- Geosciences and Environmental Change Science Center, U.S. Geological Survey, Denver, CO, United States
The Cordilleran Ice Sheet covered most of southeastern Alaska during the Last Glacial Interval (LGI: Marine Isotope Stage 2). Ice began to recede from western Alexander Archipelago ∼17,000 ± 700 yr BP. In this study, pollen analysis and radiocarbon dating of three sediment cores were used to reconstruct, for the first time, the postglacial development of vegetation in the northwestern Alexander Archipelago during the past ∼15,240 cal yr. Hummingbird Lake (HL), on southwestern Baranof Island, yielded a sediment core with one of the longest dated records from southeastern Alaska. The earliest part of the HL pollen record (∼15,240–14,040 yr BP) indicates that the earliest vegetation was pine (Pinus contorta subsp. contorta) parkland with willows (Salix), heaths (Ericaceae), sedges (Cyperaceae), grasses (Poaceae), herbs and ferns. Starting at ∼14,040 yr BP, alder (Alnus) rapidly colonized the area as pine populations declined. By 11,400 yr BP, Sitka spruce (Picea sitchensis) colonized the area, and soon became the dominant conifer. Mountain hemlock (Tsuga mertensiana) also colonized the area by ∼11,400 yr BP, followed by western hemlock (Tsuga heterophylla) at ∼10,200 yr BP. By ∼9200 yr BP, western hemlock had become the dominant tree species in the area. During the late Holocene yellow cedar (Chamaecyparis nootkatensis) became established. Two marine sediment cores were also analyzed for pollen, with the oldest core from Lower Sitka Sound, between Kruzof and Baranof Islands. The lower part of the core consists of interlayered tephras and freshwater lake muds that are estimated to be ∼14,000 to 13,150 yr BP. Pollen evidence indicates that the early postglacial vegetation around Sitka Sound was pine parkland with alders and abundant ferns. Damage to vegetation around Sitka Sound by volcanic eruptions is suggested by abrupt, large shifts in percentages of alder and pine pollen, and fern spores in samples adjacent to tephra layers. A marine sediment core from Slocum Arm, a fiord on the western coast of Chichagof Island, has a basal age of ∼10,000 yr BP. The pollen record is similar to the Holocene pollen record at Hummingbird Lake. The sequence of vegetation changes interpreted from the three northwestern Alexander Archipelago pollen records is similar to those from other well-dated sites in southeastern Alaska, although chronologies differ between sites.
Introduction
The vast conifer forests of present-day southeastern Alaska (Figure 1B) are part of the world’s largest temperate rainforest that extends from northern California, along the Oregon, Washington, British Columbia (B.C.) and Gulf of Alaska coasts as far northwest as the Cook Inlet region (Figure 1A) in south-central Alaska (Heusser, 1960). This northeast Pacific coastal rainforest is not uniform in its composition, as the number of plant species diminishes with cooler, wetter climates at higher latitudes. Fewer species along the northern coasts is also a result of the time required for plants from distant locations to colonize areas that were buried under ice during the Last Glacial Interval (LGI), roughly equivalent to Marine Isotope Stage 2, ∼29,000–14,000 yr BP (Lisiecki and Raymo, 2005). Glacial ice extended from Puget Sound in Washington State northward and westward to the Alaska Peninsula and beyond, into the Aleutians during the LGI (Blaise et al., 1990; Kaufman and Manley, 2004). Many plant species that now grow in southeastern (SE) Alaska appear to have spread northward along the coast into the region perhaps from as far south as coastal areas of Washington State or refugia along the B.C. coast and its exposed continental shelf (Heusser, 1960, 1989; Luternauer et al., 1989; Barrie et al., 1994; Fedje and Josenhans, 2000; Lacourse et al., 2003; Lacourse, 2005; Lacourse and Mathewes, 2005; Mathewes and Clague, 2017). However, the early, rapid spread of some tree species, especially pine, during and following deglaciation suggests that some plant populations probably survived in refugia within SE Alaska, and the Queen Charlotte Islands (Haida Gwaii) of B.C. (Figure 1B), as well as on unglaciated areas of the adjacent exposed continental shelves, allowing plants to quickly colonize newly deglaciated lands in the region.
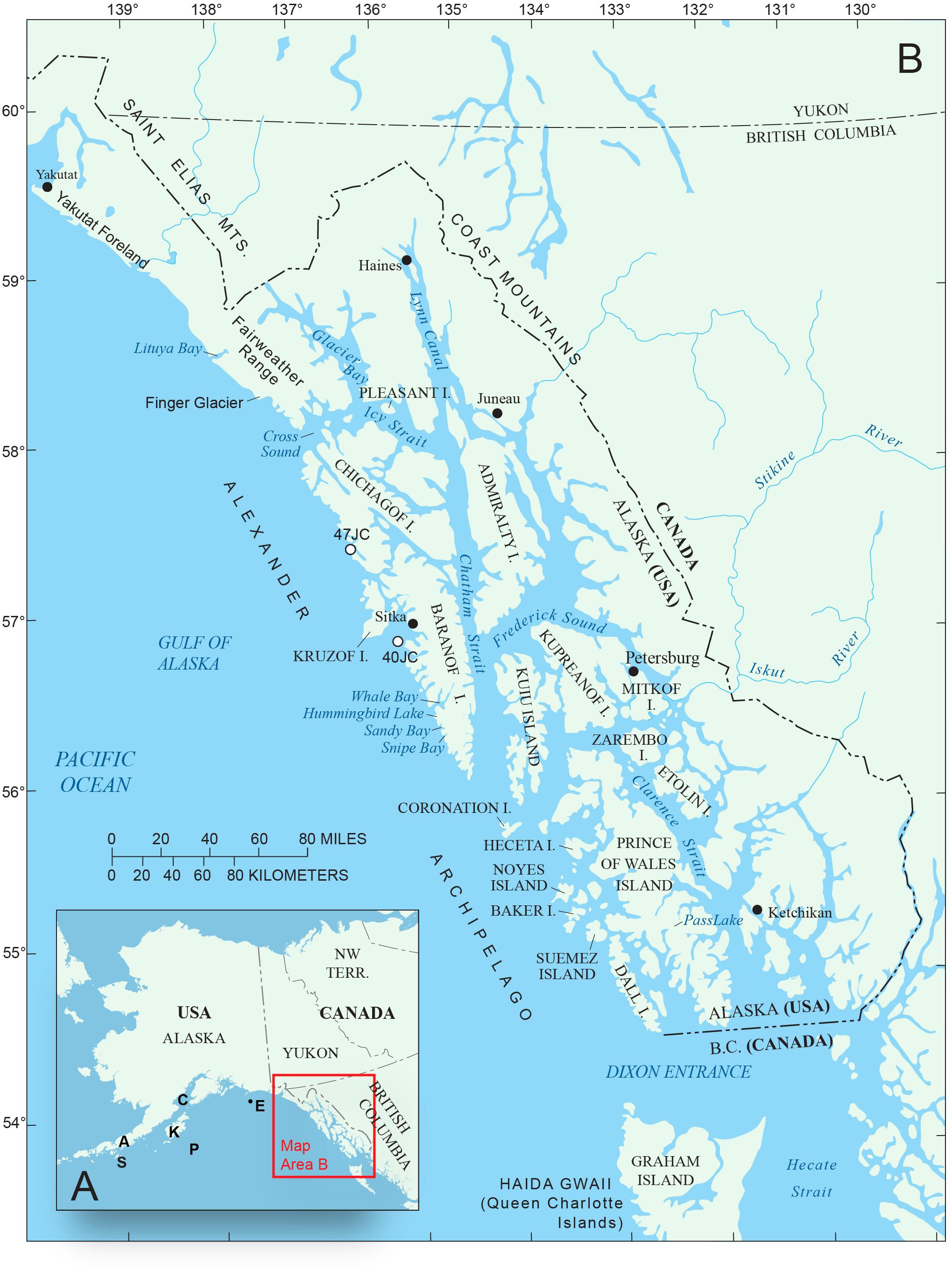
Figure 1. (A) Inset map of Alaska showing Gulf of Alaska sites mentioned in text: A – Alaska Peninsula; S – Sanak Islands (Misarti et al., 2012); C – Cook Inlet; K – Kodiak Island (Peteet and Mann, 1994); P – Patton Seamounts marine coring sites (de Vernal and Pedersen, 1997; Méheust et al., 2018); E – EW0408-85JC marine coring site (Barron et al., 2009; Davies et al., 2011; Praetorius et al., 2016). (B) Map of southeastern Alaska showing geographic features and research locations discussed in text.
The modern conifer forests of southeastern Alaska are classified as perhumid temperate rainforest, with climate characterized by heavy precipitation and no dry season (Alaback and Pojar, 1997). This region extends southward to northern Vancouver Island in B.C. South of northern Vancouver Island, seasonal rainforests of the Pacific Northwest prevail with a summer dry season. Northwest of SE Alaska is another subregion: subpolar rainforest, which has a cooler climate and vegetation more subalpine in character, fewer tree species, and larger areas covered by wetlands, shrublands, muskeg and meadows (Alaback and Pojar, 1997). In spite of the distances involved in postglacial colonizing of SE Alaska, largely from southern seed sources, the modern flora of southeastern Alaska is extraordinarily rich, with at least ∼862 species and subspecies of known native vascular plants. That number represents ∼56% of the native vascular flora of the entire State of Alaska, according to Muller (1982), and species range maps in Hultén (1968) and Pojar and MacKinnon (1994). This species richness is remarkable, given that SE Alaska’s present land area (94,175 km2) represents only 6.2% of the land area of the State of Alaska. In addition to the rich vascular plant flora (coniferous and deciduous trees, shrubs, and low woody plants, graminoids, forbs, ferns and fern allies), there are 572 known species of mosses and liverworts, 425 species of lichens (Vitt et al., 1988), and over 230 known species of fungi (O’Clair et al., 1997).
The species richness of today’s SE Alaskan vegetation, and the ancient appearance of its dense, conifer-dominated rainforests, laden with epiphytic lichens, mosses and fungi, seems at odds with the fact that massive glaciers from the Cordilleran Ice Sheet (CIS) to the east, and local glaciers within the Alexander Archipelago expanded during the LGI to fill all of the fiords with ice, and cover nearly all of the land areas in the region, as well as large areas of the adjacent continental shelf (Carrara et al., 2003, 2007; Kaufman and Manley, 2004). The history of colonization of SE Alaskan and B.C. coastal regions by plants and animals during and following deglaciation has been an intriguing subject for research since 1950. Palynological investigations and radiocarbon dating of postglacial peat and sediment cores have been conducted to reconstruct the chronology and stages by which the vegetation developed. Coastal forests similar to today’s existed in the region during the last interglacial (MIS 5), as indicated by pollen analysis of an exposure of organic rich interglacial sediments from the Echo Section, near Lituya Bay (Figure 1B). The site was discovered by Worley (1980), and later was sampled and analyzed by Mann (1986) to obtain an informative pollen record. The most abundant pollen types from that interglacial site included Pinus, Picea, Tsuga heterophylla, Tsuga mertensiana, Alnus, Cyperaceae, and Lysichiton americanum, all of which are common in the present-day forests of the region. Interstadial vegetation (MIS 3) of Haida Gwaii in northern coastal B.C. has been reconstructed from pollen evidence and indicates that conifer species were present prior to 32,200 ± 370 yr BP, before the beginning of the LGI (Warner et al., 1984). Interstadial pollen types included fir (Abies amabilis was reported, but it may have been Abies lasiocarpa), Picea, T. mertensiana, Pinus, Alnus, Salix, Cyperaceae, Poaceae, various herbs as well as fern spores. These pre-LGI pollen records indicate that the vegetation that has developed in southeastern Alaska and coastal B.C. since the end of the last glaciation is composed of essentially the same key species as were present during Marine Isotope Stages 3 (interstadial) and 5 (interglacial).
This paper focuses on reconstructing the postglacial vegetation history of western coastal areas of northwestern Alexander Archipelago (Figure 1B), a large area of southeastern Alaska for which no dated pollen records have been published prior to this study. The area under investigation includes western Baranof and Chichagof Islands and adjacent marine waters, a region directly exposed to the full force of North Pacific storms. This investigation is based on pollen and spore analysis of radiocarbon-dated sediment cores from a coastal lake and two marine sediment cores from the region. These data were collected in order to reconstruct the stages and chronology of vegetation development, and to evaluate an area on the southwestern coast of Baranof Island that may have been a refugium where some plants and animals survived during the LGI. The idea that refugia for plants and animals existed along the western edge of the CIS has long been a subject of discussion and research (e.g., Heusser, 1960, 1989; Worley, 1980; Wheeler and Guries, 1982; Heaton et al., 1996; Heaton and Grady, 2003; Lacourse and Mathewes, 2005; Reimchen and Byun, 2005; Carrara et al., 2007). These investigations provide strong biological evidence for the existence of refugia in southeastern Alaska, composed of terrestrial and marine ecosystems robust enough to support a genetically distinct population of brown bears, implying long-term isolation from other populations (Heaton et al., 1996).
The reconstruction of changing postglacial environmental conditions in coastal regions of the Gulf of Alaska are also highly relevant to understanding the challenges faced by the first maritime-adapted human colonists who began to reach the area by boat from the northwest Pacific region at some as-yet unknown time after deglaciation, but prior to the beginning of the Holocene (Dixon, 1999, 2001). Human skeletal remains found in the Shuká Káa site (formerly known as On Your Knees Cave) on northwestern Prince of Wales Island (Figure 1B) indicate that maritime-adapted humans were present in the Alexander Archipelago by ∼10,300 yr BP, and probably much earlier (Dixon, 1999, 2001; Dixon et al., 2014; Carlson and Baichtal, 2015; Lesnek et al., 2018). A recent archeological discovery of human footprints preserved in buried beach sands on Calvert Island in northern coastal B.C. suggests that humans were present in the region at least as early as 13,000 yr BP (Mackie et al., 2018; McLaren et al., 2018). This paper provides evidence of the terrestrial plant resources and climatic conditions faced by human colonists and their descendants in this dynamic region with its history of earthquakes, tsunamis, volcanic eruptions, rapid sea level changes, and changing climate, faunas, and vegetation. The changes in regional marine and terrestrial faunas during the past ∼17,000 years are recorded in rich fossil vertebrate faunas excavated from caves in southeastern Alaska (Heaton and Grady, 2003; Lesnek et al., 2018) and in Haida Gwaii, B.C. (Wigen, 2005).
Regional Geography and Climate
Southeastern Alaska extends from Dixon Entrance on the south, to Icy Bay (west of Yakutat Bay) to the northwest, a distance of 844 km (Figure 1B). Of the region’s land area of 94,175 km2, 12,500 km2 is presently covered by glacial ice (Molnia, 2008). Present-day climate is maritime, with relatively mild winter temperatures, frequent North Pacific storms, heavy rainfall, especially in autumn and winter, frequent fog, and heavy winter snowfall in the mountains (Table 1). The Alexander Archipelago (Figure 1B) comprises over 1100 islands, which range in size from small rocky islets to Prince of Wales Island (Figure 1B), which is the third largest island in the United States (6674 km2). The Coast Mountains on the mainland of SE Alaska and B.C. (Figure 2) and many of the larger islands (e.g., Chichagof, Baranof, Admiralty, Prince of Wales, Dall, and Revillagigedo) have rugged mountainous terrain, but the altitudes of most mountain chains on the islands are generally too low to support glaciers under present climate conditions. At present, glaciers cover only about 150 km2 in the Alexander Archipelago, mostly on Chichagof and Baranof Islands (Molnia, 2008).
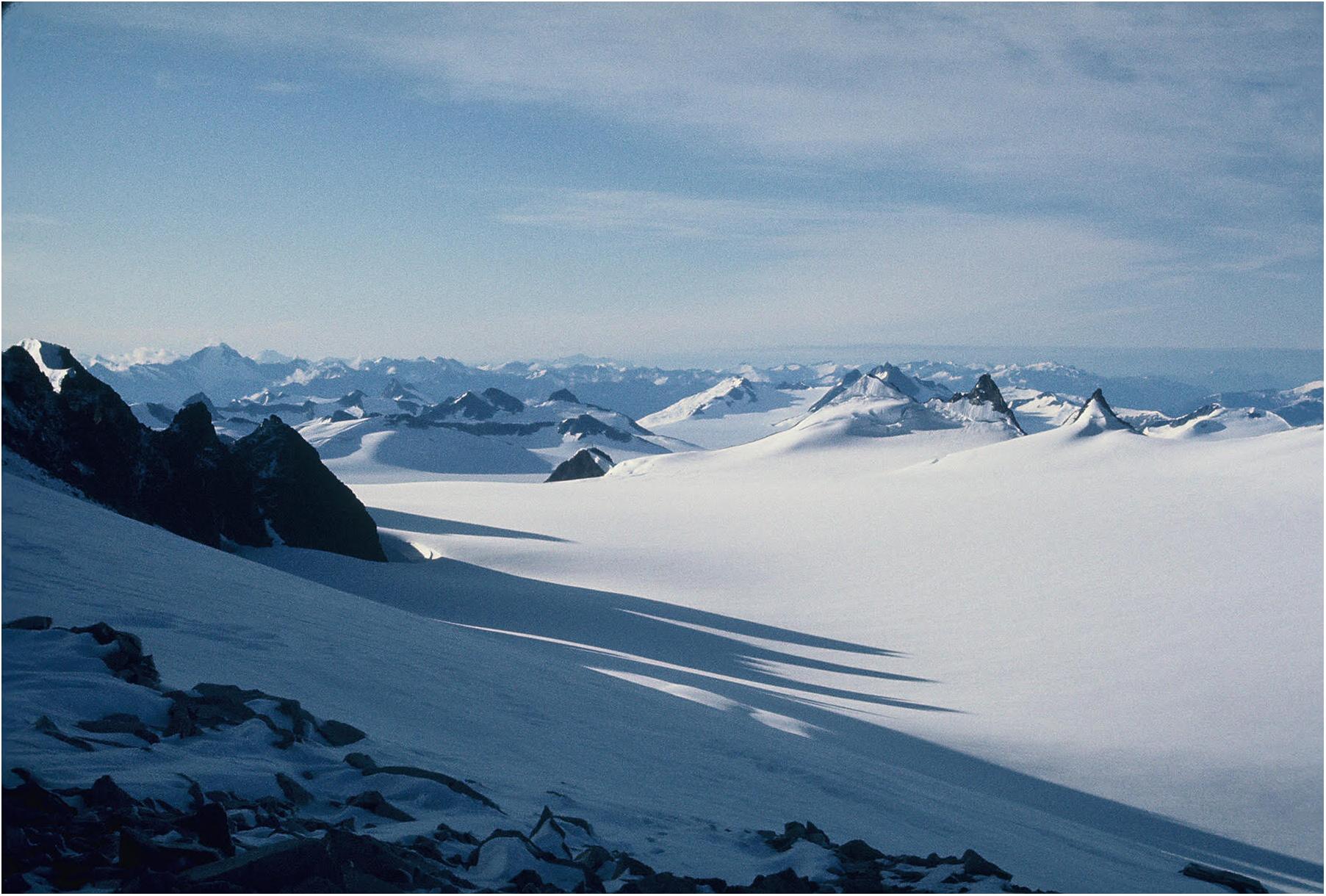
Figure 2. Juneau Icefield with its ice-filled valleys and scattered nunataks is a useful analog for what much of the Alexander Archipelago was like during the Last Glacial Interval (T. Ager photo).
Modern Vegetation
Most of present-day southeastern Alaska up to ∼700 m altitude is blanketed by dense conifer forests (Figure 3), dominated by a few tree species: T. heterophylla (western hemlock), Picea sitchensis (Sitka spruce), T. mertensiana (mountain hemlock), and Pinus contorta subsp. contorta (shore pine), closely related to lodgepole pine (P. contorta subsp. latifolia). Also common is Chamaecyparis nootkatensis (yellow cedar). In southern Alexander Archipelago, Thuja plicata (red cedar), A. lasiocarpa (subalpine fir), Taxus brevifolia (Pacific yew), and A. amabilis (Pacific silver fir) occur, but are absent from northern southeastern Alaska (except for subalpine fir near the Juneau area) and played no role in the present study area’s vegetation history. Deciduous trees are locally important, especially along streams, and include Populus balsamifera subsp. trichocarpa (black cottonwood), and Alnus rubra (red alder). The conifer forests of SE Alaska are interspersed with small to large areas of muskeg (peatlands), where mosses, sedges, heaths (Ericaceae), and slow growing or stunted conifer trees such as shore pines and mountain hemlock are found (Figure 4). Tree growth is often limited in muskeg terrain by waterlogged acidic soils, thick peat deposits, and low availability of nutrients. Muskeg generally forms on level or slightly sloping terrain in cool, wet climates. With increasing altitude in mountainous areas, the coastal forest of western hemlock-Sitka spruce transitions to a montane forest in which mountain hemlock trees become more abundant than spruce and western hemlock. Trees become smaller, are slower growing, and are interspersed with shrub thickets, especially Sitka alders (Alnus viridis subsp. sinuata). Mountain hemlocks become far more abundant at higher elevations in the subalpine zone and that species, along with less common Sitka spruce develop as low growing shrubs at the upper limits of tree growth (Figure 5; Heusser, 1989). Alpine tundra occurs above the altitudinal limits of tree growth (∼675–700 m), which has a discontinuous vegetation cover of mosses, lichens, cushion plants, prostrate woody shrubs, such as Salix (low willows), Empetrum (crowberry), Phyllodoce aleutica (mountain heather), Cassiope mertensiana (Merten’s cassiope) and many other hardy plants that grow interspersed with barren bedrock, talus, and glacial deposits (Jaques, 1973; Heusser, 1989; O’Clair et al., 1997). The flora and vegetation of subalpine and alpine zones in SE Alaska are of particular interest, as the plants that are able to grow in those cooler, snowier environments with shorter growing seasons are most likely to have survived in refugia at much lower altitudes during the LGI when mean July temperatures were probably 4–5°C cooler near sea level than today (Heusser, 1989; Table 1). Subalpine vegetation is also heavily utilized by bears, Sitka deer, and other animals as a summer food source (O’Clair et al., 1997).
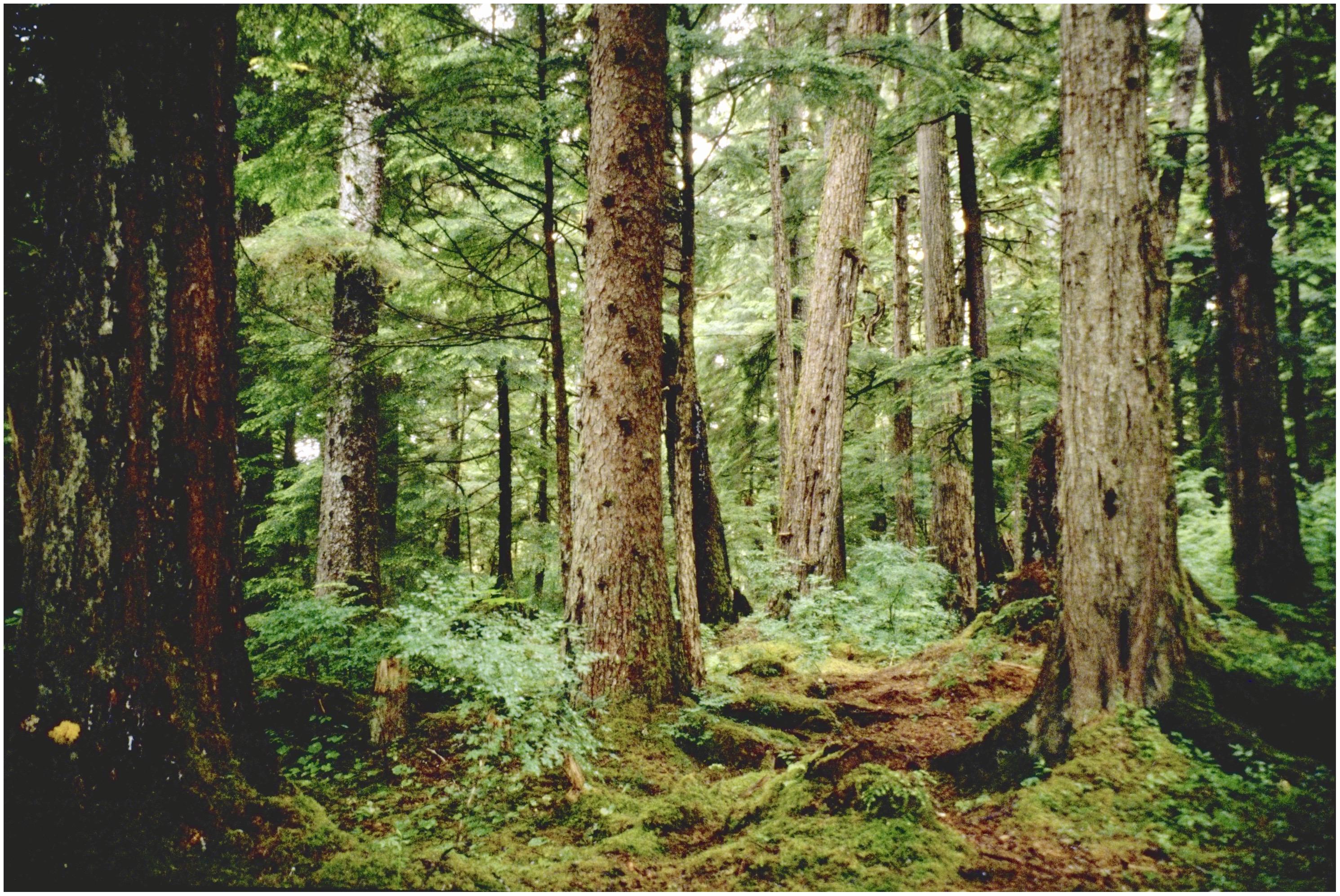
Figure 3. Temperate western hemlock-Sitka spruce coniferous rainforest in southeastern Alaska (T. Ager photo).
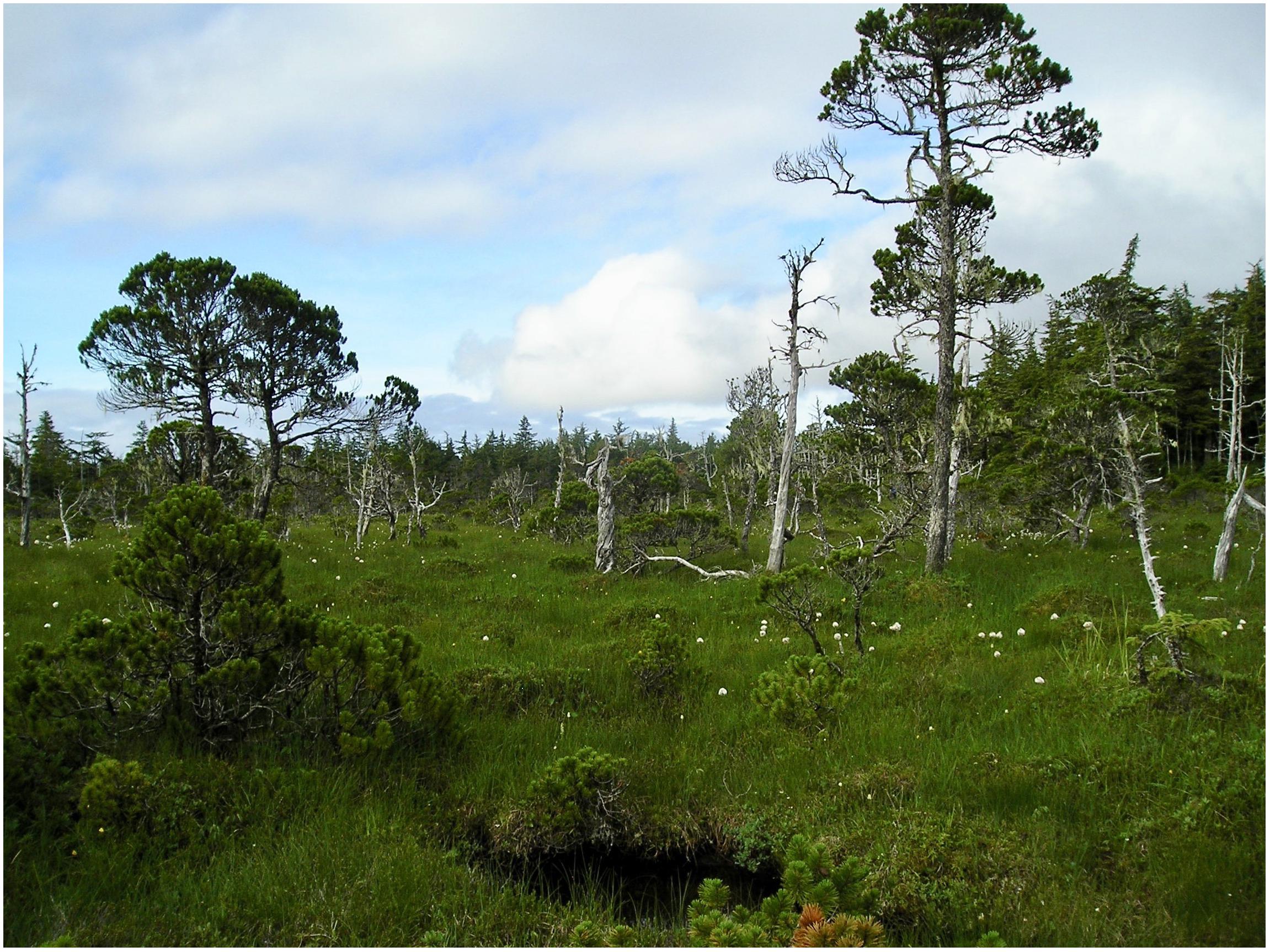
Figure 4. Muskeg (peatlands) with shore pines (Pinus contorta subsp. contorta) on Kruzof Island, northwestern Alexander Archipelago, southeastern Alaska (T. Ager photo).
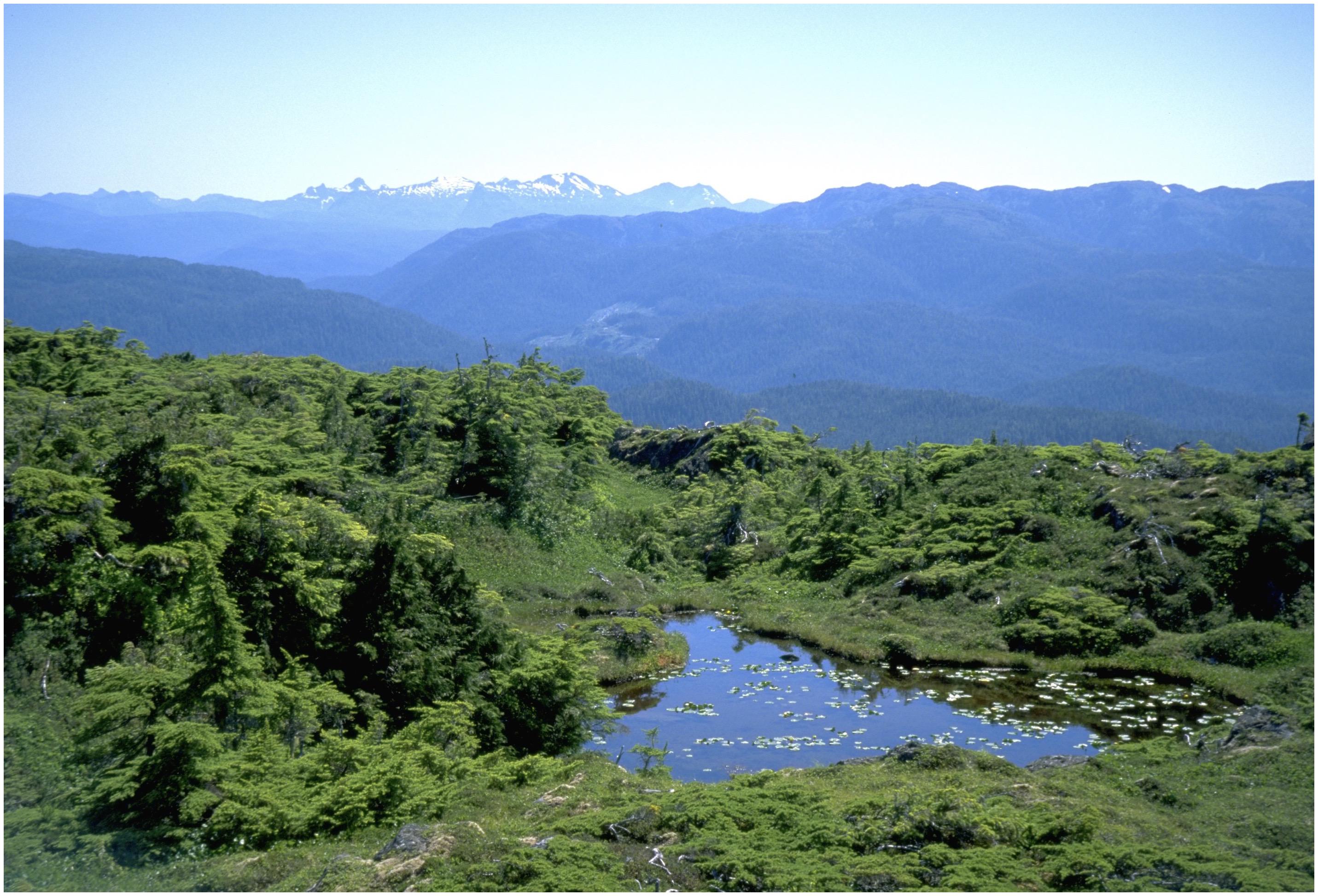
Figure 5. Upper subalpine zone vegetation with stunted mountain hemlock and Sitka spruce growing near its altitudinal limits, on Prince of Wales Island, southeastern Alaska (T. Ager photo).
Previous Research on Vegetation History of Southeastern Alaska
Calvin J. Heusser began an ambitious project in 1950 to reconstruct the postglacial history of vegetation development of the Pacific coast conifer forests by analyzing pollen records from peat deposits scattered along the coasts between northern California northward to the Gulf of Alaska and sites as far west as the Aleutians. His investigations included numerous sites in southeastern Alaska and coastal B.C. (Heusser, 1952, 1954, 1960, 1965, 1985, 1989). Heusser’s pioneering studies occurred at a time when radiocarbon dating methods were under early development, and therefore few of Heusser’s sites in the region had any age control. Those of Heusser’s sites with radiocarbon age control usually had only a single date near the core bases (Heusser, 1960). Also, the reliance on peat deposits for sampling sites limited the time depth of most of the records obtained, as most peat deposits in the region are of Holocene age (Heusser, 1960). Nevertheless, his landmark publication remains an impressive contribution and a valuable source of information about the modern vegetation and the history of the North Pacific coastal rainforests.
Updating the record of vegetation history of southeastern Alaska following Heusser’s pioneering research has proceeded slowly, beginning with reports of dated pollen records from a postglacial peat deposit near Lituya Bay (Figure 1B; Mann, 1983), Lily Lake near Haines (Figure 1B; Cwynar, 1990), a lake on Pleasant Island near Glacier Bay (Figure 1B; Hansen and Engstrom, 1996), an archeological site with a discontinuous Holocene pollen record (Hidden Falls) at Kasnyku Bay on the northeastern coast of Baranof Island (Holloway, 1989), a peat deposit on Mitkof Island (Figure 1B; Ager et al., 2010), a lake sediment deposit (Pass Lake) on central Prince of Wales Island (Figure 1B; Ager and Rosenbaum, 2009), and lake sediments and peat deposits from Heceta Island (Figure 1B; Ager, 2007). In northwestern Alexander Archipelago, previous studies are few, and include pollen records from peat deposits in the Sitka area, and near Suloia Lake on southern Chichagof Island (Figure 1B; Heusser, 1952, 1954, 1960). None of Heusser’s sites from this region had radiocarbon age control, and in the case of the Sitka area sites, only a few conifer pollen types were recorded. Those records are probably no older than mid-Holocene age, based on comparison with records discussed in this paper. The Suloia Lake peat core pollen record from Chichagof island is likely of pre-Holocene age at the base of the core. This paper provides the missing history of dated, pollen-based vegetation histories for the northwestern archipelago, and then compares the region’s history with well-dated records from other areas of SE Alaska.
Glacial and Climate History
Reviews of glacial history of southeastern Alaska (Mann, 1986; Mann and Hamilton, 1995) emphasize how little was known about the region’s glacial record as of the mid-1980s and mid-1990s. Since then, progress in interpreting the onshore history of the LGI has been limited (e.g., Briner et al., 2017; Lyles et al., 2017). Important new information derived from cosmogenic dating of erratic boulders and glacially scoured bedrock surfaces indicate that LGI glacial ice was somewhat more extensive in southwestern Alexander Archipelago than was depicted in maps by Carrara et al. (2003, 2007) and Kaufman and Manley (2004). Their studies indicate that deglaciation in that region was underway in that area by ∼17,000 ± 700 yr BP (Lesnek et al., 2018). The maximum extent of LGI ice in the archipelago appears to have occurred between ∼20,000–17,000 yr BP, based on a gap in dated vertebrate remains in cave deposits (Heaton and Grady, 2003; Lesnek et al., 2018). Farther west along the coast of Gulf of Alaska (GOA), glacial ice retreated from the continental shelf south of the Alaska Peninsula (Figure 1A) ∼17,000 yr BP based on radiocarbon dating of lake sediment cores from Sanak Island (Figure 1A) south of the peninsula (Misarti et al., 2012). On Kodiak Island (Figure 1A), investigations indicate that glacial ice retreat also occurred early: ∼16,700 yr BP (Peteet and Mann, 1994).
During the LGI the CIS vastly expanded from the Coast Mountains of SE Alaska to fill the numerous fiords with glacial ice (Figure 2). Expanding glaciers eventually overtopped many of the smaller, lower islands, especially in the eastern archipelago (e.g., Mitkof, Kupreanof, Zarembo, Gravina, Etolin, and Wrangell Islands). At its maximum LGI extent, the CIS was about the size of the present Greenland ice sheet (Clague, 1989). In many areas, the CIS and local ice from the mountains on western Alexander Archipelago islands expanded onto the continental shelf (Carrara et al., 2007), as it did along the northern and northeastern coasts of the Gulf of Alaska (Mann, 1986; Molnia, 1986). However, it is likely that some areas along the western margins of the Alexander Archipelago and parts of the adjacent subaerially exposed inner continental shelf remained free of glacial ice cover (Heaton and Grady, 2003; Carrara et al., 2007). Such areas provided likely refugia for plants and animals. Other likely refugia have been identified in the Lituya Bay area (Figure 1B) north of the Archipelago (Heusser, 1960; Worley, 1980; Mann, 1983, 1986). Likely LGI refugia also existed on the exposed continental shelf east and south of Haida Gwaii in northern coastal B.C. (Figure 1B; Heusser, 1989; Lacourse et al., 2003; Lacourse and Mathewes, 2005).
GOA coastal areas and mountain ranges underwent significant isostatic crustal adjustments as a result of the massive ice load during the LGI, with the greatest crustal depression occurring along the mainland Coast Mountains and inner (eastern) islands of the archipelago where ice was thickest (Mann, 1986). Along the western margins of the archipelago, and farther south in the area of Haida Gwaii in B.C., crustal adjustments included forebulge development caused by westward lateral movement of upper mantle material from under the Coast Mountains that had subsided under the weight of thick glacial ice. The forebulge elevated coastal areas of the western archipelago and raised large areas of the inner continental shelf well beyond the areas that were exposed by at least –125 m of eustatic sea level lowering alone (Clague, 1983; Hetherington et al., 2004; Briner et al., 2017).
Important advances in glacial-deglacial history in the region have been made recently through detailed, well-dated paleoceanographic studies of a key marine sediment core from the northern GOA region (Figure 1A, Site E: Barron et al., 2009; Davies et al., 2011; Praetorius and Mix, 2014; Praetorius et al., 2015). A marine record spans the past ∼17,400 yr and was collected from the continental slope (core EW0408-85JC) at 59.5553°N, 144.1535°W, 682 m water depth). That record indicates that seawater salinities began to freshen from glacial meltwater by 16,650 ± 170 yr BP, but ice proximal glaciomarine sediments were still being deposited on the continental slope at that time. In that area, coastal glaciers that expanded onto the continental shelf, perhaps in part as floating ice shelves, persisted until ∼14,790 ± 380 yr BP, when glaciers apparently retreated onshore and into fiords (Davies et al., 2011). The retreat by glaciers to onshore positions coincides with the onset of the Bølling-Allerød warm interval (14,700–12,900 yr BP). The withdrawal of glaciers from coastal waters of the northern GOA coincides with the disappearance of ice-rafted debris (IRD) in marine core 85JC, indicating that icebergs from calving glaciers largely disappeared from the GOA after ∼14,700 yr BP (Praetorius and Mix, 2014). Sea surface temperatures in the GOA remained cold until after ∼16,000 yr BP, when they begin to warm, rising ∼4–5°C during the Bølling, cooled somewhat during the Allerød, and cooled more during the Younger Dryas (12,900–11,700 yr BP), then warmed ∼4–5°C again during the early Holocene (11,500–10,500 yr BP; Praetorius and Mix, 2014; Praetorius et al., 2015).
Deglaciation of the continental shelf near Haida Gwaii, B.C., began as early as ∼18,000 yr BP, and the lowlands of the islands were undergoing deglaciation by about that time (Barrie and Conway, 1999). The available records from the GOA indicate the timing of deglaciation in the western Alexander Archipelago by ∼17,000 ± 700 yr BP from cosmogenic dating of erratic boulders and ice-scoured bedrock surfaces. However, stagnating glacial ice in some valleys may have persisted for longer, e.g., 15,300 ± 600 yr BP on Baker Island in the southwestern archipelago (Figure 1B; Lesnek et al., 2018).
Studies of marine diatom and dinocyst assemblages in Gulf of Alaska sediment cores, including sites in the Patton Seamounts southeast of Kodiak Island (Figure 1A, site P) provide information about the extent and timing of sea ice during and after the LGI (de Vernal and Pedersen, 1997; Barron et al., 2009, 2016; Méheust et al., 2018). The marine microfossil assemblages indicate that LGI winter sea ice was very widespread in the Gulf of Alaska, probably lasting for ∼six months a year, and extending seaward from the Gulf of Alaska coastlines perhaps as far south as ∼52°N in central GOA (Méheust et al., 2018), and along the coasts of SE Alaska and probably B.C., as indicated by the presence of sea ice diatoms preserved in marine sediment records (Barron et al., 2009, 2016). Other evidence for coastal sea ice includes late glacial-age vertebrate fossils of mammals preserved in caves in southeastern Alaska that include species associated with arctic sea ice, such as ringed seals (Phoca hispida) and arctic foxes (Alopex lagopus; Heaton and Grady, 2003; Lesnek et al., 2018). Winter sea ice disappeared from most of the Gulf of Alaska during the Bølling warm interval, starting ∼14,700 yr BP (Méheust et al., 2018), but persisted at least intermittently during winters, well into the Holocene in coastal areas, where cold winters and input of cool freshwater from runoff from rain and snow and glacial melting reduced local sea surface salinity, promoting winter sea ice development (Barron et al., 2009, 2016). Coastal sea ice was more widespread during the Younger Dryas cold interval (Barron et al., 2009) when summer sea surface temperatures dropped ∼4°C (Praetorius et al., 2015). The existence of winter-spring sea ice in fiords during and soon after deglaciation may have allowed animals to disperse over ice from island to island, although they are known to swim across marine waterways today in spite of strong currents and variable sea conditions. Sea ice history has implications for human colonization of Alaskan coastal regions, reducing winter mobility by boat, and making access to marine food resources more challenging. Colder temperatures during the Younger Dryas resulted in less productive marine ecosystems, reducing potential food resources, such as fish, shellfish and other intertidal faunas and floras (Hetherington and Reid, 2003; Davies et al., 2011).
Climate shifts within the Holocene began with early Holocene warming ∼11,500–10,500 (e.g., Pellatt and Mathewes, 1997; Praetorius et al., 2015), followed by cooling of the GOA between ∼10,500–8000 ka, followed by gradual warming (Praetorius and Mix, 2014). During the mid- to late Holocene, temperatures shifted between warmer and cooler intervals, during which several Neoglacial ice advances and retreats occurred in the mountains rimming the Gulf of Alaska region, with many glaciers reaching tidewater (McKenzie and Goldthwait, 1971; Heusser et al., 1985; Mann et al., 1998; Moss et al., 2007). The Neoglacial began in the Gulf of Alaska region ∼5600 yr BP and included the Little Ice Age that began ∼1350 AD and ended ∼1900 AD (Mann et al., 1998).
Materials and Methods
The lake sediments in Hummingbird Lake (HL) were sampled during the summer of 2000 with a modified Livingstone piston corer (Aaby and Digerfeldt, 1986), using aluminum core tubes with an inside diameter of 5 cm. Sediment cores were taken in 1-m-long drives (or less), from an anchored platform with inflatable floats. Soon after the field season the sealed cores were extruded in the laboratory, described, and sampled for pollen analysis and radiocarbon dating. Samples for pollen analysis were collected at 2 to 10 cm intervals and varied in volume between 2 and 5 cm3. Core samples were processed in the U.S. Geological Survey (USGS) Cenozoic Palynology Laboratory in Denver, Colorado, using pollen extraction methods modified from those described by Doher (1980) and Brown (2008). Processing treatments began with screening to remove coarse plant detritus > 150 μm, then washed in dilute HCl to remove carbonates, HF in a hot water bath to remove silicate minerals, then timed washes in Shultze solution (10% HNO3 and potassium chlorate (KClO3) to oxidize fine plant detritus remaining after sieving. A 5% solution of potassium hydroxide (KCl) was used to remove oxidized organic material without damaging pollen and spores. Pollen residues were mounted on glass slides in glycerine jelly. Pollen slides were examined with a transmitted light binocular microscope at magnifications of 400×, 630×, and occasionally 1000× (oil immersion). Pollen and spores were identified using a modern pollen reference slide collection at the USGS in Denver.
Core samples selected for radiocarbon dating, many of which consisted of detrital peat, were prepared at the USGS Radiocarbon Laboratory in Reston, Virginia, and dated by Accelerator Mass Spectrometry (AMS) at the National Science Foundation-Arizona dating facility in Tucson, Arizona, or at the Center for Accelerator Mass Spectrometry (CAMS), Lawrence Livermore National Laboratory in Livermore, California. The lowest sample in the HL core selected for AMS radiocarbon dating contained more silt than detrital peat, and the organic material used for dating that sample consisted of excess pollen concentrates resulting from the processing procedures for pollen analysis (Brown et al., 1989). Radiocarbon ages and their calibrations for the Hummingbird Lake core are presented in Table 2. The 14C ages were calibrated using the IntCal13 dataset and CALIB 7.1 html (Stuiver and Reimer, 1993; Reimer et al., 2013). Ages are presented in calibrated years, yr BP (Before Present; 0 yr BP = 1950 A.D.), and uncertainties are given at the 95% (2-sigma) confidence level. In the event that multiple ranges were permitted during calibration, ages discussed in the text and shown in the figures are based on the mean of the ranges weighted by their probabilities and are presented without uncertainties. For example, an age of a single calibrated range would be given as 13,090 ± 100 yr BP, whereas it would be presented as ∼13,100 yr BP if multiple ranges were permitted.
Additional samples for pollen analysis and radiocarbon dating were collected from two cores obtained during the August–September 2004 oceanographic research cruise of the R/V Maurice Ewing. The cores were obtained with a jumbo piston corer with a 10 cm inside diameter, in single drives up to 18 m long. These cores, along with several others, were sampled at the Oregon State University marine core repository in Corvallis, Oregon. The generally rapid sedimentation rates in the marine cores allowed larger sampling intervals, sometimes 50 cm or more. In core EW0408-40JC, some sampling intervals in the lower part of the core were much closer in order to evaluate the influence of volcanic activity on vegetation changes. The radiocarbon chronologies for these two cores were developed by dating shell fragments, wood, and plant material, as discussed in Addison et al. (2010) for EW0408-40JC from lower Sitka Sound (Figure 1B), and in Barron et al. (2016) for EW0408-47JC in Slocum Arm (Figure 1B). Corrections for marine reservoir effect (MRE) and calibrations for the radiocarbon ages are presented in those publications. Pollen and spore percentage diagrams for the sites discussed in this paper were drafted using Tilia and TiliaGraph software (Grimm, 1991, 1993).
Results
Hummingbird Lake Core
The longest and most complete late Quaternary pollen record currently available from northwestern Alexander Archipelago was obtained from Hummingbird Lake (informal name), near the northern shore of Still Harbor on the remote southwestern coast of Baranof Island (Figures 1B, 6). The lake is located at 56.54931°N, 135.01652°W, at an altitude of 46 m. The lake has a surface area of 6.47 ha, and a maximum depth of 18 m. The lake formed in a trough between bedrock ridges and outcrops striking NW-SE. The water depth at the coring site was 15.2 m. The bedrock underlying the area and exposed in landslide scars is Sitka Graywacke of Late Jurassic to Early Cretaceous age (Loney et al., 1975). The lake was selected as a coring target from air photos because of its location within a suspected refugium, an area that does not appear to have been overridden by glacial ice during the LGI (Carrara et al., 2003, 2007). The northwest-southeast trending bedrock ridges within the suspected refugium may have diverted valley glaciers that were flowing from the mountains farther inland to the northwest. If this interpretation is correct, the diverted glaciers would have flowed into the Whale Bay glacier system (Figure 1B) rather than flowing directly southwest over a series of perpendicular sharp bedrock ridges to the (present) coast. During our brief ground surveys of the Still Harbor and Sandy Bay areas (Figure 1B), no glacial landforms, erratic boulders, or glacial deposits were observed in landslide scars and coastal exposures. During the LGI, the glacier within Whale Bay advanced onto the adjacent continental shelf, and glaciers to the south of Snipe Bay (Figure 1B) also reached part of the exposed inner shelf (Carrara et al., 2003, 2007). A large expanse of the subaerially exposed continental shelf south of Whale Bay glacial ice would likely have provided a significant refugium for plants and animals during the LGI (Carrara et al., 2003, 2007). The area of the exposed shelf would likely have been considerably larger than that depicted in Carrara et al. (2007), if the forebulge raised the shelf in this area.
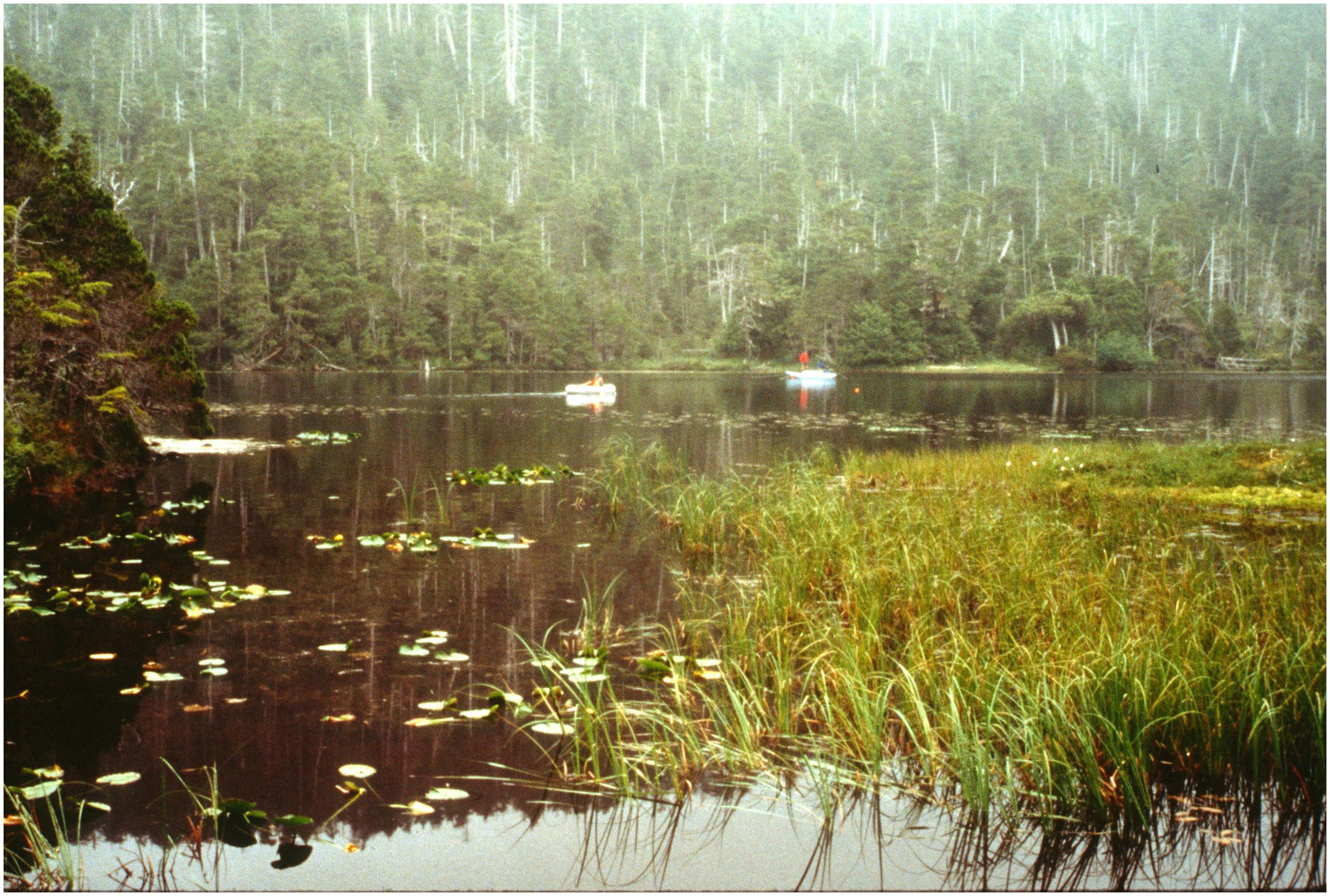
Figure 6. Hummingbird Lake with coring platform in distance. Also shown is hemlock-yellow cedar forest on steep slopes at Still Harbor, southwestern coast, Baranof Island (Paul Carrara photo).
The immediate area around HL has a present-day vegetation of mixed conifer coastal forest that is composed of T. heterophylla, P. contorta, subsp. contorta, and C. nootkatensis. P. sitchensis and T. mertensiana trees and Alnus shrubs were not observed in the immediate vicinity of the lake, but the pollen record from the lake indicates that those trees and shrubs are present in the area. Common shrubs observed in the lake area include Menziesia ferruginea, Vaccinium spp., Empetrum nigrum, and Juniperus communis. Aquatic and semiaquatic plants observed in the lake areas include Nuphar polysepalum, Eriophorum russeolum, Sparganium angustifolium, Menyanthes trifoliata, Isoëtes, Potamogeton natans, and Carex spp. Muskeg areas near the lake include numerous moss species including Sphagnum spp., along with Cyperaceae, mostly Carex spp., along with Vaccinium spp., Kalmia polifolia, Ledum groenlandicum, Andromeda polifolia, Oxycoccus oxycoccus, Cornus canadensis, E. nigrum, Drosera rotundifolia, Gentiana douglasiana, Tofieldia glutinosa, Coptis trifoliata, and Geum calthifolium.
The lake sediments were sampled with a 5 cm-diameter piston corer to a total penetration of 450 cm below the lake bottom. The lowest 48 cm of sediments encountered could only be penetrated by percussion coring, using a cylindrical 10 kg hammer with an anvil attachment on a coring extension rod. The piston corer hit a hard layer at ∼450 cm core depth that may have been bedrock, which ended the sampling operations. The lowermost 48 cm of sediments fell out of the core tube when the piston corer was raised to the surface, but a small amount of sediment recovered indicates that the lost sediments were fine sand, perhaps eolian sand, perhaps from the terrain around Whale Bay that was probably deglaciated or in ice retreat. The recovered sediments from the 402 cm long Hummingbird Lake core consisted of 118 alternating layers of fine detrital peat, and coarser, more fibrous peat. Most of the coarse peat layers were 1–2 cm thick. Those coarse layers may represent rapid deposition during major rainstorm events that may have washed plant detritus into the lake from the surrounding steep upland slopes (Figure 6). Far fewer layers (3) of coarse peat occurred below 315 cm core depth, where the sediments contained more silt. The limited number of those coarser detrital peat layers in the lower part of the core may reflect less accumulation of plant detritus on the slopes adjacent to the lake during the first ∼4000 years of the record, or it may indicate fewer major rainfall or meltwater events during that period.
Hummingbird Lake Pollen Record
The pollen and spore record recovered from Hummingbird Lake (HL) is shown in Figure 7 and Supplementary Table S1. Pollen sums (the total of all pollen grains counted in a single sample) exceeded 300 in all but two samples, and sums ranged from 217 to 366 grains. The pollen plus spore sums ranged between 354 to 1184 grains. The pollen and spore percentage diagram is divided into five pollen assemblage zones, the boundaries of which were drawn where significant changes in pollen assemblages occur, such as the initial local arrival of pollen of a tree species, such as Sitka spruce. The chronology for the core is provided by 11 AMS radiocarbon-dated core samples (Table 2), all but one of which fall close to the regression line that indicates an average sedimentation rate (∼37.9 yr/cm) over the past ∼15,240 years (Figure 8). The uppermost calibrated radiocarbon age plots far from the regression line and is clearly anomalously old. It probably represents plant material that was eroded from older deposits on steep slopes adjacent to the lake and transported into lake sediments much younger than the dated material. That sample was not used to calculate the slope of the regression line and was not used for interpreting the core chronology.
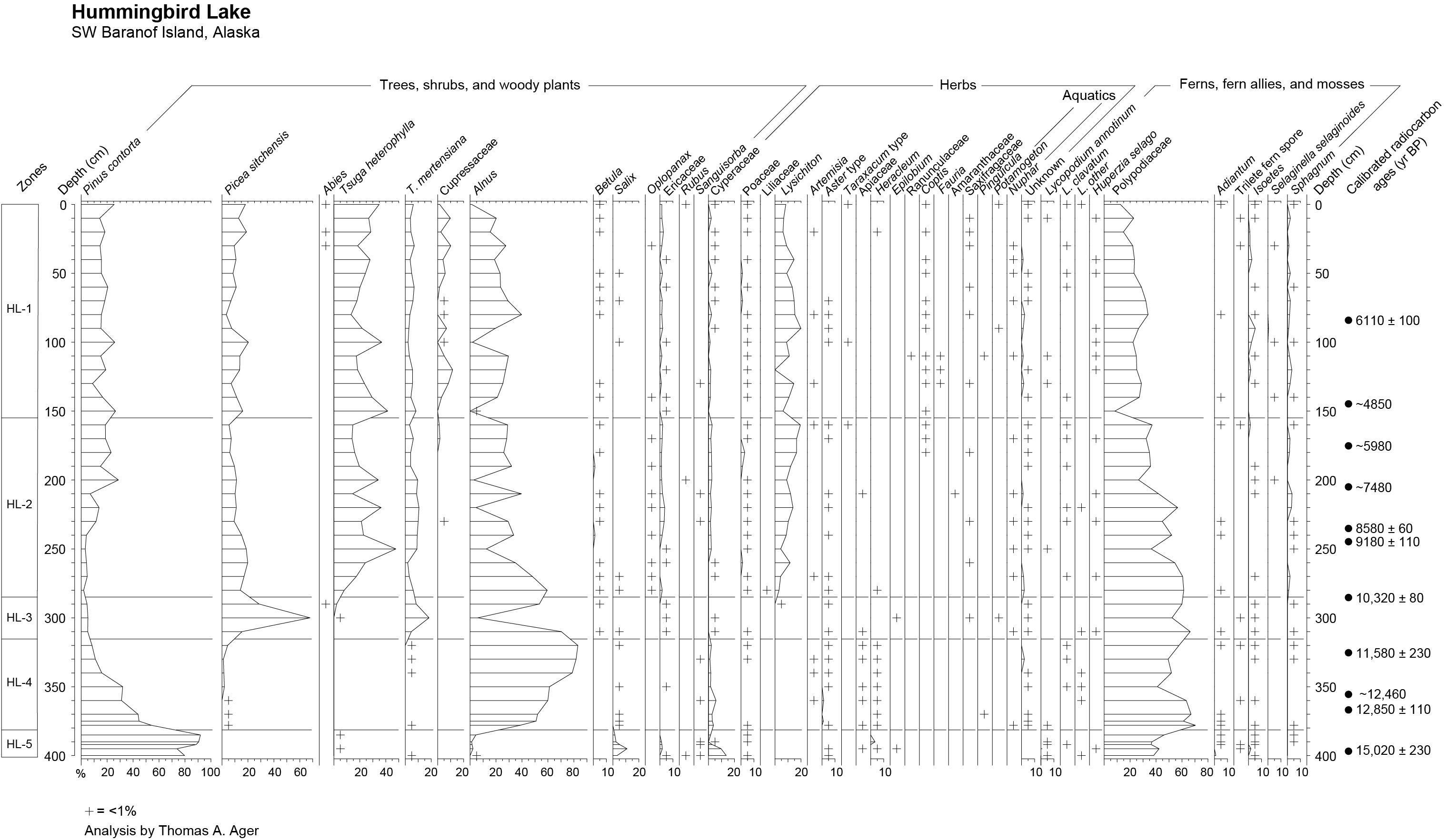
Figure 7. Pollen and spore percentage diagram with calibrated radiocarbon ages for the Hummingbird Lake sediment core, from the southwestern coast of Baranof Island. Pollen data are shown in Supplementary Table S1.
Pollen Zone HL-5
Beginning with the lowest, and oldest pollen and spore assemblage zone (HL-5, 400–382 cm core depth; ∼15,240 to ∼14,040 yr BP), the dominant pollen and spore types include abundant Pinus pollen and fern spores, mostly of the Polypodiaceae type (monolete, psilate). Pollen types that are present in low percentages include Salix, Ericaceae, Cyperaceae, Poaceae, Aster type, Heracleum and other Apiaceae, and Epilobium type. Isoëtes microspores are the only aquatic plant fossils represented in HL-5 pollen samples. The oldest radiocarbon age obtained from the core within lower zone HL-5 is 15,020 ± 230 yr BP (Table 2). That radiocarbon sample was obtained from excess pollen and spore concentrates remaining after sample processing to obtain material for pollen analysis. Such pollen concentrates have been shown to be reliable material for AMS radiocarbon dating (Brown et al., 1989). The depth vs. age plot (Figure 8) shows that the lowermost dated sample from the HL core falls close to the regression line calculated for the core, and therefore the age is likely to be valid. The abundance of pine pollen (>75% of the pollen sum) in even the lowermost samples in Zone HL-5 suggests that pines were well established in the area before the extrapolated age of the base of the core (∼15,240 yr BP), and perhaps were growing within the hypothesized refugium on southwestern Baranof Island (Carrara et al., 2007) and probably on the adjacent subaerially exposed continental shelf. It is noteworthy that the calculated pollen accumulation rates for pine from a sediment core from an unnamed lake on Pleasant Island in the early postglacial (pre-13,000 yr BP) are much higher (up to ∼4000 grains cm-2 yr-1) than at any time during the Younger Dryas cold interval (12,900–11,700 yr BP) that followed, or during the Holocene (Hansen and Engstrom, 1996). The earliest approximate age for pine at Hummingbird Lake (∼15,240 yr BP) predates the onset of the Bølling-Allerød (BA) warmer climate interval (∼14,700–12,900 yr BP). Pine populations may have been responding to the beginnings of ocean warming at ∼16,000 yr BP that preceded the BA warming in the Gulf of Alaska (Praetorius et al., 2015). The BA is recognized from Gulf of Alaska marine sediments as a warmer climate event, with some cooling events during the Allerød (Barron et al., 2009; Davies et al., 2011; Praetorius and Mix, 2014; Praetorius et al., 2015).
Two tephras were discovered in the lower part of the Hummingbird Lake core within Zone HL-5 and at the boundary between Zones HL-5 and HL-4. The upper, slightly younger tephra is from 378 to 381.6 cm core depth, and is light gray to white in color. The lower tephra is from 390.5 to 392.2 cm and is pinkish-gray in color. The approximate ages of the two tephras, calculated by interpolating between dated core samples are ∼13,760 yr BP for the upper tephra, and 14,600 yr BP for the lower tephra. Both tephra samples were analyzed by microprobe by John Westgate at University of Toronto, and he concluded that the upper tephra was rhyolitic in composition and came from one of the early postglacial eruptions from the Mount Edgecumbe volcanic field on Kruzof Island west of Sitka (Riehle et al., 1992a,b; Addison et al., 2010). The lower tephra is also of rhyolitic composition, but came from an unidentified source, perhaps from the Alaska Peninsula. Neither tephra layer is associated with changes in pollen and spore percentages that might indicate damage to vegetation as a result of the ash deposition, in contrast to the record of EW0408-40JC, to be described below.
Zone HL-4
The age of Pollen Zone HL-4’s lower boundary at 382 cm core depth is ∼14,040 yr BP (interpolated age), and the upper boundary is at 317 cm core depth, ∼11,330 yr BP (interpolated age) which is near the base of the Holocene. The pollen samples within this zone record an apparently rapid expansion by Alnus, probably Sitka alder (A. viridis subsp. sinuata) which is the most common alder shrub in southeastern Alaska today, often forming nearly impenetrable thickets along shorelines, in gullies, on avalanche scars, and on mountain slopes in the upper subalpine zone. Within HL-4, the rapid increase in alder pollen percentages coincides with a dramatic decline in pine pollen percentages. This may be a response to somewhat cooler climates during the Allerød, as suggested by paleoceanographic records from the Gulf of Alaska and the even colder conditions during the Younger Dryas cold interval (12,900–11,700 yr BP) that followed (Davies et al., 2011; Praetorius and Mix, 2014). P. contorta subsp. contorta grows most commonly in lowland areas of SE Alaska, but it is sometimes found growing in the cooler subalpine zone of southeastern Alaska, where such climatic conditions result in slow, stunted growth habit in conifers, and it is generally uncommon. Alnus shrubs, on the other hand, are more tolerant of colder climates, as indicated by their abundant growth in the upper subalpine zone in SE Alaska. Alder populations first began to expand during the Allerød (∼14,200–12,900 yr BP), but expanded much more during the Younger Dryas in sites across southeastern Alaska. Alder populations in eastern Canada, although the alder species are different than in southeastern Alaska, also expanded during the Younger Dryas (Mayle et al., 1993). Fern spores are abundant in HL-4 samples, and that may reflect their common occurrence within alder thickets and as colonizing plants in disturbed terrain. Pollen types present in HL-4 in low percentages include Cyperaceae, Salix, Artemisia. and Heracleum and other Apiaceae. Pollen of the aquatic plant Nuphar makes its first appearance within Zone HL-4. The only other aquatic plant represented in HL-4 is Isoëtes (quillwort), a spore-producing plant. Very low percentages of pollen of P. sitchensis occur in lower HL-4 samples and increase slightly near the top of the zone. This initial appearance of spruce pollen suggests that the species was probably present on southern Baranof Island as early as ∼12,400 yr BP, but was probably not yet present near Still Harbor. Its slight percentage increase in upper HL-4 samples suggests that spruce trees were approaching the Still Harbor area by ∼11,200 yr BP.
Another site sampled during this investigation on southwestern Baranof Island during the 2000 field season was a peat bog located 9.5 km south of Hummingbird Lake, near the north shore of Sandy Bay (Figure 1B) at 56.47657°N, 134.95691°W, at 18 m asl. The bog site was selected as a coring target from air photos of the area, when no suitable lakes were found to be accessible from the shores of Sandy Bay. We recovered a peat and lake sediment core 755 cm long that has a pollen record quite similar to that in Hummingbird Lake, but the record is somewhat younger at the base (∼14,600 yr BP). The Sandy Bay bog pollen record is not presented here, as it is quite similar to that from Hummingbird Lake. One unusual discovery from within the Sandy Bay core is worthy of mention, however. Three well-preserved seed cones of P. sitchensis were recovered from the Sandy Bay core. The stratigraphically lowest of the cluster of three cones (625–630 cm core depth) yielded a radiocarbon age of 10,000 ± 40 yr BP (WW-4387: calibrated age ∼11,500 ± 142 yr BP), near the beginning of the Holocene. The cone’s position in that core coincides with a major rise in spruce pollen in the core sediments that enclosed the seed cone, and demonstrates that Picea was present in the Sandy Bay area, only slightly before it began to colonize the Still Harbor area ∼11,300 yr BP. Since conifers are wind pollinated, the presence of low percentages of pollen alone may not indicate that the trees grew at or very near the sampling site. The presence of macrofossils such as seed cones, needles, or stomatal cells derived from decomposed conifer needles (Hansen, 1995; Lacourse et al., 2012, 2016) provide convincing evidence of local presence. At Sandy Bay, the strongest evidence for its arrival locally comes from the presence of spruce seed cones coinciding with rising spruce pollen percentages. No spruce macrofossils were found in the HL core. At Cape Ball, on Graham Island in Haida Gwaii, B.C. (Figure 1B), Warner and Chmielewski (1987) recovered seed cones of spruce of latest Pleistocene and early Holocene age that had morphological characteristics of hybrids between P. sitchensis and Picea glauca. It is therefore possible that the early colonization of southeastern Alaska by spruce included such hybrids. However, spruce needles found in association with the seed cones at Cape Ball had characteristics of Sitka spruce (Warner and Chmielewski, 1987), so the possible presence of early postglacial spruce hybrids in the region remains uncertain.
Zone HL-3
Pollen Zone HL-3 in the Hummingbird Lake core spans core depths 315–285 cm, between ∼11,330 and 10,350 yr BP. The main features in the HL-3 pollen record are the rapid rise in spruce percentages (rising above 60% of the pollen sum in one sample), and the smaller rise in percentages of T. mertensiana (mountain hemlock) pollen. Mountain hemlock grows in southeastern Alaska from sea level to the upper subalpine zone, but greatly increases in abundance at higher altitudes. However, the trees become increasingly stunted at higher altitudes where there are deep winter snows and shorter, cooler growing seasons. Mountain hemlock develops low shrubby growth forms near its upper altitudinal limits of growth in the upper subalpine zone at ∼685 m in the Sitka area (Yehle, 1974). Trace amounts of mountain hemlock pollen in the oldest postglacial records available from southeastern Alaska suggest that it is likely to have survived in refugia in the region during the LGI. Its pollen is usually underrepresented in pollen samples (Heusser, 1960) so even small amounts of its pollen in lowland samples may indicate a significant population of the species in the area. Pollen percentages of mountain hemlock are generally much higher in subalpine zone records, reflecting the greater local abundance of those trees. Alnus pollen and Polypodiaceae type fern spores are also well represented in Zone HL-3. The abundance of alder pollen and fern spores suggests that the developing spruce forests were growing in open stands or scattered denser stands, interspersed with alder shrublands rather than spruce trees covering most of the landscape. Pollen types represented in low percentages in Zone HL-3 include Betula, Salix, Ericaceae, Cyperaceae, Aster type, and Apiaceae. Betula (birch) shrubs or trees have not been found in the present-day vegetation anywhere on Baranof Island, but its pollen can be wind transported long distances. The shrub Betula glandulosa has been found growing on uplands of northern Chichagof Island and along the northern coast of Cross Sound, however (Viereck and Little, 2007), and that appears to be the closest known source of Betula pollen in the northwestern archipelago. The boreal forest paper birch tree, Betula papyrifera, has colonized some areas of western slopes of the Coast Mountains, particularly near Juneau, and is likely to have reached the coast from interior British Columbia along major rivers that flow through the mountains (e.g., the Taku River valley south of Juneau). It is possible that those trees were a source of some of the birch pollen that often shows up in trace amounts in pollen samples from across the region.
Zone HL-2
Zone HL-2 spans the time interval of 10,350 to ∼5000 yr BP, between core depths 285–155 cm. The main feature of the HL-2 pollen record is the evidence for colonization of the Still Harbor area by the conifer T. heterophylla (western hemlock), beginning ∼10,350 yr BP, and which appears to have become the dominant tree in the HL area by ∼9500 yr BP, when its pollen percentages first exceeded that of spruce. As seen from other pollen records in southeastern Alaska, western hemlock spread across most of southeastern Alaskan forest lands in the early Holocene, usually after spruce had become established, but soon became the dominant conifer in most areas. Whereas Sitka spruce is able to colonize barren ground successfully, western hemlock generally requires some organic material and moister soils to successfully develop seedlings that survive, and likely also benefit from the nitrogen added to soils by the alders that preceded it (Cooper, 1939; Lawrence, 1958). Most pollen records from southeastern Alaska that are old enough to record pre-Holocene and early Holocene vegetation histories show pine as the earliest tree to colonize, often with minor amounts of mountain hemlock, followed much later by Sitka spruce, then western hemlock (Heusser, 1960; Hansen and Engstrom, 1996; Ager and Rosenbaum, 2009; Ager et al., 2010). Zone HL-2 shows a significant increase in pine pollen percentages after ∼8000 yr BP, which may reflect the early onset of warmer, moister conditions in the region (Praetorius and Mix, 2014). Sitka spruce percentages decline gradually within HL-2, reflecting the growing dominance of western hemlock trees in the area. Alder pollen and fern spores remain abundant in HL-2, suggesting open or discontinuous conifer forests that did not crowd out the alders. The appearance of L. americanum indicates local swampy areas with organic rich soils along with some mineral soils in the vicinity of Hummingbird Lake throughout the past ∼10,000 yr. Skunk cabbage contributes to the paludification processes that contribute to the expansion of muskeg (peatland) vegetation, which often gradually replaces stands of conifer forest (Heusser, 1960).
Zone HL-1
Pollen Zone HL-1 pollen assemblages are quite similar to those in Zone HL-2. The zone extends from core depth 155 cm to the top of the core (lake floor), and spans the past ∼5000 yrs. The main difference between HL-2 and HL-1 pollen assemblages is that small amounts of Cupressaceae type pollen are present in HL-1. The cedar type pollen is most likely to have been derived from C. nootkatensis (yellow cedar), as yellow cedar is abundant in the Still Harbor area forests today. T. plicata (red cedar) is absent from the area, so the Cupressaceae pollen in HL-1 could not have come from that species. J. communis shrubs produce pollen that is very similar to that of yellow cedar and red cedar, and junipers do occur in the Still Harbor area, but they are low growing, tend to grow in muskeg areas, and are less likely than yellow cedar to contribute much pollen to the lake record. Cupressaceae pollen is often poorly preserved in sediments, or not at all, so it is often either unrepresented in pollen records, or is underrepresented. Therefore the postglacial history of Cupressaceae in coastal Alaska is very poorly known. Heusser (1952, 1954, 1960, 1965) did not record the presence of Cupressaceae type pollen in any of his many pollen diagrams. P. sitchensis pollen is slightly more abundant in HL-1 than in HL-2. Fern spores remain abundant in Zone HL-1. Pollen types that are present in low percentages include Betula, Ericaceae, Cyperaceae, Poaceae, Artemisia, Aster type, Coptis, Nephrophyllidium crista-galli, and Saxifragaceae. Aquatic plants represented by pollen include Potamogeton and Nuphar. The persistence of fairly abundant alder pollen in Zone HL-1 within an area that now has a widespread cover of conifer forest may result from alders colonizing shorelines, streambanks and landslide scars that are common on steep slopes in the area.
Lower Sitka Sound Core (EW0408-40JC)
Pollen samples from two marine sediment cores obtained during the 2004 oceanographic research cruise of the R/V Maurice Ewing were analyzed, in order to evaluate whether informative vegetation histories areas could be reconstructed from nearshore marine cores, for areas where no pollen records were available onshore. The analyses of pollen assemblages from the EW0408-40JC and 47JC cores represent the first attempts to reconstruct coastal terrestrial vegetation histories from marine sediment cores from the Gulf of Alaska region. It had been demonstrated previously that pollen analysis of marine deposits from an anoxic coastal inlet (Saanich Inlet) in southern Vancouver Island, British Columbia yielded high-quality records of coastal temperate rainforest history (Heusser, 1983; Pellatt et al., 2001). At another site in B.C., on the east coast of Moresby Island in Haida Gwaii, early postglacial freshwater lake deposits within an isolation basin in Logan Inlet was later inundated by marine waters as sea level rose. A 12-m long core was obtained from the Logan Inlet site, at a depth of ∼200 m below present sea level (Josenhans et al., 1997). Pollen analysis of the freshwater sediment portion of the Logan Inlet core (between 4 and 12 m depth) yielded a pre-Holocene record spanning ∼14,060–12,300 yr BP (Lacourse and Mathewes, 2005).
The first marine record from SE Alaska to be discussed here is from core EW0408-40JC, located at 56.98573°N, 135.47757°W, in lower Sitka Sound (Figure 1B), 53 km NW of Hummingbird Lake. The jumbo piston core was collected from a water depth of 216 m. The core is 1145 cm in length, and consists of marine lithogenic mud in the upper ∼890 cm, and of freshwater lake muds interbedded with tephras and pyroclastic deposits below 900 cm core depth, representing the lower 245 cm of the core (Addison et al., 2010). The volcanic deposits were derived from multiple eruptions of volcanoes in nearby Kruzof Island’s Mount Edgecumbe volcanic field (MEVF; Figure 1B; Riehle et al., 1992a; Addison et al., 2010). The pollen and spore percentage diagram from 40JC is shown in Figure 9 and Supplementary Table S1. Calibrated radiocarbon ages are also shown in Figure 9. Pollen sums from 40JC samples ranged from 252 to 360 grains, with most sums more than 300 grains, while pollen plus spore sums ranged between 354 and 1049 specimens. Pollen preservation was good to excellent in 40JC samples.
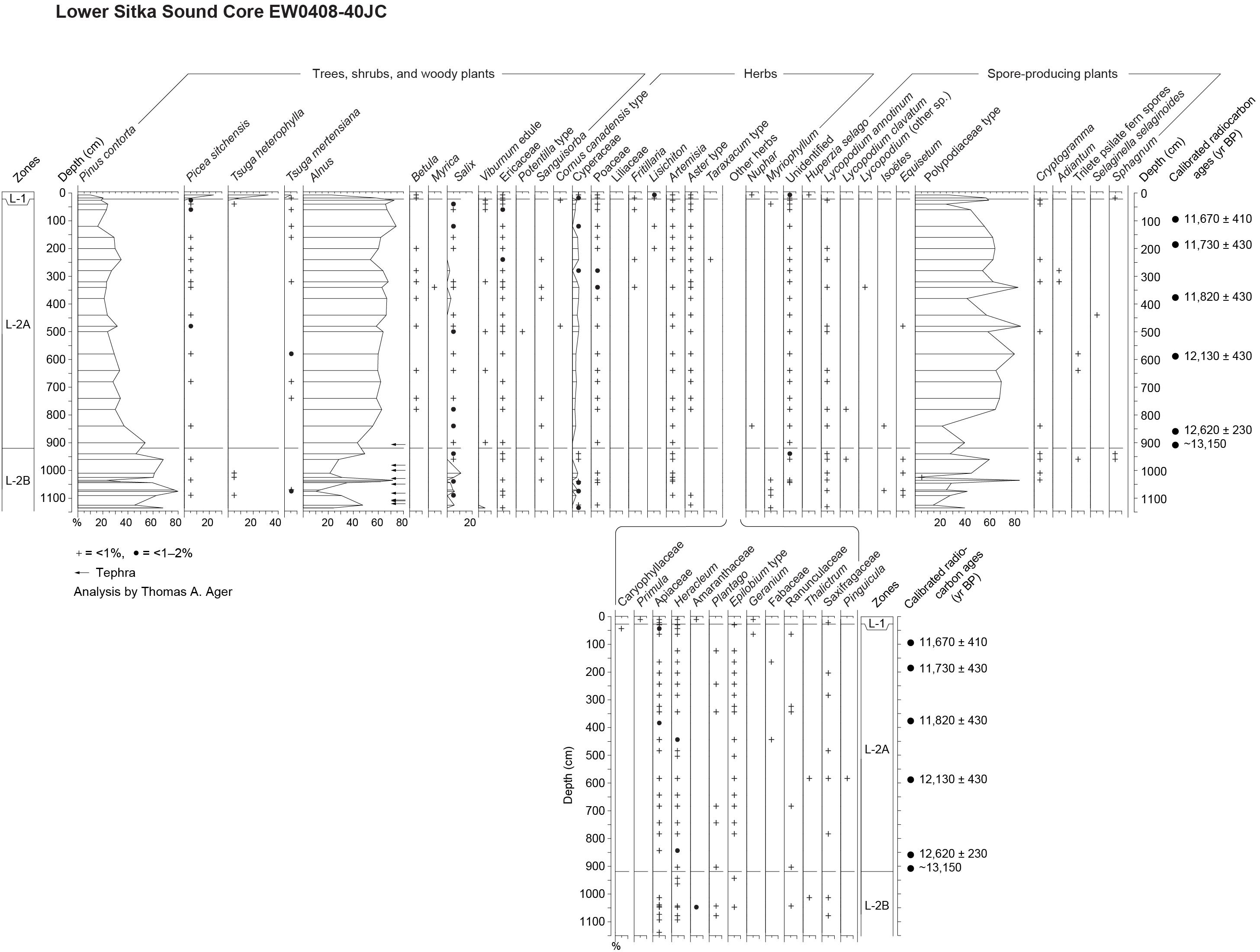
Figure 9. Pollen and spore pollen percentage diagram with calibrated radiocarbon ages for Core EW0408-40 JC in Lower Sitka Sound. Pollen data are shown in Supplementary Table S1.
The base of the 40JC core is undated because of the lack of organic material within the volcanic deposits at the base of the core, but in NE Gulf of Alaska marine cores, tephras consisting largely of lapilli were deposited between ∼14,300 and 13,300 yr BP, and that may represent the approximate age range of multiple early postglacial MEVF eruptions on Kruzof Island (Praetorius and Mix, 2014). The pollen assemblages in the lowermost Subzone L-2B include abundant alder pollen. In the previously described Hummingbird Lake core, alder pollen did not become abundant until after ∼14,040 yr BP, near the base of Zone HL-4. That ∼14,040 yr BP age provides an approximate maximum limiting age for the lowermost pollen samples in the 40JC core, assuming the timing of the alder population increase is about the same in both areas. Alders are prolific pollen producers, and alder pollen is readily transported by wind. Therefore it is unlikely that alder would be largely absent from 40JC’s Subzone L-2B at the same time when alder was abundant at HL.
Sitka Sound was filled with glacial ice during the LGI (Kaufman and Manley, 2004), and therefore the volcanic deposits in the Sitka region must be younger than the time of local deglaciation, which may have been underway by ∼17,000 ± 700 yr BP in the western Alexander Archipelago (Lesnek et al., 2018). In the Sitka area east of Kruzof Island, volcanic ash deposits 1–3 m thick overlie glacial till and bedrock (Yehle, 1974). The volcanic deposits recovered in 40JC do not represent the entire postglacial eruptive sequence, as a longer series of tephras have been described from an undated lake sediment core from Chichagof Island (Riehle et al., 1992b; Addison et al., 2010). The older tephras in the lower part of that Chichagof lake core included a basaltic andesite tephra that does not occur in 40JC, and was produced during an early stage of the eruption sequence (Addison et al., 2010).
Maximum global eustatic sea level lowering during the LGI was ∼125 m below present sea level (Fairbanks, 1989). The freshwater lake sediments discovered within the 40JC core are below 900 cm core depth, and the top of the core is 216 m below modern sea level. Therefore, the top of the freshwater lake sediments is now more than 225 m below present sea level, and ∼100 m below the approximate low stand global eustatic sea level position during the LGI. What remains unknown is the depth of the former lake, and the position and current depth of its paleoshoreline below present sea level, which could be used to calculate the amount of seafloor subsidence more accurately. It is likely that a crustal forebulge developed along the western margins of the Alexander Archipelago during LGI, as a westward lateral migration of mantle rocks responded to the massive weight of the thick ice in the CIS that depressed the Coast Mountains, as has been documented in the Haida Gwaii area (Clague, 1983, 1989). Since deglaciation, isostatic rebound has raised marine shell-bearing deposits up to 220 m along the west flank of the Coast Mountains (Mann, 1986). With rapid deglaciation of the western margins of the CIS and local glaciers ∼17,000 yr BP, the forebulge of mantle material migrated back eastward (Clague, 1983) and caused marine waters to flood the continental shelf in excess of that caused by postglacial eustatic sea level rise alone. This widespread submergence inundated the floor of lower Sitka Sound (Addison et al., 2010). Flooding of the freshwater lake by marine waters in what is now Sitka Sound occurred by ∼12,620 ± 230 yr BP, based on a marine shell date from above the lake sediments. This inundation of freshwater lake deposits in 40JC by rising sea level and crustal adjustments during and after deglaciation is very similar to the situation at the Logan Inlet core near Moresby Island in Haida Gwaii, in terms of present-day water depths, and where both deposits contain pollen and diatom records with overlapping ages with those in 40JC (Josenhans et al., 1997; Lacourse and Mathewes, 2005; Addison et al., 2010). The crustal adjustments during deglaciation included forebulge lateral migration, isostatic rebound, and perhaps major earthquakes caused by nearby fault movements along the Queen Charlotte-Fairweather Fault system on the outer shelf (Carlson et al., 1988). These events may have triggered the multiple volcanic eruptions on Kruzof Island soon after local deglaciation (Addison et al., 2010; Praetorius et al., 2016).
Core 40JC, Pollen Subzone L-2B
Pollen Assemblage Zone L-2B spans the lower part of 40JC from 900 to 1145 cm core depths. The Zone L-2A/L-2B boundary is marked by a dacitic tephra that is correlated with the dacitic tephra that has been dated on western Kruzof Island and Yakobi Island northwest of Chichagof Island (Beget and Motyka, 1998) at ∼13,150 yr BP (calibration range 13,050–13,250 yr BP). The lower end of the Zone L-2B (core base) is not dated, but the abundance of alder pollen within Zone L2B suggests that the core base is no older than the rise of alder pollen percentages in lower Zone H-4 in Hummingbird Lake (∼14,040 yr BP). Freshwater lake sediments (diatomaceous mud) are interbedded with tephras in Subzone L-2B. Freshwater diatoms in the lacustrine sediments in the lower part of 40JC were identified by Scott Starratt at the U.S. Geological Survey in Menlo Park, California (Addison et al., 2010).
The large abrupt shifts in percentages of pine, alder, and fern spores within Zone L-2B, as seen in the 40JC pollen diagram (Figure 9) are likely to be a result of widespread and repeated destruction of vegetation around Kruzof Island and the Sitka Sound coastal areas on Baranof Island during multiple eruptions, some of which may not be represented by tephras preserved in 40JC (Addison et al., 2010). Deposition of tephras at any location depends on the magnitude and duration of the eruptions, distance from the volcano, and wind directions at the time of the event. A total of nine tephras were identified within Subzone L-2B in the 40JC core between 900 cm and the core base at 1145 cm (left-pointing arrows mark positions of tephra layers in the Alnus column in Figure 9). The tephras were described by Addison et al. (2010). There are also dense layers of pyroclastic deposits in the lower part of the core, which probably prevented the heavily weighted jumbo piston corer from penetrating deeper.
Freshwater lake mud deposits from 40JC below ∼900 cm contained pollen and spore assemblages in Zone L-2B that indicate that the vegetation around the Sitka Sound area at the time of the eruptions was pine parkland with abundant alders and ferns, Salix, Ericaceae, Cyperaceae, and herbaceous plants such as Artemisia, Aster, Poaceae, Apiaceae, Heracleum, Plantago, Epilobium, and Saxifragaceae. Shallow water aquatic plants are represented by trace amounts of pollen of Myriophyllum and microspores of Isoëtes. Also present are spores of Lycopodium annotinum and Equisetum. This assemblage is similar, but not identical to Zone HL-4 in the Hummingbird Lake (∼14,040 to ∼11,330 yr BP). The percentages of pollen of pine, alder, and fern spores in HL-4 do not show the large variations as in 40JC’s Zone L-2B. This suggests that the vegetation around Still Harbor was not significantly damaged by tephra deposition during the eruptions from the MEVF, a result of Still Harbor’s remoteness from the volcanoes and its relatively unfavorable direction for wind transport of volcanic ash plumes from its source on Kruzot Island.
Core 40JC, Pollen Subzone L-2A
Pollen Subzone L-2A extends from ∼25 to 900 cm core depth, and spans the time interval between ∼11,400 to ∼13,150 yr BP. The record in this zone is dominated by abundant pollen of alder, pine, and fern spores, an assemblage quite similar to samples in Subzone L-2B, but the percentages of the dominant taxa differ enough to justify defining a separate zone. Pine percentages are generally 20% lower, while alder and fern spore percentages are generally 20–30% higher than in L-2B. Other pollen types that are present, but in low percentages, and include Salix, Ericaceae, Cyperaceae, Poaceae Artemisia, Aster type, Heracleum and other Apiaceae, Plantago, Epilobium type, and other herbs, as well as L. annotinum. All of these plant types are present in the region today and are also present in Pollen Zone HL-4 from Hummingbird Lake. The sediments in Zone L-2B were deposited very rapidly (∼1.68 yr/cm), perhaps related to the subsidence of a crustal forebulge in Sitka Sound during or soon after deglaciation (Addison et al., 2010). The Zone L-2A pollen assemblages are quite similar to those in Hummingbird Lake’s Zone HL-4, as in Zone L-2B. Assemblages are dominated by pollen of alder, somewhat declining pine, and abundant fern spores, and the chronologies of the two zones (HL-4 and L-2A) are quite similar. The abundance of alder pollen and fern spores suggests that the Sitka Sound area vegetation was probably heavily dominated by alder shrublands, in which ferns often grow as an understory.
Core 40JC, Pollen Zone L-1
Only two samples from the top of 40JC (Figure 9, 0–25 cm core depth) appear to be of Holocene age, but are undated, as no wood or shell fragments were found in those core sediments. The uppermost marine sediments at this site were dispersed by the impact of the jumbo piston corer, so some Holocene sediments were not recovered in this core. The abundance of pollen of western hemlock in the uppermost core sample suggests that it may have an age of <9800 yr BP, about the time when western hemlock first became fairly abundant at Hummingbird Lake. The lowermost sample in Zone L-1 contains spruce pollen but little western hemlock pollen, and it is therefore probably of very early Holocene age (∼11,500–10,000 BP). Pollen Zone L-1 samples also contain pollen of mountain hemlock, alder, and abundant fern spores (Polypodiaceae type). Minor taxa (low percentages) in Zone L-1 include Ericaceae, Betula, Cyperaceae, Lysichiton, Artemisia and Aster. The near-absence of Holocene sediments at this coring site may be a result of strong bottom currents, or because of entrapment of sediments in deep fiords on adjacent Baranof Island. The base of Pollen Zone L-1 is at ∼25 cm core depth. Since these early Holocene samples are undated, they are not used for vegetation reconstruction for the Sitka Sound region.
Slocum Arm Core (EW0408-47JC)
The 40JC record from lower Sitka Sound discussed above has only a short, undated Holocene pollen record (two samples near the top of the core). In order to fill in most of the missing marine Holocene record for the far northwestern edge of the Alexander Archipelago, pollen samples were analyzed from marine sediment core EW0408-47JC, collected from near the entrance to Slocum Arm, a fiord on the southern coast of Chichagof Island (Figure 1B). The jumbo piston coring site was located at 57.57546°N, 136.06297°W, at a water depth of 114 m. As with 40JC, core 47JC was collected during the 2004 cruise of the R/V Maurice Ewing, and was subsequently sampled by the author and resampled later by Jason Addison. The core is 1800 cm long, and spans most, but not all of the Holocene. The age of the base of the core is ∼10,000 yr BP. A detailed study of the paleoceanographic record of that core, based primarily on analysis of marine diatom and silicoflagellate assemblages, was reported by Barron et al. (2016). The calibrated AMS radiocarbon chronology reported in that publication is used here in the pollen diagram for 47JC (Figure 10). The chronology is based upon 12 calibrated radiocarbon dates on bivalve fragments, a wood sample, and detrital plant material. The radiocarbon ages of bivalve samples were adjusted for MRE, reducing the reported radiocarbon age by 735 years (Barron et al., 2016) prior to calibrating the dates. Pollen sums from analyzing the 47JC samples for this study ranged from 305 to 358 grains, while pollen plus spore sums ranged from 444 to 937 specimens.
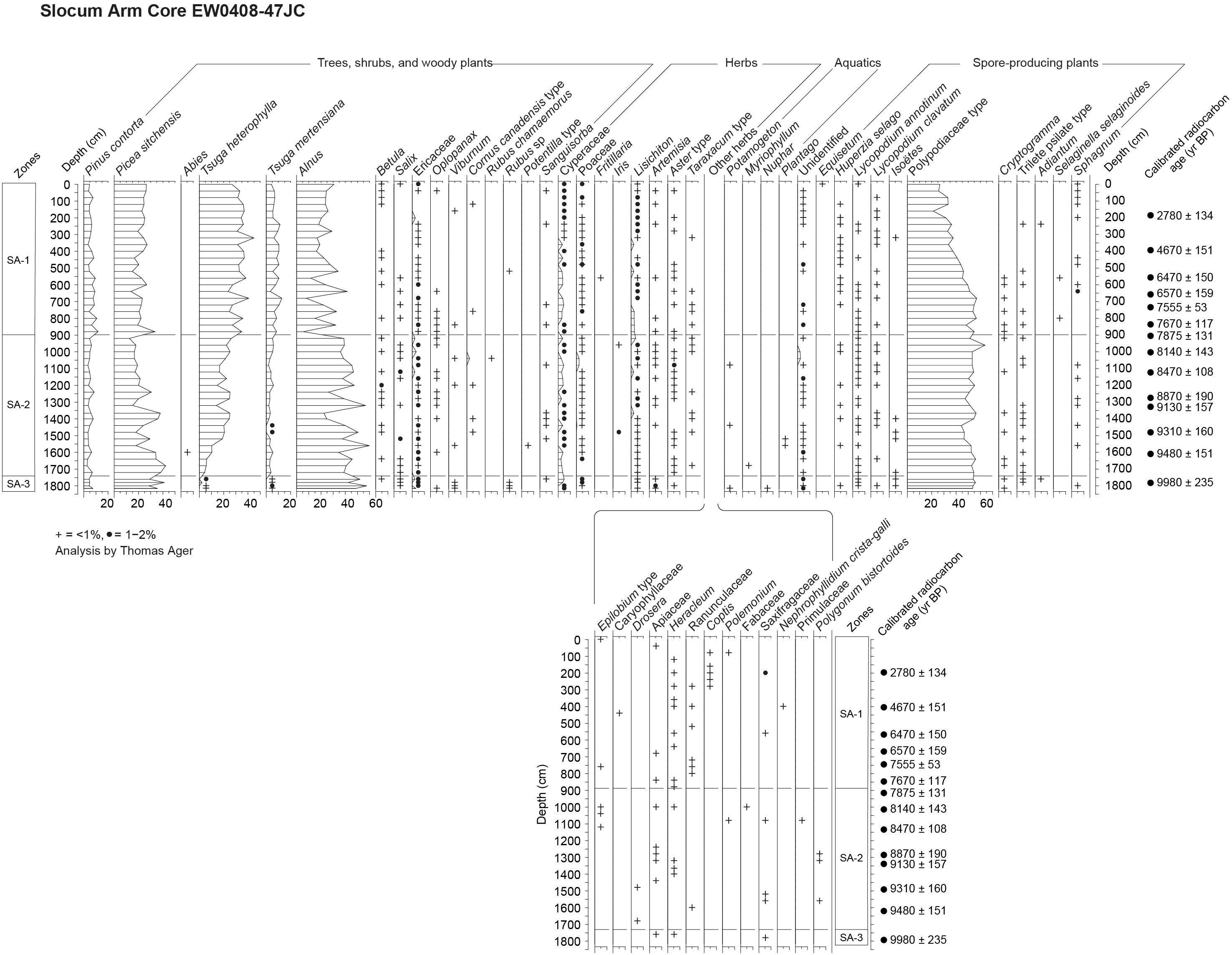
Figure 10. Pollen and spore pollen percentage diagram with calibrated radiocarbon ages for Core EW0408-47 JC in Slocum Arm, southern coast of Chichagof Island. Pollen data are shown in Supplementary Table S1.
Core 47JC, Pollen Zone SA-3
The pollen record from 47JC (Figure 10 and Supplementary Table S1) is divided into three pollen and spore assemblage zones. A core sediment description is provided in Barron et al. (2016). The oldest Pollen Zone SA-3 is ∼10,000 yr BP at the base of the core at 1800 cm core depth, and its upper boundary is at 1740 cm core depth (∼9840 yr BP). That short core interval contains pollen assemblages dominated by pollen of Sitka spruce, alder, and fern spores. At Hummingbird Lake, which has a much longer record than 47JC, the base of the Sitka spruce-alder-fern zone (HL-3) began ∼11,300 yr BP and ended at ∼10,300 yr BP. This indicates that the arrival time of spruce in the Slocum Arm area is unknown, but the spruce dominance lasted longer than at HL. The local increase in spruce pollen in the lake coring site on Pleasant Island lake (Figure 1B) began at ∼10,500 yr BP (Hansen and Engstrom, 1996). Therefore the ∼10,000 yr BP date for the base of the 47 JC core should be considered a minimum age for spruce arrival locally. Pollen types present in low percentages in Zone SA-3 include Pinus, Salix, Ericaceae, Viburnum, Rubus, Cyperaceae, Poaceae, Artemisia, Plantago and other herbs. The abundance of alder pollen and fern spores suggests that the forest was probably more open than the denser western hemlock-Sitka spruce forest that exists today around Slocum Arm.
Core 47JC, Pollen Zone SA-2
Pollen Zone SA-2 extends from 1740 cm up to 900 cm core depth, representing the time interval of ∼9840 to ∼7850 yr BP (Barron et al., 2016). Zone SA-2 records the colonization of the Slocum Arm area by western hemlock, which became the dominant conifer pollen type by ∼8500 yr BP. Zone SA-2 is characterized by abundant pollen of Sitka spruce, western hemlock, alder, and fern spores. Low percentages of pine and mountain hemlock pollen indicate their presence in the area, but their local populations were probably small. Pollen types present in low percentages in SA-2 include Betula, Salix, Ericaceae, Cyperaceae, Poaceae, Lysichiton, and Aster type, plus other herbs.
Core 47JC, Pollen Zone SA-1
Pollen Zone SA-1 extends from 900 cm core depth to the top of core 47JC, representing the time interval of ∼7850 yr BP to near the present day. The upper ∼42 cm of core 47JC is missing from the impact of the piston corer as it penetrated the sediments (Barron et al., 2016). The missing sediments represents ∼54 years (Barron et al., 2016). The pollen assemblages in SA-1 differ little from those in SA-2 in terms of taxa present, but relative abundances of some pollen types are significant enough to differentiate Zone SA-1 from SA-2. Spruce, western hemlock and mountain hemlock pollen percentages are all higher than in upper SA-2. Alder pollen percentages are generally lower than in SA-2, and fern spores decline in abundance within SA-1. These percentage changes suggest that a denser forest (more area with closed canopy and reduced alder shrublands) developed in the area during the past ∼7850 years. Pollen types that are present in low percentages within SA-1 include Betula, Ericaceae, Cyperaceae, Poaceae, Lysichiton, Aster type, and occasional occurrences of other herb taxa. The steep walls of Slocum Arm fiord do not favor the development of extensive muskeg terrain, and therefore limits the area where shore pines can grow. However, pine percentages are somewhat higher in SA-1, so there was apparently some expansion of the pine population after ∼7850 yr BP, perhaps linked to muskeg expansion in areas of low relief on the Khaz Peninsula, which forms the southern side of Slocum Arm fiord.
Discussion
If deglaciation of northwestern Alexander Archipelago occurred ∼17,000 ± 700 yr BP, as indicated for the southwestern archipelago (Lesnek et al., 2018), then there may be an earlier stage in the vegetation history that has yet to be discovered in the Baranof-Chichagof Island area. Such very early, radiocarbon-dated postglacial histories are rare at present in southeastern Alaska, but two are known from widely separated locations: the Pleasant Island lake sediment core in Icy Strait (Figure 1B; ∼15,460 ± 360 yr BP, Hansen and Engstrom, 1996), and a lake sediment core from Heceta Island (Figure 1B; ∼16,700 ± 228 yr BP, Ager, 2007). These two early records indicate that lowland tundra developed in SE Alaska soon after local deglaciation. This tundra vegetation included willows, heaths, including Empetrum (crowberry), as well as sedges, grasses, various herbs, and ferns. Pine pollen occurs in both of these early postglacial tundra-dominated sites, indicating its local presence during or soon after local deglaciation.
The dated pollen records from Hummingbird Lake and the marine cores EW0408-40JC and EW0408-47JC provide the first dated vegetation histories for the far northwest coastal region of the Alexander Archipelago. The HL record serves as the key vegetation history for northwestern Alexander Archipelago. The pollen record from the lower HL core indicates a very early local abundance of pine pollen, and presumably local pine trees as early as ∼15,240 yr BP, several centuries before the onset of the Bølling warm event that began ∼14,700 yr BP, but during a time when sea surface temperatures were already beginning to warm (Davies et al., 2011). This early appearance of pine suggests that the hypothesized refugium on SW Baranof Island, where HL is located, and the adjacent subaerially exposed continental shelf (Carrara et al., 2007), may have supported a pine population during the LGI. Pine-fern-willow-sedge vegetation developed into alder-sedge-pine vegetation during the Allerød, when the pine population declined, with further substantial decline in pine occurring during the Younger Dryas cold event, while alder increased. Spruce and mountain hemlock, with alder and ferns became the dominant regional vegetation during the early Holocene warm event, followed by the colonization of the region by western hemlock by ∼10,000 yr BP. At Hummingbird Lake, pollen evidence indicates that yellow cedar trees colonized NW Alexander Archipelago by ∼5000 yr BP, although its pollen does not appear in the 47 JC Holocene record from Slocum Arm.
Plant Colonization of Deglaciated Terrain
The rapid colonization of southeastern Alaska during and after deglaciation by plants occurred as plant propagules were dispersed across large areas of barren terrain by various means. Spore-producing plants such as mosses, liverworts, fungi, lichens, ferns, and fern allies such as Equisetum, and Lycopodiaceae have a distinct advantage in dispersing their abundant microscopic spores by means of wind, as each spore (unlike pollen) has the potential for developing into a complete plant. Many vascular plants produce seeds adapted to wind transport, including Salix, Populus, Dryas, Epilobium, Eriophorum, Betula, Alnus, and most of the conifers (Pinus, Picea, Tsuga, Chamaecyparis and Abies). During gale-force North Pacific storms, most common in autumn and winter, some seeds that are ordinarily unlikely to be transported far from the parent plant are apparently carried much farther. In general, most conifer seeds are wind transported within 30–60 m of the source trees. Sitka spruce seeds are sometimes transported by wind up to 800 m from the parent trees (Harris, 1990), while western hemlock seeds are known to be transported up to 1600 m, but most hemlock seeds reach the ground within 610 m (Packee, 1990). Seeds of conifers (and other plants) are also wind transported on the surface of snowbeds (Lotan and Critchfield, 1990), and possibly on drifting sea ice. Plants that produce edible fruits and berries are aided in the rapid and relatively long-distance dispersal of their seeds by birds and over shorter distances by mammals. In southeastern Alaska birds and mammals disperse seeds of Empetrum, Vaccinium, Rubus spectabilis, C. canadensis, Ribes, Oplopanax, Sorbus, J. communis, and other plants. Migratory wildfowl feed on aquatic plants and carry seeds from lake to lake along their flight routes (e.g., Nuphar, Potamogeton, Sparganium, Utricularia, Carex). Given that humans have been present in southeastern Alaska and coastal B.C. for over 10,300 years (Dixon, 1999), and perhaps ≥ 13,000 years (Mackie et al., 2018; McLaren et al., 2018), plant propagules may have been transported by boat from island to island, perhaps inadvertently. During high water stages, streams carry plant detritus, including seeds, many of which germinate downstream (Jaques, 1973, and observations in Alaska by the author). Plants adapted to growing along coastal shorelines are apparently able to distribute their propagules through transport in marine waters, as suggested by the widespread distribution of coastal floras all across the Gulf of Alaska and beyond. Examples of such plants found along the coasts of the Gulf of Alaska include Mertensia maritima, Zostera marina, Ruppia spiralis, Honckenya peploides, Stellaria humifusa, Fragaria chiloensis, Potentilla egedii, Lathyrus maritimus, Ligusticum scoticum, Plantago maritima and others (Hultén, 1968). What is currently unknown is whether conifer seeds immersed in salt water will remain afloat, and viable, and if so, for how long? The Alaska Coastal Current flows northward along the continental shelf of southeast Alaska, then turns northwest and west to follow the Gulf of Alaska coast, carrying driftwood logs and rafts of plant detritus, including conifer cones. If seeds can survive such marine transport and deposition by high tides and waves on coastal beaches, it would be a rapid method of dispersal along the western coastline. As pointed out by Peteet (1991), viable conifer seed transport by sea is a testable idea that has yet to be studied experimentally.
The initial colonization of southeastern Alaska by pine trees during and soon after deglaciation raises some interesting questions. How were pines able to spread so rapidly over vast areas of newly deglaciated terrain, when under present-day conditions, they are largely restricted to open muskeg terrain, and are poor competitors where Sitka spruce and western hemlock trees are present? They are intolerant of shade and competition from other plant species (Heusser, 1960; Lotan and Critchfield, 1990), so their seedlings do not survive for long in mixed stands of spruce and hemlocks. In Glacier Bay, in northern southeastern Alaska (Figure 1B), a late Holocene (Little Ice Age) expansion of glaciers filled the entire bay to its entrance in Icy Strait by the mid-1700’s, but since the late 1700s, glaciers have retreated over 100 km and exposed large tracts of barren bedrock, glacial till, and outwash deposits (Stowell, 2006). The conifer forests that survived just beyond the areas that had been buried under ice provided local seed sources for rapid colonization by plants as local ice receded. For more than a century, Glacier Bay has served as an outdoor laboratory for the study of plant succession, starting with recently deglaciated barren terrain of known ages, and involving repeat visits to study areas over decades to record floral and vegetation changes. In most cases, pioneer herbs such as dwarf fireweed, horsetails, willows, and mosses are initial colonizers of recently deglaciated terrain. These are soon followed by alders and cottonwoods, along with or followed by Sitka spruce and western hemlock (e.g., Cooper, 1931, 1939; Lawrence, 1958; Goldthwait et al., 1966). In a very few areas of outer Glacier Bay, pines have been observed to colonize glacial deposits, particularly in outwash sediments near the entrance to the bay (Cooper, 1931). This suggests that shore pines can act as effective colonizers of barren deglaciated terrain, but perhaps only in the local absence, or near absence of competing conifers such as Sitka spruce and western hemlock trees. A lack of competition from other conifer species, along with its apparent advantage of having survived in the region in refugia during the LGI allowed pines to develop pine woodlands over most, if not all of lowland southeastern Alaska during and soon after deglaciation. Figure 11 shows the earliest available calibrated radiocarbon ages for pines across the region. These dates should be considered minimal ages for the local appearance of pines at any given locality.
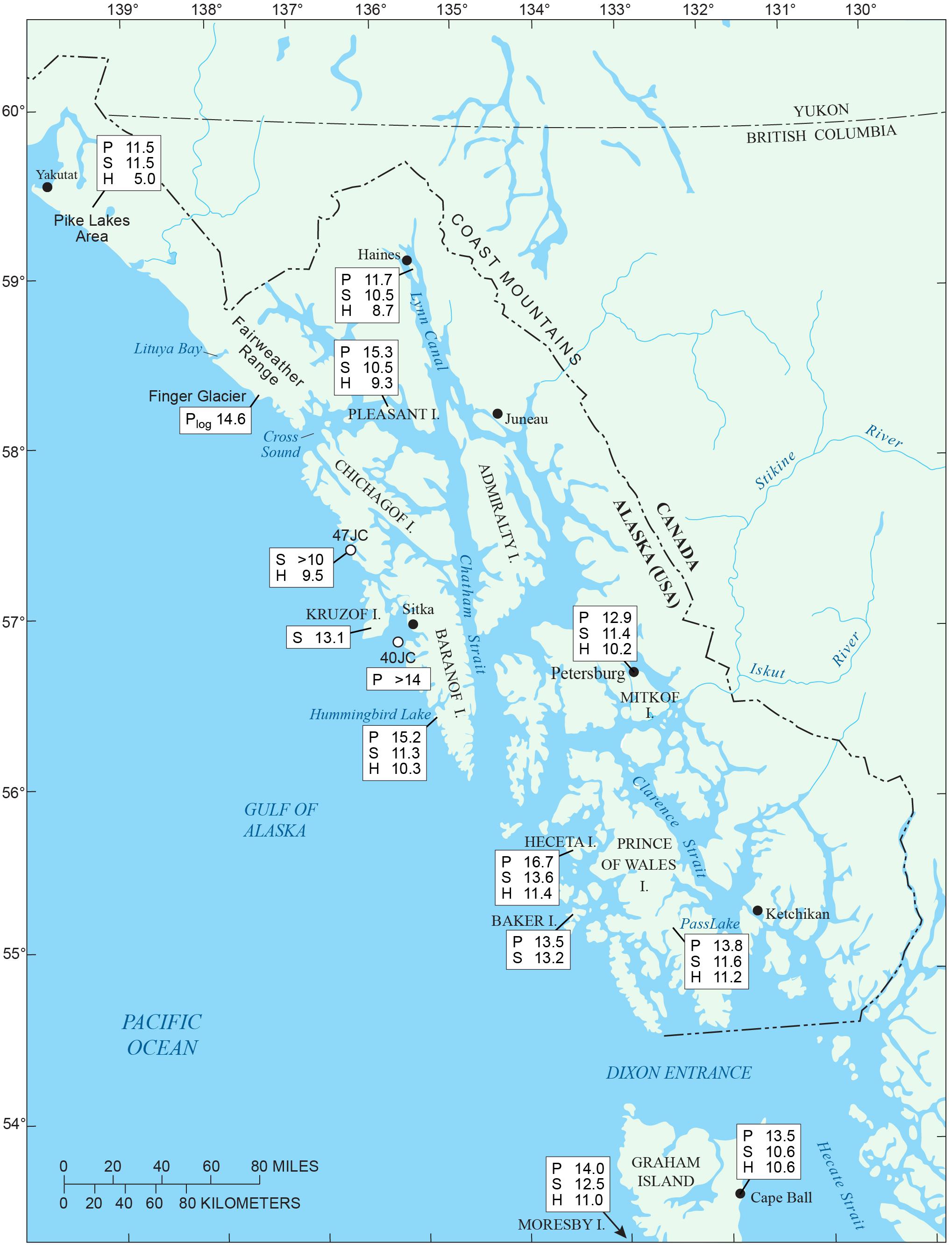
Figure 11. Map of southeastern Alaska showing abbreviated calibrated 14C ages (yr BP × 1000) of earliest occurrences of shore pine: Pinus contorta ssp. contorta (P), Sitka spruce: Picea sitchensis (S), and western hemlock: Tsuga heterophylla (H). References for data sources: Yakutat area cores (Peteet, 1991); Finger Glacier pine log (Mann, 1986); Kruzof Island spruce tree trunk (Baichtal, 2014); 47JC marine sediment core (this paper); 40JC marine and freshwater lake sediment core (this paper); Hummingbird Lake core (this paper); Pleasant Island lake core (Hansen and Engstrom, 1996); Lily Lake core near Haines (Cwynar, 1990); Mitkof Island peat core (Ager et al., 2010); Pass Lake core, Prince of Wales Island (Ager and Rosenbaum, 2009); Baker Island lake core (Wilcox et al., 2016); Heceta Island lake core (Ager, 2007 and unpublished data); Cape Ball coastal exposure, Graham Island, Haida Gwaii (Queen Charlotte Islands) (Warner, 1984; Lacourse and Mathewes, 2005); Moresby Island/Hippa Island sites, Haida Gwaii (Fedje, 1993; Lacourse and Mathewes, 2005; Lacourse et al., 2012).
The history of Sitka spruce colonization in SE Alaska is more difficult to interpret, in that its earliest appearances in pollen records and a few macrofossil sites across much of southeastern Alaska are more varied than one might expect. The oldest occurrences of Sitka spruce pollen first occur in southwestern Alexander Archipelago at ∼13,600 yr BP on Heceta Island (Figures 1B, 11; Ager, 2007), and at ∼13,200 yr BP at nearby Baker Island (Figure 11; Wilcox et al., 2016), assuming these early dates are accurate. This suggests possible refugia for spruce in the SW Alexander Archipelago or on the adjacent continental shelf. At most other sites in the region spruce first appears close to the beginning of the Holocene ∼11,600–11,300 yr BP or soon thereafter (Figure 11). Spruce was somewhat later arriving in the northeast, however, both at Pleasant Island (Figure 1B) and Lily Lake near Haines (Figures 1B, 11) at ∼10,500 yr BP, suggesting a south to north migration from Mitkof Island of 240–300 km over a millennium (Ager et al., 2010), or a shorter west to east (∼80 km) migration from the outer coast (Figure 1B). Farther to the northwest, near Yakutat (Figures 1B, 11), both pollen and macrofossil evidence indicate that spruce arrived in that area about 11,500 yr BP (Peteet, 1991), while at Hummingbird Lake and Sandy Bay on the SW coast of Baranof Island (Figure 1B), pollen and seed cones indicate that spruce was locally present at about the same time (∼11,300–11,500 yr BP). At Pass Lake on Prince of Wales Island (Figure 1B), pollen evidence suggests that spruce arrived there ∼11,500 yr BP (Ager and Rosenbaum, 2009).
Two spruce macrofossil records from northern Alexander Archipelago and Glacier Bay (Figure 1B) are seemingly out of place when compared with the available pollen records from the region. At Adams Inlet in Glacier Bay, a fossil seed cone identified as spruce yielded an unusually old date of ∼13,070 ± 370 yr BP. The cone was collected from an exposure of the early postglacial Forest Creek Formation (McKenzie and Goldthwait, 1971). If the cone was correctly identified, it would be a surprising discovery, given the absence of fossil spruce pollen in the region until about 3000 years later. A younger sample from the same Forest Creek Formation dated to ∼12,900 yr BP, and yielded a fossil pollen assemblage that included pine and alder, along with other shrubs and herbs, but with no spruce pollen. The absence of spruce pollen in that dated sample casts doubt upon the original identification of the older seed cone. The other report of early spruce in northern Alexander Archipelago is from a charred standing tree trunk buried by pyroclastic deposits on the southern coast of Kruzof Island. The charred wood was identified as spruce and was dated to ∼13,100 ± 91 cal yr BP (Baichtal, 2014). It is possible that spruce persisted in southeastern Alaskan refugia during the LGI, perhaps in small scattered populations, and perhaps producing little or no pollen for long intervals until climatic conditions more favorable for sexual reproduction developed. Fossil evidence in support of an early population of spruce producing little pollen has been reported from Hippa Island in Haida Gwaii (Lacourse et al., 2012) where spruce stomata derived from decomposed spruce needles are present 14,000–13,800 yr BP in samples containing very low percentages of spruce pollen. Also present were stomata of other conifer species that had not yet shown up in the pollen record (western hemlock, and red cedar (T. plicata). It is not yet understood how conifers are able to survive and persist without producing much, if any pollen for sexual reproduction (e.g., Peteet, 1991), although many conifers can reproduce vegetatively by layering of lower branches in contact with soil, as has often been observed near altitudinal and latitudinal treelines. Rapid expansion of spruce across much of SE Alaska during the early Holocene suggests that a climate threshold may have been crossed that allowed scattered in situ populations to quickly expand across the region. The early Holocene warming reported from marine records (Praetorius et al., 2015) and from studies of tree line fluctuations (Pellatt and Mathewes, 1997) may have been a triggering event for regionwide spruce expansion from scattered populations.
The calibrated radiocarbon dates that record the colonization of southeastern Alaska and Haida Gwaii by western hemlock during the early Holocene are more consistent than the postglacial spread of spruce when plotted on a map (Figure 11), with somewhat older dates for initial occurrences in the south, and with younger ages to the north, suggesting northward migration from southern sources. Summer temperatures that favor western hemlock reproduction and seedling survival tend to be warmer (≥11.3°C mean July temperature: Packee, 1990) than for Sitka spruce (∼8°C MJT: Heusser, 1989), and mountain hemlock (MJT probably ∼8°C). Western hemlock seedlings survive most often where alders and other nitrogen-fixing plants precede its arrival, adding nitrogen to soil and producing some organic material in the soil which provide a more favorable seed bed (Lawrence, 1958; Goldthwait et al., 1966). These factors of temperature and soil conditions may explain why western hemlock trees colonized southeastern Alaska centuries or more after Sitka spruce and long after alder shrubs were locally established.
In order to place the new records of late Quaternary vegetation history of NW Alexander Archipelago into a broader regional context, a comparison chart summarizing vegetation histories from the oldest, well-dated pollen records from the Alexander Archipelago, southeastern Alaska is shown in Figure 12. An additional comparison chart (Figure 13) summarizes vegetation histories from key sites in Haida Gwaii.
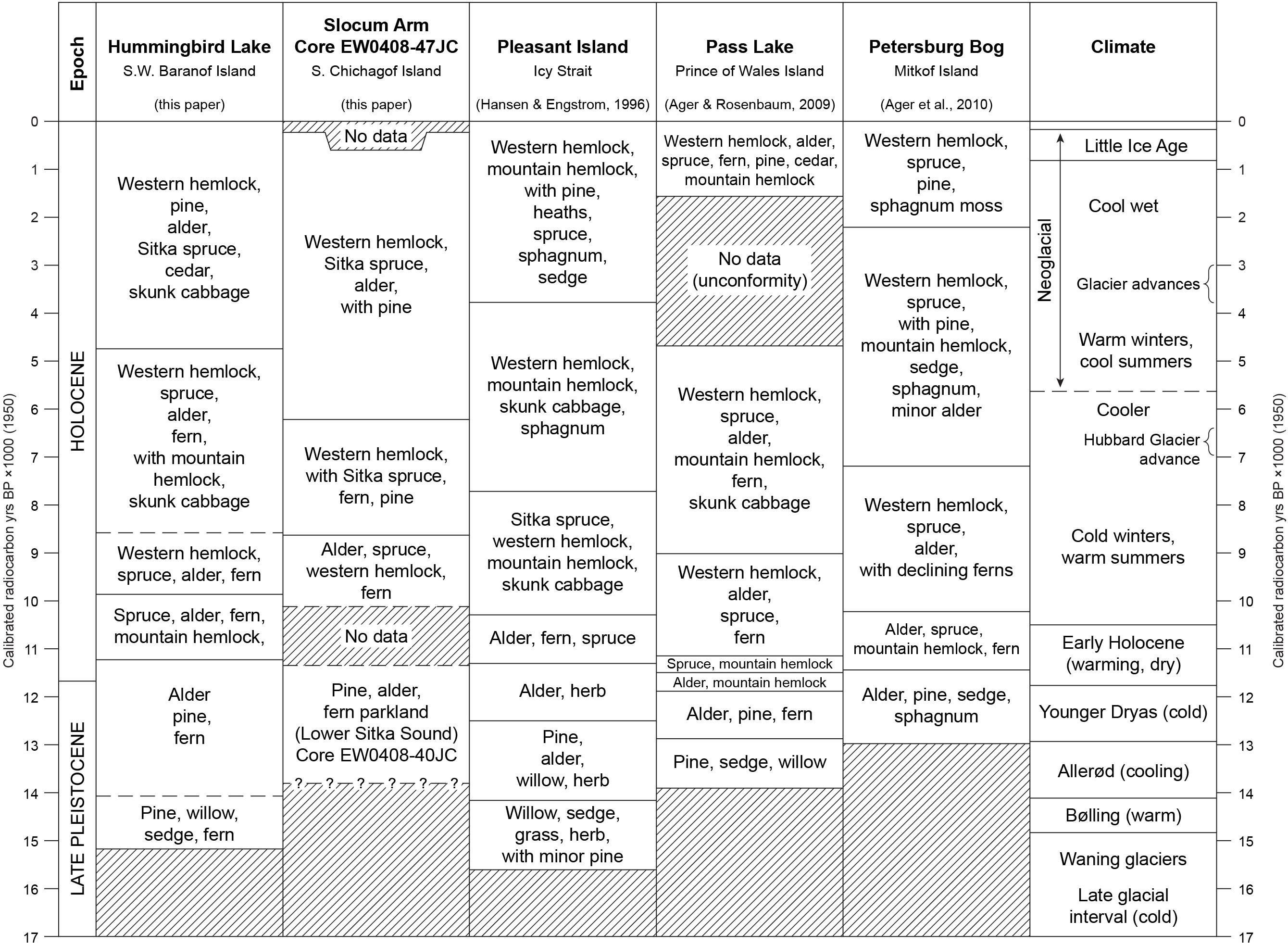
Figure 12. Comparison chart of key vegetation histories from the Alexander Archipelago and vicinity, southeastern Alaska.
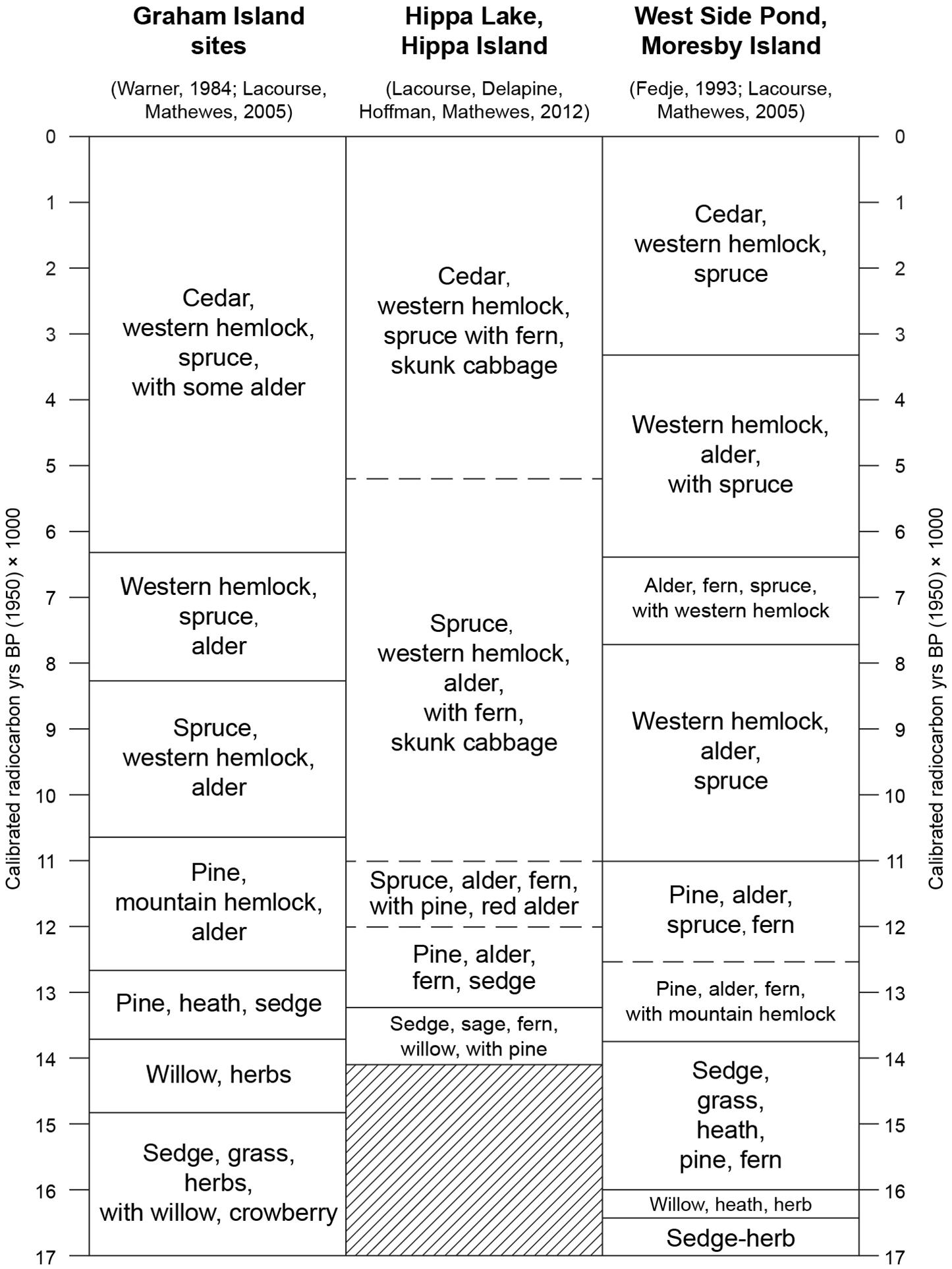
Figure 13. Comparison chart of key vegetation histories from Haida Gwaii (Queen Charlotte Islands) in northern coastal British Columbia, Canada.
Conclusion
Deglaciation along the western edge of the Alexander Archipelago was underway by 17,000 ± 700 yr BP, and soon thereafter plants began to colonize newly exposed lands as the ice retreated to the east. Spore and seed sources for colonization are likely to have included refugia for plants along the western margins of the Alexander Archipelago and the adjacent inner continental shelf that had been subaerially exposed by eustatic sea level lowering during glaciation. Plants also spread from sources along the coasts of British Columbia and from areas of vegetated exposed B.C.’s continental shelf. Stages in colonization include early postglacial lowland herb-shrub tundra (known so far from only from two sites in SE Alaska), followed by pine parkland with ferns, shrubs, and herbs. The high percentages of pine pollen in sediments up to ∼15,240 yr BP at Hummingbird Lake suggest that pines were well established very early at that site, even before the onset of the Bølling warming that began ∼14,700 yr BP. This suggests that pine trees survived during the LGI in the local hypothesized refugium on the SW coast of Baranof Island and adjacent shelf (Carrara et al., 2003, 2007). Biological evidence for the existence of refugia in the western Alexander Archipelago and Haida Gwaii and adjacent shelf areas is strong, but as yet no suspected continuously ice-free refugium has been proven by pollen or other evidence dating to the LGI.
Pine parklands all across SE Alaska declined as alder shrub populations expanded during the Allerød and Younger Dryas. During the early Holocene, Sitka spruce populations expanded over most of SE Alaska, although fossil evidence indicates that earlier populations appear have existed in SW Alexander Archipelago and Haida Gwaii to the south. Western hemlock trees spread across SE Alaska centuries or more after the establishment of Sitka spruce populations. Western hemlocks soon became the dominant conifer tree species in the region, as it dominates most of the conifer forests today. Plant colonization may still be underway, as suggested by the presence of more tree species in coastal B.C. and southern Alexander Archipelago than in the north, although climatic limitations imposed by increasing latitude may explain some of this varied distribution of species. During the late Holocene, under generally wetter and cooler climates, the modern dense temperate conifer forests of SE Alaska developed, during which Cupressaceae (yellow cedar in the north, and yellow and red cedar in southern SE Alaska) became well established. Muskeg vegetation also expanded in the late Holocene, at the expense of dense conifer forests.
Early Human Colonists in Southeastern Alaska
The western margins of the Alexander Archipelago were undergoing deglaciation by 17,000 ± 700 yr BP (Lesnek et al., 2018), but paleoceanographic evidence from the north-central coast of the GOA indicates the persistence of glaciers on the continental shelf, with calving icebergs, and sea ice for many months of the year (Méheust et al., 2018) until the beginning of the Bølling warm interval ∼14,700 yr BP. Large glaciers on the shelf along the northern coast would have posed a challenging obstacle for boat travel along that coast until after the onset of Bølling-Allerød (BA) warming (Davies et al., 2011). Coastal conditions during the Younger Dryas cooling would have been less favorable for human occupation, but the warmer early Holocene would have been more favorable for humans, as ocean productivity was relatively high, and with terrestrial ecosystems rapidly increasing in diversity of plants and animals. Vertebrate fossil assemblages from caves indicate that both marine and terrestrial mammals were present in southeastern Alaska before and during the BA and through the Holocene, along with fish, and birds, but the faunas changed through time in response to climatic and ecosystem changes both onshore and offshore (Heaton and Grady, 2003).
Human colonization of southeastern Alaska occurred at least as early as shortly before the beginning of the Holocene: e.g., Ground Hog Bay site east of Glacier Bay (Ackerman et al., 1979); Hidden Falls site, northeastern Baranof Island (Davis, 1996) and Shuká Káa [On Your Knees Cave]: Dixon (1999, 2001). Earlier human presence, perhaps ≥ 13,000 yr BP, is suggested by the recent discovery of dated human footprints preserved in buried beach sands from Calvert Island in central coastal B.C. and archeological evidence from other B.C. coastal sites (Mackie et al., 2018; McLaren et al., 2018). Numerous early Holocene archeological sites have been found recently in southern Alexander Archipelago (Carlson and Baichtal, 2015), indicating that a marine-adapted human population was well established in the region by that time. The paleoecological evidence from NW Alexander Archipelago suggests that pine parkland was well developed in that area at least as early as ∼15,240 yr BP. Pine and mountain hemlock trees would have provided potential sources of fuel, shelter, resin and perhaps material for boat building for human colonists, along with smaller wood from willow shrubs, and after about 14,000 yr BP, alder wood and bark. By earliest Holocene time, Sitka spruce was colonizing SE Alaska rapidly, providing another source of wood products, fiber, resin, and other products for human use.
Author Contributions
TA conceived the project, collected the samples, analyzed the pollen samples, developed and interpreted the data, and wrote the manuscript.
Funding
This research was supported by the U.S. Geological Survey Land Change Science Program.
Conflict of Interest Statement
The author declares that the research was conducted in the absence of any commercial or financial relationships that could be construed as a potential conflict of interest.
Acknowledgments
Field investigations within Tongass National Forest were made possible through the cooperation and logistical support of the U.S. Forest Service, and the collaboration of Forest Service (FS) scientists Jim Baichtal and Michael Shephard during the 2000 summer field season, and Mary Stensvold during the 2007 and 2008 visits to Sitka. The USGS-FS field party that assisted in carrying loads and sampling Hummingbird Lake and Sandy Bay bog on SW Baranof Island included Larry Phillips, Paul Carrara, and Josh Beene (all from USGS) and Jim Baichtal and Michael Shephard (FS). The author especially thanks the U.S. Forest Service, Tongass National Forest, for arranging for boat transport to reach the remote SW coast of Baranof Island during a circumnavigation of Baranof Island aboard the Chugach Ranger, an historic wooden Forest Service vessel captained by Bob Kinville during the 2000 summer field season. John Westgate, University of Toronto, analyzed two tephra samples from Hummingbird Lake and one from Sandy Bay Bog (Addison et al., 2010). Ager’s participation in the 2004 oceanographic research cruise of the R/V Maurice Ewing was at the invitation of Alan Mix, Oregon State University (OSU). Marine sediment cores obtained during the cruise were archived at the OSU marine core repository in Corvallis. Sampling of selected cores by the author during visits to the OSU core facility was expedited by core curators Bobbi Conard and Misti Weber. Pollen samples from Hummingbird Lake, Core EW0408-40JC and Core EW0408-47JC were processed in the U.S. Geological Survey’s Cenozoic Palynology Laboratory in Denver, Colorado by Jeff Honke. Pollen diagrams were prepared by Rebecca Brice (USGS, Denver). Figures were prepared by computer graphics specialist Jeremy Havens. Samples of plant material and shell fragments collected from the three cores discussed in this paper were prepared for AMS radiocarbon dating at the U.S. Geological Survey Radiocarbon Laboratory in Reston, Virginia, by John McGeehin and his staff. AMS dating of those samples was done at the National Science Foundation-Arizona AMS facility in Tucson. Calibrations for the Hummingbird Lake radiocarbon samples were provided by Jeff Pigati (USGS Denver). The author also expresses appreciation to the reviewers Laura Strickland, RM, LW, and constructive comments from guest editor DP, and Specialty Chief Editor Steven L. Forman. Any use of trade, firm, or product names is for descriptive purposes only and does not imply endorsement by the U.S. Government.
Supplementary Material
The Supplementary Material for this article can be found online at: https://www.frontiersin.org/articles/10.3389/feart.2019.00104/full#supplementary-material
TABLE S1 | Raw pollen and spore counts for samples analyzed from Hummingbird Lake (Baranof Island), Core EW0408-40JC (Lower Sitka Sound), and Core EW0408-47JC (Slocum Arm Fiord, Chichagof Island, Alaska. Percentages calculated from these counts were used to construct the pollen and spore diagrams.
References
Aaby, B., and Digerfeldt, G. (1986). “Sampling techniques for lakes and bogs,” in Handbook of Holocene Palaeoecology and Palaeohydrology, ed. B. E. Berglund (Chichester: John Wiley & Sons), 181–194.
Ackerman, R. E., Hamilton, T. D., and Stuckenrath, R. (1979). Early cultural complexes on the Northwest Coast. Can. Jour. Archaeol. 3, 195–209.
Addison, J. A., Beget, J. E., Ager, T. A., and Finney, B. P. (2010). Marine tephrochronology of the Mt. Edgecumbe volcanic field, southeast Alaska, U.S.A. Quat. Res. 73, 277–292. doi: 10.1016/j.yqres.2009.10.007
Ager, T. A. (2007). Vegetation development on Heceta Island, southeastern Alaska during the Late Glacial and Holocene. Geol. Soc. Amer. Abstr. Prog. 39:17.
Ager, T. A., Carrara, P. E., Smith, J. L., Anne, V., and Johnson, J. (2010). Postglacial vegetation history of Mitkof Island, Alexander Archipelago, southeastern Alaska. Quat. Res. 73, 259–268. doi: 10.1016/j.yqres.2009.12.005
Ager, T. A., and Rosenbaum, J. G. (2009). “Late Glacial-Holocene pollen-based vegetation history from Pass Lake, Prince of Wales Island, Southeastern Alaska,” in Studies by the U.S. Geological Survey in Alaska, 2007. U.S. Geological Survey Professional Paper 1760-G, eds P. J. Haeussler and J. P. Galloway, 1–19.
Alaback, P., and Pojar, J. (1997). “Vegetation from Ridgetop to Seashore,” in The Rain Forests of Home: Profile of a North American Bioregion, eds P. K. Schoonmaker, B. von Hagen, and W. C. Wolf (Washington, D.C: Island Press), 69–87.
Baichtal, J. F. (2014). The Buried Forest of Alaska’s Kruzof Island: A Window Into the Past. United States Department of Agriculture Blog Archives. Available at: https://www.usda.gov/media/blog/2014/03/07/buried-forest-alaskas-kruzof-island-window-past (accessed May 13, 2019).
Barrie, J. V., Conway, K. M., Mathewes, R. W., Josenhans, H. W., and Johns, M. J. (1994). Submerged late Quaternary terrestrial deposits and paleoenvironment of northern Hecate Strait, British Columbia continental shelf, Canada. Quat. Internat. 20, 123–129. doi: 10.1016/1040-6182(93)90041-d
Barrie, J. V., and Conway, K. W. (1999). Late Quaternary glaciation and postglacial stratigraphy of the northern Pacific margin of Canada. Quat. Res. 51, 113–123. doi: 10.1006/qres.1998.2021
Barron, J. A., Bukry, D., Addison, J. A., and Ager, T. A. (2016). Holocene evolution of diatom and silicoflagellate paleoceanography in Slocum Arm, a fjord in southeastern Alaska. Mar. Micropaleontol. 126, 1–18. doi: 10.1016/j.marmicro.2016.05.002
Barron, J. A., Bukry, D., Dean, W. E., Addison, J. A., and Finney, B. (2009). Paleoceanography of the Gulf of Alaska during the past 15,000 years: Results from diatoms, silicoflagellates, and geochemistry. Mar. Micropaleontol. 72, 176–195. doi: 10.1016/j.marmicro.200904.006
Beget, J. E., and Motyka, R. J. (1998). New dates on Late Pleistocene dacitic tephra from the Mount Edgecumbe volcanic field, southeastern Alaska. Quat. Res. 49, 123–125. doi: 10.1006/qres.1997.1945
Blaise, B., Clague, J. J., and Mathewes, R. W. (1990). Time of maximum late Wisconsin glaciation, west coast of Canada. Quat. Res. 34, 282–295. doi: 10.1016/0033-5894(90)90041-i
Briner, J. P., Tulenko, J. P., Kaufman, D. S., Young, N. E., Baichtal, J. F., and Lesnek, A. (2017). The last deglaciation of Alaska. Cuad. Invest. Geográfica 43, 429–448.
Brown, C. A. (2008). Palynological Techniques, 2nd Edn. Dallas, TX: Amer. Assoc. of Stratigraphic Palynologists Foundation.
Brown, T. A., Nelson, D. E., Mathewes, R. W., Vogel, J. S., and Southon, J. R. (1989). Radiocarbon dating of pollen by accelerator mass spectrometry. Quat. Res. 32, 205–212. doi: 10.1016/0033-5894(89)90076-8
Carlson, P. R., Bruns, T. R., and Plafker, G. (1988). Late Cenozoic offsets on the offshore connection between the Fairweather and Queen Charlotte faults off southeast Alaska. Mar. Geol. 85, 89–97. doi: 10.1016/0025-3227(88)90085-0
Carlson, R. J., and Baichtal, J. F. (2015). A predictive model for locating early holocene archaeological sites based on raised shell-bearing strata in Southeast Alaska. Geoarchaeology 30, 120–138. doi: 10.1002/gea.21501
Carrara, P. E., Ager, T. A., and Baichtal, J. F. (2007). Possible refugia in the Alexander Archipelago of southeastern Alaska during the late Wisconsin glaciation. Can. J. Earth Sci. 44, 229–244. doi: 10.1139/e06-081
Carrara, P. E., Ager, T. A., Baichtal, J. F., and Van Sistine, D. P. (2003). Map of Glacial Limits and Possible Refugia in the Southern Alexander Archipelago, Alaska During the Late Wisconsin Glaciation. United States Geological Survey Misc. Field Studies, Map 2424.
Clague, J. J. (1983). “Glacio-isostatic effects of the Cordilleran Ice Sheet, British Columbia, Canada,” in Shorelines and Isostasy, eds D. E. Smith and A. G. Dawson (London: Academic Press), 321–343.
Clague, J. J. (1989). “Quaternary geology of the Canadian Cordillera,” in Quaternary Geology of Canada and Greenland: Geology of Canada 1, ed. R. J. Fulton (Ottawa: Geological Survey of Canada), 17–96.
Cooper, W. S. (1931). A third expedition to Glacier Bay, Alaska. Ecology 12, 61–95. doi: 10.2307/1932934
Cooper, W. S. (1939). A fourth expedition to Glacier Bay, Alaska. Ecology 20, 130–155. doi: 10.2307/1930735
Cwynar, L. C. (1990). A late Quaternary vegetation history from Lily Lake, Chilkat Peninsula, southeast Alaska. Can. Jour. Bot. 68, 1106–1112. doi: 10.1139/b90-139
Davies, M. H., Mix, A. C., Stoner, J. S., Addison, J. A., Jaeger, J. A., Finney, B., et al. (2011). The deglacial transition on the southeastern Alaskan margin: meltwater input, marine productivity, and sedimentary anoxia. Paleoceanography 26:A2233. doi: 10.1029/2010PA002051
Davis, S. D. (1996). “Hidden Falls,” in American Beginnings: The Prehistory and Palaeoecology of Beringia, ed. F. H. West (Chicago: University of Chicago Press), 413–424.
de Vernal, A., and Pedersen, T. F. (1997). Micropaleontology and palynology of core PAR87A-10: A 23,000 year record of paleoenvironmental changes in the Gulf of Alaska, northeast Pacific. Paleoceanography 12, 821–830. doi: 10.1029/97PA02167
Dixon, E. J. (1999). Bones Boats & Bison: Archeology and the First Colonization of Western North America. Albuquerque: University of New Mexico Press.
Dixon, E. J. (2001). Human colonization of the Americas: timing, technology, and process. Quat. Sci. Rev. 20, 277–299. doi: 10.1016/s0277-3791(00)00116-5
Dixon, E. J., Heaton, T. H., Lee, C. M., Fifield, T. E., Coltrain, J. B., Kemp, B. M., et al. (2014). “Evidence of maritime adaptation and coastal migration from Southeast Alaska,” in Kennewick Man: The Scientific Investigation of an Ancient American Skeleton, eds D. W. Owsley and R. L. Jantz (Texas: Texas A&M University Press).
Doher, L. I. (1980). Palynomorph Preparation Procedures Currently used in Paleontology and Stratigraphy Laboratories. United States Geological Survey Circular 830.
Fairbanks, R. G. (1989). A 17,000-year glacio-eustatic sea level record – influence of glacial melting rates on the Younger Dryas event and deep ocean circulation. Nature 342, 637–642. doi: 10.1038/342637a0
Fedje, D. W. (1993). Sea-Levels and Prehistory in Gwaii Haanas. Ph.D. thesis, University of Calgary, Calgary.
Fedje, D. W., and Josenhans, H. (2000). Drowned forests and archaeology on the continental shelf of British Columbia. Can. Geol. 28, 99–102. doi: 10.1130/0091-7613(2000)028
Goldthwait, R. P., Loewe, F., Ugolini, F. C., Decker, H. F., DeLong, D. M., Troutman, M. B., et al. (1966). Soil Development and Ecological Succession in a Deglaciated Area of Muir Inlet, Southeast Alaska. Columbus: Ohio State University Institute of Polar Studies.
Grimm, E. (1991). TILIAGRAPH 2.0.b.5 (Computer Software). Springfield, IL: Illinois State Museum, Research and Collections Center.
Grimm, E. (1993). TILIA v. 2.0 (Computer Software). Springfield, IL: Illinois State Museum, Research and Collections Center.
Hansen, B. C. S. (1995). Conifer stomate analysis as a paleoecological tool: an example from the Hudson Bay Lowlands. Can. Jour. Bot. 73, 244–252. doi: 10.1139/b95-027
Hansen, B. C. S., and Engstrom, D. R. (1996). Vegetation history of Pleasant Island, southeastern Alaska, since 13,000 yr BP. Quat. Res. 46, 161–175. doi: 10.1006/qres.1996.0056
Harris, A. S. (1990). “Picea sitchensis (Bong.) Carr.: Sitka Spruce,” in Silvics of North America, Vol. 1, eds R. M. Burns and B. H. Honkala (Washington, D.C: U.S. Dept. of Agriculture Forest Service), 260–267.
Heaton, T. H., and Grady, F. (2003). “The late Wisconsin vertebrate history of Prince of Wales Island, southeast Alaska,” in Ice Age Cave Faunas of North America, eds B. W. Schubert, J. I. Mead, and R. W. Graham (Bloomington: Indiana University Press), 17–53.
Heaton, T. H., Talbot, S. L., and Shields, G. F. (1996). An ice age refugium for large mammals in the Alexander Archipelago, southeastern Alaska. Quat. Res. 46, 186–192. doi: 10.1006/qres.1996.0058
Hetherington, R., Barrie, J. V., Reid, R. G. B., MacLeod, R., and Smith, D. J. (2004). Paleogeography, glacially induced crustal displacement, and late Quaternary coastlines on the continental shelf of British Columbia. Can. Quat. Sci. Rev. 23, 295–318. doi: 10.1016/j.quascirev.2003.04.001
Hetherington, R., and Reid, R. G. B. (2003). Malacological insights into the marine ecology and changing climate of the late Pleistocene-early Holocene Queen Charlotte Islands archipelago, western Canada and implications for early humans. Can. Jour. Zool. 81, 626–661. doi: 10.1139/z03-024
Heusser, C. J. (1952). Pollen profiles from southeastern Alaska. Ecol. Monog. 22, 331–352. doi: 10.2307/1948473
Heusser, C. J. (1954). Additional pollen profiles from southeastern Alaska. Amer. Jour. Sci. 252, 106–119. doi: 10.2475/ajs.252.2.106
Heusser, C. J. (1960). Late Pleistocene Environments of North Pacific North America. New York, NY: American Geographical Society.
Heusser, C. J. (1965). “A Pleistocene sketch of the Pacific Northwest and Alaska,” in The Quaternary of the United States, eds H. E. Wright Jr. and D. G. Frey (Princeton: Princeton University Press), 469–483.
Heusser, C. J. (1985). “Quaternary pollen records from the Pacific Northwest Coast: Aleutians to the Oregon-California boundary,” in Pollen Records of Late-Quaternary North American Sediments, eds V. M. Bryant Jr. and R. G. Holloway (Dallas: American Association of Stratigraphic Palynologists Foundation), 141–165.
Heusser, C. J. (1989). “North Pacific coastal refugia – the Queen Charlotte Islands in perspective,” in The Outer Shores, eds G. G. Scudder, N. Gessler, and B. C. Skidegate (Washington, D.C: Islands Museum Press), 91–106.
Heusser, C. J., Heusser, L. E., and Peteet, D. M. (1985). Late-quaternary climate change on the American North Pacific coast. Nature 315, 485–487. doi: 10.1038/315485a0
Heusser, L. E. (1983). Palynology and paleoecology of postglacial sediments in an anoxic basin, Saanich Inlet, British Columbia. Can. J. Earth Sci. 20, 873–885. doi: 10.1139/e83-077
Holloway, R. G. (1989). “Analysis of botanical materials,” in The Hidden Falls Site: Baranof Island, Alaska, ed. S. D. Davis (Anchorage, AK: Anthropological Assoc. Monograph Series), 61–92.
Hultén, E. (1968). Flora of Alaska and Neighboring Territories. Stanford: Stanford University Press.
Jaques, D. R. (1973). Reconnaissance Botany of Alpine Ecosystems of Prince of Wales Island, Southeast Alaska. master’s thesis, University of Oregon, Eugene, OR.
Josenhans, H., Fedje, D., Pienitz, R., and Southon, J. (1997). Early humans and rapidly changing Holocene sea levels in the queen Charlotte Islands - Hecate Strait, British Columbia, Canada. Science 277, 71–74. doi: 10.1126/science.277.5322.71
Kaufman, D. S., and Manley, W. F. (2004). “Pleistocene maximum and late Wisconsin glacier extents across Alaska, U.S. A,” in Quaternary Glaciations – Extent and Chronology. Part II. North America: Developments in Quaternary Science, 2, eds J. Ehlers and P. I. Gibbard (Amsterdam: Elsevier), 9–27. doi: 10.1016/s1571-0866(04)80182-9
Lacourse, T. (2005). Late Quaternary dynamics of forest vegetation on northern Vancouver Island, British Columbia, Canada. Quat. Sci. Rev. 24, 105–121. doi: 10.1016/j.quascirev.2004.05.008
Lacourse, T., Beer, K. W., and Hoffman, E. H. (2016). Identification of conifer stomata in pollen samples from western North America. Rev. Palaeobot. Palynol. 232, 140–150. doi: 10.1016/j.revpalbo.2016.05.005
Lacourse, T., Delepine, J. M., Hoffman, E. H., and Mathewes, R. W. (2012). A 14,000 year vegetation history of a hypermarine island on the outer Pacific coast of Canada based on fossil pollen, spores and conifer stomata. Quat. Res. 78, 572–582. doi: 10.1016/j.qres.2012.08.008
Lacourse, T., and Mathewes, R. W. (2005). “Terrestrial paleoecology of the Queen Charlotte Islands and the continental shelf: vegetation, climate, and plant resources of the coastal migration route,” in Haida Gwaii: Human History and Environment From the Time of Loon to the Time of the Iron People, eds D. W. Fedje and R. W. Mathewes (Vancouver, B.C: Univ. of British Columbia Press), 38–58.
Lacourse, T., Mathewes, R. W., and Fedje, D. W. (2003). Paleoecology of late-glacial terrestrial deposits with in situ conifers from the submerged continental shelf of western Canada. Quat. Res. 60, 180–188. doi: 10.1016/s0033-5894(03)00083-8
Lesnek, A. J., Briner, J. P., Lindqvist, C., Baichtal, J. F., and Heaton, T. H. (2018). Deglaciation of the Pacific coastal corridor directly preceded the human colonization of the Americas. Sci. Adv. 2018:eaar5040. doi: 10.1126/sciadv.aar5040
Lisiecki, L., and Raymo, M. (2005). A Pliocene-Pleistocene stack of 57 globally distributed benthic del 180 records. Paleoceanography 20:A1003. doi: 10.1029/2004P001003
Loney, R. A., Brew, D. A., Muffler, L. J. P., and Pomeroy, J. S. (1975). Reconnaissance Geology of Chichagof, Baranof, and Kruzof Islands, Southeastern Alaska. United States Geological Survey Professional Paper 792.
Lotan, J. E., and Critchfield, W. B. (1990). “Pinus contorta Dougl. Ex. Loud., Lodgepole Pine,” in Silvics of North America, Vol. 1, Conifers, eds R. M. Burns and B. H. Honkala (Washington, D.C: U.S. Dept. of Agriculture Forest Service), 302–315.
Luternauer, J. L., Clague, J. J., Conway, K. W., Barrie, J. V., Blaise, B., and Mathewes, R. W. (1989). Late Pleistocene terrestrial deposits on the continental shelf of western Canada: evidence for rapid sea-level change at the end of the last glaciation. Geology 17, 357–360. doi: 10.1130/0091-7613(1989)017
Lyles, A. S., Baichtal, J. F., and Karl, S. M. (2017). Deciphering Flow Path of Ice Across the Southern Alaska Panhandle Based on Geomorphological Interpretation and Field Data. Geological Society of America Program and Abstracts 49,6.
Mackie, Q., Fedje, D., and McLaren, D. (2018). Archaeology and sea level change on the British Columbia coast. Can. J. Archaeol. 42, 74–91.
Mann, D. H. (1983). The Quaternary History of the Lituya Bay Refugium, Alaska. Ph.D. thesis, University of Washington, Seattle, WA.
Mann, D. H. (1986). “Wisconsin and Holocene glaciation of southeast Alaska,” in Glaciation in Alaska – The Geologic Record, eds T. D. Hamilton, K. M. Reed, and R. M. Thorson (Anchorage: Alaska Geological Society), 237–265.
Mann, D. H., Crowell, A. L., Hamilton, T. D., and Finney, B. P. (1998). Holocene geologic and climatic history around the Gulf of Alaska. Arctic. Anthro. 35, 112–131.
Mann, D. H., and Hamilton, T. D. (1995). Late Pleistocene and Holocene paleoenvironments of the North Pacific Coast. Quat. Sci. Rev. 14, 449–471. doi: 10.1016/0277-3791(95)00016-i
Mathewes, R. W., and Clague, J. J. (2017). Paleoecology and ice limits of the early Fraser glaciation (Marine Isotope Stage 2) on Haida Gwaii, British Columbia, Canada. Quat. Res. 88, 277–292. doi: 10.1017/qua.2017.36
Mayle, F. E., Levesque, A. J., and Cwynar, L. C. (1993). Alnus as an indicator taxon of the Younger Dryas cooling in eastern North America. Quat. Sci. Rev. 12, 295–305. doi: 10.1016/0277-3791(93)90038-n
McKenzie, G. D., and Goldthwait, R. P. (1971). Glacial history of the last eleven thousand years in Adams Inlet, southeastern Alaska. Geol. Soc. Amer. Bull. 82, 1767–1782.
McLaren, D., Fedje, D., Dyck, A., Mackie, Q., Gauvreau, A., and Cohen, J. (2018). Terminal Pleistocene Epoch human footprints from the Pacific coast of Canada. PLoS One 13:e0193522. doi: 10.1371/journal.pone.0193522
Méheust, M., Stein, R., Fahl, K., and Gersonde, R. (2018). Sea-ice variability in the subarctic North Pacific and adjacent Bering Sea during the past 25 ka: new insights from IP25 and Uk’37 proxy records. Arktos 4:8. doi: 10.1007/s41063-018-0043-1
Misarti, N., Finney, B. P., Jordan, J. W., Maschner, H. D. G., Addison, J. A., Shapley, M. D., et al. (2012). Early retreat of the Alaska Peninsula Glacier Complex and the implications for coastal migrations of first Americans. Quat. Sci. Rev. 48, 1–6. doi: 10.1016/j.quascirev.2012.05.014
Molnia, B. F. (1986). “Glacial history of the northeastern Gulf of Alaska – A synthesis,” in Glaciation in Alaska – The Geologic Record, eds T. D. Hamilton, K. M. Reed, and R. M. Thorson (Anchorage, AK: Alaska Geological Society), 219–235.
Molnia, B. F. (2008). Glaciers of Alaska: Satellite Image Atlas of Glaciers of the World. United States Geological Survey Professional Paper 1386-K.
Moss, M. L., Peteet, D. M., and Whitlock, C. (2007). “Mid-Holocene culture and climate on the northwest coast of North America,” in Climate Change and Cultural Dynamics: A Global Perspective on Mid-Holocene Transitions, eds D. H. Sandweiss, K. Maasch, and D. Anderson (Amsterdam: Elsevier, Inc), 491–529. doi: 10.1016/b978-012088390-5.50019-4
Muller, M. C. (1982). A Preliminary Checklist of the Vascular Plants of Southeastern Alaska. United States Forest Service Wildlife and Fisheries Habitat Notes, Alaska Region, Administrative Document 112.
O’Clair, R. M., Armstrong, R. H., and Carstensen, R. (1997). The Nature of Southeast Alaska: A Guide to the Plants, Animals, and Habitats. Anchorage: Alaska Northwest Books.
Packee, E. C. (1990). “Tsuga heterophylla (Raf.) Sarg.: Western Hemlock,” in Silvics of North America, Vol. 1, Conifers, eds R. M. Burns and B. H. Honkala (Washington, D.C: U.S. Dept. of Agriculture Forest Service),613–622.
Pellatt, M. G., Hebda, R. J., and Mathewes, R. W. (2001). High-resolution Holocene vegetation history and climate from Hole 1034B, ODP leg 169S, Saanich Inlet, Canada. Mar. Geol. 174, 211–226. doi: 10.1016/s0025-3227(00)00151-1
Pellatt, M. G., and Mathewes, R. W. (1997). Holocene tree line and climate change on the Queen Charlotte Islands, Canada. Quat. Res. 48, 88–99. doi: 10.1006/qres.1997.1903
Peteet, D. M. (1991). Postglacial migration history of lodgepole pine near Yakutat. Alaska. Can. J. Bot. 69, 786–796. doi: 10.1139/b91-102
Peteet, D. M., and Mann, D. H. (1994). Late-glacial vegetational, tephra, and climatic history of southwestern Kodiak Island. Alaska. Ecoscience 1, 255–267. doi: 10.1080/11956860.1994.11682250
Pojar, J., and MacKinnon, A. (eds) (1994). Plants of the Pacific Northwest Coast: Washington, Oregon, British Columbia, & Alaska. Redmond: Lone Pine Publishing.
Praetorius, S. K., Mix, A., Jensen, B., Froese, D., Milne, G., Wolhowe, M., et al. (2016). Interaction between climate, volcanism, and isostatic rebound in Southeast Alaska during the last deglaciation. Earth Planet. Sci. Lett. 452, 79–89. doi: 10.1016/j.epsl.2016.07.033
Praetorius, S. K., and Mix, A. C. (2014). Synchronization of North Pacific deglacial hypoxic events linked to abrupt ocean warming. Science 345, 444–448. doi: 10.1126/science.1252000
Praetorius, S. K., Mix, A. C., Walczak, M. H., Wolhowe, M. D., Addison, J. A., and Prahl, F. G. (2015). North Pacific deglacial hypoxic events linked to abrupt ocean warming. Nature 527, 362–366. doi: 10.1038/nature15753
Reimchen, T., and Byun, A. (2005). “The evolution of endemic species in Haida Gwaii,” in Haida Gwaii: Human History and Environment from the Time of the Loon to the Time of the Iron People, eds D. W. Fedje and R. W. Mathewes (Vancouver, B.C: University of British Columbia Press), 77–95.
Reimer, P. J., Bard, E., Bayliss, A., Beck, J. W., Blackwell, P. G., Bronk Ramsey, C., et al. (2013). IntCal13 and Marine13 radiocarbon age calibration curves 0-50,000 years cal BP. Radiocarbon 55, 1869–1887. doi: 10.1016/j.dib.2018.10.040
Riehle, J. R., Champion, D. E., Brew, D. A., and Lanphere, M. A. (1992a). Pyroclastic deposits of the Mount Edgecumbe volcanic field, southeast Alaska: eruptions of a stratified magma chamber. Jour. Volcanol. Geotherm. Res. 53, 117–143. doi: 10.1016/0377-0273(92)90078-r
Riehle, J. R., Mann, D. H., Peteet, D. M., Engstrom, D. R., Brew, D. A., and Meyer, C. E. (1992b). The Mount Edgecumbe tephra deposits, a marker horizon in southeastern Alaska near the Pleistocene-Holocene boundary. Quat. Res. 37, 183–202. doi: 10.1016/0033-5894(92)90081-s
Stowell, H. H. (2006). Geology of Southeast Alaska: Rock and Ice in Motion. Fairbanks: University of Alaska Press.
Stuiver, M., and Reimer, P. J. (1993). Extended 14C data base and revised CALIB 3.0 14C age calibration program. Radiocarbon 35, 215–230. doi: 10.1017/s0033822200013904
Viereck, L. A., and Little, E. L. Jr. (2007). Alaska Trees and Shrubs, 2nd edn. Fairbanks: University of Alaska Press.
Vitt, D. H., Marsh, J. E., and Bovey, R. B. (1988). Mosses, Lichens & Ferns of Northwest North America. Edmonton: Lone Pine Publishing.
Warner, B. G. (1984). Late Quaternary paleoecology of eastern Graham Island, Queen Charlotte Islands, British Columbia, Canada. Ph.D. thesis, Simon Fraser University, Burnaby, BC.
Warner, B. G., and Chmielewski, J. G. (1987). Biometric analysis of modern and late Pleistocene cones of Picea from western Canada. New Phytol. 107, 449–457. doi: 10.1111/j.1469-8137.1987.tb00196.x
Warner, B. G., Clague, J. J., and Mathewes, R. W. (1984). Geology and paleoecology of a mid-Wisconsin peat from the Queen Charlotte Islands, British Columbia, Canada. Quat. Res. 21, 337–350. doi: 10.1016/0033-5894(84)90073-5
Western Regional Climate Center (2018). Recent Climate in The West. Available at: wrcc.dri.edu (accessed May 14, 2018).
Wheeler, N. C., and Guries, R. P. (1982). Biogeography of lodgepole pine. Can. J. Bot. 60, 1805–1814. doi: 10.1139/b82-227
Wigen, R. J. (2005). “History of the vertebrate fauna in Haida Gwaii,” in Human History and Environment from the Time of Loon to the Time of the Iron People,”, eds D. W. Fedje and R. W. Mathewes (Vancouver, B.C: University of British Columbia Press), 96–115.
Wilcox, P., Fowell, S. J., Bigelow, N. H., and Baichtal, J. F. (2016). Increasing humidity during Younger Dryas revealed by palynological and sedimentological analysis of cores from Baker Island, southeast Alaska (Abstr) Annual Meeting, Denver, Colorado, Paper No. 73-75. Boulder, CO: Geological Society of America.
Worley, I. A. (1980). “Ancient environments and age of non-glaciated terrain in southeastern Alaska,” in National Geographic Society Research Reports, Vol. 12, eds P. H. Oehser, J. S. Lea, and N. L. Powars (Washington, DC: National Geographic Society), 733–747.
Keywords: Alaska, late quaternary, deglaciation, lake sediments, marine sediments, vegetation, pollen analysis, radiocarbon dating
Citation: Ager TA (2019) Late Quaternary Vegetation Development Following Deglaciation of Northwestern Alexander Archipelago, Alaska. Front. Earth Sci. 7:104. doi: 10.3389/feart.2019.00104
Received: 30 October 2018; Accepted: 24 April 2019;
Published: 31 May 2019.
Edited by:
Dorothy M. Peteet, Goddard Institute for Space Studies (NASA), United StatesReviewed by:
Rolf Walter Mathewes, Simon Fraser University, CanadaLi Wu, Anhui Normal University, China
Copyright © 2019 Ager. This is an open-access article distributed under the terms of the Creative Commons Attribution License (CC BY). The use, distribution or reproduction in other forums is permitted, provided the original author(s) and the copyright owner(s) are credited and that the original publication in this journal is cited, in accordance with accepted academic practice. No use, distribution or reproduction is permitted which does not comply with these terms.
*Correspondence: Thomas A. Ager, dG9tYWdlcjc1QGdtYWlsLmNvbQ==