- 1Department of Physical Geography, Stockholm University, Stockholm, Sweden
- 2Bolin Centre for Climate Research, Stockholm University, Stockholm, Sweden
- 3Institute for Atmospheric and Climate Science, ETH Zürich, Zurich, Switzerland
- 4Geophysical Institute, University of Bergen, Bergen, Norway
- 5Bjerknes Centre for Climate Research, Bergen, Norway
- 6Department of Geography, University of Zurich, Zurich, Switzerland
- 7Department of Aquatic Sciences and Assessment, Swedish University of Agricultural Sciences, Uppsala, Sweden
To better understand the small-scale variability of rainfall and its isotopic composition it is advantageous to utilize rain samplers which are at the same time low-cost, low-tech, robust, and precise with respect to the collected rainwater isotopic composition. We assessed whether a self-built version of the Kennedy sampler is able to collect rainwater consistently without mixing with antecedent collected water. We called the self-built sampler made from honey jars and silicon tubing the Zurich sequential sampler. Two laboratory experiments show that high rainfall intensities can be sampled and that the volume of water in a water sample originating from a different bottle was generally less than 1 ml. Rainwater was collected in 5 mm increments for stable isotope analysis using three (year 2011) and five (years 2015 and 2016) rain samplers in Zurich (Switzerland) during eleven rainfall events. The standard deviation of the total rainfall amounts between the different rain gauges was <1%. The standard deviation of δ18O and δ2H among the different sequential sampler bottles filled at the same time was generally <0.3‰ for δ18O and <2‰ for δ2H (8 out of 11 events). Larger standard deviations could be explained by leaking bottle(s) with subsequent mixing of water with different isotopic composition of at least one out of the five samplers. Our assessment shows that low-cost, low-tech rain samplers, when well maintained, can be used to collect sequential samples of rainfall for stable isotope analysis and are therefore suitable to study the spatio-temporal variability of the isotopic composition of rainfall.
Introduction
The stable isotopologues of water (H216O, H218O 1H2H16O, hereafter referred to as isotopes), are valuable tracers to study long-term changes in climatic conditions (Dansgaard et al., 1993), atmospheric processes at the weather system timescale (Pfahl et al., 2012), and are useful to understand how catchments transform rainfall into runoff. The regional variations in the isotopic composition of rainfall are relatively well understood thanks to the monthly dataset from the Global Network of Isotopes in Precipitation (GNIP; Aragus-Aragus et al., 2000). In contrast, the small-scale spatial variability of the isotopic composition of rainfall (<10 km2) has been much less studied and is often assumed to be homogenous. To better understand the isotopic composition of rainfall it is necessary to collect rainwater which is temporally resolved, and in a spatially distributed way.
Rainfall collection for stable isotope analysis started with holding a bottle of beer and a funnel in the rain (Dansgaard, 2004). Bottles have been used since then, e.g., for crowdsourced snapshot information of super storm Sandy (Good et al., 2014). Despite successful examples of manual sampling by Hrachowitz et al. (2011) or Graf et al. (2019) the need for several people and the right-on-time presence of staff and logistics during an event, makes this type of sampling demanding.
Higher quality information can be obtained by using rain samplers that collect sequential samples of rainwater in either volumetric or temporal intervals. Ideally, the rain sampler should collect rainwater at fixed temporal or volumetric intervals without any mixing of different samples. Furthermore, a sampler should be low-cost, compact, work autonomously, and consume low amounts of electricity. In addition, the sampler needs to be easy to handle, enable fast sample collection, and allow for repair in the field.
Different volume- or time-based rain samplers exist (Laquer, 1990). Rain samplers for stable isotope analysis can be self-built (Prechsl et al., 2014) or commercial (Gröning et al., 2012) cumulative precipitation collectors. However, as shown in several studies, the rainfall isotopic composition changes during the rainfall event (McDonnell et al., 1990; Munksgaard et al., 2012; Aemisegger et al., 2015; Fischer et al., 2017b; Graf et al., 2019). Therefore, it is crucial to collect sequential samples of rainfall with a high temporal resolution to capture these variations in stable isotope composition. Commercially available sequential samplers such as revolver type samplers (e.g., Rücker et al., 2019) usually need electricity to be operated and are costly. Self-built sequential samplers, using open low-budget microcontrollers, e.g., ArduinoTM (Aemisegger et al., 2015; Nelke and Selker, 2015; Hartmann et al., 2018; Ankor et al., 2019; Michelsen et al., 2019) are flexible but need energy, and a certain level of electronic knowledge is required. Instead, field-deployed laser spectrometers allow the isotopic composition of rainfall to be measured directly in the field at a high temporal frequency (Berman et al., 2009; Munksgaard et al., 2012; Tweed et al., 2016; von Freyberg et al., 2016). However, the high investment cost and high-tech character make it unfeasible to use this type of sampler to collect rainfall at a high spatial resolution in small catchments. In contrast to such high-tech high cost samplers, the Kennedy sequential sampler (Kennedy et al., 1979), which is used in many hydrological studies (McDonnell et al., 1990; James and Roulet, 2009; Šanda et al., 2014; Fischer et al., 2017b), meets many aforementioned requirements of an ideal sampler. However, it is not clear whether this sampler is able to collect rainwater without any mixing of subsequent samples. Therefore, in this study we built a version of the Kennedy sampler and evaluated its functioning in two ways: (1) a laboratory experiment using deionized water and a salt solution and, (2) a field experiment based on the comparison of the isotopic composition of sequentially sampled rainfall collected by multiple samplers during eleven rainfall events.
Materials and Methods
The Zurich Sequential Sampler
The Zurich sequential sampler (ZRS-sampler) is an adapted version of the Kennedy type volume-based sequential rainfall sampler (Kennedy et al., 1979) and uses low-cost materials such as 100 ml honey jars, silicon tubing, plastic connectors (which can be potentially 3D-printed), and a plastic box enclosure (Figure 1). The ZRS-sampler design resulted from experimenting with different bottles using rubber plugs, tube diameters and materials, radii of transport tubes, and air vents to minimize the mixing of new rainwater collected, with water which was previously collected and stored in the series of interconnected bottles. With too small sample volumes, water droplets in the tubing might introduce memory effects. Therefore, a bottle volume of 100 ml was chosen. To also collect data on the rainfall amounts over time, we directly connected the ZRS-sampler to a tipping bucket rain gauge by attaching a small funnel to each of the two drains at the base of the tipping bucket rain gauge (Figures 1A,D, Rain collector II – tipping bucket; 0.2 mm; Davis Instruments Corp., United States, rim height installed at 1.5 m above ground level). To each funnel, a 10 cm silicon tube is connected with a Y-connector (6–7 mm, Kartell, Italy) which is connected to the sampler with a silicon tube (1.5 m). The ZRS-sampler consists of a frame (plastic sheet, 350 × 250 × 3 mm, L × W × H), where 12 × 100 ml screw top glass bottles, each representing 5 mm of rainwater, are attached with their metal screw lids (2 × M3 screws and silicone adhesive to ensure a sealed watertight connection). The different bottles are connected serially to each other using silicon tubing (Ø 9 mm OD). To divert rainwater into a bottle, a bifurcation is made using a Y-connector connected to a 100 mm vertical silicon tube (Ø 9 mm OD) reaching the bottom of the bottles. Each bottle has a smaller second silicon tube attached to the lid (Ø 3 mm OD, 500 mm, small Ø chosen to prevent fractionation from evaporation) acting as an air vent to regulate the atmospheric pressure in each bottle and prevent the water from siphoning. For a correct functioning of the sampler, these air vents always need to be vertical. Once the water level reaches the air vent, a headspace of 0.5 cm filled with air remains, no additional rainwater can enter into the bottle, and water flows to the next empty bottle without mixing with the antecedent collected water. For transport, protection and to minimize solar radiation, each ZRS-sampler is enclosed in a plastic box (UTZ-Rako 400 × 300 × 120 mm). After the last bottle, excess rainfall flows through a tube into the plastic box. It is also possible to connect a second sequential sampler to capture large rainfall amounts.
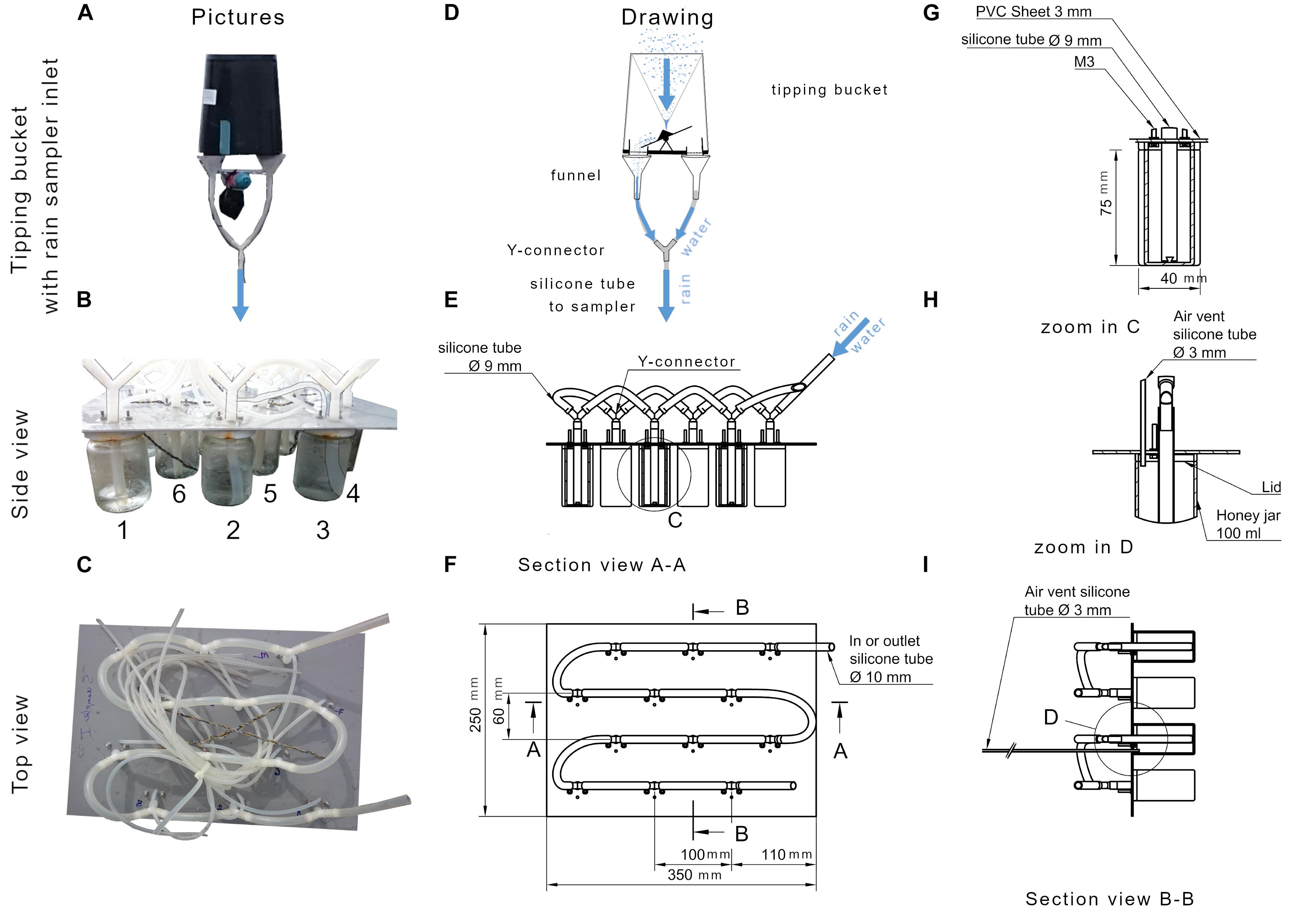
Figure 1. Overview of the ZRS-sampler tipping bucket with attached funnels (A), side view (numbers indicate sample bottles, each 100 ml or 5 mm of precipitation), side view (B) is an example of a malfunctioning sampler where in rainfall event June 2016, honey jar 1 and 2 (left to right) were leaking (not full) and therefore not properly sampling, resulting in mixing of water of different isotopic composition and top view (C). Drawing of the tipping bucket (D), section view A–A (E), top view (F), zoom in C (bottle and connections) (G), zoom in D (air vent and connection of glass) (H), and section view B–B (I). In case the study requires a high or lower temporal resolution, i.e., rainwater samples representing <5 mm or >5 mm rainfall amount, it is possible to connect honey jars with smaller or larger volumes (having a similar honey jar lid diameter).
The cost per sampler is approximately €330 including tipping bucket or €85 when using a 214 cm2 funnel instead of a tipping bucket (for price per sampler, see Supplementary Table S1).
Experimental Setup, Isotope Analysis, and Comparison
The mixing of different water samples was assessed in two laboratory experiments: I. testing the maximum rainfall intensity before sampling errors occur, and II. assessing the mixing, i.e., sampling error and memory effect within the sampler (Supplementary Material and Supplementary Figure S1, laboratory experiment).
Furthermore, the mixing of different water samples was additionally assessed by collecting rainwater during rainfall events using three (year 2011) and five (years 2015 and 2016) ZRS-samplers installed within a distance of 2 m (Supplementary Material and Supplementary Figure S2, laboratory experiment).
Results and Discussion
Laboratory Experiment
The rainfall sampler was able to collect water correctly for rainfall intensities up to 1 mm s–1 (comparable to a very high rain burst, e.g., pour 1, Supplementary Video S1). For higher rainfall intensities, which rarely occur in natural rainfall events, water entered not only in the first empty bottle but in multiple bottles (pour two onward, Supplementary Video S1).
The second laboratory experiment revealed that in some bottles, mixing of different water samples occurred, which was visible from the color of the water (Supplementary Videos S2–S6). In addition to the color indicator, the electrical conductivity increased or decreased between 0 and 50 μS cm–1, to what the electrical conductivity of the originally collected water was (Supplementary Table S2). Despite the increase or decrease in electrical conductivity due to mixing of different water samples, from the mass balance (Supplementary Equation S1), the volume of water in a water sample originating from a different bottle was generally less than 1 ml (<1% of the sample volume, 10 out of 18 bottles, Supplementary Table S2). Mixing of more than 1 ml was due to memory effects, i.e., antecedent water remained in the tubing and mixed with the newly poured water. In 3 out of 18 pours, the water was accidentally poured at rates >1 mm s–1, resulting in a non-correct sampling due to trapped air bubbles in the tubing and consequently a volume of water which mixed with water of a different bottle of more than 4 ml or more than 4% of the sample volume (Test 3 in Supplementary Table S2 and Supplementary Videos S2–S6).
These results indicate that overall the sampler can be used to collect sequential samples of rainfall with a minimal mixing between different bottles. These samples can subsequently be used to determine the isotopic composition of rainfall increments.
Sampled Rainfall Amount and Its Isotopic Composition of Different Events
The different ZRS-samplers collected rainwater samples of 11 rainfall events (Ptot 1 to 30 mm, SDPtot < 1%) with an isotopic composition that is aligned along the global meteoric water line, Figure 2, Supplementary Figure S3, and Supplementary Table S3.
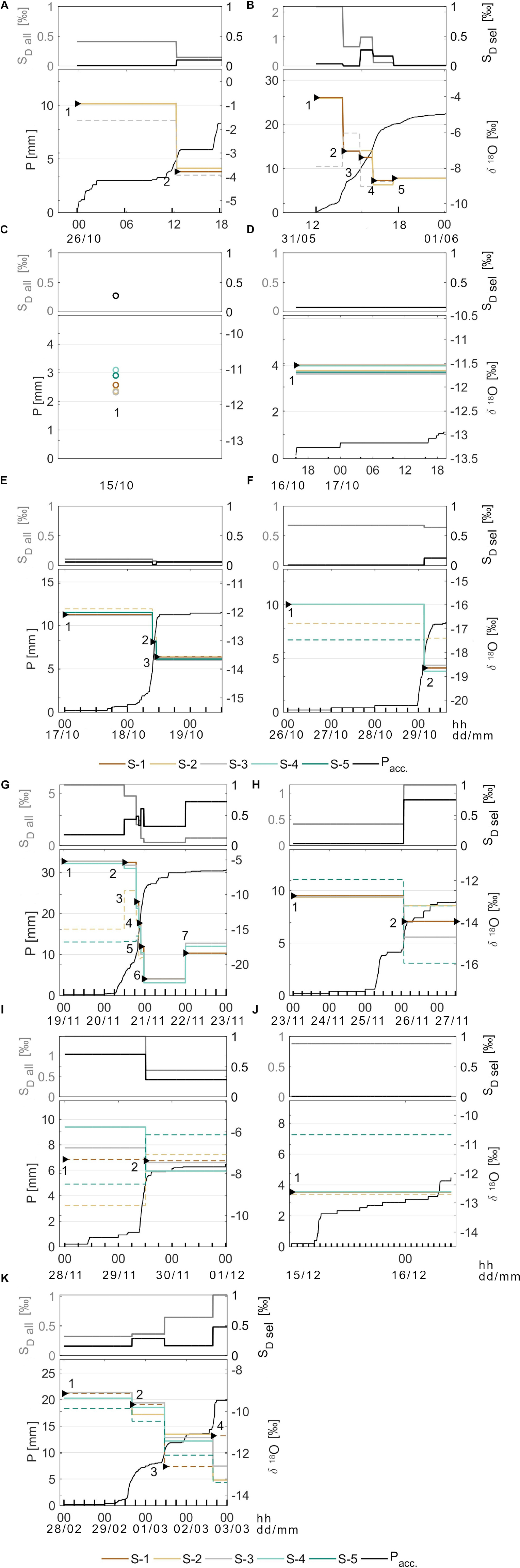
Figure 2. For the 11 rainfall events (A–K), the mean accumulated rainfall (black line, left axis) and the δ18O of rainfall (5 mm increments) of the different rain samplers (S-1 to S-5; colored lines). The triangles indicate the start of a new bottle which are consecutively numbered number 1 to n. The solid lines indicate a correct δ18O sample while a dashed line indicates δ18O samples, for which the rain samplers malfunctioned due to technical problems. On top of each panel the temporal evolution of the SD δ18O all rain samplers (gray line, left y-axis) and SD δ18O sel rain samplers excluding malfunctioning rain samplers (black line, right y-axis).
The rooftop location was chosen for practical reasons, but does not comply with WMO recommendations for rainfall measurements (WMO, 2008) because of wind exposure. However, the variation of the measured rainfall amounts between the gauges was small for all events (SD Ptot <1%). As the different ZRS-samplers received a similar amount of rainwater, it can be assumed that the isotopic composition of the water samples collected by the different samplers should be similar.
For all events with rainfall Ptot >5 mm the δ18O decreased with subsequent samples, i.e., in time (Figure 2 and Supplementary Table S3). For events with low rainfall amounts (e.g., October 15, 2015; October 16, 2015; and October 17, 2015) the last sample bottle in each sampler was partly filled. No difference in the isotopic composition between samplers with and without tipping buckets could be observed (individual samples within the SD of δ18O or δ2H to the mean δ18O or δ2H of all samplers, Supplementary Table S3). Also, no correlation was found between the rainfall amount and SD (Supplementary Figure S5). Comparing the isotopic composition of the water in the bottles filled at the same time, the SD 18O generally ranged from 0.01 to 1.5‰ and SD 2H generally ranged from 0.01 to 4‰ (Supplementary Table S3). Only for three events, some water samples taken at the same time had an SD 18O up to 5.8‰ and SD 2H up to 45‰ (Supplementary Table S3). This considerable standard deviation, which is almost as large as the temporal variability of the δ18O from bottle to bottle, can be explained by malfunctioning (leaking bottles by which water samples of different isotopic composition were mixing) of one or some ZRS-sampler(s) during the collection of samples (Figure 1B). During some events, one or more of the honey jar lids were not closed tightly enough, and water and air were leaking. This malfunctioning resulted in only partially filled bottles and mixing of different water samples (e.g., ZRS-sampler S-3 for events May 25, 2011 and June 1, 2011, and ZRS-sampler S-5 event November 19, 2015). When removing these outliers (known from field notes and indicated in Supplementary Table S3 with the letter L), the SD was generally near the laboratory analytical precision (7 out of 11 events the SD 18O <0.3‰ and SD 2H <2‰). After October 2015, the SD 18O remained higher and the samplers showed signs of aging (after sampling approximately 50 rainfall events over 4 years). The frequent screw movements of the bottles, deformed the thin honey jar lids and the seals degraded. Despite maintenance and repairs, from October 2015 onward, bottles started to leak more often and were more challenging to repair, resulting in larger differences (SD 18O >0.3‰ or SD 2 >2‰) caused by mixing of the water samples with a different isotopic composition. This shows that it is necessary to continuously monitor the state of the sampler, performing maintenance and repairs to achieve a recommended deployment time of approximately 4 years.
Conclusion and Recommendations
Our assessment of the self-built ZRS-sampler showed that by using honey jars and silicon tubing, it is possible to collect rainwater samples in incremental volumes with minimal mixing between sample bottles (less than 1 ml or 1% of the sample volume). During natural rainfall events, the standard deviation among the different sequential sampler bottles filled at the same time was generally <0.3‰ for δ18O and <2‰ for δ2H.
Similar samplers can be built from any material, e.g., honey jars, PET, beer, milk, and laboratory glass bottles and any kind of tubing. Notably, the low-cost, low-tech character makes this type of sequential sampler useful for investigating the spatial-temporal variability in the isotopic composition of rainfall.
However, to work correctly, and to minimize both technical and human errors when collecting rainwater samples for stable isotope analysis, from the findings in this study, we recommend:
1. Air vents need to be vertical at all times to function as intended.
2. It is essential to test for leaking bottles. Before sampling rainfall events this can be tested by filling the sampler with water and examining if all bottles fill with water to the level of the air vents. In the field, when samples are picked up, leaking bottles can be detected by checking if bottles are not correctly filled, e.g., water does not reach the air vent. Leaking bottles can be repaired using O-rings or by replacing the metal honey jar lid.
3. Full samplers must be lifted vertically. Tilting might cause mixing or spilling of rainwater collected in the different honey jars (bottles).
4. Before transferring the rainwater into transport bottles (labeled with the sampler number, bottle number and date) slightly shake each bottle to homogenize collected water.
5. To avoid unintentional mixing of rainwater of different rainfall events, remove excess water from the sequential sampler’s tubing (shake thoroughly), as well as from honey jars (use a cloth) and rain gauge bucket (Supplementary Figure S4). After controlling all tubing connections for leaks, the sampler is ready for a next rainfall event.
6. It should be noted that during frontal passages with substantial temperature changes or pressure drops during the event, stronger cross-contamination might occur between the samples due to pressure fluctuations within the vials. However, in an experiment we found no evidence for cross-contamination between the samples caused by an expansion of air and water due to temperature fluctuations.
Furthermore, from our experience using this sampler we recommend the following additional points be considered:
7. The water samples should be collected directly after a rainfall event. A dedicated test in a climate or sprinkling chamber, simulating different environmental changes in a controlled way, could be done to quantify the fractionation and importance of cross-contamination effects in the case of delayed collection of samples.
8. Lids and tubes should be replaced about every 4 years. Instead of using honey jars, more durable threaded caps and wide mouth bottles (100 or 250 ml; Duran or Nalgene) could be used.
Author Contributions
BF designed and built the sampler and wrote the first draft of the manuscript. BF, FA, and PG developed the field concept and design of the project and collected the data. All authors contributed to the manuscript revision, and read and approved the submitted version.
Conflict of Interest Statement
The authors declare that the research was conducted in the absence of any commercial or financial relationships that could be construed as a potential conflict of interest.
Acknowledgments
We thank all the people who helped and contributed to this study: Bruno Kägi, Claudia Schreiner, Michael Hilf, Sandra Röthlisberger, Roland Werner, Peter Isler, and Urs Beyerle. Barbara Herbstritt for the isotope analysis of rainwater samples (2015) and Valentin Mansanarez for lending his video equipment used in the laboratory experiments. In particular, we thank Heini Wernli for support and feedback to an earlier version of the manuscript and Ivan Woodhatch for his help, good humor, and useful input to the different versions of the ZRS-sampler. We thank Tracy Ewen for proofreading the manuscript, and the editor and the three reviewers for their constructive comments and suggestions that helped to significantly improve the manuscript.
Supplementary Material
The Supplementary Material for this article can be found online at: https://www.frontiersin.org/articles/10.3389/feart.2019.00244/full#supplementary-material
References
Aemisegger, F., Spiegel, J. K., Pfahl, S., Sodemann, H., Eugster, W., and Wernli, H. (2015). Isotope meteorology of cold front passages: a case study combining observations and modeling. Geophys. Res. Lett. 42, 5652–5660. doi: 10.1002/2015GL063988
Ankor, M. J., Jonathan, T. J., and Hughes, C. E. (2019). Development of an autonomous, monthly and daily, rainfall sampler for isotope research. J. Hydrol. 575, 31–41. doi: 10.1016/j.jhydrol.2019.04.074
Aragus-Aragus, L., Froehlich, K., and Rozanski, K. (2000). Deuterium and oxygen-18 isotope composition of precipitation and atmospheric moisture. Hydrol. Process. 14, 1341–1355. doi: 10.1002/1099-1085(20000615)14:8<1341::AID-HYP983>3.0.CO;2-Z
Berman, E. S. F., Gupta, M., Gabrielli, C., Garland, T., and McDonnell, J. J. (2009). High-frequency field-deployable isotope analyzer for hydrological applications. Water Resour. Res. 45, 1–7. doi: 10.1029/2009WR008265
Coplen, T. B. (2011). Guidelines and recommended terms for expression of stable-isotope-ratio and gas-ratio measurement results. Rapid Commun. Mass Spectrom. 25, 2538–2560. doi: 10.1002/rcm.5129
Dansgaard, W. (2004). Frozen Annals, Greenland Ice Sheet Research. Copenhagen: The Niels Bohr Institute for Astronomy.
Dansgaard, W., Johnsen, S. J., Clausen, H. B., Dahl-Jensen, D., Gundestrup, N. S., Hammer, C. U., et al. (1993). Evidence for general instability of past climate from a 250-kyr ice-core record. Nature 364, 218–220. doi: 10.1038/364218a0
Fischer, B. M. C., Stähli, M., and Seibert, J. (2017a). Pre-event water contributions to runoff events of different magnitude in pre-alpine headwaters. Hydrol. Res. 48, 28–47. doi: 10.2166/nh.2016.176
Fischer, B. M. C., (Ilja), van Meerveld, H. J., and Seibert, J. (2017b). Spatial variability in the isotopic composition of rainfall in a small headwater catchment and its effect on hydrograph separation. J. Hydrol. 547, 755–769. doi: 10.1016/j.jhydrol.2017.01.045
Good, S. P., Mallia, D. V., Lin, J. C., and Bowen, G. J. (2014). Stable isotope analysis of precipitation samples obtained via crowdsourcing reveals the spatiotemporal evolution of superstorm sandy. PLoS One 9:e91117. doi: 10.1371/journal.pone.0091117
Graf, P., Wernli, H., Pfahl, S., and Sodemann, H. (2019). A new interpretative framework for below-cloud effects on stable water isotopes in vapour and rain. Atmos. Chem. Phys. 19, 747–765. doi: 10.5194/acp-19-747-2019
Gröning, M., Lutz, H. O., Roller-Lutz, Z., Kralik, M., Gourcy, L., and Pöltenstein, L. (2012). A simple rain collector preventing water re-evaporation dedicated for δ18O and δ2H analysis of cumulative precipitation samples. J. Hydrol. 448–449, 195–200. doi: 10.1016/j.jhydrol.2012.04.041
Hartmann, A., Luetscher, M., Wachter, R., Holz, P., Eiche, E., and Neumann, T. (2018). Technical note: GUARD – an automated fluid sampler preventing sample alteration by contamination, evaporation and gas exchange, suitable for remote areas and harsh conditions. Hydrol. Earth Syst. Sci. 22, 4281–4293. doi: 10.5194/hess-22-4281-2018
Hrachowitz, M., Bohte, R., Mul, M. L., Bogaard, T. A., Savenije, H. H. G., and Uhlenbrook, S. (2011). On the value of combined event runoff and tracer analysis to improve understanding of catchment functioning in a data-scarce semi-arid area. Hydrol. Earth Syst. Sci. 15, 2007–2024. doi: 10.5194/hess-15-2007-2011
James, A. L., and Roulet, N. T. T. (2009). Antecedent moisture conditions and catchment morphology as controls on spatial patterns of runoff generation in small forest catchments. J. Hydrol. 377, 351–366. doi: 10.1916/j.jhydrol.2009.08.039
Kennedy, V. C., Zellweger, G. W., and Avanzino, R. J. (1979). Variation of rain chemistry during storms at two sites in northern California. Water Resour. Res. 15, 687–702. doi: 10.1029/WR015i003p00687
Laquer, F. C. (1990). Sequential precipitation samplers: a literature review. Atmos. Environ. Part A Gen. Top. 24, 2289–2297. doi: 10.1016/0960-1686(90)90322-E
McDonnell, J. J., Bonell, M., Stewart, M. K., and Pearce, A. J. (1990). Deuterium variations in storm rainfall: implications for stream hydrograph separation. Water Resour. Res. 26, 455–458. doi: 10.1029/WR026i003p00455
Michelsen, N., Laube, G., Friesen, J., Weise, S. M., Bait Said, A. B. A., and Müller, T. (2019). Technical note: a microcontroller-based automatic rain sampler for stable isotope studies. Hydrol. Earth Syst. Sci. 23, 2637–2645. doi: 10.5194/hess-23-2637-2019
Munksgaard, N. C., Wurster, C. M., Bass, A., and Bird, M. I. (2012). Extreme short-term stable isotope variability revealed by continuous rainwater analysis. Hydrol. Process. 26, 3630–3634. doi: 10.1002/hyp.9505
Nelke, M., and Selker, J. (2015). OPEnSampler. Available at: http://www.open-sensing.org/opensampler (accessed January 12, 2018).
Penna, D., Stenni, B., Šanda, M., Wrede, S., Bogaard, T. A., Gobbi, A., et al. (2010). On the reproducibility and repeatability of laser absorption spectroscopy measurements for δ2H and δ18O isotopic analysis. Hydrol. Earth Syst. Sci. 14, 1551–1566. doi: 10.5194/hess-14-1551-2010
Pfahl, S., Wernli, H., and Yoshimura, K. (2012). The isotopic composition of precipitation from a winter storm a case study with the limited-area model COSMOiso. Atmos. Chem. Phys. 12, 1629–1648. doi: 10.5194/acp-12-1629-2012
Prechsl, U. E., Gilgen, A. K., Kahmen, A., and Buchmann, N. (2014). Reliability and quality of water isotope data collected with a low-budget rain collector. Rapid Commun. Mass Spectrom. 28, 879–885. doi: 10.1002/rcm.6852
Rücker, A., Zappa, M., Boss, S., and Freyberg, J. V. (2019). An optimized snowmelt lysimeter system for monitoring melt rates and collecting samples for stable water isotope analysis. J. Hydrol. Hydromech. 67, 20–31. doi: 10.2478/johh-2018-0007
Šanda, M., Vitvar, T., Kulasová, A., Jankovec, J., and Císlerová, M. (2014). Run-off formation in a humid, temperate headwater catchment using a combined hydrological, hydrochemical and isotopic approach (Jizera Mountains, Czech Republic): catchment run-off formation: hydrology and isogeochemistry. Hydrol. Process. 28, 3217–3229. doi: 10.1002/hyp.9847
Tweed, S., Munksgaard, N., Marc, V., Rockett, N., Bass, A., Forsythe, A. J., et al. (2016). Continuous monitoring of stream δ18O and δ2H and stormflow hydrograph separation using laser spectrometry in an agricultural catchment. Hydrol. Process. 30, 648–660. doi: 10.1002/hyp.10689
von Freyberg, J., Studer, B., and Kirchner, J. W. (2016). A lab in the field: high-frequency analysis of water quality and stable isotopes in streamwater and precipitation. Hydrol. Earth Syst. Sci. Discuss. 2016, 1–32. doi: 10.5194/hess-2016-585
Keywords: rainfall and its isotopic composition, sequential rainwater sampler, laboratory experiments, field test, stable isotopes (18O and 2H), low-cost/low-tech self-built sampler
Citation: Fischer BMC, Aemisegger F, Graf P, Sodemann H and Seibert J (2019) Assessing the Sampling Quality of a Low-Tech Low-Budget Volume-Based Rainfall Sampler for Stable Isotope Analysis. Front. Earth Sci. 7:244. doi: 10.3389/feart.2019.00244
Received: 31 January 2019; Accepted: 02 September 2019;
Published: 27 September 2019.
Edited by:
Theresa Blume, German Research Centre for Geosciences, Helmholtz Centre Potsdam, GermanyReviewed by:
Ahmed M. ElKenawy, Mansoura University, EgyptChristoff Andermann, German Research Centre for Geosciences, Helmholtz Centre Potsdam, Germany
Natalie Orlowski, Albert-Ludwigs-Universität Freiburg, Germany
Copyright © 2019 Fischer, Aemisegger, Graf, Sodemann and Seibert. This is an open-access article distributed under the terms of the Creative Commons Attribution License (CC BY). The use, distribution or reproduction in other forums is permitted, provided the original author(s) and the copyright owner(s) are credited and that the original publication in this journal is cited, in accordance with accepted academic practice. No use, distribution or reproduction is permitted which does not comply with these terms.
*Correspondence: Benjamin M. C. Fischer, YmVuamFtaW4uZmlzY2hlckBuYXRnZW8uc3Uuc2U=