- 1Max Planck Institute for Biogeochemistry, Jena, Germany
- 2Aquatic Geomicrobiology, Institute of Biodiversity, Friedrich Schiller University Jena, Jena, Germany
- 3German Centre for Integrative Biodiversity Research (iDiv) Halle-Jena-Leipzig, Leipzig, Germany
- 4Hydrogeology, Institute of Geosciences, Friedrich Schiller University Jena, Jena, Germany
- 5Thüringer Landesamt für Umwelt, Bergbau und Naturschutz, Jena, Germany
Surface ecosystems are rapidly changing on a global scale and it is important to understand how this influences aquifers in the subsurface, as groundwater quality is a major concern for future generations. Dissolved organic matter (DOM) contains molecular and isotopic signals from surface-derived inputs as well as from the biotic and abiotic subsurface environment and is therefore ideal to study the connectivity between both environments. We evaluated a 3-year time series of DOM composition using ultrahigh resolution mass spectrometry and age using 14C accelerator mass spectrometry along a hillslope well transect in the fractured bedrock of the Hainich Critical Zone Exploratory, Germany. We found a wide range of DOM 14C depletion, from Δ14C = −47.9 to Δ14C = −782.4, within different zones of the shallow groundwater. The 14C content of DOM mirrored the connectivity of the aquifers to the surface. The composition of DOM was highly interrelated with its 14C age. The proportions of surface-derived DOM components decreased with DOM age, whereas microorganism-derived DOM components increased. The intensity of surface-sourced DOM signals differed between the wells and likely reflected the hydrological complexity of fractured-rock environments. During recharge, DOM was more enriched in Δ14C, contained more surface-derived molecular components and was more diverse. As a potential response to the varying DOM substrate, bacterial 16S rRNA gene analysis revealed community evolution and increased bacterial diversity during recharge. The influx of diverse, surface-derived DOM potentially fueled evolution within the autochthonous bacterial communities, as in contrast to DOM, the bacterial community did not retreat to the initial diversity and community composition during the recession period. Our results demonstrate on the one hand that combined analyses of the composition and age of groundwater DOM strongly contribute to the understanding of interconnections, community evolution and the functioning of subsurface ecosystems and on the other hand that changes in surface ecosystems have an imprint on subsurface ecosystems.
Introduction
Shallow groundwater ecosystems can rely on surface-fed inputs of carbon and nutrients, which are largely controlled by the hidden subsurface flow paths and recharge events (Ben Maamar et al., 2015; Ward et al., 2017). Along the percolation paths, molecular and isotopic signals of dissolved organic matter (DOM) are incorporated from the surface biosphere as well as from abiotic and biotic interactions in the soil and in the unsaturated and saturated subsurface compartments (Katz et al., 1998; Kujawinski et al., 2009; Kaiser and Kalbitz, 2012; Kallenbach et al., 2016; Van Stan and Stubbins, 2018; Gross and Harrison, 2019). Disentangling and tracing these integrated signals has emerged as a key objective in exploring the links between surface and subsurface systems within the Critical Zone (CZ), the near-surface environment that hosts nearly all terrestrial life (Küsel et al., 2016).
Informative subsections of the DOM pool that potentially contain signaling information can be identified using non-targeted ultrahigh resolution mass spectrometry (HR-MS; Hertkorn et al., 2008, 2013). HR-MS facilitates the analysis of the detailed molecular composition of DOM, generating information on individual molecular formulae and enabling the interpretation of molecular patterns across natural gradients (Jaffé et al., 2012; Ohno et al., 2014; Kellerman et al., 2015; Kothawala et al., 2015; Roth et al., 2015). Natural gradients of DOM age occur in groundwater following recharge events that lead to mixing or exchange of waters containing surface-sourced or in situ produced DOM (Sukhija et al., 2006; McMahon et al., 2011). Combined analyses of HR-MS and 14C age can therefore open new perspectives regarding the evolution of DOM, its sources and fate (Singer et al., 2012; Spencer et al., 2014).
Even though analyses of DOM composition and age have a long history in CZ research, holistic studies of DOM evolution in the CZ and the environmental signals that are integrated are still missing (e.g., Wassenaar et al., 1991; Purdy et al., 1992; Aravena and Wassenaar, 1993; Artinger et al., 2000). Time series of the composition of DOM can be used to identify sources, interactions and flow paths within the CZ (Jin et al., 2014; Shen et al., 2015), that all vary, for instance following groundwater recharge events, causing a high connectivity of surface and subsurface compartments (Baker et al., 2000; Kaiser and Guggenberger, 2005). Surface-derived inputs that enter an aquifer system have been shown to contain signals linking to the decay of plant biomass (Shen et al., 2015). Increasing proportions of by-products resulting from the degradation of the phenolic biopolymer lignin are therefore expected during groundwater recharge (Benk et al., 2018). Continuous sorption/desorption (Kaiser and Guggenberger, 2000; Kleber et al., 2015; Leinemann et al., 2018) and microbial processing (Grøn et al., 1992; Einsiedl et al., 2007; Simon et al., 2010; Fasching et al., 2014; Smith et al., 2018) of surface-derived DOM along its path give rise to new signals from the residues of the in situ microbial communities. Specifically, the predominance of microorganism-derived proteins and lipids are expected during the recession periods (Kallenbach et al., 2016; Kujawinski et al., 2016; Ding et al., 2018). Furthermore, the interactions of microorganisms with DOM can drive community succession (Li et al., 2013; Zhang et al., 2015; Wu et al., 2018). Previous investigations have indicated that aquifer-surface connection and recharge events are reflected in bacterial diversity and bacterial community composition (Kolehmainen et al., 2007; Li et al., 2012; Cooper et al., 2016; Hubalek et al., 2016).
We hypothesize that the infiltration of surface-derived DOM with high molecular diversity could fuel bacterial diversity and bacterial community evolution in the subsurface. To identify the molecular and isotopic signals of aquifer-surface connection and seasonal flow variation, we analyze a 3-year time series of DOM composition and age from a well transect in a fractured bedrock groundwater system. Further, we discuss potential responses of the groundwater bacterial community to changes in DOM diversity during recharge events.
Materials and Methods
Study Site and Groundwater Sampling for HR-MS Analysis
The study site is located in the Hainich Critical Zone Exploratory (CZE) in NW Thuringia (central Germany) and represents a hillslope sub-catchment of used groundwater resources under temperate climate (Figures 1A,B). The Hainich CZE monitoring well transect accesses a shallow groundwater flow system in sloping thin-bedded limestone-mudstone alternations (Lazar et al., 2019; Figure 1C). Along the eastern hillslope of the Hainich low-mountain range, the contribution areas of the wells exhibit forest, pasture and cropland use, whereas the outcrop areas of the main aquifer are predominantly covered by forest or unmanaged woodland (national park) (Kohlhepp et al., 2016). Each of the five well sites features up to three groundwater wells, giving access to different sampling depths. Lithostratigraphically, the accessed bedrock belongs to the Upper Muschelkalk (Germanic Triassic) and is of marine origin. The Upper Muschelkalk hosts minor groundwater resources in mudstone-rich strata as well as a regional main aquifer in thick bioclastic beds. Flow in the minor groundwater resources (accessed by the wells H32, H42, H43, H52, H53) was characterized as slow and diffusive, taking place in fractures of dense limestones with low primary porosity (Kohlhepp et al., 2017). The fractured to karstified regional main aquifer (accessed by the wells H31, H41, H51) allows for faster flow and surface connectivity. These differences in the presumed flow modes result in a well oxygenated regional main aquifer and partly oxygen-deficient minor groundwater resources, leading to distinctly different biogeochemical milieus regarding metabolic processes as well as microbial habitats (Opitz et al., 2014). Groundwater samples were collected every 3 months (July 2014 – May 2017) from the midslope to footslope wells (sites H3, H4, H5) that continuously provided water. Before sampling, stagnant water was removed from the wells using a submersible sampling pump (MP1, Grundfos, Denmark) until steady conditions of physicochemical parameters were reached. Ten liters of groundwater were collected in duplicate for each time point of DOM measurement. Filtration to <0.3 μm was carried out immediately on site over pre-combusted (500°C/5 h) glass-fiber filters in sequence with pumping (Schwab et al., 2017). The filtered groundwater was stored at 2°C in the dark until further processing.
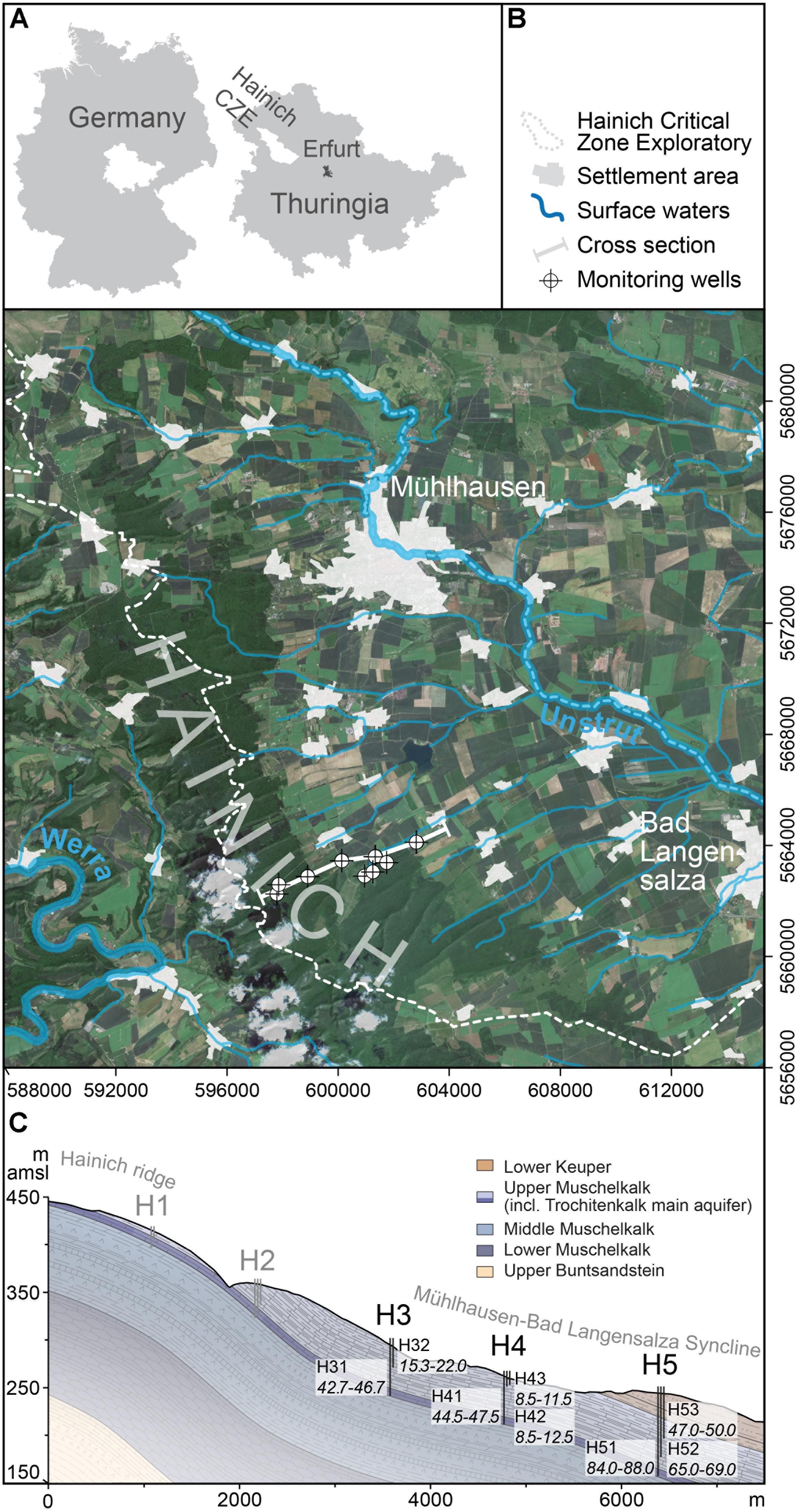
Figure 1. (A,B) Location of the study site in Central Germany. (C) Schematic cross-section of the Hainich CZE multi-well groundwater transect showing the bedrock strata configuration and the screen sections in m below ground level (after Lazar et al., 2019). Data sources: DEM GDI-Th, DLM250 GeoBasis-DE/BKG 2016; dl652 d/by-2-0, http://govdata.de/dl-de/by-2-0; satellite image: Copernicus Service information, 2018.
Sample Preparation and HR-MS Analysis
Dissolved organic matter was extracted from the filtered groundwater using solid phase extraction over PPL (styrene-divinylbenzene polymer) cartridges according to Dittmar et al. (2008). The extraction efficiency was ∼50% on a carbon basis. Together with the samples, procedural blanks of ultrapure water acidified to pH = 2 were extracted for each sampling campaign. HR-MS analysis was carried out at the Max Planck Institute for Biogeochemistry (MPI-BGC) in Jena, Germany on an Orbitrap Elite (Thermo Fisher Scientific; Makarov and Scigelova, 2004) using an UPLC system (Thermo Fisher Scientific) without chromatographic column for automated injection. The instrument was run in negative ionization mode with a continuous flow of 20 μL/min H2O/MeOH (50/50) and ESI needle voltage of 2.65 kV. 100 μL of DOM extract at a concentration of 20 mg/L were injected and 100 scans of m/z 175–1000 and maximum 200 ms ion accumulation time were acquired. Between each sample the injection system was flushed for 10 min at 100 μL/min with H2O/MeOH. Only peaks detected in both replicates and with a signal/noise ratio (S/N) >4 were analyzed further. Peak intensities were normalized to the sum intensity of the respective spectra to account for known competitive ionization effects (Hertkorn et al., 2008; Herzsprung et al., 2012). Molecular formulae were assigned constrained by a maximum stoichiometry of C ≤ 60, H ≤ 120, O ≤ 60, N ≤ 4, S ≤ 2, P ≤ 1. To identify bulk trends, only mass peaks with successful sum formula assignment and present in at least 10% of the total data set were considered for statistical analyses. Molecular subgroups were assigned based on the elemental ratios and the aromaticity index (AImod; Koch and Dittmar, 2006, 2016) according to the classification of Riedel et al. (2016). We focused on the four most abundant molecular subgroups in our dataset: “Highly unsaturated” compounds with AImod < 0.5 and H/C < 1.5, which also contain phenols with aliphatic side chains (Santl-Temkiv et al., 2013). “Polyphenols” with 0.50 < AImod < 0.66, containing next to plant-derived polyphenolic substances also carboxyl rich alicyclic molecules (CRAM; Hertkorn et al., 2006) and condensed aromatics with aliphatic side chains (Santl-Temkiv et al., 2013; Kellerman et al., 2015). “Unsaturated aliphatics” were assigned for formulae with H/C ≥ 1.5 (Riedel et al., 2016) and “peptide-like” compounds for H/C ≥ 1.5 and N ≥ 1. We determined the relative proportions of these molecular subgroups by summing the relative intensities of all the HR-MS peaks within each group. To allow comparison of the within-group trends, we normalized the relative proportion values by the group’s respective maxima.
Radiocarbon Analysis
PPL extracts were dried into tin capsules, combusted to CO2 in an elemental analyzer and subsequently graphitized. The PPL extraction procedure was checked for potential 14C contamination in a previous study (Benk et al., 2018). Radiocarbon analysis was carried out on a 3 MV Tandetron 14C accelerator mass spectrometer at the MPI-BGC in Jena, Germany, together with size-matched modern (Oxalic Acid II) and 14C-depleted standard materials (Steinhof, 2013; Steinhof et al., 2017). Age calibration was performed using OxCal 4.3.2 (Bronk Ramsey, 2017) and the IntCal13 atmospheric curve (Reimer et al., 2013). Radiocarbon data is reported as Δ14C, indicating the relative difference in activity with respect to the standard, normalized for δ13C and corrected for the decay between 1950 and the measurement time y (Trumbore et al., 2016).
The scale of Δ14C is given in parts per thousand, or ‰, and typically ranges between +1000‰ (doubling of 14C relative to 1950) and −1000‰ (no 14C in sample) in natural samples. In this study, we found only negative values of Δ14C and therefore refer to DOM in relative terms as Δ14C-old or Δ14C-depleted for strongly negative values and correspondingly as Δ14C-young or Δ14C-enriched for only slightly negative values.
Stable Isotope Analysis
Stable hydrogen (δ2H) and oxygen (δ18O) isotopes of groundwater were analyzed at the MPI-BGC in Jena, Germany, using a setup of a high-temperature reactor (TC/EA) coupled on-line via a ConFlo III interface to a Delta+ XL isotope ratio mass spectrometer (all units from Finnigan MAT). Measurements were calibrated using in-house standards. The δ2H and δ18O values of the in-house standards were regularly verified against Vienna Standard Mean Ocean water (VSMOW: δ2H = 0‰, δ18O = 0‰) and Standard Light Antarctic Precipitation (SLAP: δ2H = −428.0‰, δ18O = −55.5‰). A detailed description of the instrument method can be found in Gehre et al. (2004).
Bacterial Community Analysis
Groundwater samples for bacterial community analysis were taken every 3 months between July 2014 and May 2017 on the same days as sampling for DOM analysis. Genomic DNA of groundwater (10 L) was enriched on conventional-sized (0.2 μm) PES (Supor, Pall Corporation, until June 2016) or polycarbonate filters (Nuclepore, Whatman; Merck-Millipore, after June 2016). Filters were stored at −80°C prior to DNA extraction. Genomic DNA was extracted using a PowerSoil DNA Isolation kit (MO BIO laboratories Inc., United States). The primers Bakt_341F (CCTACGGGNGGCWGCAG) and Bakt_805R (GACTACHVGGGTATCTAATCC) (Herlemann et al., 2011) were used to amplify bacterial partial 16S rRNA genes (V3–V4 region). The amplicons were purified using NucleoSpin Gel and PCR Clean-Up Kit (Macherey-Nagel, Düren, Germany). The amplicon sequencing was performed on Illumina’s MiSeq platform using v3 chemistry (Illumina, Eindhoven, Netherlands). The detailed amplicon library preparation procedures were described by Kumar et al. (2018). The sequence data was analyzed using mothur V.1.39.5 (Schloss et al., 2009) according to the MiSeq SOP by Kozich et al. (2013). The good-quality bacterial sequences were assigned into OTUs based on VSEARCH abundance-based greedy clustering (AGC) at 97% similarity (Edgar, 2010; He et al., 2015; Rognes et al., 2016). The samples were rarefied to equal sequencing depth (3000 sequences) prior to analysis. The sequence data were deposited in the European Nucleotide Archive (ENA) and can be retrieved via their accession numbers listed in Supplementary Table S1.
Statistical Analysis and Visualization
All statistical analyses and visualizations shown in this study were done in R 3.4.1 (R Core Team, 2019), using the packages “vegan” 2.4-5 (Oksanen et al., 2018) and “ggplot2” 2.2.1 (Wickham, 2016). Principal component analysis (PCA) was performed on DOM data with base R function “prcomp.” Using function “envfit” in the package “vegan,” we fitted vectors showing the proportions of major molecular subgroups and the Δ14C values onto the DOM ordination. The data was not normalized prior to fitting. Only parameters that were determined to have a significant fit using function “envfit” are shown. The vectors indicate the direction within the ordination space of the strongest increasing gradient of the respective parameter. Principal coordinates analysis (PCoA) was performed on bacterial community composition data of well H41. The PCoA was based on Bray-Curtis dissimilarity (calculated using function “vegdist” in “vegan”) to account for the described problem of double absences, which in microbial ecology should be considered as lack of information (Ramette, 2007). Shannon alpha diversity indices were calculated using the function “diversity” in “vegan.” Rarefying bacterial community samples to equal sequencing depth and adjusting DOM samples to equal carbon concentration prior to HR-MS injection allowed comparing the resulting diversity indices of bacterial and molecular data sets.
Results and Discussion
Spatial Patterns in DOM Age
The 14C depletion of DOM in the studied aquifers ranged between Δ14C = −47.9 in well H32 and −782.4 in well H53. The wells could be classified into three groups based on their Δ14C values (Figure 2). H31, H32 and H51 possessed the least depleted organic carbon with mean Δ14C = −129.0, corresponding to calibrated 14C ages of ∼1000 years before present (BP). H42, H43, and H52 were characterized by medium 14C depletion with mean Δ14C = −386.6, representing calibrated 14C ages of ∼4500 years BP. H53 showed the strongest depletion with mean Δ14C = −722.0, equal to ∼12000 years BP. The 14C depletion of DOM from the wells in these three groups was relatively stable over time with the between-well variability being larger than the within-well variability. DOM from well H41 stood out, because its variability over the 3 years of observations was remarkably larger than in any of the other wells. The respective Δ14C maxima and minima for H41 ranged between the little depleted and the medium depleted well groups.
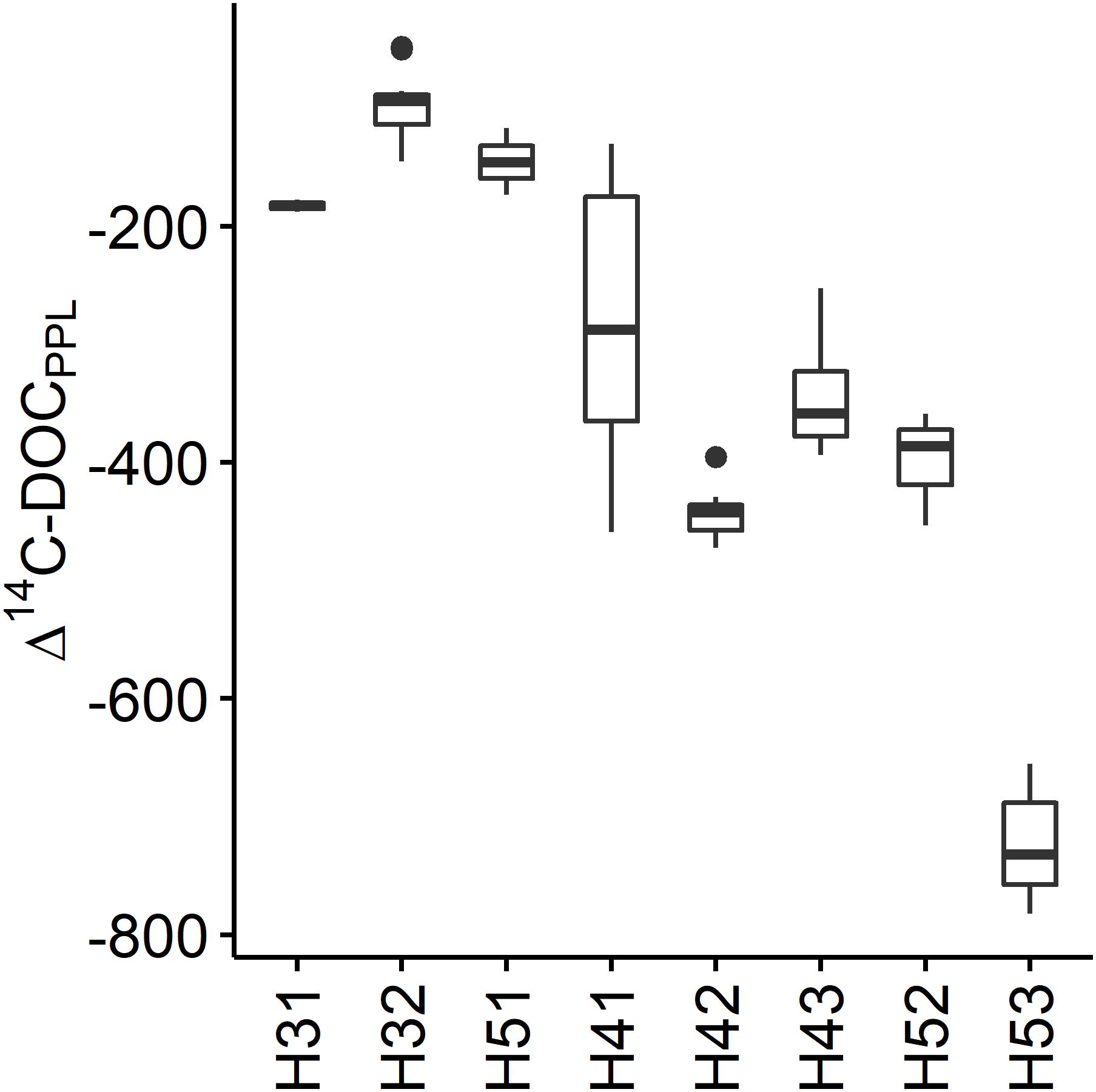
Figure 2. Distribution of Δ14C values of SPE-DOM in the Hainich CZE aquifers. Data from 07/2014 to 07/2017.
The distribution of Δ14C values between the wells followed more likely the hydrogeological than the stratigraphic layout of the aquifer system. The wells H31 and H51 are both part of the regional main aquifer that is likely well connected to a large recharge area on the Hainich ridge (Kohlhepp et al., 2017). Compared to the overlying minor groundwater resources, inflow of a relatively larger proportion of surface-derived modern carbon contributing to 14C-enriched DOM in those wells is therefore probable. H32, the other well with 14C-enriched DOM, follows an area of mixing between different groundwaters and infiltration-recharge that is favored by uphill sinkholes at the upper midslope. The medium 14C-depleted wells H42, H43, and H52 share low-permeable rocks in laying and hanging strata (Lazar et al., 2019) and are therefore most likely cut off from direct surface-derived inputs, resulting in the moderate Δ14C values of DOM in the water from these wells. While H53 had similar characteristics to H52 regarding the Δ14C of inorganic carbon (Nowak et al., 2017) and major ion composition (Kohlhepp et al., 2017), DOM showed strong 14C depletion of in H53 relative to H52. The strong 14C depletion of DOM in H53 supported the indicated ecosystem compartmentalization by Lehmann and Totsche (2019), who described those zones as hydrogeologically isolated within the shallow groundwater flow system. Differences between the two sites were previously observed in their fungal (Nawaz et al., 2016) and archaeal communities (Lazar et al., 2017), suggesting potential links between the 14C-depletion of DOM and the in situ biosphere. Based on the Δ14C values of microbial phospholipid fatty acids in selected groundwaters from this transect, Schwab et al. (2019) showed that bacteria metabolize different proportions of sedimentary, 14C-dead carbon. Similarly, the large between-well variability of DOM Δ14C values in this study suggested that varying proportions of in situ incorporated and/or microbial transformed organic carbon have mixed with inputs of 14C-enriched surface-derived DOM. To further elucidate the organic matter dynamics suggested by the distribution of Δ14C values, an in-depth analysis of the DOM composition is required.
Spatial Patterns in DOM Composition
A PCA of the molecular composition of DOM summarized 53.3% of the variability by the first two principal components. Additionally, we fitted parameters such as the proportions of major molecular subgroups and the Δ14C values onto the DOM ordination as vectors. Their directions indicated the strongest increasing gradient of the respective parameter. A well-separated clustering was produced along the two axes with the distribution on PC1 strongly reflecting the Δ14C-based groupings and variabilities (Figure 3). The gradient vector of Δ14C was parallel to PC1, which supported the role of 14C depletion as a major determinant of DOM composition (Flerus et al., 2012).
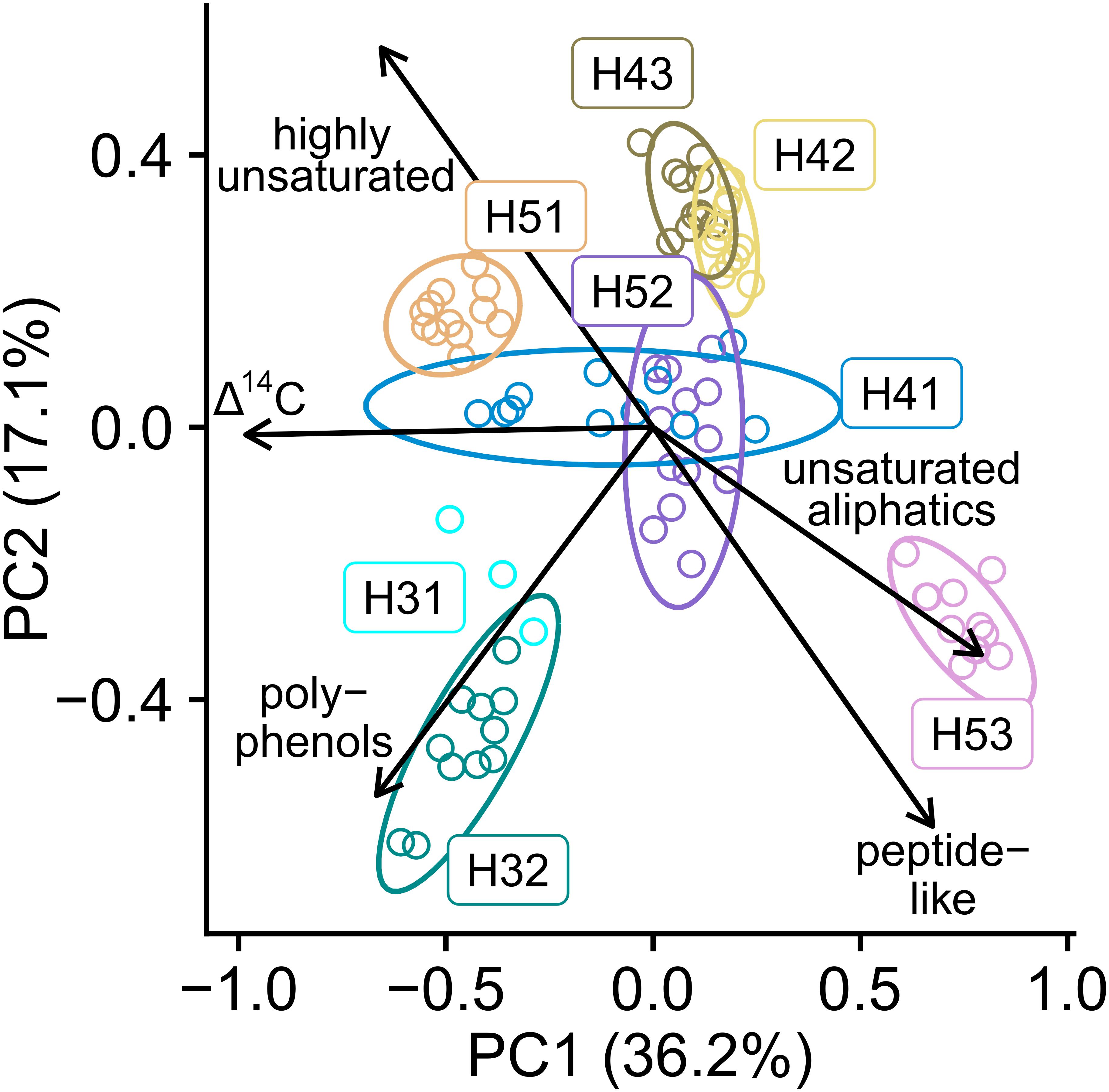
Figure 3. Principal component analysis (PCA) graph based on the molecular composition of SPE-DOM in the Hainich Critical Zone aquifers. Δ14C values and relative proportions of major molecular subgroups were fitted as vectors indicating the direction of the strongest increasing gradients.
The gradient vectors of major molecular subgroups in DOM indicated that DOM was spatially characterized by distinctly differing proportions of these groups. We found that DOM from the wells H31, H32, and H51 contained higher proportions of highly unsaturated and polyphenolic compounds. Together, these molecular subgroups identify labile, surface-derived DOM components, likely derived from the degradation of plant material in the recharge areas. Plant-derived DOM has been previously characterized as more unsaturated and aromatic than microorganism-derived DOM components (Einsiedl et al., 2007; Fellman et al., 2008). The high proportions of polyphenols in H31, H32, and H51 likely identify contributions from the decay of the plant biopolymer lignin (Benk et al., 2018; Jia et al., 2019). In contrast to the contributions of plant-derived DOM to wells H31, H32, and H51, we found high proportions of unsaturated aliphatic and peptide-like DOM components in well H53, indicating microorganism-derived DOM (Kallenbach et al., 2016). Since the peptide-like assigned group of compounds could contain a multitude of different N-containing molecules, we aimed at exploring this compound group in more detail. We compiled structure suggestions from the ChEBI database for the sum formulas that contained three or more nitrogen atoms and whose relative intensities rose with increasing 14C age (Supplementary Table S2). For half of the 26 nitrogen-rich sum formulae oligopeptide structures were suggested by the database, confirming the assignment of the peptide-like group. The results of the chemical structure assignment via the ChEBI database supported our indication of high proportions of microorganism-derived DOM in H53. Our findings from the spatial patterns in DOM composition support our initial hypothesis that surface-connection of the aquifers would be a strong driver for DOM composition. We found high proportions of surface-derived DOM in the wells with high surface connectivity (H31, H31, H51). Isolation from surface-derived inputs (H53) resulted in higher proportions of microorganism-derived DOM. The wells with medium surface-connectivity were not clearly characterized in the ordination by PCA. Therefore, a mixing of 14C enriched plant material with 14C depleted microbial carbon is likely valid over the whole Hainich CZ groundwater transect.
Relationship of DOM Composition and Age
The spatial patterns observed in the wells indicated that DOM composition and age were highly interrelated. To generalize these indications to the whole 3 years of observations from all wells of the transect, we normalized the proportions of each molecular subgroup to their respective maximum and followed their evolution with respect to 14C depletion (Figure 4). We note that as a result of the normalization, the shown trends refer to within-group effects. The non-normalized relative proportions are shown separately in Supplementary Figure S1. The order of relative proportion between the groups was: highly unsaturated molecules >> unsaturated aliphatics > polyphenols > peptide-like compounds. We found that with increasing 14C age, the relative proportions highly unsaturated molecules decreased by ∼10% and those of polyphenols almost halved. In contrast, unsaturated aliphatic and peptide-like substances more than tripled. We observed a shift from the compositional signatures of plant-derived DOM to microorganism-derived DOM. This trend of continuous recycling and microbial transformation of DOM in contrast to the accumulation of recalcitrant molecules (that are chemically resistant to degradation), has been the topic of extensive discussion in the soil community (Marschner et al., 2008; Kaiser and Kalbitz, 2012; Lehmann and Kleber, 2015). Recently, Roth et al. (2019) indicated that during the passage of DOM through soil, small plant-derived molecules were preferentially consumed and transformed into larger microorganism-derived molecules. Our results show that the transition from plant-derived to microorganism-derived DOM is reflected in groundwater as well. Based on this finding and the previously discussed link between aquifer-surface connection and DOM composition and age, we hypothesize that temporally changing proportions of plant-derived and microorganism-derived DOM in groundwater will reflect hydrological system shifts that affected DOM transport.
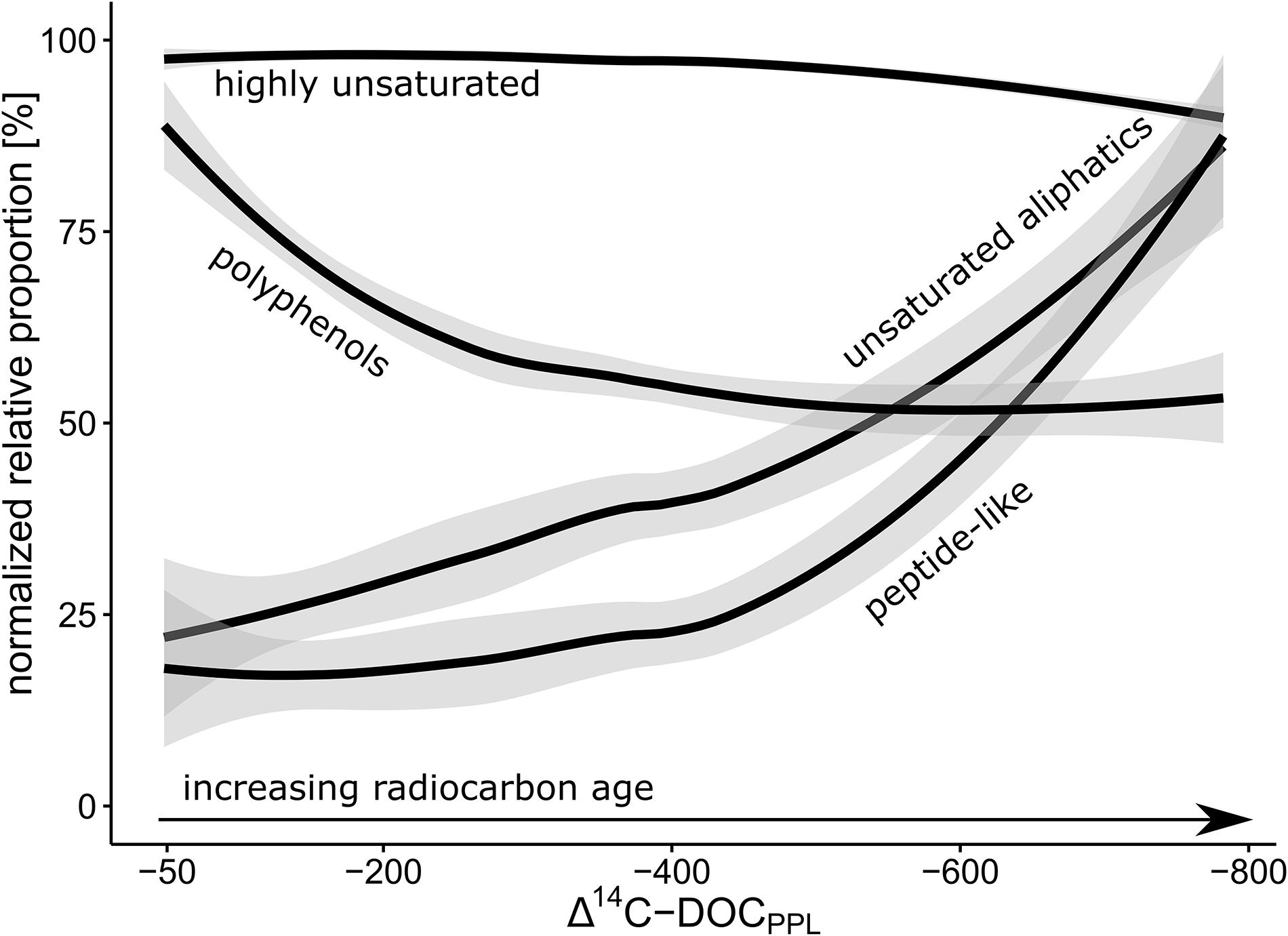
Figure 4. Relative proportions of major molecular subgroups in DOM with respect to 14C depletion, normalized to the respective within-group maxima. Lines result of a locally estimated scatterplot smoothing (LOESS), gray areas indicate 95% confidence intervals.
Variable Input During Groundwater Recharge Events
Investigating the temporal variability of Δ14C in DOM showed that the wells H32, H41, H42, and H43 were characterized by a yearly reoccurring pattern that was potentially linked to their hydraulic head configuration. Their Δ14C values were more enriched during the first quarter of the year and retreated back to more depleted values in the remaining 9 months (Figure 5A). This pattern was most pronounced in well H41. The shift to less depleted Δ14C values coincided with the onset of rising hydraulic heads as well as rising air temperatures and precipitation at the end of the winter period (Figures 5B,C). The groundwater levels (Figure 5B) showed pronounced seasonal fluctuation with maxima in early spring (well H32) or early summer (other wells) and minima occurring in late autumn (2015, 2016) or late winter (2017). The latter is the consequence of high (2014, 2015) or low amounts (2016) of precipitation in the dormancy period and points to a fast-responding shallow groundwater flow system. In the shallow well H32, responses to single recharge events are contained in the hydrograph, whereas responses are dampened and delayed in the wells with larger contribution areas (Kohlhepp et al., 2017). The varying hydraulic heads represented phases of elevated to reduced groundwater recharge, as well as the variation in subsurface flow patterns (Lehmann and Totsche, 2019). More 14C-enriched DOM and correspondingly higher relative intensities of surface-derived molecular species during phases of rising hydraulic heads point to a fast subsurface passage. The configuration of the fractured aquifer system in the Hainich CZE gave rise to an uneven distribution of observed responses to the shifts in hydraulic pressure associated with recharge events. It would be reasonable to expect the strongest responses of the DOM composition and age to recharge-related system shifts in wells close to the recharge sources, such as H31 and H32 at the upper midslope. However, we observed the largest variability in well H41 with regards to intra-annual fluctuations of the hydraulic heads as well as 14C depletion and molecular composition of DOM. Our observations suggest that the exceptionally strong recharge signals in H41 were not solely caused by directly surface-sourced input, but instead potentially reflect a complex temporal flow dynamic in the Hainich CZE fractured aquifer system. Underlying aquifers have been suggested as sources for upward to lateral cross-stratal exchange between sites H4 and H5, as inferred from exceptionally high sulfate concentrations in waters from well H51 that are likely derived from evaporite layers contained in the underlying Middle Muschelkalk strata (Kohlhepp et al., 2017). Recharge-related episodic integration of H4 into upward flow patterns could have caused the large variability in H41. This hypothesis was supported by sulfate concentrations in H41 being highest when DOM was 14C-enriched (Nov 2015: SO42– = 75 mg/L, Δ14C = −383; Feb 2016: SO42– = 117 mg/L, Δ14C = −156). Thus, at the lower midslope, the input of surface-derived DOM to the main aquifer is likely coupled to ascending groundwater flow of meteoric origin. A meteoric origin was likely, considering that stable water isotopes at H41 did not show strong changes during the phases of the observed shifts in organic matter composition and age (Nov 2015: δ18O = −9.3, δ2H = −63.4; Feb 2016: δ18O = −9.0, δ2H = −63.0).
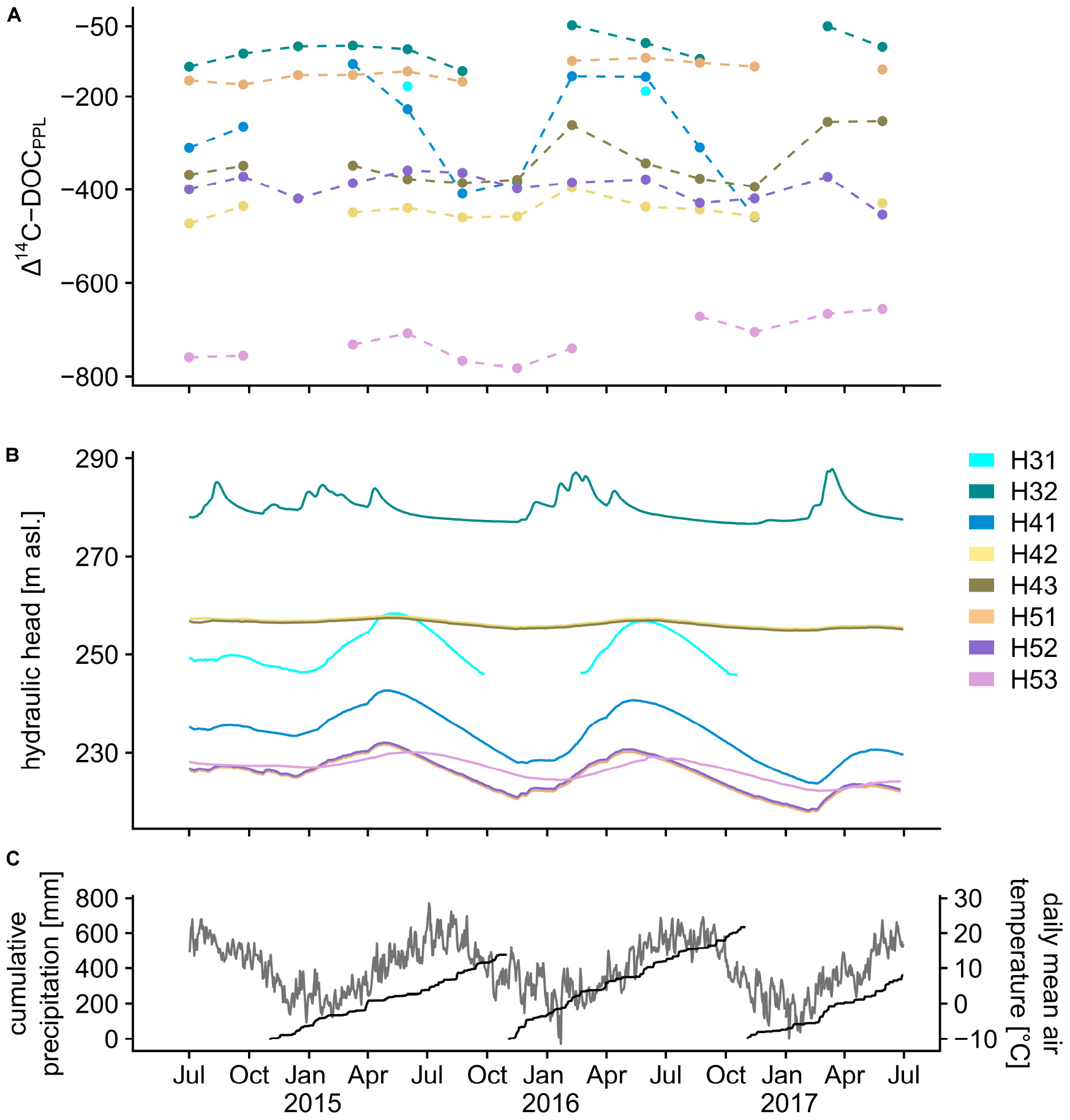
Figure 5. Combined graph highlighting the connection of the 14C depletion of SPE-DOM and the hydraulic pressure layout of the Hainich Critical Zone aquifers over three consecutive years. (A) Δ14C in SPE-DOM. Missing values resulted from either non-productive wells during the time of sampling or measurement failure. (B) Hydraulic head configuration. (C) Cumulative 12-monthly precipitation and daily mean air temperature recorded by the meteorological station in Weberstedt (MeteoGroup Deutschland GmbH, Germany; 270 m asl).
Responses of the in situ Biosphere
As microorganisms are key mediators for the transformation of DOM (Carlson et al., 2004; Young et al., 2004; Jiao et al., 2010; D’Andrilli et al., 2019), we hypothesized that the variable DOM input following groundwater recharge potentially affected the bacterial communities in the aquifers. Here, we focused on the temporal variation of DOM composition and age in well H41, which showed the strongest responses to groundwater recharge, disregarding already reported microbiological differences between the transect sites (Opitz et al., 2014). In addition to composition and age, also the Shannon alpha diversity of DOM in well H41 followed a yearly cycle corresponding to groundwater recharge (Figure 6). During late recharge phases at the end of the winter season, DOM was more diverse compared to the rest of the year. There was no significant net change of DOM diversity in well H41 over the three observed years. In contrast, the Shannon alpha diversity of the bacterial community in well H41 showed an increase of diversity over time, overlain by yearly cycles corresponding to the DOM dynamics. Our data suggested that in our fractured-rock ecosystem the alpha diversities of DOM and bacteria were linked. A coupling between the diversities of DOM and bacteria during groundwater recharge could occur as a result of differing processes: (1) combined transport of surface-derived DOM and diverse, allochthonous bacterial communities into the aquifer (Pronk et al., 2006, 2009; Herrmann et al., 2019). (2) Stimulation of the autochthonous bacterial community in response to influx of diverse DOM substrate from the surface (Zhang et al., 2015; Rajala and Bomberg, 2017; Wu et al., 2018). The two potential drivers are not mutually exclusive and could have a combined effect. Previous studies in comparable carbonate-rock environments, though karst aquifers, reported stable autochthonous bacterial communities (Farnleitner et al., 2005), that were overprinted during recharge events by allochthonous communities together with a peak of inflowing DOM from the surface (Pronk et al., 2006, 2009). Combined transport of DOM and allochthonous bacteria are likely common phenomena, with potential stimulation effects of autochthonous bacteria fading during the recession phase. In our study, the molecular diversity of DOM returned to the pre-event state after the recharge periods had ended, however, the bacterial community diversity did not. A PCoA graph of the bacterial community composition in well H41 further revealed that, similar to the diversity dynamics, also the bacterial community composition did not return to the original state in the recession phases (Figure 7). Instead, the ordination suggested responses to recharge that entailed community evolution. The overprinting return, which happened for DOM during every observed recession phase, was lacking for bacterial communities in H41. This suggested that the autochthonous bacterial community was stimulated by diverse, surface-derived DOM during recharge. During groundwater recharge, the input of relatively more diverse DOM potentially represented a wide range of food types, allowing a diverse bacterial community to flourish, as has been reported from culture experiments (Farjalla et al., 2009; Landa et al., 2013). Mixtures of allochthonous and autochthonous carbon, simultaneously provided to freshwater bacterial communities, can cause community shifts (Attermeyer et al., 2014). In the fractured and stratified subsurface of the Hainich CZE, mixing of subsurface waters is likely during intensive recharge events in the winter season. In contrast to pronounced karst aquifers, showing more distinct event-scale responses, our retarded flow system within limestone-mudstone alternations covers low-frequency responses, leading to the observed trends in molecular and bacterial diversity and community composition. The observed phenomena of the main aquifer well H41 are the most pronounced along the well transect, however, they highlight the rarely studied dynamics within shallow groundwater resources. In this study we cannot finally disentangle the individual effects of transport from shallow or soil sources and stimulation by mobile DOM. However, our data indicated that surface-derived DOM is a contributor to bacterial community evolution.
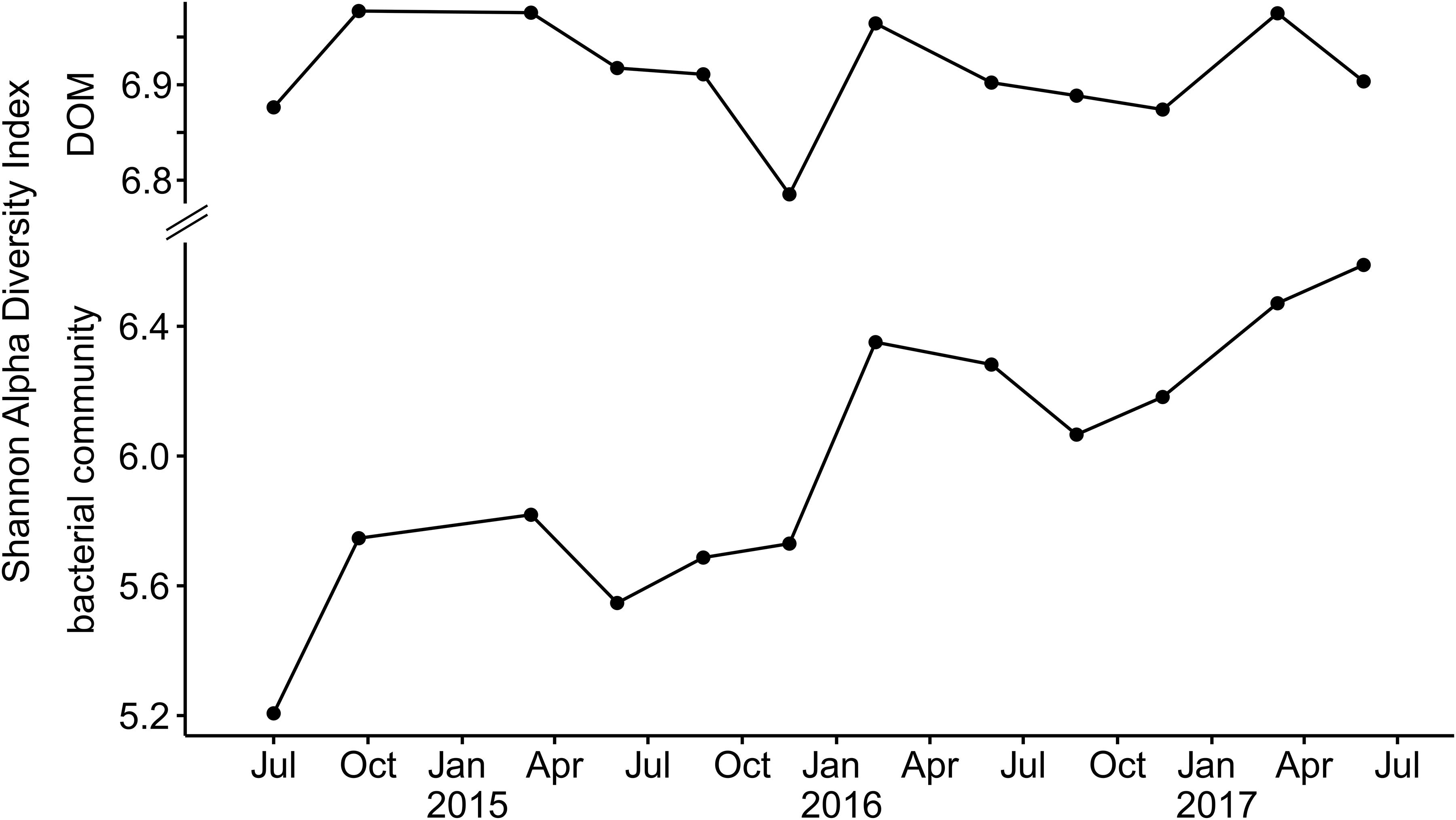
Figure 6. Shannon alpha diversity values of DOM and the bacterial community in well H41 in response to groundwater recharge.
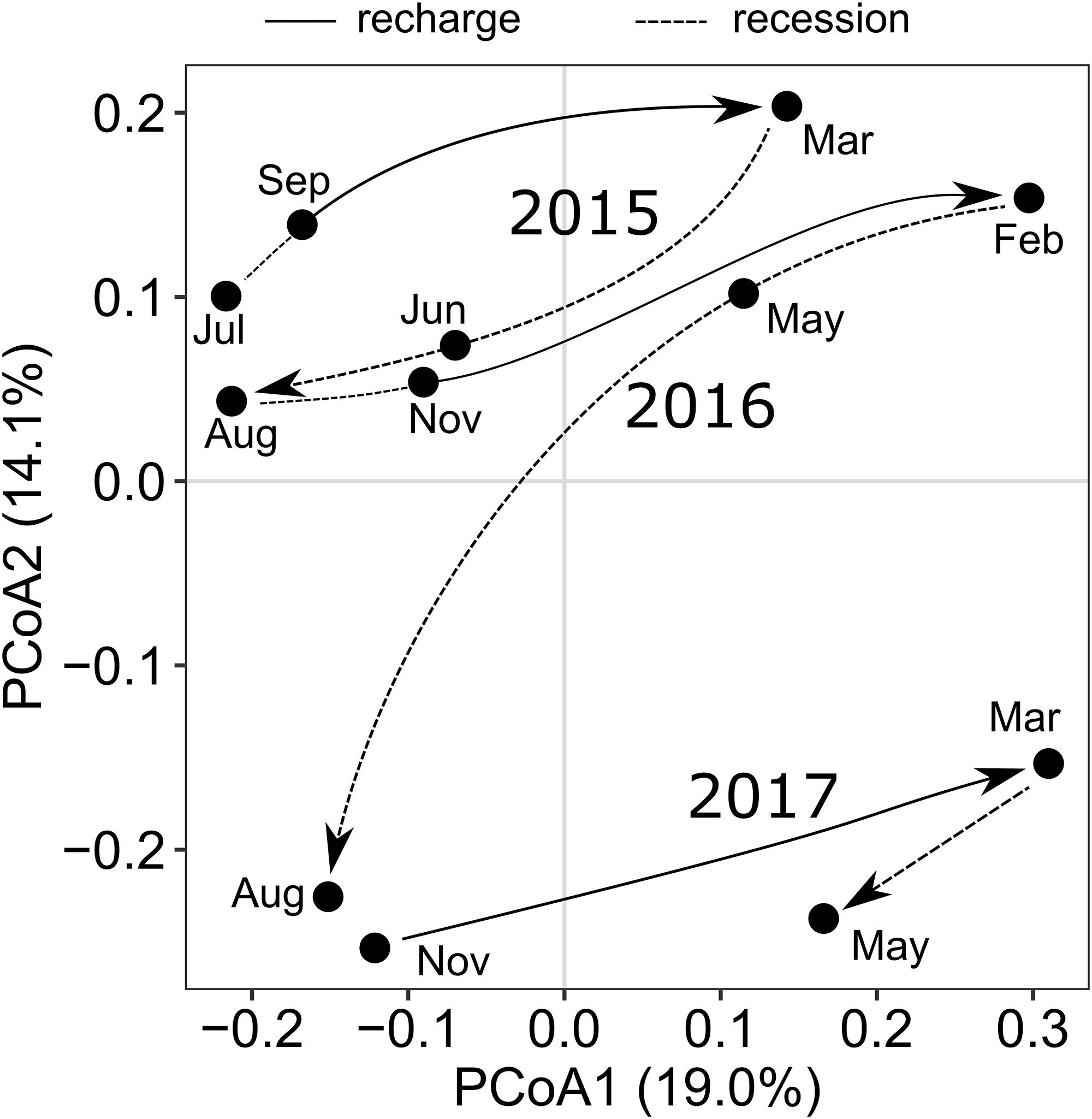
Figure 7. Principal coordinates analysis (PCoA) graph based on Bray-Curtis dissimilarity, summarizing the bacterial community composition in well H41 over 3 years of recharge and recession cycles.
Conclusion
Our study showed that the analysis of composition and age of groundwater DOM, in combination with hydrochemical and environmental data, creates a better understanding of links between surface and subsurface ecosystems and of drivers of microbial community dynamics in groundwater ecosystems. Mostly overlooked dynamics of DOM diversity are likely an important factor for the groundwater bacterial community structure. We suggest that future studies take into account the metabolic pathways that are relevant for the biotransformation of DOM in groundwater. Extracting metabolic pathway information from DOM will lead to an increased specificity in targeting the coupling between DOM and microbial communities, which will help in separating autochthonous stimulation from transport effects (Anantharaman et al., 2016; Bomberg et al., 2016). Advances in assessing these links between DOM and microbial communities in the CZ will aid future management of ecosystem services such as crop production and water quality as human demands on the environment are constantly increasing (Brantley et al., 2007; Frimmel and Abbt-Braun, 2009; Richter and Mobley, 2009).
Data Availability Statement
The datasets generated for this study can be found in the European Nucleotide Archive PRJEB33923.
Author Contributions
V-NR and GG conceived the study idea. SB managed the DOM instrumental analysis and data analysis, and drafted the manuscript. RL and KT provided the physicochemical data. LY and KK provided the bacterial community data. SB, VS, and GG interpreted the data. All authors contributed to reviewing and improving the manuscript.
Funding
This study was part of the Collaborative Research Centre AquaDiva (CRC 1076 AquaDiva) of the Friedrich Schiller University Jena, funded by the Deutsche Forschungsgemeinschaft.
Conflict of Interest
The authors declare that the research was conducted in the absence of any commercial or financial relationships that could be construed as a potential conflict of interest.
Acknowledgments
We thank the radiocarbon group of A. Steinhof, the routine measurement and analysis group of I. Hilke, and the stable isotope analysis group of H. Moossen for measurement contributions and fruitful discussions. We further acknowledge M. Förster for her work in preparing and extracting the DOM samples. We thank the AquaDiva scientific coordinators and on-site crew for conducting the regular sampling campaigns. Field work permits were issued by the responsible state environmental offices of Thüringen. SB acknowledges the International Max Planck Research School for Global Biogeochemical Cycles (IMPRS-gBGC) for funding.
Supplementary Material
The Supplementary Material for this article can be found online at: https://www.frontiersin.org/articles/10.3389/feart.2019.00296/full#supplementary-material
References
Anantharaman, K., Brown, C. T., Hug, L. A., Sharon, I., Castelle, C. J., Probst, A. J., et al. (2016). Thousands of microbial genomes shed light on interconnected biogeochemical processes in an aquifer system. Nat. Commun. 7:13219. doi: 10.1038/ncomms13219
Aravena, R., and Wassenaar, L. I. (1993). Dissolved organic carbon and methane in a regional confined aquifer, southern Ontario, Canada: carbon isotope evidence for associated subsurface sources. Appl. Geochem. 8, 483–493. doi: 10.1016/0883-2927(93)90077-T
Artinger, R., Buckau, G., Geyer, S., Fritz, P., Wolf, M., and Kim, J. I. (2000). Characterization of groundwater humic substances: influence of sedimentary organic carbon. Appl. Geochem. 15, 97–116. doi: 10.1016/S0883-2927(99)00021-9
Attermeyer, K., Hornick, T., Kayler, Z. E., Bahr, A., Zwirnmann, E., Grossart, H.-P., et al. (2014). Enhanced bacterial decomposition with increasing addition of autochthonous to allochthonous carbon without any effect on bacterial community composition. Biogeosciences 11, 1479–1489. doi: 10.5194/bg-11-1479-2014
Baker, M. A., Valett, H. M., and Dahm, C. N. (2000). Organic carbon supply and metabolism in a shallow groundwater ecosystem. Ecology 81, 3133–3148.
Ben Maamar, S., Aquilina, L., Quaiser, A., Pauwels, H., Michon-Coudouel, S., Vergnaud-Ayraud, V., et al. (2015). Groundwater isolation governs chemistry and microbial community structure along hydrologic flowpaths. Front. Microbiol. 6:1457. doi: 10.3389/fmicb.2015.01457
Benk, S. A., Li, Y., Roth, V.-N., and Gleixner, G. (2018). Lignin dimers as potential markers for 14C-young terrestrial dissolved organic matter in the critical zone. Front. Earth Sci. 6:168. doi: 10.3389/feart.2018.00168
Bomberg, M., Lamminmäki, T., and Itävaara, M. (2016). Microbial communities and their predicted metabolic characteristics in deepfracture groundwaters of the crystalline bedrock at Olkiluoto, Finland. Biogeosciences 13, 6031–6047. doi: 10.5194/bg-13-6031-2016
Brantley, S. L., Goldhaber, M. B., and Ragnarsdottir, K. V. (2007). Crossing Disciplines and Scales to Understand the Critical Zone. Elements 3, 307–314. doi: 10.2113/gselements.3.5.307
Carlson, C. A., Giovannoni, S. J., Hansell, D. A., Goldberg, S. J., Parsons, R., and Vergin, K. (2004). Interactions among dissolved organic carbon, microbial processes, and community structure in the mesopelagic zone of the northwestern Sargasso Sea. Limnol. Oceanogr. 49, 1073–1083. doi: 10.4319/lo.2004.49.4.1073
Cooper, K. J., Whitaker, F. F., Anesio, A. M., Naish, M., Reynolds, D. M., and Evans, E. L. (2016). Dissolved organic carbon transformations and microbial community response to variations in recharge waters in a shallow carbonate aquifer. Biogeochemistry 129, 215–234. doi: 10.1007/s10533-016-0226-4
D’Andrilli, J., Junker, J. R., Smith, H. J., Scholl, E. A., and Foreman, C. M. (2019). DOM composition alters ecosystem function during microbial processing of isolated sources. Biogeochemistry 142, 281–298. doi: 10.1007/s10533-018-00534-5
Ding, S., Kohlhepp, B., Trumbore, S., Küsel, K., Totsche, K.-U., Pohnert, G., et al. (2018). In situ production of core and intact bacterial and archaeal tetraether lipids in groundwater. Organ. Geochem. 126, 1–12. doi: 10.1016/j.orggeochem.2018.10.005
Dittmar, T., Koch, B., Hertkorn, N., and Kattner, G. (2008). A simple and efficient method for the solid-phase extraction of dissolved organic matter (SPE-DOM) from seawater. Limnol. Oceanogr. 6, 230–235. doi: 10.4319/lom.2008.6.230
Edgar, R. C. (2010). Search and clustering orders of magnitude faster than BLAST. Bioinformatics 26, 2460–2461. doi: 10.1093/bioinformatics/btq461
Einsiedl, F., Hertkorn, N., Wolf, M., Frommberger, M., Schmitt-Kopplin, P., and Koch, B. P. (2007). Rapid biotic molecular transformation of fulvic acids in a karst aquifer. Geochim. Cosmochim. Acta 71, 5474–5482. doi: 10.1016/j.gca.2007.09.024
Farjalla, V. F., Marinho, C. C., Faria, B. M., Amado, A. M., Esteves, F., de, A., et al. (2009). Synergy of fresh and accumulated organic matter to bacterial growth. Microb. Ecol. 57, 657–666. doi: 10.1007/s00248-008-9466-8
Farnleitner, A. H., Wilhartitz, I., Ryzinska, G., Kirschner, A. K. T., Stadler, H., Burtscher, M. M., et al. (2005). Bacterial dynamics in spring water of alpine karst aquifers indicates the presence of stable autochthonous microbial endokarst communities. Environ. Microbiol. 7, 1248–1259. doi: 10.1111/j.1462-2920.2005.00810.x
Fasching, C., Behounek, B., Singer, G. A., and Battin, T. J. (2014). Microbial degradation of terrigenous dissolved organic matter and potential consequences for carbon cycling in brown-water streams. Sci. Rep. 4:4981. doi: 10.1038/srep04981
Fellman, J. B., D’Amore, D. V., Hood, E., and Boone, R. D. (2008). Fluorescence characteristics and biodegradability of dissolved organic matter in forest and wetland soils from coastal temperate watersheds in southeast Alaska. Biogeochemistry 88, 169–184. doi: 10.1007/s10533-008-9203-x
Flerus, R., Lechtenfeld, O. J., Koch, B. P., McCallister, S. L., Schmitt-Kopplin, P., Benner, R., et al. (2012). A molecular perspective on the ageing of marine dissolved organic matter. Biogeosciences 9, 1935–1955. doi: 10.5194/bg-9-1935-2012
Frimmel, F. H., and Abbt-Braun, G. (2009). “Dissolved Organic Matter (DOM) in Natural Environments,” in Biophysico-Chemical Processes Involving Natural Nonliving Organic Matter in Environmental Systems, eds N. Senesi, B. Xing, and P. M. Huang, (Hoboken, NJ: John Wiley & Sons, Inc.), 367–406. doi: 10.1002/9780470494950.ch10
Gehre, M., Geilmann, H., Richter, J., Werner, R. A., and Brand, W. A. (2004). Continuous flow 2H/1H and 18O/16O analysis of water samples with dual inlet precision. Rapid Commun. Mass Spectr. 18, 2650–2660. doi: 10.1002/rcm.1672
Grøn, C., Tørsløv, J., Albrechtsen, H.-J., and Jensen, H. M. (1992). Biodegradability of dissolved organic carbon in groundwater from an unconfined aquifer. Sci. Total Environ. 11, 241–251. doi: 10.1016/0048-9697(92)90091-6
Gross, C. D., and Harrison, R. B. (2019). The case for digging deeper: soil organic carbon storage, dynamics, and controls in our changing world. Soil Syst. 3:28. doi: 10.3390/soilsystems3020028
He, Y., Caporaso, J. G., Jiang, X.-T., Sheng, H.-F., Huse, S. M., Rideout, J. R., et al. (2015). Stability of operational taxonomic units: an important but neglected property for analyzing microbial diversity. Microbiome 3:20. doi: 10.1186/s40168-015-0081-x
Herlemann, D. P., Labrenz, M., Jurgens, K., Bertilsson, S., Waniek, J. J., and Andersson, A. F. (2011). Transitions in bacterial communities along the 2000 km salinity gradient of the Baltic Sea. ISME J. 5, 1571–1579. doi: 10.1038/ismej.2011.41
Herrmann, M., Wegner, C.-E., Taubert, M., Geesink, P., Lehmann, K., Yan, L., et al. (2019). Predominance of Cand. Patescibacteria in groundwater is caused by their preferential mobilization from soils and flourishing under oligotrophic conditions. Front. Microbiol. 10:1407. doi: 10.3389/fmicb.2019.01407
Hertkorn, N., Benner, R., Frommberger, M., Schmitt-Kopplin, P., Witt, M., Kaiser, K., et al. (2006). Characterization of a major refractory component of marine dissolved organic matter. Geochim. Cosmochim. Acta 70, 2990–3010. doi: 10.1016/j.gca.2006.03.021
Hertkorn, N., Frommberger, M., Witt, M., Koch, B. P., Schmitt-Kopplin, P., and Perdue, E. M. (2008). Natural organic matter and the event horizon of mass spectrometry. Anal. Chem. 80, 8908–8919. doi: 10.1021/ac800464g
Hertkorn, N., Harir, M., Koch, B. P., Michalke, B., and Schmitt-Kopplin, P. (2013). High-field NMR spectroscopy and FTICR mass spectrometry: powerful discovery tools for the molecular level characterization of marine dissolved organic matter. Biogeosciences 10, 1583–1624. doi: 10.5194/bg-10-1583-2013
Herzsprung, P., von Tumpling, W., Hertkorn, N., Harir, M., Buttner, O., Bravidor, J., et al. (2012). Variations of DOM quality in inflows of a drinking water reservoir: linking of van Krevelen diagrams with EEMF spectra by rank correlation. Environ. Sci. Technol. 46, 5511–5518. doi: 10.1021/es300345c
Hubalek, V., Wu, X., Eiler, A., Buck, M., Heim, C., Dopson, M., et al. (2016). Connectivity to the surface determines diversity patterns in subsurface aquifers of the Fennoscandian shield. ISME J. 10, 2447–2458. doi: 10.1038/ismej.2016.36
Jaffé, R., Yamashita, Y., Maie, N., Cooper, W. T., Dittmar, T., Dodds, W. K., et al. (2012). Dissolved organic matter in headwater streams: compositional variability across climatic regions of North America. Geochim. Cosmochim. Acta 94, 95–108. doi: 10.1016/j.gca.2012.06.031
Jia, J., Feng, X., Graf Pannatier, E., Wacker, L., McIntyre, C., van der Voort, T., et al. (2019). 14C characteristics of dissolved lignin along a forest soil profile. Soil Biol. Biochem. 135, 407–410. doi: 10.1016/j.soilbio.2019.06.005
Jiao, N., Herndl, G. J., Hansell, D. A., Benner, R., Kattner, G., Wilhelm, S. W., et al. (2010). Microbial production of recalcitrant dissolved organic matter: long-term carbon storage in the global ocean. Nat. Rev. Microbiol. 8, 593–599. doi: 10.1038/nrmicro2386
Jin, J., Zimmerman, A. R., Moore, P. J., and Martin, J. B. (2014). Organic and inorganic carbon dynamics in a karst aquifer: Santa Fe River Sink-Rise system, north Florida, USA: Carbon dynamics in a karst aquifer. J. Geophys. Res. Biogeosci. 119, 340–357. doi: 10.1002/2013JG002350
Kaiser, K., and Guggenberger, G. (2000). The role of DOM sorption to mineral surfaces in the preservation of organic matter in soils. Organ. Geochem. 31, 711–725. doi: 10.1016/S0146-6380(00)00046-2
Kaiser, K., and Guggenberger, G. (2005). Storm flow flushing in a structured soil changes the composition of dissolved organic matter leached into the subsoil. Geoderma 127, 177–187. doi: 10.1016/j.geoderma.2004.12.009
Kaiser, K., and Kalbitz, K. (2012). Cycling downwards – dissolved organic matter in soils. Soil Biol. Biochem. 52, 29–32. doi: 10.1016/j.soilbio.2012.04.002
Kallenbach, C. M., Frey, S. D., and Grandy, A. S. (2016). Direct evidence for microbial-derived soil organic matter formation and its ecophysiological controls. Nat. Commun. 7:13630. doi: 10.1038/ncomms13630
Katz, B. G., Catches, J. S., Bullen, T. D., and Michel, R. L. (1998). Changes in the isotopic and chemical composition of ground water resulting from a recharge pulse from a sinking stream. J. Hydrol. 211, 178–207. doi: 10.1016/S0022-1694(98)00236-4
Kellerman, A. M., Kothawala, D. N., Dittmar, T., and Tranvik, L. J. (2015). Persistence of dissolved organic matter in lakes related to its molecular characteristics. Nat. Geosci. 8, 454–457. doi: 10.1038/ngeo2440
Kleber, M., Eusterhues, K., Keiluweit, M., Mikutta, C., Mikutta, R., and Nico, P. S. (2015). Mineral–organic associations: formation, properties, and relevance in soil environments. Adv. Agron. 130, 1–140. doi: 10.1016/bs.agron.2014.10.005
Koch, B. P., and Dittmar, T. (2006). From mass to structure: an aromaticity index for high-resolution mass data of natural organic matter. Rapid Commun. Mass Spectr. 20, 926–932. doi: 10.1002/rcm.2386
Koch, B. P., and Dittmar, T. (2016). From mass to structure: an aromaticity index for high-resolution mass data of natural organic matter. Rapid Commun. Mass Spectr. 30, 250–250. doi: 10.1002/rcm.7433
Kohlhepp, B., Lehmann, R., Seeber, P., Küsel, K., Trumbore, S. E., and Totsche, K. U. (2016). Pedological and hydrogeological setting and subsurface flow structure of the carbonate-rock CZE Hainich in western Thuringia, Germany. Hydrol. Earth Syst. Sci. Discuss. 3, 1–32. doi: 10.5194/hess-2016-2374
Kohlhepp, B., Lehmann, R., Seeber, P., Küsel, K., Trumbore, S. E., and Totsche, K. U. (2017). Aquifer configuration and geostructural links control the groundwater quality in thin-bedded carbonate–siliciclastic alternations of the Hainich CZE, central Germany. Hydrol. Earth Syst. Sci. 21, 6091–6116. doi: 10.5194/hess-21-6091-2017
Kolehmainen, R. E., Langwaldt, J. H., and Puhakka, J. A. (2007). Natural organic matter (NOM) removal and structural changes in the bacterial community during artificial groundwater recharge with humic lake water. Water Res. 41, 2715–2725. doi: 10.1016/j.watres.2007.02.042
Kothawala, D. N., Ji, X., Laudon, H., Agren, A. M., Futter, M. N., Köhler, S. J., et al. (2015). The relative influence of land cover, hydrology, and in-stream processing on the composition of dissolved organic matter in boreal streams. J. Geophys. Res. Biogeosci. 120, 1491–1505. doi: 10.1002/2015JG002946
Kozich, J. J., Westcott, S. L., Baxter, N. T., Highlander, S. K., and Schloss, P. D. (2013). Development of a dual-index sequencing strategy and curation pipeline for analyzing amplicon sequence data on the miseq illumina sequencing platform. Appl. Environ. Microbiol. 79, 5112–5120. doi: 10.1128/AEM.01043-1013
Kujawinski, E. B., Longnecker, K., Barott, K. L., Weber, R. J. M., and Kido Soule, M. C. (2016). Microbial community structure affects marine dissolved organic matter composition. Front. Mar. Sci. 3:45. doi: 10.3389/fmars.2016.00045
Kujawinski, E. B., Longnecker, K., Blough, N. V., Vecchio, R. D., Finlay, L., Kitner, J. B., et al. (2009). Identification of possible source markers in marine dissolved organic matter using ultrahigh resolution mass spectrometry. Geochim. Cosmochim. Acta 73, 4384–4399. doi: 10.1016/j.gca.2009.04.033
Kumar, S., Herrmann, M., Blohm, A., Hilke, I., Frosch, T., Trumbore, S. E., et al. (2018). Thiosulfate- and hydrogen-driven autotrophic denitrification by a microbial consortium enriched from groundwater of an oligotrophic limestone aquifer. FEMS Microbiol. Ecol. 94:fiy141. doi: 10.1093/femsec/fiy141
Küsel, K., Totsche, K. U., Trumbore, S. E., Lehmann, R., Steinhäuser, C., and Herrmann, M. (2016). How deep can surface signals be traced in the critical zone? Merging biodiversity with biogeochemistry research in a central german muschelkalk landscape. Front. Earth Sci. 4:32. doi: 10.3389/feart.2016.00032
Landa, M., Cottrell, M., Kirchman, D., Blain, S., and Obernosterer, I. (2013). Changes in bacterial diversity in response to dissolved organic matter supply in a continuous culture experiment. Aquat. Microb. Ecol. 69, 157–168. doi: 10.3354/ame01632
Lazar, C. S., Lehmann, R., Stoll, W., Rosenberger, J., Totsche, K. U., and Küsel, K. (2019). The endolithic bacterial diversity of shallow bedrock ecosystems. Sci. Total Environ. 679, 35–44. doi: 10.1016/j.scitotenv.2019.04.281
Lazar, C. S., Stoll, W., Lehmann, R., Herrmann, M., Schwab, V. F., Akob, D. M., et al. (2017). Archaeal diversity and CO2 fixers in Carbonate-/Siliciclastic-rock groundwater ecosystems. Archaea 2017:2136287. doi: 10.1155/2017/2136287
Lehmann, J., and Kleber, M. (2015). The contentious nature of soil organic matter. Nature 528, 60–68. doi: 10.1038/nature16069
Lehmann, R., and Totsche, K. U. (2019). Multi-directional flow dynamics shape groundwater quality in sloping bedrock strata. J. Hydrol. (in press). doi: 10.1016/j.jhydrol.2019.124291
Leinemann, T., Preusser, S., Mikutta, R., Kalbitz, K., Cerli, C., Höschen, C., et al. (2018). Multiple exchange processes on mineral surfaces control the transport of dissolved organic matter through soil profiles. Soil Biol. Biochem. 118, 79–90. doi: 10.1016/j.soilbio.2017.12.006
Li, D., Alidina, M., Ouf, M., Sharp, J. O., Saikaly, P., and Drewes, J. E. (2013). Microbial community evolution during simulated managed aquifer recharge in response to different biodegradable dissolved organic carbon (BDOC) concentrations. Water Res. 47, 2421–2430. doi: 10.1016/j.watres.2013.02.012
Li, D., Sharp, J. O., Saikaly, P. E., Ali, S., Alidina, M., Alarawi, M. S., et al. (2012). Dissolved organic carbon influences microbial community composition and diversity in managed aquifer recharge systems. Appl. Environ. Microbiol. 78, 6819–6828. doi: 10.1128/AEM.01223-1212
Makarov, A., and Scigelova, M. (2004). “Orbitrap mass analyzer,” in Encyclopedia of Genetics, Genomics, Proteomics and Bioinformatics, eds L. B. Jorde, P. F. Little, M. J. Dunn, and S. Subramaniam, (Hoboken, NJ: John Wiley and Sons), doi: 10.1002/047001153X.g301222
Marschner, B., Brodowski, S., Dreves, A., Gleixner, G., Gude, A., Grootes, P. M., et al. (2008). How relevant is recalcitrance for the stabilization of organic matter in soils? J. Plant Nutr. Soil Sci. 171, 91–110. doi: 10.1002/jpln.200700049
McMahon, P. B., Plummer, L. N., Böhlke, J. K., Shapiro, S. D., and Hinkle, S. R. (2011). A comparison of recharge rates in aquifers of the United States based on groundwater-age data. Hydrogeol. J. 19, 779–800. doi: 10.1007/s10040-011-0722-5
Nawaz, A., Purahong, W., Lehmann, R., Herrmann, M., Küsel, K., Totsche, K. U., et al. (2016). Superimposed pristine limestone aquifers with marked hydrochemical differences exhibit distinct fungal communities. Front. Microbiol. 7:666. doi: 10.3389/fmicb.2016.00666
Nowak, M. E., Schwab, V. F., Lazar, C. S., Behrendt, T., Kohlhepp, B., Totsche, K. U., et al. (2017). Carbon isotopes of dissolved inorganic carbon reflect utilization of different carbon sources by microbial communities in two limestone aquifer assemblages. Hydrol. Earth Syst. Sci. 21, 4283–4300. doi: 10.5194/hess-21-4283-2017
Ohno, T., Parr, T. B., Gruselle, M. C., Fernandez, I. J., Sleighter, R. L., and Hatcher, P. G. (2014). Molecular composition and biodegradability of soil organic matter: a case study comparing two new England forest types. Environ. Sci. Technol. 48, 7229–7236. doi: 10.1021/es405570c
Oksanen, J., Blanchet, F. G., Friendly, M., Kindt, R., Legendre, P., McGlinn, D., et al. (2018). vegan: Community Ecology Package version 2.5-2.
Opitz, S., Küsel, K., Spott, O., Totsche, K. U., and Herrmann, M. (2014). Oxygen availability and distance to surface environments determine community composition and abundance of ammonia-oxidizing prokaroytes in two superimposed pristine limestone aquifers in the Hainich region, Germany. FEMS Microbiol. Ecol. 90, 39–53. doi: 10.1111/1574-6941.12370
Pronk, M., Goldscheider, N., and Zopfi, J. (2006). Dynamics and interaction of organic carbon, turbidity and bacteria in a karst aquifer system. Hydrogeol. J. 14, 473–484. doi: 10.1007/s10040-005-0454-5
Pronk, M., Goldscheider, N., and Zopfi, J. (2009). Microbial communities in karst groundwater and their potential use for biomonitoring. Hydrogeol. J. 17, 37–48. doi: 10.1007/s10040-008-0350-x
Purdy, C. B., Burr, G. S., Rubin, M., Helz, G. R., and Mignerey, A. C. (1992). Dissolved organic and inorganic 14 C concentrations and ages for coastal plain aquifers in Southern Maryland. Radiocarbon 34, 654–663. doi: 10.1017/S0033822200063943
R Core Team (2019). R: A Language and Environment for Statistical Computing. Vienna: R Foundation for Statistical Computing.
Rajala, P., and Bomberg, M. (2017). Reactivation of deep subsurface microbial community in response to methane or methanol amendment. Front. Microbiol. 08:431. doi: 10.3389/fmicb.2017.00431
Ramette, A. (2007). Multivariate analyses in microbial ecology: multivariate analyses in microbial ecology. FEMS Microbiol. Ecol. 62, 142–160. doi: 10.1111/j.1574-6941.2007.00375.x
Reimer, P. J., Bard, E., Bayliss, A., Beck, J. W., Blackwell, P. G., Ramsey, C. B., et al. (2013). Selection and treatment of data for radiocarbon calibration: an update to the International Calibration (IntCal) criteria. Radiocarbon 55, 1923–1945. doi: 10.2458/azu_js_rc.55.16955
Richter, D. Jr., and Mobley, M. L. (2009). Monitoring earth’s critical zone. Science 326, 1067–1068. doi: 10.1126/science.1179117
Riedel, T., Zark, M., Vähätalo, A. V., Niggemann, J., Spencer, R. G. M., Hernes, P. J., et al. (2016). Molecular signatures of biogeochemical transformations in dissolved organic matter from ten world rivers. Front. Earth Sci. 4:85. doi: 10.3389/feart.2016.00085
Rognes, T., Flouri, T., Nichols, B., Quince, C., and Mahé, F. (2016). VSEARCH: a versatile open source tool for metagenomics. PeerJ 4:e2584. doi: 10.7717/peerj.2584
Roth, V.-N., Dittmar, T., Gaupp, R., and Gleixner, G. (2015). The molecular composition of dissolved organic matter in forest soils as a function of pH and temperature. PLoS One 10:e0119188. doi: 10.1371/journal.pone.0119188
Roth, V.-N., Lange, M., Simon, C., Hertkorn, N., Bucher, S., Goodall, T., et al. (2019). Persistence of dissolved organic matter explained by molecular changes during its passage through soil. Nat. Geosci. 12, 755–761. doi: 10.1038/s41561-019-0417-4
Santl-Temkiv, T., Finster, K., Dittmar, T., Hansen, B. M., Thyrhaug, R., Nielsen, N. W., et al. (2013). Hailstones: a window into the microbial and chemical inventory of a storm cloud. PLoS One 8:e53550. doi: 10.1371/journal.pone.0053550
Schloss, P. D., Westcott, S. L., Ryabin, T., Hall, J. R., Hartmann, M., Hollister, E. B., et al. (2009). Introducing mothur: open-source, platform-independent, community-supported software for describing and comparing microbial communities. Appl. Environ. Microbiol. 75, 7537–7541. doi: 10.1128/AEM.01541-9
Schwab, V. F., Herrmann, M., Roth, V.-N., Gleixner, G., Lehmann, R., Pohnert, G., et al. (2017). Functional diversity of microbial communities in pristine aquifers inferred by PLFA- and sequencing-based approaches. Biogeosciences 14, 2697–2714. doi: 10.5194/bg-14-2697-2017
Schwab, V. F., Nowak, M. E., Elder, C. D., Trumbore, S. E., Xu, X., Gleixner, G., et al. (2019). 14C-free carbon is a major contributor to cellular biomass in geochemically distinct groundwater of shallow sedimentary bedrock aquifers. Water Resour. Res. 55, 2104–2121. doi: 10.1029/2017wr022067
Shen, Y., Chapelle, F. H., Strom, E. W., and Benner, R. (2015). Origins and bioavailability of dissolved organic matter in groundwater. Biogeochemistry 122, 61–78. doi: 10.1007/s10533-014-0029-4
Simon, K. S., Pipan, T., Ohno, T., and Culver, D. C. (2010). Spatial and temporal patterns in abundance and character of dissolved organic matter in two karst aquifers. Fund. App. Lim. 177, 81–92. doi: 10.1127/1863-9135/2010/0177-81
Singer, G. A., Fasching, C., Wilhelm, L., Niggemann, J., Steier, P., Dittmar, T., et al. (2012). Biogeochemically diverse organic matter in Alpine glaciers and its downstream fate. Nat. Geosci. 5, 710–714. doi: 10.1038/ngeo1581
Smith, H. J., Tigges, M., D’Andrilli, J., Parker, A., Bothner, B., and Foreman, C. M. (2018). Dynamic processing of DOM: insight from exometabolomics, fluorescence spectroscopy, and mass spectrometry. Limnol. Oceanogr. Lett. 3, 225–235. doi: 10.1002/lol2.10082
Spencer, R. G. M., Guo, W., Raymond, P. A., Dittmar, T., Hood, E., Fellman, J., et al. (2014). Source and biolability of ancient dissolved organic matter in glacier and lake ecosystems on the Tibetan Plateau. Geochim. Cosmochim. Acta 142, 64–74. doi: 10.1016/j.gca.2014.08.006
Steinhof, A. (2013). Data Analysis at the Jena 14C Laboratory. Radiocarbon 55, 282–293. doi: 10.1017/s0033822200057386
Steinhof, A., Altenburg, M., and Machts, H. (2017). Sample preparation at the jena 14C laboratory. Radiocarbon 59, 815–830. doi: 10.1017/RDC.2017.50
Sukhija, B. S., Reddy, D. V., Nagabhushanam, P., Bhattacharya, S. K., Jani, R. A., and Kumar, D. (2006). Characterisation of recharge processes and groundwater flow mechanisms in weathered-fractured granites of Hyderabad (India) using isotopes. Hydrogeol. J. 14, 663–674. doi: 10.1007/s10040-005-0461-6
Trumbore, S. E., Sierra, C. A., and Hicks Pries, C. E. (2016). “Radiocarbon nomenclature, theory, models, and interpretation: measuring age, determining cycling rates, and tracing source pools,” in Radiocarbon and Climate Change, eds E. A. G. Schuur, E. Druffel, and S. E. Trumbore, (Cham: Springer International Publishing), 45–82. doi: 10.1007/978-3-319-25643-6_3
Van Stan, J. T., and Stubbins, A. (2018). Tree-DOM: dissolved organic matter in throughfall and stemflow: DOM in throughfall and stemflow. Limnol. Oceanogr. 3, 199–214. doi: 10.1002/lol2.10059
Ward, N. D., Bianchi, T. S., Medeiros, P. M., Seidel, M., Richey, J. E., Keil, R. G., et al. (2017). Where carbon goes when water flows: carbon cycling across the aquatic continuum. Front. Mar. Sci. 4:7. doi: 10.3389/fmars.2017.00007
Wassenaar, L., Aravena, R., Hendry, J., and Fritz, P. (1991). Radiocarbon in dissolved organic carbon, a possible groundwater dating method: case studies from western Canada. Water Resour. Res. 27, 1975–1986. doi: 10.1029/91WR00504
Wu, X., Wu, L., Liu, Y., Zhang, P., Li, Q., Zhou, J., et al. (2018). Microbial interactions with dissolved organic matter drive carbon dynamics and community succession. Front. Microbiol. 9:1234. doi: 10.3389/fmicb.2018.01234
Young, K. C., Maurice, P. A., Docherty, K. M., and Bridgham, S. D. (2004). Bacterial degradation of dissolved organic matter from two northern michigan streams. Geomicrobiol. J. 21, 521–528. doi: 10.1080/01490450490888208
Zhang, P., Wu, W. M., Van Nostrand, J. D., Deng, Y., He, Z., Gihring, T., et al. (2015). Dynamic succession of groundwater functional microbial communities in response to emulsified vegetable oil amendment during sustained In Situ U(VI) reduction. Appl. Environ. Microbiol. 81, 4164–4172. doi: 10.1128/AEM.00043-15
Keywords: Orbitrap, radiocarbon, groundwater, DOM, diversity, AquaDiva
Citation: Benk SA, Yan L, Lehmann R, Roth V-N, Schwab VF, Totsche KU, Küsel K and Gleixner G (2019) Fueling Diversity in the Subsurface: Composition and Age of Dissolved Organic Matter in the Critical Zone. Front. Earth Sci. 7:296. doi: 10.3389/feart.2019.00296
Received: 26 June 2019; Accepted: 29 October 2019;
Published: 15 November 2019.
Edited by:
Samuel Abiven, University of Zurich, SwitzerlandReviewed by:
Malin Bomberg, VTT Technical Research Centre of Finland Ltd., FinlandJakob Zopfi, University of Basel, Switzerland
Copyright © 2019 Benk, Yan, Lehmann, Roth, Schwab, Totsche, Küsel and Gleixner. This is an open-access article distributed under the terms of the Creative Commons Attribution License (CC BY). The use, distribution or reproduction in other forums is permitted, provided the original author(s) and the copyright owner(s) are credited and that the original publication in this journal is cited, in accordance with accepted academic practice. No use, distribution or reproduction is permitted which does not comply with these terms.
*Correspondence: Gerd Gleixner, Z2VyZC5nbGVpeG5lckBiZ2MtamVuYS5tcGcuZGU=