- 1LabMAM, Chemistry Department, Pontifical Catholic University of Rio de Janeiro, Rio de Janeiro, Brazil
- 2Alfred Wegener Institute, Helmholtz Centre for Polar and Marine Research, Bremerhaven, Germany
Branched glycerol dialkyl glycerol tetraethers (brGDGTs) are membrane lipids produced by bacteria usually ascribed to soil and peat deposits. The presence of brGDGTs in marine sediments can thus be used to track terrigenous organic matter inputs to the continental margin and to infer the local continental mean annual air temperature (MAT) and soil pH. The proxy rationale is based on the degree methylation and cyclization of the brGDGTs from terrestrial bacteria, but recently evidence was found of river and oceanic production of brGDGTs with similar configuration, indicating the necessity to better constrain the applicability of the soil brGDGTs in the marine realm. Here we considered crenarchaeol and brGDGTs obtained in 46 core-top sediments from cross-margin transects in the Campos Basin in the Southwest Atlantic, with the goal to evaluate the effectiveness of the brGDGT-associated proxies in a region in the southeastern tropical Brazilian continental margin influenced by upwelling events and low terrigenous inputs. The separation of the 5- and 6-methyl brGDGTs proved to be essential for a better evaluation of the sources of brGDGTs in the environment. Direct evidence of input of terrigenous organic matter by the medium-sized Paraíba do Sul River and other small rivers in the region were observed. More importantly, the high proportions of ring-containing brGDGTs—and the consequent increased values of the #ringstetra–in the sediments deposited between 75 and 400 m water depths (mid-shelf to upper slope) were clear evidence of marine in situ production of brGDGTs. In some stations deeper than 1,900 m, an increase in the acyclic 6-methyl hexamethylated compounds can also be ascribed to in situ production. Our results revealed that the initial soil signal is lost during transport and after river discharge in the Campos Basin, which compromise the use of brGDGTs to reconstruct the soil pH and MAT of the nearby land area.
Introduction
Glycerol dialkyl glycerol tetraethers (GDGTs) are lipids present in the cell membranes of Archaea and bacteria and occur in the environment in the isoprenoid (isoGDGTs) and branched (brGDGTs) forms (Schouten et al., 2013). While isoGDGTs are most likely to occur in the terrestrial aquatic and marine environments, brGDGTs are attributed to anaerobic and heterotrophic bacteria present in peat and soil (Pancost and Sinninghe Damsté, 2003; Weijers et al., 2006a, 2007b). Therefore, to evaluate the input and distribution of terrigenous OM in the continental margin, brGDGTs can be used as alternative proxies to the more common tracers derived from higher plants (Hopmans et al., 2004).
BrGDGT structures vary in the degree of methylation (4-6) and cyclization (up to 2 cyclopentane rings) (Weijers et al., 2006a, 2007b, Figure 1). The relative abundance of the individual forms of brGDGTs in the lipid membranes varies as a function of different environmental conditions, which could possibly be ascribed to a necessity to maintain the bacterial cellular structure and its vital functions. Weijers et al. (2007b) found a significant correlation between the cyclic brGDGTs and the pH of terrigenous materials, and between the methylation of the brGDGTs and both soil pH and the mean annual air temperature (MAT), leading to the definition of the cyclization of branched tetraethers (CBT), and methylation of branched tetraethers (MBT) indices. The MBT was later simplified by Peterse et al. (2012) to the MBT' index. Both indices are widely used in paleoenvironmental studies to evaluate soil pH and MAT, based on regional and/or global calibrations. When applied to marine sediments, brGDGT-reconstructed MAT usually corresponds well with temperatures from the nearby land (Weijers et al., 2007b; Rueda et al., 2009) in areas with a relatively high amount of terrestrial OM input (Peterse et al., 2009). However, even though the brGDGTs are predominantly terrigenous, it was demonstrated that they can potentially be derived from in situ production in the river (Zell et al., 2013a, 2015; De Jonge et al., 2014b) and in the marine realm (Peterse et al., 2009; Zhu et al., 2011; Hu et al., 2012; Liu et al., 2014; Zell et al., 2014a,b; Sinninghe Damsté, 2016), which may constrain the use of the MBT'/CBT proxies.
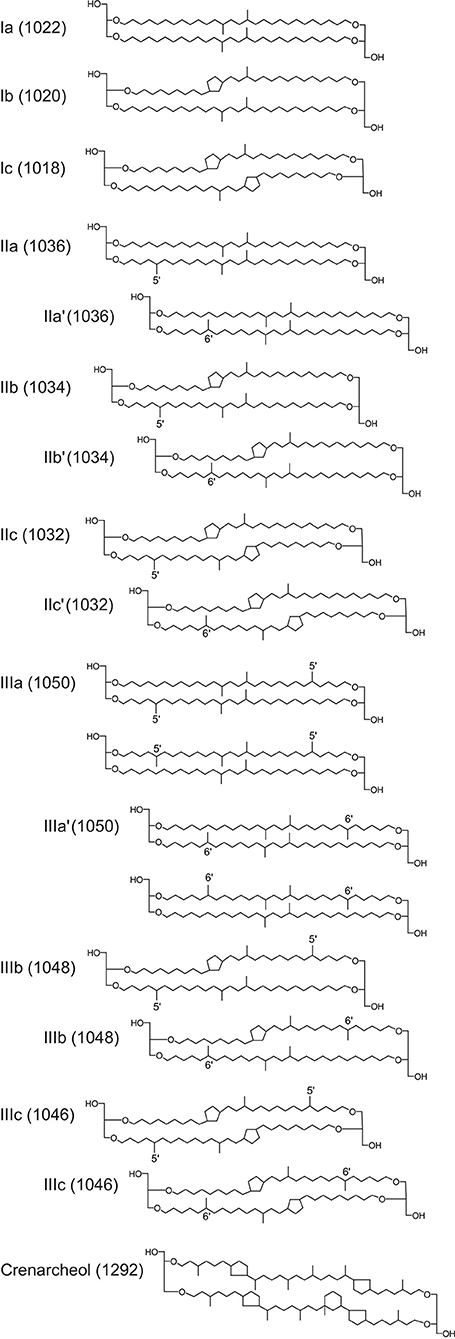
Figure 1. Chemical structure of brGDGTs and crenarchaeol with the correspondent masses (m/z) of the protonated molecules.
Recently, a new chromatographic method developed using two HPLC silica columns enabled the separation of the 5- and 6-methyl compounds (De Jonge et al., 2013, 2014a; Hopmans et al., 2016, Figure 1). This new method revealed that the 6-methyl isomer can be present in the water column and sediment from a lake, even if not detected in the soil of the lake's catchment (Weber et al., 2015). Moreover, the predominance of 6-methyl brGDGT in suspended particulate matter from a Siberian river was not consistent with the soil characteristics from the watershed (De Jonge et al., 2014b). Based on the evidences that in situ production of brGDGTs can only be ruled out by the separate quantification of the 5- and 6-methyl isomers, as well as on the observation of a good correlation between the abundance of 6-methyl brGDGTs with soil pH, De Jonge et al. (2014a) proposed the new MBT'5ME and CBT' indices. The first excludes the 6-methyl brGDGT from the equation and removes the pH dependency from the MAT global calibration reducing the residual mean error from 5 to 4.6°C, whereas the second includes the 6-methyl and reduces the error from 0.8 to 0.5 in soil pH reconstructions (Peterse et al., 2012; De Jonge et al., 2014a). The BIT index (Hopmans et al., 2004) was proposed to infer the contributions of soil OM to the ocean. It is based on the ratio of the continentally derived brGDGTs and the marine crenarchaeol, an isoprenoid GDGT produced by cosmopolitan pelagic Thaumarchaeota. In addition to the characterization of soil OM, the BIT index also provides information about the applicability of the GDGT-derived proxies in a particular region (Zhu et al., 2011). As there is evidence for limited terrestrial sources of crenarchaeol, confidence in the BIT values requires evaluation of the relative contributions of allochthonous and autochthonous sources to the total isoprenoid GDGTs, which are derived from archaea, in continental margin sediments (Weijers et al., 2006b).
The Campos Basin, in the south-eastern Brazilian continental margin, is of particular ecological and biogeochemical relevance because of the great diversity in the pelagic and benthic ecosystems (Falcão et al., 2017) that results from the influence of large to meso-scale hydrodynamic fronts in the region (Brandini et al., 2018). An important feature is the coastal and shelf break upwelling system off Cabo Frio (~23°S; Figure 2A), which supports primary production rates of 0.04–0.45 mg C m−2 d−1 (Gonzalez-Rodriguez et al., 1992; Metzler et al., 1997; Brandini et al., 2014). These values are among the highest measured in the Brazilian continental margin and contrast with the overall oligotrophic conditions induced by the warm and nutrient-poor waters transported by the Brazil Current (Silveira et al., 2017). As a consequence of the upwelling events, predominance of autochthonous OM in the basin's sediments on a regional scale is observed (Yoshinaga et al., 2008; Carreira et al., 2010; Cordeiro et al., 2018), a scenario also favored by a relatively low input of allochthonous OM (Carreira et al., 2015). The major contributor to continentally-derived materials to the Campos Basin is the Paraíba do Sul river, located to the north of the basin (Figure 2), as evidenced by isotopic and molecular markers (Souza et al., 2010; Albuquerque et al., 2014; Carreira et al., 2015), dissolved black carbon (Dittmar et al., 2012) and metals (Lacerda et al., 1993; Carvalho et al., 2002; Araujo et al., 2017). Even though the Paraíba do Sul River plays a minor role as source of material to the global oceans when compared to other rivers, the influence of Paraíba do Sul River is relevant on a regional scale (Carvalho et al., 2002).
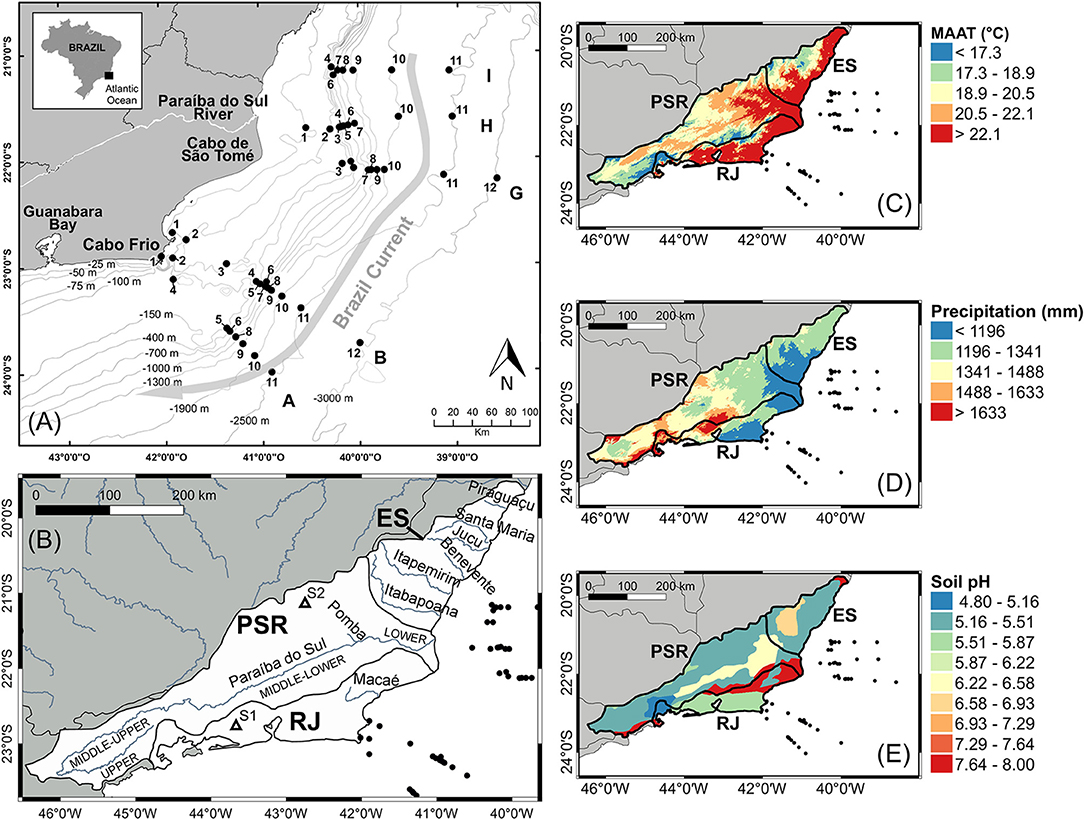
Figure 2. (A) Map of the Campos Basin with locations of the surface (0–2 cm) sediment sampling stations. Each transect (A, B, G, H, and I) was sampled on isobaths as follows: 1, 25 m; 2, 50 m; 3, 75 m; 4, 100 m; 5, 150 m; 6, 400 m; 7, 700 m; 8, 1,000 m; 9, 1,300 m; 10, 1,900 m; 11, 2,300 m, and 12, 3,000 m. (B) Map showing the three drainage basins adjacent to the Campos Basin, their main rivers and location of the two soil samples included in the global soil calibration set (Weijers et al., 2007b; Peterse et al., 2012; De Jonge et al., 2014a) used as a reference in our study. (C) Mean annual air temperature, (D) annual precipitation (Fick and Hijmans, 2017) and (E) pH of the 30 cm topsoil (FAO/IIASA/ISRIC/ISS-CAS/JRC, 2012) in the three drainage basins. PSR, Paraíba do Sul River drainage basin; RJ, coastal drainages of the Rio de Janeiro State; ES, coastal drainages of the Espírito Santo State.
The south-eastern Brazilian continental margin has proved to be a crucial region for paleoclimate studies (Rühlemann and Butzin, 2006; de Mahiques et al., 2009; Nagai et al., 2009, 2016; Souto et al., 2011; Pivel et al., 2013; Chiessi et al., 2014; Cordeiro et al., 2014; Evangelista et al., 2014; Lessa et al., 2014, 2016; Lazzari et al., 2018). The particular interest in the region stems from the relatively low input of continental material and enhanced autochthonous production due to cold and nutrient-rich deep-waters, a scenario contrasting with the overall oligotrophic conditions in the water column (Brandini et al., 2018). Here we provide for the first-time insights on the use of brGDGTs related proxies as additional tools in the ongoing research in the South-Western Atlantic. To do that, we considered the distribution of crenarchaeol and brGDGTs in core-top sediments collected along cross-margin transects (25–3,000 m water depths, Figure 2). Our main goal was to investigate the potential marine in situ production of brGDGTs that may compromise the use of the GDGT-based proxies to the sediments of the basin and contribute to the characterization of the OM.
Study Area
The Campos Basin (Figure 2) extends over an area of 100,000 km2, in the south-eastern Brazilian continental margin between 20.5°S (Vitoria High) and 24°S (Cabo Frio High). The continental shelf has an average width of 100 km and the shelf break water depth varies from 80 m in the northern area to 130 m in the south. The slope, marked by the presence of several submarine canyons and channels, extends over 40 km with an average gradient of 2.5° (Viana et al., 1998).
The circulation in the Campos Basin is mainly controlled by the Brazil Current, formed at 10°S as a southern branch of the South Equatorial Current (Peterson and Stramma, 1991). The Brazil Current flows southward following the 200 m isobath and transports the oligotrophic warm Tropical Waters in its upper levels and the nutrient-rich and cold South Atlantic Central Water (SACW) between 200 and 700 m (Campos et al., 2000). In the southern portion of the basin, south of Cabo de São Tomé (22°S), changes in the coastline orientation affects the Brazil Current flow and favor the formation of strong cyclonic and anticyclonic frontal eddies. Those features induce the intrusion of SACW into the photic zone on the shelf break and the uppermost slope (Campos et al., 2000; Calado et al., 2010). Near Cabo Frio, wind-driven intrusions of SACW occur through Ekman transport during austral spring and summer, when NE winds prevail (Rodrigues and Lorenzzetti, 2001; Castelao and Barth, 2006).
In the northern Campos Basin, the Paraíba do Sul River (Figure 2), a medium sized river with approximately 1,145 km of extension, flows into the ocean and is the major source of terrestrial material to the basin. The river drains an area of 55,400 km2 (Figures 2B–D), crossing three of the most industrialized states of Brazil: Minas Gerais, São Paulo, and Rio de Janeiro (Carvalho et al., 2002). Other rivers bring in additional terrigenous material to the Campos Basin throughout the coastal drainage basins of Rio de Janeiro and Espírito Santo States (Figure 2B) but drain much smaller catchments than the Paraíba.
The climate in the Paraíba do Sul River drainage area is subtropical with a well-defined precipitation regime. The MAT varies from 10.1°C in the upper basin to 24.1°C (Figure 2B) in the lower basin, with an average of 20.1°C (Fick and Hijmans, 2017). The average rainfall in the river basin is 1,377 mm year−1 (Figure 2D) with higher precipitation in the upper and middle sectors of the drainage basin (maxima of 2,311 mm year−1) and less humidity in the lower basin (minimum of 998 mm year−1, Fick and Hijmans, 2017). Half of the annual rainfall occurs between November and January, while the period between June and August is the driest of the year (Carvalho and Torres, 2002; Dittmar et al., 2012). Several studies in the Paraíba do Sul River have shown a strong dependence of the river water discharge and transported material on the rainfall regime (Carvalho et al., 2002; Souza and Knoppers, 2003; Souza et al., 2010; Figueiredo et al., 2011; Ovalle et al., 2013). A maximum river flow of 4,380 m3 s−1 is observed during the rainy season, when more than 80% of the total annual load is transported, decreasing to 180 m3 s−1 in the dry season (Carvalho et al., 2002; Souza et al., 2010).
The vegetation in the drainage basin of the Paraíba do Sul River is approximately 70% pastures, 27% agriculture and reforestation areas, and only 3% of the original Atlantic Forest (Ovalle et al., 2013), which is distributed over small patches (Dittmar et al., 2012). The river serves as an important water source for agriculture, industry and human use for approximately 13 million people and 5,000 industries especially in the State of Rio de Janeiro (Ovalle et al., 2013), but also receives back the sewage, generally without efficient treatment (Carvalho et al., 2002). Most of the industries are located in the middle and upper basin sectors, while extensive sugarcane plantations occupy the lower basin (Dittmar et al., 2012; Ovalle et al., 2013).
The texture of the soil along the Paraíba do Sul River basin is mainly silt/clay, with predominance of clay and sand/clay in the upper basin and clay/sand and clay/silt organic sediments (peat) in the flood-plain areas (Carvalho and Torres, 2002). The soil pH varies from 4.8 in the river's upper basin to 8.0 in the lower basin. The average soil pH across the entire drainage area adjacent to the Campos Basin is 6.1 (Figure 2E; FAO/IIASA/ISRIC/ISS-CAS/JRC, 2012).
Materials and Methods
Sampling
Surface sediment samples (0–2 cm) were collected from five cross-shelf transects (A, B, G, H, and I) along 12 isobaths (25, 50, 75, 100, 150, 400, 700, 1,000, 1,300, 1,900, 2,500, and 3,000 m) in the Campos Basin as a part of the Habitats Project (CENPES/PETROBRAS) in 2008 and 2009 (Figure 2). Undisturbed sediments were collected with an adapted large volume Van Veen sampler at the continental shelf stations and with a box corer at the slope stations.
Lipid Extraction and GDGT Analysis
Fractions of about 5 g (±0.01 g) of freeze-dried and homogenized samples were extracted by use of an accelerated solvent extraction system (ASE 200, Dionex), with a mixture of dichloromethane:methanol 9:1 (v:v) at a temperature of 100°C and a pressure of 1,000 psi. A known amount of C46-GDGT was added as internal standard prior to the extraction. The volume of the bulk extracts was rotary evaporated under vacuum and saponified for 2 h at 80°C with 1 mL of KOH (0.1 M) in methanol:H2O (9:1; v:v). The extracts were fractionated into separate fractions using silica gel column chromatography. The polar fraction containing the GDGTs was obtained by eluting with dichloromethane:methanol 1:1 (v:v) and dried under a stream of nitrogen at 40°C.
The GDGT fractions were filtered to remove particles and analyzed using an Agilent 1200 series high performance liquid chromatography system coupled via an atmospheric pressure chemical ionization interface to an Agilent 6120 mass spectrometry (HPLC–APCI-MS). A method enabling the separation of 5- and 6-methyl brGDGTs slightly modified from the one proposed by Hopmans et al. (2016) was used. In this method, the GDGTs were separated on two UPLC silica columns in series (Waters Acquity BEH HILIC, 1.7 μm, 150 mm ×2.1 mm), with a 2.1 mm x 5 mm pre-column of the same material maintained at a temperature of 30°C. Mobile phases A and B consisted of n-hexane/chloroform (99:1, v/v) and n-hexane/2-propanol/chloroform (89:10:1, v/v/v), respectively. After sample injection (20 μl) and 25 min isocratic elution with 18% mobile phase B, the proportion of B was increased to 50% within 25 min, thereafter to 100% within the next 30 min. After 5 min at 100% B and prior to the analysis of the next sample, the column was re-equilibrated with 18% of phase B for 15 min. The flow rate was 0.22 mL min−1 and resulted in a maximum back pressure of 220 bar. The total run time was 100 min.
The identification of the GDGTs was performed using single ion monitoring (SIM) as described by Schouten et al. (2007). The ion masses (m/z) monitored were 1,050, 1,048, 1,046, 1,036, 1,034, 1,032, 1,022, 1,020, 1,018 for brGDGTs, 1,292 for crenarchaeol and 744 for the C46-GDGT standard. Contents were calculated by comparing the peak areas of the GDGTs analyzed relative to the peak area of the internal standard (C46-GTGT). Due to the lack of appropriate standards, the individual response factors of the analyzed C86-GDGTs relative to the C46-GDGTs standard was not evaluated and the reported contents should be considered semi-quantitative. The extract of samples A3, G1, G2, I1, I2, and I3 were lost during handling and no results were obtained for these samples.
Calculation of the GDGT-Based Indices
The BIT index was calculated according to Hopmans et al. (2004), using the Equation (1) as follows:
Here and in all following equations, the roman numerals identify the different brGDGTs as illustrated in Figure 1, and “cren” is for crenarchaeol.
The weighted average number of cyclopentane moieties was calculated for the tetramethylated brGDGTs according to Sinninghe Damsté (2016) using Equation (2) as follows:
To evaluate the fractional abundance of the 6-methyl brGDGTs, the isomer ratio (IR) was calculated according to De Jonge et al. (2015), using Equation (3). The IRpenta and IRhexa were calculated using only the pentamethylated and hexamethylated compounds, respectively (De Jonge et al., 2014a).
The most recent CBT' and MBT'5ME indices were calculated according to De Jonge et al. (2014a), using the Equations (4) and (5), below:
The calculation of the CBT'-derived pH and the MBT'5ME-derived MAT were performed based on the global soil calibrations proposed by De Jonge et al. (2014a), using Equations (6) and (7), respectively:
Results
GDGT Distribution and BIT, IR, and #Ringstetra Indices
The content of brGDGTs in the surface sediments showed a geographic distribution similar to the total isoGDGTs (Ceccopieri et al., 2018) and to other lipid biomarkers analyzed in the Campos Basin sediments (Oliveira et al., 2013; Cordeiro et al., 2018). Higher contents of total brGDGTs were found in the slope samples on all transects and in the middle shelf samples of the CFUS region, which are areas where preferential accumulation of OM, mostly from autochthonous processes, was already demonstrated (Oliveira et al., 2013; Albuquerque et al., 2014; Cordeiro et al., 2018).
Based on the sediment TOC contents reported by Cordeiro et al. (2018), the total brGDGTs contents varied from 5 to 32 μg gTOC−1, with a sharply decreasing gradient in contents from the coastal to deeper waters (Figure 3A). A similar trend can also be observed for the contents of the acyclic brGDGTs (sum of Ia, Ia', IIa, IIa', IIIa, and IIIa', Figure 3B), with the highest contents of up to 21 μg gTOC−1 at stations near to the river mouth decreasing to 3 μg gTOC−1 in the slope region. Opposite to their content distribution, the relative abundance of the acyclic compounds increased with distance from the coast, decreasing the #ringstetra ratio to <0.4 in the downslope stations (Figure 3E). A few exceptions to this trend occur in the mid and outer shelf areas, where the lowest contribution of acyclic brGDGTs (<44%, Table 1) and the highest #ringstetra values (>0.8, Figure 3E) were observed.
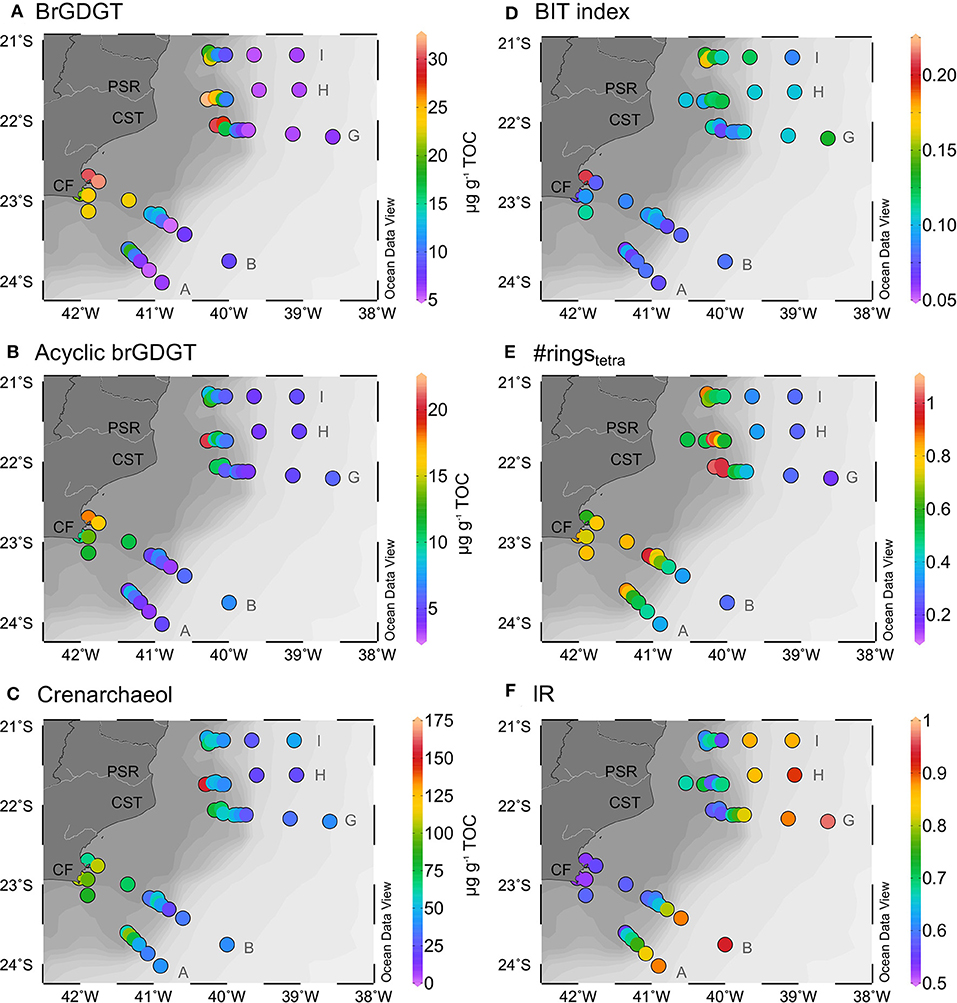
Figure 3. Contents of (A) total brGDGTs (μg gTOC−1); (B) acyclic brGDGTs (μg gTOC−1); (C) crenarchaeol (μg gTOC−1), (D) BIT index; (E) #ringstetra index and (F) IR. The total brGDGT and crenarchaeol normalized by the TOC were not determined in sample H1 because the TOC content of this sample was below quantification limit, but the contents in the sediment were comparable to sample H2 (Supplementary Table 1).
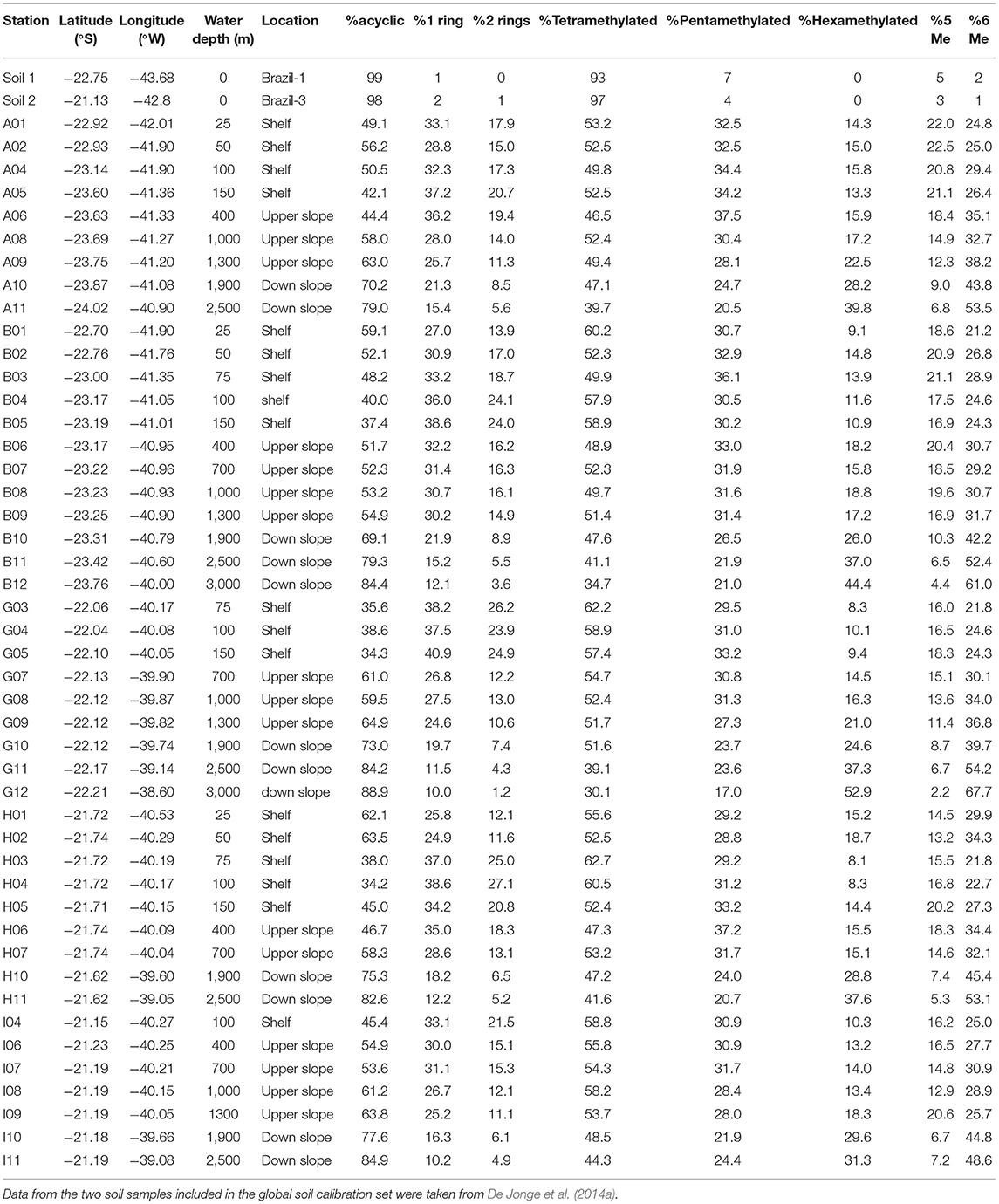
Table 1. Relative abundance (%) of each group of brGDGT in the surface sediment samples of the Campos Basin.
The tetramethylated brGDGTs (Ia, Ib, and Ic) were the most abundant in most of the samples with an average abundance of 51% (Table 1), followed by the pentamethylated (IIa, IIb, and IIc) with 29%. In the soil samples, the tetramethylated compounds comprised more than 93% of the total brGDGTs (Table 1, De Jonge et al., 2014a). The hexamethylated brGDGTs (IIIa, IIIIb, and IIIc) had an overall lower abundance (average <20% of the total brGDGTs) in the Campos Basin sediment samples but are relatively more representative in the downslope samples (>1,900 m depth, 25–53%).
The novel 6-methyl brGDGTs represented 21–68% of the total brGDGTs content (Table 1; Supplementary Table 2). Their relative abundance and the associated IR index exhibited lower values (<30% and <0.6, respectively, Figure 3F) in the inner shelf stations of transects A and B but, on the other hand, higher values (>40% or >0.8) in the down slope stations. The IRpenta presented values slightly lower than IRhexa, with averages of 0.65 and 0.75, respectively (Supplementary Table 1).
The contents of crenarchaeol varied between 21 and 153 μg gTOC−1, with higher values in the Cabo Frio coastal upwelling area and near the Paraíba do Sul River mouth (Figure 3C). The BIT index values ranged from 0.07 to 0.20 with no clear bathymetric gradient. Only the low BIT values from the slope samples of transect A were noticeable (Figure 3D).
CBT' and MBT'5ME Indices
The CBT' values varied between −0.17 and 0.39, resulting in a CBT'-based estimated pH range from 6.9 to 7.8 and an average of 7.3 ± 0.2 (Figure 4A; Supplementary Table 4). The MBT'5ME ranged from 0.70 to 0.93, resulting in MBT'5ME-based MAT varying from 13.6 to 20.8°C (Figure 4B) with an average of 16.3 ± 1.8°C. Both CBT'-based pH and MBT'5ME-based MAT became lower in the inner shelf (<75 m depth) and higher in the slope stations (>400 m depth).
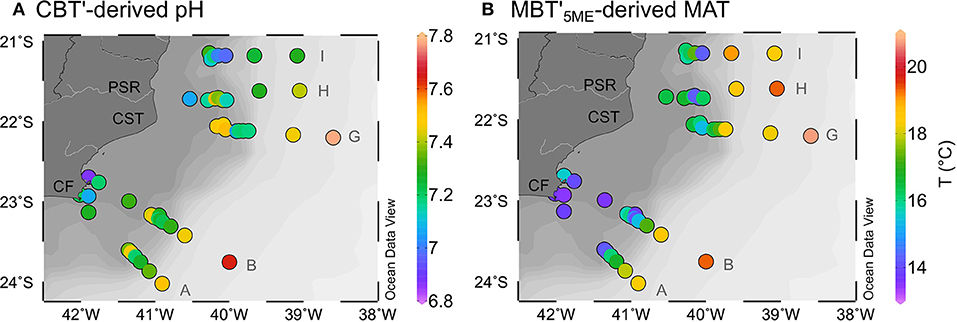
Figure 4. (A) CBT'-derived pH (De Jonge et al., 2014a); (B) MBT'5ME-derived MAT (De Jonge et al., 2014a) in the Campos Basin surface sediments.
Discussion
Sources and Fate of GDGTs in the Marine Environment
The BIT index represents the ratio between the sum of Ia, IIa, and IIIa branched compounds and crenarchaeol, and provides information on soil OM contributions to the marine sediments. The BIT is expected to be near 0 in sediments from the open ocean and near 1 in soils (Hopmans et al., 2004), and thus a decreasing trend in the BIT values is usually observed with distance from the river mouth (Hopmans et al., 2004; Zell et al., 2014a,b; Sinninghe Damsté, 2016). The data for the Campos Basin showed no such trend, as all samples exhibited low (<0.20) BIT values (Figure 3C). Similar behavior was observed in several systems (Herfort et al., 2006; Schmidt et al., 2010; Fietz et al., 2011; Smith et al., 2012) and were attributed to a higher influence of in situ production of crenarchaeol than the continental input of brGDGTs upon the BIT values, which in turn limit the application of this index to trace soil organic carbon in the ocean. In fact, the TOC-normalized crenarchaeol contents were higher at the shelf stations of both the transects influenced by upwelling (A and B) and by the Paraíba do Sul River flow (H and I). This process is favored by aggregation of the small cells of Thaumarchaeota with phytoplankton and other suspended matter (Wuchter et al., 2005; Huguet et al., 2006, 2007; Kim et al., 2008), which seems to also occur in our study region as discussed in a related previous paper (Ceccopieri et al., 2018).
A gradient of decreasing concentration in brGDGTs is commonly observed along the river-estuary-coastal ocean continuum (Hopmans et al., 2004; Kim et al., 2006; Walsh et al., 2008; Zhu et al., 2011; Hu et al., 2012; Zell et al., 2014a,b; Sinninghe Damsté, 2016). This may be explained considering a major production of these compounds by soil bacteria and later transport by rivers to the sea. The brGDGT content in the shelf is dependent on the river flow and dilution with other terrestrial and marine-derived materials. The TOC-normalized brGDGTs distribution in the Campos Basin show the same trend, as high content in the shelf samples (depth <75 m) and a gradual decrease toward deeper stations on the slope and rise in all transects is observed (Figure 3A). Two samples with the highest contents are close to the Paraíba do Sul River mouth (25 and 50 m depths, transect H), which confirm the role of this river as the main source of brGDGTs to the Campos Basin. In addition, relatively high contents of TOC-normalized brGDGTs (up to 31 μg gTOC−1) were also found at shallow stations from transects A and B, in the southern basin near Cabo Frio (Figure 1), suggesting coastal transport of continental materials delivered by small rivers along the coast, including contribution from the Paraíba do Sul river, located further north (Albuquerque et al., 2014).
The relative contributions of acyclic and cyclic compounds showed marked differences along the sedimentary provinces in the margin. The acyclic compounds represented more than 50–60% of the total brGDGTs in most of the samples at all depths in all transects (A, B, G, H, and I; Table 1). Also in Table 1 the two soils from the Paraíba do Sul River drainage basin included in the global calibration of De Jonge et al. (2014a), with more than 97% of brGDGTs being acyclic. Contrary to this general predominance of acyclic compounds, however, in several samples at 75–150 m (mid to outer shelf) and at 400 m (upper slope) water depths, the acyclic compounds were <40% of the brGDGTs, and consequently the #ringstetra exhibited the highest values (Figure 3E). In fact, the abundance of the correspondent cyclic compounds was higher in these samples compared to the shallow stations, closer to the coast and more influenced by the continental discharges of cyclic brGDGTs (Table 1). This points out to an autochthonous production of cyclic brGDGTs in the intermediate water depths (75–400 m) of the Campos Basin. The same process was also observed at intermediate water depths (i.e., 50–500 m) in many other margins worldwide, like the East China Sea (Zhu et al., 2011), the Portuguese Margin (Zell et al., 2015), the Berau Delta (Sinninghe Damsté, 2016), and the Svalbard fjords (Peterse et al., 2009; Dearing Crampton-Flood et al., 2019). However, the exact process inducing the in situ production of brGDGTs is still unclear (Dearing Crampton-Flood et al., 2019). In some places they appear to be derived from heterotrophic bacterial activity thriving in the alkaline pore waters of productive sediments (Peterse et al., 2009; Zhu et al., 2011; Weijers et al., 2014; Zell et al., 2015; Sinninghe Damsté, 2016; Dearing Crampton-Flood et al., 2019). The sediments in the depth-range where in situ production of brGDGTs seems to occur (75–400 m) are also enriched in labile organic matter (Cordeiro et al., 2018), which could support an enhanced bacterial activity in these sediments of the Campos Basin.
It is also noteworthy that the contribution of cyclic compounds (one or two rings, i.e., Ib, IIb, IIb', IIIb, IIIb', and Ic, IIc, IIc', IIIc, and IIIc') decreases for the stations from mid to the lower slope (Table 1). This was reflected in the lowering of the index #ringtetra of these deep-water samples (Figure 3E). In the stations from 1,900 to 3,000 m, the acyclic compounds (Ia, Ia', IIa, IIa', IIIa, and IIIa') represented more than 69% of the total brGDGTs. Moreover, the TOC-normalized contents of the acyclic 6-methyl hexamethylated brGDGT (IIIa') were higher in the lower slope stations in all transects (Figure 5; Supplementary Table 3). Similarly increased proportions of acyclic brGDGTs were observed in the anoxic and OM-rich sediments of the Black Sea and Cariaco Basin (Liu et al., 2014), but for the oxic sediments and the water column of the Campos Basin further research is needed to understand this process.
The separation and individual quantification of the 5- and 6-methyl brGDGTs provided new insights about the production and sources of autochthonous and allochthonous brGDGTs in aquatic systems (De Jonge et al., 2014a, 2016; Weber et al., 2015; Dang et al., 2016; Naafs et al., 2017). In particular, the relative abundance of 6-methyl brGDGTs showed potential to be used as an indicator of aquatic in situ produced compounds to the brGDGT signature in continental margin sediments (De Jonge et al., 2015, 2016; Sinninghe Damsté, 2016). In the Campos Basin surface sediments, the 6-methyl brGDGT predominates over the 5-methyl brGDGTs in all stations, including the ones close to the river mouth, representing on average a contribution to total brGDGTs of 34 and 15% (Table 1), respectively. These are values much higher than the fractional abundance found by De Jonge et al. (2014a) in the two soils from the global calibration considered here (average of 4% for the 5-methyl and 2% for the 6-methyl isomers, Table 1). From the cyclization and methylation degree perspective, the brGDGT distribution in the shallow stations near the river outflow were also strongly different from the soil samples (Table 1), as can be clearly seen in the ternary plots of Figures 6C,D. This means that the soil signal is lost before the river discharge into the ocean and a considerable riverine in situ production of brGDGTs is possibly occurring inside the Paraiba do Sul River. Additionally, IR values (>0.8, Figure 3F) of the down slope sediments, coupled to an increase in the TOC-normalized contents of the acyclic 6-methyl hexamethylated brGDGT (Figure 5) in the same stations, as previously shown, seem to support the idea of a local marine in situ production at deeper stations. This means that the 6-methyl brGDGTs is probably related to aquatic in situ production not only in lakes or river systems, but also in the marine environment. It is important to highlight that IRpenta and IRhexa correlate (R = 0.82), which indicates a common source of the 6-methyl isomers for both penta- and hexamethylated brGDGTs.
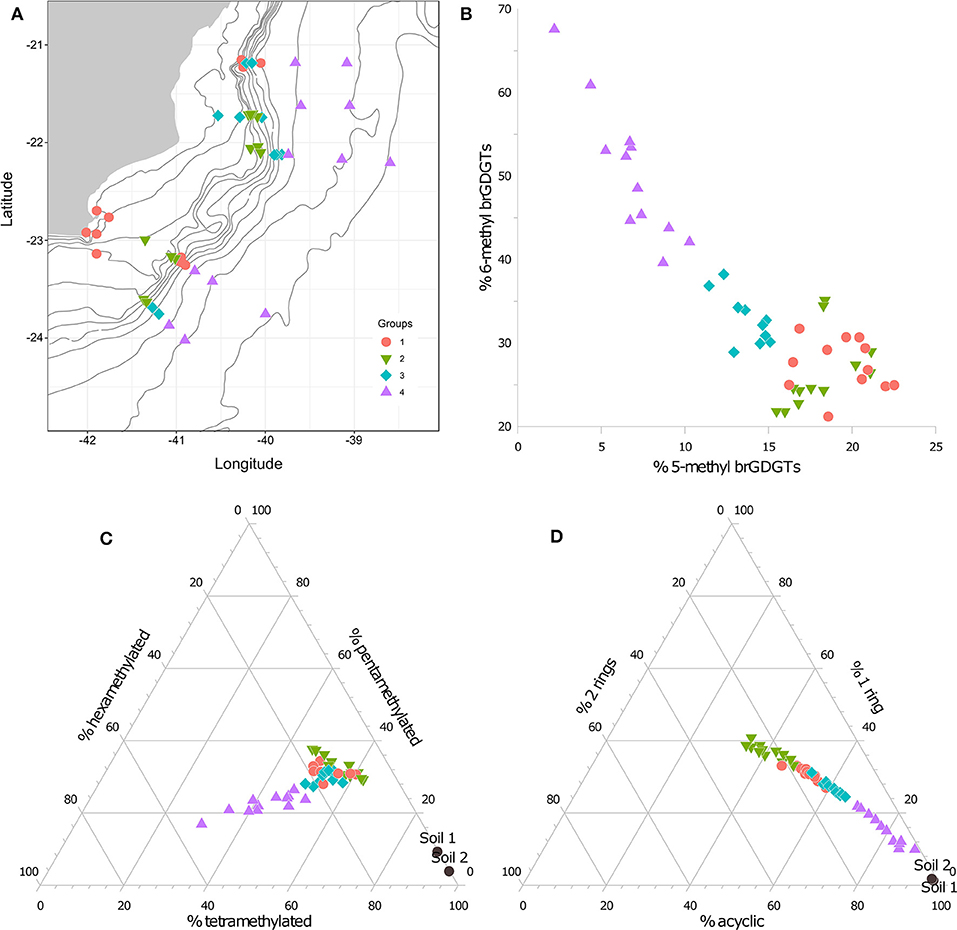
Figure 6. (A) Distribution of groups using Ward's hierarchical cluster analysis based on the brGDGTs relative abundance. Group 1 is characterized by samples with predominance of tetramethylated and acyclic brGDGTs; group 2 represents the samples with predominance of cyclic over acyclic brGDGTs and the highest #ringstetra; group 3 represents the samples that are more influenced by the riverine input; group 4 represents the lower slope and rise samples (>1,900 m) with predominance of non-cyclic brGDGTs and relevant contribution of 6-methyl hexamethylated compounds. (B) Cross plots of the relative abundance of the 5- and 6-methyl brGDGTs. (C) Ternary diagram of the relative abundance of the tetra-, penta- and hexamethylated brGDGTs. (D) Ternary diagram of the relative abundance of the acyclic and cyclic (with 1 and 2 cyclopentane rings in the structure) brGDGTs.
Applying Ward's hierarchical clustering method (Ward, 1963) with the dataset of relative distribution of brGDGT allowed the division of samples in four groups (Figure 6A). This statistical approach confirmed the previous observations on the distribution of brGDGTs. Group 1 comprised the coastal upwelling samples of transect A and B, the upper slope samples of transect B and some of the outer-shelf and upper slope samples of transect I, which is characterized by predominance of tetramethylated (Figure 6B) and acyclic compounds (Figure 6D). Group 2 represents all the samples that contain a predominance of cyclic over acyclic brGDGTs (Figure 6D) and, consequently, the highest #ringstetra. Therefore, group 2 encompass the intermediate water depths samples (75–400 m) where in situ production of cyclic compounds occurs, as previously discussed (see previous discussion). Group 3 comprises both the samples most strongly influenced by continental inputs (H1 and H2) and the upper slope samples of transects A, G and I. These samples are characterized by high IR values with 6-methyl brGDGTs representing more than twice the amount of the 5-methyl brGDGTs (Figure 6B). Group 4 includes the lower slope and rise samples (>1,900 m) that contain a predominance of acyclic brGDGTs (Figure 6D) with considerable contribution of 6-methyl (Figure 6B) and hexamethylated (Figure 6D) compounds, with a particular increase in the content of the IIIa' compound, probably caused by a marine in situ production at deeper stations.
Implications for Soil pH and Continental MAT Reconstructions in the Campos Basin Sediments
The brGDGTs in the marine sediments were first assumed to represent an integrated signal of the entire river basin from where the soil brGDGTs derive (Weijers et al., 2007a). However, it has been shown that the reconstructed MAT and soil pH can be biased toward a certain area and/or period of increased input of brGDGTs to the river (Freymond et al., 2017). In addition, the hydrodynamics and microbial activity during fluvial transport might influence the distribution of brGDGTs along the river (Zell et al., 2013a,b, 2014a; De Jonge et al., 2014b, 2016), and the marine in situ production of brGDGTs may affect the original signal of the soil-derived material (Peterse et al., 2009; Zhu et al., 2011; Hu et al., 2012; Liu et al., 2014; Zell et al., 2014a,b; Sinninghe Damsté, 2016). These effects must be considered in the evaluation of whether or not the MBT'5ME and CBT' proxies reflect the MAT and soil pH of the adjacent drainage basins.
The CBT'-based pH (6.9–7.8, Figure 4A) values for all analyzed samples in the Campos Basin were within the same range of the topsoil pH measured along the Paraíba do Sul River drainage basin and along the states of RJ and ES (4.8–8.0, Figure 2E). The same can be observed when comparing the MBT'5ME-based estimated MAT (13.6–20.8°C; Figure 4B) with the MAT of the Paraíba do Sul River drainage basin (10.1–24.1°C, Figure 2B). The estimated pH of 7 for both H1 and H2 samples, the nearest to the Paraíba do Sul River, seems to reflect the soil pH from the lower basin of this river, while the MAT of 16.6°C for these samples agrees more with the upper basin temperatures. The Paraíba do Sul River discharge and the associated transport of terrigenous/riverine materials is marked by a seasonal rainfall regime, with most of the precipitation measured in the summer in the middle-upper lands (Carvalho and Torres, 2002; Ovalle et al., 2013). Therefore, our data suggest that in addition to the flow regime, factors like erosional patterns along the river bank and intermediate storage of materials may also influence the transport of terrigenous/riverine material along the Paraíba do Sul River drainage basin and final export to the coastal ocean. Additionally, and perhaps more importantly, the evidence of in situ marine production of brGDGTs in the Campos Basin revealed that the initial soil signal is overprinted by a marine brGDGT signal, especially in the zones between 75 and 400 m depth and >1,900 depth (Figure 6A). This means that reconstructed soil pH and MAT values from the marine sediments probably do not represent the actual conditions in the catchment soils. Nonetheless, our brGDGTs data and associated proxies provided useful baseline information regarding the transport and distribution of terrigenous biomarkers in offshore sediments for a relevant portion of the SE Brazilian continental margin.
Conclusions
The highest brGDGTs contents were found near the Paraíba do Sul River outflow, which confirmed this river as the main source of soil brGDGTs to the study region. The BIT seems to be more strongly influenced by the contents of crenarchaeol rather than brGDGTs and no trend from coast to open ocean was observed, which limits the use of brGDGTs as proxy for input of soil organic carbon in the study region.
The spatial heterogeneity observed for our brGDGTs highlights the existence of different sources and post-depositional effects that compromise the use of brGDGTs to reconstruct the soil pH and MAT of the nearby land area. The strong variation in the estimated pH and MAT along the cross-margin transects might be ascribed to the occurrence of two distinct zones of in situ marine production of brGDGTs: the first takes place between 75 and 400 m depth, where the brGDGTs with cyclopentane moieties are more abundant relative to the adjacent areas; and the second occurs on a smaller scale at the down slope region >1,900 m depth, where an increase in the contents of acyclic 6-methyl hexamethylated brGDGTs is observed.
Therefore, our data contribute to the general observation that the application and interpretation of brGDGTs-estimated MAT and soil pH in marine records must consider the limitations of the proxies. Finally, analyses of soil and water samples from the Paraíba do Sul River drainage basin could provide new insights regarding the potential riverine in situ production of brGDGTs in the Paraíba do Sul River.
Data Availability Statement
The datasets generated for this study are available on request to the corresponding author.
Author Contributions
MC, RC, AW, and GM contributed conception and design of the study. MC and JH executed chemical analysis and organized the database. MC performed the statistical analysis and wrote the first draft of the manuscript. All authors contributed to manuscript revision, read and approved the submitted version.
Funding
This study was funded in part by the Coordenação de Aperfeiçoamento de Pessoal de Nível Superior—Brasil (CAPES)—Finance Code 001. MC thanks the National Council for Scientific and Technological Development (CNPq, grant 142451/2015-0), CAPES (grant PDSE 88881.134877/2016-01), and the German Academic Exchange Service (DAAD, short-term grant 2015/91591964) for her Ph.D. scholarships. RC was supported by research fellowships from CNPq (grant 309347/2017-3) and CAPES (grant 88881.121009/2016-01). The research was partially funded by CNPq (process 402459/2012-1).
Conflict of Interest
The authors declare that the research was conducted in the absence of any commercial or financial relationships that could be construed as a potential conflict of interest.
Acknowledgments
The authors thank PETROBRAS (Brazilian Petroleum Company) for providing samples under the Habitats project. Our thanks to Ralph Kreutz and Dr. Enno Schefuβ (MARUM, University of Bremen) for assistance with the sediment extractions and to Prof. Carlos Massone for helping with the statistical analysis using software R. We also thank the comments provided by two reviewers which significantly contributed to improve the manuscript.
Supplementary Material
The Supplementary Material for this article can be found online at: https://www.frontiersin.org/articles/10.3389/feart.2019.00291/full#supplementary-material
References
Albuquerque, A. L. S., Belém, A. L., Zuluaga, F. J. B., Cordeiro, L. G. M., Mendoza, U., Knoppers, B. A., et al. (2014). Particle fluxes and bulk geochemical characterization of the Cabo Frio Upwelling System in Southeastern Brazil: sediment trap experiments between spring 2010 and summer 2012. An. Acad. Bras. Cienc. 86, 601–619. doi: 10.1590/0001-37652014107212
Araujo, B. F., Hintelmann, H., Dimock, B., Almeida, M. G., and Rezende, C. E. (2017). Concentrations and isotope ratios of mercury in sediments from shelf and continental slope at Campos Basin near Rio de Janeiro, Brazil. Chemosphere 178, 42–50. doi: 10.1016/j.chemosphere.2017.03.056
Brandini, F. P., Nogueira, M., Simião, M., Codina, J. C. U. J., and Almeida Noernberg, M. (2014). Deep chlorophyll maximum and plankton community response to oceanic bottom intrusions on the continental shelf in the South Brazilian Bight. Cont. Shelf Res. 89, 61–75. doi: 10.1016/j.csr.2013.08.002
Brandini, F. P., Tura, P. M., and Santos, P. P. G. M. (2018). Ecosystem responses to biogeochemical fronts in the South Brazil Bight. Prog. Oceanogr. 164, 52–62. doi: 10.1016/j.pocean.2018.04.012
Calado, L., da Silveira, I. C. A., Gangopadhyay, A., and de Castro, B. M. (2010). Eddy-induced upwelling off Cape São Tomé (22°S, Brazil). Cont. Shelf Res. 30, 1181–1188. doi: 10.1016/j.csr.2010.03.007
Campos, E. J. D., Velhote, D., and Silveira, I. C. A. (2000). Shelf break upwelling driven by Brazil current cyclonic meanders. Geophys. Res. Lett. 27, 751–754. doi: 10.1029/1999GL010502
Carreira, R. S., Araújo, M. P., Costa, T. L. F., Ansari, N. R., and Pires, L. C. M. (2010). Lipid biomarkers in deep sea sediments from the Campos Basin, SE Brazilian continental margin. Org. Geochem. 41, 879–884. doi: 10.1016/j.orggeochem.2010.04.017
Carreira, R. S., Cordeiro, L. G. M. S., Oliveira, D. R. P., Baêta, A., and Wagener, A. L. R. (2015). Source and distribution of organic matter in sediments in the SE Brazilian continental shelf influenced by river discharges: an approach using stable isotopes and molecular markers. J. Mar. Syst. 141, 80–89. doi: 10.1016/j.jmarsys.2014.05.017
Carvalho, C., and Torres, J. P. M. (2002). “The ecohydrology of the Paraíba do Sul River, Southeast Brazil,” in The Ecohydrology of South America Rivers and Wetlands, ed M. McClain (Wallingford: International Association of Hydrological Sciences, 179–191.
Carvalho, C. E. V., Salomão, M. S. M. B., Molisani, M. M., Rezende, C. E., and Lacerda, L. D. (2002). Contribution of a medium-sized tropical river to the particulate heavy-metal load for the South Atlantic Ocean. Sci. Total Environ. 284, 85–93. doi: 10.1016/S0048-9697(01)00869-5
Castelao, R. M., and Barth, J. A. (2006). Upwelling around Cabo Frio, Brazil: the importance of wind stress curl. Geophys. Res. Lett. 33:L03602. doi: 10.1029/2005GL025182
Ceccopieri, M., Carreira, R. S., Wagener, A. L. R., Hefter, J. H., and Mollenhauer, G. (2018). On the application of alkenone- and GDGT-based temperature proxies in the south-eastern Brazilian continental margin. Org. Geochem. 126, 43–56. doi: 10.1016/j.orggeochem.2018.10.009
Chiessi, C.M., Mulitza, S., Groeneveld, J., Silva, J. B., Campos, M. C., and Gurgel, M. H. C. (2014). Variability of the Brazil current during the late Holocene. Palaeogeogr. Palaeoclimatol. Palaeoecol. 415, 28–36. doi: 10.1016/j.palaeo.2013.12.005
Cordeiro, L. G. M. S., Belem, A. L., Bouloubassi, I., Rangel, B., Sifeddine, A., Capilla, R., et al. (2014). Reconstruction of southwestern Atlantic sea surface temperatures during the last Century: Cabo Frio continental shelf (Brazil). Palaeogeogr. Palaeoclimatol. Palaeoecol. 415, 225–232. doi: 10.1016/j.palaeo.2014.01.020
Cordeiro, L. G. M. S., Wagener, A. L. R., and Carreira, R. S. (2018). Organic matter in sediments of a tropical and upwelling influenced region of the Brazilian continental margin (Campos Basin, Rio de Janeiro). Org. Geochem. 120, 86–98. doi: 10.1016/j.orggeochem.2018.01.005
Dang, X., Yang, H., Naafs, B. D. A., Pancost, R. D., and Xie, S. (2016). Evidence of moisture control on the methylation of branched glycerol dialkyl glycerol tetraethers in semi-arid and arid soils. Geochim. Cosmochim. Acta 189, 24–36. doi: 10.1016/j.gca.2016.06.004
De Jonge, C., Hopmans, E. C., Stadnitskaia, A., Rijpstra, W. I. C., Hofland, R., Tegelaar, E., et al. (2013). Identification of novel penta- and hexamethylated branched glycerol dialkyl glycerol tetraethers in peat using HPLC–MS2, GC–MS and GC–SMB-MS. Org. Geochem. 54, 78–82. doi: 10.1016/j.orggeochem.2012.10.004
De Jonge, C., Hopmans, E. C., Zell, C. I., Kim, J.-H., Schouten, S., and Sinninghe Damsté, J. S. (2014a). Occurrence and abundance of 6-methyl branched glycerol dialkyl glycerol tetraethers in soils: implications for palaeoclimate reconstruction. Geochim. Cosmochim. Acta 141, 97–112. doi: 10.1016/j.gca.2014.06.013
De Jonge, C., Stadnitskaia, A., Cherkashov, G., and Sinninghe Damsté, J. S. (2016). Branched glycerol dialkyl glycerol tetraethers and crenarchaeol record post-glacial sea level rise and shift in source of terrigenous brGDGTs in the Kara Sea (Arctic Ocean). Org. Geochem. 92, 42–54. doi: 10.1016/j.orggeochem.2015.11.009
De Jonge, C., Stadnitskaia, A., Hopmans, E. C., Cherkashov, G., Fedotov, A., and Sinninghe Damsté, J. S. (2014b). In situ produced branched glycerol dialkyl glycerol tetraethers in suspended particulate matter from the Yenisei River, Eastern Siberia. Geochim. Cosmochim. Acta 125, 476–491. doi: 10.1016/j.gca.2013.10.031
De Jonge, C., Stadnitskaia, A., Hopmans, E. C., Cherkashov, G., Fedotov, A., Streletskaya, I. D., et al. (2015). Drastic changes in the distribution of branched tetraether lipids in suspended matter and sediments from the Yenisei River and Kara Sea (Siberia): implications for the use of brGDGT-based proxies in coastal marine sediments. Geochim. Cosmochim. Acta 165, 200–225. doi: 10.1016/j.gca.2015.05.044
de Mahiques, M. M., Coaracy Wainer, I. K., Burone, L., Nagai, R., de Mello e Sousa, S. H., Lopes Figueira, R. C., et al. (2009). A high-resolution Holocene record on the Southern Brazilian shelf: paleoenvironmental implications. Quater. Int. 206, 52–61. doi: 10.1016/j.quaint.2008.09.010
Dearing Crampton-Flood, E., Peterse, F., and Sinninghe Damsté, J. S. (2019). Production of branched tetraethers in the marine realm: Svalbard fjord sediments revisited. Org. Geochem. 138:103907. doi: 10.1016/j.orggeochem.2019.103907
Dittmar, T., Rezende, C. E., Manecki, M., Niggemann, J., Coelho Ovalle, A. R., Stubbins, A., et al. (2012). Continuous flux of dissolved black carbon from a vanished tropical forest biome. Nat. Geosci. 5:618. doi: 10.1038/ngeo1541
Evangelista, H., Gurgel, M., Sifeddine, A., Rigozo, N. R., and Boussafir, M. (2014). South tropical Atlantic anti-phase response to Holocene Bond events. Palaeogeogr. Palaeoclimatol. Palaeoecol. 415, 21–27. doi: 10.1016/j.palaeo.2014.07.019
Falcão, A. P. C., Curbelo-Fernandez, M. P., Borges, A. L. N., Filgueiras, V. L., Kowsmann, R. O., and Martins, R. P. (2017). “Importância ecológica e econômica da Bacia de Campos: ambiente transicional na margem continental do Oceano Atlântico Sudoeste,” in Ambiente Bentônico: Caracterização Ambiental Regional Da Bacia de Campos, Atlântico Sudoeste, Vol. 3, eds M. P. Curbelo-Fernandez and A. C. Braga (Rio de Janeiro: Elsevier; Habitats, 1–13.
FAO/IIASA/ISRIC/ISS-CAS/JRC (2012). Harmonized World Soil Database (Version 1.2). Rome; Laxenburg: FAO; IIASA.
Fick, S., and Hijmans, R. (2017). WorldClim 2: new 1-km spatial resolution climate surfaces for global land areas. Int. J. Climatol. 37, 4302–4315. doi: 10.1002/joc.5086
Fietz, S., Martínez-Garcia, A., Huguet, C., Rueda, G., and Rosell-Melé, A. (2011). Constraints in the application of the Branched and Isoprenoid Tetraether index as a terrestrial input proxy. J Geophys. Res. Oceans 116:C10032. doi: 10.1029/2011JC007062
Figueiredo, R., Ovalle, A., Rezende, C., and Martinelli, L. (2011). Carbon and Nitrogen in the Lower Basin of the Paraíba do Sul River, Southeastern Brazil: element fluxes and biogeochemical processes. Ambiente Água Interdiscipl. J. Appl. Sci. 6, 7–37. doi: 10.4136/ambi-agua.183
Freymond, C. V., Peterse, F., Fischer, L. V., Filip, F., Giosan, L., and Eglinton, T. I. (2017). Branched GDGT signals in fluvial sediments of the Danube River basin: method comparison and longitudinal evolution. Org. Geochem. 103, 88–96. doi: 10.1016/j.orggeochem.2016.11.002
Gonzalez-Rodriguez, E., Valentin, J. L., André, D. L., and Jacob, S. A. (1992). Upwelling and downwelling at Cabo Frio (Brazil): comparison of biomass and primary production responses. J. Plankton Res. 14, 289–306. doi: 10.1093/plankt/14.2.289
Herfort, L., Schouten, S., Boon, J. P., Woltering, M., Baas, M., Weijers, J. W. H., et al. (2006). Characterization of transport and deposition of terrestrial organic matter in the southern North Sea using the BIT index. Limnol. Oceanogr. 51, 2196–2205. doi: 10.4319/lo.2006.51.5.2196
Hopmans, E. C., Schouten, S., and Sinninghe Damsté, J. S. (2016). The effect of improved chromatography on GDGT-based palaeoproxies. Org. Geochem. 93, 1–6. doi: 10.1016/j.orggeochem.2015.12.006
Hopmans, E. C., Weijers, J. W. H., Schefuß, E., Herfort, L., Sinninghe Damsté, J. S., and Schouten, S. (2004). A novel proxy for terrestrial organic matter in sediments based on branched and isoprenoid tetraether lipids. Earth Planet. Sci. Lett. 224, 107–116. doi: 10.1016/j.epsl.2004.05.012
Hu, J., Meyers, P. A., Chen, G., Peng, P., and Yang, Q. (2012). Archaeal and bacterial glycerol dialkyl glycerol tetraethers in sediments from the Eastern Lau Spreading Center, South Pacific Ocean. Org. Geochem. 43, 162–167. doi: 10.1016/j.orggeochem.2011.10.012
Huguet, C., Cartes, J. E., Sinninghe Damsté, J. S., and Schouten, S. (2006). Marine crenarchaeotal membrane lipids in decapods: implications for the TEX86 paleothermometer. Geochem. Geophys. Geosyst. 7:Q11010. doi: 10.1029/2006GC001305
Huguet, C., Schimmelmann, A., Thunell, R., Lourens, L. J., Sinninghe Damsté, J. S., and Schouten, S. (2007). A study of the TEX86 paleothermometer in the water column and sediments of the Santa Barbara Basin, California. Paleoceanography 22:PA3203. doi: 10.1029/2006PA001310
Kim, J.-H., Schouten, S., Buscail, R., Ludwig, W., Bonnin, J., Sinninghe Damsté, J. S., et al. (2006). Origin and distribution of terrestrial organic matter in the NW Mediterranean (Gulf of Lions): exploring the newly developed BIT index. Geochem. Geophys. Geosyst. 7:Q11017. doi: 10.1029/2006GC001306
Kim, J. H., Schouten, S., Hopmans, E. C., Donner, B., and Sinninghe Damsté, J. S. (2008). Global sediment core-top calibration of the TEX86 paleothermometer in the ocean. Geochim. Cosmochim. Acta 72, 1154–1173. doi: 10.1016/j.gca.2007.12.010
Lacerda, L. D., Carvalho, C. E. V., Rezende, C. E., and Pfeiffer, W. C. (1993). Mercury in sediments from the Paraíba do Sul River continental shelf, S.E. Brazil. Mar. Pollut. Bull. 26, 220–222. doi: 10.1016/0025-326X(93)90626-U
Lazzari, L., Wagener, A. L. R., Carreira, R. S., Godoy, J. M. O., Carrasco, G., Lott, C. T., et al. (2018). Climate variability and sea level change during the Holocene: insights from an inorganic multi-proxy approach in the SE Brazilian continental shelf. Quater. Int. 508, 125–141. doi: 10.1016/j.quaint.2018.11.011
Lessa, D. V. O., Ramos, R. P., Barbosa, C. F., da Silva, A. R., Belem, A., Turcq, B., et al. (2014). Planktonic foraminifera in the sediment of a western boundary upwelling system off Cabo Frio, Brazil. Mar. Micropaleontol. 106, 55–68. doi: 10.1016/j.marmicro.2013.12.003
Lessa, D. V. O., Venancio, I. M., dos Santos, T. P., Belem, A. L., Turcq, B. J., Sifeddine, A., et al. (2016). Holocene oscillations of Southwest Atlantic shelf circulation based on planktonic foraminifera from an upwelling system (off Cabo Frio, Southeastern Brazil). Holocene 26, 1175–1187. doi: 10.1177/0959683616638433
Liu, X.-L., Zhu, C., Wakeham, S. G., and Hinrichs, K.-U. (2014). In situ production of branched glycerol dialkyl glycerol tetraethers in anoxic marine water columns. Mar. Chem. 166, 1–8. doi: 10.1016/j.marchem.2014.08.008
Metzler, P. M., Glibert, P. M., Gaeta, S. A., and Ludlam, J. M. (1997). New and regenerated production in the South Atlantic off Brazil. Deep Sea Res. Part I Oceanogr. Res. Pap. 44, 363–384. doi: 10.1016/S0967-0637(96)00129-X
Naafs, B. D. A., Inglis, G. N., Zheng, Y., Amesbury, M. J., Biester, H., Bindler, R., et al. (2017). Introducing global peat-specific temperature and pH calibrations based on brGDGT bacterial lipids. Geochim. Cosmochim. Acta 208, 285–301. doi: 10.1016/j.gca.2017.01.038
Nagai, R., Martins, V., Burone, L., Wainer, I., Sousa, S., Figueira, R., et al. (2016). In-phase inter-hemispheric changes in two upwelling regions: the Southeast Brazilian and and NW Iberian margins. J. Sediment. Environ. 1, 43–67. doi: 10.12957/jse.2016.21463
Nagai, R. H., Sousa, S. H. M., Burone, L., and Mahiques, M. M. (2009). Paleoproductivity changes during the Holocene in the inner shelf of Cabo Frio, southeastern Brazilian continental margin: benthic foraminifera and sedimentological proxies. Quater. Int. 206, 62–71. doi: 10.1016/j.quaint.2008.10.014
Oliveira, D. R. P., Cordeiro, L. G. M. S., and Carreira, R. S. (2013). Characterization of organic matter in cross-margin sediment transects of an upwelling region in the Campos Basin (SW Atlantic, Brazil) using lipid biomarkers. Biogeochemistry 112, 311–327. doi: 10.1007/s10533-012-9726-z
Ovalle, A. R. C., Silva, C. F., Rezende, C. E., Gatts, C. E. N., Suzuki, M. S., and Figueiredo, R. O. (2013). Long-term trends in hydrochemistry in the Paraíba do Sul River, southeastern Brazil. J. Hydrol. 481, 191–203. doi: 10.1016/j.jhydrol.2012.12.036
Pancost, R. D., and Sinninghe Damsté, J. S. (2003). Carbon isotopic compositions of prokaryotic lipids as tracers of carbon cycling in diverse settings. Chem. Geol. 195, 29–58. doi: 10.1016/S0009-2541(02)00387-X
Peterse, F., Kim, J., Schouten, S., Klitgaard, D., Koç, N., and Sinninghe, J. S. (2009). Constraints on the application of the MBT/CBT palaeothermometer at high latitude environments (Svalbard, Norway). Org. Geochem. 40, 692–699. doi: 10.1016/j.orggeochem.2009.03.004
Peterse, F., van der Meer, J., Schouten, S., Weijers, J. W. H., Fierer, N., Jackson, R. B., et al. (2012). Revised calibration of the MBT-CBT paleotemperature proxy based on branched tetraether membrane lipids in surface soils. Geochim. Cosmochim. Acta 96, 215–229. doi: 10.1016/j.gca.2012.08.011
Peterson, R. G., and Stramma, L. (1991). Upper level circulation in the South Atlantic Ocean. Prog. Oceanogr. 26, 1–73. doi: 10.1016/0079-6611(91)90006-8
Pivel, M. A. G., Santarosa, A. C. A., Toledo, F. A. L., and Costa, K. B. (2013). The Holocene onset in the southwestern South Atlantic. Palaeogeogr. Palaeoclimatol. Palaeoecol. 374, 164–172. doi: 10.1016/j.palaeo.2013.01.014
Rodrigues, R. R., and Lorenzzetti, J. (2001). A numerical study of the effects of bottom topography and coastline geometry on the Southeast Brazilian coastal upwelling. Cont. Shelf Res. 21, 371–394. doi: 10.1016/S0278-4343(00)00094-7
Rueda, G., Rosell-melé, A., Escala, M., Gyllencreutz, R., and Backman, J. (2009). Comparison of instrumental and GDGT-based estimates of sea surface and air temperatures from the Skagerrak. Org. Geochem. 40, 287–291. doi: 10.1016/j.orggeochem.2008.10.012
Rühlemann, C., and Butzin, M. (2006). Alkenone temperature anomalies in the Brazil-Malvinas confluence area caused by lateral advection of suspended particulate material. Geochem. Geophys. Geosyst. 7:Q10015. doi: 10.1029/2006GC001251
Schmidt, F., Hinrichs, K.-U., and Elvert, M. (2010). Sources, transport, and partitioning of organic matter at a highly dynamic continental margin. Mar. Chem. 118, 37–55. doi: 10.1016/j.marchem.2009.10.003
Schouten, S., Hopmans, E. C., and Sinninghe Damsté, J. S. (2013). The organic geochemistry of glycerol dialkyl glycerol tetraether lipids: a review. Org. Geochem. 54, 19–61. doi: 10.1016/j.orggeochem.2012.09.006
Schouten, S., Huguet, C., Hopmans, E. C., Kienhuis, M. V. M., and Sinninghe Damsté, J. S. (2007). Analytical methodology for TEX86 paleothermometry by high-performance liquid chromatography/atmospheric pressure chemical ionization-mass spectrometry. Anal. Chem. 79, 2940–2944. doi: 10.1021/ac062339v
Silveira, I. C. A., Foloni Neto, H., Costa, T. P., Schmidt, A. C. K., Pereira, A. F., Castro Filho, B. M., et al. (2017). “Physical oceanography of Campos Basin continental slope and ocean region,” in Meteorology and Oceanography: Regional Environmental Characterization of the Campos Basin, Southwest Atlantic, Vol. 2, eds R. P. Martins and G. S. Grossmann-Matheson (Rio de Janeiro: Elsevier; Habitats, 135–190.
Sinninghe Damsté, J. S. (2016). Spatial heterogeneity of sources of branched tetraethers in shelf systems: the geochemistry of tetraethers in the Berau River delta (Kalimantan, Indonesia). Geochim. Cosmochim. Acta 186, 13–31. doi: 10.1016/j.gca.2016.04.033
Smith, R. W., Bianchi, T. S., and Li, X. (2012). A re-evaluation of the use of branched GDGTs as terrestrial biomarkers: implications for the BIT Index. Geochim. Cosmochim. Acta 80, 14–29. doi: 10.1016/j.gca.2011.11.025
Souto, D. D., Lessa, D. V. O., Albuquerque, A. L. S., Sifeddine, A., Turcq, B. J., and Barbosa, C. F. (2011). Marine sediments from southeastern Brazilian continental shelf: a 1200year record of upwelling productivity. Palaeogeogr. Palaeoclimatol. Palaeoecol. 299, 49–55. doi: 10.1016/j.palaeo.2010.10.032
Souza, T. A., Godoy, J. M., Godoy, M. L. D. P., Moreira, I., Carvalho, Z. L., Salomão, M. S. M. B., et al. (2010). Use of multitracers for the study of water mixing in the Paraíba do Sul River estuary. J. Environ. Radioact. 101, 564–570. doi: 10.1016/j.jenvrad.2009.11.001
Souza, W. F. L., and Knoppers, B. A. (2003). Fluxos de água e sedimentos a costa leste do Brasil: relações entre a tipologia e as pressões antrópicas. Geochim. Brasil. 17, 57–74. doi: 10.21715/gb.v17i1.198
Viana, A. R., Faugeres, J. C., Kowsmann, R. O., Lima, J. A. M., Caddah, L. F. G., and Rizzo, J. G. (1998). Hydrology, morphology and sedimentology of the Campos continental margin, offshore Brazil. Sediment. Geol. 115, 133–157. doi: 10.1016/S0037-0738(97)00090-0
Walsh, E. M., Ingalls, A. E., and Keil, R. G. (2008). Sources and transport of terrestrial organic matter in Vancouver Island fjords and the Vancouver-Washington Margin: a multiproxy approach using d13Corg, lignin phenols, and the ether lipid BIT index. Limnol. Oceanogr. 53, 1054–1063. doi: 10.4319/lo.2008.53.3.1054
Ward, J. H. (1963). Hierarchical grouping to optimize an objective function. J. Am. Stat. Assoc. 58, 236–244. doi: 10.1080/01621459.1963.10500845
Weber, Y., De Jonge, C., Rijpstra, W. I. C., Hopmans, E. C., Stadnitskaia, A., Schubert, C. J., et al. (2015). Identification and carbon isotope composition of a novel branched GDGT isomer in lake sediments: evidence for lacustrine branched GDGT production. Geochim. Cosmochim. Acta 154, 118–129. doi: 10.1016/j.gca.2015.01.032
Weijers, J. W. H., Schefuß, E., Kim, J.-H., Sinninghe Damsté, J. S., and Schouten, S. (2014). Constraints on the sources of branched tetraether membrane lipids in distal marine sediments. Org. Geochem. 72, 14–22. doi: 10.1016/j.orggeochem.2014.04.011
Weijers, J. W. H., Schefuss, E., Schouten, S., and Sinninghe Damsté, J. S. (2007a). Coupled thermal and hydrological evolution of tropical africa over the last deglaciation. Science 315, 1701–1704. doi: 10.1126/science.1138131
Weijers, J. W. H., Schouten, S., Hopmans, E. C., Geenevasen, J. A. J., David, O. R. P., Coleman, J. M., et al. (2006a). Membrane lipids of mesophilic anaerobic bacteria thriving in peats have typical archaeal traits. Environ. Microbiol. 8, 648–657. doi: 10.1111/j.1462-2920.2005.00941.x
Weijers, J. W. H., Schouten, S., Spaargaren, O. C., and Sinninghe Damsté, J. S. (2006b). Occurrence and distribution of tetraether membrane lipids in soils: implications for the use of the TEX86 proxy and the BIT index. Org. Geochem. 37, 1680–1693. doi: 10.1016/j.orggeochem.2006.07.018
Weijers, J. W. H., Schouten, S., van den Donker, J. C., Hopmans, E. C., and Sinninghe Damsté, J. S. (2007b). Environmental controls on bacterial tetraether membrane lipid distribution in soils. Geochim. Cosmochim. Acta 71, 703–713. doi: 10.1016/j.gca.2006.10.003
Wuchter, C., Schouten, S., Wakeham, S. G., and Sinninghe Damsté, J. S. (2005). Temporal and spatial variation in tetraether membrane lipids of marine Crenarchaeota in particulate organic matter: implications for TEX86 paleothermometry. Paleoceanography 20:PA3013. doi: 10.1029/2004PA001110
Yoshinaga, M., Sumida, P., and Wakeham, S. G. (2008). Lipid biomarkers in surface sediments from an unusual coastal upwelling area from the SW Atlantic Ocean. Org. Geochem. 39, 1385–1399. doi: 10.1016/j.orggeochem.2008.07.006
Zell, C., Kim, J.-H., Abril, G., Sobrinho, R. L., Dorhout, D., Moreira-Turcq, P., et al. (2013a). Impact of seasonal hydrological variation on the distributions of tetraether lipids along the Amazon River in the central Amazon basin: implications for the MBT/CBT paleothermometer and the BIT index. Front. Microbiol. 4:228. doi: 10.3389/fmicb.2013.00228
Zell, C., Kim, J.-H., Dorhout, D., Baas, M., and Sinninghe Damsté, J. S. (2015). Sources and distributions of branched tetraether lipids and crenarchaeol along the Portuguese continental margin: implications for the BIT index. Cont. Shelf Res. 96, 34–44. doi: 10.1016/j.csr.2015.01.006
Zell, C., Kim, J.-H., Fernandes, M. J., Dorhout, D., Fernandes, C., Baas, M., et al. (2014a). Transport of branched tetraether lipids from the Tagus River basin to the coastal ocean of the Portuguese margin: consequences for the interpretation of the MBT'/CBT paleothermometer. Biogeosci. Discuss. 11, 5637–5655. doi: 10.5194/bgd-11-3731-2014
Zell, C., Kim, J.-H., Moreira-Turcq, P., Abril, G., Hopmans, E. C., Bonnet, M.-P., et al. (2013b). Disentangling the origins of branched tetraether lipids and crenarchaeol in the lower Amazon River: implications for GDGT-based proxies. Limnol. Oceanogr. 58, 343–353. doi: 10.4319/lo.2013.58.1.0343
Zell, C., Kim, J. H., Hollander, D., Lorenzoni, L., Baker, P., Silva, C.G., et al. (2014b). Sources and distributions of branched and isoprenoid tetraether lipids on the Amazon shelf and fan: implications for the use of GDGT-based proxies in marine sediments. Geochim. Cosmochim. Acta 139, 293–312. doi: 10.1016/j.gca.2014.04.038
Keywords: organic matter, terrestrial biomarker, soil pH, MAT, Paraíba do Sul River, Campos Basin, in situ production
Citation: Ceccopieri M, Carreira RS, Wagener ALR, Hefter J and Mollenhauer G (2019) Branched GDGTs as Proxies in Surface Sediments From the South-Eastern Brazilian Continental Margin. Front. Earth Sci. 7:291. doi: 10.3389/feart.2019.00291
Received: 26 March 2019; Accepted: 22 October 2019;
Published: 08 November 2019.
Edited by:
Timothy Ferdelman, Max Planck Institute for Marine Microbiology (MPG), GermanyReviewed by:
Francien Peterse, Utrecht University, NetherlandsXiao-Lei Liu, University of Oklahoma, United States
Copyright © 2019 Ceccopieri, Carreira, Wagener, Hefter and Mollenhauer. This is an open-access article distributed under the terms of the Creative Commons Attribution License (CC BY). The use, distribution or reproduction in other forums is permitted, provided the original author(s) and the copyright owner(s) are credited and that the original publication in this journal is cited, in accordance with accepted academic practice. No use, distribution or reproduction is permitted which does not comply with these terms.
*Correspondence: Milena Ceccopieri, bWlsZW5hLmNlY2NvcGllcmlAZ21haWwuY29t