- 1Department of Civil and Environmental Engineering, Imperial College London, London, UK
- 2Grantham Institute for Climate Change and the Environment, Imperial College London, London, UK
- 3Departamento de Ingeniería Civil y Ambiental, Escuela Politécnica Nacional, Quito, Ecuador
- 4Department of Civil Engineering, Universiti Putra Malaysia, Serdang, Malaysia
- 5Consortium for the Sustainable Development of the Andean Ecoregion (CONDESAN), Lima, Peru
- 6School of Civil and Water Resources Engineering, Institute of Technology, Bahir Dar University, Bahir Dar, Ethiopia
- 7Institute of Development Policy and Management, University of Antwerp, Antwerp, Belgium
- 8Society of Hydrologists and Meteorologists (SOHAM Nepal), Kathmandu, Nepal
- 9School of Geography, Earth and Environmental Sciences, University of Birmingham, Birmingham, UK
- 10Public Administration and Policy Group, Wageningen University, Wageningen, Netherlands
- 11Mountain Societies Research Institute, University of Central Asia, Bishkek, Kyrgyzstan
- 12Department of Biological and Environmental Engineering, Cornell University, Ithaca, NY, USA
The participation of the general public in the research design, data collection and interpretation process together with scientists is often referred to as citizen science. While citizen science itself has existed since the start of scientific practice, developments in sensing technology, data processing and visualization, and communication of ideas and results, are creating a wide range of new opportunities for public participation in scientific research. This paper reviews the state of citizen science in a hydrological context and explores the potential of citizen science to complement more traditional ways of scientific data collection and knowledge generation for hydrological sciences and water resources management. Although hydrological data collection often involves advanced technology, the advent of robust, cheap, and low-maintenance sensing equipment provides unprecedented opportunities for data collection in a citizen science context. These data have a significant potential to create new hydrological knowledge, especially in relation to the characterization of process heterogeneity, remote regions, and human impacts on the water cycle. However, the nature and quality of data collected in citizen science experiments is potentially very different from those of traditional monitoring networks. This poses challenges in terms of their processing, interpretation, and use, especially with regard to assimilation of traditional knowledge, the quantification of uncertainties, and their role in decision support. It also requires care in designing citizen science projects such that the generated data complement optimally other available knowledge. Lastly, using 4 case studies from remote mountain regions we reflect on the challenges and opportunities in the integration of hydrologically-oriented citizen science in water resources management, the role of scientific knowledge in the decision-making process, and the potential contestation to established community institutions posed by co-generation of new knowledge.
1. Introduction
Citizen science refers to the participation of the general public (i.e., non-scientists) in the generation of new scientific knowledge. A large variety of approaches exist, ranging from community-based data collection, to soliciting contributions, to carrying out various scientific tasks with the help of large groups of people through use of the internet (i.e., crowd-sourcing, Dickinson et al., 2012).
New developments in sensing technology (e.g., Hart and Martinez, 2006), data processing and analysis, and knowledge communication are opening new pathways for citizen science. Inexpensive yet robust sensors now can allow amateurs to collect large volumes of data and document them with appropriate metadata such as location and time. Information and communication technologies (ICT) facilitate the flow of data and knowledge, both for uploading collected data to centralized databases, and for querying datasets using rich and tailor-made interfaces. Lastly, communication technologies such as cellular networks and the internet also can allow for a much more dynamic and interactive approach to the formulation of hypotheses, research design, data analysis, and knowledge generation.
The provision of water resources is one of the most fundamental ecosystem services for humanity. Hydrological science underpins most decision-making on water resources and is the basis for assessing risks related to water such as floods and droughts. But despite its critical societal relevance, this area of science is characterized by an acute scarcity of data in both the spatial and temporal domains (e.g., Hannah et al., 2011), which contrasts significantly with the heterogeneity and complexity of actual water management and governing processes. As such, it is pertinent to reflect upon the potential role that citizen science could play in the generation of new knowledge in relation to the water cycle and related ecosystem services, and the use of citizen science in decision-making.
This paper therefore aims to provide a critical review of the available literature on citizen science in a context of hydrology, water resources, and wider ecosystem services management, and to seek and develop a new perspective on the major challenges and opportunities that may lie ahead. Section 2 explores the philosophy and motivations behind the citizen science approach. Section 3 reviews existing citizen science applications in hydrology and water resources sciences. This is followed by an overview of some of the major challenges and opportunities for citizen science for ecosystem services management and sustainable development (Section 4). Lastly, in Section 5, we illustrate the discussed concepts by means of four case studies from remote mountain regions in Peru, Ethiopia, Nepal, and Kyrgyzstan, with different levels of citizen science activities and interests. In these regions, environmental pressures on ecosystem services are particularly acute and a lack of information on physical processes hinders the generation of relevant knowledge for adaptation. As a result, there may be a strong potential for citizen science to complement classic scientific knowledge generation and bring benefit to local stakeholders.
2. The Concept of Citizen Science
2.1. Definitions
Citizen science is not an entirely new concept, but it is becoming increasingly popular in natural science research (Silvertown, 2009). In broader terms, public involvement in science has also been referred to as civic science (Irwin, 1995; Kruger and Shannon, 2000), community science (Carr, 2004), and more recently, public participation in scientific research (Bonney et al., 2009; Shirk et al., 2012). The philosophy is also affiliated to concepts such as community-based monitoring (Whitelaw et al., 2003; Fry, 2011), community-based management (Keough and Blahna, 2006), and crowd-sourced data collection (Lowry and Fienen, 2013). What separates citizen science from less collaborative forms of public participation in scientific research is the element of an “active” engagement (Wiggins and Crowston, 2011, p. 1); it is an approach whereby non-scientists are actively involved, to differing degrees, in the generation of new scientific knowledge, from which they also actively stand to benefit either intrinsically (e.g., increased scientific literacy) or extrinsically (e.g., increased social capital).
Citizen participation in science is a cross-cutting concept that has emerged from and across many disciplines. As a methodology, it has been promoted for interdisciplinary research as it automatically fosters collective reflection (Wechsler, 2014). In the social development context, public participation as a strategy for achieving good governance and human rights has been embedded into mainstream discourse for decades (Gaventa and Barrett, 2012). Political economists also promote democratization of information to encourage debates and improve policy-making (Ostrom, 1990; Macknick and Enders, 2012). Recent developments in information science (e.g., remote-sensing, open-source software tools, cloud computing) have been central to the emergence of citizen science in ecology and biogeography (Silvertown, 2009; Dickinson et al., 2012), where citizen science is also prominently applied [e.g., Fernandez-Gimenez and Ballard, 2008; Danielsen et al., 2009; Blossom, 2012; Stevens et al., 2013; Open Air Laboratories (OPAL), 2014; Paul et al., 2014]. In hydrology, the adoption has been relatively limited but is rapidly gaining momentum (see Section 3).
2.2. Motivation for Engaging in Citizen Science
Citizen science scholarship highlights the importance of citizen science in facilitating a better representation of local experiences and priorities. A strong motivation for the citizens i.e., the volunteers therefore is the scope that citizen science can offer for them to be part of the scientific process, and ultimately have a much broader role in decision making as part of a bottom-up, participatory process.
This debate on citizen science is a long standing one, and its roots can be traced back to the growing mistrust of citizens about positivist science, especially in the aftermath of catastrophic failures of risk regulation (Freudenburg, 2003). For example, in the 1970s at Love Canal, at Three Mile Island, and at Wolburn, Massachusetts (to name a few prominent cases) citizens conducted their own “science” assessments to better understand the risks associated with their own exposure to toxics, in response to the discovery of toxic waste presence (Levine, 1982; Brown and Mikkelsen, 1990). Through a process that Brown (1992) coined as “popular epidemiology,” they exercised their own political power in science communication, and used their own scientific assessments to challenge expert opinion. An important outcome of this was that citizens involved in these assessments were able to assert their authority as knowledge brokers and to become more centrally involved in the planning of decisions that concerned the area where they lived and worked. The appropriate technology movement and approaches to participatory technology development that became popular in the 1970s also reached similar conclusions, highlighting the importance of citizens in the choice, design and delivery of technological development.
Citizen science often emerges at the interface of political activism and volunteering. While on the outward they may come off as a hobby, motivational aspects are often complex. Volunteers' involvement may be self-initiated as driven by an environmental concern, as well as scientific curiosity and a sense of fulfillment for being part of finding answers (Cohn, 2008). On the other hand, members of a community whose livelihood depends on their local environment (e.g., subsistence farmers in developing countries) may also seek scientific knowledge to form a better understanding of their environment, and further use this to gain political leverage in their community (Overdevest and Orr, 2004).
Another strong motivational aspect of citizen science, but perhaps currently more from the professional scientists' point of view, is about showcasing diversity, such as in terms of the ecologies as well as practices that people depend upon to sustain their livelihoods. In mountainous socio-ecological systems, which form the basis of the case study examples represented later in this article, the diversity of interactions between social and ecological changes vary significantly not only across regions but also across communities. The case of Kyrgyzstan (Section 5.4) shows for example how communities are trying to adapt to, as well as comprehend, the change brought about in the aftermath of the collapse of the Soviet Union, and the particular economic and social restructuring process that subsequently followed. In the Nepalese context (Section 5.3), we see increasing interest by the citizens themselves to understand better the complexity of their own environments. In the light of this, it requires advocating that a stronger citizen involvement in information sharing and collection may ultimately lead to greater preparedness against abrupt change, and can potentially provide an additional buffer for dealing with those shocks and stresses that are likely to be brought about as climate change and variability becomes exacerbated. Living with uncertainty and change therefore also presents new opportunities for citizens to become key actors in knowledge co-generation processes, actively contributing toward comprehending “fuzzy” science arenas (i.e., climate change and hydrology) that are at present not just scientifically contestable, but at least to some extent unknown (Stirling et al., 2007).
On the other hand, professional scientists and donors are also driven by a number of motives. Apart from purely scientific interest in the data collected and self-advancement, they may also be humanistic-altruistically motivated, for example in scientific outreach and education, promoting conservation, and improving societal conditions (Macknick and Enders, 2012). However, in spite of any goodwill on the side of the professionals, volunteer motivations are highly dynamic, changing over time even if collective project goals remain constant (Rotman et al., 2012). Therefore, Rotman et al. (2012) suggests that particular attention should be paid to first contact between a citizen and the professionals, and also in the final stage when volunteers decide whether they will contribute to other initiatives. Interview studies in USA, Costa Rica and India found that motivations not only changed with time, but were also influenced by complex cultural and institutional settings. Specifically, Costa Rican projects benefited from a national culture of environmental awareness and social responsibility, together with shallow hierarchical structures that encouraged regular mentoring and the building of trust between scientists and citizens (Rotman et al., 2014a). For citizen science projects to achieve sustained volunteer participation, communication pathways between scientists, citizen scientists and non-participating community members need to be carefully considered. Attention needs to be paid to the clarity of training and the delivery of feedback throughout the project (Rotman et al., 2014a). If volunteers feel patronized or perceive that they are undervalued by professionals, the projects are likely to suffer from high attrition rates. This is likely to be of particular significance in cultures where science and society are clearly demarcated (e.g., India, Rotman et al., 2014b).
Research into collective intelligence suggests that bringing people together from diverse backgrounds promotes innovative thinking (Woolley et al., 2010). Furthermore, there is an opportunity in the design of citizen science projects by means of citizen involvement to affect outcomes (Shirk et al., 2012) e.g., the type of knowledge generated and the benefits to be gained from it. Involvement of volunteers from multiple segments of a community can result in an improved relationship between different institutional actors (Ridder and Pahl-Wostl, 2005), and this improves the prospects of future joint actions (e.g., continued monitoring, planning and implementation of adaptation programs). However, the opposite outcome is equally probable—conflicts may arise from failures in communication or goals (a case in point is the Ethiopian case described in Section 5.2). Indeed, citizen science questions the classical divide between “science” as the knowledge producing process, and the “users of that knowledge,” such as communities who might want to participate in “science” for educational purposes or to inform their actions/arguments with “true knowledge.” Instead, citizen science suggests a process of co-generation of knowledge, with a possible decentering of the central and key role of the scientific process in the knowledge production process. The complexity of social-ecological systems, their degree of inherent uncertainty and partially unpredictable interactions, may require a more interactive view on knowledge encounters and joint knowledge production in particular, in view of the creation of a sufficiently shared knowledge base to motivate adequately coordinated and coherent social action in poly-centric governance of ecosystem services (i.e., an evolving, emergent outcome of interactions between multiple sources of governance in state, market or civic realms and at different scales of action, Ostrom and Cox, 2010). We begin to see traces of this polycentricity and the centrality of knowledge in ensuring its sustenance when we explore the intricate knowledge sharing mechanisms that have begun to form ties between upstream and downstream water user communities in the Peruvian case (Section 5.1). But inevitably this brings in interests and power in terms of whose reality will prevail to support actions.
2.3. Levels of Engagement
The broad range of citizen science activities yields different classifications or spectrum of models. The Cornell Lab of Ornithology initially proposed a continuum of research models, based on the extent of citizen-scientist collaboration across each stage of the scientific process. This included the scientific consulting research model, participatory action model, and representing the most inclusive approach, the adaptive citizen science model which adopts a central platform for an interactive feedback mechanism (Cooper et al., 2007). Bonney et al. (2009) later proposed three models: contributory, collaborative, and co-created projects. Shirk et al. (2012) sub-divides projects even further with additional contractual and collegial models at either end of the public involvement spectrum. There is a high parallelism to the Extreme Citizen Science (ExCiteS) framework, which separates them into crowd-sourcing, distributed intelligence, participatory science, and extreme citizen science (Seymour and Regalado, 2014). Such schemes may be drawing inspiration from the debate about participation in development, e.g., Pretty's (1995) typology of participation: with control over the process running from full external control to dialogue and full internal control. From a monitoring perspective, Danielsen et al. (2009) identified five types of protocols based on the relative involvement of citizens and scientists in design, data collection, interpretation and utilization. Wiggins and Crowston (2011) developed five (i.e., Action, Conservation, Investigation, Virtual and Education) citizen science typologies by focusing more on the organizational and macrostructural elements of project design and implementation. In all, the different models essentially describe the same continuum of engagement as well as the benefit obtained by the public volunteers from the scientific research. There is more emphasis on building social capital (by training) and local adaptive capacity at the extreme end of this spectrum where the objective of public engagement is to improve transparency and accountability, and to encourage discourse, learning, and collective action between multiple stakeholders (da Silva Wells et al., 2013). This is complementary to the tendency now to move away from merely capitalizing on volunteers as a low-cost workforce but to involve them in the intellectual aspects of the science (Lakshminarayanan, 2007; Conrad and Hilchey, 2010).
3. Citizen Science in Hydrology and Water Resources Management
Given the advanced technology needed for monitoring many aspects of the water cycle, hydrology is not an evident scientific discipline for the application of citizen science. But the development of more robust, cheaper and lower maintenance sensing equipment creates new opportunities for data collection in a citizen science context. At the same time, the importance of water resources for human development, and the threats that emerge from environmental change, freshwater contamination, population growth and other stressors (Milly et al., 2008; Hipsey and Arheimer, 2014; IPCC, 2014) highlight the need for novel approaches to both the generation of new knowledge about the water cycle, as well as new insights as to how this knowledge can be used in water resources management that supports sustainable development. The next sections review existing citizen science applications and opportunities brought by new technology in hydrology, before exploring their relevance for water resources management in Section 4.
3.1. Literature Review
Table 1 summarizes our review of documented citizen science projects in hydrology and water resources. From this list, we draw several inferences. Firstly, the scope is rather limited to water quality monitoring, possibly due to the relative ease of conducting measurements of water quality parameters and therefore also coordinating these activities at large scale that include training and distribution/sale of measurement kits. Secondly, there is a clear geographic bias, with activities primarily occurring in developed countries. There are more than 1800 programs in the United States alone (Volunteer Water Quality Monitoring, 2014). These observations are not inconsistent with citizen science applications which are more advanced in other fields such as ecology and biogeography. Thirdly, in all the reviewed cases except one, the level of involvement by non-scientists are limited to data gathering. This clearly represents only a small subset of the levels of engagement reviewed in Section 2.3. As a result, the full potential for problem-identification, data co-creation, and a joint analysis and co-generation of actionable knowledge (fully explored in Section 4) is not realized.
3.2. Problem Definition, Project Design, and Experimental Science
One explanation for the limited depth of engagement by non-scientists as revealed in Section 3.1 may be the geographical bias toward wealthy regions, instead of in areas where socio-ecological problems have more serious consequences (Halkay, 2011). Indeed, especially in cases that aim for sustainable development and poverty alleviation, such as those presented further in this paper, there is a clear need for a more bottom-up, inclusive approach to the identification of most pressing concerns for action, problem definition and project design (Srinivasan et al., 2013). The social objective of citizen science projects in wealthy regions more often is to increase awareness and scientific literacy, as opposed to developing regions where the goals are more related to enhancement of community well-being such as poverty alleviation (Gura, 2013). Such definition of objectives and priorities necessarily results from political and social processes of constructing management narratives within specific frameworks or “epistemic communities,” consisting of “experts sharing a belief in a common set of cause-and-effect relationships as well as common values to which policies governing these relationships will be applied” (Haas, 1989, p. 384). These processes in their turn will have an impact on data collection and knowledge generation, e.g., the variables that will be monitored and the points of data collection. As a result, it is important that the citizen science process takes explicitly into account these multi-stakeholder processes of constructing the problems and frameworks, rather than a belief in an “objective” or “neutral” scientist-initiated approach. The application of citizen science in a water resources management context is clearly in its infancy in this regard, and in need of the adaptation of existing and the development of new frameworks to move from a technocratic-expert view on decision-making support toward a joint creation-knowledge exchange model. This is further discussed in Section 4.1.
A second potential reason for the hitherto limited uptake of citizen science in water related sciences is the difficulty of conducting experiments. In experimental science, system variables are manipulated and the resulting outcome is observed to improve the understanding of processes and cause - effect relations. Such experiments are promising in a citizen science context given the direct link between cause and effect and thus their potential educational value. However, in hydrology and water resources controlled experiments are difficult and often risky to set up, not only because of the large variety of uncontrolled boundary conditions (e.g., land use change, climate conditions), but also because of the potentially wide-ranging and irreversible effects of perturbations to the water cycle. Yet examples exist. Section 5.2 discusses the implementation and success of a small-scale experimental approach to assess the effectiveness of restoration strategies in the Ethiopian uplands. In the Peruvian case (Section 5.1), such a controlled approach is not possible, and therefore a comparative analysis of land-cover types is used as a proxy (sometimes also known as a “trading-space-for-time approach” Singh et al., 2011). However, in other cases simulation experiments will be the only alternative to experiments (Beven et al., 2012, Section 3.5)
3.3. Monitoring, Data Collection
Hydrological data collection typically relies on technologically complex and expensive measurements. Additionally, because of the large temporal variability of the water cycle, hydrological data analysis requires repeated measurements such as long time series of hydrological states and fluxes (e.g., precipitation, discharge). Classic hydrometric practice has often been confined to the professional environment and tailored to the specific needs of official monitoring networks (Herschy, 2009). This contrasts with a citizen science approach to data collection, which may require the compromise of data quality due to the use of different equipment, less frequent sampling, and less trained workforce, and which may be ephemeral in nature (Cohn, 2008; Devictor et al., 2010). The design of the monitoring may need to be technically simplified, and the procedure unified, so consistent samples can be generated; additionally, inferences need to be made from a larger volume of potentially lower quality data, which requires a different approach to data analysis (Cohn, 2008). At the same time, new technological developments also provide new opportunities for citizen science based monitoring (Table 2).
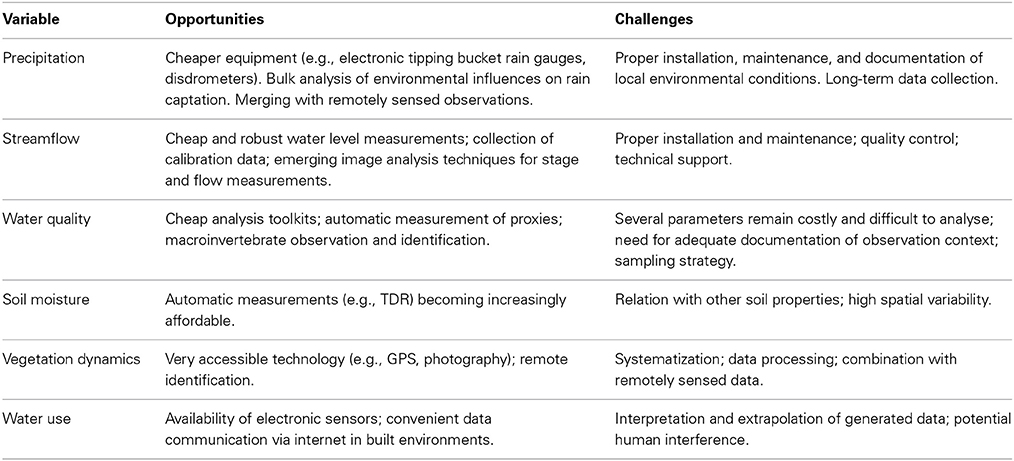
Table 2. Commonly measured hydrological variables, and identified challenges and opportunities emerging from citizen science applications.
3.3.1. Precipitation
The simple design and affordability of rain gauges makes them particularly suited for application outside the traditional monitoring environment. Electronic sensors such as tipping bucket switches allow for convenient automatization. Yet, rain gauge measurements are very sensitive to the local aerodynamic conditions (World Meteorological Organization, 1994), and non-standard locations or varying local conditions (e.g., undergrowth, vegetation blockage), thus affecting the quality and interpretability of measurements. Hence, in a citizen science context, it will be crucial to document properly the local setting of the rain gauge and to apply and/or develop robust quality control protocols. New sensor technologies have the potential to make precipitation measurements more affordable and less prone to errors. For instance, disdrometers are increasingly used in precipitation monitoring, and are both cheap and robust in their measurements (e.g., Löffler-Mang and Joss, 2000). Other, more experimental methods for precipitation measurements, such as cellular communication interference (Overeem et al., 2013), are under development and may eventually provide new possibilities for citizen involvement.
3.3.2. Streamflow
Streamflow measurements are complex by nature, because most methods are based on indirect measurements such as flow velocity, cross sectional area and/or river stage, which are subsequently converted into volumetric flux (Herschy, 2009). Traditional water level monitoring is complex and requires extensive maintenance, and is further complicated by property laws, regulations and other legal issues. Nevertheless, cases of successful measurement of river level and flow in a citizen science context exist, such as the Peruvian case (Section 5.1). At the same time, new methods are emerging that are more compatible with citizen science approaches. For example, camera-based water level measurements have been shown to have a good relative agreement (within 16%) with a traditional installation (Royem et al., 2012). These can be further improved by combination with emerging remote sensing methods, such as high-resolution digital elevation and river bed mapping from terrestrial, airborne and space measurements (e.g., Alsdorf et al., 2007; Smith and Pavelsky, 2008; Sampson et al., 2012).
3.3.3. Water quality
Citizen participation in water quality monitoring is often limited to the collection of data in the form of water samples, basic hydrochemical parameters, or other indicators (e.g., macroinvertebrates) because of the specialized knowledge and/or equipment needed for more advanced analysis (Overdevest and Orr, 2004). Benchmarking studies of the accuracy of the data collected by citizen scientists have reported differing outcomes (Au et al., 2000; Nicholson et al., 2002; Canfield et al., 2002; Shelton, 2013). Nevertheless, new sensing technologies open up perspectives for a wider range of data collection. Aquatic sensors increasingly incorporate water quality related measurements such as temperature, dissolved oxygen, turbidity, conductivity/salinity, pressure, redox potential, and pH, so there is already opportunities to automate the data collection for continuous sampling. An example of this under development is the WaterBot system that includes a Sensor that continuously measures temperature conductivity, a Gateway that relays this information using wifi to a central data server, and a Wiewer that enables real-time observation (CREATE Lab, Carnergie Mellon University, 2014).
3.3.4. Soil moisture
Soil moisture measurements are increasingly automated, e.g., by using time domain reflectometry (TDR) sensors and related technology. Automated measurements are typically still less accurate for estimating actual volumes, and may suffer from specific technical limitations related to the range of soil conditions for which the sensor is useful operationally, such as variable apparent permittivity, and frozen soils. Yet, the measurements can be useful to capture moisture fluctuations in the ground after precipitation events. The increasing affordability and robustness of the technology also allows for a broadening array of temporary and continuous uses, especially in an agricultural context, e.g., irrigation.
3.3.5. Vegetation dynamics
In a hydrological context, vegetation dynamics are important for their impact on the distribution of hydrological fluxes including interception of precipitation, canopy evaporation and surface runoff generation. Some of these fluxes can be measured with above mentioned technologies such as rain gauges and soil moisture sensors. But the spatial interpolation of these processes, for instance in hydrological models, still relies on a limited number of highly generalized vegetation parameterization (e.g., Clark et al., 2011). While remotely sensed methods for vegetation characterization are improving rapidly, there remains a need for field verification of vegetation types, spatiotemporal dynamics of vegetation change, and aspects of ecosystem system stability, which cannot be observed through remote sensing. The use of accessible technologies such as geotagged photography may provide opportunities for stronger involvement of non-experts in data generation and local evaluation of remotely sensed products. Such data may even be sourced from public repositories of photographs if adequate metadata (e.g., location, time) are available.
3.3.6. Water use
While the abovementioned methods focus on the measurement of fluxes that determine water availability, sustainable water resources management is often as much hindered by a lack of a proper characterization of water demand. Therefore, quantification of abstraction volumes and other types of water use may play a crucial role in the optimization of water resource management. Technology exists for the direct (e.g., water meters) or indirect (e.g., canal level) measurement of such fluxes. The advent of smart sensors allows for such measurements to be distributed and integrated in more complex analysis systems. However, conflicts, misuse, and data tampering may emerge if the role of water use data for example, in water allocation, becomes too direct.
3.4. Data Transmission and Processing
Most hydrological measurements have a low temporal frequency (e.g., hourly rainfall, daily streamflow measurement) which facilitates automatic transmission using internet or mobile phone connections. Even in remote regions such as mountains, mobile phone coverage is increasingly common. For instance, statistics of the (International Telecommunication Union, 2014) show that mobile phone penetration in countries such as the Kyrgyz Republic amounts to 92%. Even when mobile phone networks are not equipped for internet access, data transmission via daily text messages is a viable option. Telephone coverage in Nepal, a less developed country with extreme topographical variations, has crossed 70% of the population covering the whole country, out of which around 11% have access to internet. But low education levels and unavailability of data transmission in local language have limited its use in rural areas.
As sensors become increasingly automated and easy-to-use, standards are emerging for interfaces that plug into a variety of devices such as mobile phones and home networks. Web-based services make it easy to connect devices and products with applications to provide real-time control and data storage (Williams et al., 2011; Vitolo et al., in press). Data exchange standards such as the OGC Sensor Observation Service (e.g., Williams et al., 2011) and related assimilation methods facilitate the integration of data from citizen science with other data sources and process them in near-real time.
Data robustness can be evaluated by comparing across samples (Cohn, 2008), and it is possible for this quality-assurance procedure to be fully integrated in the real-time data collection. For explicit treatment of uncertainties, benchmarking studies of citizen science against conventional technologies/strategies could be completed before data collection commences, and examples of these are as discussed above in Section 3.3.
Assimilation of uncertain data in hydrological simulation models is a well developed and active research field (Beven, 2009). Yet, hydrological models still typically require specific, high-quality and long-term time series for calibration and validation, which may not be compatible with the distributed, temporary, and potentially lower-quality nature of citizen-science generated data. The integration of citizen science data with traditional data sources through interpolation or other merging techniques to create combined datasets may be one approach to solve this issue. For instance, methods to merge rain gauge data with satellite imagery to generate gridded precipitation products are well developed (e.g., Sheffield et al., 2006) and may highlight the value of short duration ground based measurements to locally calibrate large scale products.
As for the calibration of hydrological models, Seibert and Beven (2009), among others, highlight the value of short-duration discharge time series for the calibration of hydrological models. Distributed measurements, even of short duration, may also be particularly useful for regionalization of hydrological models (Vandewiele and Elias, 1995; Parajka et al., 2005; Buytaert and Beven, 2009). Lastly, multi-objective calibration methods can address the issue of heterogeneous data availability of river basin modeling (e.g., Vaché et al., 2004; Parajka et al., 2007).
For hydrological models to be incorporated in decision support systems, modeling systems will need to represent the decision-making processes. Common methods include multi-agent system modeling, agent based modeling, and coupled human-environment system modeling (Gunkel, 2005; Srinivasan et al., 2013), among many others (e.g., Harrison et al., 2007; Matrosov et al., 2013; Zhang et al., 2013). One particular value of this modeling approach for participatory data collection is in the integration of uncertainties, the treatment of incomplete data (Uusitalo, 2007), and the continuous updating of the model parameters with the incoming stream of data (Castelletti and Soncini-Sessa, 2007a).
3.5. Communication of Results, Scenario Building, and Participatory Modeling
Citizen science participation in data collection is probably the more straightforward type of engagement. A more fundamental way to enhance citizens' participation to the science production process is through joint analysis of the obtained data, which requires platforms for visualization and communication of results, joint scenario and hypothesis formulation, as well as data processing and hypothesis testing. Many relevant concepts and technologies have recently been developed in the context of science-supported decision support mechanisms (e.g., Olsson and Andersson, 2007; McIntosh et al., 2011).
Visualization and communication ensures that scientific content is comprehensible for the layperson, so that citizen scientists can directly gain from participation, which will also incentivise them for further engagement. This process is facilitated by the increasing availability of web-based technologies, which allow for rich, easy to update graphics potentially embedded in tailored applications and user experiences intended for a non-technical audience (so-called infographics, Spiegelhalter et al., 2011). Such systems improve communication outside the scientific discipline. Their interactive nature allows for feedback, commenting and rebuttal. The most immersive and interactive of such applications are sometimes referred to as Environmental Virtual Observatories (Beven et al., 2012; Buytaert et al., 2012), referring to the combined use of observed and virtually enhanced environmental data. But given the potentially very different nature and quality of citizen-science generated data, novel methods for data quality control, assimilation, and simulation will need to be developed. A major challenge in such applications is the communication of indirect observations and modeling results (e.g., interpolation and simulations), including their assumptions and uncertainties (Olsson and Andersson, 2007; Beven et al., 2012).
Such virtual platforms also often provide a convenient and engaging platform for facilitating scenario building and hypothesis formulation, for instance as a graphical support for classic participatory scenario building (Andersson et al., 2008; Faysse et al., 2012). More advanced virtual approaches to scenario building are simulation games (Le Bars and Grusse, 2008). An example of such approach is the Spatial Information Exploration and Visualization Environment (SIEVE, O'Connor et al., 2005), which employs a gaming engine for 3D visualization of geospatial data, which can be both raw (e.g., digital terrain) and modeling output. Their work focused on the visual communication, for example, by introducing a switch option between environments that are tailored toward experts vs. non-expert (“multiskilled environment”). This approach assumes that a layperson connects better to the information that is visualized using textures (“realistic”) instead of symbols (“abstract”). Additionally, the environment allows for multi-way interaction via a chat window. This again enables joint-testing of new hypothesis (i.e., co-creation of new knowledge), even if the modeling is done offline by the only by the expert, who returns to report the outcomes of the experiment.
A similar early experiment utilized the virtual environment of the local landscape projected in 3D on a large screen for an immersive experience (Stock and Bishop, 2005). Here, a group of people in the same physical location interact with this virtual environment using a handheld computer device, voting on different scenarios and varying degrees of land use changes that are more aligned with their local experience. The impact on water volumes and quality as associated with each land cover change as modeled by simple GIS-based models is also displayed. This system allows the simultaneous incorporation of traditional knowledge from multiple non-scientists and is a useful method to finding a point of agreement between the experts and non-experts in terms of the most relevant hypotheses (in the form of scenarios) to test.
Yet, the most immersive collaboration between scientists and non-scientists is through joint data analysis aiming at hypothesis testing and knowledge generation. The most common approach to this is the use of participatory modeling (Castelletti and Soncini-Sessa, 2007b; Olsson and Andersson, 2007). In this process, actors from different backgrounds jointly construct a model of the system under study. The purpose of this process is often direct decision-making, but may extend to social learning (Hare, 2011). In the latter case, models are used to facilitate sharing perspectives and to clarify arguments.
Bayesian belief networks are often suggested as tools for participatory modeling, e.g., in the groundwater application of Henriksen et al. (2012). In this modeling approach, a system network is constructed that link various biophysical processes to environmental outcomes, and various decision scenarios can be explored to predict impact to the outcomes. Advantages of Bayesian networks include their conceptual simplicity, graphical support, friendly semantics, and possibility for interaction among different actors (Castelletti and Soncini-Sessa, 2007a; Henriksen et al., 2007). The development of a Bayesian network representation of the system under study, either offline or online, may help in structuring the thinking process, identifying causal relations, integrating different knowledge bases, and making assumptions and limitations of datasets and models more transparent (Henriksen et al., 2007; Olsson and Andersson, 2007). Nevertheless, setting up successful participatory monitoring activities requires not only strong buy-in from relevant stakeholders, the process itself is time-consuming and relies upon a high level of leadership and interpersonal skills (Henriksen et al., 2007).
4. New Challenges and Opportunities for the Use of Citizen Science in Sustainable Water Resources Management
If citizen science aims to be effective in producing positive effects on the governance of natural resources such as water, it needs to relate to the myriad of actors whose actions interact with the hydrological processes and who play a role in the explicit or implicit governance of the resources. Since the governance of natural resources in general, and water in particular, is inherently multi-scalar and polycentric (Ostrom and Cox, 2010), we cannot suffice with a too simple technocratic model, where the “objective” expert-scientist (making use of citizens to co-generate data for the scientific models) provides the “real knowledge” to users-decision-makers. The relationships between “knowledge,” “scientific knowledge,” “decision-making,” and “actions” should be conceptualized differently, and more in line with ideas of polycentric, multi-scalar governance in complex social-ecological system (Ostrom and Cox, 2010).
The concept of citizen science with the term “citizen” and its political connotations, suggests a political—as opposed to a technical-neutral—dimension, and it requires a decentering and different articulation of the scientific knowledge process within the broader social process of co-creation of sufficiently shared “actionable knowledge” that becomes the basis of changed “actions” within the management of resources. It is crucial to think about this process in terms of “knowledge encounters,” or even “battlefields of knowledge,” where different bodies of knowledge and meaning, are vying with each other to make sense of complex reality, and strive to occupy a prominent place in the multi-scalar, poly-centric decision-making about the actions that affect livelihoods (also to be understood beyond a mere material content as sense-making for a “life one has reason to value”) and the use of the resources such as water about which science makes a knowledge contribution in interaction with the stakeholders. Of course, different from certain scientists, people are not hydrologists; they are not only interested in water, even when it might be of crucial importance to them. This also implies that the ideas and institutions that, respectively, inspire and guide the actions of people are usually not specialized, nor focused on particular issues. The inevitably reductionist approach of most scientific analysis, however, requires a focus on particular resources, and therefore also tends to look for, or contributes to the creation of specialized institutions for the governance of that particular resource. In practice, much of the relevant actions of citizens are however not inspired and determined by such specifically crafted institutions, but rather the outcome of a process of “bricolage” (Cleaver, 2002), an interactive encounter of existing (multi-purpose) institutions (i.e., contributing to make sense of the life of people and not just of water or another ecosystem service) in which sensible ideas, rules and practices can -interactively- become incorporated in the received wisdom of existing societies (Hall et al., 2014).
4.1. Toward a Model for Joint Knowledge Creation and Exchange
The space for most productive collaboration is where citizens' and scientists' questions overlap and where the mutual benefit of actors can be optimized. Citizen's questions can be quite different from those of professional scientists. Even in such conditions, citizen science projects may be successful if mutual benefits outweigh the compromise of adjusting research questions to the interests of the other parties. From a professional scientist perspective, immediate benefits will often consist of help with data collection and analysis with the aim of creating general knowledge, while for the involved citizens, immediate benefits may result from the relevance of the gathered data for local issues.
However, as argued above, citizen science may open perspectives for a more fundamental model of mutual benefit, which relies on joint knowledge creation and exchange. Especially in the context of ecosystem services management, multiple forms of local or situated knowledge exist within rural communities. These include traditional knowledge, i.e., intergenerational understanding of ecosystems and beliefs that condition ecosystem services (Berkes, 1993), as well as more recent situated understandings of ecosystem services and associated management practices (Robertson and McGee, 2003) and personal insights derived from individual experience (Fazey et al., 2006). Such local knowledge contrasts with more formalized scientific knowledge on ecosystem services, often generated by external agencies (including extension agencies, rural experts and researchers) and based on hypothesis building and testing. Connecting these different forms of knowledge goes beyond the recording of data and requires sustained interaction between citizens and scientists such that scientific and local knowledge systems can enrich each other.
Research on ecosystem services management confirms the benefits of knowledge exchange and knowledge co-generation, both within remote rural communities and between communities and external agencies (Cundill and Fabricius, 2009). These include the production of hybrid (local/science), user-oriented knowledge, potentially enabling more efficient and effective resource use in remote rural areas (Berkes, 2009); greater community involvement within marginalized groups, leading to enhanced social capital (Cundill and Fabricius, 2010) and resilience (Lebel et al., 2010); and improved trust and reciprocity between external agencies and rural communities (Jodha, 2005). Overall, several potential benefits emerge from establishing forms of knowledge co-generation. The most direct outcome may be a better management and benefit extraction from ecosystem services. Secondly, new knowledge can improve access of marginalized and excluded groups to ecosystem services. Lastly, the process of co-generating knowledge affords greater participation by marginalized groups in the development of community-wide strategies and plans to access ecosystem services.
The potential of citizen science to achieve such objective is strongly related to the nature of social action and governance of natural resources. The real world governance of natural resources is an evolving, emergent outcome of interactions between multiple sources of governance in state, market or civic realms and at different scales of action (Ostrom and Cox, 2010). Policy-oriented scientific activity in general, and the citizen science mode in particular, cannot escape and will therefore need to engage actively in these interaction, which generate actionable knowledge in and for multiple arenas.
Citizen science that aims to promote democratic inclusion of poorer groups in society can select problems that are primordially raised and framed by the concerns of these priority groups. Scientific knowledge (and scientific activity) about these problems can then not only increase evidence-based understanding of these particular concerns, but also contribute to enhanced legitimacy of these issues within the local political arenas and contribute to balancing unequal power relations without being too optimistic or naïve about the possibilities to achieve substantial results. The link of the participative scientific activity with critical concerns and explicit or implicit struggles in local political arenas will be an important source of motivation for local people to participate in the scientific knowledge generation process. We elaborate on some of the preconditions for this process in the following sections.
4.2. Legitimacy of the Generated Knowledge
If citizen science generated knowledge is to be used to support decision-making, it is important to ensure that there is a strong foundation of trust and legitimacy among the involved actors, and to be attentive toward how those become effectively ascribed to different bodies of knowledge. This also implies a need to focus explicitly on discursive struggles within local decision-making arenas, as the construction of evidence-based “expert knowledge” can quickly become decontextualized inputs for the generation of certain policies and institutional logics molded by local power geographies (e.g., Büscher, 2012). Often in water resources related bureaucracies of the developing world (i.e., where decision-making around hydrology, water resource management and technology development tends to take place almost exclusively by scientists), it may be difficult for citizen science accounts to feed into decision-making – that is, even when that knowledge is being collected through an institutionalized process (Birkenholtz, 2008; Mollinga, 2009). This is partly because it may not adhere to the same evaluation and validation criteria that scientific hydrological knowledge needs to adhere to or because of misalignment and contestation between what citizen knowledge is proposing and what is being proposed by the scientific knowledge in question (Fischer, 1993; Dunn et al., 2008).
This apparent tension between classic scientific knowledge and citizen science highlights that questions around how knowledge is created and represented within a socio-institutional context are at least as important as the design and practice of collecting citizen-based evidence in the first place. This issue of knowledge representation and legitimacy can be complex, for example, when citizen accounts rest primarily on tacit or experience-based accounts of context specific concerns. While this paper is focused more on the useful inputs of citizen science in hydrology, as opposed to processes of contestation and legitimacy per se, we recognize that in addition to questions about the characteristics of the citizen knowledge itself, we also have to ask how we can ensure that this kind of knowledge is likely to feed into democratic institutional structures and has an equally meaningful and durable impact on deliberative decision-making processes, given that we also recognize that knowledge is political. In low-income countries where citizen based information may open opportunities for achieving a range of ecosystem service safeguards and poverty alleviation outcomes, raising this question is particularly pertinent (Daw et al., 2011).
4.3. Improving Citizen Access to Decision Making
Citizen science's democratic nature may promote a more equitable form of decision-making by giving citizens more information about natural resources and allowing for meaningful contributions to discussions and policy-making (Bulkeley and Mol, 2003). The use of collective intelligence to refashion more traditional scientific practices can push the boundaries for understanding and inventiveness using the ideas from a larger set of people, thus promoting inclusiveness (Seymour and Regalado, 2014). However, in practice, inclusion may still be biased as volunteers in citizen science projects tend to be people who are interested in and committed to closer engagement with specific projects, or forms of scientific enquiry (Cohn, 2008). It may also appear that the outcome of participatory workshops, for example, are democratic, consensual conclusions by all participants, whereas in practice they often mainly represent the dominant mainstream views that are allowed to surface into the public arenas, thus transforming “participation” into a tyranny of the dominant and the mainstream. Similarly, although new technologies can attract a large sector of the society, the exclusion of the remaining sector who are averse to them or lack the competency to learn them is not fully preventable (Newman et al., 2012). Thus, a significant amount of outreach efforts is required to reach out to the segments of society for whom the project can have the most life-affecting outcomes.
Furthermore, in a context of sustainable development, almost by definition (Bastiaensen et al., 2005), the poorer people will have difficulty to voice their concerns and even more to articulate them to (scientific) outsiders. So more often than otherwise, we therefore expect that participative citizen science will address more mainstream concerns of the local majority, or even of small dominant local elites. But in view of the post-research application of scientific findings, this usually has the advantage to facilitate rallying more and more relevant local support to implement recommendations through social action, while evidently risking to bypass critical concerns of disadvantaged groups and even to contribute to the deepening of their dispossession and exclusion. This constitutes a tricky, but often unavoidable dilemma in the implementation of citizen science, and requires a careful analysis of the social interface between the citizen science practices and the learning and struggle in local political arenas. By no means, however, does this dilemma reduce the potential contribution that science and citizen science can make to local bricolage toward better governance.
4.4. Feedback Loops in the Uptake of Citizen Science Based Knowledge in Decision-Making
Being locally relevant and legitimate is a precondition for citizen science-based knowledge to be useful for decision-making. But to ensure knowledge uptake, the link between citizen science and decision-making needs to be clear and transparent. This link can be understood in terms of feedback loops at different levels, and is probably most promising when it is multi-functional across these different levels. At the individual level, an immediate feedback loop depends on the relevance and usefulness of the information collected by the citizen scientist for his or her individual decision-making. This depends also on technical possibilities or design features such that the individual citizen scientist can actually read out, understand and record the information rather than just passing on unaccessible or unintelligible bits. Another feedback loop is possible at the level of collective decision-making, be that national, regional or local, e.g., when a water user association relies on information collected by one or some of its members to take decisions about the distribution of irrigation water. Lastly, yet another feedback loop is possible when scientists are involved in a process of knowledge co-generation, where they combine locally collected data by citizen scientists with other existing datasets to add value to that information, and make the results accessible for individual and collective decision-making arenas.
4.5. Fostering Long-Term Citizen Involvement
Involving citizens from the start has the potential to enhance the prospects that the knowledge generated will be locally relevant of its long-term sustainability, especially if the setup is interweaved within existing institutional structures and if the connection to local societal benefit exists (Danielsen et al., 2005). An ideal outcome would be that the most underprivileged sections of the community have a sense of ownership over the system being collaboratively set up and can attune it toward answering questions that concerns them. For this to be realized in practice however, each knowledge and valuation system would need to be understood as legitimate in its own right, even when citizen- and science-based knowledges are pursued separately (Tengö et al., 2014). In pluralizing knowledge systems for water resource management, one must also remain attentive to the power relationships which influence cooperation and learning between disparate knowledge systems (Pahl-Wostl et al., 2007). Toward this end, it is fundamental to acknowledge that there will always be power issues when connecting different branches of science with citizen-derived knowledge (Agrawal and Gibson, 1999; Keeley and Scoones, 2000; Karpouzoglou and Zimmer, 2011). Thus, despite existing opportunities in hydrology for identifying areas of complementarity between different knowledge systems, it is essential to remain attentive to the fact that there are always aspects of each knowledge system that cannot be fully translated into each other, partly because of the different approaches that exist (within disciplines and across different knowledge systems) to generate, validate or award legitimacy to knowledge (Schweizer, 1998; Martinez-Alier, 2014).
A different kind of institutional support is necessary to foster the kind of dialogue across different knowledge perspectives. This may imply, for instance, that research funding includes support for the development of strong interpersonal connections in more open knowledge arenas (Cornell et al., 2013). Without these connections any efforts to address the important perspectives of citizens are likely to appear piecemeal or biased by the established scientific disciplines and “epistemic communities,” and institutional norms toward the validation of knowledge in research programmes.
In practice however, a more pragmatic approach may be needed. For instance, experience from the Tana Lake case study (Section 5.2) learns that unlike citizen science in developed countries where volunteering is a pastime activity, in developing countries, the citizen observers very often derive their income from their engagement. The concept of volunteering and sending data to a central point is not very well established, and it may be necessary to employ people at local wages, to incentivise participants while the value of their participation in and the outcome of the monitoring activity are still uncertain to them. However, one must be aware of the danger of “motivation crowding-out,” i.e., the fact that monetary or in-kind payments (i.e., extrinsic motivation) for “socially-expected behavior” can crowd out more intrinsic behavior (originally treated in more psychology-related and economics literature; e.g., Frey, 1997) and future social motivations.
5. Case Studies
In this last section, we present 4 geographically dispersed case studies with either active citizen science initiatives or local interest in setting up such activities. They serve as an illustration to the viability and relevance of the concepts reviewed above. The cases are located in remote mountain environments, which are typically environments that combine fragile and understudied natural systems with accelerated environmental change and vulnerable populations that are strongly reliant on ecosystem services. In such a context, it is pertinent to investigate the potential of more inclusive methods to generate knowledge on improved uses of ecosystem services that supports sustainable development and improves human well-being. The discussion focuses particularly on:
• The motivation and level of engagement by the non-scientists,
• The type of data monitored, and potential for participatory modeling and knowledge exchange,
• The legitimacy of the generated knowledge,
• The sustainability of the citizen science activity,
• Potential uptake in decision making/resource governance.
5.1. Peruvian Andes
Andean water resources are extremely variable in time and space. This is most notable on the Pacific side of the Peruvian Andes, where annual rainfall varies from virtually no precipitation at sea level around the city of Piura (except for El Niño years), to 1500 mm at 3500 m altitude (Figure 1). Humid air masses originating from the Amazonian region pass the Huancabamba depression and penetrate a few tens of kilometers into the basins of the Chira and Piura rivers on the Pacific slopes of the Andes. The water that drains to the Pacific side of the Andes through this mechanism is of vital importance to small-scale as well as industrial-scale agriculture in the desert plains in several thousands hectares irrigation schemes such as San Lorenzo, Alto Piura and Bajo Piura. A new project of diverting a tributary of the Amazon, the Huancabamba river, through a trans-Andean tunnel, toward new desert lands, is currently under construction.
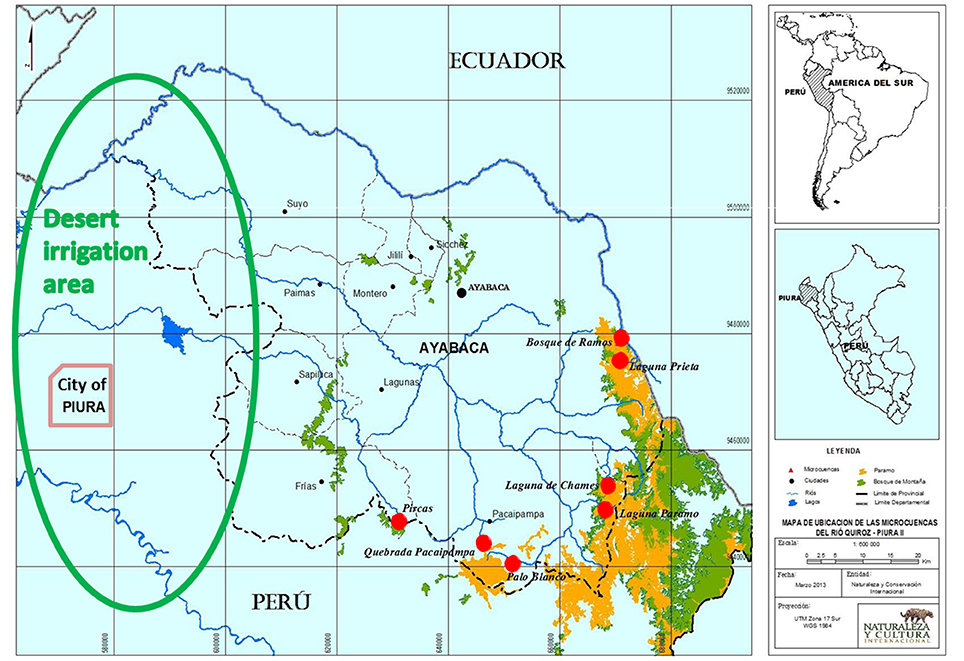
Figure 1. Regional map of the Piura region showing the location of the iMHEA citizen science monitoring in the wet uplands (red dots).
Despite the economical importance of these irrigated lands and the crucial dependence on highland hydrology, attention for highland conservation was marginal until recently and knowledge of the highlands hydrology, including basic parameters such as rainfall input is very limited. However, in the Quiroz river subcatchment, local stakeholders have now established a common platform to discuss benefit sharing mechanisms in the catchment. These stakeholders include upstream subsistence farmers, downstream agrobusinesses and smaller farmers depending on irrigated agriculture in the desert, water authorities, and regional environmental authorities. This platform is in urgent need of relevant information that can feed into discussions and negotiations. Key information needs are current surface water availability and its spatiotemporal variability, as well as the potential impact of changing land uses in the upper catchment. The case is one of several that are accompanied by the “Incubator of Mechanisms for Retribution for Ecosystem Services,” an initiative of the Ministry of Environment of Peru.
As a result of these drivers, the highlands of Piura have pioneered in citizen science-based hydrological monitoring led by a regional NGO (Consortium for Sustainable Development of the Andean Ecoregion, CONDESAN). The initiative for Hydrological Monitoring of Andean Ecosystems (iMHEA in Spanish, CONDESAN, 2014), was established in 2009 (Célleri et al., 2010). The network focuses on pairwise catchment monitoring to identify the hydrological impacts of land-use change, and the benefits of restoration activities. At present, it consists of 20 catchments in the tropical Andes (Venezuela to Bolivia), including 5 catchments in the Piura highlands (Figure 2). Monitored variables are rainfall and streamflow, in some sites complemented with meteorological variables to calculate reference evapotranspiration. Field monitoring is performed by non-specialist/non-researcher staff, but regular capacity strengthening events are organized by a coordinating NGO with support from regional and international scientists. In the case of the Rio Quiroz catchment, the monitoring is operated by an NGO, which acts as facilitator for the discussion platform, in close collaboration with the upland municipalities that are interested in quantifying the water they are “offering.”
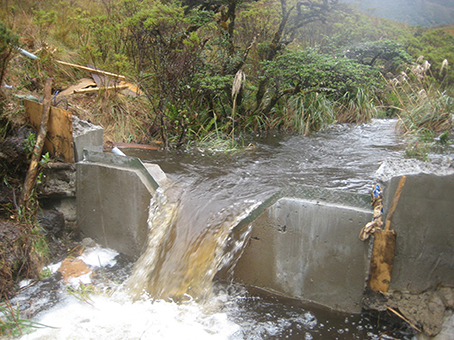
Figure 2. V-notch weir for river flow measurements installed as part of the iMHEA monitoring network in the Peruvian Andes.
The case is a clear example of a bottom-up initiative emerging from a local awareness about the need for better information on ecosystem services, and facilitated by the availability of cheap and robust technology (electronic rain gauges and water level sensors). As such, it has obvious potential to influence negotiation processes and decision-making in the catchment. This contrasts with information that originates in the standard national hydrometeorological network operated by the national hydrometeorological office (SENAMHI), which does not reach the remote upland areas that are key for water supply, but is also not focused on the specificity of the water yield and regulation of specific ecosystems and land use regimes. Additionally, local actors find difficult to access and expensive.
While the long-term sustainability of the initiative is difficult to assess given its short time span, the local buy-in provides it with a stronger legacy compared to traditional, external sources of information. Identified bottlenecks toward increased uptake of the generated information include the need for data processing, quality control, visualization and the development of modeling systems for scenario analysis and prediction.
5.2. Lake Tana Basin, Ethiopia
Livelihood security in the rural area around Lake Tana, Ethiopia is strongly dependent on local ecosystem services generated from the land and the land cover. Rapid population increase has led to rapid land use and land cover change (Moges et al., 2014), and current management of the land providing ecosystem services such as food, feed, and fuel for the current generation is unsustainable. The increase in cultivated land, combined with traditional practices such as plowing land continuously and along the slopes, causes land degradation including hardpan formation and a decreasing soil depth. The degrading resources in turn hamper the ability of the land to provide further essential ecosystem services such as groundwater recharge and carbon fixation. To minimize such degradation, and to maintain the productive capacity of the land, various natural resources management options have been developed by the central government and implemented locally under governmental directive. These practices are usually ineffective in increasing short term and long term benefits, and in some cases these practices accelerated the decrease in poverty alleviating ecosystem services.
A main problem is the disconnect between the governmental or donor recommended practices and what farmers are willing to maintain voluntarily without governmental interventions. For instance, government agencies recommend the installation of 60 cm deep and 30–40 cm wide swales on the contour to intercept excess rainfall and to allow it infiltrate into the subsoil. In contrast, the indigenous soil conservation practice is to plow small 10–15 cm furrows just off the contour to transport the excess rainfall, which ponds temporarily over slowly permeable layer. This minimizes erosion by preventing long rills going down the slope. This dichotomy in practices, and the resulting lack of local buy-in, often results in poorly implemented land-management practices that increase erosion and land degradation (Mitiku et al., 2006) despite the fact that upland and gully erosion in the watersheds are well understood (Tebebu et al., 2010). Another large source of erosion are gullies that form in the periodically saturated lower lands. Rehabilitation of these gullies has not been very successful in the past, mainly because there is little experience and farmers have not been involved.
Nevertheless, a citizen science experiment in the upper Birr catchment has shown promise in addressing this issue that fails to be addressed at a governmental level (Ayele et al., 2014, Figure 3). The study initiated local collaboration, by first discussing with the religious leaders and local respected elders, and later with local village farmers about some ideas for restoring gullies. The religious leaders, local elders and farmers were originally skeptical and hesitant, as they believed these gullies were created by God as a punishment for their wrongdoings. Only after they were shown photos of other rehabilitated gullies and two farmers were brought to visit a rehabilitated gully, that an agreement was reached for them to start a trial on one location. Twenty farmers of the community and surrounding farmers agreed to contribute labor and wood to fence for the protection of the gully and share equally the produced grass at the end of the rainy season. They also set a financial punishment for anyone who allowed their animal into the enclosed gully. Following this agreement, a meeting was organized with the local community, religious leaders, administrators, extension workers, and the village expert to share responsibilities. Nevertheless, the process was not always smooth. Grass grew well in the gully after closure during the rainy season, but then a conflict emerged when the 20 farmers whose land surrounded the gully refused to share the resulting benefits, i.e., the grass produced on the protected area. The conflict was resolved by the elders in the village by having the 20 farmers apologize and sign a promissory note to contribute labor and wood in the next program to rehabilitate on the second village's gully (Figure 3).
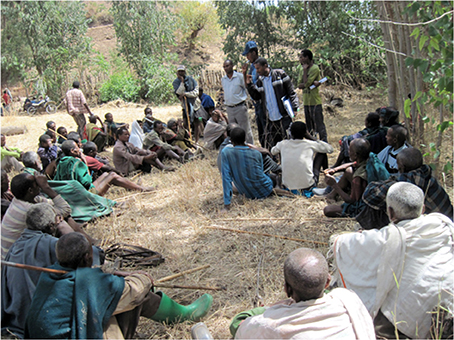
Figure 3. Discussing ecosystem degradation resulting from farmers whose land surrounding the rehabilitated gully being unwilling to share the grass in the Tana Lake Region, Ethiopia.
Clearly the success of this program was due to capitalizing on the informal decision process. Although the formal decision making process is strongly centralized following the decision from regional and federal administrators, an informal decision making process exists in parallel, and is centered around the the elders and clergymen whose authority is very well respected. Therefore, any new knowledge generated within the community, particularly with the key community representatives such as local elders and religious leaders, can be easily received if channeled by/through these figures. One could also argue that the farmers' willingness to participate is the conviction, due to the trust in their elders, that there is something for them to gain.
A key element in this process is the reconciliation of views rather than falsication of a particular view. Gully creation is the result of a combination of human activities and natural causes such as soil instabilities (i.e., external factors). Elders explain the external factors from a religious perspective but both perspectives acknowledge that part of the erosive process is inevitable, yet that impacts should be minimized. To achieve this, the religious leaders preached to the community on Sunday that God sends a messenger (the researcher) to give a solution. As a result, they requested the community to give help to the researcher for rehabilitating gully.
The case illustrates that a collaborative engagement in a citizen science experiment is achievable in the context of an Ethiopian farmers community, and how citizen-science generated results can have on dynamics of ecosystem management and benefit. Tailoring the design to local decision-making processes improved the relevance and legitimacy of the results, and thus their assimilation. At the same time, it shows the potential risks and pitfalls of altering local balances of resource use. Lastly, despite the straightforward experimental design, the use of educational material such as photos helped in setting up the experiment and creating local buy-in.
5.3. Upper Kaligandaki Basin, Nepal
The Upper Kaligandaki basin is located in the Mustang district of the Trans-Himalayan region of Nepal, bordering the Tibet Autonomous Region of China to the northeast. The Mustang district has a population of around 15,600 inhabitants, and a literacy rate of 57%. It is the the driest region of Nepal, with an annual precipitation under 200 mm/year. The local communities are mostly agro-pastoralists, cultivating barley, wheat, potato and buckwheat in isolated areas close to the river, and raising yak, sheep, and goat.
Traditionally, water resources were exclusively used for irrigating croplands (through canals) and managed by cooperative management practices among local communities (Messerschmidt, 1986). More recently, there are competing demands from domestic use, hydropower generation and cultural significance (National Trust for Nature Conservation, 2008). Furthermore, there is an increasing water stress due to changes in snowfall and rainfall. The available water resource became highly contested resource among individuals, communities and social groups (National Trust for Nature Conservation, 2008). To adapt with the changing environment, they have developed sophisticated methods of crop cultivation and water harvesting that allows them to cope and sustain their livelihoods (often with little or limited government influence, Chapagain and Bhusal, 2013).
For example, two adjacent communities, Phalyak and Dhakarjong, used to share a common stream by dividing total flow into parts, using a wooden trunk with equal opening spaces placed in the stream bed (Figure 4). This was in practice for several decades but also created disputes and misunderstandings among communities. According to a senior community member (aged 88 years), the problems were: (i) small debris often blocked or altered the flow at respective openings, (ii) some community members deliberately changed the water flow manually to get more water into their side, and (iii) the wooden structures were usually difficult to fix at the stream bed and continued washed away by occasional flash floods. This pushed local people to find a better sharing mechanism, and the stream is now diverted in days turn—3 days for Phalyak and 2 days for Dhakarjong. This sharing practice seems more sustainable as it is operating smoothly.
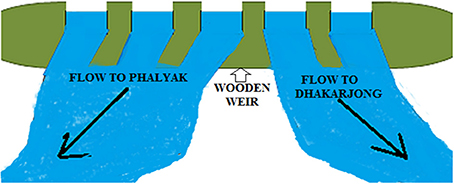
Figure 4. Water distribution system made from wooden trunk used in the Upper Kaligandaki basin, Nepal.
Despite a high level of creativity in their response to change, there remain concerns being voiced by farmers about the direction of future trajectories of change. The first phase of research in Mustang has explored for example aspects of farmer perceived climate change. In this context, farmers have referred to significant changes in local hydrological processes including disappearances of small lakes and water reservoirs, as well as changes in glacier composition. Farmers further recognize the long term implications of changing climate dynamics for their livelihoods and potential risks that these may present for their agricultural based economies. Perhaps because of this we perceive great interest by the farmers themselves to work together with natural and social scientists to develop a better sense of their surrounding and the impact of emerging socio-economic and environmental drivers upon their livelihoods. There are indications that this can only work as a two-way dialogue and knowledge co-generation process between scientists and local communities. Insights into local experiences can furthermore facilitate the formulation of a more contextually specific understanding of change on the basis of a more balanced representation of citizen and expert knowledge.
In recognition of the data scarcity and high uncertainties, there is evidence of policy making acknowledging the fact that new understanding and potential solutions can be achieved through wider public participation. One example is the involvement of volunteers in the collection of hydrological and meteorological data since the establishment of hydrological and meteorological services in Nepal. Although the volunteers receive a monthly wage from the Nepalese Hydrology and Meteorology Office, it is very small (the price of a cup of tea) and do not form a part of income contribution.
Little is known about the precise mechanisms that motivate volunteers to contribute to data collection, though it is clear that complex range of factors are involved. This may include for example a sense of national pride, since these volunteers perceive that they are helping the Nepalese meteorological authorities to collect basic data. Recently, the successful implementation of community-based flood warning system on the West Rapti, Babai, and Karnali Rivers in Nepal has also demonstrated that there is an ample scope of saving lives and properties in an inexpensive manner if the government organizations work with non-government organizations, and communities (Practical Action, 2008/2009). Thus, citizen science approaches (or people's participation) in water resources monitoring/management could become a useful alternative to address many challenging issues for water resource management practices in a high mountainous region of Nepal.
5.4. Naryn Region, Kyrgyz Republic
The Naryn region in Kyrgyzstan covers a remote mountainous watershed in the Central Tien Shan range in Central Asia. While for many centuries this area was inhabited mainly by Kyrgyz herders following traditional resource use patterns, in the Soviet era collectivized state farms were established. As these farms were specialized in livestock production, all arable land was used for the production of fodder crops to feed a variety of livestock, mainly sheep, goats, and cattle. Within the collective farms, specialized employees were given instruction by educated pasture, cropland and water management specialists from academic institutions, and supported with well-equipped infrastructure to monitor climate, river runoff and pasture land conditions. However, despite training and equipment, since the 1980s Soviet scientists reported increasing pasture degradation attributed mainly to overuse of the natural resources (Kerven et al., 2012).
As aftermath of the collapse of the Soviet Union, Kyrgyzstan faced another restructuring process, with consequent major political and societal upheavals. Moving from a planned economy to a market-based economy through the 1990s, privatization and land reforms led to a significant redistribution of assets such as cropland, livestock and state farm equipment. Simultaneously there was a drastic increase in poverty following independence due to economic factors and changes in livelihoods (United Nation Development Programme, 2011/2012). Additionally, the majority of families became very dependent on subsistence farming, with livestock as the primary source of household income. This major socio-economic transition was accompanied by deterioration of the transport and irrigation networks and the environmental monitoring infrastructure, matched also by inadequate collaboration and data-sharing among institutions involved henceforth in more localized (decentralized) decision-making. This trend has continued to the present, however the patterns and drivers of degradation have changed over time. Ongoing climate change is further exacerbating the above challenges and is impacting natural resources, leading to higher frequencies of natural disaster occurrence.
From a perspective of water availability and management, the main challenges in Naryn include limited public investment, high inter-sectoral competition (e.g., hydropower vs. irrigation), scarcity due to shortage and waste, and increasing occurrence of upstream/downstream tensions. Regarding water shortage and related resource management, a dual strategic response is generally adopted: increasing irrigation water availability with construction and rehabilitation of infrastructure, and strengthening and improving water governance institutions. Current development projects in the region mostly address the organization of water administration including the promotion and institutional development of water users associations (WUAs), improvement of water allocation mechanisms among farmers and between village WUAs, and the promotion of technical improvements for more efficient water use.
At present there is little opportunity or local capacity in rural areas of Naryn to monitor water resources. However, a participatory citizen science approach is promising for such a data scarce region – and could be used profitably not only to fill data and information gaps, but also to work collaboratively with pastoral communities to generate relevant management-oriented knowledge. The use of citizen science can help provide a mobilizing and empowering effect within the community if appropriate motivational factors are clearly identified. Through development of long-term partnerships and joint elaboration of key research questions or problems to address, both scientists and community members may value such collaborative work and together develop participatory processes to monitor water flow and collect relevant environmental data, and to collaborate in data processing, analysis, knowledge generation, and transparent monitoring. Local motivation for participation in joint work is likely to include both intrinsic and extrinsic factors, including both personal development and curiosity as well as an anticipation of future economic advantages. Finding an inclusive research approach that reconciles traditional (“pre-Soviet”) and modern knowledge types presumably will be another significant factor for the acceptance and successful application of citizen science in the top-down, “expert”-oriented decision-making legacy that still remains in Kyrgyzstan. Furthermore, the effective application of citizen science for knowledge generation is likely to depend on identifying and working alongside unique innovative individuals who are willing to consider and trial new collaborative management approaches. From a technical perspective, a variety of new mobile tools and instruments, e.g., for canal runoff measurements or precipitation monitoring, are now available at low cost and can easily be used even by inexperienced community partners or students from nearby colleges.
There are, however, some challenges to the uptake of citizen science as a community-endorsed concept. Of particular importance is the persistence of a “Soviet mindset.” While citizen science has a potential to overcome entrenched legacies by empowering communities to engage in the creation and production of “their own” relevant knowledge, this participatory approach to science faces challenges due to many people in transitional post-Soviet contexts still tending to rely on external expertise rather than developing their own capacity. Some beneficiaries of the current social status quo – often former (Soviet era) elites – may also have an interest in preserving their status and privileges. Yet in the democratizing Republic there is generally a sustained growth of local people's involvement in governing processes, even though sometimes there remain (or are increases in) asymmetric power relations that complicate the development of strong civil institutions and consensual decision making. Additionally, as a result of recent changes in national pasture legislation, there is a potential for increased village-level engagement in the development of pasture management plans. Individual herders thus have increasing opportunity to make their own natural resource management decisions, as well as to be represented on village pasture management committees. Thus, in the context of recent socio-political development in the Kyrgyz Republic, despite some continuing legacy of Soviet times, there are clear opportunities for the emergence of citizen partnerships.
As part of a UK-funded Ecosystem Services for Poverty Alleviation (ESPA) project, community meetings have now taken place over several months along with the collection of baseline information (e.g., regional maps, demographic datasets) for the research team to better understand the social-ecological system in Naryn. Through this process, several ecosystem services have been identified including the provision of grassland forage, fodder, livestock products such as milk and wool, water for irrigation, water flow regulation, soil erosion control, biodiversity and carbon sequestration. All these ecosystem services benefit or impact directly on pastoral livelihoods, and nearly all are related to the water cycle. From this perspective, the project team deems it to be a sensible hypothesis that the legitimacy of local knowledge will be increasingly recognized and its uptake into local decision-making will increase during the process of production of new local knowledge about water and related environmental variables with engaged community members. The process of identifying topics of common interest and specific questions deemed to be of local significance is on-going.
6. Conclusions and Challenges
Despite being an intrinsic part of the scientific discovery and knowledge generation process, the concept and potential of citizen science in itself only recently received increasing scientific attention. New technological developments are supporting novel and more efficient methods for data collection and processing, visualization and communication. These opportunities make reflecting upon the challenges and opportunities of citizen science, especially in a context of managing natural resources and leveraging them for human well-being, timely and relevant. This is particularly the case for water resources, which is often one of the most fundamental ecosystem services and a significant bottleneck for sustainable development and poverty alleviation. Our review of technologies reveals a large potential for increasing involvement of citizens in data collection because of the availability of inexpensive, robust and highly automated sensors, and the possibility to combine them with powerful environmental models to create rich and interactive visualization methods. But in order to leverage citizen science for water resources management and poverty alleviation, we identify the following major research and implementation challenges.
• Scientists' perceptions: Although met with enthusiasm in some quarters, citizen science is still seen as a rather vague term in much of the scientific community. If value and validity of citizen science continues to be poorly communicated, such ambiguity has the potential to damage the reputation of researchers, and ultimately, discourage innovation. We suggest that, whilst it is important to remain optimistic about the potential of citizen science, limitations need to be reflected by setting realistic goals that minimize disappointment if projects fail to deliver (Riesch and Potter, 2014).
• Problem definition: Given the multi-level nature of decision-making and conflicting interests of managing scarce natural resources, there is a need to understand better how resource management issues can be defined in a transparent and deliberative process that favors the inclusion of traditionally marginalized and resource-poor actors. Even participatory approaches have a tendency to introduce bias in the participation process, which can only to some extent be used to alleviate (and, if not applied properly, may reinforce) this bias.
• Resource intensity: Despite the potential to distribute the effort among multiple actors, citizen science is human-resource intense, especially in the need for coordination among actors and the need for adequate training and education, and maintaining an acceptable level of participation in the project (Gura, 2013).
• Technology: While evolving quickly, the technology underpinning citizen science requires further scientific effort. Ensuring that technological applications are affordable, user friendly, reliable, compatible, and versatile will be vital for their uptake (Silvertown, 2009; Royem et al., 2012, among others). However, the selection of technology, if effective, would contribute to an empowerment of local communities to take charge and deliver for themselves some solutions to particular needs (Newman et al., 2010).
• Communication and exchange of knowledge: Citizen science results need to be presented in a way that little to no technical knowledge should be required to enable interpretation (Royem et al., 2012). A further consideration is that sharing data and opening up to a larger scientific audience of their local environment may cause some apprehension (Seymour and Regalado, 2014). Furthermore, communication of results needs to be done carefully to avoid making impressions that can potentially become a permanent misunderstanding.
• Legitimacy and polycentric governance: Governance of natural resources in general, and water in particular, is inherently multi-scalar and poly-centric (Ostrom and Cox, 2010). The citizen science concept clearly shows the deficiencies of the technocratic model of knowledge generation. Therefore, it is a useful environment to explore the political—as opposed to a technical-neutral—dimension. This requires a decentering and different articulation of the scientific knowledge process within the broader social process of co-creation of sufficiently shared actionable knowledge that becomes the basis of changed actions within the management of resources.
Author Contributions
Wouter Buytaert, Bert De Bièvre, Art Dewulf, Julian Clark and David M. Hannah led the conception and design of the paper. Zed Zulkafli, Sam Grainger, Timothy Karpouzoglou, Gert Van Hecken, Johan Bastiaensen and Munavar Zhumanova led the literature review. Bert De Bièvre, Luis Acosta, Tammo Steenhuis, Seifu Tilahun, Tilashwork C. Alemie, Bhopal Pandeya, Jagat Bhusal, Keshav Sharma, Deepak Paudel, Munavar Zhumanova, Christian Hergarten, Marc Foggin and Aiganysh Isaeva led the description and analysis of the case studies. All authors contributed to the development of ideas and to the interdisciplinary reflection process. From the 4th author, the names are listed alphabetically and not according to the individual contributions. We also acknowledge input from Geteneh Ayele (Bahir Dar University) and Santosh Regmi (Society of Hydrology and Meteorology Nepal) on the case studies.
Conflict of Interest Statement
The authors declare that the research was conducted in the absence of any commercial or financial relationships that could be construed as a potential conflict of interest.
Acknowledgments
The research consortium to which the authors belong is funded by the UK Natural Environment Research Council project NE-K010239-1 (Mountain-EVO). Wouter Buytaert and Zed Zulkafli also acknowledge funding from the UK Natural Environment Research Council project NE/I004017/1 (VO-ESPA). Wouter Buytaert acknowledges support from the Ecuadorian Government (SENESCYT Prometeo programme).
References
Agrawal, A., and Gibson, C. C. (1999). Enchantment and disenchantment: the role of community in natural resource conservation. World Dev. 27, 629–649. doi: 10.1016/S0305-750X(98)00161-2
Alsdorf, D. E., Rodríguez, E., and Lettenmaier, D. P. (2007). Measuring surface water from space. Rev. Geophys. 45:RG2002. doi: 10.1029/2006RG000197
Andersson, L., Olsson, J. A., Arheimer, B., and Jonsson, A. (2008). Use of Participatory Scenario Modelling as Platforms in Stakeholder Dialogues. Water SA: Special HELP Edition: Special Issue 4, Vol. 34. Available online at: http://www.wrc.org.za/Pages/KH_WaterSA.aspx?dt=5&L0=1&L1=4
Au, J., Bagchi, P., Chen, B., Martinez, R., Dudley, S., and Sorger, G. (2000). Methodology for public monitoring of total coliforms, escherichia coli and toxicity in waterways by Canadian high school students. J. Environ. Manag. 58, 213–230. doi: 10.1006/jema.2000.0323
Ayele, G. K., Gessess, A. A., Addisie, M. B., Tilahun, S. A., Tibebu, T. Y., Tenessa, D. B., et al. (2014). “Biophysical and financial impacts of community-based gully rehabilitation in the Birr watershed, upper Blue Nile basin, Ethiopia,” in Proceedings of the 2nd International Conference on the Advancements of Science and Technology (Bahir Dar: Ethiopia), 194–200.
Bastiaensen, J., De Herdt, T., and D'Exelle, B. (2005). Poverty reduction as a local institutional process. World Dev. 33, 979–993. doi: 10.1016/j.worlddev.2004.09.019
Berkes, F. (1993). “Traditional ecological knowledge in perspective,” in Traditional Ecological Knowledge, Concepts and Cases, ed J. T. Inglis (Ottawa, ON: Canadian Museum of Nature/International Development Research Centre), 1–9.
Berkes, F. (2009). Evolution of co-management: role of knowledge generation, bridging organisations and social learning. J. Environ. Manag. 90, 1692–1702. doi: 10.1016/j.jenvman.2008.12.001
Pubmed Abstract | Pubmed Full Text | CrossRef Full Text | Google Scholar
Beven, K., Buytaert, W., and Smith, L. A. (2012). On virtual observatories and modelled realities (or why discharge must be treated as a virtual variable). Hydrol. Process. 26, 1906–1909. doi: 10.1002/hyp.9261
Birkenholtz, T. (2008). Contesting expertise: the politics of environmental knowledge in northern Indian groundwater practices. Geoforum 39, 466–482. doi: 10.1016/j.geoforum.2007.09.008
Blossom, T. (2012). Fishing for Data: Potential for Citizen Science to Conserve Freshwater Ecosystems. Master's thesis, Imperial College London.
Bonney, R., Ballard, H., Jordan, R., McCallie, E., Phillips, T., Shirk, J., et al. (2009). “Public participation in scientific research: defining the field and assessing its potential for informal science education,” in A CAISE Inquiry Group Report (Washington, DC: Centre for Advancement of Informal Science Education).
Brown, P. (1992). Popular epidemiology and toxic waste contamination: lay and professional ways of knowing. J. Health Soc. Behav. 33, 267–281. doi: 10.2307/2137356
Pubmed Abstract | Pubmed Full Text | CrossRef Full Text | Google Scholar
Brown, P., and Mikkelsen, E. (1990). No Safe Place: Toxic Waste, Leukimia and Community Control. Berkeley, CA: University of California Berkeley Press.
Bulkeley, H., and Mol, A. P. (2003). Participation and environmental governance: consensus, ambivalence and debate. Environ. Values 12, 143–154. doi: 10.3197/096327103129341261
Büscher, B. (2012). Payments for ecosystem services as neoliberal conservation: (reinterpreting) evidence from the Maloti-Drakensberg, South Africa. Conserv. Soc. 10:29. doi: 10.4103/0972-4923.92190
Buytaert, W., Baez, S., Bustamante, M., and Dewulf, A. (2012). Web-Based environmental simulation: bridging the gap between scientific modeling and decision-making. Environ. Sci. Technol. 46, 1971–1976. doi: 10.1021/es2031278
Pubmed Abstract | Pubmed Full Text | CrossRef Full Text | Google Scholar
Buytaert, W., and Beven, K. (2009). Regionalization as a learning process. Water Resour. Res. 45:W11419. doi: 10.1029/2008WR007359
Canfield, D. E., Brown, C. D., Bachmann, R. W., and Hoyer, M. V. (2002). Volunteer lake monitoring: testing the reliability of data collected by the florida lakewatch program. Lake Reserv. Manag. 18, 1–9. doi: 10.1080/07438140209353924
Carr, A. (2004). Why do we all need community science? Soc. Nat. Res. 17, 841–849. doi: 10.1080/08941920490493846
Castelletti, A., and Soncini-Sessa, R. (2007a). Bayesian networks and participatory modelling in water resource management. Environ. Model. Softw. 22, 1075–1088. doi: 10.1016/j.envsoft.2006.06.003
Castelletti, A., and Soncini-Sessa, R. (2007b). Coupling real-time control and socio-economic issues in participatory river basin planning. Environ. Model. Softw. 22, 1114–1128. doi: 10.1016/j.envsoft.2006.05.018
Célleri, R., Buytaert, W., De Bièvre, B., Tobón, C., Crespo, P., Molina, J., et al. (2010). “Understanding the hydrology of Tropical Andean ecosystems through an Andean network of basins,” in Status and Perspectives of Hydrology of Small Basins, Proceedings of the Workshop Held in Goslar-Hahnenklee (Hahnenklee: International Association for Hydrological Sciences), 336.
Chapagain, P. S., and Bhusal, J. K. (2013). Changing water regime and adaptation strategies in Upper Mustang valley of Upper Kaligandaki basin in Nepal. Sci. Cold Arid Reg. 5, 0133–0139. doi: 10.3724/SP.J.1226.2013.00133
Clark, D., Mercado, L., Sitch, S., Jones, C., Gedney, N., Best, M., et al. (2011). The joint uk land environment simulator (jules), model description–part 2: carbon fluxes and vegetation dynamics. Geosci. Model Dev. 4, 701–722. doi: 10.5194/gmd-4-701-2011
Cleaver, F. (2002). Development Through Bricolage: Rethinking Institutions for Natural Resource Management. London: Routledge.
Cohn, J. P. (2008). Citizen science: can volunteers do real research? BioScience 58:192. doi: 10.1641/B580303
Community Collaborative Rain, Hail and Snow Network. (2014). Community Collaborative Rain, Hail and Snow Network. Available online at: http://www.cocorahs.org/ (Accessed July 25 2014).
CONDESAN. (2014). Condesan. Available online at: imhea.condesan.org (Accessed July 25 2014).
Conrad, C. C., and Hilchey, K. G. (2010). A review of citizen science and community-based environmental monitoring: issues and opportunities. Environ. Monitor. Assess. 176, 273–291. doi: 10.1007/s10661-010-1582-5
Cooper, C. B., Dickinson, J., and Phillips, T. (2007). Citizen science as a tool for conservation in residential ecosystems. Ecol. Soc. 12:11. Available online at: http://hdl.handle.net/10535/2968
Cornell, S., Berkhout, F., Tuinstra, W., Tàbara, J. D., Jger, J., Chabay, I., et al. (2013). Opening up knowledge systems for better responses to global environmental change. Environ. Sci. Policy 28, 60–70. doi: 10.1016/j.envsci.2012.11.008
CREATE Lab, Carnergie Mellon University. (2014). Waterbot. Available online at: http://waterbot.org/ (Accessed July 25 2014).
Cundill, G., and Fabricius, C. (2009). Monitoring in adaptive co-management: toward a learning based approach. J. Environ. Manag. 90, 3205–3211. doi: 10.1016/j.jenvman.2009.05.012
Pubmed Abstract | Pubmed Full Text | CrossRef Full Text | Google Scholar
Cundill, G., and Fabricius, C. (2010). Monitoring the governance dimension of natural resource co-management. Ecol. Soc. 15:15. doi: 10.1016/j.jenvman.2009.05.012
da Silva Wells, C., van Lieshout, R., and Uytewaal, E. (2013). Monitoring for learning and developing capacities in the WASH sector. Water Policy 15:206. doi: 10.2166/wp.2013.120
Danielsen, F., Burgess, N. D., and Balmford, A. (2005). Monitoring matters: examining the potential of locally-based approaches. Biodivers. Conserv. 14, 2507–2542. doi: 10.1007/s10531-005-8375-0
Danielsen, F., Burgess, N. D., Balmford, A., Donald, P. F., Funder, M., Jones, J. P. G., et al. (2009). Local participation in natural resource monitoring: a characterization of approaches. Conserv. Biol. 23, 31–42. doi: 10.1111/j.1523-1739.2008.01063.x
Pubmed Abstract | Pubmed Full Text | CrossRef Full Text | Google Scholar
Daw, T., Brown, K., Rosendo, S., and Pomeroy, R. (2011). Applying the ecosystem services concept to poverty alleviation: the need to disaggregate human well-being. Environ. Conserv. 38, 370–379. doi: 10.1017/S0376892911000506
Devictor, V., Whittaker, R. J., and Beltrame, C. (2010). Beyond scarcity: citizen science programmes as useful tools for conservation biogeography. Divers. Distribut. 16, 354–362. doi: 10.1111/j.1472-4642.2009.00615.x
Dickinson, J. L., Shirk, J., Bonter, D., Bonney, R., Crain, R. L., Martin, J., et al. (2012). The current state of citizen science as a tool for ecological research and public engagement. Front. Ecol. Environ. 10, 291–297. doi: 10.1890/110236
Dunn, C. E., Crowley, P., Bush, J., Pless-Mulloli, T., and McKinney, P. A. (2008). Expertise and scientific uncertainty: understanding trust amongst professional stakeholders in environment and health. Environ. Plan. A 40:696. doi: 10.1068/a3993
Faysse, N., Rinaudo, J.-D., Bento, S., Richard-Ferroudji, A., Errahj, M., Varanda, M., et al. (2012). Participatory analysis for adaptation to climate change in Mediterranean agricultural systems: possible choices in process design. Reg. Environ. Change 14, 57–70. doi: 10.1007/s10113-012-0362-x
Fazey, I., Fazey, J. A., Salisbury, J. G., Lindenmayer, D. B., and Dovers, S. (2006). The nature and role of experiential knowledge for environmental conservation. Environ. Conserv. 33, 1–10. doi: 10.1017/S037689290600275X
Fernandez-Gimenez, M. E., and Ballard, H. L. (2008). Adaptive management and social learning in collaborative and community-based monitoring: a study of five community-based forestry organizations in the western USA. Ecol. Soc. 13:4. Available online at: http://hdl.handle.net/10535/3213
Fischer, F. (1993). Citizen participation and the democratization of policy expertise: from theoretical inquiry to practical cases. Policy Sci. 26, 165–187. doi: 10.1007/BF00999715
Freudenburg, W. (2003). “Institutional failure and the organizational amplification of risks,” in The Social Amplification of Risk, eds N.F. Pdgeon, R. Kasperson, and P. Slovic (Cambridge, UK: Cambridge University Press), 102–122.
Frey, B. (1997). Not Just for the Money: An Economic Theory of Personal Motivation. Cheltenham: Edward Elgar.
Fry, B. P. (2011). Community forest monitoring in REDD+: the ‘M’ in MRV? Environ. Sci. Policy 14, 181–187. doi: 10.1016/j.envsci.2010.12.004
Gaventa, J., and Barrett, G. (2012). Mapping the outcomes of citizen engagement. World Dev. 40, 399–2410. doi: 10.1016/j.worlddev.2012.05.014
Pubmed Abstract | Pubmed Full Text | CrossRef Full Text | Google Scholar
Gunkel, A. (2005). The Application of Multi-Agent Systems for Water Resources Research–Possibilities and Limits. Ph.D. thesis, Institut für Hydrologie der Albert-Ludwigs-Universität Freiburg, Freiburg.
Gura, T. (2013). Citizen science: amateur experts. Nature 496, 259–261. doi: 10.1038/nj7444-259a
Pubmed Abstract | Pubmed Full Text | CrossRef Full Text | Google Scholar
Haas, P. M. (1989). Do regimes matter? Epistemic communities and Mediterranean pollution control. Int. Org. 43, 377–403. doi: 10.1017/S0020818300032975
Halkay, M. (2011). “Extreme citizen science and the internet of things - participatory sensing and sense making,” in ESF Exploratory Workshop (Göteborg), 1–36.
Hall, K., Cleaver, F., Franks, T., and Maganga, F. (2014). Capturing critical institutionalism: a synthesis of key themes and debates. Eur. J. Dev. Res. 26, 71–86. doi: 10.1057/ejdr.2013.48
Hannah, D. M., Demuth, S., van Lanen, H. A. J., Looser, U., Prudhomme, C., Rees, G., et al. (2011). Large-scale river flow archives: importance, current status and future needs. Hydrol. Process. 25, 1191–1200. doi: 10.1002/hyp.7794
Hare, M. (2011). Forms of participatory modelling and its potential for widespread adoption in the water sector. Environ. Policy Gov. 21, 386–402. doi: 10.1002/eet.590
Harrison, J. R., Lin, Z., Carroll, G. R., and Carley, K. M. (2007). Simulation modeling in organizational and management research. Acad. Manag. Rev. 32, 1229–1245. doi: 10.5465/AMR.2007.26586485
Hart, J. K., and Martinez, K. (2006). Environmental Sensor Networks: a revolution in the earth system science? Earth Sci. Rev. 78, 177–191. doi: 10.1016/j.earscirev.2006.05.001
Henriksen, H. J., Rasmussen, P., Brandt, G., Von Buelow, D., and Jensen, F. V. (2007). Public participation modelling using bayesian networks in management of groundwater contamination. Environ. Model. Soft. 22, 1101–1113. doi: 10.1016/j.envsoft.2006.01.008
Henriksen, H. J., Zorrilla-Miras, P., de la Hera, A., and Brugnach, M. (2012). Use of Bayesian belief networks for dealing with ambiguity in integrated groundwater management. Int. Environ. Assess. Manag. 8, 430–444. doi: 10.1002/ieam.195
Pubmed Abstract | Pubmed Full Text | CrossRef Full Text | Google Scholar
Hipsey, M., and Arheimer, B. (2014). “Challenges for water-quality research in the new iahs decade,” in Understanding Freshwater Quality Problems in A Changing World. IAHS Red Book Published 361 (Wallingford, CT: IAHS Press), 17–29.
International Telecommunication Union. (2014). “The world in 2014: ICT facts and figures,” in Technical Report (Geneva: International Telecommunication Union).
IPCC. (2014). Climate Change 2014: Impacts, Adaptation, and Vulnerability. Part B: Regional Aspects. Contribution of Working Group II to the Fifth Assessment Report of the Intergovernmental Panel on Climate Change. Cambridge; New York: Cambridge University Press.
Irwin, A. (1995). Citizen Science: A Study of People, Expertise and Sustainable Development. Abingdon: Psychology Press.
Jodha, N. S. (2005). Changing ecosystem-social system links and future of drylands in India. Ann. Arid Zone 44, 387–402. Available online at: http://lib.icimod.org/record/16859?ln=en
Karpouzoglou, T., and Zimmer, A. (2011). “Our power rests with numbers; re-visiting the divide between expert and lay knowledge in contemporary urban wastewater governance: New Delhi, India,” in Liquid Dynamics II: STEPS Centre Water and Sanitation Symposium (Brighton: Institute of Development Studies).
Keeley, J., and Scoones, I. (2000). Knowledge, power and politics: the environmental policy-making process in Ethiopia. J. Modern Afr. Stud. 38, 89–120. doi: 10.1017/S0022278X99003262
Keough, H. L., and Blahna, D. J. (2006). Achieving integrative, collaborative ecosystem management. Conserv. Biol. 20, 1373–1382. doi: 10.1111/j.1523-1739.2006.00445.x
Pubmed Abstract | Pubmed Full Text | CrossRef Full Text | Google Scholar
Kerven, C., Steimann, B., Dear, C., and Ashley, L. (2012). Researching the future of pastoralism in Central Asia's mountains: examining development orthodoxies. Mount. Res. Dev. 32, 368–377. doi: 10.1659/MRD-JOURNAL-D-12-00035.1
Kruger, L. E., and Shannon, M. A. (2000). Getting to know ourselves and our places through participation in civic social assessment. Soc. Nat. Resour. 13, 461–478. doi: 10.1080/089419200403866
Lakshminarayanan, S. (2007). Using citizens to do science versus citizens as scientists. Ecol. Soc. 12:r2. Available online at: http://www.ecologyandsociety.org/vol12/iss2/resp2/
Le Bars, M., and Grusse, P. L. (2008). Use of a decision support system and a simulation game to help collective decision-making in water management. Comput. Electron. Agricult. 62, 182–189. doi: 10.1016/j.compag.2008.01.003
Lebel, L., Grothmann, T., and Siebenhuner, B. (2010). The role of social learning in adaptiveness: insights from water management. Int. Environ. Agreem. 10, 33–53. doi: 10.1007/s10784-010-9142-6
Löffler-Mang, M., and Joss, J. (2000). An optical disdrometer for measuring size and velocity of hydrometeors. J. Atmospher. Ocean. Technol. 17, 130–139. doi: 10.1175/1520-0426(2000)017<0130:AODFMS>2.0.CO;2
Lowry, C. S., and Fienen, M. N. (2013). CrowdHydrology: crowdsourcing hydrologic data and engaging citizen scientists. Ground Water 51, 151–156. doi: 10.1111/j.1745-6584.2012.00956.x
Pubmed Abstract | Pubmed Full Text | CrossRef Full Text | Google Scholar
Macknick, J. E., and Enders, S. K. (2012). Transboundary forestry and water management in Nicaragua and Honduras: from conflicts to opportunities for cooperation. J. Sustain. Forest. 31, 376–395. doi: 10.1080/10549811.2011.588473
Martinez-Alier, J. (2014). The environmentalism of the poor. Geoforum 54, 239–241. doi: 10.1016/j.geoforum.2013.04.019
Matrosov, E. S., Woods, A. M., and Harou, J. J. (2013). Robust decision making and info-gap decision theory for water resource system planning. J. Hydrol. 494, 43–58. doi: 10.1016/j.jhydrol.2013.03.006
McIntosh, B., Ascough II, J., Twery, M., Chew, J., Elmahdi, A., Haase, D., et al. (2011). Environmental decision support systems (edss) development–challenges and best practices. Environ. Model. Soft. 26, 1389–1402. doi: 10.1016/j.envsoft.2011.09.009
Messerschmidt, D. (1986). “People and resources in nepal: customary resource management systems of the upper Kali Gandaki,” in Proceedings of the Conference on Common Property Resource Management (Washington, DC: National Academy Press), 455–480.
Milly, P. C. D., Betancourt, J., Falkenmark, M., Hirsch, R. M., Kundzewicz, Z. W., Lettenmaier, D. P., et al. (2008). Stationarity is dead: whither water management? Science 317, 573–574. doi: 10.1126/science.1151915
Minnesota Pollution Control Agency. (2014). Citizen Water Monitoring. Available online at: http://www.pca.state.mn.us/index.php/water/water-monitoring-and-reporting/volunteer-water-monitoring/volunteer-surface-water-monitoring.html (Accessed July 25 2014).
Mitiku, H., Herweg, K., and Stillhardt, B. (2006). Sustainable Land Management – a New Approach to Soil and Water Conservation in Ethiopia. Technical report, Bern, Switzerland: Land Resource Management and Environmental Protection Department, Mekelle University, Mekelle, Ethiopia, Center for Development and Environment (CDE), University of Bern and Swiss National Center of Competence in Research (NCCR) North-South.
Moges, M. A., Tilahun, S. A., Ayana, E. K., Moges, M. M., and Steenhuis, T. S. (2014). “Assessing non-point source pollution in an agricultural watershed: the Awramba watershed in the Lake Tana basin,” in Proceedings of the 2nd International Conference on the Advancements of Science and Technology, Bahir Dar, Ethiopia, 6-17 May, 2014 (Bahir Dar), 182–188.
Mollinga, P. P. (2009). Towards the transdisciplinary engineer: Incorporating ecology, equity and democracy concerns into water professionals' attitudes, skills and knowledge. Irrigat. Drain. 58, S195–S204. doi: 10.1002/ird.510
National Trust for Nature Conservation. (2008). Sustainable Development Plan of Mustang. Lalitpur: Technical report, NTNC/GoN/UNEP.
New Jersey Department of Environmental Protection. (2014). Volunteer Monitoring. Available online at: http://www.state.nj.us/dep/wms/bwqsa/vm/
New York City Water Trail Association. (2014). 2014 Citizens' Water Quality Testing Program. Available online at: http://www.nycwatertrail.org/waterquality.html
Newman, G., Wiggins, A., Crall, A., Graham, E., Newman, S., and Crowston, K. (2012). The future of citizen science: emerging technologies and shifting paradigms. Front. Ecol. Environ, 10, 298–304. doi: 10.1890/110294
Newman, G., Zimmerman, D., Crall, A., Laituri, M., Graham, J., and Stapel, L. (2010). User-friendly web mapping: lessons from a citizen science website. Int. J. Geograph. Inf. Sci. 24, 1851–1869. doi: 10.1080/13658816.2010.490532
Nicholson, E., Ryan, J., and Hodgkin, D. (2002). Community data–where does the value lie? assessing confidence limits of community collected water quality data. Water Sci. Technol. 45, 193–200. Available online at: http://www.ncbi.nlm.nih.gov/pubmed/12171352
O'Connor, A., Bishop, I., and Stock, C. (2005). “3d visualisation of spatial information and environmental process model outputs for collaborative data exploration,” in Proceedings of the Ninth International Conference on Information Visualisation (London, UK: IEEE), 758–763.
Olsson, J. A., and Andersson, L. (2007). “Possibilities and problems with the use of models as a communication tool in water resource management,” Integrated Assessment of Water Resources and Global Change: A North-South Analysis, eds E. Craswell, M. Bonnell, D. Bossio, S. Demuth, and N. van de Giesen (Bonn: Springer), 97–110.
Open Air Laboratories (OPAL). (2014). Opal. Available online at: http://www.opalexplorenature.org/
Ostrom, E. (1990). Governing the Commons: The Evolution of Institutions for Collective Action. Cambridge, UK: Cambridge University Press. doi: 10.1017/CBO9780511807763
Ostrom, E., and Cox, M. (2010). Moving beyond panaceas: a multi-tiered diagnostic approach for social-ecological analysis. Environ. Conservat. 37, 451–463. doi: 10.1017/S0376892910000834
Overdevest, C., and Orr, C. H. (2004). Volunteer stream monitoring and local participation in natural resource issues. Hum. Ecol. Rev. 11, 177–185. Available online at: http://www.humanecologyreview.org/pastissues/her112/overdevestorrstepenuck.pdf
Overeem, A., Leijnse, H., and Uijlenhoet, R. (2013). Country-wide rainfall maps from cellular communication networks. Proc. Natl. Acad. Sci. U.S.A. 110, 2741–2745. doi: 10.1073/pnas.1217961110
Pubmed Abstract | Pubmed Full Text | CrossRef Full Text | Google Scholar
Pahl-Wostl, C., Craps, M., Dewulf, A., Mostert, E., Tabara, D., and Taillieu, T. (2007). Social learning and water resources management. Ecol. Soc. 12:5. Available online at: http://www.ecologyandsociety.org/vol12/iss2/art5/
Parajka, J., Merz, R., and Blöschl, G. (2005). A comparison of regionalisation methods for catchment model parameters. Hydrol. Earth Syst. Sci. 9, 157–171. doi: 10.5194/hess-9-157-2005
Parajka, J., Merz, R., and Blöschl, G. (2007). Uncertainty and multiple objective calibration in regional water balance modelling: case study in 320 austrian catchments. Hydrol. Process. 21, 435–446. doi: 10.1002/hyp.6253
Paul, K., Quinn, M. S., Huijser, M. P., Graham, J., and Broberg, L. (2014). An evaluation of a citizen science data collection program for recording wildlife observations along a highway. J. Environ. Manage. 139, 180–187. doi: 10.1016/j.jenvman.2014.02.018
Pubmed Abstract | Pubmed Full Text | CrossRef Full Text | Google Scholar
Practical Action. (2008/2009). Annual Report. Kathmandu: Technical report, Practical Action Nepal Office.
Pretty, J. N. (1995). Participatory learning for sustainable agriculture. World Dev. 23, 1247–1263. doi: 10.1016/0305-750X(95)00046-F
Ridder, D., and Pahl-Wostl, C. (2005). Participatory Integrated Assessment in local level planning. Region. Environ. Change 5, 188–196. doi: 10.1007/s10113-004-0089-4
Riesch, H., and Potter, C. (2014). Citizen science as seen by scientists: methodological, epistemological and ethical dimensions. Public Underst. Sci. 23, 107–120. doi: 10.1177/0963662513497324
Pubmed Abstract | Pubmed Full Text | CrossRef Full Text | Google Scholar
Robertson, H. A., and McGee, T. K. (2003). Applying local knowledge: the contribution of oral history to wetland rehabilitation at kanyapella basin, Australia. J. Environ. Manag. 69, 275–287. doi: 10.1016/S0301-4797(03)00155-5
Pubmed Abstract | Pubmed Full Text | CrossRef Full Text | Google Scholar
Rotman, D., Hammock, J., Preece, J., Hansen, D., Boston, C., Bowser, A., et al. (2014a). “Motivations affecting initial and long-term participation in citizen science projects in three countries,” in iConference 2014 Proceedings, 110–124.
Rotman, D., Hammock, J., Preece, J. J., Boston, C. L., Hansen, D. L., Bowser, A., et al. (2014b). “Does motivation in citizen science change with time and culture?” in Proceedings of the Companion Publication of the 17th ACM Conference on Computer Supported Cooperative Work & Social Computing, CSCW Companion '14 (New York, NY: ACM), 229–232.
Rotman, D., Preece, J., Hammock, J., Procita, K., Hansen, D., Parr, C., et al. (2012). “Dynamic changes in motivation in collaborative citizen-science projects,” in Proceedings of the ACM 2012 Conference on Computer Supported Cooperative Work, CSCW '12 (New York, NY: ACM), 217–226.
Royem, A. A., Mui, C. K., Fuka, D. R., and Walter, M. T. (2012). Technical note: proposing a low-tech, affordable, accurate stream stage monitoring system. Trans. ASABE 55, 237–2242. doi: 10.13031/2013.42512
Sampson, C. C., Fewtrell, T. J., Duncan, A., Shaad, K., Horritt, M. S., and Bates, P. D. (2012). Use of terrestrial laser scanning data to drive decimetric resolution urban inundation models. Adv. Water Res. 41, 1–17. doi: 10.1016/j.advwatres.2012.02.010
Schweizer, T. (1998). “Epistemology: the nature and validation of anthropological knowledge,” in Research Methods in Anthropology: Qualitative and Quantitative Approaches, ed H. Bernard (Altamira: Rowman), 39–87.
Seibert, J., and Beven, K. J. (2009). Gauging the ungauged basin: how many discharge measurements are needed? Hydrol. Earth Syst. Sci. 13, 883–892. doi: 10.5194/hess-13-883-2009
Seymour, V., and Regalado, C. (2014). “Extreme citizen science (excites): one end of the citizen science spectrum,” in British Hydrological Society South East Section Meeting (London, UK).
Sheffield, J., Goteti, G., and Wood, E. F. (2006). Development of a 50-year high-resolution global dataset of meteorological forcings for land surface modeling. J. Clim. 19, 3088–3111. doi: 10.1175/JCLI3790.1
Shelton, A. (2013). The Accuracy of Water Quality Monitoring Data: a Comparison Between Citizen Scientists and Professionals. Master's thesis, Saint Mary's University, Halifax, Nova Scotia.
Shirk, J. L., Ballard, H. L., Wilderman, C. C., Phillips, T., Wiggins, A., Jordan, R., et al. (2012). Public participation in scientific research: a framework for deliberate design. Ecol. Soc. 17:29. doi: 10.5751/ES-04705-170229
Silvertown, J. (2009). A new dawn for citizen science. Trends Ecol. Evol. 24, 467–471. doi: 10.1016/j.tree.2009.03.017
Pubmed Abstract | Pubmed Full Text | CrossRef Full Text | Google Scholar
Singh, R., Wagener, T., van Werkhoven, K., Mann, M. E., and Crane, R. (2011). A trading-space-for-time approach to probabilistic continuous streamflow predictions in a changing climate – accounting for changing watershed behavior. Hydrol. Earth Syst. Sci. 15, 3591–3603. doi: 10.5194/hess-15-3591-2011
Smith, L. C., and Pavelsky, T. M. (2008). Estimation of river discharge, propagation speed, and hydraulic geometry from space: Lena River, Siberia. Water Res. Res. 44:W03427. doi: 10.1029/2007WR006133
Spiegelhalter, D., Pearson, M., and Short, I. (2011). Visualizing uncertainty about the future. Science 333, 1393–1400. doi: 10.1126/science.1191181
Pubmed Abstract | Pubmed Full Text | CrossRef Full Text | Google Scholar
Srinivasan, V., Thomas, B. K., Jamwal, P., and Lele, S. (2013). Climate vulnerability and adaptation of water provisioning in developing countries: approaches to disciplinary and research-practice integration. Curr. Opin. Environ. Sustainabil. 5, 378–383. doi: 10.1016/j.cosust.2013.07.011
Stevens, M., Vitos, M., Lewis, J., and Haklay, M. (2013). “Participatory monitoring of poaching in the Congo basin,” in 21st GIS Research UK Conference (Liverpool, UK).
Stirling, A., Leach, M., Mehta, L., Scoones, I., Smith, A., Stagl, S., et al. (2007). “Empowering designs: towards more progressive appraisal of sustainability,” in STEPS Working Paper 3 (Brighton: STEPS Centre).
Stock, C., and Bishop, I. (2005). “Helping rural communities envision their future,” in Visualization for Landscape and Environmental Planning, eds I. Bishop and E. Lange (Oxford: Taylor & Francis), 145–151.
Tebebu, T. Y., Abiy, A. Z., Zegeye, A. D., Dahlke, H. E., Easton, Z. M., Tilahun, S. A., et al. (2010). Surface and subsurface flow effect on permanent gully formation and upland erosion near lake Tana in the northern highlands of Ethiopia. Hydrol. Earth Syst. Sci. 14, 2207–2217. doi: 10.5194/hess-14-2207-2010
Tengö, M., Brondizio, E. S., Elmqvist, T., Malmer, P., and Spierenburg, M. (2014). Connecting diverse knowledge systems for enhanced ecosystem governance: the multiple evidence base approach. AMBIO, 43, 579–591. doi: 10.1007/s13280-014-0501-3
Pubmed Abstract | Pubmed Full Text | CrossRef Full Text | Google Scholar
Turner, D., and Richter, H. (2011). Wet/dry mapping: using citizen scientists to monitor the extent of perennial surface flow in dryland regions. Environ. Manag. 47, 497–505. doi: 10.1007/s00267-010-9607-y
Pubmed Abstract | Pubmed Full Text | CrossRef Full Text | Google Scholar
United Nation Development Programme. (2011/2012). Annual Report. New York, NY: Technical report, United Nation Development Programme.
US Environment Protection Agency. (2014). Monitoring and Assessing water Quality - Volunteer Monitoring. Available online at: http://water.epa.gov/type/rsl/monitoring/
Uusitalo, L. (2007). Advantages and challenges of Bayesian networks in environmental modelling. Ecol. Model. 203, 312–318. doi: 10.1016/j.ecolmodel.2006.11.033
Vaché, K. B., McDonnell, J. J., and Bolte, J. (2004). On the use of multiple criteria for a posteriori model rejection: Soft data to characterize model performance. Geophys. Res. Lett. 31:L21504. doi: 10.1029/2004GL021577
Vandewiele, G., and Elias, A. (1995). Monthly water balance of ungauged catchments obtained by geographical regionalization. J. Hydrol. 170, 277–291. doi: 10.1016/0022-1694(95)02681-E
Vitolo, C., Elkhatib, Y., Reusser, D., Macleod, C. J. A., and Buytaert, W. (in press). Web technologies for environmental big data. Environ. Model. Softw. doi: 10.1016/j.envsoft.2014.10.007
Volunteer Water Quality Monitoring. (2014). Volunteer Water Quality Monitoring and Master Naturalist Programs in the US. Available online at: http://www.usawaterquality.org/volunteer/VolunteerMonPrograms/index.html
Water Reporter. (2014). Water Reporter. Available online at: http://waterreporter.org/
Wechsler, D. (2014). Crowdsourcing as a method of transdisciplinary research - tapping the full potential of participants. Futures 60, 14–22. doi: 10.1016/j.futures.2014.02.005
Whitelaw, G., Vaughan, H., Craig, B., and Atkinson, D. (2003). Establishing the Canadian community monitoring network. Environ. Monitor. Assess. 88, 409–418. doi: 10.1023/A:1025545813057
Pubmed Abstract | Pubmed Full Text | CrossRef Full Text | Google Scholar
Wiggins, A., and Crowston, K. (2011). “From conservation to crowdsourcing: a typology of citizen science,” in 2011 44th Hawaii International Conference on System Sciences (HICSS 2011) (Koloa, HI).
Williams, M., Cornford, D., Bastin, L., Jones, R., and Parker, S. (2011). Automatic processing, quality assurance and serving of real-time weather data. Comput. Geosci. 37, 353–362. doi: 10.1016/j.cageo.2010.05.010
Woolley, A. W., Chabris, C. F., Pentland, A., Hashmi, N., and Malone, T. W. (2010). Evidence for a collective intelligence factor in the performance of human groups. Science 330, 686–688. doi: 10.1126/science.1193147
Pubmed Abstract | Pubmed Full Text | CrossRef Full Text | Google Scholar
World Meteorological Organization. (1994). Guide to hydrological practices, data acquisition and processing, analysis, forecasting and other applications. WMO-No. 168, 5th Edn. Geneva: Technical report, World Meteorological Organisation.
World Water Monitoring Challenge. (2014). World water monitoring challenge. Availble online at: http://www.monitorwater.org/
Keywords: citizen science, hydrological sensing, co-generation of knowledge, water resources management, poly-centric governance
Citation: Buytaert W, Zulkafli Z, Grainger S, Acosta L, Alemie TC, Bastiaensen J, De Bièvre B, Bhusal J, Clark J, Dewulf A, Foggin M, Hannah DM, Hergarten C, Isaeva A, Karpouzoglou T, Pandeya B, Paudel D, Sharma K, Steenhuis T, Tilahun S, Van Hecken G and Zhumanova M (2014) Citizen science in hydrology and water resources: opportunities for knowledge generation, ecosystem service management, and sustainable development. Front. Earth Sci. 2:26. doi: 10.3389/feart.2014.00026
Received: 04 June 2014; Accepted: 25 September 2014;
Published online: 22 October 2014.
Edited by:
Rolf Hut, Delft University of Technology, NetherlandsReviewed by:
Guy Jean-Pierre Schumann, University of California Los Angeles, USALuciano Raso, Institut National de Recherche en Sciences et Technologies pour l'Environnement et l'Agriculture (IRSTEA), France
Maurits Ertsen, Delft University, Netherlands
Copyright © 2014 Buytaert, Zulkafli, Grainger, Acosta, Alemie, Bastiaensen, De Bièvre, Bhusal, Clark, Dewulf, Foggin, Hannah, Hergarten, Isaeva, Karpouzoglou, Pandeya, Paudel, Sharma, Steenhuis, Tilahun, Van Hecken and Zhumanova. This is an open-access article distributed under the terms of the Creative Commons Attribution License (CC BY). The use, distribution or reproduction in other forums is permitted, provided the original author(s) or licensor are credited and that the original publication in this journal is cited, in accordance with accepted academic practice. No use, distribution or reproduction is permitted which does not comply with these terms.
*Correspondence: Wouter Buytaert, Department of Civil and Environmental Engineering, Imperial College London, Skempton Building, South Kensington Campus, London SW7 2AZ, UK e-mail: w.buytaert@imperial.ac.uk