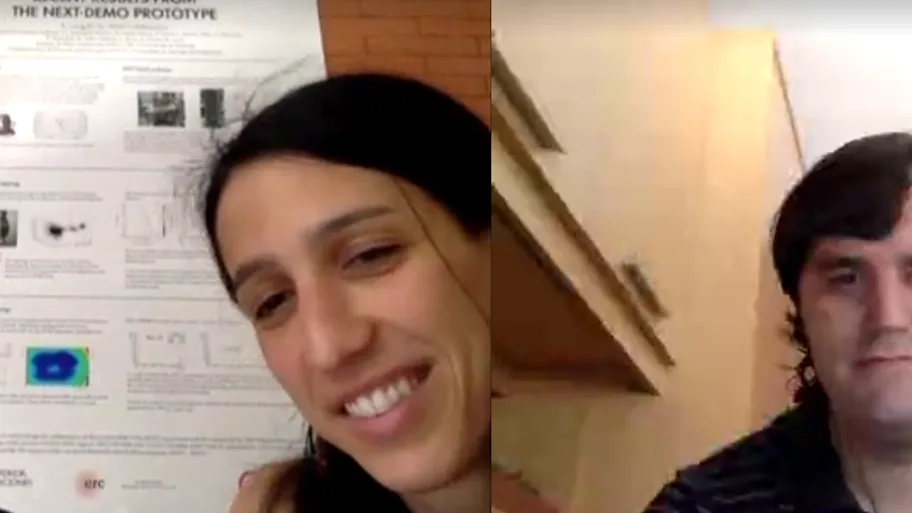
- Science News
- Life sciences
- A brief history of neutrinos: from past problems to future challenges
A brief history of neutrinos: from past problems to future challenges
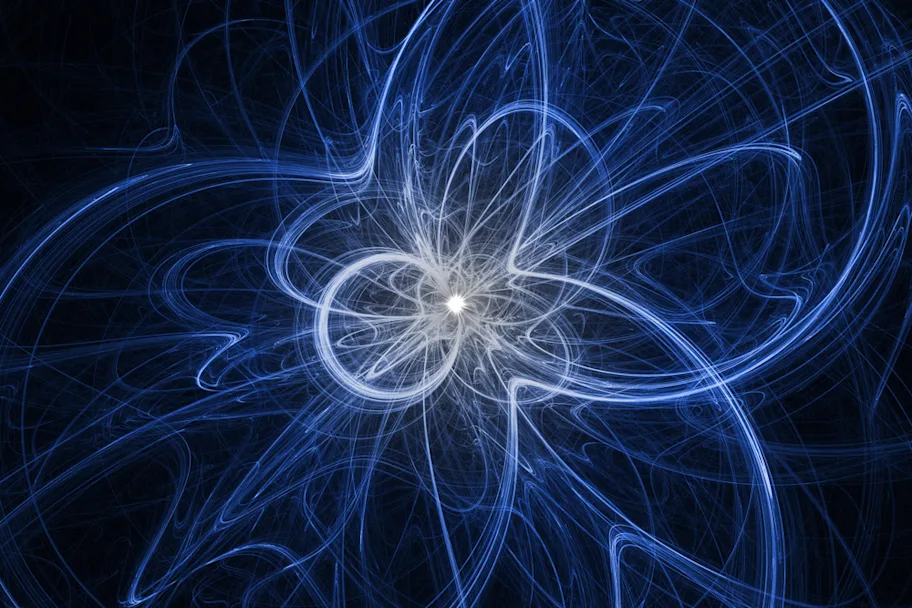
By Claudio Bogazzi, Frontiers
Frontiers in Physics recently launched a new Research Topic, titled “The Physics associated with Neutrino Masses”. This topic was conceived by three physicists from different institutes: Dr. Aristizabal, Santa Maria University (Chile), Dr. Deppisch, University College of London (UK) and Dr. Merle, Max Planck Institute for Physics (Germany). The idea behind it is to provide a platform for broad discussion about phenomenological and theoretical aspects of neutrino masses and their implications.
This topic focuses on one of the biggest challenges the physics community is currently facing. Neutrinos are subatomic particles with a mass so small and so hard to detect that even Wolfgang Pauli, the brilliant physicist who in the 1930s first postulated their existence, announced: “I have done a terrible thing, I have postulated a particle that cannot be detected”. To give you an idea, stop reading for just one second, and look at the palm of your hand. Did you notice anything? No? Well, you probably haven’t felt anything but about 1012 (a trillion!) neutrinos passed through your hand in that time. No wonder that Pauli was so pessimistic about their detection.
Fortunately, Pauli was wrong. In 1955, two American physicists, Frederick Reines and Clyde L. Cowan Jr. set up an experiment able to detect neutrinos emitted in a nuclear reactor. That’s right, a real nuclear reactor. Nuclear reactors are indeed expected to produce neutrino fluxes on the order of 1012-1013 neutrinos per second per cm2.
Since then, neutrinos have been a source of continuous surprises. First, the discovery that neutrinos exist in three types, or rather, three flavours, each one associated with a different charged particle. We have electron, muon and tau neutrinos. Then, the so-called solar neutrino puzzle: in the 1960s original studies by John Bahcall described in detail the flux of electron neutrinos we should expect from the Sun due the nuclear fusion reactions that power our star. Yet, when Raymond Davis, Jr. tried to measure this neutrino flux in the mine of Homestack, South Dakota, to shield his experiment from cosmic rays, he found approximately a third of what Bahcall’s calculations suggested. What happened to the missing neutrinos?
The answer of this question came almost two decades later, in 1989, when the Kamiokande experiment, based in the mine of Kamioka, Japan, managed to measure all neutrino flavours coming from the Sun. The combined flux of all three neutrino types exactly corresponded with Bahcall’s predictions. The consequences of this result, confirmed by other neutrino experiments in the following years, were shocking: neutrinos, while travelling, can change flavours or, to use a more scientific term, “oscillate” from one type to another.
The discovery of neutrino oscillations was the first hint of what physicists referred to as “physics beyond the Standard Model”. In order to oscillate, neutrinos must be massive. This is something not predicted by the Standard Model, a theory which mathematically describes all the known nuclear forces, electromagnetic, strong and weak, as well as classifies all the particles known. A massive neutrino could be a good candidate to partially contribute to that 23% of undetected matter in the Universe called Dark Matter. And it could also help to understand the asymmetry mass – anti-mass that we see in our Universe today.
A theory able to explain how and why neutrino have masses would represent a huge step forward in our understanding of the physical world. Neutrinos are the most interesting and at the same time the most mysterious among the particle zoo. And the best is yet to come.
Keep following Frontiers in Physics and our Research Topic: The Physics Associated with Neutrino Masses, for more news and articles about neutrinos. Oh, did I mention that neutrinos could be their own anti-particle?