- 1Environment Department, York Institute for Tropical Ecosystems, University of York, York, United Kingdom
- 2Department of Archaeology and Ancient History, Uppsala University, Uppsala, Sweden
- 3Department of History, University of Dar es Salaam, Dar es Salaam, Tanzania
- 4Department of Archaeology, University of York, York, United Kingdom
- 5School of Geography, Archaeology and Environmental Studies, University of the Witwatersrand, Johannesburg, South Africa
This study presents a multidisciplinary perspective for understanding environmental change and emerging socio-ecological interactions across the Amboseli region of southwestern Kenya. We focus on late Holocene (<5,000 cal yr. BP) changes and continuities reconstructed from sedimentary, archeological, historical records and socio-ecological models. We utilize multi-disciplinary approaches to understand environmental-ecosystem-social interactions over the longue durée and use this to simulate different land use scenarios supporting conservation and sustainable livelihoods using a socio-ecological model. Today the semi-arid Amboseli landscape supports a large livestock and wildlife population, sustained by a wide variety of plants and extensive rangelands regulated by seasonal rainfall and human activity. Our data provide insight into how large-scale and long-term interactions of climate, people, livestock, wildlife and external connections have shaped the ecosystems across the Amboseli landscape. Environmental conditions were dry between ~5,000 and 2,000 cal yr. BP, followed by two wet periods at ~2,100–1,500 and 1,400–800 cal yr. BP with short dry periods; the most recent centuries were characterized by variable climate with alternative dry and wet phases with high spatial heterogeneity. Most evident in paleo and historical records is the changing woody to grass cover ratio, driven by changes in climate and fire regimes entwined with fluctuating elephant, cattle and wild ungulate populations moderated by human activity, including elephant ivory trade intensification. Archeological perspectives on the occupation of different groups (hunter-gatherers, pastoralists, and farmers) in Amboseli region and the relationships between them are discussed. An overview of the known history of humans and elephants, expanding networks of trade, and the arrival and integration of metallurgy, livestock and domesticated crops in the wider region is provided. In recent decades, increased runoff and flooding have resulted in the expansion of wetlands and a reduction of woody vegetation, compounding problems created by increased enclosure and privatization of these landscapes. However, most of the wetlands outside of the protected area are drying up because of the intensified water extraction by the communities surrounding the National Park and on the adjacent mountains areas, who have increased in numbers, become sedentary and diversified land use around the wetlands.
Introduction
Palynological expeditions have been undertaken in East Africa for over 80 years with the first publication of results, by Dubois and Dubois, appearing in 1939 (Van Zinderen and Coetzee, 1988) followed in 1948 by Hedberg and his “Swedish East Africa Expedition” which collected samples from Lake Victoria, Lake Tanganyika, Mt. Kenya and the Ruwenzori Mountains (Fries and Fries, 1948). Early palynological work in eastern Africa described Pleistocene to Holocene-aged lacustrine and peat sediment sequences, largely collected from mesic and wet regions in montane western Uganda (Osmaston, 1958, 1965; Livingstone, 1962, 1967; Morrison, 1968) and the Kenyan highlands (Cherangani Hills, Van Zinderen, 1962; Mount Kenya, Coetzee, 1964, 1967). These early studies focused on Afromontane taxa timing and response to climatic variability during the Late Pleistocene glacial retreat and subsequent broad-scale at the Late Pleistocene-Early Holocene transition (Livingstone, 1967; Morrison, 1968). Further research used pollen data to chart anthropogenic deforestation of Afromontane forests to explain vegetation changes (Morrison and Hamilton, 1974; Hamilton, 1982; Hamilton et al., 1986; Perrott, 1987). Pollen results from longer sequences found that these forests had previously expanded, as Afromontane glaciers coverage increased leading into the global Last Glacial Maximum (Bonnefille and Riollet, 1988). Montane vegetation patterns were described as altitudinal bands (Mackinder, 1900; Fries and Fries, 1948; Hedberg, 1951), but further examination of pollen records and forest biogeographies showed a high degree of complexity in montane forests from the lower montane to forest-ericaceous zone transitions and non-linearity in vegetation responses to climatic variability (Bussmann and Beck, 1995a,b; Hemp, 2006a,b; Platts et al., 2013). A review by Van Zinderen and Coetzee (1988) broadly summarizes the last 32,000 cal yr. BP of East Africa as warm and humid (32,000–20,000 cal yr. BP), cool and dry from 20,000 to 14,000 cal yr. BP, warm and dry until 12,000 cal yr. BP, warm and humid from 12,000 cal yr. BP to 4,000 cal yr. BP and then regionally arid from 4,000 cal yr. BP.
Examinations of climate and anthropogenic modifications to montane forests in East Africa remains an important topic to which modern ecology, geoarcheology, and pollen studies continue to contribute (Finch and Marchant, 2011; Heckmann, 2014; Heckmann et al., 2014; Finch et al., 2016). The increase in the number of paleoerecords and proxies in recent years, as well as wide-ranging reference collections, has led to interpretations that are more complex as well as a better understanding of the drivers of the changes observed in the records. Notable topics of interest have been the influences of increasing atmospheric CO2 concentrations on vegetation changes over the latter millennia of the Late Pleistocene and into the Holocene (Jolly and Haxeltine, 1997), and the effects of disturbances like fire (Bussmann, 2001; Hemp and Beck, 2001; Rucina et al., 2010; Finch et al., 2016). Further palynological investigations have focused on long-term deposits in lakes that provide Late Quaternary vegetation histories (DeBusk, 1998; Nelson et al., 2012) and on small lakes and wetland sites to reveal high-resolution environmental histories from the late Holocene to present (Muiruri, 2008; Rucina et al., 2010; Colombaroli et al., in press). Although few in number, there is a growing number of co-located study sites linking high-resolution paleoenvironmental data with archeological sites to build concise descriptions of human-environmental interactions during the late Holocene (Robertshaw, 1997; Lejju et al., 2003, 2005; Taylor et al., 2005; Iles et al., 2014).
Building on these previous studies, this paper summarizes the integrated results of several studies of the Amboseli area of southern Kenya, equatorial eastern Africa (Figure 1). By combining newly collated and analyzed palynological, archeological and historical data sets with longitudinal socio-ecological data generated by previous research we provide fresh insights into the origins and drivers of spatial heterogeneity in this semi-arid savannah ecosystem and its temporal variability over the last several thousand years. In particular, we first review (I) pollen-based reconstructions of Holocene vegetation and environmental change in Amboseli, and (II) archeological and historical evidence for changes and continuities in livelihood strategies and land use management practices within Amboseli, and between Mt Kilimanjaro and Amboseli communities, before going on to (III) combine insights drawn from different data sources to model impacts of land use choices in Amboseli with data from the last 50 years. Given the rapidity of land cover change in the area and current challenges surrounding human-ecosystem-climate interactions in Amboseli, a particular concern is to demonstrate how knowledge of the historical ecology of this landscape can contribute to sustainable land use management and conservation, which forms the focus of our concluding discussion.
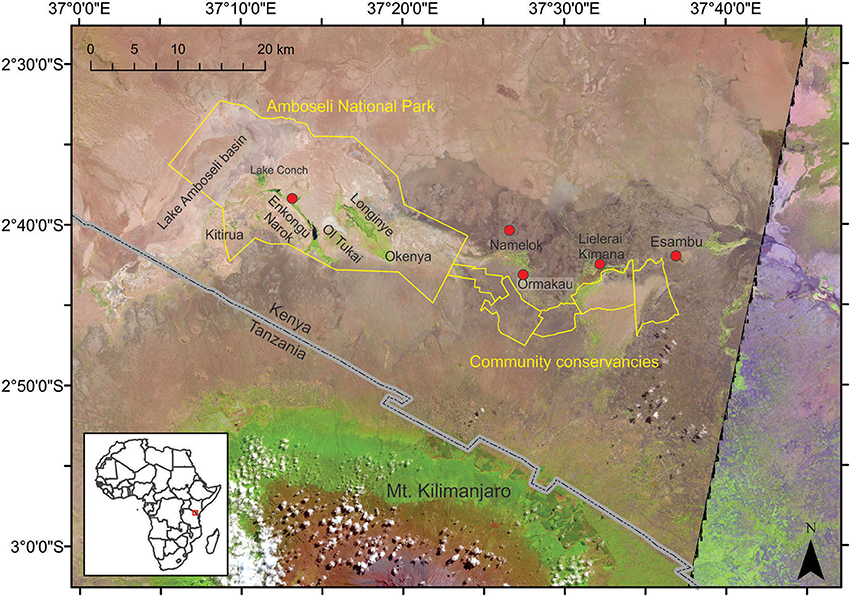
Figure 1. Location of the study region in Africa map (bottom left inset). Esambu, Kimana, Ormakau, Namelok, and Enkongu coring sites (red dots) and the community conservancies (yellow delimitations) that create a corridor through the wetlands westward toward Amboseli National Park (main map). Base Landsat images LC81680622014194LGN00 (left side and inset) LC81670622015014LGN00 (right side).
Physiography of Amboseli
Located north of Kilimanjaro (Figure 1), the Amboseli basin formed through isostatic down warping of country rock due to the mass of the Kilimanjaro volcano upon the crust (Pickford, 1986). Regional lithology can be divided into three major groups (Touber et al., 1983):
1. The Precambrian metamorphic basement rocks present across much of the northern region (Williams, 1972).
2. Late Pleistocene lacustrine-derived plain and clayey, alkaline soils of the Amboseli Lake bed, which were deposited under wetter-than-present conditions; these soils are comprised of impure limestone, marls, and local diatomite, with sepiolitic clays near springs and minor inputs of volcanic ash (Williams, 1972; Stoessell and Hay, 1978; Hay and Stoessell, 1984).
3. Tertiary to Quaternary volcanic rocks and lavas around the footsteps of Mt. Kilimanjaro and reworked Pleistocene pyroclastic deposits elsewhere in the southern part of the region.
Hydrology in Amboseli is controlled by climate, geology, and topography. Groundwater originates from Kilimanjaro and supply is controlled by fractures, rock porosity, and drainage network. Rainfall within the lower elevations of the Amboseli watershed is currently about 350 mm per year concentrated within two rainy seasons (November to January and March to May), with a negative evapotranspiration-precipitation budget with warm mean temperatures (21–25°C) and a range of 12–35°C (Meijerink and Van Wijngaarden, 1997; Altmann et al., 2002; Worden et al., 2003). Hydrological models estimate that spring discharge could exist at 1,450 m asl on the slopes of Mt. Kilimanjaro (Meijerink and Van Wijngaarden, 1997), and wetlands persist currently at elevations between 1,150 and 1,250 m asl. Lake Amboseli (1,125 m asl) is recharged by diffuse flows from groundwater seepage and minor surface flow from the Namanga and Sinet Rivers (Meijerink and Van Wijngaarden, 1997). Currently, the lake ephemerally fills with shallow water, but geological evidence suggests the lake was much larger and deeper during most of the Late Pleistocene (100,000–20,000 cal yr. BP) concomitant with higher water levels at Lake Challa (Williams, 1972; Moernaut et al., 2010). Since the end of the Last Glacial Maximum, eastern Africa has experienced high hydroclimatic variability evidenced by fluctuating lake levels owing to wetter intervals and arid phases (Nicholson and Yin, 2001; Kiage and Liu, 2006; Verschuren and Charman, 2008; Moernaut et al., 2010; Tierney et al., 2011) likely resulting in highly variable water depths at Lake Amboseli with the possibility of intermittent desiccation (Stager and Johnson, 2008). To date, however, there are no detailed paleolimnological studies of Lake Amboseli either to constrain the ages of the lacustrine deposits or to reconstruct past water depth through paleo shoreline mapping. Boreholes (Williams, 1972) have not ascertained the maximum depth of the lacustrine deposits above the basement metamorphic rocks.
Currently, the Amboseli groundwater levels have been increasing since the 1950s, caused by neo-tectonism increasing the outflow of the deeper diffuse part of the flow system and increased runoff caused by overgrazing in the catchment, without a corresponding increase in long-term rainfall (Meijerink and Van Wijngaarden, 1997). This contributed to localized tree mortalities (Acacia) due to increased salt concentration and hydric soils but, not enough water to re-fill of the Lake Amboseli Basin. Riverine wetlands persist on flat topographies where river flows can overbank as flow slows down and broadens and hydric soils are maintained by high groundwater levels. Some of these wetlands, such as Kimana, are supplemented by groundwater seeps and springs, whereas others are primarily sourced from them, e.g., Enkongu.
Controls on the characteristics of wetlands result from interactions between local and regional environmental drivers. For Amboseli, regional-scale drivers include hydroclimatic variability, degree of forest cover and land use type on Kilimanjaro. Local-scale drivers include micro-topographic gradients, animals and anthropogenic activity, which contribute to the geohydrology through trampling, wading and wallowing, creation of wells and digging into springs. As well as influencing vegetation in the catchment, these processes can change surface and subsurface hydrology and forest-atmosphere exchanges in montane elevations. High wildlife and livestock grazing intensities in the area have been suggested as drivers of watershed vegetation changes and increased surface channeling during short wet periods (Meijerink and Van Wijngaarden, 1997; Western and Maitumo, 2004). The changing nature of the agro-pastoral-protected area economic system in the region has also contributed to land cover and wetland changes (Western and Van Praet, 1973; Worden et al., 2003).
Paleoenvironmental Prospects in Semi-arid Amboseli
Pollen records provide information of past vegetation compositional changes and can be used to explore interacting environmental and anthropogenic drivers of land cover change. Currently, there are 14 records of late Holocene vegetation change in semi-arid savannah ecosystems of eastern Africa (Table 1). Amboseli, being a semi-arid region and prone to both multi-year wetter and drier phases, poses multiple challenges and opportunities for paleoenvironmental reconstructions (Pigati et al., 2014). Sedimentological and glacial studies from the highlands of Kilimanjaro provide a long-term context for change in the lowlands (Thompson et al., 2002; Zech, 2006; Zech et al., 2011; Schüler et al., 2012), but offer limited insights regarding environmental change at a local scale in Amboseli, or at a fine temporal scale, both of which are of interest to various stakeholders, including current inhabitants, conservation workers, land managers, and demographers.
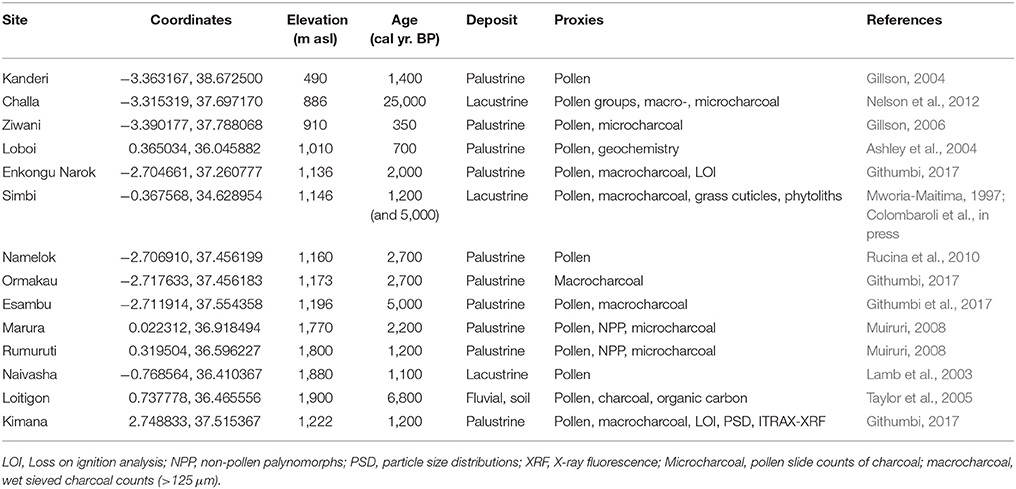
Table 1. Main data from paleovegetation studies derived from woody savannah ecosystems in eastern Africa.
Paleobotanical studies of semi-arid ecosystem deposits from older analogous sedimentary sequences, such as those of Olduvai, can be used to interpret current changes at Amboseli. Wetland deposits on low gradient landscapes exposed in 1.85 million year old rock at Olduvai Gorge, Tanzania, suggest that permanently saturated ground from groundwater seepage can persist through arid climate periods and fill with shallow standing water during wetter climates (Ashley et al., 2016). At Olduvai, these landscape features are associated with bone deposit evidence suggesting continued potential for exploitation by mammal and proto-human populations (Magill et al., 2012a,b; Ashley et al., 2016). One inference from such studies is that the spatial complexity of the landscape combined with temporal complexity provides an environment that is rich in research potential yet patchy. Paleoenvironmental records therefore require integration of different data sets to capture more of the spatiotemporal variability necessary for understanding these environmental complexities in relation to human land use and land cover modifications.
Paleoenvironmental studies have been published on ecosystem change in the lowlands surrounding Mt. Kilimanjaro, including Lake Challa (Blaauw et al., 2010; Nelson et al., 2012; Barker et al., 2013) and Namelok wetland (Rucina et al., 2010). These studies provide evidence of late Holocene environmental change at a decadal to centennial scale and are being complemented by ongoing sedimentological (Githumbi et al., 2016) and multidisciplinary historical ecology studies (Courtney-Mustaphi et al., 2015). Several spatially isolated, perennial and ephemeral wetlands persist across Amboseli today (Table 2). These wetlands are topographically divided at 1,250 m asl causing groundwater and channelized flowing westward to Lake Amboseli or eastward toward the Chyulu Hills.
The wetlands are maintained by groundwater seepage, springs and runoff. Wetland hydro geomorphology is modified by wetting and drying cycles, herbivores (Laws, 1968; Murray-Rust, 1972; McCarthy et al., 1998; Deocampo, 2002), and anthropogenic modifications (Murray-Rust, 1972; Rapp et al., 1972; Payton et al., 1992). Hydrology, topography, pedology, lithology, vegetation, and land-use types and intensities (Poesen et al., 2010) control surface channeling, uphill gullying and erosion. These interacting long-term processes include top-down hydroclimatic controls and local scale bottom-up controls of micro-topography, geology, animals, and people, forming a very complex and heterogeneous landscape. These processes present two main challenges for paleoenvironmental reconstruction studies: interruptions in sediment accumulation rates and reworking of previously deposited sediments. Hydroclimatic conditions can result in periods of lower water levels (regression) leading to no deposition and/or subaqueous or subaerial redeposition of sediments resulting in stratigraphic hiatuses. Bioturbation by mega herbivores, invertebrates and plants can also contribute to crosscutting and reworking of sediments. Bioturbation by plant roots and formation of rhizoliths are common characteristics of sedimentary deposits from the margin of paleowetlands in arid environments (Mount and Cohen, 1984; Liutkus and Ashley, 2003; Liutkus et al., 2005).
Methodology
This paper applied a quantitative and qualitative multi-proxy approach. They study involved radiocarbon dating, pollen and charcoal analysis, archeological surveys, analysis of documentary and oral historical sources and agent based modeling (ABM). Through combined pollen and charcoal analyse, and archeological and historical research we were able to track the extent to which humans, climate, livestock and wildlife have shaped the course of ecosystem dynamics in the Amboseli landscape. Charcoal and pollen samples were analyzed to get an in-depth understanding of the interactions and dynamics in the landscape. While pollen and charcoal analysis provided a deep understanding over a longue durée, historical methods were useful for understanding contemporary and more recent dynamics.
Coring
Site selection for this study was based on the depth and availability of a sedimentary archive. A suitable coring spot was determined by probing with fiberglass rods to locate the thickest sediment accumulation. The cores were retrieved using a Russian D-shaped corer (Jowsey, 1966) in 50 cm drives with 10 cm overlapped sections. Cores were transferred to PVC tubes, wrapped in plastic film and aluminum foil, shipped to the University of York, UK, and refrigerated at 4°C. Sediment cores were collected from seven locations (Table 3) in different Amboseli wetlands, of which five sites have been radiocarbon dated (Figure 2 and Table S1). Pollen analysis was carried out on 4 cores and 138 pollen types identified from Esambu, Namelok, Enkongu and Kimana swamps.
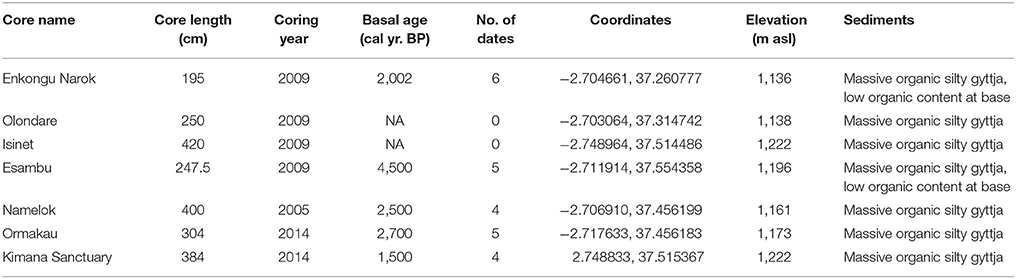
Table 3. List of sediment cores collected from Amboseli wetlands using a hand-operated, D-shaped barrel (5 cm diameter) Russian peat corer (Jowsey, 1966).
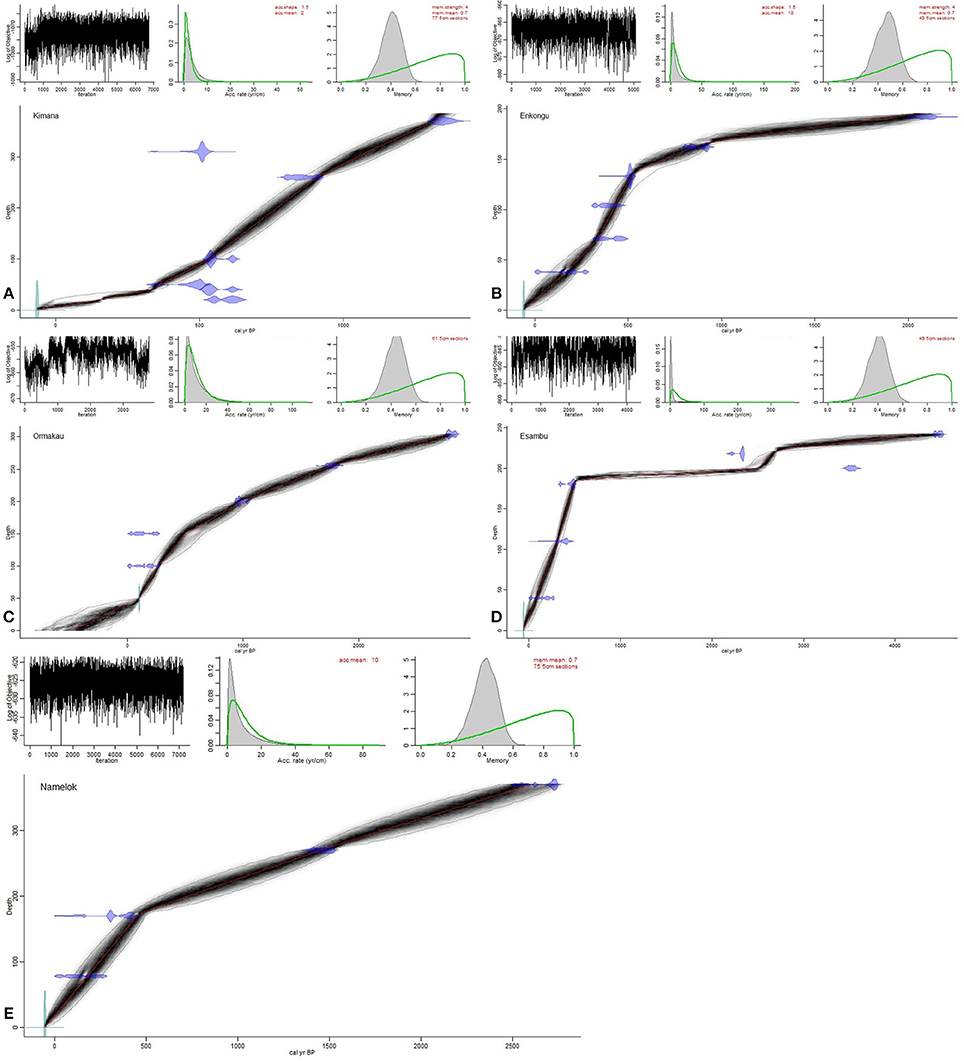
Figure 2. BACON age-depth models of (A) Kimana, (B) Enkongu, (C) Ormakau, (D) Esambu, and (E) Namelok wetlands from the radiocarbon dates acquired from the various labs.
Radiocarbon Dating
Bulk sediment samples were sampled from the sediment cores for radiocarbon dating, as there were no macrofossils present to pick for dating except one Acacia spp. wood fragment from the Esambu sediment. The bulk sediment samples were picked from each of the cores for radiocarbon dating at Direct AMS, the NERC Radiocarbon Facility-East Kilbride and the SUERC AMS Laboratory for 14C analysis. Six samples were selected from the Esambu sediment core; four from Namelok, seven samples were picked from the Kimana core, six from the Ormakau core and six from the Enkongu site. The samples were selected from sections where there was a change in sedimentary features such as texture and color. The number of samples selected for dating were limited by budgetary constraints.
The IntCal13 curve (Reimer et al., 2013) was used to calibrate the dates and presented in calibrated year BP (AD 1950) with the year 1950 chosen as it was around the time the first 14C dates were obtained (Birks et al., 2012). An age-depth model was developed for each site using BACON (Blaauw et al., 2010). The priors set were different for each age-depth model, for Kimana the minimum depth was set as 0 cm and maximum depth as 384 cm, the accumulation mean (acc.mean) was changed to 2 yr/cm as suggested by BACON during the analysis, a run of cal BP and calibrated 14C dates were used to run the model with 77.5 sections, acc.shape:1.5, mem.strength:4 and mem.mean:0.7 to produce the age-depth model. The Enkongu priors were set as minimum depth = 0 cm, maximum depth = 195 cm, acc.shape:1.5, acc.mean:20, mem.strength:4 and mem.mean:0.7. The Ormakau priors were set at minimum depth = 0 cm, maximum depth = 304 cm, acc.shape:1.5, acc.mean:10, mem.strength:4 and mem.mean:0.7 and 61.5 sections using a run of cal BP and calibrated 14C dates. The Esambu age-depth model was developed with minimum depth = 0 cm, maximum depth = 247.5 cm, acc.shape:1.5, acc.mean:20, mem.strength:4 and mem.mean:0.7 and 49 sections using a run of cal BP and calibrated 14C dates. An age-depth model was developed for Namelok, the priors were; minimum depth = 0 cm, maximum depth = 400 cm, acc.shape:1.5, acc.mean:10, mem.strength:4 and mem.mean:0.7 and 75 sections using a run of cal BP and calibrated 14C dates.
Pollen Analysis
A 1 cm3 sub-sample was obtained every 20 cm for pollen and spore analysis from Kimana, every 10 cm from Namelok, every 5 cm from Enkongu and every 2.5 cm from Esambu following the standard protocol (Moore et al., 1991). Pollen analysis was not carried out on the Ormakau sediment core. An exotic marker (Lycopodium spores) was added prior to pollen analysis to aid in calculation of absolute concentrations (Stockmarr, 1971). The 1 cm3 was sampled into 100 ml beakers together with the one Lycopodium tablet with a known number of spores (9,666 spores) added to each sample. 10 ml of HCl (to remove the calcium carbonate in the samples) was added to the samples, vortexed to encourage mixing of the sample with the acid and placed on a hot water bath for 2 min. Samples were then centrifuged at 3,000 revolutions per minute (rpm) for 3 min and the liquid decanted. Seven milliliter of distilled water was added to each sample, vortexed, centrifuged and the liquid decanted. This was done twice to remove all the HCl. Seven milliliter of distilled water was added to each sample, vortexed, centrifuged and the liquid decanted. This was done twice to remove all the HCl.
Twenty milliliter of 10% KOH (to digest organic matter) was added to the samples, which were then gently boiled while mixing on a hot plate to facilitate peptisation for 5 min, and then left to cool. The samples were sieved (to remove large unwanted particles) through 250 m mesh screens into 15 ml polypropylene centrifuge tubes and centrifuged for 1 min at 2,000 rpm and the liquid decanted. Samples were then washed twice with deionized water by adding 2–3 ml deionized water, thoroughly shaking the samples, topping up with water and centrifuging at 2,000 rpm for 1 min.
If carbonates were still present 1 ml ethanol and 1 ml H2O were added and the sample vortexed. Seven ml of 96% Glacial acetic acid was added and carefully mixed into the mixture and left for 8–12 h. Samples were then washed with deionized water to prepare for acetolysis. During acetolysis samples were washed twice with acetic acid, i.e., 4 ml, Acetic acid was added to the samples and centrifuged at 2,000 rpm for 1 min and the clear liquid decanted. Acetolysis mixture 96% H2SO4: Acetic anhydride at a ratio of 1:9 was added to the samples, which were heated in aluminum block heater to 100C. The acetolysis mixture digests the cellulose covering the pollen making the exine features distinct. The tubes were vortexed and heated further for 10 min. Samples were centrifuged and decanted to remove acetolysis mixture and washed twice in deionized water.
Heavy liquid separation using Sodium polytungstate (3NaWO4.9WO3.H2O with d = 2) was carried out to separate the pollen from the remaining organic material. Three ml of the heavy liquid was added to samples and vortexed, water was carefully added using a glass rod to prevent mixture of the two liquids, the mixture was centrifuged at 3,000 rpm for 1 min and an organic suspension layer appeared at the boundary between the heavy liquid and water before the organic suspension was then carefully transferred to another test tube and samples were washed twice in deionized water. Prepared samples were transferred to residue tubes using 96% alcohol, centrifuged and decanted. Glycerine (same volume as residue) was then added to the sample and the tubes left to evaporate in stove at 60C. Pollen samples were mounted onto the pollen slides where the identification and enumeration of the pollen, spores and micro charcoal was carried out at a magnification of 400–1,000 and from each slide a minimum of 300 pollen grains, excluding Poaceae and Cyperaceae, using a Leica DM4000B. The pollen grains were identified using images and descriptions from the African Pollen Database and published atlases (Hamilton, 1976; Hamilton and Perrott, 1980).
For each of the sites, a b-stick analysis was carried out to determine the suitable number of pollen zones. The zones were then delineated by carrying out a constrained incremental sum of squares (CONISS) analysis.
Charcoal Analysis
Subsamples of 1 cm3 of sediment were extracted at 1 cm intervals from the Kimana and Ormakau cores, every 0.5 cm from the Enkongu Narok core, and between 1 and 5 cm from the Esambu core. Macrocharcoal analysis was not carried out on the Namelok sediment core. The samples were soaked in sodium hexametaphosphate solution and a drop of hydrogen peroxide to disaggregate the samples and aid in the separation of the organic material and the clay particles (Bamber, 1982; Schlachter and Horn, 2010; Whitlock et al., 2010). Samples were wet sieved through a 125 μm mesh, the retained charcoal were identified by visual inspection and probed with a metal needle, and pieces were tallied under a Zeiss Axio Zoom V16 microscope at 10–40 X magnifications. All pieces above 125 μm were counted and the counts converted to charcoal concentration values, i.e., number of particles per unit of volume (pieces/cm−3) and charcoal concentration rates (number/cm2/yr−1).
Archeological and Historical Synthesis
This paper provides an overview of the literature pertaining to human occupation of the Amboseli area and pastoralism in East Africa more generally in the mid to late Holocene. Both primary (archival documents, oral histories, preliminary data on archeological surveys and excavations) and secondary sources were consulted to generate insight on human-environmental interaction in the pre-colonial and colonial era (Chuhila, 2016; Shoemaker, in prep). More detailed results on archeological research in Amboseli investigating change and continuity in pastoral livelihoods over the last thousand years is forthcoming (Shoemaker, in prep). Further examination of the history of twentieth century land use change on Kilimanjaro is available in Chuhila 2016. Yet rather than summarizing these archeological and historical studies, which were guided by complimentary but not identical research questions, this section instead explores potential ways that people may have directly and indirectly modified the vegetation and water catchment system in the wider Amboseli region, and how livelihood adaptations may have responded to changing wetland environments over the last c. 5,000 years. In order to avoid overly reducing the complexities of ecosystem dynamics on this landscape, or evoking environmentally deterministic explanations for livelihood change, this section is necessarily vague. The complications of combining and interpreting paleoenvironmental and archeological records are fully acknowledged. Yet, as the incompleteness of paleoenvironmental and archeological records will never be resolved, this must not preclude attempts to understand their interaction. The integration of historical, archeological and paleoenvironmental data in contemporary human-environmental models is critical, and this section is in contribution to their ongoing synthesis.
Modeling
We developed an ABM to understand the interactions between biophysical and socio-economic drivers of land use change by exploring the role of rainfall, socio-economic circumstances, and governance factors, in driving land use decisions by pastoralists and the impact of land use change on wildlife densities across the Amboseli landscape from 1950 to present. Coupled biophysical and socio-economic data can be used to link the interaction between natural and human factors (Boone et al., 2011). The biophysical variables (i.e., the availability of grazing resources) were simulated by the LPJ-GUESS (Smith et al., 2001) dynamic global vegetation model and were provided as an input to link together with other socio-economic variables in the ABM. LPJ-GUESS is a deterministic, process-based vegetation model that simulates plant physiological and biogeochemical processes as a function of changing climates (Lindeskog et al., 2013; Pachzelt et al., 2013; Bodin et al., 2016). It is driven by temperature, rainfall and atmospheric CO2 concentrations, with simulations run at both daily and annual time steps at a spatial resolution of 0.5 degrees (Bodin et al., 2016). Vegetation is represented as plant functional types distinguished by bioclimatic limits, morphology, phenology, photosynthetic pathway and life history strategy (Ahlström et al., 2015; Jönsson et al., 2015). The plant functional types compete for water and light (Lehsten et al., 2016) and their changing distribution at regional and functional scales is simulated (Quillet et al., 2010; Scheiter et al., 2013). ABMs can be used for linking the biophysical and socio-economic components of social-ecological systems characterized by multiple, stochastic and non-linear interactions (Matthews and Bakam, 2007; Rounsevell et al., 2012; Bert et al., 2014). Applications of ABMs offers a mechanism for incorporating human decision-making criteria on land use change at multiple scales (Matthews et al., 2007; Schindler, 2013; Bert et al., 2014).
The period for the ABM is from 1950 to present. Long-term mean rainfall from Climatic Research Unit (CRU) was used in LPJ-GUESS to simulate vegetation biomass for Amboseli for the period between 1950 and 2005 at 0.5 × 0.5 degree resolution. The simulated biomass was converted to kilograms per kilometers squared and used as input data in the ABM to simulate the biomass available to wildlife and livestock. To parameterize other ecological and socio-economic variables used in the ABM, we used data on animal densities, grazing rates, income levels, household densities and irrigation probabilities from literature focussed on pastoralists/agropastoralists in Amboseli (De Leeuw and Tothill, 1990; BurnSilver, 2009; Nkedianye et al., 2009) and from the 2009 Kenya census (KNBS, 2010). For each parameter, we used the mean and standard deviation to incorporate stochasticity in the ABM. To capture multiple land use change behaviors observed in Amboseli in the ABM, we applied the principle of pattern oriented modeling (POM) (Grimm et al., 2005) in the model design. We used insights from semi-structured interviews conducted with pastoralists in Amboseli in January and February 2016. The interviews focussed on the history and drivers of land use types, land tenure, livelihood strategies and land management.
The ABM was built in NetLogo (version 6.0.2; Wilensky, 1999). The model design adopted the “pattern oriented modeling” (Grimm et al., 2005) approach, using multiple land use change behaviors observed in the Amboseli ecosystem as a guide. Some of these system behaviors include: (1) pastoralism is hampered in subdivided rangelands that are densely populated, (2) high rainfall areas are the first to be converted to rain fed agriculture, (3) there is a high probability of irrigated agro pastoralism near wetlands, and (4) with high wildlife density and significant benefit from wildlife conservation initiatives, landowners are likely to use their land for conservation. Using empirical data from local community experts in Amboseli, from literature (De Leeuw and Tothill, 1990; BurnSilver, 2009; Nkedianye et al., 2009; Okello et al., 2016), and the latest (2009) Kenyan census, we derived parameters and values for socio-economic and some ecological factors such as income levels per land use types, household density, number of tropical livestock units and wildlife density. The influence of grazing resources and socio-economic factors on land use type and the impact of adopted land use types on wildlife was then simulated.
Results and Discussion
Amboseli Chronology
Five wetlands (Figure 2) across Amboseli have been radiocarbon dated to provide a chronological framework for past ecosystem histories. Although the sites have complex age-depth relationships, and were treated with large chronological uncertainty regarding their interpretation, there are coherent patterns of paleoenvironmental change at individual sites and across the sites.
Five dates from Kimana wetland when modeled give the basal date of Kimana core to be ~1,200 cal yr. BP. The model recognizes the age from 310 cm as an outlier as it is younger than the depths from the two levels above it (Figure 2A). The Enkongu core has a basal date of ~2,000 cal yr. BP modeled from the six radiocarbon dates. There is an age reversal where the sample from 100 cm was older than the samples from two lower depths, as a result three dates were noted as outliers and not modeled (Figure 2B). At Ormakau, the six dates are modeled to give a basal date of ~2,700 cal yr. BP with one date left out of the model (118 from 150 cm) which was also recognized as an outlier due to being younger than the two dates above it (Figure 2C). Linear interpolation of the six Esambu radiocarbon dates show that the sediment record spans from ~4,974 cal yr. BP (Figure 2D). The Namelok age-depth model (Figure 2E) incorporated all four radiocarbon dates spanning from ~2,594 cal yr BP to present.
There are several probable causes for the abrupt changes in sedimentation rates, the potential hiatus or reversed ages observed in these cores: they may have an erosional cause resulting from the change from dominantly semi-arid conditions to increased moisture, or a depositional cause by increased aridity with subsequent cessation or very low sediment accumulation. Significant bioturbation by the wildlife that utilize these semi-arid wetlands could also disturb the stratigraphic order of the sediments. Despite these chronological challenges, coherent and repeatable age-depth models can be constructed from the sites and the proxy evidence appears similarly robust with coherent signals of change detected through the sedimentary sequences.
Amboseli Vegetation Dynamics since the Mid-Holocene
The pollen data from Esambu, Namelok and Enkongu (Figure 3) are summarized into broad ecological groups that enable comparison between the sites and engender linkages to other stands of evidence. Across the landscape sedges (Cyperaceae and Typha sp.), grasses (Cenchrus ciliaris, Cynodon dactylon, Digitaria ciliaris, and Pennisetum sp.), shrub and tree species in the adjacent riverine areas (Acacia spp., Amaranthaceae, Balanites spp., Commiphora spp., Euphorbia spp., and Tabernaemontana elegans) currently dominate the wetlands. The assemblage indicates that vegetation mosaics had different compositions in the past, and in what follows contrasts between these will be treated as successive discrete time periods that characterize the main phases of environmental change.
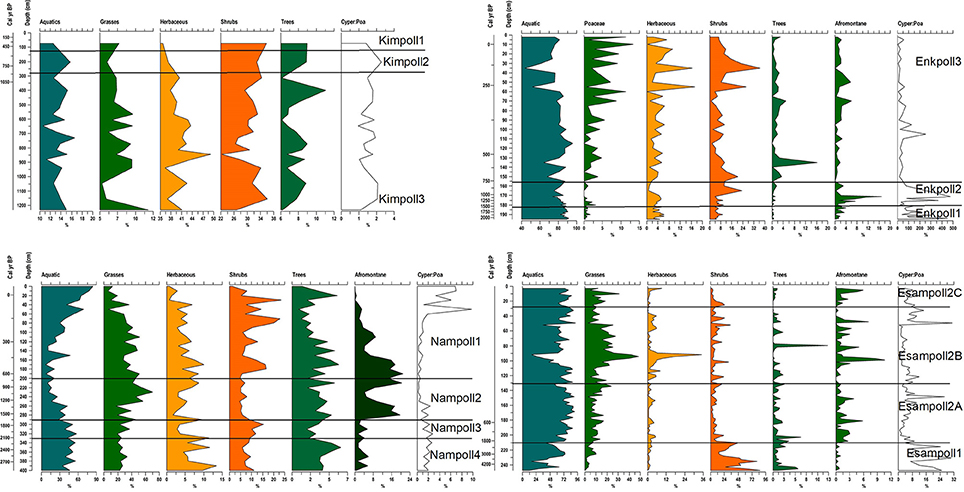
Figure 3. Pollen taxa identified from Enkongu, Namelok, Kimana and Esambu sediment records since the mid Holocene classified into vegetation types (aquatic, grasses, herbaceous, shrubs, trees, Afromontane and the Cyperaceae:Poaceae ratio). Table S2 lists all the pollen taxa and can be referred to for taxa in each group.
Between ~5,000 and 2,000 cal yr. BP, as seen in ESAM1 (Figure 3), the local Esambu ecosystem was a semi-arid woodland environment with Amaranthaceae/Chenopodiaceae and Acacia spp. as the dominant taxa followed by Asteraceae and Capparis sp. Low abundances of aquatic and semi-aquatic taxa (i.e., Cyperaceae, Nymphaea sp., Typha sp., and Tapura sp.) indicate that the Esambu wetland was much more restricted than today. There was a high presence of non-pollen palynomorphs (NPP) abundance and the most dominant species suggest a high herbivore density, implying wild herbivores were concentrated within a relatively small area to access water and grazing. The Namelok record also suggests that the vegetation around the wetland was more open from ~3,000 to 2,100 cal yr. BP with low abundance of tree taxa coupled with an increase in the abundance of shrubs and herbaceous taxa. Charcoal concentration at Esambu (Figure 4) was very low suggesting few fires, due to the reduced fuel connectivity because of the low vegetation cover.
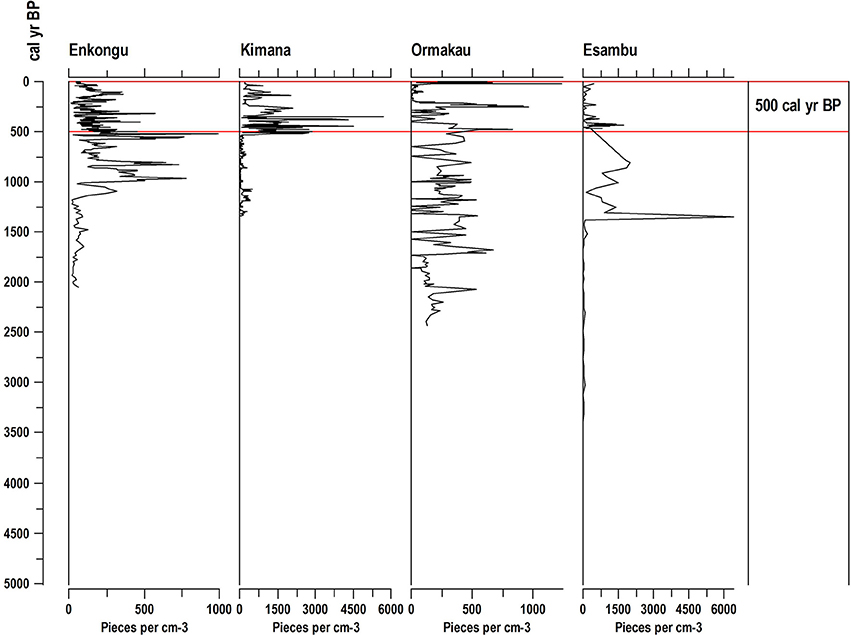
Figure 4. Macrocharcoal records from the Esambu, Kimana, Ormakau and Enkongu sediment records since the mid Holocene. The ~500 cal yr. BP zone where macrocharcoal concentration changes differ significantly across the sites is highlighted.
From ~2,000 to 400 cal yr. BP (ESAM2) there was increased moisture and fuel connectivity linked to macrophyte expansion around the Esambu wetland margin. There was an increase in pollen diversity with increased shrub and woodland (Balanites sp. and Cordia sp.) and aquatic taxa as well as Afromontane taxa (Celtis sp., Commiphora sp., Croton sp., Juniperus sp., Olea sp., and Schefflera sp.) on the adjacent highlands. The Namelok record (Figure 3) captures an arid phase maintaining the open semi-arid shrubs and low aquatic taxa abundance between ~2,100 and 1,700 cal yr. BP (NAM2). From ~1,700 to 1,200 cal yr. BP, the Namelok record (NAM3) indicates a mesic phase with the increase of herbaceous taxa and Syzygium sp., followed by a decrease in Syzygium sp. coupled with an increase in Acacia spp., Amaranthaceae/Chenopodiaceae, Euphorbia sp. and particularly Poaceae up to ~700 cal yr. BP. The Enkongu record (ENK1 and ENK2) indicates that the period between ~2,000 to 900 cal yr. BP was relatively mesic compared to the rest of the record, dominated by aquatic taxa, Poaceae, Asteraceae, Amaranthaceae, Solanum sp. and Maesa sp. forming a woodland savannah where woody taxa dominated over the non-woody taxa. There was an increase in charcoal concentration relative to the previous period suggesting greater fire frequency as the increased shrub and herbaceous taxa increased fuel load and connectivity. From ~700 cal yr. BP, there was a significant increase in the Cyperaceae and Poaceae abundance, in the Esambu record Cyperaceae, Poaceae and Typha sp. (aquatic taxa and grasses) dominate ESAM3. Within the Namelok record (NAM4) Asteraceae, Cissampelos sp. and Syzygium sp. decreased with increases in Acacia spp., Amaranthaceae/Chenopodiaceae, Cyperaceae and particularly Poaceae from 500 cal yr. BP. Within Enkongu (ENK3), the dominating taxa are the shrubs Amaranthaceae/Chenopodiaceae, Commelina sp., Solanum spp. and Asteraceae while the Poaceae abundance significantly increases.
Across the sites, the last ~500 cal yr. BP is identified as a significant period with drastic differences in macro charcoal records (Figures 3, 4). There is a significant decrease in macro charcoal concentration in the Esambu record however within the Kimana record there is a significant increase in the macro charcoal concentration (Figure 4). The pollen records point to increased standing water (~400 cal yr. BP) and within the recent past (100 cal yr. BP to present) an intensification of human modifications to the channels and wetland area for agriculture within the Esambu wetland. The Cyperaceae: Poaceae ratios (Figure 3) can be used as an indicator of water level where an increase in only Cyperaceae is due to falling water levels exposing sediment and providing a greater habitat for expansion of marginal wetland plants. Within the last ~500 cal yr. BP, the Cyperaceae: Poaceae ratio (Figure 3) indicates a consistently open landscape after a drop in the water level, reducing the size of the wetland at Enkongu. The Namelok record shows a previously open landscape and a continued reduction in the size of the open water area while Esambu shows a fluctuating extent of open water area.
Livelihood Strategies in Amboseli from the Mid Holocene
Archeological evidence suggests that many of the changes in vegetation in East Africa during Late Holocene were influenced by changes in human land use (Table 4). The following sections outline regional and local archeological and historical records to provide further detail concerning possible anthropogenic landscape modifications in Amboseli. Also considered are the potential ways that people in Amboseli responded to environmental changes.
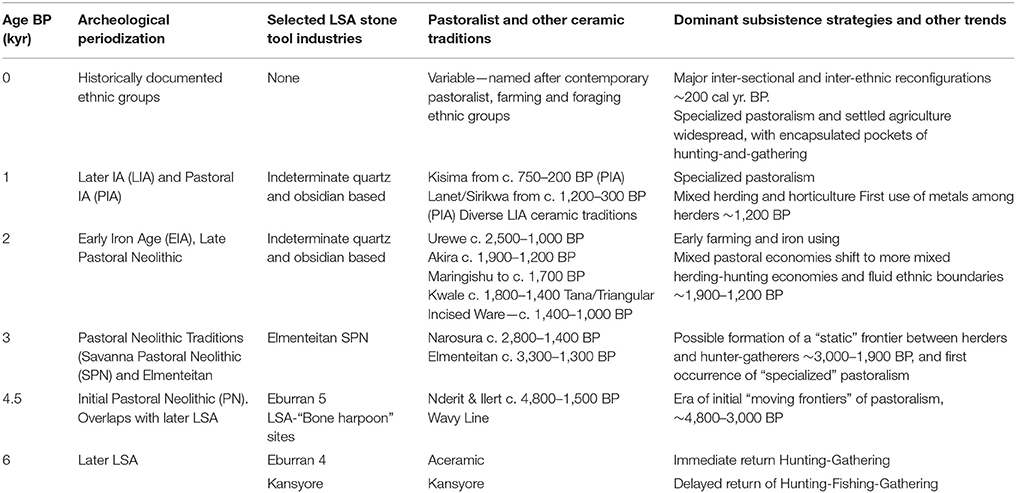
Table 4. Outline chronology of different archeological periods and material traditions in eastern Africa (after Lane, 2013).
Late Stone Age >c. 4,500 BP
In the early–mid Holocene, Late Stone Age (LSA) hunter-gatherer groups occupied eastern Africa including the Rift Valley in Kenya and Tanzania and adjacent highlands (Kusimba, 2013). Archeological evidence in the Central Rift suggests that LSA hunter-gatherer groups used rock shelters at forest-grassland ecotones and open-air savannahs (Mehlman, 1979; Ambrose, 1984, 1998; Marean, 1992; Kusimba, 1999, 2001). Faunal assemblages indicate hunting of forest-savannah game, though honey was also an important food (Ambrose, 1984; Marean, 1992). Little is known about LSA hunter-gatherer plant use in east Africa, but ethnographic analogies suggest people would have been consuming, tending, and transplanting wild plants to varying degrees (Marshall, 2001; Marlowe and Berbesque, 2009). On the shores of Lake Turkana and Lake Victoria, hunter-fisher-gatherer peoples also intensively exploited lacustrine resources including fish and shellfish (Robbins, 1972; Lane et al., 2006, 2007; Dale and Ashley, 2010; Prendergast and Lane, 2010; Prendergast and Beyin, in press).
Prior to the adoption of crops and livestock, regional aridity caused forests to recede to higher elevations, grassy savannah expanded as lake levels fell, and rainfall decreased from ~ 5,000 cal yr. BP (Ricketts and Johnson, 1996; Damnati, 2000; Gasse, 2000; Marchant and Hooghiemstra, 2004; Russell and Johnson, 2005). In the Central Rift, LSA hunter-gatherers may have shifted settlement locations to higher elevations as savannah-montane forest ecotonal areas advanced in elevation (Ambrose and Sikes, 1991). In Amboseli, the Esambu pollen record from this study suggests that while the local ecosystem was dry, the permanent water availability of the wetlands still served to attract herbivores in relatively dense concentrations. During this period of intense aridity, Amboseli wetlands were perhaps one of a few permanent sources of water in an otherwise dry landscape. These wetlands may have been of particular importance to hunter-gatherers and wildlife at this time. Conversely, during the mid-Holocene Dry Phase foragers may have preferentially occupied higher elevation zones, such as on nearby Mt. Kilimanjaro. More intensive surveys and excavations focused on elucidating the history of Holocene hunter-gatherers in Amboseli is required to clarify these issues.
Regionally, evidence suggests that some pre-pastoral LSA foragers engaged in moderate delayed-return subsistence strategies (Dale and Ashley, 2010), and fire was quite possibly used in Amboseli as a tool for landscape management from an early time. Contemporary hunter-gatherers in southern Africa are known to strategically burn grasslands (Lee, 1979), for example, as periodic burning in savannah environments serves to reduce and break up homogenous bush cover, promoting the productivity and diversity of biomes (Butz, 2009; Bond and Parr, 2010; Kamau and Medley, 2014). There are no known securely dated LSA hunter-gatherer occupation sites in Amboseli, however, making it difficult to surmise the behavior of early-mid Holocene inhabitants. It is likely that early foragers in Amboseli incorporated wild edible plant foods into their diets, consuming a variety of the starchy tuberous roots, fruits, legumes, grains, and leafy greens that occur in the landscape today (Altmann et al., 1987). It is possible that fishing also formed a component of hunter-gatherer diets in Amboseli at some time in the past. Catfish and other fish bones have been found in the dried Amboseli lakebed (Foley, 1981), and fish currently persist in the wetlands; although, they are not exploited as is the case in some wetlands of Tanzania (Hamidu et al., 2017). Hunting and scavenging of terrestrial fauna, also potentially contributed to LSA forager livelihoods in Amboseli.
Pastoral Neolithic (c. 4.5–1.5 ka BP)
Livelihood strategies based on domesticates began emerging as early as c. 4,800 years ago in northern Kenya, and by c. 4,100 cal. BP in south-central Kenya and northern Tanzania, marking the beginning of the Pastoral Neolithic (PN) in these areas (Lane, 2011a; Crowther et al., in press). Pottery wares such as Nderit and Ileret are found in association with wild fauna and the rare caprine dating to this time, indicating the nominal incorporation of domesticates into hunter-fisher-gatherer livelihoods (Gifford-Gonzalez, 2000). The transition to pastoralism in East Africa was neither immediate, nor comprehensive (Lane, 2004; Prendergast and Beyin, in press). Overall, during the mid-5th–4th millennium BP, domestic taxa constituted increasingly larger proportions of faunal assemblages and new technological traditions emerged, including the Savannah Pastoral Neolithic (SPN) (Marshall, 1990). SPN encompasses a diversity of archeological contexts associated with the onset of pastoralism in eastern Africa that bear certain material signifiers, such as Narosura ceramics and stone bowls (Wandibba, 1980; Robertshaw and Collett, 1983).
Around Amboseli, the earliest and closest archeological evidence for pastoralist habitation comes from an SPN site on the lower western slopes of Mt. Kilimanjaro (4,100 cal yr. BP) (Mturi, 1986). On the Galana River in nearby Tsavo livestock also appear in archeological deposits dated to c. 3,800 BP (Wright, 2005, 2007). At present, evidence for PN occupation of Amboseli is entirely based on survey finds, including ceramics bearing affinity to Narosura and Akira pottery wares and a fragmented stone bowl (Foley, 1981; Weissbrod, 2010; Shoemaker, in prep), making inferences about settlement and subsistence strategies difficult. The cultural provenience of Akira ware is also not well established (Ashley and Grillo, 2015), though radiocarbon dates from the type site GuJi 2 range between 1,255 and 1,695 cal yr. BP (Bower et al., 1977), spanning the Pastoral Neolithic–Iron Age time period. The association elsewhere between Akira with PN sites containing predominantly wild faunal assemblages has led to the suggestion that Akira pottery was produced by hunter-gatherer groups, and its wide distribution perhaps indicates its value as a trade good (Robertshaw, 1990). Akira ware may also be associated with a “final phase” of SPN expansion wherein wild resources were more wholly embraced (Bower, 1991).
Transitions to pastoral livelihoods were nevertheless underway before the end of the Mid-Holocene Dry Phase in East Africa. Early herders in Kenya contended with novel epizootic challenges and comparative aridity, factors which may have contributed to the lag in time between the first appearance of domesticates and the emergence of specialized pastoralism (Gifford-Gonzalez, 1998, 2000). Lake levels in northern Kenya appear to have been returning to higher stands around 3,300 cal yr. BP (Garcin et al., 2012), though the Esambu and Namelok pollen records from this study (Figure 3) indicate that wetter conditions did not prevail in Amboseli until after 2000 years ago. Long-term trends in aridity are not the sole considerations; the improved predictability and volume of bimodal rainfall regimes after 3000 years ago may have also encouraged specialized pastoralism across the region (Western and Finch, 1986; Marshall, 1990, 1994; Bower, 1991). However, the processes by which pastoralists became woven into the socio-economic fabric of East Africa were not entirely dependent on weather patterns. Livestock herding emerged and reproduced in multiple and dynamic ways across a myriad of landscapes during the span of millennia, and while the climatic context of this history is important, it did not determine it.
There is also increasing recognition that PN herders were not only responsive to environmental factors but that the arrival of livestock in East Africa also conditioned “emerging ecosystems” (Milton, 2003; Hobbs et al., 2006), with new species compositions and relative abundances driven by human interventions that had not previously existed. The soil nutrient and water retention enhancing properties of livestock dung, for instance, are known to lead to distinct successions of vegetation on abandoned pastoral settlements (Dunne et al., 1978; Western and Dunne, 1979; Reid and Ellis, 1995; Muchiru et al., 2009; Boles and Lane, 2016). There may have also been an acceleration of anthropogenic burning activity and a decline in dense bushland and woodland vegetation due to interventions by emergent herders in the latter half of the Holocene. After the inception of herding, the burning of vegetation in Amboseli may have served to improve the quality of pasture (Archibald and Bond, 2004), and to moderate tsetse-harboring woodlands and bushlands (Gifford-Gonzalez, 2000). The introduction of livestock to Amboseli would certainly have exerted considerable influence on the ecological development of the wetlands and surrounding environs. The timing and local circumstances under which livestock were adopted in Amboseli are still under evaluation, however. What is known is that the wetlands of Amboseli remained a source of permanent water on the landscape, no doubt attracting wildlife and livestock to some degree.
Emergence of Farming Communities (c. 2 ka BP)
Current evidence suggests that from around 2,000 years ago the first farming communities begin to be established in the western part of the region, spreading steadily eastwards and likely co-existing with established PN and LSA groups (Lane, 2004). In regional archeological terminology, the arrival of the first farmers marks the start of the Iron Age, traditionally distinguished (Oliver, 1966) by knowledge of iron working, the introduction of proto-Bantu languages, new pottery types, and the cultivation of African root (yams) and cereal (sorghum, pearl millet, finger millet) crops, supplemented by various legumes (De Langhe et al., 1995; Fuller and Hildebrand, 2013). Indian Ocean maritime exchange between Southeast Asia and Africa's eastern coast in the latter part of the (~1,500–1,000 cal yr. BP), if not earlier, was also responsible for the introduction of additional crops and animal species (De Langhe, 2007; Fuller and Boivin, 2009; Fuller et al., 2011a,b; Boivin et al., 2013, 2014; Prendergast et al., 2017; Crowther et al., in press).
Direct evidence for iron production (e.g., slag, tuyères) has not yet been recovered in the Amboseli area; although various Iron Age pottery wares and habitation sites are certainly present (Soper, 1976; Shoemaker, in prep). Furthermore, while the low-rainfall in the Amboseli basin (Figure 5) suggests this would not have been a viable area for agriculture, the potential for cultivation immediately surrounding the wetlands or at higher-rainfall altitudinal, zones within the Amboseli ecosystem cannot be dismissed. The Esambu and Namelok pollen records (Figure 3) show periods of increased precipitation where there is increased shrub and tree taxa (Nampoll4 and Esampoll1). Finds of grinding-stones, stone rings (potentially used as digging stick weights) and Early Iron Age Kwale pottery provide indirect evidence for farming and iron manufacturing communities on the northwestern slopes of Mt. Kilimanjaro going back as far as two millennia (Fosbrooke and Sassoon, 1965; Odner, 1971). Still, the higher precipitation rates on the southern and eastern slopes of Mt. Kilimanjaro were probably more attractive to agricultural settlement than the northern and western slopes, which lie in the rain shadow of the mountain (Stump and Tagseth, 2009). Livestock herding and foraging inhabitants of Amboseli would have been well situated to trade with iron working and farming communities on Mt. Kilimanjaro and in other highland areas to the southeast.
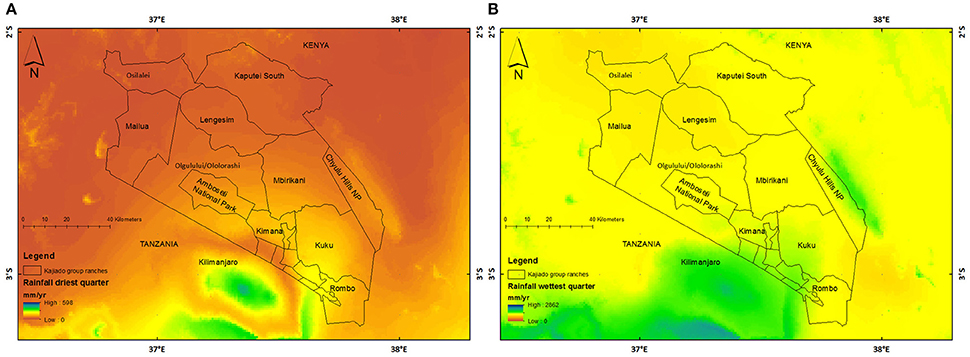
Figure 5. Rainfall driest quarter (A) and rainfall wettest quarter (B) in Kajiado County. The data is derived from WorldClim and it includes any consecutive 3 months of the year. (https://www.york.ac.uk/environment/research/kite/resources/#citation). In the driest quarter (A), areas near Mt. Kilimanjaro and the eastern side of Amboseli National park are wetter than other parts of Amboseli. These areas have been dry season grazing reserves for both wildlife and livestock but are now challenged with agricultural encroachment and habitat fragmentation. In the wettest quarter (B), a rainfall gradient is observed in the Amboseli area with the eastern areas being wetter than the western areas.
Concurrent with the arrival of Iron Age farming communities, there is some evidence to suggest a transition in pastoral economies in the Central Rift Valley between 1,900 and 1,300 cal yr. BP with herders shifting away from specialized pastoralism toward more highly mobile settlement strategies and a greater reliance on wild foods (Bower, 1991). Settlement sites in southwestern Kenya also appear to become smaller in comparison to earlier PN occupations (Robertshaw, 1990), perhaps being a factor of increased mobility. There is no indication that PN diets or mobility patterns changed substantially on the Galana River in Tsavo during the second millennium BP, however, (Wright, 2007), highlighting the potential diversity of pastoral adaptations during this time.
With the adoption of agriculture and metallurgy in the wider region after 2,000 cal yr. BP came opportunities for the intensification of trade and interactions between pastoralists, foragers, farmers and iron working communities in Amboseli. As documented elsewhere (Lane, 2011a; Crowther et al., in press), a dynamic mosaic of livelihoods including foraging, hunting, specialized herding, farming, and combinations thereof would have existed in the wider Amboseli area. Specialized relationships between farmers and herders may have even facilitated new, more intensive management and production systems by pastoralists and agriculturalists (Robertshaw, 1990; Davies, 2015). Unfortunately, compared to the coastal strip (Helm, 2000) and the Lower Pangani Valley to the south (Walz, 2010), little is known about the scale and dimensions of economic activity in Amboseli during the earliest Iron Age.
As agricultural and iron producing communities in East Africa emerged, a transformation in the capacity for people to modify their environments to suit their habitation (i.e., human niche construction) occurred (Boivin et al., 2016). Introductions of novel agricultural crops to the region would have also brought new pathogens, non-domesticated “weed” species, and some pre-existing taxa would have thrived on the biomes emerging on cultivated plots (Baker, 1991). The degree to which regional vegetation was modified by iron working is still under investigation though iron manufacturing in East Africa required charcoal, the production of which can be, though certainly not always, be linked to reductions in forest cover (Iles, 2016). Anthropogenically driven transformations of flora and fauna, potentially including land clearance and soil erosion on Mt. Kilimanjaro may have intensified, as has been observed in the Pare Mountain bloc over the last 2,000 years (Heckmann, 2014), with a spike in human landscape modifications occurring ~500 cal yr. BP, and c. 200 cal yr. BP (Finch et al., 2016). The impacts of such anthropogenic activities and potential modifications to the water catchment system may be responsible in part for the apparent shift in the hydrology and biota of the wetlands in Amboseli 500 years ago, though the mechanisms of this have yet to be discerned. The increased opening up of the landscape implied by increase in Poaceae and Cyperaceae accompanied by an increase in local fires suggested by the macro charcoal is one such indication of anthropogenic driven change.
The Later Iron Age (c. 0.5 ka BP−1900 CE)
During the last thousand years, there is mounting evidence that major socioeconomic and environmental changes were underway in the wider Amboseli region. A change in land use was taking place in locales across the region by the mid-first millennium BP, if not earlier, in the form of irrigation agriculture (Stump and Tagseth, 2009). Progressive anthropogenic land modification linked to agriculture was already occurring ~600 cal yr. BP in North Pare (Heckmann, 2014). In South Pare, greater levels of forest disturbance and an increase in pioneer taxa in the last 500 years similarly indicates intensive anthropogenic activity at montane elevations (Finch et al., 2016). Taken together, these lines of evidence suggest a certain intensification in agricultural activity over the last 500 years in the wider area.
Also occurring over the last 500 years was the introduction to Africa of domesticated plants, including maize, from the Americas as part of the “Columbian Exchange” (Crosby, 2003). Maize is now one of the most important crops consumed in Amboseli, although the date and pathway of its introduction to East Africa is largely unknown. “New World” crops such as maize likely arrived at trading ports along the Swahili coast with the Portuguese. Historical sources indicate maize was present on Zanzibar by 1643 (White, 1949), and being sold at markets in Pare by 1861 (Von der Decken, 1869). Paleoecological records find maize considerably earlier at Lake Naivasha ~500 cal yr. BP (Lamb et al., 2003), in the South Pare Mountains by ~400 cal yr. BP (Finch et al., 2016), and on the Laikipia Plateau by ~200 yr. cal BP (Taylor et al., 2005). The trajectory of maize cultivation on Mt. Kilimanjaro remains to be discovered, though it was present in the Loitoktok area in the nineteenth century (Meyer, 1900).
The hunting of elephants in the interior of eastern and southern Africa to supply external ivory markets has also been ongoing for centuries (Alpers, 1992; Håkansson, 2004; Shalem, 2005). This trade in ivory from the Swahili coast intensified considerably during the nineteenth century (Beachey, 1967; Cutler, 1985; Håkansson, 2004; Lane, 2010). With increasing involvement in the long-distance ivory trade, an expansion of irrigation agriculture and centralization of Chagga-speaking polities is suggested to have occurred on Mt Kilimanjaro's southern and eastern slopes (Stahl, 1964; Håkansson, 2008), resulting in the amalgamation of previously semi-autonomous clans of diverse ethnic origins, including Maa-speaking groups (Chuhila, 2016). Cattle, as “the lifeblood of political relationships” in the regional economy were in high demand in the nineteenth century (Håkansson, 2008). The entangled consumption of livestock and crops 200 years ago with the ivory trade elevated their status from mostly of significance to local and regional exchange, to that of international commerce.
The depopulation of elephants in Amboseli due to hunting may have also induced ecosystem shifts. In nearby Tsavo, for instance, an increase in elephant hunting has been suggested to have caused an expansion in scrub vegetation (Kusimba, 2009), although this link has not been conclusively established. Available paleoecological records from Kanderi Wetland (Gillson, 2004) indicate a marked phase transition from woodland to grassland in the Tsavo area commencing ~420 cal yr. BP and lasting to ~180 cal yr. BP, after which there was a return to more wooded conditions. Gillson (2004) tentatively attributes the opening up of the Tsavo landscape around 420 cal yr. BP to increased herbivory, probably elephant, but notes that phase transitions from grassland to woodland may be attributed to factors other than a decline in herbivore density. Elephants are known to exert a great deal of control over the vegetation in Amboseli, as has been shown by the loss of trees and shrubs in Amboseli National Park since the mid-1960s (Western, 2006). As rising numbers of elephants have come to seek refuge in the protected space, Acacia xanthophloea and Acacia tortilis woodlands have been heavily grazed and impeded from recovery (Western and Maitumo, 2004). However, very little is known about the local circumstances under which elephant populations were hunted in Amboseli over the last 500 years, making it difficult to evaluate the hypothesis that woodlands expanded over this time due to the removal of elephants. The exact extent of defaunation of elephants in the interior of East Africa is under investigation (Coutu et al., 2016). More information is available for the nineteenth century. Various historical sources indicate that elephants were rarely seen in the area east of Kilimanjaro in the late 1800s (Wimmelbücker, 2009, pp. 130–131). While it is conceivable that elephant populations were similarly depleted in Amboseli, it is also possible that elephant numbers increased here at this time, as these animals sought refuge from comparatively more extensive hunting activity to the east. Early European traveler accounts reveal that Amboseli was known to have had wild animals in abundance, but they also indicate that ivory hunters were present in the area (Rebmann, 1848; Thomson, 1885). Either way elephants with large tusks existed in the Kilimanjaro region in the nineteenth century, suggesting that herds had not been entirely decimated. This is best attested by a pair of tusks with a combined weight of just under 200 kg from a large bull elephant reportedly killed on the mountain in 1898, before being transported to Zanzibar and ultimately landing up in the Natural History Museum in London (Kunz, 1926), where they remain.
It is evident that adding to all of these transformations would have been the accumulation of landscape transformations driven by pastoral activity in Amboseli over the course of millennia. Herders continued to influence the spatial and temporal availability of soil nutrients across the region, with implications for the composition and distribution of plant and animal taxa. Anthropogenic transformations in Amboseli were not on a linear trajectory however, major disruptions occurring due to droughts, pathogens, and socio-economic upheavals introduced hiatuses and reversals in long-term trends.
The intensification and transformation of slavery and slave trading which occurred during the nineteenth century in East Africa (Alpers, 1975; Sheriff, 1987; Glassman, 1995) may have been a particularly insidious cause of turbulence in the wider region. In the Taita district of Kenya, Kusimba and Kusimba (2005) suggest that people relocated from the lowland plains to defensive rock shelters to protect themselves from slave raiding. There is little to indicate slave trading had a similar impact in Amboseli, though recognizing slavery in archeological contexts is challenging (Alexander, 2001; Lane, 2011b). Historical sources do indicate that early nineteenth century Maa-speaking pastoralists who are ancestrally linked to the current inhabitants of Amboseli were involved in the slave trade however (Krapf, 1854).
Considerable disruption and social upheaval among various livestock herding groups certainly occurred between 1840 and 1870 during the so-called Iloikop Wars (Waller, 1979; Galaty, 1993; Jennings, 2003). Maasai in Amboseli today recount that during the 1800s groups of Maasai, many of them from Tanzania, moved into Amboseli and the pastoralists they encountered were assimilated, migrated elsewhere, or killed. Regional paleoenvironmental reconstructions are now also indicating a drought of acute severity at the turn of the nineteenth century (Verschuren et al., 2000) with a number of social consequences for pastoral communities (Sobania, 1980; Anderson, 2016; Petek and Lane, 2016). It may be no coincidence that the Loikop Wars began toward the end of the 1830s, coinciding with the recovery of lake levels immediately following the intense drought and a corresponding recovery of rangeland (Anderson, 2016). Another period of drought in northeastern Tanzania in the 1830s may have further catalyzed Maasai movements north into Kenya (Wimmelbücker, 2009). The impacts of such a severe drought on societies in East Africa must have been radical, causing widespread famine, and the loss of livestock and human lives on a grand scale, and probably major migrations. This great drought at the end of the eighteenth century may have created the conditions for the emergence or perhaps re-instigation of the Maasai in the following years.
Another disturbance to pastoral livelihoods occurred in Amboseli in the late 1800s when devastation was brought to livestock herders across East Africa in the form of bovine pleuropneumonia, rinderpest, smallpox epidemics, and failed rains (Ofcansky, 1981; Waller, 1988). Cattle losses are estimated as high as 90%, while Maasai mortality rates have been suggested to have been up to 50% of the population overall (Leys, 1924). European traveler accounts indicate that stockless Maasai had taken up the cultivation of beans, maize and cassava in the Loitokitok area of Mt Kilimanjaro, though there were still pastoralists tending their herds in the Amboseli basin (Meyer, 1900; Bernsten, 1976).
Amboseli in the Colonial Era, c. 1900
At the turn of the twentieth century, the colonial encounter began in Amboseli ushering in an era of socio-economic sanctions and geo-political borders inconsistently enforced by two colonial governments varying in their motivations to maximize the potential of “Maasailand.” The general trend during the twentieth century in Amboseli was toward the confinement of livestock and herders within ever diminishing parcels of land (BurnSilver and Mwangi, 2007). Rangelands that were once flexibly and communally managed became increasingly fenced and settled (Western and Manzolillo-Nightingale, 2003; Mwangi, 2006; BurnSilver et al., 2007). There has also been an increase in the area of land cultivated using irrigation, particularly around the wetlands of Amboseli (Campbell et al., 2003). These changes have disrupted previous patterns of mobility and presented challenges to pastoralists living in Amboseli.
In the early colonial period Maasai people received little support to develop their livestock production sector and were at times actively hindered by the colonial administration (Dresang and Sharkansky, 1975; Spencer, 1983; Rutten, 1992); consequently, diversification of livelihoods toward agriculture proved attractive. In addition, as European settlers appropriated large swaths of East Africa (Hughes, 2006) and as populations rose, conditions were created for the scarcity of land, prompting the influx of farmers from other areas into the Amboseli region (Rutten, 1992, pp. 189, 191). The conversion of land for growing cash crops such as coffee for export also diminished the level of food security in the Kilimanjaro region (Wimmelbücker, 2009, p. 395), further encouraging the development of agriculture in lowland areas that had previously not been cultivated to the same degree (Chuhila, 2016).
The trend toward the influx of agriculturalists was not linear: in the 1950s, for instance, there was a reduction in cultivation throughout Kajiado District due to the eviction of Kikuyu families suspected to be connected to Mau Mau, though in this same decade irrigation farming projects were underway in Loitokitok and Kimana (Rutten, 1992). Irrigation farming schemes were common in the years following World War II as colonial authorities turned their attention to the development of semi-arid rangelands such as Amboseli (e.g., Swynnerton, 1955; ALDEV, 1962). Policies were implemented that aimed to facilitate the conversion of rangelands to farmlands, and enhance the productivity of the Maasai livestock economy through destocking and sedentarization, policies that have had enduring and often negative impacts on pastoral livelihoods and resources in Amboseli (Overton, 1989).
Another major development in the later colonial era was the increased pressure on the government to protect Amboseli wildlife from livestock, leading to interventions with little regard for consultation with local pastoralists (Lindsay, 1989). According to colonial administration reports from the 1950s, it was perceived that competition between pastoralists and wildlife had reached an untenable level. A narrative was put forth at this time that continued grazing in the Amboseli National Reserve would result in depletion of the area's resources and wildlife. For example, Donald Ker, a Kenyan hunter, safari guide, and conservationist of British descent, warned in 1955 that there had been an increase in the number of Maasai herds and that due to overstocking the Maasai must be relocated to new grazing and watering zones outside the central basin (Ker, 1955). Ker observed “many of the indigenous herds of game have been forced to leave what is now a dust-covered desert for grazing grounds many miles away, and travel back to their natural watering places to find them occupied by vast herds of Maasai stock during day-time, so that most of the grass-eating animals are compelled to drink at night or perish (1955).” In the late 1950s government authorities attempted to move livestock concentrations away from the central basin by constructing boreholes and dams in peripheral areas and regulating livestock grazing patterns (Lindsay, 1989). In 1974 a 488 km2 portion of the Amboseli basin was gazetted as a National Park, and Maasai access to this area was severely restricted.
Estimates of livestock populations between 1948 and 1984 indicate the number of cattle in Kajiado District has fluctuated a great deal but over the long term the population remained stable (Grandin, 1991), yet per capita livestock holdings have been falling steadily in Amboseli since the 1960s (Western and Manzolillo-Nightingale, 2003). Change in economic and population dynamics coupled with severe successive droughts for the pastoralist Maasai in the Amboseli ecosystem over the last 25 years has resulted in a 3.5-fold increase in crop cultivation supplemented by irrigation (Norman, 2010). Agriculture is more profitable than pastoralism or conservation, and much of the farming in Amboseli is dependent on the wetlands (Okello, 2005). Gaps in our knowledge regarding the wetlands, especially their hydrology, biodiversity and ecosystem functions hinder their sustainable management.
Modeling Recent Socio-Ecological Changes in Amboseli
Paleoecological, archeological, historical and ecological research has determined that many challenges currently facing pastoral systems in sub-Saharan Africa are attributable to environmental factors, caused by climate variability and habitat fragmentation, driven by land use change (Hailegiorgis et al., 2010). Climatic variability affects the availability of pasture and distribution of water points (Hailegiorgis et al., 2010) with pasture production being influenced by rainfall seasonality and storm patterns independent of total rainfall (Western and Manzolillo-Nightingale, 2003). In Amboseli, rainfall is highly variable across months and between years (Altmann et al., 2002; Figure 5), and droughts are a common occurrence (BurnSilver et al., 2007). This leads pastoralists to adapt different coping mechanisms that largely involve changing their land use types or diversifying their livelihoods. Ongoing modeling research seeks to understand the feedback between land use change and the ecology of Amboseli by exploring pastoral land use change decisions under different climatic and land tenure scenarios.
The high rates of pastoral land use change in Amboseli are driven by interacting biophysical, political and socio-economic factors operating at different time scales. The colonial and independent governments in Kenya perceived pastoralism as an inefficient method of managing livestock in Kenyan rangelands (Seno and Shaw, 2002; Western and Manzolillo-Nightingale, 2003; Mwangi and Ostrom, 2009) and advocated for policies that supported communal group ranch subdivision (Seno and Shaw, 2002; BurnSilver et al., 2007; Mwangi and Ostrom, 2009; Sundstrom et al., 2012). Private land ownership was perceived to bring economic development and a national level policy in their support was formulated in 1983 (BurnSilver et al., 2007). Around the same time, poor leadership and management of group ranch resources led to dissatisfaction among group members prompting the subdivision of most Kajiado group ranches from the mid-1970s (Galaty, 1993; Sundstrom et al., 2012). Additionally, between 1973 and 1984, immigrant communities from Central and Eastern Kenya, as well as Maasai herders, further influenced land use change in Amboseli by farming on the slopes of Kilimanjaro leading to rapid expansion of irrigated and rainfed agriculture (Campbell et al., 2003).
Livestock activities by pastoralists are well adapted to the variable habitat and shifting patch dynamics of arid and semi-arid areas (Bulte et al., 2008). Pastoralists' land use decisions are based on prevailing environmental and socio-economic factors and have differential impacts on the ecology and wildlife structure of dry areas. When deciding their livelihood strategies, contemporary pastoralists diversify their economic options and reduce their drought vulnerability using different strategies, such as agriculture, wage employment, diversification of livestock production and diversification of wildlife based revenues (Western and Manzolillo-Nightingale, 2003; Homewood et al., 2009; Reid et al., 2014). Most (92%) of Amboseli is classified as arid or semi-arid and droughts are characterized by the failure of either or both the short and long rains (BurnSilver et al., 2007). After experiencing severe droughts in 1977 and 1984, most pastoralists in Amboseli settled near the wetlands and practiced agriculture as a temporary survival solution and as a strategy to rebuild their herds (BurnSilver et al., 2007). Though they planned to go back to pastoralism, most became sedentary and shifted to agro-pastoralism (BurnSilver et al., 2007). Agriculture was perceived to have significantly higher and immediate income compared to wildlife conservation, which has minimal direct benefits for most households (Okello and D'Amour, 2008). Readily available water and pastoralists willing to lease their land to farming communities further promoted commercial agriculture around the Amboseli wetlands (Okello and Kioko, 2011). Additionally, wetter parts of Kajiado, which formed dry season grazing zones for livestock, had been taken up by agriculture posing a challenge to livestock herding (Western and Manzolillo-Nightingale, 2003). From these activities, a gradient of pastoral land use across the Amboseli landscape ensued with agro-pastoralism practiced around wetlands, pastoralism on subdivided lands and extensive pastoralism in the interior, dry rangelands zones (Homewood et al., 2009).
Similar trends of sedentarization have been observed in other pastoral areas in East Africa over the last ~100 cal yr. BP. The pastoral Pokot community of northwestern Kenya have changed from extensive livestock grazing land use to more sedentary and diversified livelihoods such as rain-fed agriculture, livestock marketing and honey production (Bollig, 2016; Greiner and Mwaka, 2016). Like in Amboseli, sedentarization among the Pokot began in fertile and wet areas, largely the highlands, and spread to the lowlands (Greiner et al., 2013). Agriculture was unsuccessfully introduced in the lowlands in the 1980s; however, by the 1990s, it had expanded to the lowlands where about 30% of households presently practice it (Greiner and Mwaka, 2016). In Loliondo, located in Ngorongoro District of northern Tanzania, most pastoralists have adopted agriculture combined with traditional pastoralism with peak levels of agriculture expansion documented in the 1970s (McCabe et al., 2010). Interestingly, most of the households adopt agriculture by choice and not out of necessity (McCabe et al., 2010; Greiner and Mwaka, 2016).
Understanding these interactions between natural factors and human activities in Amboseli is challenging because the system is dynamic, complex and constantly evolving. Our model outcomes show land use types as number of grids in kilometer squared (Figure 6). Actors are taken to be autonomous with an ability to interact with each other and with their environment (Valbuena et al., 2010). The dominant land use types are livestock grazing, smallholder agriculture, livestock grazing with conservation activities and urban or built-up areas. They overlap across the Amboseli landscape but show some underlying trends based on key drivers of change. On the whole, communal land tenure is predominated by livestock grazing while, the levels of livestock grazing and agriculture do not differ much on private land tenure (Figures 6A,B). During a wet year, the prevalence of agriculture rises in communal and private land, where in the communal land there are fewer numbers of grids with agriculture land use compared to private land. Availability of financial support from Non-Governmental Organizations (NGOs) and investors supporting wildlife conservation initiatives shows a trade-off between conservation and other land use types where pastoralists are willing to use their land for wildlife conservation if the conservation budget increases (Figures 6C–F). Consequently, wildlife density is correlated with livestock grazing and conservation land use and increases as the wildlife budget increase. Wildlife density is also slightly higher on wet years relative to dry years and where there is no support for conservation; it is inversely related to livestock grazing and agriculture in both communal and private land tenure. The interaction between rainfall and conservation budget show that on a wet year, when the conservation budget is small, agriculture levels are high but reduce as the conservation budget increases. We note that the proportion of land used for urban areas remains constant as the conservation budget increases. However, this is because, in the model, urban areas are largely dependent on current household density in Amboseli, which is static.
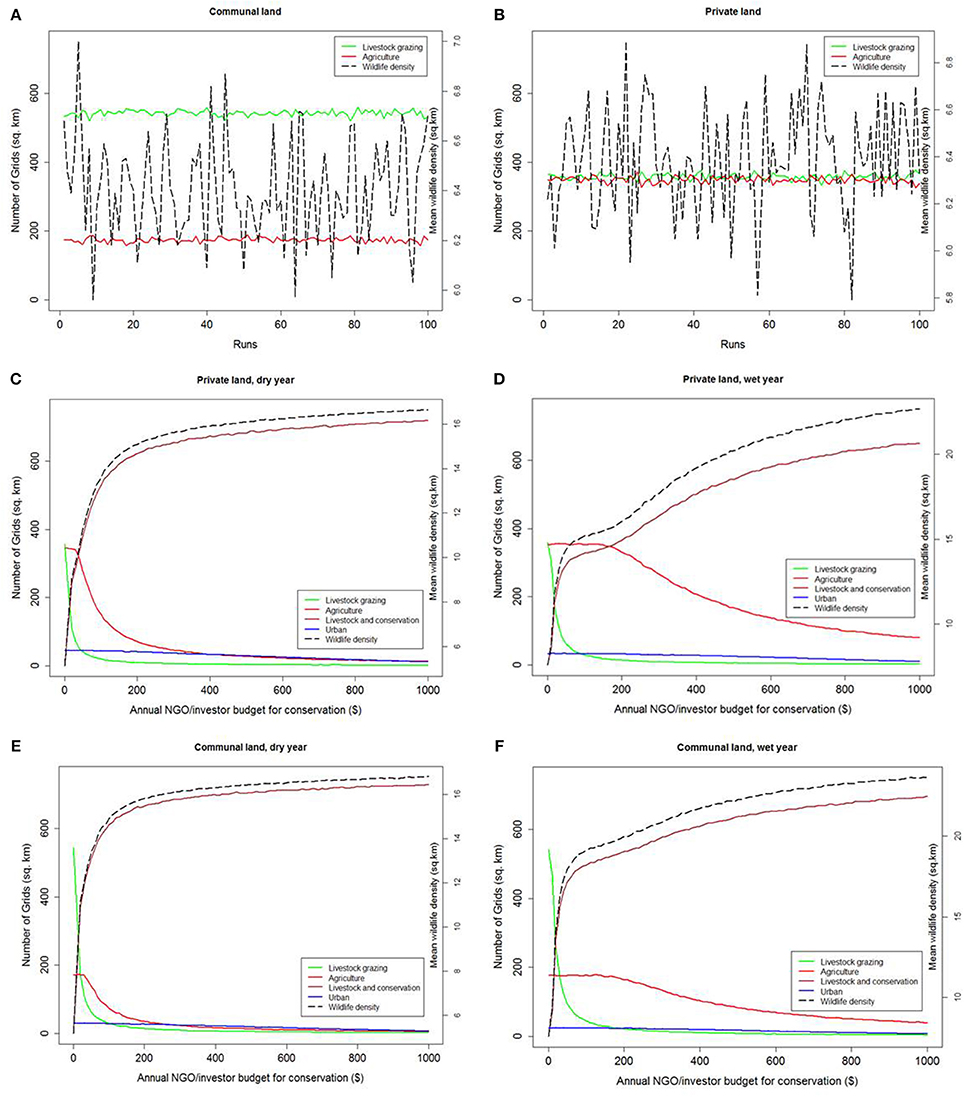
Figure 6. The relationship between land use types and wildlife densities as simulated from a socio-ecological model. (A,B) Show the trends between livestock grazing, agriculture and wildlife densities in community and private land tenure in Amboseli. (C,D) Compare the relationship between land use types and wildlife density in private land between dry and wet rainfall years as Non-Governmental Organizations (NGOs) or investors into conservation initiatives in Amboseli invest more money. (E,F) Compare land use trends, monetary investment into conservation initiatives and wildlife density between dry and wet rainfall years in community land.
By combining insights from vegetation data, interviews with local communities and other secondary data sources, our model shows the interaction of rainfall, land tenure types and availability of conservation budget in shaping land use patterns among pastoralists in Amboseli. It also shows the impact of land use choices on wildlife densities and how that relationship changes in different rainfall years and across land ownership types. Though our socio-ecological model treats different land use types independently, it also shows that the ability of pastoral communities to depend entirely on livestock grazing for their subsistence is declining, leading them to diversify their livelihoods and employ different strategies on and off their land.
Outcomes and Next Steps
The Late Holocene social-ecological history of Amboseli is characterized by a diversification in anthropogenic consequences for the landscape that we have traced in this paper from around the initial inception of pastoralist livelihoods c.4000 years ago to the emergence of agriculture, through the colonial period and into present times. These livelihood strategies emerged upon a context of a longer history of hunter-gatherer use of the animal, plant and water natural capital of Amboseli. As noted above, the temporal and spatial scale of the environmental effects of early herders in sub-Saharan Africa are only just beginning to be understood. However, despite the older assumption that livestock unilaterally denude the environments in which they live (Sinclair and Fryxell, 1985; Schlesinger et al., 1990), we have demonstrated that heterogeneous, dynamic, and biodiverse East African savannah ecologies have existed with pastoralists as active participants for millennia. After two millennia of what was likely relatively low-density livestock herding and continuing hunting and gathering by different social groups, the cultivation of diverse African and other domesticated crops began around the margins of the ecosystem, most notably on the slopes of Kilimanjaro, creating a mosaic of ethnic groups, land use strategies and settlement systems. These dynamic groups engaged in exchange relationships with each other and were linked to wider networks. The latter most conspicuously involved commodities trade, such as ivory, from at least the late second millennium CE, and connected Amboseli inhabitants to neighboring and distant regions that included the Indian Ocean and, ultimately, also the Atlantic world system. From the late nineteenth century, colonial era policies disrupted and transformed livelihoods and land use practices in East Africa. Pastoralism has nonetheless continued to the present and remains the food production system that culturally and economically defines Amboseli.
Most socio-ecological systems have undergone massive climatic, landscape and institutional changes over the last century (Goldman and Riosmena, 2013) and recent studies have shown that their adaptive and resilient nature plays an important role in mediating impacts of environmental changes and allowing social responses that sustain production (Anderson and Bollig, 2016). Pastoralists in Amboseli never stop practicing livestock grazing entirely despite the environmental, socio-economic and political challenges they face. Instead, they apply different pastoral and agro-pastoral land use strategies. Like most pastoral systems in East African drylands, livestock grazing remains the main land use type (Seno and Shaw, 2002; Homewood et al., 2009) and plays a major role in Amboseli's culture and economy (Sundstrom et al., 2012). Over the past decades, populations have increased markedly and the introduction of intensive agriculture surrounding the Amboseli wetlands has resulted in unprecedented change. Given the rapidity of land cover change and challenges surrounding human-ecosystem-climate interactions, there is increasing interest in exploiting the potential of paleoenvironmental and archeological records characterizing these changes to contextualize processes governing long-term dynamics and development of the ecosystem.
As outlined above, a comprehensive understanding of interactions between environmental change, social use and the important drivers that have determined ecosystem composition and structure within the Amboseli ecosystem since ~5,000 cal yr. BP is emerging. Further extensive archeological surveys and intensive archival work are needed to enhance and fill in the gaps about the movement of people and animals as well as the decisions influencing their behavior. Long-term investment is needed from all stakeholders, i.e., the local communities, the local and national government, the conservation and tourism industry as well as researchers, to continue in the development of adaptive and sustainable management plans for current and future Amboseli communities.
Undoubtedly, finer resolution data will improve our understanding of past socio-ecological dynamics within the landscape, especially due to the different responses that have been observed, likely indicating local and regional drivers of change. However, alongside gaining a greater comprehension of events that have occurred in the past the next step is also to build on the combined datasets and the insights from them by fully exploring their application to present-day and future issues. One such issue that has formed a theme throughout this paper concerns the longevity and adaptive capacity of the pastoralist lifestyle in Amboseli. However, this is thought to be increasingly under threat from factors such as competing land uses, changing weather patterns and climate, the increasing move to sedentarization, degradation of the wider landscape and restrictions on access to labor as more children go into (and stay in) education and/or then move away (Seno and Tome, 2013).
The current predictions for population growth in Kenya suggest that numbers will almost double by 2050 (United Nations, Department of Economic and Social Affairs, Population Division, 2017); climate change projections suggest that temperatures on the African continent are expected to rise faster than the rest of the world (Adhikari et al., 2015); and rainfall patterns will be substantially altered (Platts et al., 2015). These predictions lead to questions such as what are the implications of these changes to pastoralism in Amboseli? Will pastoralists continue to adapt and change? Will a tipping point be reached and when? We cannot know the answers to these questions from the data and insights presented alone, but this does not mean that they are of no relevance.
The final technique that we utilized was ABM. This approach was used to help understand the complex and shifting biophysical and socio-economic factors affecting pastoral livelihoods in the very recent past. However, by continuing to work alongside modelers there is potential to combine techniques such as ABM or Bayesian modeling with the datasets from the past in order to interrogate them predictively for the future. Combining the different datasets presented here has already illustrated the adaptive capacity of pastoralists over the longue durée changing environmental and social pressures, the next step is to actively use these insights within models with specific questions in mind. This would not only provide estimates and models that are potentially useful to a range of stakeholders, e.g., the Amboseli community, planners, conservation specialists etc., but they would also allow us (as researchers) to pose research questions—at what point may a pastoralist lifestyle become unviable in Amboseli or will it thrive? What are likely to be the factor/s driving these changes? The Amboseli landscape has seen a long history of change, affected by both local and regional factors, and we are only just coming to comprehend this complexity using the interdisciplinary approach taken here. Landscape evolution does not stop at the end of a paleoecological core or an oral history account, but it continues to evolve, we need to find nuanced methods for linking these valuable historical insights into the current, and informing the future, management of the these important conservation and pastoral landscapes.
Author Contributions
Primary research on the paleoecological records of Amboseli was undertaken by EG, CC-M, supervised by RM; primary research on the archeology of Amboseli was undertaken by AS, supervised by PL; MC, and AS undertook the historical research relating to Kilimanjaro and RK developed the socio-ecological models, supervised by RM. All authors contributed to the drafting and writing of the manuscript. All authors approved it for publication.
Conflict of Interest Statement
The authors declare that the research was conducted in the absence of any commercial or financial relationships that could be construed as a potential conflict of interest.
Acknowledgments
We gratefully acknowledge those who helped in sediment collection and processing, such as Veronica Muiruri, Rahab Kinyanjui, Rebecca Muthoni and Stephen Rucina from the National Museums of Kenya; and Nik Petek, Geert van der Plas and Aynalem Zenebe from the REAL project. John Kanyingi helped survey wetland areas of Amboseli. We thank Rebecca Muthoni and Lauren Shotter for collecting data in the laboratory. We thank David Western, and the wider African Conservation Centre, for continued discussions on the Amboseli research. National Commission issued permits for this research for Science, Technology and Innovation permits NACOSTI/P/14/6965/1096. This research was partially funded by a European Commission Marie Skłodowska-Curie Initial Training Network grant to PL and RM titled “Resilience in East African Landscapes (REAL)” (FP7-PEOPLE-2013-ITN project number 606879). CCM was supported through the “Adaptation and Resilience to Climate Change (ARCC)” project under the Sustainability and resilience—tackling climate and environmental changes programme funded by Vetenskapsrådet, Sida and Formas (2016-06355) awarded to PL and RM. The British Institute in Eastern Africa are thanked for their continued support.
Supplementary Material
The Supplementary Material for this article can be found online at: https://www.frontiersin.org/articles/10.3389/feart.2017.00113/full#supplementary-material
Table S1. Radiocarbon dates from four Amboseli sites with lab ID and sample information. Bulk sediment was dated at all the sites except at *Esambu 180.5 cm.
Table S2. Table containing a list of all the pollen identified from the four Amboseli records, i.e., Enkongu, Kimana, Esambu and Namelok. The taxa identified, family and Plant Function Type are listed. Pioneer and disturbance taxa are highlighted. X means present N means absent. Classification into groups is based on http://www.africanplants.senckenberg.de/root/index.php
References
Adhikari, U., Nejadhashemi, A. P., and Woznicki, S. A. (2015). Climate change and eastern Africa: a review of impact on major crops. Food Energy Sec. 4, 110–132. doi: 10.1002/fes3.61
Ahlström, A., Xia, J., Arneth, A., Luo, Y., and Smith, B. (2015). Importance of vegetation dynamics for future terrestrial carbon cycling. Environ. Res. Lett. 10:054019. doi: 10.1088/1748-9326/10/5/054019
Alexander, J. (2001). Islam, archaeology and slavery in Africa. World Archaeol. 33, 44–60. doi: 10.1080/00438240120047627
Alpers, E. A. (1975). Ivory and Slaves in East Central Africa: Changing Patterns of Internal Trade to the Late Nineteenth Century. London: Heinemann.
Alpers, E. A. (1992). “The ivory trade in Africa. An historical overview,” in Elephant; The Animal and its Ivory in African Culture, ed D. Ross (Los Angeles CA: University of California), 349–360.
Altmann, J., Alberts, S. C., Altmann, S. A., and Roy, S. B. (2002). Dramatic change in local climate patterns in the Amboseli basin, Kenya. Afr. J. Ecol. 40, 248–251. doi: 10.1046/j.1365-2028.2002.00366.x
Altmann, S. A., Post, D. G., and Klein, D. F. (1987). Nutrients and toxins of plants in Amboseli, Kenya. Afr. J. Ecol. 25, 279–293. doi: 10.1111/j.1365-2028.1987.tb01119.x
Ambrose, S. (1984). “The introduction of pastoral adaptations to the highlands of east africa,” in From Hunters to Farmers: The Causes and Consequences of Food Production in Africa, eds J. D. Clark and S. A. Brandt (Berkeley, CA: University of California Press), 212–239.
Ambrose, S. (1998). Chronology of the later stone age and food production in East Africa. J. Archaeol. Sci. 25, 377–392. doi: 10.1006/jasc.1997.0277
Ambrose, S. H., and Sikes, N. E. (1991). Soil carbon isotope evidence for Holocene habitat change in the Kenya Rift Valley. Science 253:1402. doi: 10.1126/science.253.5026.1402
Anderson, D. M. (2016). The beginning of time? Evidence for catastrophic drought in Baringo in the early nineteenth century. J. East Afr. Stud. 10, 45–66. doi: 10.1080/17531055.2015.1134532
Anderson, D. M., and Bollig, M. (2016). Resilience and collapse: histories, ecologies, conflicts and identities in the Baringo-Bogoria basin, Kenya. J. East Afr. Stud. 10, 1–20. doi: 10.1080/17531055.2016.1150240
Archibald, S., and Bond, W. J. (2004). Grazer movements: spatial and temporal responses to burning in a tallgrass African savannah. Int. J. Wildland Fire 13, 377–385. doi: 10.1071/WF03070
Ashley, C. Z., and Grillo, K. M. (2015). Archaeological ceramics from eastern Africa: past approaches and future directions. Azania 50, 460–480. doi: 10.1080/0067270X.2015.1102939
Ashley, G. M., Maitima-Mworia, J., Muasya, A. M., Owen, R. B., Driese, S. G., Hover, V. C., et al. (2004). Sedimentation and recent history of a freshwater wetland in a semi-arid environment: Loboi Swamp, Kenya, East Africa. Sedimentology 51, 1301–1321. doi: 10.1111/j.1365-3091.2004.00671.x
Ashley, G. M., Wet, C. B., Barboni, D., and Magill, C. R. (2016). Subtle signatures of seeps: record of groundwater in a dryland, DK, Olduvai Gorge, Tanzania. Depositional Rec. 2, 4–21. doi: 10.1002/dep2.11
Baker, H. G. (1991). The continuing evolution of weeds. Econ. Bot. 45, 445–449. doi: 10.1007/BF02930705
Bamber, R. N. (1982). Sodium hexametaphosphate as an aid in benthic sample sorting. Mar. Environ. Res. 7, 251–255. doi: 10.1016/0141-1136(82)90017-4
Barker, P. A., Hurrell, E. R., Leng, M. J., Plessen, B., Wolff, C., Conley, D. J., et al. (2013). Carbon cycling within an East African lake revealed by the carbon isotope composition of diatom silica: a 25-ka record from Lake Challa, Mt. Kilimanjaro. Quat Sci. Rev. 66, 55–63. doi: 10.1016/j.quascirev.2012.07.016
Beachey, R. W. (1967). The East African ivory trade in the nineteenth century. J. Afr. Hist. 8, 269–290. doi: 10.1017/S0021853700007052
Bernsten, J. L. (1976). The maasai and their neighbours: variables of interaction. Afr. Econ. Hist. 2, 1–11.
Bert, F. E., Rovere, S. L., Macal, C. M., North, M. J., and Podestá, G. P. (2014). Lessons from a comprehensive validation of an agent based-model: the experience of the Pampas Model of Argentinean agricultural systems. Ecol. Modell. 273, 284–298. doi: 10.1016/j.ecolmodel.2013.11.024
Birks, H., Lotter, A. F., Juggins, S., and Smol, J. P. (2012). Tracking Environmental Change Using Lake Sediments: Data Handling and Numerical Techniques, Vol. 5.: Springer Netherlands.
Blaauw, M., van Geel, B., Kristen, I., Plessen, B., Lyaruu, A., Engstrom, D. R., et al. (2010). High-resolution 14C dating of a 25,000-year lake-sediment record from equatorial East Africa. Quat. Sci. Rev. 30, 3043–3059. doi: 10.1016/j.quascirev.2011.07.014
Bodin, P., Olin, S., Pugh, T. A. M., and Arneth, A. (2016). Accounting for interannual variability in agricultural intensification: the potential of crop selection in Sub-Saharan Africa. Agric. Sys. 148, 159–168. doi: 10.1016/j.agsy.2016.07.012
Boivin, N., Crowther, A., Helm, R., and Fuller, D. Q. (2013). East Africa and Madagascar in the Indian Ocean world. J. World Prehist. 26, 213–281. doi: 10.1007/s10963-013-9067-4
Boivin, N., Crowther, A., Prendergast, M., and Fuller, D. Q. (2014). Indian Ocean food globalisation and Africa. Afr. Archaeol. Rev. 31, 547–581. doi: 10.1007/s10437-014-9173-4
Boivin, N., Zeder, M. A., Fuller, D. Q., Crowther, A., Larson, G., Erlandson, J. M., et al. (2016). Ecological consequences of human niche construction: examining long-term anthropogenic shaping of global species distributions. Proc. Natl. Acad. Sci. U.S.A. 113, 6388–6396. doi: 10.1073/pnas.1525200113
Boles, O. J. C., and Lane, P. J. (2016). The Green, Green Grass of Home: an archaeo-ecological approach to pastoralist settlement in central Kenya. Azania 51, 507–530. doi: 10.1080/0067270X.2016.1249587
Bollig, M. (2016). Adaptive cycles in the savannah: pastoral specialization and diversification in northern Kenya. J. East Afr. Stud. 10, 21–44. doi: 10.1080/17531055.2016.1141568
Bond, W. J., and Parr, C. L. (2010). Beyond the forest edge: ecology, diversity and conservation of the grassy biomes. Biol Cons. 143, 2395–2404. doi: 10.1016/j.biocon.2009.12.012
Bonnefille, R., and Riollet, G. (1988). The Kashiru pollen sequence (Burundi) palaeoclimatic implications for the last 40,000 yr. B.P. in tropical Africa. Quat Res. 30, 19–13. doi: 10.1016/0033-5894(88)90085-3
Boone, R., Galvin, K., BurnSilver, S., Thornton, P., Ojima, D., and Jawson, J. (2011). Using coupled simulation models to link pastoral decision making and ecosystem services. Ecol. Soc. 16:6. doi: 10.5751/ES-04035-160206
Bower, J. R. (1991). The Pastoral Neolithic of East Africa. J. World Prehis. 5, 49–82. doi: 10.1007/BF00974732
Bower, J. R. F., Nelson, C. M., Waibel, A. F., and Wandibba, S. (1977). The University of Massachusetts' later stone age/pastoral ‘Neolithic’ comparative study in Central Kenya: an overview. Azania 12, 119–146.
Bulte, E. H., Boone, R. B., Stringer, R., and Thornton, P. K. (2008). Elephants or onions? Paying for nature in Amboseli, Kenya. Environ. Dev. Econ. 13, 395–414. doi: 10.1017/S1355770X08004312
BurnSilver, S. B. (2009). “Pathways of continuity and change: maasai livelihoods in Amboseli, Kajiado District, Kenya,” in Staying Maasai?, eds K. Homewood, P. Kristjanson, and P. C. Trench (New York, NY: Springer), 161–207.
BurnSilver, S., and Mwangi, E. (2007). Beyond Group Ranch Subdivision: Collective Action for Livestock Mobility, Ecological Viability and Livelihoods. Washington, DC: International Food Policy Research Institute.
BurnSilver, S., Worden, J., and Boone, R. B. (2007). “Processes of fragmentation in the amboseli ecosystem, Southern Kajiado District, Kenya,” in Fragmentation of Semi-Arid and Arid Landscapes: Consequences for Human and Natural Systems, eds K. Galvin, R. Reid, R. Behnke, and T. Hobbs (New York, NY: Verlag Publishers), 225–254.
Bussmann, R. W. (2001). Succession and regeneration patterns of East African mountain forests: a review. Syst. Geogr. Plants. 1, 959–974. doi: 10.2307/3668731
Bussmann, R. W., and Beck, E. (1995a). The forests of Mount Kenya (Kenya), a phytosociological synopsis. Phytocoenologia 25, 467–560. doi: 10.1127/phyto/25/1995/467
Bussmann, R. W., and Beck, E. (1995b). Regeneration and succession processes in the Cedar-Forests (Juniperion procerae) of Mt. Kenya. Ecotropica 1, 79–84.
Butz, R. J. (2009). Traditional fire management: historical fire regimes and land use change in pastoral East Africa. Int. J. Wildland Fire 18, 442–450. doi: 10.1071/WF07067
Campbell, D. J., Lusch, D. P., Smucker, T. A., and Wangui, E. E. (2003). Land Use Change Impacts and Dynamics (LUCID). Project working paper 19. ILRI, Nairobi, Kenya.
Chuhila, M. J. (2016). Coming down the Mountain: History of Land Use Change in Kilimanjaro, ca. 1920 to 2000s. Ph.D. thesis, University of Warwick.
Coetzee, J. A. (1964). Evidence for a considerable depression of the vegetation belts during the Upper Pleistocene on the East African mountains. Nature 204, 564–566.
Coetzee, J. A. (1967). “Pollen analytical studies in East and Southern Africa,” in Palaeoecology of Africa and of the Surrounding Islands and Antarctica, ed E. M. van Zinderen Bakker (Amsterdam: CRC Press Taylor and Francis Group), 1–146.
Colombaroli, D., van der Plas, G., Rucina, S., and Verschuren, D. (in press). Determinants of savanna-fire dynamics in the eastern Lake Victoria catchment (western Kenya) during the last 1200 years. Quat Int. doi: 10.1016/j.quaint.2016.06.028
Courtney-Mustaphi, C. J., Shoemaker, A. C., Githumbi, E. N., Kariuki, R., Muriuki, R. M., Rucina, S., et al. (2015). Historical ecology perspectives of changes in Amboseli, Kenya. GLP 12, 26–29. Available online at: https://glp.earth/how-we-work/glp-newsletters/glp-newsletter-issue-no-12-november-2015
Coutu, A. N., Lee-Thorp, J., Collins, J. M., and Lane, P. J. (2016). Mapping the elephants of the 19th Century East African Ivory trade with a multi-isotope approach. PLoS ONE 11:e0163606. doi: 10.1371/journal.pone.0163606
Crosby, A. (2003) [1972]. The Columbian Exchange: Biological and Cultural Consequences of 1492. Westport, CN: Praeger.
Crowther, A., Prendergast, M. E., Fuller, D. Q., and Boivin, N. (in press). Subsistence mosaics, forager-farmer interactions, the transition to food production in eastern Africa. Quat. Int. doi: 10.1016/j.quaint.2017.01.014
Cutler, A. (1985). The Craft of Ivory: Sources, Techniques, and Uses in the Mediterranean World, AD 200–1400. Washington, DC: Dumbarton Oaks.
Dale, D., and Ashley, C. Z. (2010). Holocene hunter-fisher-gatherer communities: new perspectives on Kansyore-using communities of western Kenya. Azania 45, 24–48. doi: 10.1080/00672700903291716
Damnati, B. (2000). Holocene lake records in the Northern Hemisphere of Africa. J. Afr. Earth Sci. 31, 253–262. doi: 10.1016/S0899-5362(00)00089-0
Davies, M. I. J. (2015). Economic specialisation, resource variability, and the origins of intensive agriculture in Eastern Africa. Rural Landscapes Soc. Environ. Hist. 2, 1–18. doi: 10.16993/rl.af
DeBusk, G. H. (1998). A 37,500-year pollen record from Lake Malawi and implications for the biogeography of Afromontane forests. J. Biogeogr. 25, 479–500. doi: 10.1046/j.1365-2699.1998.2530479.x
De Langhe, E. (2007). “The establishment of traditional plantain cultivation in the african rain forest: a working hypothesis,” in Rethinking Agriculture: Archaeological and Ethnoarchaeological Perspectives, eds T. P. Denham, J. Iriate, and L. Vrydaghs (Walnut Creek: Left Coast Press), 361–370.
De Langhe, E., Swennen, R., and Vuylsteke, D. (1995). Plantain in the Early Bantu World. Azania 29, 147–160.
De Leeuw, P. N., and Tothill, J. C. (1990). The Concept of Rangeland Carrying Capacity in Sub-Saharan Africa: Myth or Reality. London: Overseas Development Institute, Pastoral Development Network.
Deocampo, D. M. (2002). Sedimentary structures generated by Hippopotamus amphibius in a lake-margin wetland, Ngoro Ngoro Crater, Tanzania. Palaios 17, 212–217. doi: 10.1669/0883-1351(2002)017<0212:SSGBHA>2.0.CO;2
Dresang, D. L., and Sharkansky, I. (1975). Sequences of Change and the Political Economy of public Corporations: Kenya. J. Polit. 37, 163–186. doi: 10.2307/2128895
Dunne, T., Dietrich, W. E., and Brunengo, M. J. (1978). Recent and past erosion rates in semi-arid Kenya. Z. Geomorphol. 29, 130–140.
Finch, J., and Marchant, R. (2011). A palaeoecological investigation into the role of fire and human activity in the development of montane grasslands in East Africa. Veg. Hist. Archaeobot. 20, 109–124. doi: 10.1007/s00334-010-0276-9
Finch, J., Marchant, R., and Courtney-Mustaphi, C. J. (2016). Ecosystem change in the South Pare Mountain bloc, Eastern Arc Mountains of Tanzania. Holocene 27, 796–810. doi: 10.1177/0959683616675937
Foley, R. (1981). Off-Site Archaeology and Human Adaptation in Eastern Africa: An Analysis of Regional Artefact Density in the Amboseli, Southern Kenya. Cambridge: Cambridge Monographs in African Archaeology 3. BAR International Series 97.
Fosbrooke, H. A., and Sassoon, H. (1965). Archaeological Remains on Kilimanjaro. Tanganyika Notes Records 64, 62–64.
Fries, R. E., and Fries, T. C. E. (1948). “Phytogeographical researches on Mt. Kenya and Mt. Aberdare, British East Africa” in Kungl. Svenska Vetenskapsakademiens Handlingar, Tbedje Serien (Stockholm: Band 25. No 5. Almqvist & Wiksellr Boktryckeri-a.-b), 1–83.
Fuller, D. Q., and Boivin, N. (2009). “Crops, cattle and commensals across the Indian Ocean: current and potential archaeobiological evidence,” in Plantes et Sociétés, Études océan Indien 42–43, ed G. Lefèvre (Paris: CEROI-INALCO), 3–46.
Fuller, D. Q., Boivin, N., Hoogervorst, T., and Allaby, R. (2011b). Across the Indian Ocean: the prehistoric movement of plants and animals. Antiquity 84, 544–558. doi: 10.1017/S0003598X00067934
Fuller, D. Q., and Hildebrand, E. A. (2013). “Domesticating plants in Africa,” in The Oxford Handbook of African Archaeology, eds P. Mitchell and P. J. Lane (Oxford: University of Oxford Press), 507–525.
Fuller, D. Q., van Etten, J., Manning, K., Castillo, C., Kingwell-Banham, E., Weisskopf, A., et al. (2011a). The contribution of rice agriculture and livestock pastoralism to prehistoric methane levels: an archaeological assessment. Holocene 21, 743–759. doi: 10.1177/0959683611398052
Galaty, J. G. (1993). “Maasai expansion and the New East African Pastoralism,” in Being Maasai: Ethnicity and Identity in East Africa, eds R. Spear and J. Waller (London: Currey), 61–86.
Garcin, Y., Melnick, D., Strecker, M. R., Olago, D., and Tiercelin, J. J. (2012). East African mid-Holocene wet–dry transition recorded in palaeo-shorelines of Lake Turkana, northern Kenya Rift. Earth Planet Sci. Lett. 331, 322–334. doi: 10.1016/j.epsl.2012.03.016
Gasse, F. (2000). Hydrological changes in the African tropics since the Last Glacial Maximum. Quat. Sci. Rev. 19, 189–211. doi: 10.1016/S0277-3791(99)00061-X
Gifford-Gonzalez, D. P. (1998). Early pastoralists in East Africa: ecological and social dimensions. J. Anthropol. Archaeol. 17, 166–200. doi: 10.1006/jaar.1998.0322
Gifford-Gonzalez, D. P. (2000). Animal disease challenges to the emergence of pastoralism in sub-Saharan Africa. Afr. Archaeol. Rev. 17, 95–139. doi: 10.1023/A:1006601020217
Gillson, L. (2004). Testing non-equilibrium theories in savannahs: 1400 years of vegetation change in Tsavo National Park, Kenya. Ecol. Comp. 1, 281–298. doi: 10.1016/j.ecocom.2004.06.001
Gillson, L. (2006). A ‘large infrequent disturbance’ in an East African savannah. Afr. J. Ecol. 44, 458–467. doi: 10.1111/j.1365-2028.2006.00662.x
Githumbi, E. (2017). Holocene Environmental and Human Interactions in East Africa. Unpublished Ph.D., thesis, University of York, Dordrecht.
Githumbi, E., Courtney-Mustaphi, C., and Marchant, R. (2016). Holocene ecosystem, social and landscape dynamics in East Africa. Quat. Int. 404, 199–200. doi: 10.1016/j.quaint.2015.08.175
Githumbi, E., Courtney-Mustaphi, C., Yun, K. J., Muiruri, V., Rucina, S. R., and Marchant, R. (2017). Late Holocene Wetland Transgression and 500 Years of Vegetation and Fire Variability in the Semi-Arid Amboseli Landscape, Southern Kenya. Dordrecht: AMBIO.
Glassman, J. (1995). Feasts and Riots: Revelry, Rebellion, and Popular Consciousness on the Swahili Coast, 1856–888. London: James Currey.
Goldman, M. J., and Riosmena, F. (2013). Adaptive capacity in Tanzanian Maasailand: changing strategies to cope with drought in fragmented landscapes. Glob. Environ. Chang. 23, 588–597. doi: 10.1016/j.gloenvcha.2013.02.010
Grandin, B. E. (1991). “The Maasai: socio-historical context and group Ranches,” in Maasai Herding – An Analysis of the Livestock Production System of Maasai Pastoralists in Eastern Kajiado District, Kenya, eds S. Bekure, P. N. de Leeuw, B. E. Grandin, and P. J. H. Neate (Addis Ababa: International Livestock Centre for Africa), 21–39.
Greiner, C., Alvarez, M., and Becker, M. (2013). From cattle to corn: attributes of emerging farming systems of former pastoral nomads in East Pokot, Kenya. Soc. Nat. Resour. 26, 1478–1490. doi: 10.1080/08941920.2013.791901
Greiner, C., and Mwaka, I. (2016). Agricultural change at the margins: adaptation and intensification in a Kenyan dryland. J. East Afr. Stud. 10, 130–149. doi: 10.1080/17531055.2015.1134488
Grimm, V., Berger, U., Bastiansen, F., Eliassen, S., Ginot, V., Giske, J., et al. (2005). A standard protocol for describing individual-based and agent-based models. Ecol. Model., 198, 115–126. doi: 10.1016/j.ecolmodel.2006.04.023
Hailegiorgis, A. B., Kennedy, W. G., Rouleau, M., Bassett, J. K., Coletti, M., Balan, G. C., et al. (2010). An Agent Based Model of Climate Change and Conflict among Pastoralists in East Africa. Doctoral dissertation, International Environmental Modelling and Software Society.
Håkansson, N. T. (2004). The human ecology of world systems in East Africa: the impact of the ivory trade. Hum. Ecol. 32, 561–591. doi: 10.1007/s10745-004-6097-7
Håkansson, N. T. (2008). “The decentralized landscape: regional wealth and the expansion of production in Northern Tanzania before the eve of colonialism,” in Economies and the Transformation of Landscape, eds L. Cliggett and C. A. Pool (Lanham: Altamira Press), 239–265.
Hamidu, A. S., Shirima, D. D., Courtney-Mustaphi, C. J., Marchant, R., and Munishi, P. K. T. (2017). The impact of land use and land cover change on biodiversity within and adjacent Kibasira Swamp in Kilombero valley, Tanzania. Afr. J. Ecol. doi: 10.1111/aje.12488. [Epub ahead of print].
Hamilton, A. C. (1976). Identification of East African Urticales Pollen. Pollen Et Spores 18, 27–66.
Hamilton, A. C. (1982). Environmental History of East Africa: A Study of the Quaternary. London: Academic Press.
Hamilton, A. C., and Perrott, R. (1980). “Modern pollen deposition on a tropical african mountain,” in ‘Pollen Et Spores,' Chapter Modern Pol, Vol. 22 (Amsterdam), 437–468.
Hamilton, A., Taylor, D., and Vogel, J. C. (1986). Early forest clearance and environmental degradation in south-west Uganda. Nature 320, 164–167. doi: 10.1038/320164a0
Hay, R. L., and Stoessell, R. K. (1984). Sepiolite in the Amboseli Basin of Kenya: a new interpretation. Dev. Sed. 37, 125–136. doi: 10.1016/S0070-4571(08)70032-3
Heckmann, M. (2014). Farmers, smelters and caravans: two thousand years of land use and soil erosion in North Pare, NE Tanzania. Catena 113, 187–201. doi: 10.1016/j.catena.2013.07.010
Heckmann, M., Muiruri, V., Boom, A., and Marchant, R. (2014). Human-environment interactions in an agricultural landscape: a 1400-yr. sediment and pollen record from North Pare, NE Tanzania. Palaeogeog. Palaeoclim. Palaeoecol. 406, 49–61 doi: 10.1016/j.palaeo.2014.04.005
Hedberg, O. (1951). Vegetation belts of the East African mountains. Svensk Botanisk Tidskrift. 45, 140–202.
Helm, R. (2000). Conflicting Histories: The Archaeology of Iron-Working, Farming Communities in the Central and Southern Coast Region of Kenya. Unpublished Ph.D., thesis, University of Bristol.
Hemp, A. (2006a). Continuum or zonation? Altitudinal gradients in the forest vegetation of Mt. Kilimanjaro. Plant Ecol. 184, 27–42. doi: 10.1007/s11258-005-9049-4
Hemp, A. (2006b). Vegetation of Kilimanjaro: hidden endemics and missing bamboo. Afr. J. Ecol. 44, 305–328. doi: 10.1111/j.1365-2028.2006.00679.x
Hemp, A., and Beck, E. (2001). Erica excelsa as a fire-tolerating component of Mt. Kilimanjaro's forests. Phytocoenologia 31, 449–475. doi: 10.1127/phyto/31/2001/449
Hobbs, R. J., Arico, S., Aronson, J., Baron, J. S., Bridgewater, P., Cramer, V. A., et al. (2006). Novel ecosystems: theoretical and management aspects of the new ecological world order. Global Ecol Biogeog. 15, 1–7. doi: 10.1111/j.1466-822X.2006.00212.x
Homewood, K., Kristjanson, P., and Trench, P. (2009). Staying Maasai: Livelihoods, Conservation and Development in East African rangelands, Vol. 5. New York, NY: Springer Science & Business Media.
Iles, L. (2016). The role of metallurgy in transforming global forests. J. Archaeol. Meth. Theory. 23, 1219–1241. doi: 10.1007/s10816-015-9266-7
Iles, L., Robertshaw, P., and Young, R. (2014). A furnace and associated iron working remains at Munsa, Uganda. Azania 49, 45–63. doi: 10.1080/0067270X.2013.877619
Jennings, C. C. (2003). “They called themselves Iloikop: rethinking pastoralist history in nineteenth-century East Africa,” in Sources and Methods in African History: Spoken, Written, Unearthed, eds T. Falola and C. C. Jennings (Rochester, NY: University of Rochester Press), 173–194.
Jolly, D., and Haxeltine, A. (1997). Effect of low glacial atmospheric CO2 on tropical African montane vegetation. Science 276, 786–788. doi: 10.1126/science.276.5313.786
Jönsson, A. M., Lagergren, F., and Smith, B. (2015). Forest management facing climate change-an ecosystem model analysis of adaptation strategies. Mitig. Adapt. Strat. Glob. Change 20, 201–220. doi: 10.1007/s11027-013-9487-6
Jowsey, P. C. (1966). An improved peat sampler. New Phytol. 65, 245–248. doi: 10.1111/j.1469-8137.1966.tb06356.x
Kamau, P. N., and Medley, K. E. (2014). Anthropogenic fires and local livelihoods at Chyulu Hills, Kenya. Landscape Urban Plan. 124, 76–84. doi: 10.1016/j.landurbplan.2014.01.010
Kenya National Bureau of Statistics (KNBS) (2010). Kenya 2009 Population and Housing Census Highlights KNBS Government of Kenya, Nairobi. Nairobi: Kenya National Bureau of Statistics.
Ker, D. (1955). Letter to the Editor – The East African Standard. Nairobi: The East African Standard.
Kiage, L. M., and Liu, K. B. (2006). Late Quaternary paleoenvironmental changes in East Africa: a review of multiproxy evidence from palynology, lake sediments, and associated records. Prog. Phys. Geog. 30, 633–658. doi: 10.1177/0309133306071146
Krapf, J. L. (1854). Vocabulary of the Engutuk Eloikob or the Wakuafi-Nation in the Interior of Equatorial East Africa. Tubingen: L. F. Fues.
Kunz, G. F. (1926). Ivory and the Elephant in Art, in Archaeology and in Science. New York, NY: Doubleday.
Kusimba, C. M. (2009). “Landscape at two scales: economy and trade in East Africa,” in Polities and Power: Archaeological Perspectives on the Landscapes of Early States, eds S. E. Falconer and C. L. Redman (Tucson: University of Arizona Press), 163–178.
Kusimba, C. M., and Kusimba, S. B. (2005). “Mosaics and Interactions: East Africa, 2000 B. P. to the Present,” in African Archaeology: A Critical Introduction, ed A. B. Stahl (Oxford: Blackwell), 392–419.
Kusimba, S. B. (1999). Hunter-gatherer land use patterns in later stone age East Africa. J. Anthrop. Archaeol. 18, 165–200. doi: 10.1006/jaar.1998.0335
Kusimba, S. B. (2001). The Pleistocene Later Stone Age in East Africa: excavations and lithic assemblages from Lukenya Hill. Afr. Archaeol. Rev. 18, 77–123. doi: 10.1023/A:1011032025300
Kusimba, S. B. (2013). “Hunter-gatherer-fishers of eastern and South-Central Africa since 20,000 years ago,” in The Oxford Handbook of African Archaeology, eds P. Mitchell and P. J. Lane (Oxford: Oxford University Press), 461–472.
Lamb, H., Darbyshire, I., and Verschuren, D. (2003). Vegetation response to rainfall variation and human impact in central Kenya during the past 1100 years. Holocene 13, 285–292. doi: 10.1191/0959683603hl618rr
Lane, P. J. (2004). The ‘moving frontier’ and the transition to food production in Kenya. Azania 39, 243–264. doi: 10.1080/00672700409480402
Lane, P. J. (2010). Developing landscape historical ecologies in Eastern Africa: an outline of current research and potential future directions. Afr. Stud. 69, 299–322. doi: 10.1080/00020184.2010.499203
Lane, P. J. (2011a). An outline of the later Holocene archaeology and pre-colonial history of the Ewaso Basin, Kenya. Smithson. Contrib. Zool. 632, 11–30. doi: 10.5479/si.00810282.632.11
Lane, P. J. (2011b). “Slavery and slave trading in Eastern Africa: exploring the intersections of historical sources and archaeological evidence,” in Slavery in Africa: Archaeology and Memory, eds P. J. Lane and K. C. MacDonald (London: Oxford University Press), 281–314.
Lane, P. J. (2013). “Trajectories to pastoralism in northern and central Kenya: an overview of the archaeological and environmental evidence,” in Pastoralism in Africa: Past Present and Future, eds M. Bollig and H. P. Wotzka (Oxford: Berghahn Books), 104–143.
Lane, P. J., Ashley, C., and Oteyo, G. (2006). New dates from Kansyore and Urewe wares from northern Nyanza, Kenya. Azania. 41, 123–138. doi: 10.1080/00672700609480438
Lane, P. J., Seitsonen, O., Harvey, P., Mire, S., and Odede, F. (2007). The transition to farming in eastern Africa: new faunal and dating evidence from Wadh Lang'o and Usenge, Kenya. Antiquity 81, 62–81. doi: 10.1017/S0003598X00094849
Laws, R. M. (1968). Interactions between elephant and Hippopotamus populations and their environments. East Afr. Agric. For. 33, 140–147.
Lee, R. B. (1979). The !Kung San: Men, Women, and Work in a Foraging Society. Cambridge: Cambridge University Press.
Lehsten, V., Arneth, A., Spessa, A., Thonicke, K., and Moustakas, A. (2016). The effect of fire on tree–grass coexistence in savannahs: a simulation study. Int. J. Wildland Fire 25, 137–146. doi: 10.1071/WF14205
Lejju, B. J., Robertshaw, P., and Taylor, D. (2003). Vegetation history and archaeology at Munsa, western Uganda. Azania 38, 155–165. doi: 10.1080/00672700309480363
Lejju, B. J., Taylor, D., and Robertshaw, P. (2005). Late-Holocene environmental variability at Munsa archaeological site, Uganda: a multicore, multiproxy approach. Holocene 15, 1044–1061. doi: 10.1191/0959683605hl877ra
Lindeskog, M., Arneth, A., Bondeau, A., Waha, K., Seaquist, J., Olin, S., et al. (2013). Implications of accounting for land use in simulations of ecosystem carbon cycling in Africa. Earth Syst. Dyn. 4, 385–407. doi: 10.5194/esd-4-385-2013
Lindsay, W. K. (1989). “Integrating parks and pastoralists: some lessons from Amboseli,” in Conservation in Africa: People, Policies and Practice, eds D. Anderson and R. Grove (Cambridge: Cambridge University Press), 149–167.
Liutkus, C. M., and Ashley, G. M. (2003). Facies model of a semiarid freshwater wetland, Olduvai Gorge, Tanzania. J. Sed. Res. 73, 691–705. doi: 10.1306/021303730691
Liutkus, C. M., Wright, J. D., Ashley, G. M., and Sikes, N. E. (2005). Paleoenvironmental interpretation of lake-margin deposits using δ13C and δ18O results from early Pleistocene carbonate rhizoliths, Olduvai Gorge, Tanzania. Geology 33, 377–380. doi: 10.1130/G21132.1
Livingstone, D. A. (1962). Age of deglaciation in the ruwenzori range, Uganda. Nature 194, 859–860. doi: 10.1038/194859b0
Livingstone, D. A. (1967). Postglacial vegetation of the Ruwenzori Mountains in equatorial Africa. Ecol. Monogr. 37, 25–52. doi: 10.2307/1948481
Mackinder, H. J. (1900). A journey to the summit of Mount Kenya, British East Africa. Geogr. J. 15, 453–476. doi: 10.2307/1774261
Magill, C. R., Ashley, G. M., and Freeman, K. H. (2012a). Ecosystem variability and early human habitats in eastern Africa. Proc. Natl Acad. Sci. U.S.A. 110, 1167–1174. doi: 10.1073/pnas.1206276110
Magill, C. R., Ashley, G. M., and Freeman, K. H. (2012b). Water, plants, and early human habitats in eastern Africa. Proc. Natl Acad. Sci. U.S.A. 110, 1175–1180. doi: 10.1073/pnas.1209405109
Marchant, R. A., and Hooghiemstra, H. (2004). Rapid environmental change in African and South American tropics around 4000 years before present: a review. Earth Sci. Rev. 66, 217–260. doi: 10.1016/j.earscirev.2004.01.003
Marean, C. W. (1992). Hunter to Herder: large mammal remains from the Hunter-gatherer Occupation at Enkapune ya Muto Rock-shelter. Afr. Archaeol. Rev. 10, 65–127. doi: 10.1007/BF01117697
Marlowe, F. W., and Berbesque, J. C. (2009). Tubers as fallback foods and their impact on Hadza hunter-gatherers. Am. J. Phys. Anthrop. 140, 751–758. doi: 10.1002/ajpa.21040
Marshall, F. (1990). Origins of specialized pastoral production in East Africa. Am. Anthrop. 92, 873–894. doi: 10.1525/aa.1990.92.4.02a00020
Marshall, F. (1994). “Archaeological perspectives on East African pastoralism,” in African Pastoralist Systems, eds E. Fratkin, J. Galvin and E. Roth (Boulder: Lynne-Reinner), 17–44.
Marshall, F. (2001). Agriculture and use of wild and weedy greens by the Piik ap Oom Okiek of Kenya. Econ. Bot. 55, 32–46. doi: 10.1007/BF02864544
Matthews, R. B., and Bakam, I. (2007). “A combined agent-based and biophysical modelling approach to address GHG mitigation policy issues,” in MODSIM International Congress on Modelling and Simulation (Christchurch).
Matthews, R. B., Gilbert, N. G., Roach, A., Polhill, J. G., and Gotts, N. M. (2007). Agent-based land-use models: a review of applications. Landsc. Ecol. 22, 1447–1459. doi: 10.1007/s10980-007-9135-1
McCabe, J. T., Leslie, P. W., and DeLuca, L. (2010). Adopting cultivation to remain pastoralists: the diversification of Maasai livelihoods in northern Tanzania. Hum. Ecol. 38, 321–334. doi: 10.1007/s10745-010-9312-8
McCarthy, T. S., Ellery, W. N., and Bloem, A. (1998). Some observations on the geomorphological impact of hippopotamus (Hippopotamus amphibius L.) in the Okavango Delta, Botswana. Afr. J. Ecol. 36, 44–56. doi: 10.1046/j.1365-2028.1998.89-89089.x
Mehlman, M. J. (1979). Mumba Hohle Revisited: the relevance of a forgotten excavation to some current issues in East African prehistory. World Archaeol. 11, 80–94. doi: 10.1080/00438243.1979.9979751
Meijerink, A. M. J., and Van Wijngaarden, W. (1997). “Contribution to the groundwater hydrology of the Amboseli ecosystem, Kenya,” in Groundwater/Surface Water Ecotones: Biological and Hydrological Interactions and Management Options, eds J. Gilbert, J. Mathieu, and F. Fournier (Cambridge: Cambridge University Press), 111–118.
Milton, S. J. (2003). ‘Emerging ecosystems’: a washing-stone for ecologists, economists and sociologists? S. Afr. J. Sci. 99, 404–406. Available online at: https://hdl.handle.net/10520/EJC97706
Moernaut, J., Verschuren, D., Charlet, F., Kristen, I., Fagot, M., and De Batist, M. (2010). The seismic-stratigraphic record of lake-level fluctuations in Lake Challa: hydrological stability and change in equatorial East Africa over the last 140kyr. Earth Plan Sci Lett. 290, 214–223. doi: 10.1016/j.epsl.2009.12.023
Moore, P. D., Webb, J. A., and Collison, M. E. (1991). Pollen Analysis. Oxford: Blackwell publishers.
Morrison, M. E. S. (1968). Vegetation and climate in the uplands of southwestern Uganda during the Later Pleistocene Period, 1. Muchoya Swamp, Kigezi District. J. Ecol. 56, 363–384. doi: 10.2307/2258239
Morrison, M. E. S., and Hamilton, A. C. (1974). Vegetation and climate in the uplands of southwestern Uganda during the later Pleistocene Period: II. Forest clearance and other vegetational changes in the Rukiga Highlands during the past 8000 Years. Ecology 62, 1–31.
Mount, J. F., and Cohen, A. S. (1984). Petrology and geochemistry of rhizoliths from Plio-Pleistocene fluvial and marginal lacustrine deposits, east Lake Turkana, Kenya. J. Sed. Res. 54, 263–275.
Mturi, A. A. (1986). The Pastoral Neolithic of West Kilimanjaro. Azania 21, 53–63. doi: 10.1080/00672708609511367
Muchiru, A. N., Western, D., and Reid, R. S. (2009). The impact of abandoned pastoral settlements on plant and nutrient succession in an African savanna ecosystem. J. Arid. Environ. 73, 322–331. doi: 10.1016/j.jaridenv.2008.09.018
Muiruri, V. M. (2008). Detecting Environmental Change and Anthropogenic Activities on the Laikipia Plateau, Kenya. Master's degree, Earth Sciences Department, Palynology Section, National Museums of Kenya, Nairobi, and Department of Botany, University of Free State South Africa.
Murray-Rust, D. H. (1972). Soil erosion and reservoir sedimentation in a grazing area west of Arusha, northern Tanzania. Geografiska Ann. Series A. Phys. Geog. 54, 325–343. doi: 10.1080/04353676.1972.11879872
Mwangi, E. (2006). The footprints of history: path dependence in the transformation of property rights in Kenya's Maasailand. J. Inst. Ecol. 2, 157–180. doi: 10.1017/S1744137406000324
Mwangi, E., and Ostrom, E. (2009). “A century of institutions and ecology in East Africa's rangelands: linking institutional robustness with the ecological resilience of Kenya's maasailand,” in Institutions and Sustainability: Political Economy of Agriculture and the Environment - Essays in Honour of Konrad Hagedorn, eds V. Beckmann and M. Padmanabhan (Dordrecht: Springer).
Mworia-Maitima, J. (1997). Prehistoric fires and land-cover change in western Kenya: evidences from pollen, charcoal, grass cuticles and grass phytoliths. Holocene 7, 409–417. doi: 10.1177/095968369700700404
Nelson, D. M., Verschuren, D., Urban, M. A., and Hu, F. S. (2012). Long-term variability and rainfall control of savannah fire regimes in equatorial East Africa. Glob. Change Biol. 18, 3160–3170. doi: 10.1111/j.1365-2486.2012.02766.x
Nicholson, S. E., and Yin, X. (2001). Rainfall conditions in equatorial East Africa during the nineteenth century as inferred from the record of Lake Victoria. Clim. Change 48, 387–398. doi: 10.1023/A:1010736008362
Nkedianye, D., Radeny, M., Kristjanson, P., and Herrero, M. (2009). “Assessing returns to land and changing livelihood strategies in Kitengela,” in Staying Maasai?, eds K. Homewood P. Kristjanson, and P. C. Trench (New York, NY: Springer), 115–149.
Norman, R. (2010). Kimana Wetlands Spring and Water Use Inventory. Nairobi: African Wildlife Institution.
Odner, K. (1971). A preliminary report on an archaeological survey on the slopes of Kilimanjaro. Azania 6, 131–149. doi: 10.1080/00672707109511549
Ofcansky, T. P. (1981). The 1889–1897 rinderpest epidemic and the rise of British and German colonialism in eastern and southern Africa. J. Afr. Stud. 8, 31–38.
Okello, M. M. (2005). Land use changes and human–wildlife conflicts in the Amboseli Area, Kenya. Hum. Dim. Wildlife. 10, 19–28. doi: 10.1080/10871200590904851
Okello, M. M., and D'Amour, D. E. (2008). Agricultural expansion within Kimana electric fences and implications for natural resource conservation around Amboseli National Park, Kenya. J. Arid. Environ. 72, 2179–2192. doi: 10.1016/j.jaridenv.2008.07.008
Okello, M. M., and Kioko, J. M. (2011). A field study in the status and threats of cultivation in Kimana and Ilchalai swamps in Amboseli dispersal area, Kenya. Nat. Resour. 2, 197–211. doi: 10.4236/nr.2011.24026
Okello, M. M., Kiringe, J. W., Njumbi, S. J., and Isiche, J. (2016). Prevalence of human elephant conflicts in Amboseli ecosystem, Kenya: current opinions of local community. Int. J. Biodivers. Conserv. 8, 60–71. doi: 10.5897/IJBC2015.0865
Oliver, R. (1966). The problem of the Bantu expansion. J. Afr. Hist. 7, 361–376. doi: 10.1017/S0021853700006472
Osmaston, H. A. (1958). Pollen Analysis in the Study of the Past Vegetation and Climate of Ruwenzori and its Neighbourhood. Unpublished BSc., thesis, Oxford University.
Osmaston, H. A. (1965). The Past and Present Climate and Vegetation of the Rwenzori and its Neighbourhood. Unpublished DPhil, thesis, University of Oxford, Oxford, UK.
Overton, J. D. (1989). Social control and social engineering: African Reserves in Kenya 1895–1920. Environ. Plann D Soc. Space 8, 163–174.
Pachzelt, A., Rammig, A., Higgins, S., and Hickler, T. (2013). Coupling a physiological grazer population model with a generalized model for vegetation dynamics. Ecol. Model. 263, 92–102. doi: 10.1016/j.ecolmodel.2013.04.025
Payton, R. W., Christiansson, C., Shishira, E. K., Yanda, P., and Eriksson, M. G. (1992). Landform, soils and erosion in the northeastern Irangi Hills, Kondoa, Tanzania. Geografiska Anna. Series A. Phys Geog. 74, 65–79. doi: 10.1080/04353676.1992.11880351
Perrott, R. A. (1987). Early forest clearance and the environment in south-west Uganda. Nature 325, 89–90. doi: 10.1038/325089b0
Petek, N., and Lane, P. (2016). Ethnogenesis and surplus food production: communitas and identity building among nineteenth- and early twentieth-century Ilchamus, Lake Baringo, Kenya. World Archaeol. 49, 40–60. doi: 10.1080/00438243.2016.1259583
Pickford, M. (1986). “Sedimentation and fossil preservation in the Nyanza Rift System, Kenya,” in Sedimentation in the African Rifts, eds L. E. Frostick, R. W. Renaut, I. Reid, and J. J. Tiercelin (Oxford: Blackwell Scientific Publications), 339–363.
Pigati, J. S., Rech, J. A., Quade, J., and Bright, J. (2014). Desert wetlands in the geologic record. Earth Sci. Rev. 132, 67–81. doi: 10.1016/j.earscirev.2014.02.001
Platts, P. J., Gereau, R. E., Burgess, N. D., and Marchant, R. (2013). Spatial heterogeneity of climate change in an Afromontane centre of endemism. Ecography 36, 518–530. doi: 10.1111/j.1600-0587.2012.07805.x
Platts, P. J., Omeny, P., and Marchant, R. (2015). AFRICLIM: high-resolution climate projections for ecological applications in Africa. Afr. J. Ecol. 53, 103–108. doi: 10.1111/aje.12180
Poesen, J. W. A., Torri, D. B., and Vanwalleghem, T. (2010). “Gully erosion: procedures to adopt when modelling soil erosion,” in Landscapes Affected by Gullying, eds R. P. C. Morgan and M. A. Nearing (Chichester: John Wiley & Sons), 360–386.
Prendergast, M. E., and Beyin, A. (in press). Fishing in a fluctuating landscape: terminal Pleistocene early Holocene subsistence strategies in the Lake Turkana Basin, Kenya. Quat Int. doi: 10.1016/j.quaint.2017.04.022
Prendergast, M. E., and Lane, P. J. (2010). Middle Holocene Fishing Strategies in East Africa: zooarchaeological analysis of Pundo, a Kansyore shell midden in northern Nyanza (Kenya). Int. J. Osteoarchaeol. 20, 88–112. doi: 10.1002/oa.1014
Prendergast, M., Quintana Morales, E., Crowther, A., Horton, M. C., and Boivin, N. (2017). Dietary diversity on the Swahili coast: the fauna from two Zanzibar trading locales. Int. J. Osteoarchaeol. 27, 621–637. doi: 10.1002/oa.2585
Quillet, A., Peng, C., and Garneau, M. (2010). Toward dynamic global vegetation models for simulating vegetation–climate interactions and feedbacks: recent developments, limitations, and future challenges. Environ. Rev. 18, 333–353. doi: 10.1139/A10-016
Rapp, A., Axelsson, V., Berry, L., and Murray-Rust, D. H. (1972). Soil erosion and sediment transport in the Morogoro River catchment, Tanzania. Geografiska Ann. Series A. Phys. Geog. 54, 125–155. doi: 10.1080/04353676.1972.11879863
Rebmann, J. (1848). “Tagebuch des missionars vom 14 February 1848-16,” in Veröffentlichungen des Archivs der Stadt Gerlingen: Band 3 (Gerlingen: Herausgegeben vom Stadtarchiv Gerlingen), 1997.
Reid, R. S., and Ellis, J. E. (1995). Impacts of pastoralists on woodlands in South Turkana, Kenya: livestock-mediated tree recruitment. Ecol. Appl. 5, 978–992. doi: 10.2307/2269349
Reid, R. S., Fernández-Giménez, M. E., and Galvin, K. A. (2014). Dynamics and resilience of rangelands and pastoral peoples around the globe. Ann. Rev. Environ. Resour. 39, 217–242. doi: 10.1146/annurev-environ-020713-163329
Reimer, P. J., Bard, E., Bayliss, A., Beck, J. W., Blackwell, P. G., Ramsey, C. B., et al. (2013). IntCal13 and Marine13 Radiocarbon Age Calibration Curves 0–50,000 Years cal BP. Radiocarbon. 55, 1869–1887. doi: 10.2458/azu_js_rc.55.16947
Ricketts, R. D., and Johnson, T. C. (1996). Climate change in the Turkana basin as deduced from a 4000-year long delta 0–18 record. Earth Planet Sci. Lett. 142, 7–17. doi: 10.1016/0012-821X(96)00094-5
Robbins, L. (1972). Archaeology in the Turkana District, Kenya. Science 176, 359–366. doi: 10.1126/science.176.4033.359
Robertshaw, P. (1997). Munsa earthworks: a preliminary report on recent excavations. Azania 32, 1–20. doi: 10.1080/00672709709511585
Robertshaw, P. T. (1990). “Early Pastoralists and their Herds in the Loita-Mara Region,” in Early Pastoralists of Southwestern Kenya, ed P. T. Robertshaw (Nairobi: British Institute in Eastern Africa), 293–302.
Robertshaw, P. T., and Collett, D. T. (1983). The identification of pastoral peoples in the archaeological record: an example from East Africa. World Archaeol. 15, 67–78. doi: 10.1080/00438243.1983.9979885
Rounsevell, M. D. A., Robinson, D. T., and Murray-Rust, D. (2012). From actors to agents in socio-ecological systems models. Philos. Trans. R. Soc. B 367, 259–269. doi: 10.1098/rstb.2011.0187
Rucina, S. M., Muiruri, V. M., Downton, L., and Marchant, R. (2010). Late-Holocene savannah dynamics in the Amboseli Basin, Kenya. Holocene 20, 667–677. doi: 10.1177/0959683609358910
Russell, J. M., and Johnson, T. C. (2005). A high-resolution geochemical record from Lake Edwards, Uganda Congo and the timing and causes of tropical African drought during the late Holocene. Quat. Sci. Rev. 24, 1375–1389. doi: 10.1016/j.quascirev.2004.10.003
Rutten, M. M. E. M. (1992). Selling Wealth to Buy Poverty: The Process of Individualization of Land Ownership among the Maasai Pastoralists of Kajiado District, Kenya. Saarbrücken: Verlag Breitenbach Publishers.
Scheiter, S., Langan, L., and Higgins, S. I. (2013). Next-generation dynamic global vegetation models: learning from community ecology. New Phytol. 198, 957–969. doi: 10.1111/nph.12210
Schindler, J. (2013). About the uncertainties in model design and their effects: an illustration with a land-use model. J. Artif. Soc. Soc. Simul. 16:6. doi: 10.18564/jasss.2274
Schlachter, K. J., and Horn, S. P. (2010). Sample preparation methods and replicability in macroscopic charcoal analyses. J. Paleolim. 44, 701–708. doi: 10.1007/s10933-009-9305-z
Schlesinger, W. H., Reynolds, J. F., Cunningham, G. L., Huenneke, L. K., Jarrell, W. M., Virginia, R. A., et al. (1990). Biological feedbacks in global desertification. Science 247, 1043–1048. doi: 10.1126/science.247.4946.1043
Schüler, L., Hemp, A., Zech, W., and Behling, H. (2012). Vegetation, climate and fire-dynamics in East Africa inferred from the Maundi crater pollen record from Mt Kilimanjaro during the last glacial–interglacial cycle. Quat. Sci. Rev. 39, 1–13. doi: 10.1016/j.quascirev.2012.02.003
Seno, S. K., and Shaw, W. W. (2002). Land tenure policies, Maasai traditions, and wildlife conservation in Kenya. Soc. Nat. Resour. 15, 79–88. doi: 10.1080/089419202317174039
Seno, S. K., and Tome, S. (2013). Socio-economic and ecological viability of pastoralism in Loitokitok District, Southern Kenya. Nomadic Pple. 17, 66–86. doi: 10.3167/np.2013.170104
Shalem, A. (2005). “Trade in and the availability of ivory: the picture given by the medieval sources,” in The ivories of Muslim Spain: Papers from a Symposium Held in Copenhagen from the 18th to the 20th of November 2003, eds K. von Folsach and J. Meyer (Copenhagen: The David Collection), 25–36.
Sheriff, A. (1987). Slaves, Spices and Ivory in Zanzibar: Integration of an East African Commercial Empire into the World Economy 1770–1873. London: James Currey.
Sinclair, A. R. E., and Fryxell, J. M. (1985). The Sahel of Africa: ecology of a disaster. Can. J. Zool. 63, 987–994. doi: 10.1139/z85-147
Smith, B., Prentice, I. C., and Sykes, M. T. (2001). Representation of vegetation dynamics in the modelling of terrestrial ecosystems: comparing two contrasting approaches within European climate space. Global Ecol. Biogeog. 10, 621–637. doi: 10.1046/j.1466-822X.2001.00256.x
Sobania, N. P. (1980). The Historical Traditions of the Peoples of the Eastern Lake Turkana Basin c.1840–1925. Ph.D., thesis, SOAS, University of London.
Soper, R. (1976). Archaeological Sites in the Chyulu Hills, Kenya. Azania 11, 83–116. doi: 10.1080/00672707609511232
Spencer, I. (1983). “Pastoralism and Colonial Policy in Kenya, 1895-1929,” in Imperialism, Colonialism, and Hunger: East and Central Africa, ed R. Rotberg (Lexington: D.C. Heath), 113–140.
Stager, J. C., and Johnson, T. C. (2008). The late Pleistocene desiccation of Lake Victoria and the origin of its endemic biota. Hydrobiologia 596, 5–16. doi: 10.1007/s10750-007-9158-2
Stockmarr, J. (1971). Tablets with spores used in absolute pollen analysis. Pollen Spores 13, 615–621.
Stoessell, R. K., and Hay, R. L. (1978). The geochemical origin of sepiolite and kerolite at Amboseli, Kenya. Contrib. Mineral. Pet. 65, 255–267. doi: 10.1007/BF00375511
Stump, D., and Tagseth, M. (2009). “The history of pre-colonial and early colonial agriculture on Kilimanjaro: a review,” in Culture, History and Identity: Landscapes of Inhabitation in the Mount Kilimanjaro Area, Tanzania, BAR International Series 1966, ed T. Clack (Oxford: Archaeopress), 107–124.
Sundstrom, S., Tynon, J. F., and Western, D. (2012). Rangeland privatization and the Maasai experience: social capital and the implications for traditional resource management in Southern Kenya. Soc. Nat. Resour. 25, 483–498. doi: 10.1080/08941920.2011.580420
Swynnerton, R. J. M. (1955). A Plan to Intensify the Development of African Agriculture in Kenya, Government Printer. Nairobi: Colony and Protectorate of Kenya.
Taylor, D., Lane, P. J., Muiruri, V., Ruttledge, A., Gaj McKeever, R., Nolan, T., et al. (2005). Mid-to late-Holocene vegetation dynamics on the Laikipia Plateau, Kenya. Holocene 15, 837–846. doi: 10.1191/0959683605hl857ra
Thompson, L. G., Mosley-Thompson, E., Davis, M. E., Henderson, K. A., Brecher, H. H., Zagorodnov, V. S., et al. (2002). Kilimanjaro ice core records: evidence of Holocene climate change in tropical Africa. Science 298, 598–593. doi: 10.1126/science.1073198
Tierney, J. E., Russell, J. M., Damsté, J. S. S., Huang, Y., and Verschuren, D. (2011). Late Quaternary behaviour of the East African monsoon and the importance of the Congo Air Boundary. Quat. Sci. Rev. 30, 798–807. doi: 10.1016/j.quascirev.2011.01.017
Touber, L., van der Pouw, B. J., and van Engelen, V. W. (1983). Soils and Vegetation of the Amboseli - Kibwezi area. Reconnaissance Soil Survey Report R6. Kenya Soil Survey, Nairobi.
United Nations, Department of Economic and Social Affairs, Population Division. (2017). World Population Prospects: The 2017 Revision, Key Findings and Advance Tables. Working Paper No. ESA/P/WP/248.
Valbuena, D., Verburg, P. H., Bregt, A. K., and Ligtenberg, A. (2010). An agent-based approach to model land–use change at a regional scale. Landscape Ecol. 25, 185–199. doi: 10.1007/s10980-009-9380-6
Van Zinderen, B. E. M. (1962). A late-glacial and postglacial climatic correlation between East Africa and Europe. Nature 194, 201–203. doi: 10.1038/194201a0
Van Zinderen, B. E. M., and Coetzee, J. A. (1988). A review of Late Quaternary pollen studies in East, Central and Southern Africa. Rev. Palaeobot. Palynol. 55, 155–174. doi: 10.1016/0034-6667(88)90083-8
Verschuren, D., and Charman, D. (2008). “Latitudinal linkages in late-holocene moisture-balance variation,” in Natural Climate Variability and Global Warming, eds R. W. Battarbee and H. A. Binney (Oxford: Wiley-Blackwell), 189–231.
Verschuren, D., Laird, K. R., and Cumming, B. F. (2000). Rainfall and drought in equatorial East Africa during the past 1,100 years. Nature 403, 410–414. doi: 10.1038/35000179
Von der Decken, C. C. (1869). Reisen in Ost-Afrika in den Jahren 1859 bis 1865. Leipzig: C. F. Winter'sche Verlagshandlung.
Waller, R. (1979). The Lords of East Africa: The Maasai in the mid-Nineteenth Century, c1840–1885. Ph.D., thesis, Cambridge.
Waller, R. (1988). ‘Emutai': crisis and response in Maasailand, 1883–1902,” in Ecology of Survival, eds D. Johnson and D. M. Anderson (Boulder: Westview), 73–113.
Walz, J. R. (2010). Route to a Regional Past: An Archaeology of the Lower Pangani (Ruvu) Basin, Tanzania, 500–1900 C. E. Unpublished Ph.D., thesis, University of Florida.
Wandibba, S. (1980). “The application of attribute analysis to the study of Later Stone Age/Neolithic pottery ceramics in Kenya,” in Proceedings of the Eighth Panafrican Congress of Prehistory and Quaternary Studies, Nairobi, eds R. E. Leakey and B. Ogot (Nairobi: The International Louis Leakey Memorial Institute for African Prehistory), 283–285.
Weissbrod, L. (2010). The Small Animals of Maasai Settlements: Ethnoarchaeological Investigations of the Commensalism Model. Unpublished Ph.D., dissertation, Washington University.
Western, D. (2006). A half a century of habitat change in Amboseli National Park, Kenya. Afr. J. Ecol. 45, 302–310. doi: 10.1111/j.1365-2028.2006.00710.x
Western, D., and Dunne, T. (1979). Environmental aspects of settlement site decisions among pastoral Maasai. Hum. Ecol. 7, 75–97. doi: 10.1007/BF00889353
Western, D., and Finch, V. (1986). Cattle and pastoralism: survival and production in arid lands. Hum. Ecol. 14, 77–94. doi: 10.1007/BF00889211
Western, D., and Maitumo, D. (2004). Woodland loss and restoration in a savannah park: a 20-year experiment. Afr. J. Ecol. 42, 111–121. doi: 10.1111/j.1365-2028.2004.00506.x
Western, D., and Manzolillo-Nightingale, D. L. (2003). Environmental Change and the Vulnerability of Pastoralists to Drought: A Case Study of the Maasai in Amboseli, Kenya. New York, NY: Cambridge University Press.
Western, D., and Van Praet, C. (1973). Cyclical Changes in the Habitat and Climate of an East African Ecosystem. Nature 241, 104–106. doi: 10.1038/241104a0
Whitlock, C., Higuera, P., McWethy, D. B., and Briles, C. E. (2010). Paleoecological perspectives on fire ecology: revisiting the fire-regime concept. Open Ecol. 3, 6–23. doi: 10.2174/1874213001003020006
Wilensky, U. (1999). NetLogo. Evanston, IL: Center for Connected Learning and Computer-Based Modeling, Northwestern University. Available online at: http://ccl.northwestern.edu/netlogo/
Williams, L. A. J. (1972). Geology of the Amboseli Area, Vol. 90. Nairobi: Geological Survey of Kenya, Ministry of Natural Resources, Republic of Kenya.
Wimmelbücker, L. (2009). “Local memories of famine,” in Culture, History, and Identity: Landscapes of Inhabitation in the Mount Kilimanjaro Area, Tanzania: Essays in Honour of Paramount Chief Thomas Lenana Mlanga Marealle II (1915–2007), ed T. Clack (Oxford: BAR International), 171–193.
Worden, J., Reid, R., and Gichohi, H. (2003). Land-Use Impacts on Large Wildlife and Livestock in the Swamps of the Greater Amboseli Ecosystem, Kajiado District, Kenya - Part 1, Text and Tables. LUCID working paper series number 27.
Wright, D. K. (2005). New perspectives on early regional interaction networks of East African traded; a view from Tsavo National Park, Kenya. Afr. Archaeol. Rev. 22, 111–140. doi: 10.1007/s10437-005-8041-7
Wright, D. K. (2007). Tethered mobility and riparian resource exploitation among Neolithic hunters and herders in the Galana River basin, Kenyan coastal lowlands. Environ. Archaeol. 12, 25–47. doi: 10.1179/174963107x172732
Zech, M. (2006). Evidence for Late Pleistocene climate changes from buried soils on the southern slopes of Mt. Kilimanjaro, Tanzania. Palaeogeog. Palaeoclim. Palaeoecol. 242, 303–312. doi: 10.1016/j.palaeo.2006.06.008
Keywords: Africa, groundwater, land cover, land use, paleovegetation, protected areas, vegetation, wetlands
Citation: Githumbi EN, Kariuki R, Shoemaker A, Courtney-Mustaphi CJ, Chuhilla M, Richer S, Lane P and Marchant R (2018) Pollen, People and Place: Multidisciplinary Perspectives on Ecosystem Change at Amboseli, Kenya. Front. Earth Sci. 5:113. doi: 10.3389/feart.2017.00113
Received: 28 September 2017; Accepted: 26 December 2017;
Published: 25 January 2018.
Edited by:
Encarni Montoya, Instituto de Ciencias de la Tierra Jaume Almera (CSIC), SpainReviewed by:
Charlotte Sarah Miller, Zentrum für Marine Umweltwissenschaften, Universität Bremen, GermanyAlberto Saez, University of Barcelona, Spain
Copyright © 2018 Githumbi, Kariuki, Shoemaker, Courtney-Mustaphi, Chuhilla, Richer, Lane and Marchant. This is an open-access article distributed under the terms of the Creative Commons Attribution License (CC BY). The use, distribution or reproduction in other forums is permitted, provided the original author(s) or licensor are credited and that the original publication in this journal is cited, in accordance with accepted academic practice. No use, distribution or reproduction is permitted which does not comply with these terms.
*Correspondence: Esther N. Githumbi, ZXN0aGVyZ2l0aHVtYmlAZ21haWwuY29t