Changing Eruptive Styles at the South-East Crater of Mount Etna: Implications for Assessing Lava Flow Hazards
- 1Istituto Nazionale di Geofisica e Vulcanologia, Sezione di Catania, Osservatorio Etneo, Catania, Italy
- 2Conservatoire National des Arts et Métiers, Laboratoire Modélisation Mathématique et Numérique, Paris, France
The formation of new craters or cones in active volcanoes can influence the style and frequency of eruptions and may also significantly affect volcanic hazard. Here, we explore how the lava flow hazard changed on Mount Etna in the past 20 years, while continuous shifts in the location of volcanism in the summit area were experienced. The summit area of Mount Etna has undergone large morphological variations since the formation of the South-East Crater (SEC) in 1971 and New Southeast Crater (NSEC) in 2007, which have also marked a notable change in the eruptive activity of the volcano. Indeed, in less than 50 years, the SEC area has been the source of more than two hundreds of eruptions characterized by the emission of lava fountains, pyroclastic material, and short-lasting lava flows, which are the greatest hazard presented to the tourist facilities on the flanks of Etna. Here we statistically analyze the main quantitative parameters (i.e., duration and lava volume) of 154 well-documented eruptive events occurred since 1998 with the aim of characterizing the time-volume evolution of the SEC activity. From 1998 to 2018, we find a cumulative volume of ∼135 millions of cubic meters that gives a long-term output rate of ∼0.21 m3/s, with a remarkable change in the eruptive style (longer and more voluminous events) starting from 2011. Taking advantage of this analysis and lava flow simulations run on a Pléiades-derived topography updated to 2016, we also present the new hazard map from lava flow inundation in the SEC area, which is quite different from the hazard map produced in 2011, covering an area of ∼15 km2 and reaching the lowest altitude of about 1,500 m a.s.l. This map establishes an important basis for mitigation decisions and long-term territorial planning, allowing key at risk areas to be rapidly and appropriately identified.
Introduction
Central or composite volcanoes are often characterized by persistent activity at the summit crater. Nevertheless, the geological record of some active volcanoes, including calderas (e.g., Sakurajima, Aso, Okmok, Campi Flegrei) and large edifices (e.g., Mauna Loa, Etna) has shown a more complicated eruptive pathway (Rivalta et al., 2019). Etna, in particular, has experienced one of the best-documented shifts in the location of volcanism in the summit area. Over the last 120 years, the main eruptive activity was initially located at the central crater, then migrated to the North-East Crater (NEC) and later to the South-East Crater (SEC), as the new summit craters were formed (Cappello et al., 2013).
Etna’s summit eruptions are often accompanied by emission of lava flows, which represent the greatest hazard for the touristic facilities located above 1,800 m of elevation (Rifugio Sapienza and Piano Provenzana areas, see Figure 1). Detailed maps showing areas that are likely to be inundated by future lava flows are essential to manage volcanic emergencies in terms of long-term land-use planning and mitigation actions to reduce potential effects. On Mount Etna, different hazard maps, especially for flank eruptions, have been proposed. Some of them are based on a qualitative analysis of historical eruptions (e.g., Andronico and Lodato, 2005; Behncke et al., 2005), while modern assessment combines geological history with deterministic or probabilistic computational models (e.g., Crisci et al., 2008; Favalli et al., 2011; Connor et al., 2012; Del Negro et al., 2013b).
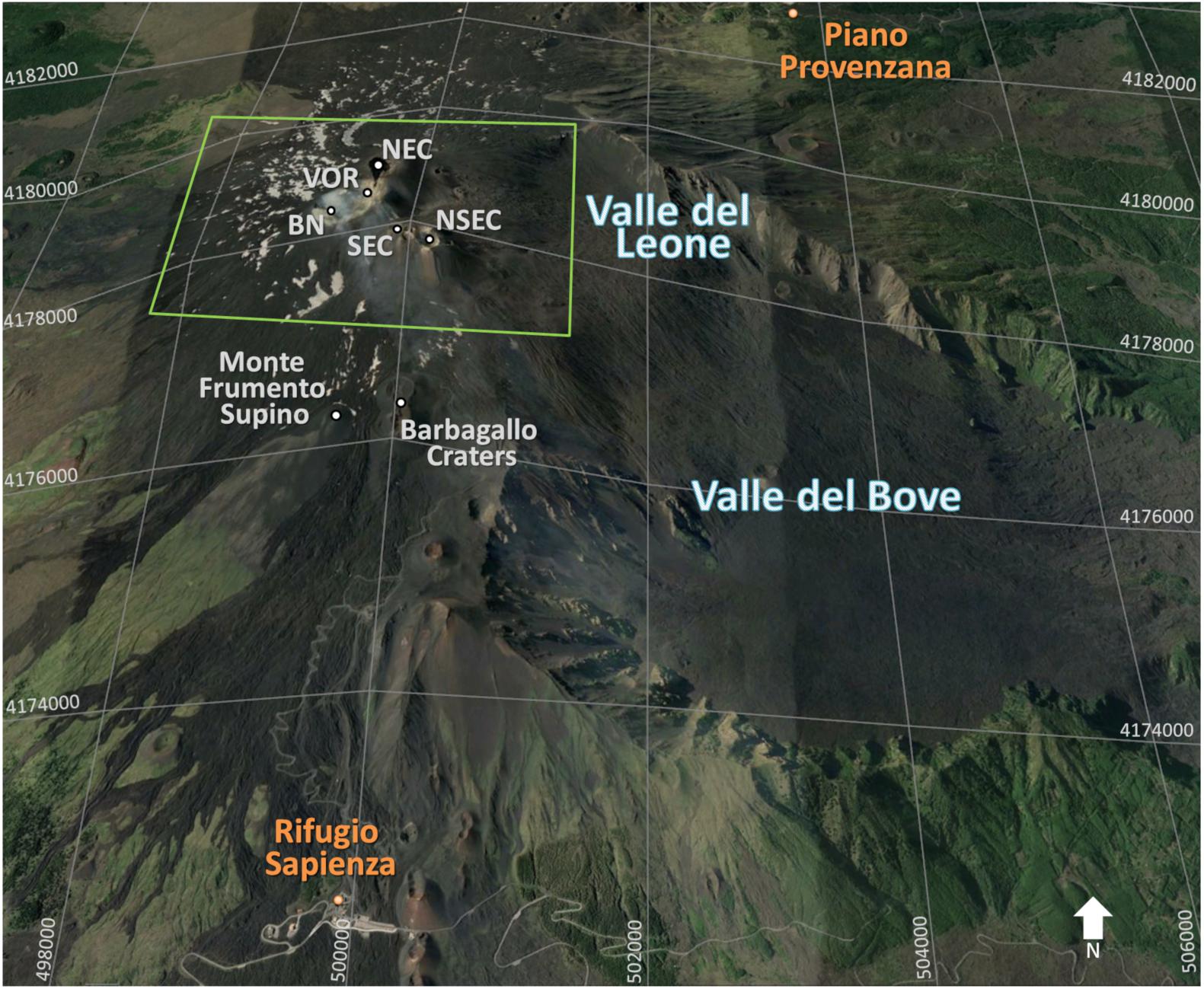
Figure 1. Google Earth 3D oblique view (from south) of Etna’s summit area. The active summit craters are indicated: North-East Crater (NEC), Voragine (VOR), Bocca Nuova (BN), South-East Crater (SEC), and New South-East Crater (NSEC). Monte Frumento Supino and Barbagallo Craters are the cones that diverted the lava flows emitted by NSEC in February and March 2017. Valle del Leone is the NE part of Valle del Bove, a large horseshoe-shaped depression located on the eastern side of the volcano. Rifugio Sapienza and Piano Provenzana are the two main touristic facilities located respectively, in the southern and northern flank of Etna. The green trapeze shows the location of Figure 2.
Here we demonstrate how the formation of Etna’s SEC had direct implications on the eruptive frequency and style, and, consequently, on the hazard from lava flow inundation. We extend the preliminary statistical analysis and lava flow hazard mapping presented in a previous study related to the 12–13 January 2011 eruption of SEC (Vicari et al., 2011b), and revise the catalog of SEC/NSEC eruptions of the last 20 years (Behncke et al., 2006; Del Negro et al., 2013a; Ganci et al., 2018; Cappello et al., 2019).
The probability of occurrence of the expected effusive eruptions was estimated by carrying out a complete revision of the main quantitative data on all 154 episodes occurred at SEC/NSEC from 1998 to 2018 (Supplementary Table S1). From the accurate analysis of the distribution of eruption durations and lava volumes, we identified a time/volume trend associated with different significant periods, and estimated new statistics of occurrence for each class of expected eruptions. The latter were combined with updated lava flow simulations to revise the hazard map from lava flow inundation in the SEC/NSEC area. Based on the classification of SEC eruptions, simulations of lava flow paths were run with the MAGFLOW model (Cappello et al., 2016b) using a Pléiades-derived Digital Elevation Model (DEM) updated to 2016 (Ganci et al., 2019a). The increase of zonation of susceptible areas for new vent opening, as well as the new time-volume evolution of eruptive activity, produced a new lava flow hazard map, which differs significantly from the previous hazard map produced in 2011 (Vicari et al., 2011b).
The Eruptions of Etna’s South-East Crater
The South-East Crater (SEC) of Mount Etna opened as a collapse pit at the southeastern base of the central cone in the middle of the 1971 eruption, which in its first phase affected the southeastern portion of the summit area, and then developed into a classical flank eruption with the opening of new eruptive fissures on the north-eastern flank (Rittmann et al., 1973). The 1971 eruption is considered an important turning point in the recent evolution of the volcano. If all the dynamics of Etna since 1971 are considered, one of the main features is a gradual, but significant, change in the composition of erupted magmas and in their amount of volatiles (e.g., Viccaro and Cristofolini, 2008; Viccaro et al., 2011; Di Renzo et al., 2018 and references therein). Moreover, the birth of the SEC marks a significant increase in the frequency of eruptive events, mostly occurred in the eastern sector of the volcano and structurally connected to the newly formed crater (Cappello et al., 2013; Acocella et al., 2016). The majority of these eruptions is accompanied by lava fountains and flows that spread within the Valle del Bove, a major morphological depression on the eastern flank of the volcano (Figure 1).
During the first 25 years of activity, SEC erupted quite frequently, but most of the growth was due to more than 140 paroxysmal episodes and effusive eruptions occurred in the time periods 1996–2001 and 2006–2012 (Figure 2a). Meanwhile, in mid-May 2007, a pit crater formed on the south-eastern flank of the SEC cone, which would be later known as New Southeast Crater or NSEC (Figure 2b). Starting from the eruptive event of 12–13 January 2011, NSEC has grown by about 210 meters, covering an area of 0.53 km2 and reaching an elevation of 3,325 m a.s.l. in 2015 with over more than 50 eruptive episodes (Ganci et al., 2018). In particular, the eruptive events of October-December 2013 caused an important change in the morphology of the southern flank of Etna (Neri et al., 2017), with lava flows on October 26th and November 11th that completely covered the entire area of the Torre del Filosofo refuge. Since 2014, eruptive activity at SEC has tended to be less violent (De Beni et al., 2015), showing a temporal frequency that has gradually decreased with no remarkable effusive activity in 2016.
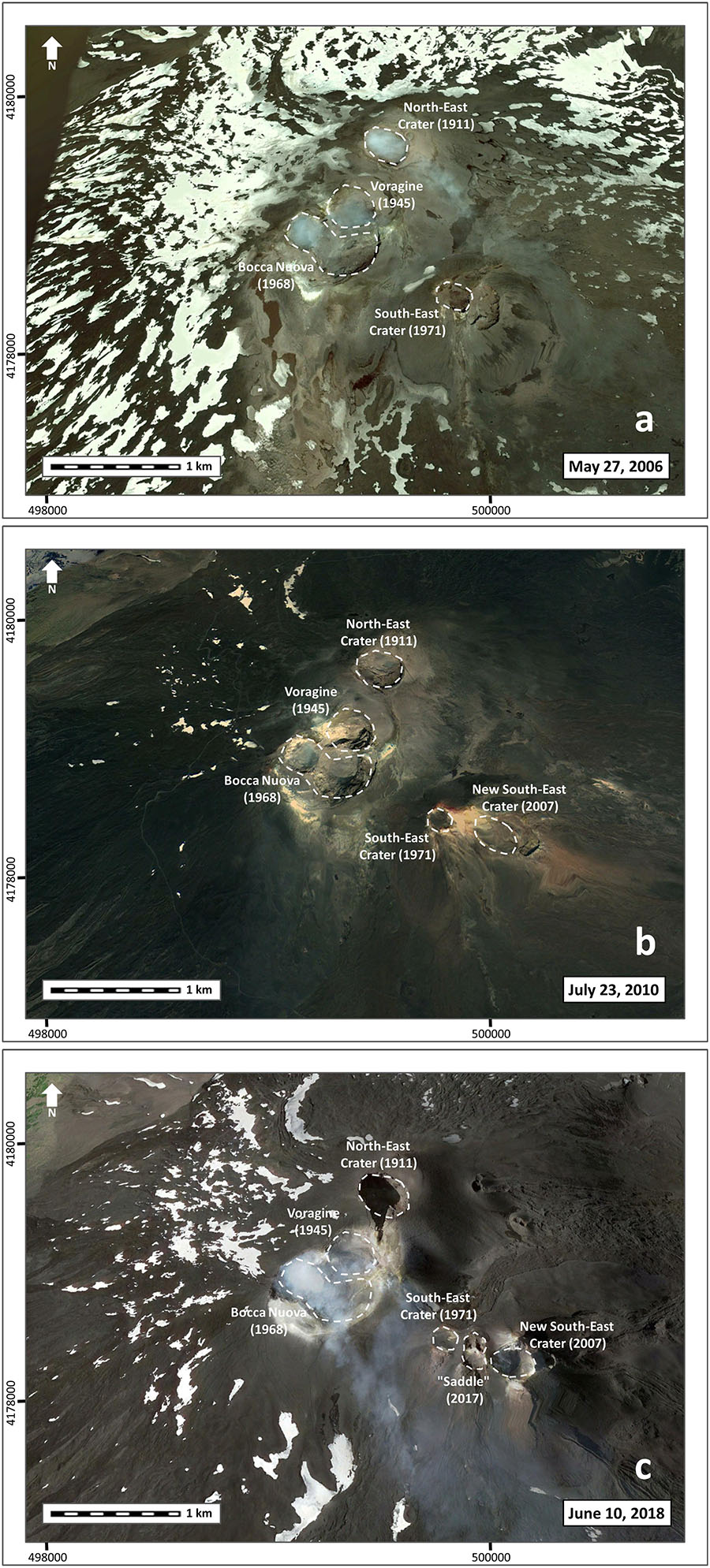
Figure 2. Morphological change of the summit area of Mount Etna seen from Google Earth’s satellite imagery acquired on 27 May 2006 (a), 23 July 2010 (b) and 10 June 2018 (c). The three views highlight the formation and growth of the cones on the southeast flank of the volcano, which have produced exceptional eruptive episodes in the historical context of Etna’s activity.
After 14 months of repose, six eruptive episodes occurred between February and April 2017, mostly from vents located in the old saddle between SEC and NSEC (Figure 2c). These produced lava flows spreading toward south-west, diverted by Monte Frumento Supino and Barbagallo Craters, toward SE, flowing in the Valle del Bove, and toward NE, directed to Valle del Leone (see Figure 1; Cappello et al., 2019). Finally, on 23 and 27 August 2018, activity resumed at the “saddle” cone with two small lava flows that flowed a few hundred meters toward the Valle del Bove and into the Valle del Leone.
Materials and Methods
Revision and Statistical Analysis of SEC Eruptions
Eruptions occurred in the SEC area have been characterized by field measurements (Behncke et al., 2006) and more recently by satellite remote sensing data, which allowed to estimate mean eruptive rates and to compute the related lava volumes (Del Negro et al., 2013a; Ganci et al., 2018, 2019b; Cappello et al., 2019). We carried out a complete revision of 154 eruptive episodes occurred between September 1998 and August 2018, collecting for each one the main quantitative volcanological data: start date, duration, lava volume, Mean Output Rate (MOR), and cumulative volumes (Supplementary Table S1). The HOTSAT system (Ganci et al., 2012a, b, 2016) was used to analyze infrared remote sensing images acquired by SEVIRI and MODIS sensors in order to detect eruptive style, eruption start and end, and to derive the lava volume emitted during each event.
The revised catalog of SEC/NSEC eruptions of the last 20 years was divided in two datasets, obtaining 97 episodes occurred from 1998 to 2008 (called “Period I”) and 57 events that took place between 2011 and 2018 (called “Period II”). The 2008 long-lasting eruption (13 May 2008–6 July 2009) was fixed as turning point since it has been the longest Etna flank eruption of the early seventeenth century, changing the summit area morphology and determining the growth of a broad lava shield on the western slope of the Valle del Bove (Behncke et al., 2016).
To detect any variation in the eruption style, we computed the minimum, maximum, average and median values of eruption duration and lava volume for all events of Period I and II. We also estimated the cumulative volume and the long-term output rate dividing the total volume of lava by the time period 1998–2018. This rate was compared with a fully stochastic model (e.g., Burt et al., 1994), which assumes the rate of lava being discharged is equal to the rate of magma entering the reservoir, and that repose times (also known as inter-event times, i.e., the time between the onset of two consecutive eruptions) are independent with respect to the volumes. The cumulative volume Vcum can be given by:
where λ is the slope (in m3/s), equal to the long-term magma supply rate, t is the time (in sec) and α is the intercept (in m3). The best estimate of average output over time is calculated as , being vi the volume of lava (in m3) emitted during each eruption i, and ri the repose time (in sec) between the eruption i end i + 1.
Finally, from the accurate analysis of the distribution of eruption durations and lava volumes, we identified nine eruptive classes, fixing two time limits for their duration, at 4 and 8 h, and two for the emitted volume, at 1 and 2 million cubic meters (Vicari et al., 2011b). In order to detect the presence of any trend in the eruptive activity of SEC in the past 20 years, we calculated the percentages of occurrence and MORs of the nine eruptive classes.
Lava Flow Hazard Mapping
Starting from the new classification of SEC eruptions, we revised the hazard map from lava flow inundation presented in Vicari et al. (2011b). To this aim, we used the probabilistic approach based on the MAGFLOW model that has been applied for the flank and summit eruptions on Mount Etna (Cappello et al., 2011a, b; Vicari et al., 2011a; Del Negro et al., 2013b, 2016) and in other volcanic areas worldwide (Kereszturi et al., 2014, 2016; Cappello et al., 2015a, b, 2016a; Pedrazzi et al., 2015). This approach consists of four different steps: (i) assessment of the spatiotemporal probability of future vent opening, (ii) estimation of the occurrence probability associated with classes of expected eruptions, (iii) simulation of lava flow paths with the MAGFLOW model, and (iv) computation of the probability that a lava flow will inundate a certain area.
For the assessment of the spatiotemporal probability of future vent opening, we assumed that the whole SEC area has the same probability of activation. We thus defined a 100-m grid of potential vents (103 in total) over an area of ∼1 km2 including all the SEC/NSEC complex and centered on the NSEC. The probability of activation was estimated dividing 1 by 103, i.e., the number of potential vents, and homogenously assigned.
As occurrence probabilities associated with the classes of expected eruptions, we used the decimal values of percentages obtained for the eruptive episodes occurred at SEC between 1998 and 2018.
Eruptive scenarios were simulated using MAGFLOW, which is based on a physical model for the thermal and rheological evolution of the flowing lava (Bilotta et al., 2012; Cappello et al., 2016b). To evaluate areas more likely to be affected by lava flow paths we computed nine simulations, corresponding to the expected classes of eruptions, for each of the potential vents of the grid defined around NSEC.
We used different effusion rate trends based on the event duration. For the three longest eruptive classes of eruptions (lasting more than 8 h), we used three bell-shaped curves retrieved from the median values of duration and volume. These curves, representing the flux rate as a function of time, subtend the total volume with the peak after 25% of the entire eruption duration. For the short-lasting events, effusion rate was assumed constant and equal to the MOR of the class (Latutrie et al., 2016; Cappello et al., 2019).
Rheological properties are modeled using a variable viscosity relationship for Etna’s basaltic rocks (Giordano and Dingwell, 2003), parameterized in terms of temperature and water content. Typical parameters of Etna’s summit events (Giordano and Dingwell, 2003; Giordano et al., 2010; Rogic et al., 2019) used for the MAGFLOW simulations are: density (2600 kg m–3), emissivity (0.93), solidification temperature (1143 K), extrusion temperature (1360 K), water content (0.02 wt%). The crystallization and lava composition are not explicitly accounted for, but they are implicitly considered in the viscosity–temperature relationship of Giordano and Dingwell (2003).
The topographic base was provided in the form of a Digital Elevation Model (DEM), a georeferenced, rectangular grid of cells describing the (average) terrain height at regularly spaced points, which has been proved to have crucial effects on the accuracy and reliability of lava flow hazard assessments (Bilotta et al., 2019). Lava flow paths were simulated on the most recent DEM available for the summit area of Etna, that we obtained by processing tri-stereo Pléiades images acquired on 18 July 2016 (Ganci et al., 2019a).
Finally, the hazard was computed for each point inundated by at least a lava flow by combining the sequence of overlapping MAGFLOW simulations with the probability of vent opening and the probability of occurrence of the nine eruptive classes.
Results
Durations, volumes and MORs of the events occurred between 1998 and 2018 are reported in Figure 3. In the Period I, durations range from 26 min to little more than half day, with a median value of 2 h (25th percentile = 1.6 h; 75th percentile = 8.3 h). In the Period II, eruptions have a median duration of 7.2 h (25th percentile = 4 h; 75th percentile = 16.9 h), with a minimum of 40 min and a maximum of 71 days for the long effusive event begun on 22 January 2014 (Figure 3B).
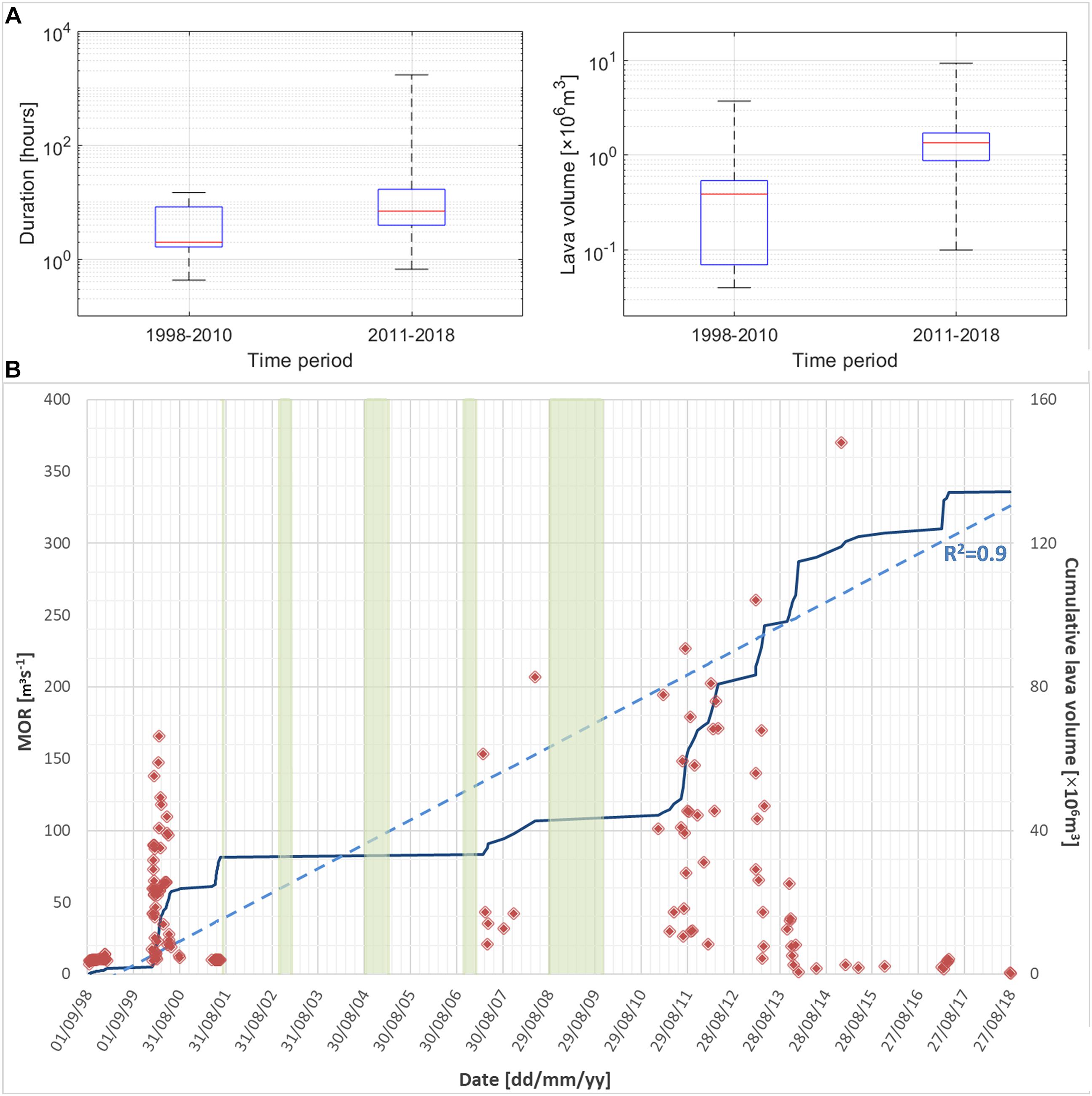
Figure 3. (A) Box plots for duration (left) and lava volume (right) of SEC eruptions showing batches of data for Period I and II. Five values are displayed: the extremes, the upper and lower hinges (quartiles), and the median. Ordinates are in logarithmic scale. (B) Cumulative lava volume curve (blue line) estimated considering only SEC eruptions from September 1998 to August 2018 whose long-term supply rate is given by slope of the fitting curve (dashed blue line). Mean Output Rate - MOR (red diamonds) is calculated as the total volume erupted during the event divided by duration of event. The green bars highlight the five effusive flank eruptions of 2011, 2002–2003, 2004–2005, 2006 and 2008–2009.
The median values of lava volumes are 0.4 × 106 m3 (Period I) and 1.33 × 106 m3 (Period II), with interquartile ranges of [0.07, 0.54] and [0.88, 1.70] × 106 m3 for the Periods I and II, respectively. The minimum volumes were recorded on 29 January 2000 (0.04 × 106 m3) and 27 August 2018 (0.2 × 106 m3), and maximum volumes on 10 May 2008 (3.72 × 106 m3) and 22 January 2014 (9.3 × 106 m3).
MORs range between ∼ 1.5 m3/s (on 27 August 2018) and 370 m3/s (on 28 December 2014), with an average value of 54 m3/s. Three bell-shaped trends can be recognized in Figure 3B, with local peaks of MOR (bigger than 100 m3/s) recorded during eruptive episodes occurred from February to May 2000, on 29 March 2007 and 10 May 2008, and between January 2011 to April 2013. Since then, eruption intensity shows a decreasing trend, reaching the minimum value during the last SEC eruption of 2018.
The cumulative volume for the entire period amounts to about 135 millions of cubic meters (Figure 3B), which gives a long-term output rate of 0.214 m3/s. Implementing the fully stochastic model (Burt et al., 1994) on our data, we found a linear trend having a slope of 0.213 m3/s, which best fits the actual cumulative volume with an R-squared value of 0.9 (Figure 3B).
The percentages of occurrence and MOR for the nine possible eruptive classes of SEC eruptions are shown in Figure 4. In Period I, only six classes were populated, with most of the eruptive episodes (66%) falling in the first one (less than 1 h and 1 × 106 m3). The percentages of Period II illustrate a very different scenario, with all occurrences ranging between 4 and 21%. In particular, the number of events belonging to the first class has drastically decreased to 7%, causing a “flattening” effect on statistics. By considering the entire period, all classes show positive percentages, with more than 60% of eruption lasting less than 4 h or greater than 8 h, and lava volume less than 1 million of cubic meters.
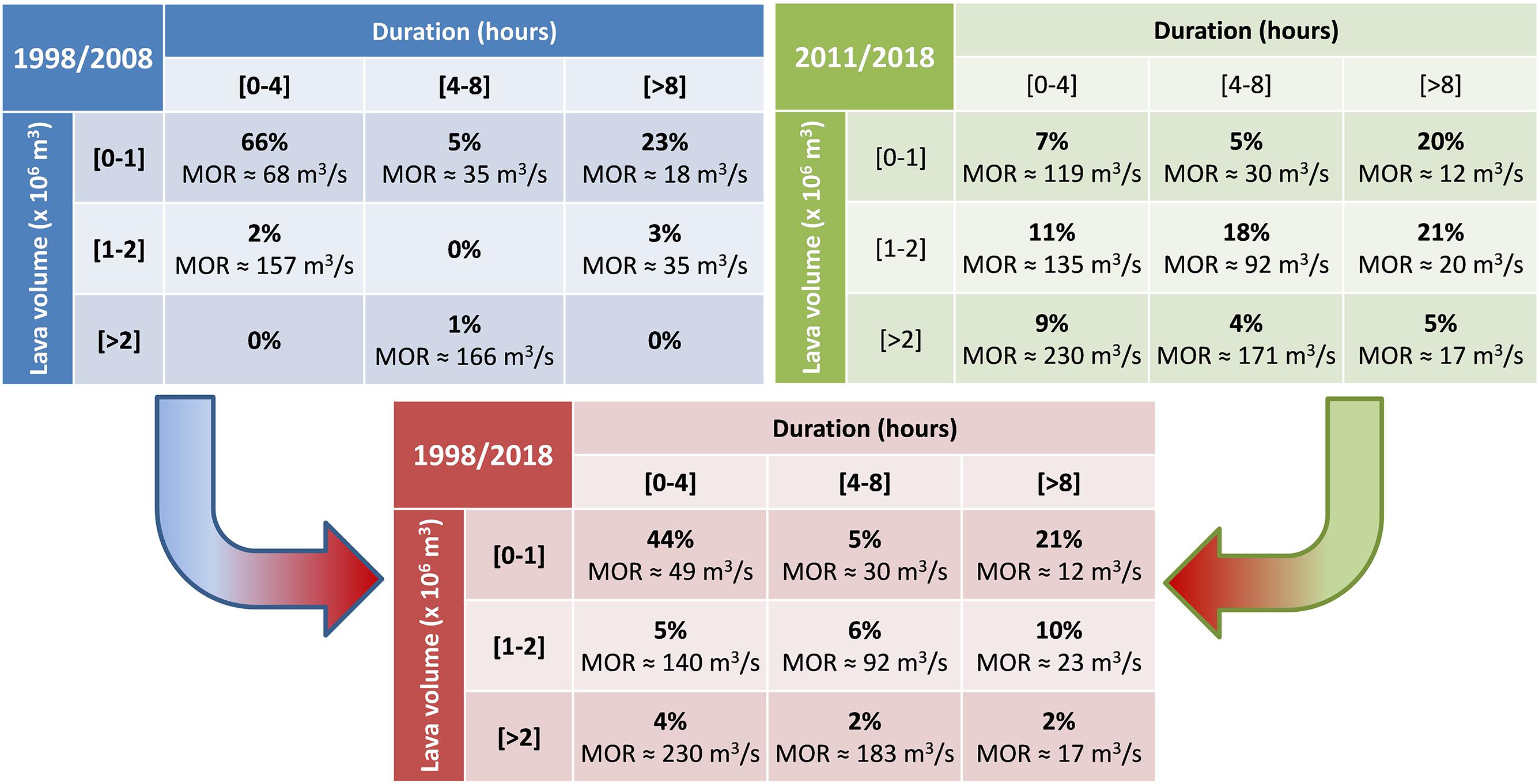
Figure 4. Classification of eruptive events at SEC based on duration and lava volume by time period: from 1998 to 2008 (blue), from 2011–2018 (green), and for the entire data range (red). Each class reports the percentage of occurrence and MOR, estimated as the ratio between the median values of erupted lava and duration.
By combining the overlapping MAGFLOW simulations with the probability of vent opening and the probability of occurrence of SEC’s eruptive classes, we obtained the new lava flow hazard map displayed in Figure 5. This map shows the probability of inundation for the areas potentially affected by lava flows emitted from vents in the SEC/NSEC complex. We have not computed absolute levels of hazard (i.e., probabilities within a specific time period), limiting our investigation to the assessment of conditional probabilities (i.e., probabilities upon the occurrence of an eruption).
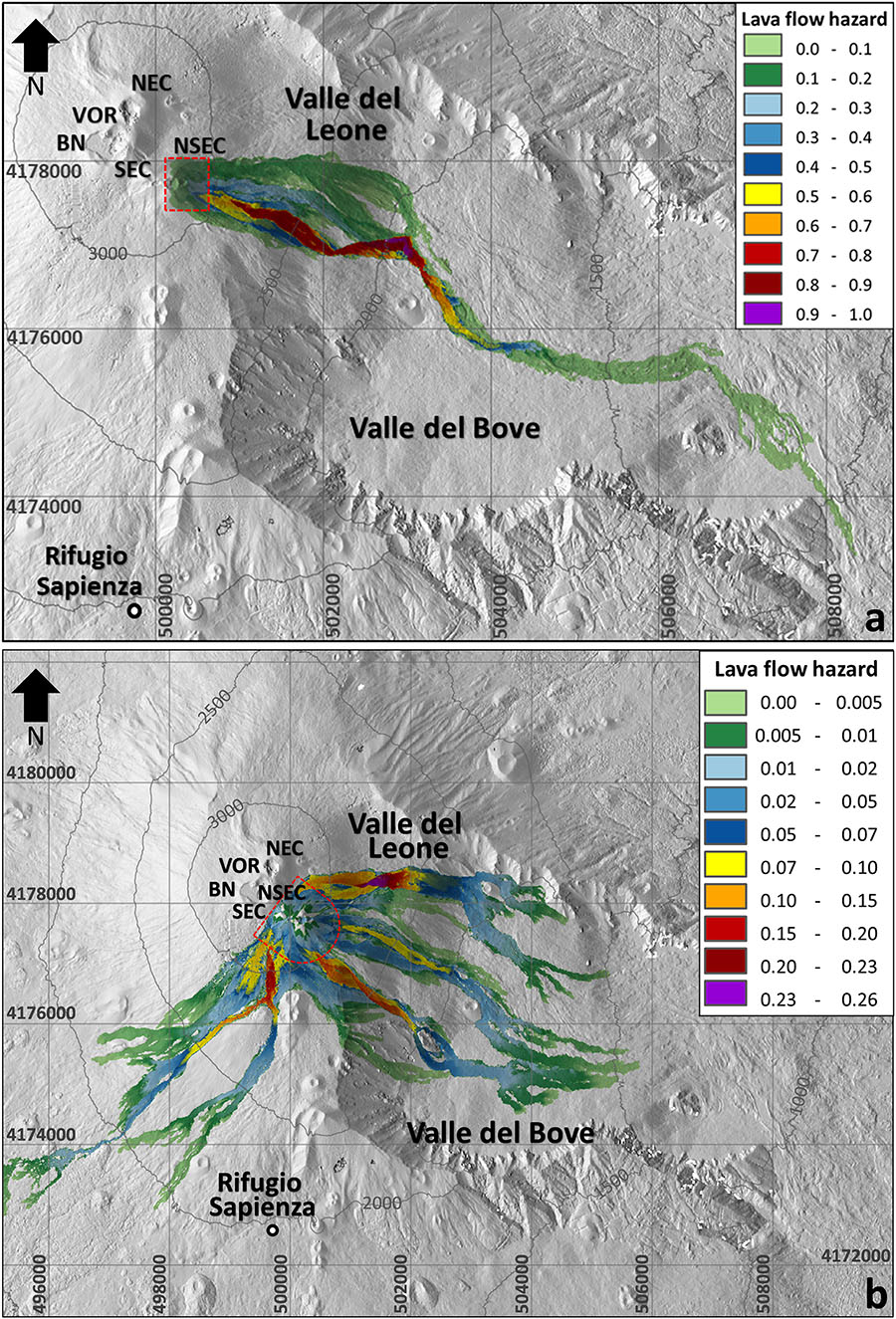
Figure 5. (a) Lava flow hazard map at the SEC updated to 2011 (redrawn from Vicari et al., 2011b). Six MAGFLOW simulations were run on the 2007 DEM from 30 potential vents around the NSEC (red dashed area). (b) Inundation hazard mapping updated to 2018 and associated to lava flows emitted from 103 potential vents opening in the SEC zone (red dashed area). MAGFLOW simulations were performed on the most recent available DEM, which was derived from the Pléiades images acquired on 18 July 2016.
While the 2011 map (Figure 5a) covers an area of 4.3 km2 with a maximum hazard of 0.97 in Valle del Bove, the total area of the new map measures about 14.8 and 1.5 km2 of which have a probability of inundation higher than 0.1. The highest hazard value (0.26) has moved from Valle del Bove to Valle del Leone.
The longest lava flow simulations travel almost 7 km along the south-west direction, while a maximum distance of ∼6.5 km is reached toward south-east (Figure 5b). Except for a small southwestern branch, none of the simulated lava flows reaches an altitude below 1,500 m a.s.l., which could threaten cultivated zones, and, possibly, inhabited centers.
Discussion
Analyzing the duration and lava volume of 154 episodes, we found a remarkable change in the eruptive activity of Etna’s SEC starting from the growth of the NSEC in 2011 (Figures 3A, 4). In addition to the increase in the number of events (Cappello et al., 2013), this change concerns a shift in the location of volcanism, as well as a considerable variation in the eruptive style from shorter to longer duration and from smaller to larger volumes.
Before the long flank eruption of 2008–2009, SEC eruptions were mostly characterized by short durations (less than 4 h) and small volumes of erupted magma (less than 1 million cubic meters). Conversely, since 2011, median values of duration and lava volume increased more than three times (from 2 to 7 h and from 0.4 to 1.35 m3). Moreover, the non-stationary trend of MOR (Figure 3B), with three local peaks on 2000, 2008, and 2013, and mainly decreasing since 2014, highlights a significant change in the data distribution.
By considering the cumulative volume (Figure 3B), we found a long-term output rate of ∼0.21 m3/s, which can grow to ∼0.58 m3/s if we also include the lava volumes (∼235 × 106 m3) erupted during the 2011, 2002–2003, 2004–2005, 2006, 2008–2009, and December 2018 flank eruptions (obtaining a total amount of ∼370 × 106 m3). Our long-term supply is comparable with the MOR (0.6 m3/s) of the 1970’s decade (Harris et al., 2011), but far below the time-averaged rate of 1.26 m3/s recorded since 1971 (Neri et al., 2011) and of 0.9 m3/s started in 2000 (Ganci et al., 2012b). This was mainly due to the small number of eruptions occurred since 2014 (only 15), with a total lava volume emitted of little more than 30 × 106 m3.
The updated lava flow hazard map (Figure 5b) is quite different from the one produced in 2011, covering an area (14.8 km2) more than three times larger than the one (∼4.3 km2) of the 2011 map (Figure 5a). Lava flows spread both toward south-west and south-east, reaching an elevation of about 1,500 m a.s.l., while the threatened zone in the 2011 map was almost completely contained within the huge uninhabited depression of the Valle del Bove (Figure 5a). This is a consequence of the migration of the SEC eruptive activity: if the eruptions occurred between 2011 and 2013 followed a northwestern–southeastern trend from SEC to NSEC, the most recent lava flows have been emitted from vents opened within and around the SEC/NSEC complex, making SEC, NSEC, and “saddle” cone almost indistinguishable (Figure 2c). For this reason a larger area of vent opening was chosen (∼1 vs 0.24 km2, i.e., 103 vs 30 potential vents), producing less channeled flows over a more spread area, as well as lower maximum values of inundation probability (0.26 vs 0.97). Indeed both a decreased probability of activation and a decreased overlapping of simulated lava flows imply a lower probability of relative hazards. Moreover, the change in the eruptive style results in more long-lasting and more voluminous lava flows capable of traveling over long distances, possibly inundating vulnerable areas on Etna’s flanks.
The remarkable enlargement of the area potentially affected by lava flows from 2011 to 2018 reveals an increase in the overall hazard, posing major challenges to authorities and decision-makers during volcanic emergencies, and emphasizing the importance of updating hazard maps at active volcanoes, especially those characterized by several interchanging and growing vents on their summit, such as Etna, Stromboli or Mauna Loa volcanoes. In general, understanding where and when lava flows are likely to occur is a vital requirement for any hazard assessment at active volcanoes (Del Negro et al., 2013b). Indeed, when a lava flow reaches towns or rural lands, destruction is usually complete. However, the effects of lava flows can be reduced by careful advance land-use planning, so that inhabited centers and vulnerable structures are built away from or above likely lava flow paths.
Conclusion
We performed a new comprehensive classification of the SEC eruptive activity of Mount Etna spanning the 1998–2018 time period, with the scope of detecting any change in the eruptive frequency and style, especially after the creation of the NSEC at the southeast base of the SEC cone in 2007.
By analyzing the distribution of duration and volume emitted during eruptions at SEC in the last two decades, we underlined how they both have grown considerably, determining a strong reduction of eruption intensity, especially after 2013. Indeed, eruptions occurred before the 2008–2009 flank eruption mostly lasted less than 4 h and emitted lava volumes less than 0.5 million of cubic meters. Starting from 2011, the duration of eruptive events grew up to more than 2 months (for the long effusive event of 22 January 2014), with a median lava volume of 1.33 × 106 m3. This increasing trend in both duration and volume of SEC eruptions is more evident after 2013, when the MOR suddenly drops to an average value of ∼6 m3/s. The long-term output rate amounts to 0.214 m3/s, which is far below the time-averaged rate of 0.9 m3/s started in 2000.
We also updated the occurrence of the nine eruptive classes identified on the basis of durations and lava volumes (≤4, 4–8 and >8 h vs ≤1, 1–2 and >2 × 106 m3) of the SEC eruptions occurring until 2018. This new classification was used to revise the 2011 hazard map of SEC, both for the estimation of the occurrence probabilities, and for deriving the eruption rate for the lava flow simulations. Lava flow paths were simulated with the physics-based MAGFLOW model on a Pléiades DEM updated to 2016 starting from one hundred of potential vents around the whole SEC/NSEC complex.
The new lava flow hazard map of SEC shows that high probabilities of inundation are reached in different areas of the south-east flank of Etna: Valle del Leone, Valle del Bove and toward SW. Nevertheless, none of the simulated lava flows emitted from vents in the SEC area should threaten nearby settlements such as Rifugio Sapienza and the tourist facility located on the southern slope of Etna at about 1,800 m, which are morphologically protected by the Barbagallo Craters opened during the 2002–2003 flank eruption.
Lava flow hazard maps can be a very powerful instrument to support timely decision-making and risk mitigation measures by civil authorities during eruptive emergencies. Moreover, knowing the areas that are the most prone to inundation by a lava flow is useful for environmental preservation and to encourage better land use planning. Clearly, our hazard map does not provide a deterministic forecast of future eruptions, but a useful prediction based on probabilistic modeling of historical eruptive activity of the SEC. Indeed, the eruptive activity of Etna is always evolving and unpredictable. Recently another pit formed on the eastern flank of the NSEC, marking a counterclockwise rotation of about 30° with respect to the main NW-SE orientation of striking dikes propagating from the SEC (Acocella et al., 2016). What would happen if this pit became the location of the next eruptive events, determining another progressive shift of the SEC eruptive activity to lower elevations?
Data Availability
The datasets generated for this study are available on request to the corresponding author.
Author Contributions
AC mainly wrote the manuscript with contributions from all authors. GG processed SEVIRI data and extracted the topography from Pléiades images. AC performed the statistical analysis on SEVIRI-derived results (with the contribution of GB, CC and AH), and produced the hazard map. CDN coordinated the research.
Funding
We gratefully acknowledge funding support from the ATHOS Research Program.
Conflict of Interest Statement
The authors declare that the research was conducted in the absence of any commercial or financial relationships that could be construed as a potential conflict of interest.
Acknowledgments
This work was developed within the framework of the Laboratory for Technological Advance in Volcano Geophysics (Tecnolab) at the INGV in Catania. Thanks are due to EUMETSAT for SEVIRI data and NASA for MODIS images. Pléiades data were available through the Space Volcano Observatory with Pléiades project (http://volcano.iterre.fr/svo_projects). This manuscript benefited from the comments and suggestions of the Specialty Chief Editor Valerio Acocella, the Associate Editor RS, and the two reviewers.
Supplementary Material
The Supplementary Material for this article can be found online at: https://www.frontiersin.org/articles/10.3389/feart.2019.00213/full#supplementary-material
TABLE S1 | Date (dd/mm/yyy), duration (in hours), lava volume (in m3), Mean Output Rate (in m3/s), cumulative volumes (in m3) and the eruptive class identity of SEC eruptions occurred between September 1998 and August 2018. Eruptive classes are numbered from 1 to 9 depending on duration and volume emitted: class 1 (<4 h, <1 × 106 m3), class 2 (between 4 and 8 h, <1 × 106 m3), class 3 (>8 h, <1 × 106 m3), class 4 (<4 h, between 1 and 2 × 106 m3), class 5 (between 4 and 8 h, between 1 and 2 × 106 m3), class 6 (>8 h, between 1 and 2 × 106 m3), class 7 (<4 h, >2 × 106 m3), class 8 (between 4 and 8 h, >2 × 106 m3), and class 9 (>8 h, >2 × 106 m3).
References
Acocella, V., Neri, M., Behncke, B., Bonforte, A., Del Negro, C., and Ganci, G. (2016). Why does a mature volcano need new vents? the case of the new southeast crater at Etna. Front. Earth Sci. 4:67. doi: 10.3389/feart.2016.00067
Andronico, D., and Lodato, L. (2005). Effusive activity at Mount Etna Volcano (Italy) during the 20th century: a contribution to volcanic hazard assessment. Nat. Hazards 36, 407–443. doi: 10.1007/s11069-005-1938-2
Behncke, B., Fornaciai, A., Neri, M., Favalli, M., Ganci, G., and Mazzarini, F. (2016). LiDAR surveys reveal eruptive volumes and rates at Etna, 2007–2010. Geophys. Res. Lett. 43, 4270–4278. doi: 10.1002/2016GL068495
Behncke, B., Neri, M., and Nagay, A. (2005). “Lava flow hazard at Mount Etna (Italy): new data from a GIS-based study,” in Kinematics and Dynamics of Lava Flows, Vol. 396, eds M. Manga, and G. Ventura (Boulder, CL: Geological Society of America), 189–208. doi: 10.1130/0-8137-2396-5.189
Behncke, B., Neri, M., Pecora, E., and Zanon, V. (2006). The exceptional activity and growth of the southeast crater, Mount Etna (Italy). Between 1996 and 2001. Bull. Volcanol. 69, 149–173. doi: 10.1007/s00445-006-0061-x
Bilotta, G., Cappello, A., Hérault, A., and Del Negro, C. (2019). Influence of topographic data uncertainties and model resolution on the numerical simulation of lava flows. Environ. Model. Softw. 112, 1–15. doi: 10.1016/j.envsoft.2018.11.001
Bilotta, G., Cappello, A., Hérault, A., Vicari, A., Russo, G., and Del Negro, C. (2012). Sensitivity analysis of the MAGFLOW cellular automaton odel for lava flow simulation. Environ. Model. Softw. 35, 122–131. doi: 10.1016/j.envsoft.2012.02.015
Burt, M. L., Wadge, G., and Scott, W. A. (1994). Simple stochastic modeling of the eruption history of a basaltic volcano: nyamuragira. Zaire Bull. Volcanol. 56, 87–97. doi: 10.1007/BF00304104
Cappello, A., Bilotta, G., Neri, M., and Del Negro, C. (2013). Probabilistic modeling of future volcanic eruptions at Mount Etna. J. Geophys. Res. 118, 1925–1935. doi: 10.1002/jgrb.50190
Cappello, A., Ganci, G., Bilotta, G., Hérault, A., Zago, V., and Del Negro, C. (2019). Satellite-driven modeling approach for monitoring lava flow hazards during the 2017 Etna eruption. Ann. Geophys. 61, doi: 10.4401/ag-7792
Cappello, A., Ganci, G., Calvari, S., Perez, N. M., Hernandez, P. A., Silva, S. V., et al. (2016a). Lava flow hazard modeling during the 2014-2015 Fogo eruption, Cape Verde. J. Geophys. Res. Solid Earth 121, 2290–2303. doi: 10.1002/2015JB012666
Cappello, A., Geshi, N., Neri, M., and Del Negro, C. (2015a). Lava flow hazards-An impending threat at Miyakejima volcano, Japan. J. Volcanol. Geother. Res. 308, 1–9. doi: 10.1016/j.jvolgeores.2015.10.005
Cappello, A., Hérault, A., Bilotta, G., Ganci, G., and Del Negro, C. (2016b). MAGFLOW: a physics-based model for the dynamics of lava-flow emplacement. Geol. Soc. Lond. 426, 357–373. doi: 10.1144/SP426.16
Cappello, A., Zanon, V., Del Negro, C., Ferreira, T. J. L., and Queiroz, M. G. P. S. (2015b). Exploring lava-flow hazards at Pico Island, Azores Archipelago (Portugal). Terra Nova 27, 156–161. doi: 10.1111/ter.12143
Cappello, A., Vicari, A., and Del Negro, C. (2011a). Assessment and modeling of lava flow hazard on Mt. Etna volcano. Bollettino di Geofisica Teorica e Applicata 52, 299–308. doi: 10.4430/bgta0003
Cappello, A., Vicari, A., and Del Negro, C. (2011b). Retrospective validation of a lava-flow hazard map for Mount Etna volcano. Ann. Geophys. 54, 634–640. doi: 10.4401/ag-5345
Connor, L. J., Connor, C. B., Meliksetian, K., and Savov, I. (2012). Probabilistic approach to modeling lava flow inundation: a lava flow hazard assessment for a nuclear facility in Armenia. J. Appl. Volcanol. 1:3.
Crisci, G. M., Iovine, G., Di Gregorio, S., and Lupaino, V. (2008). Lava-flow hazard on the SE flank of Mt. Etna (Southern Italy). J. Volcanol. Geotherm. Res. 177, 778–796. doi: 10.1093/rpd/ncu092
De Beni, E., Behncke, B., Branca, S., Nicolosi, I., Carluccio, R., D’Ajello Caracciolo, F., et al. (2015). The continuing story of Etna’s New Southeast Crater (2012-2014): evolution and volume calculations based on field surveys and aerophotogrammetry. J. Volcanol. Geoth. Res. 303, 175–186. doi: 10.1016/j.jvolgeores.2015.07.021
Del Negro, C., Cappello, A., and Ganci, G. (2016). Quantifying lava flow hazards in response to effusive eruption. Geol. Soc. Am. Bull. 128, 752–763. doi: 10.1130/B31364.1
Del Negro, C., Cappello, A., Neri, M., Bilotta, G., Herault, A., and Ganci, G. (2013a). Etna Summit Lava Flows Between 1955 and 2012. Bremen: PANGAEA Data Publisher. doi: 10.1594/PANGAEA.825013
Del Negro, C., Cappello, A., Neri, M., Bilotta, G., Herault, A., and Ganci, G. (2013b). Lava flow hazards at Mount Etna: constraints imposed by eruptive history and numerical simulations. Sci. Rep. 3:3493. doi: 10.1038/srep03493
Di Renzo, V., Corsaro, R. A., Miraglia, L., Pompilio, M., and Civetta, L. (2018). Long and short-term magma differentiation at Mt. Etna as revealed by SrNd isotopes and geochemical data. Earth Sci. Rev. 190, 112–130. doi: 10.1016/j.earscirev.2018.12.008
Favalli, M., Tarquini, S., and Fornaciai, A. (2011). DOWNFLOW code and LIDAR technology for lava flow analysis and hazard assessment at Mount Etna. Ann. Geophys. 54, 552–566. doi: 10.4401/ag-5339
Ganci, G., Bilotta, G., Cappello, A., Hérault, A., and Del Negro, C. (2016). “HOTSAT: a multiplatform system for the satellite thermal monitoring of volcanic activity,” in Detecting Modelling and Responding to Effusive Eruptions, eds A. Harris, T. De Groeve, F. Garel, and S. A. Carn, (London: Geological Society), 426.
Ganci, G., Cappello, A., Bilotta, G., Hérault, A., Zago, V., and Del Negro, C. (2018). Mapping volcanic deposits of the 2011–2015 etna eruptive events using satellite remote sensing. Front. Earth Sci. 6:83. doi: 10.3389/feart.2018.00083
Ganci, G., Cappello, A., Bilotta, G., Hérault, A., Zago, V., and Del Negro, C. (2019a). 3D Lava Flow Mapping at Etna Volcano from Pléiades-derived DEM Differences. Bremen: PANGAEA Data Publisher. doi: 10.1594/PANGAEA.899176
Ganci, G., Cappello, A., Zago, V., Bilotta, G., Hérault, A., and Del Negro, C. (2019b). 3D Lava flow mapping of the 17–25 May 2016 Etna eruption using tri-stereo optical satellite data. Ann. Geophys. 61. doi: 10.4401/ag-7875
Ganci, G., Harris, A. J. L., Del Negro, C., Guehenneux, Y., Cappello, A., Labazuy, P., et al. (2012a). A year of lava fountaining at Etna: volumes from SEVIRI. Geophys. Res. Lett. 39:L06305. doi: 10.1029/2012GL051026
Ganci, G., Vicari, A., Cappello, A., and Del Negro, C. (2012b). An emergent strategy for volcano hazard assessment: from thermal satellite monitoring to lava flow modeling. Remote Sens. Environ. 119, 197–207. doi: 10.1016/j.rse.2011.12.021
Giordano, D., and Dingwell, D. B. (2003). Viscosity of hydrous Etna basalt: implications for Plinian-style basaltic eruptions. Bull. Volcanol. 65, 8–14. doi: 10.1007/s00445-002-0233-2
Giordano, D., Polacci, M., Papale, P., and Caricchi, L. (2010). Rheological control on the dynamics of explosive activity in the 2000 summit eruption of Mt. Etna. Solid Earth 1, 61–69. doi: 10.5194/se-1-61-2010
Harris, A. J. L., Steffke, A., Calvari, S., and Spampinato, L. (2011). Thirty years of satellite-derived lava discharge rates at Etna: implications for steady volumetric output. J. Geophys. Res. 116:B08204. doi: 10.1029/2011JB008237
Kereszturi, G., Cappello, A., Ganci, G., Procter, J., Németh, K., Del Negro, C., et al. (2014). Numerical simulation of basaltic lava flows in the Auckland Volcanic Field, New Zealand – implication for volcanic hazard assessment. Bull. Volcanol. 76, 879–896. doi: 10.1007/s00445-014-0879-6
Kereszturi, G., Nemeth, K., Vioufti, M. R., Cappello, A., Murcia, H., Ganci, G., et al. (2016). Emplacement conditions of the 1256 AD Al-Madinah lava flow field in Harrat Rahat, Kingdom of Saudi Arabia—insights from surface morphology and lava flow simulations. J. Volcanol. Geotherm. Res. 309, 14–30. doi: 10.1016/j.jvolgeores.2015.11.002
Latutrie, B., Andredakis, I., De Groeve, T., Harris, A., Langlois, E., van Wyk de Vries, B., et al. (2016). “Testing a geographical information system for damage and evacuation assessment during an effusive volcanic crisis,” in Detecting, Modelling and Responding to Effusive Eruptions, eds A. Harris, T. De Groeve, F. Garel, and S. A. Carn (London: Geological Society), 426. doi: 10.1144/SP426.19
Neri, M., Acocella, V., Behncke, B., Giammanco, S., Mazzarini, F., and Rust, D. (2011). Structural analysis of the eruptive fissures at Mount Etna (Italy). Ann. Geophys. 54, 464–479. doi: 10.4401/ag-5332
Neri, M., De Maio, M., Crepaldi, S., Suozzi, E., Lavy, M., Marchionatti, F., et al. (2017). Topographic maps of Mount Etna’s summit craters, updated to December 2015. J. Maps 13, 674–683. doi: 10.1080/17445647.2017.1352041
Pedrazzi, D., Cappello, A., Zanon, V., and Del Negro, C. (2015). Impact of effusive eruptions from the Eguas-Carvao fissure system, Sao Miguel Island, Azores Archipelago (Portugal). J. Volcanol. Geother. Res. 291, 1–13. doi: 10.1016/j.jvolgeores.2014.12.012
Rittmann, A., Romano, R., and Sturiale, C. (1973). Some considerations on the 1971 Etna eruption and on the tectonophysics of the Mediterranean area. Geologische Rundschau 62, 418–430. doi: 10.1007/bf01840107
Rivalta, E., Corbi, F., Passarelli, L., Acocella, V., Davis, T., and Di Vito, M. A. (2019). Stress inversions to forecast magma pathways and eruptive vent location. Sci. Adv. 5:eaau9784. doi: 10.1126/sciadv.aau9784
Rogic, N., Cappello, A., and Ferrucci, F. (2019). Role of emissivity in lava flow ‘Distance-to-Run’ estimates from satellite-based volcano monitoring. Remote Sens. 11:662. doi: 10.3390/rs11060662
Vicari, A., Bilotta, G., Bonfiglio, S., Cappello, A., Ganci, G., Herault, A., et al. (2011a). LAV@HAZARD: a web-GIS interface for volcanic hazard assessment. Ann. Geophys. 54, 662–670. doi: 10.4401/ag-5347
Vicari, A., Ganci, G., Behncke, B., Cappello, A., Neri, M., and Del Negro, C. (2011b). Near-real-time forecasting of lava flow hazards during the 12-13 January 2011 Etna eruption. Geophys. Res. Lett. 38:L13317. doi: 10.1029/2011GL047545
Viccaro, M., and Cristofolini, R. (2008). Nature of mantle heterogeneity and its role in the short-term geochemical and volcanological evolution of Mt. Etna (Italy). Lithos 105, 272–288. doi: 10.1016/j.lithos.2008.05.001
Keywords: HOTSAT, lava volume, eruption duration, MAGFLOW, numerical simulations, lava flow hazard
Citation: Cappello A, Ganci G, Bilotta G, Corradino C, Hérault A and Del Negro C (2019) Changing Eruptive Styles at the South-East Crater of Mount Etna: Implications for Assessing Lava Flow Hazards. Front. Earth Sci. 7:213. doi: 10.3389/feart.2019.00213
Received: 30 May 2019; Accepted: 31 July 2019;
Published: 21 August 2019.
Edited by:
Roberto Sulpizio, University of Bari Aldo Moro, ItalyReviewed by:
Eugenio Nicotra, University of Calabria, ItalyLaura Pioli, University of Cagliari, Italy
Copyright © 2019 Cappello, Ganci, Bilotta, Corradino, Hérault and Del Negro. This is an open-access article distributed under the terms of the Creative Commons Attribution License (CC BY). The use, distribution or reproduction in other forums is permitted, provided the original author(s) and the copyright owner(s) are credited and that the original publication in this journal is cited, in accordance with accepted academic practice. No use, distribution or reproduction is permitted which does not comply with these terms.
*Correspondence: Annalisa Cappello, annalisa.cappello@ingv.it