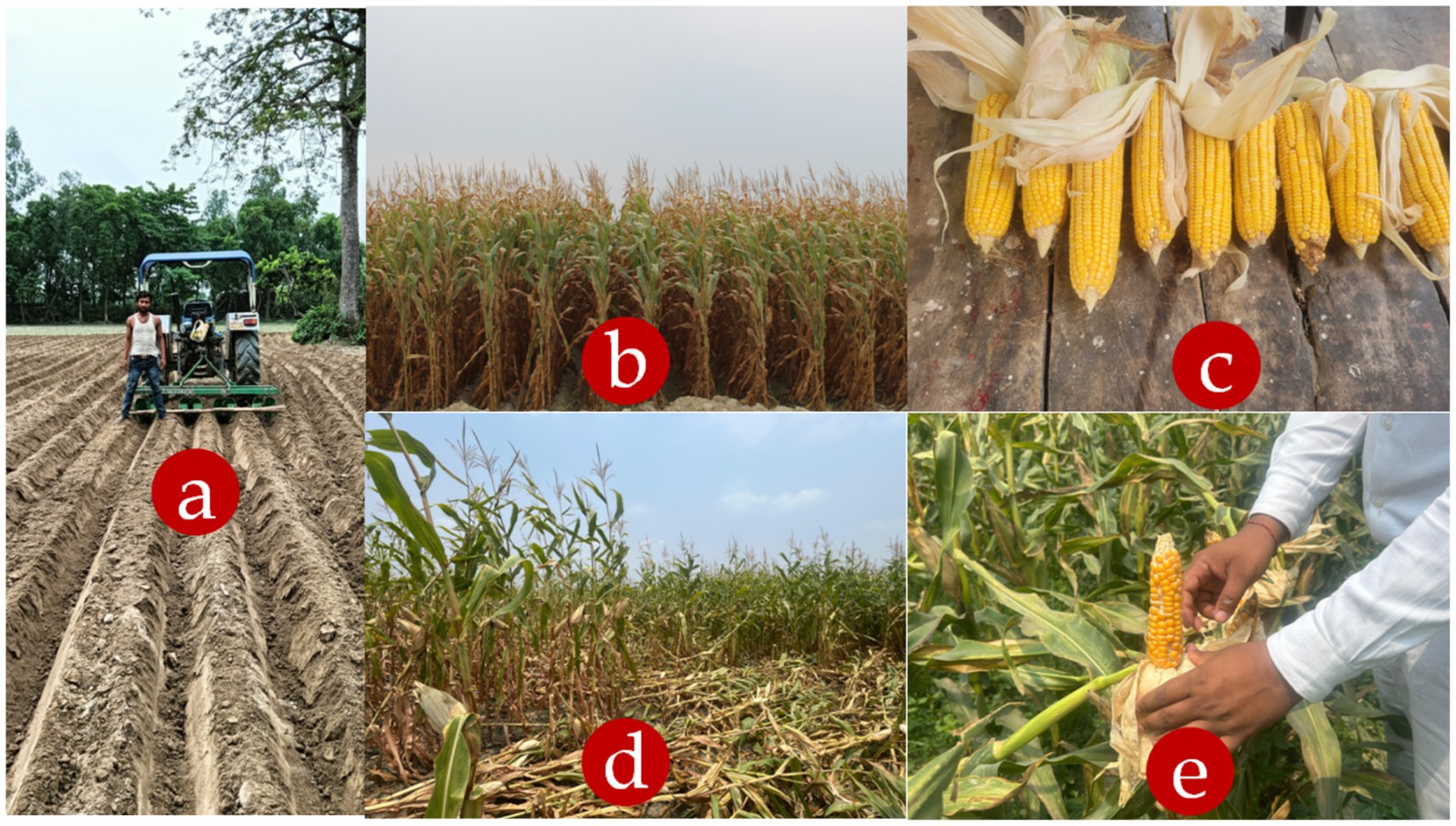
Figure 3. (a) RBP system land preparation, (b) standing crop at RBP, (c) crop size of RBP, (d) loading at FB, and (e) cob size of FB.
2.4 Crop management practices
2.4.1 Crop density
Under the RBP technique, maize (hybrid P-3355) was sown at a crop density of approximately 74,626 plants per hectare, which was accomplished with a row-to-row distance of 67 cm and an intra-row spacing of 20 cm. Conversely, for the flatbed planting (FB) system, crop density stood at approximately 88,888 plants per hectare with row spacing of 45 cm and intra-row spacing of 25 cm. This way ensures uniformity of plant density since it serves the hybrid in question, namely, P-3355 (Supplementary Table S2).
2.4.2 Nutrient management
A balanced fertilization approach was adopted to ensure optimal growth and crop development in maize farming. The basal dose of application of nutrients consisted of applying potassium (K) 75 kg/ha as muriate of potash (MOP), phosphorus (P) 125 kg/ha as diammonium phosphate (DAP), and nitrogen (N) 125 kg/ha as urea. The two split dosages were made during the growing season; the first application which constitutes 50% of the total N application was applied 25 days after planting, and the remaining 50% was applied 50 days after planting (Supplementary Table S2).
2.4.3 Pest management
After 15–20 days of sowing, a mixture of tembotrione (115 mL) + atrazine (500 g) was sprayed per acre of land to control weeds. To control pests such as stem borer (fall armyworm), emamectin benzoate 100 g/ acre was sprayed (Supplementary Table S3).
2.4.4 Water management
Irrigation—The irrigation system used in this study was a surface furrow irrigation system, which maximizes water distribution and meets the crop’s water requirement under field conditions. The furrows, as shown in Figure 2, were 37 cm wide, 15 cm deep, and 67 cm apart, aiming at effective delivery of water with less surface runoff. Water was applied using manually opened inlets installed at the upper end of every furrow to deliver controlled flow and prevent percolation losses. The irrigation schedule was established according to soil moisture depletion, measured at 15-cm depth intervals utilizing gravimetric soil sampling, and initiated as soon as the soil moisture had reached 50% depletion from available water holding capacity (WHC) to sufficiently hydrate crops without over-irrigating.
2.5 Data handling and analysis
Statistical tests were conducted on the raised bed planting (RBP) and flatbed (FB) systems in terms of differences between different parameters using analysis of variance (ANOVA) and Duncan’s multiple range test (DMRT). Parameters were productivity, profitability, water use efficiency (WUE), water productivity (WP), and nutrient use efficiency (NUE). Evapotranspiration (ET) was calculated by the pan evaporation method, in which water evaporation was quantified from a standard Class A pan under open-field conditions. The observed pan evaporation (Epan) values were corrected by a pan coefficient (Kp) of 0.7 to 0.85, considering local climatic factors such as wind speed, relative humidity, and solar radiation. To estimate crop evapotranspiration (ETc), Kc values specific to maize were used for the corrected ET0 (reference evapotranspiration). Rather than taking a constant Kc = 0.85, we took stage-specific Kc values according to FAO-56 guidelines for maize (Allen et al., 1998 and Doorenbos and Pruitt, 1977):
Kc_initial = 0.35 (Emergence up to the early vegetative stage).
Kc_development = 0.75 (Vegetative to flowering stage).
Kc_mid-season = 1.15 (Flowering up to the grain-filling stage).
Kc_late-season = 0.60 (Maturity and drying stage).
The formula for water use efficiency (Equations 1–4) was used from Howell (2001), total water productivity from Zwart and Bastiaanssen (2004), and nutrient use efficiency from Fageria et al. (2010) and Dobermann (2007).
3 Results
3.1 Impact on productivity
Data showed that productivity varied considerably between the raised bed planting (RBP) and flatbed (FB) systems, with RBP showing higher productivity across all 10 project sites (PSs) (Figure 4). The RBP system had a range of productivity from 9.28 to 10.53 t ha−1. The highest productivity was observed at PS-3 with 10.53 ± 0.49 t ha−1, whereas the lowest was recorded at PS-5 with 9.28 ± 0.80 t ha−1, thus showing significant variation. These differences show the consistent benefit of the RBP system over FB in terms of higher productivity at different locations.
The productivity under the flatbed (FB) system varied considerably across the 10 project sites (PSs), ranging from 5.70 to 8.29 t ha−1 (Figure 4). The highest productivity was recorded at PS-1 (8.29 ± 0.31 t ha−1), while the lowest was recorded at PS-9 (5.70 ± 0.42 t ha−1). In-between value situations existed for most others as well, with some considerable performances at PS-2 (8.12 ± 0.24 t ha−1) and PS-3 (7.87 ± 0.72 t ha−1). The RBP system experienced productivity advantages of 4.84 to 17.82% compared to the FB system, with the former being observed at PS-1, PS-3, and PS-8.
3.2 Impact on profitability
The average profitability across project sites under the RBP system varied from $1943 to $1998 ha−1, with significant differences. The highest profitability was noted at PS-10 ($1998.83 ± 48 ha−1), whereas the lowest was reported at PS-7 ($1943.25 ± 54.94 ha−1). Other notable performances were reported at PS-8 ($1976.50 ± 40.66 ha−1) and PS-5 ($1975.83 ± 44.79 ha−1), indicating consistent economic advantages of the RBP system. On the other hand, the FB system had a profitability range of $1627–1857 ha−1 across the project sites (Figure 5). The highest profitability was recorded at PS-10 ($1857.25 ± 60.84 ha−1), representing a 14.11% increase compared to other sites, while PS-5 recorded the lowest value at $1627.50 ± 27.87 ha−1. Intermediate profit gains were recorded at PS-3 (+12.20%, $1826.00 ± 83.46 ha−1) and PS-6 (+8.04%, $1758.50 ± 95.56 ha−1). These results highlight the significant economic advantages of using the RBP system over the FB system. The results showed that the RBP systems exhibited higher profitability compared to the FB system across all PS. Profitability increased in the RBP system from 4.64 to 20.43%, with significant improvements noted in PS-1, PS-3, and PS-8 (Figure 5).
3.3 Impact on water use efficiency
Data revealed that the WUE ranged widely among the PSs and planting methods RBP and FB (Figure 6). WUE on the RBP system varied from 2.72% (PS-4) to 30.52% (PS-9). The highest (+30.52%, 4.79 ± 0.64 kg ha−1 mm−1) WUE was reported at PS-9, followed by PS-3 (+23.71%, 4.54 ± 0.32 kg ha−1 mm−1), PS-2 (+22.89%, 4.51 ± 0.13 kg ha−1 mm−1), PS-1 (+15.26%, 4.23 ± 0.48 kg ha−1 mm−1), PS-8 (+13.89%, 4.18 ± 0.19 kg ha−1 mm−1), PS-5 (+11.99%, 4.11 ± 0.53 kg ha−1 mm−1), PS-6 (+7.91%, 3.96 ± 0.59 kg ha−1 mm−1), PS-7 (+5.72%, 3.88 ± 0.21 kg ha−1 mm−1), and PS-10 (+2.72%, 3.77 ± 0.89 kg ha−1 mm−1), and the lowest (3.67 ± 0.42 kg ha−1 mm−1) was reported at PS-4. Meanwhile, in the case of the FB system, it ranges from 15.24 to 49.39% among the different PSs. The highest (+49.39%, 2.45 ± 0.10 kg ha−1 mm−1) WUE was observed at PS-1 (Figure 6), followed by PS-2 (+43.29%, 2.35 ± 0.18 kg ha−1 mm−1), PS-7 (+29.88%, 2.13 ± 0.30 kg ha−1 mm−1), PS-6 (+25.61%, 2.06 ± 0.32 kg ha−1 mm−1), PS-3 (+25.61%, 2.06 ± 0.25 kg ha−1 mm−1), PS-8 (+24.39%, 2.04 ± 0.11 kg ha−1 mm−1), PS-5 (+21.95%, 2.00 ± 0.36 kg ha−1 mm−1), PS-10 (+20.12%, 1.97 ± 0.07 kg ha−1 mm−1), and PS-4 (+15.24%, 1.89 ± 0.18 kg ha−1 mm−1), and the lowest was 1.64 ± 0.18 kg ha−1 mm−1 at PS-9. This trend continued for successive years, and in all PSs and years, the RBP system exhibited better WUE.
3.4 Impact on water productivity
Water productivity (WP) varied significantly between the project sites (PSs) and planting techniques (Table 1). Under the RBP system, WP ranged from 3.88 to 4.58 kg ha−1 mm−1, with the highest value observed at PS-9 (+17.85%, 4.58 ± 0.94 kg ha−1 mm−1). This was closely followed by PS-3 (+16.84%, 4.54 ± 0.27 kg ha−1 mm−1) and PS-2 (+15.63%, 4.50 ± 0.23 kg ha−1 mm−1). Conversely, the lowest WP under RBP was reported at PS-5 (3.88 ± 0.64 kg ha−1 mm−1). These findings indicated substantial gains in water productivity with RBP, particularly at high-performing sites such as PS-9 and PS-3.
For the FB system, WP exhibited a broad range of variability, spanning from 1.57 to 2.41 kg ha−1 mm−1 across the sites (Table 1). PS-1 recorded the maximum WP (+53.50%, 2.41 ± 0.12 kg ha−1 mm−1), followed by PS-2 (+39.49%, 2.19 ± 0.10 kg ha−1 mm−1) and PS-7 (+33.76%, 2.09 ± 0.18 kg ha−1 mm−1). The lowest WP in the FB system was observed at PS-9 (1.57 ± 0.24 kg ha−1 mm−1), highlighting the significant advantage of RBP over FB in optimizing water productivity, especially at low-performing sites.
3.5 Impact on nitrogen use efficiency
Nitrogen use efficiency (NUE) varied significantly across the project sites (PSs) and planting techniques (Figure 7). The NUE under the RBP system ranged from 68.45 to 75.83 kg grain kg−1 N. The highest NUE was recorded at PS-9 (+10.74%, 75.83 ± 8.59 kg grain kg−1 N), followed by PS-3 (+8.94%, 74.63 ± 5.36 kg grain kg−1 N) and PS-1 (+7.38%, 73.46 ± 6.12 kg grain kg−1 N). In contrast, the lowest NUE was observed at PS-4 (68.45 ± 5.74 kg grain kg−1 N). These results highlight the efficiency of the RBP system, especially at the better-performing sites such as PS-9 and PS-3. For the FB system, NUE values varied between 42.89 and 60.69 kg grain kg−1 N across the project sites (Figure 7). The highest NUE was documented at PS-1 + 41.48%, with 60.69 ± 1.81 kg grain kg−1 N, followed by PS-2 + 36.72%, with 58.62 ± 1.94 kg grain kg−1 N, and PS-3 + 30.47%, with 55.97 ± 2.13 kg grain kg−1 N, respectively. At the bottom end, the minimum NUE was documented at PS-9 with 42.89 ± 4.63 kg grain kg−1 N; this marks a significant variance in nutritional use efficiency among RBP and FB systems. The consistently higher NUE under RBP demonstrates its effectiveness in optimizing nitrogen utilization.
3.6 Impact on phosphorous use efficiency
Phosphorus use efficiency (PUE) varied significantly across project sites (PSs) and planting techniques, with the RBP system showing higher values overall (Figure 8). Under the RBP system, PUE ranged from 164.79 to 188.66 kg grain kg−1 P. The highest efficiency was observed at PS-2 (+14.48%, 188.66 ± 13.43 kg grain kg−1 P), closely followed by PS-9 (+14.43%, 188.60 ± 14.12 kg grain kg−1 P) and PS-10 (+11.90%, 184.44 ± 15.22 kg grain kg−1 P). On the lower end, PS-6 recorded the least PUE (164.79 ± 9.91 kg grain kg−1 P), indicating variability across sites. These results highlight the consistent advantage of RBP, particularly at high-performing locations such as PS-2 and PS-9.
In contrast, the FB system exhibited lower PUE values, ranging from 103.69 to 148.89 kg grain kg−1 P across the sites (Figure 8). PS-1 achieved the highest PUE (+43.63%, 148.89 ± 11.75 kg grain kg−1 P), followed by PS-3 (+38.80%, 143.89 ± 5.89 kg grain kg−1 P) and PS-2 (+37.79%, 142.86 ± 6.88 kg grain kg−1 P). The lowest efficiency was recorded at PS-9 (103.69 ± 3.55 kg grain kg−1 P), demonstrating a significant disparity between the best- and worst-performing sites. Overall, the RBP system outperformed FB, reinforcing its effectiveness in optimizing phosphorus utilization across diverse conditions.
3.7 Impact on potassium use efficiency
Potassium use efficiency (KUE) exhibited significant variability across project sites (PSs) and planting techniques (Figure 9). In the RBP system, KUE varied from 210.56 to 240.99 kg grain kg−1 K. The highest value was recorded at PS-9 with +14.46% (240.99 ± 13.81 kg grain kg−1 K), followed closely by PS-2 with +12.58% (237.06 ± 12.50 kg grain kg−1 K) and PS-10 with +11.93% (235.68 ± 15.09 kg grain kg−1 K). At the other end, PS-6 produced the lowest KUE values (210.56 ± 15.01 kg grain kg−1 K), suggesting site-specific variability in potassium utilization efficiency. These results indicated that RBP was better at harnessing maximum KUE, especially at high-performing sites such as PS-9 and PS-2.
The FB system produced comparatively lower KUE values in the range of 109.03 to 181.81 kg grain kg−1 K for different sites (Figure 9). The highest value was found at PS-3 (+66.78%, 181.81 ± 7.96 kg grain kg−1 K), followed by PS-1 (+61.97%, 176.59 ± 6.24 kg grain kg−1 K) and PS-5 (+61.25%, 175.82 ± 7.85 kg grain kg−1 K). The lowest value was reported at PS-9 (109.03 ± 3.62 kg grain kg−1 K), which reflects a drastic difference in the performance of KUE among the sites. Overall, the RBP system outperformed FB at all locations and demonstrated its effectiveness in improving potassium utilization.
4 Discussion
4.1 Productivity
The RBP system demonstrated higher productivity than the FB system by enhancing nutrient use efficiency and supporting optimal plant density, leading to improved crop growth and yield potential. RBP demonstrated superior yield performance across all production systems, with the highest and most stable productivity observed at certain sites. In contrast, the FB system showed lower and more variable yields. These findings highlight the effectiveness of RBP in enhancing maize productivity and emphasize the influence of site-specific factors on optimizing yield outcomes. The increase in production can be attributed to various elements that are inherent (+ aeration, + drainage ~ temperature) in the RBP system. The RBP system is particularly beneficial in high and intensive rainfall areas (Ahmad et al., 2020; Jaseem et al., 2024). This system also improves (10–35%) productivity and soil sustainability (Chauhdary et al., 2019). In addition, the RBP system improves germination, plant growth, and development (Singh et al., 2023). RBP system improves the loading resistance and optimizes the yield (Pooniya and Sharma, 2023; Jat et al., 2020; Du et al., 2021b). The RBP system showed its superiority to the FB system.
4.2 Profitability
The results showed that the RBP system significantly improved both productivity and resource use efficiency compared to the FB system. The profitability of RBP was much higher than the FB, with a spread of improvements from 15 to 42% across various project sites. PS-5 exhibited the highest profitability under RBP, and PS-10 had the highest total profitability. Instead, the FB system was characterized by a relatively lower profit margin with a narrower scope of increases at all sites. These results indicate that in addition to increasing crop yields, the RBP system provides even better economic benefits (Bakhsh et al., 2018), especially on selected sites, and therefore deserves more serious consideration for application in sustainable farming (Yigezu et al., 2021). Ahmad et al. (2020) reported that the RBP system has a favorable condition for root growth and development. RBP system also inputs use efficiency (water 1,244% and nutrients 18–34%) at different soil and climatic conditions. Pooniya and Sharma (2023) and Chauhdary et al. (2019) reported that it improved soil sustainability and crop productivity. It also improves the soil biological health of different cropping systems (Sinha and Kumar, 2023).
4.3 Water use efficiency and water productivity
WUE and WP vary across planting techniques and production systems. The RBP method improved WUE, highlighting its role in water conservation (Supplementary Figure S2). While some systems benefited more, others showed lower efficiency, reflecting management influences (Chauhdary et al., 2019). By means of improved infiltration and lower evaporation, the RBP approach guarantees more efficient water use and less waterlogging (Jaseem et al., 2024).
The RBP system improves water use efficiency, is more effective with limited inputs, and supports soil sustainability in high moisture conditions (Singh et al., 2023). Chauhdary et al. (2019) and Connor et al. (2023) reported that this system saved more water (40–50%) than the FB system.
4.4 Nutrient use efficiency
NUE, PUE, and KUE varied among the tested planting systems (i.e., RBP system and FB system). The RBP system showed significantly higher efficiency compared to the FB system. NUE demonstrated notable differences, with the RBP system achieving higher efficiency. Similarly, PUE and KUE were also higher in the RBP system, indicating improved phosphorus and potassium utilization. These findings emphasize the role of RBP in enhancing NUE, contributing to improved resource utilization and sustainable crop production. The adoption of the RBP system improves NUE (25–30%) than the FB system (Ahmad et al., 2020). Adoption of the RBP system improves NUE and reduces soil loss (Pooniya and Sharma, 2023; Chauhdary et al., 2019). The RBP system also helps to improve biological health, contributes to the nutrient mineralization process, and improves productivity and profitability (Sinha and Kumar, 2023). Climate conditions during the experiment also affected WUE and NUE (Supplementary Figure S3). Greater temperatures in 2023 and 2024 would probably have raised evapotranspiration levels (Lahlali et al., 2024), impacting soil moisture balance and nutrient mobility. Similarly, changes in rainfall patterns impact irrigation requirements. However, surplus rainfall in 2021 would have resulted in nutrient leaching losses, while deficit rainfall in 2022 and 2024 necessitated greater dependence on supplemental irrigation to sustain optimal plant growth. Soil texture contributed significantly to the efficiency of water retention at the study sites (Bhushan et al., 2007). Sandy loams in some of the sites showed quicker drainage and needed more regular irrigation adjustments, whereas loamy alluvial stored water longer and enhanced the water productivity ratio. These emphasize the need for climate-responsive management of nutrients and irrigation to achieve maximum resource use efficiency (Katerji and Mastrorilli, 2009) in dynamic agro-ecological settings.
5 Policy recommendations for RBP systems
The current study emphasizes the practical relevance of adopting the raised bed planting (RBP) system for improving maize production in resource-scarce regions (Figure 10). The results illustrate that RBP considerably boosts productivity, profitability, and use efficiency of resources (WUE, WP, and NUE), thereby giving it much more importance for confronting the issues of water shortage, NUE, and soil health in maize cropping. Given the unique benefits of RBP, especially regarding sustainability and economic viability, policy recommendations are important for facilitating its widespread adoption. For this purpose, the government needs to introduce financial incentives in terms of subsidies, low-interest loans, and insurance schemes for the easy adoption of RBP. Extension services and training of farmers are also very important as they need to be informed and educated about the benefits of RBP and supported by demonstration plots and information campaigns. Infrastructure development, especially irrigation, and targeted research funding are very important to optimize RBP techniques and ensure sustainable farming practices. These measures would not only enhance productivity and profitability but also improve resilience and food security in smallholder maize farming systems, thereby laying the foundation for long-term agri-food security and sustainability. In addition, the shift to RBP involves specialized machinery, including bed formers and furrowers, which may not be accessible to smallholder farmers. In response to these issues, policies need to target long-term trials, site-specific nutrient management practices, and enhancing access to affordable mechanization to promote wider adoption and sustainability of the RBP system.
6 Conclusion
This 4-year on-farm comparative trial underlines the significant advantages that accrue from adopting the raised bed planting (RBP) system in maize farming (Figure 11). The productivity, profitability, and resource-use efficiency (WUE and NUE) gains of RBP over the flatbed system were consistently evident. Specifically, RBP showed significant increases in yield (9.28–10.53 t ha−1) and profitability ($1943–1998 ha−1) compared to FB (5.70–8.29 t ha−1 and $1627–1857 ha−1, respectively). In addition, RBP showed enhanced WUE (3.67–4.79 kg ha−1 mm−1) and WP (3.88–4.58 kg ha−1 mm−1), in addition to higher NUE, PUE, and KUE values, which indicated better nutrient management.
RBP is a valuable strategy for enhancing maize productivity and long-term sustainability, particularly in waterlogged and resource-limited regions. By optimizing water use and improving nutrient efficiency, RBP supports productivity and profitability across diverse agroecological zones. However, its effectiveness during prolonged dry periods requires further research. Implementing RBP in suitable environments can strengthen agri-food security, economic viability, and sustainable farming practices.
Data availability statement
The original contributions presented in the study are included in the article/Supplementary material, further inquiries can be directed to the corresponding authors.
Author contributions
RajJ: Conceptualization, Funding acquisition, Methodology, Project administration, Resources, Supervision, Validation, Writing – review & editing. VM: Conceptualization, Project administration, Visualization, Writing – original draft, Writing – review & editing. IR: Project administration, Writing – review & editing. RKS: Funding acquisition, Project administration, Writing – review & editing. RNS: Funding acquisition, Methodology, Project administration, Writing – review & editing. SD: Investigation, Writing – original draft, Writing – review & editing. SuK: Methodology, Writing – review & editing. SP: Data curation, Writing – review & editing. SM: Data curation, Formal analysis, Methodology, Resources, Writing – review & editing. KuS: Investigation, Writing – review & editing. SuS: Investigation, Writing – review & editing. RamS: Investigation, Writing – review & editing. SeK: Investigation, Writing – review & editing. KMS: Investigation, Writing – review & editing. GK: Investigation, Writing – review & editing. AmK: Writing – review & editing. AC: Data curation, Methodology, Writing – review & editing. RaS: Data curation, Investigation, Methodology, Writing – review & editing. GC: Investigation, Writing – review & editing. PN: Investigation, Supervision, Writing – review & editing. PK: Investigation, Methodology, Writing – review & editing. AbK: Funding acquisition, Investigation, Methodology, Writing – review & editing. RatJ: Funding acquisition, Investigation, Project administration, Supervision, Writing – review & editing. UK: Funding acquisition, Methodology, Project administration, Supervision, Writing – review & editing. AD: Funding acquisition, Resources, Supervision, Validation, Writing – review & editing. AJ: Funding acquisition, Resources, Supervision, Validation, Writing – review & editing. DT: Project administration, Supervision, Writing – review & editing. SwS: Investigation, Supervision, Methodology, Writing – review & editing.
Funding
The author(s) declare that financial support was received for the research and/or publication of this article. We acknowledge the financial and technical support from Government of Bihar for initiating (CRA Program) for funds and technical support. Indian Council of Agricultural Research (ICAR), CGIAR and all donors who supported this research through their contributions are highly acknowledged.
Acknowledgments
The authors sincerely acknowledge the financial and technical support provided by the Government of Bihar through the Climate-Resilient Agriculture (CRA) Program. We also extend our gratitude to the Indian Council of Agricultural Research (ICAR), CGIAR, and all donors whose contributions made this research possible. We deeply appreciate the farmers for their time, resources, and invaluable insights, as well as the field technicians for their dedicated efforts in facilitating this study.
Conflict of interest
The authors declare that the research was conducted in the absence of any commercial or financial relationships that could be construed as a potential conflict of interest.
The author(s) declared that they were an editorial board member of Frontiers, at the time of submission. This had no impact on the peer review process and the final decision.
Publisher’s note
All claims expressed in this article are solely those of the authors and do not necessarily represent those of their affiliated organizations, or those of the publisher, the editors and the reviewers. Any product that may be evaluated in this article, or claim that may be made by its manufacturer, is not guaranteed or endorsed by the publisher.
Supplementary material
The Supplementary material for this article can be found online at: https://www.frontiersin.org/articles/10.3389/fsufs.2025.1484079/full#supplementary-material
References
Ahmad, A., Shahid, M., and Khan, A. (2020). Raised bed planting improves soil properties and productivity in maize-wheat cropping system. Soil Tillage Res. 197:104519. doi: 10.1016/j.still.2019.104519
Allen, R. G., Pereira, L. S., Raes, D., and Smith, M. (1998). Crop evapotranspiration: guidelines for computing crop water requirements. FAO irrigation and drainage paper no. 56. Rome: Food and Agriculture Organization of the United Nations.
Amanullah Iqbal, M., and Khalil, S. K. (2007). Maize phenology and growth response to seed rates and nitrogen levels. Agron. J. 99, 645–653. doi: 10.2134/agronj2006.0077
Bakhsh, A., Abid, M., Shahid, M., and Ahmad, M. (2018). Economic benefits of raised bed planting in maize cultivation. Agric. Econ. 48, 437–448. doi: 10.1111/agec.12345
Bhushan, L., Ladha, J. K., Gupta, R. K., Singh, S., Tirol-Padre, A., Saharawat, Y. S., et al. (2007). Saving of water and labor in a rice–wheat system with no-tillage and direct seeding technologies. Agron. J. 99, 1288–1296. doi: 10.2134/agronj2006.0227
Chauhdary, J. N., Rashid, M., and Khan, A. A. (2019). Raised bed planting in cereal cropping systems: a review. J. Agric. Sci. 64, 155–170. doi: 10.2298/JAS1902155C
Connor, D. J., Loomis, R. S., and Cassman, K. G. (2023). Crop ecology: Productivity and Management in Agricultural Systems. 2nd Edn. Cambridge: Cambridge University Press.
Dar, M. A., Singh, S., and Kumar, A. (2020). Sustainable agriculture: an overview. Sustain. Agric. Rev. 39, 1–22. doi: 10.1007/978-3-030-42587-6_1
Das, P. K., Sharma, R. C., and Singh, R. (2018). Maize as a component crop in rice-based systems in South Asia. Field Crop Res. 217, 113–124. doi: 10.1016/j.fcr.2017.12.012
Dobermann, A. (2007). “Nutrient use efficiency-measurement and management” in Fertilizer best management practices: General principles, strategy for their adoption and voluntary initiatives versus regulations. eds. A. Krauss, K. Isherwood, and P. Heffer (Paris: International Fertilizer Industry Association), 1–28.
Doorenbos, J., and Pruitt, W.O. (1977). Guidelines for predicting crop water requirements. FAO irrigation and drainage paper no. 24. Rome: Food and Agriculture Organization of the United Nations.
Du, J., Li, W., and Wang, J. (2021a). Benefits of raised bed planting on soil chemical and biological properties. Soil Sci. Soc. Am. J. 85, 691–702. doi: 10.1002/saj2.20228
Du, J., Li, W., and Wang, J. (2021b). The impact of raised bed planting on soil salinity and crop yield. Soil Water Manag. J. 7, 1–12. doi: 10.1016/j.sawma.2021.02.001
Fageria, N. K., Baligar, V. C., and Jones, C. A. (2010). Growth and mineral nutrition of field crops. 3rd Edn. Boca Raton, FL: CRC Press.
FAOSTAT (2022). Global maize production statistics. Rome: Food and Agriculture Organization of the United Nations.
Fischer, R. A., Byerlee, D., and Edmeades, G. O. (2019). Can technology deliver on the yield challenge to 2050? Philos. Trans. Royal Soc. B 369:20120261. doi: 10.1098/rstb.2012.0261
Foley, J. A., Ramankutty, N., Brauman, K. A., Cassidy, E. S., Gerber, J. S., Johnston, M., et al. (2011). Solutions for a cultivated planet. Nature 478, 337–342. doi: 10.1038/nature10452
Godfray, H. C. J., Beddington, J. R., Crute, I. R., Haddad, L., Lawrence, D., Muir, J. F., et al. (2010). Food security: the challenge of feeding 9 billion people. Science 327, 812–818. doi: 10.1126/science.1185383
Hartkamp, A. D., White, J. W., and Hoogenboom, G. (2001). Maize production in a changing climate: impacts, adaptation, and vulnerability. Agric. Syst. 67, 33–45. doi: 10.1016/S0308-521X(00)00090-5
Herrero, M., Thornton, P. K., Bernués, A., Baltenweck, I., Vervoort, J., van de Steeg, J., et al. (2014). Exploring future changes in smallholder farming systems by linking socio-economic scenarios with regional and household models. Glob. Environ. Chang. 24, 165–182. doi: 10.1016/j.gloenvcha.2013.12.008
Hoda, S., Kumar, M., and Sharma, V. K. (2021). Maize production challenges and solutions in Bihar. Agric. Res. 10, 245–257. doi: 10.1007/s40003-021-00516-4
Howell, T. A. (2001). Enhancing water use efficiency in irrigated agriculture. Agron. J. 93, 281–289. doi: 10.2134/agronj2001.932281x
Jaseem, K. P., Meena, V. S., Hasanain, M., Meena, S. K., and Jat, R. K. (2024). Raised bed planting for boosting soybean yields and profits. Indian Farming, 74, 27–29.
Jat, M. L., Kumar, V., and Saharawat, Y. S. (2011). Conservation agriculture in cereal systems of South Asia: a review. Agric. Res. 2, 193–207. doi: 10.1007/s40003-011-0024-8
Jat, M. L., Kumar, V., and Saharawat, Y. S. (2020). Precision nutrient management in maize production: advances and opportunities. Agric. Res. 9, 137–147. doi: 10.1007/s40003-020-00437-7
Jat, R. K., Meena, V. S., Durgude, S., Sohane, R. K., Jha, R. K., Kumar, A., et al. (2025). Bridging the gap: challenges and adoption of climate-resilient agriculture technologies in agricultural landscapes across agro-climatic zones of Bihar, India. Front. Sustain. Food Syst. 8:1504388. doi: 10.3389/fsufs.2024.1504388
Katerji, N., and Mastrorilli, M. (2009). The effect of soil texture on the water use efficiency of irrigated crops: results of a multi-year experiment carried out in the Mediterranean region. Eur. J. Agron. 30, 95–100. doi: 10.1016/j.eja.2008.07.009
Krupnik, T. J., Schulthess, U., Ahmed, Z. U., and McDonald, A. J. (2017). Farmer-led approaches to developing improved crop management practices in maize-rice cropping systems in Bangladesh. Field Crop Res. 203, 94–105. doi: 10.1016/j.fcr.2016.12.013
Lahlali, R., Taoussi, M., Laasli, S.-E., Gachara, G., Ezzouggari, R., Belabess, Z., et al. (2024). Effects of climate change on plant pathogens and host-pathogen interactions. Crop Environ. 3, 159–170. doi: 10.1016/j.crope.2024.05.003
Mandal, B., Thakur, A. K., and Ghosh, A. (2020). Impact of climate change on maize production in Bihar. Indian J. Agric. Sci. 90, 421–428. doi: 10.56093/ijas.v90i3.99999
Montgomery, D. R. (2007). Soil erosion and agricultural sustainability. Proc. Natl. Acad. Sci. 104, 13268–13272. doi: 10.1073/pnas.0611508104
Pimentel, D., Burgess, M., and Pimentel, M. (2010). Soil erosion: a food and environmental threat. Environ. Dev. Sustain. 12, 449–463. doi: 10.1007/s10668-009-9201-7
Pooniya, V., and Sharma, V. (2023). Mitigating stem borer and root rot in maize using raised bed planting. Crop Prot. 152:105869. doi: 10.1016/j.cropro.2021.105869
Rockström, J., Steffen, W., Noone, K., Persson, Å., Chapin, F. S., Lambin, E. F., et al. (2009). A safe operating space for humanity. Nature 461, 472–475. doi: 10.1038/461472a
Sahu, A., Gupta, R. K., and Singh, S. P. (2024). Mechanized raised bed planting: a sustainable practice for the future. J. Agric. Eng. 61, 13–24. doi: 10.1007/s10099-023-00598-3
Sayre, K. D., and Ramos, O. (1997). Applications of raised bed planting systems to wheat. Mexico: CIMMYT.
Singh, V. K., Kumar, A., and Sharma, V. K. (2023). Water use efficiency in maize-based cropping systems: a comprehensive review. J. Water Manage. 10, 14–28. doi: 10.1016/j.jwm.2023.01.002
Singh, R. K., Singh, V. K., and Chaturvedi, V. K. (2014). Agricultural productivity and food security in Bihar. Indian J. Agric. Econ. 69, 210–221. doi: 10.22004/ag.econ.206244
Singh, A. K., Singh, S., and Kumar, R. (2023). Recent advances in raised bed planting systems: a review. Agric. Rev. 44, 21–34. doi: 10.18805/ag.R-2356
Sinha, P., and Kumar, R. (2023). Precision farming and profitability in maize cultivation. J. Precision Agric. 34, 89–103. doi: 10.1007/s11119-022-09872-0
Tilman, D., Balzer, C., Hill, J., and Befort, B. L. (2011). Global food demand and the sustainable intensification of agriculture. Proc. Natl. Acad. Sci. 108, 20260–20264. doi: 10.1073/pnas.1116437108
Yigezu, Y. A., Aw-Hassan, A., and Shiferaw, B. (2021). Maize productivity, profitability, and adoption of sustainable farming practices: a review. Agric. Syst. 187:103025. doi: 10.1016/j.agsy.2020.103025
Yuan, S., and Peng, S. (2017). Improving crop productivity and resource use efficiency to ensure food security and environmental quality in China. J. Exp. Bot. 68, 4219–4231. doi: 10.1093/jxb/erx233
Keywords: maize, raised bed planting, productivity, net returns, water and nutrient use efficiency
Citation: Jat RK, Meena VS, Reddy IR, Sohane RK, Singh RN, Durgude S, Kumar S, Pazhanismy S, Meena SK, Sharda K, Singh S, Singh RK, Kumari S, Singh KM, Kumar G, Kumar Lenka A, Chaurasiya A, Sahu R, Choudhary GL, Nath P, Kumar Yadav P, Kumar A, Jha RK, Kumar U, Das A, Jha AK, Tripathi DP and Sagar S (2025) Optimizing maize systems with raised beds: boosting productivity, profitability, and sustainability. Front. Sustain. Food Syst. 9:1484079. doi: 10.3389/fsufs.2025.1484079
Edited by:
Srdjan Šeremešić, University of Novi Sad, SerbiaReviewed by:
Vesna Dragicevic, Maize research Institute Zemun Polje, SerbiaBorivoj Pejic, University of Novi Sad, Serbia
Copyright © 2025 Jat, Meena, Reddy, Sohane, Singh, Durgude, Kumar, Pazhanismy, Meena, Sharda, Singh, Singh, Kumari, Singh, Kumar, Kumar Lenka, Chaurasiya, Sahu, Choudhary, Nath, Kumar Yadav, Kumar, Jha, Kumar, Das, Jha, Tripathi and Sagar. This is an open-access article distributed under the terms of the Creative Commons Attribution License (CC BY). The use, distribution or reproduction in other forums is permitted, provided the original author(s) and the copyright owner(s) are credited and that the original publication in this journal is cited, in accordance with accepted academic practice. No use, distribution or reproduction is permitted which does not comply with these terms.
*Correspondence: Raj Kumar Jat, ci5qYXRAY2dpYXIub3Jn; Vijay Singh Meena, dmlqYXlzc2FjLmJodUBnbWFpbC5jb20=; Sunita Kumari Meena, c3VuaXRhLm1lZW5hQHJwY2F1LmFjLmlu; Shubham Durgude, cy5kdXJndWRlQGNnaWFyLm9yZw==