- 1Institute of Particle Physics, ETH Zurich, Zurich, Switzerland
- 2Institute of Geological Sciences, University of Bern, Bern, Switzerland
- 3EDYTEM Lab, CNRS, Université Savoie Mont Blanc, Chambéry, France
The Arp Nouva peat bog located in the upper Ferret Valley in the Mont Blanc massif was critically evaluated since published radiocarbon dates have led to controversial conclusions on the formation of this swamp. Radiocarbon dating of woody fragments from three pits of up to 1 m depth was used to discuss the question of whether the historically documented rock avalanche occurring in 1717 CE overran the peat bog or settled prior to its formation. For the deepest samples in the pits, calibrated radiocarbon ages between 1,652 and 1950 CE (95.4%; confidence level) were obtained, which fit very well into the time frame of the historical documented 1717 CE rock avalanche event. It can, therefore, be concluded that the Arp Nouva peat bog was formed by blockage of the Bella Combe torrent by the rock avalanche deposits. Furthermore, careful sample preparation with consequent separation of woody fragments from the bulk peat sample has shown that the problem of too old 14C ages can be circumvented. This work demonstrates that a combined geomorphological and geochronological approach is the most reliable way to reconstruct landscape evolution. The key to successful 14C dating is careful sample selection and the identification of the material that might not be ideal for chronological reconstructions.
Introduction
Not all changes in the landscape caused by mass movements can be reconstructed from historical accounts. Such records help to understand the mechanisms behind the events. Some cases, however, might remain confounded despite historical information. On September 12, 1717 CE, a rock avalanche occurred on the Mont Blanc massif's south flank in the upper part of Val Ferret (Figure 1). As described by Porter and Orombelli (1980), the written records spoke of the Triolet catastrophe, which was described as a collapse of ice and rock onto the Triolet Glacier. The sudden collapse sent masses of rocks mixed with water and ice down the valley, filling it with debris. Pastures and settlements were destroyed, and seven men were killed as well as 120 cows (Porter and Orombelli, 1980, and references therein).
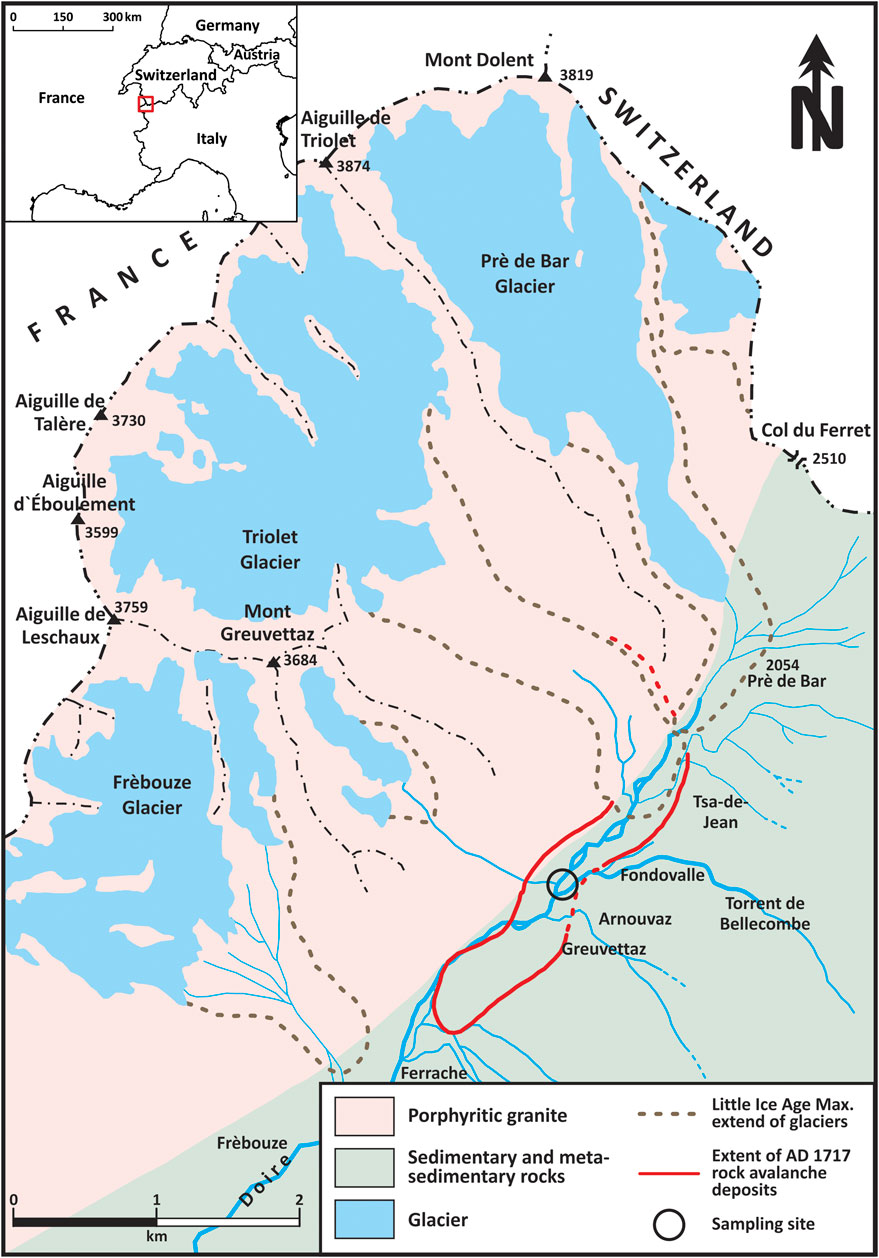
FIGURE 1. Location of the study site, Arp Nova plain in the Ferret Valley, Italy (45.871341157559634, 7.05089817967189). Figure modified after Akçar et al. (2012).
This tragic rock avalanche was recounted for a few decades with the early historical report written by a local inhabitant Michael-Jospeh Pennard. However, over time, the event's memories became obscured, leading to various speculations for the nature of deposits filling the valley (Porter and Orombelli, 1980 and references therein). De Saussure (1786), who visited the valley in 1781, attributed it to the rock avalanche but later in the 19th-century glacial deposits (Agassiz 1845) and a glacial outburst flood (Virgilio 1883) have been proposed. In the 20th century, both glacial and rock avalanche scenarios were active. Sacco (1918) advocated for 16th-19th century glacial drift, Zienert (1965) for late-glacial glaciers activity, and Mayr (1969) argued for mixed sources: rock avalanche and the glacial movement. Porter and Orombelli (1980) revisited the site. They described the boulder deposits as mostly angular and non-sorted, with the largest specimen as high as 20 m (4 × 12 × 20 m) on the Triolet deposit surface about 100 m for the terminus (Photo 3 in Porter and Orombelli 1980). They estimated that the 2 km-long and 500 m-wide valley was filled with debris raising its level by 4–6 m. Their estimated volume of rocks mixed with ice and snow was 6–20 million m3, which collapsed from the elevation of 1860 m and traveled with the velocity of 125–160 km/h, covering a horizontal distance of 7 km. To support their observations, Porter and Orombelli (1980) used chronological tools of dendrochronology and lichenometry. Most notable were the dates of trees, all of which colonized the valley after a few decades. However, the Porter and Orombelli (1980) scenario, which excludes glacial activity as a source of geomorphological changes, has been debated. Aeschlimann (1983) applied radiocarbon dating on peat sampled on the valley floor covered by the Triolet deposit and obtained an age of 885 ± 60 BP (14C years Before Present; Stuiver and Polach 1977). In consequence, he prescribed the older deposits to past glacial activity. In their response, Orombelli and Porter (1988) highlighted the inconsistency of radiocarbon ages on peat. The authors pointed out the possibility of the re-deposition of old peat from nearby locations, which could have happened during the catastrophic mass movement. The potential of hard water effect has been suggested as a possible explanation for the old ages. Hard water effect (depleted 14C content or too old 14C ages) can be expected when plants growing underwater incorporate dissolved CO2 of a mixed 14C signal, typically older than the atmosphere (Deevey and Stuiver, 1964).
Orombelli, Porter, and Aeschliman revisited the site in 1984 and took additional samples from the peat bog near the boulder accumulation of Arp Nuova (location P2 Figure 1; Orombelli and Porter, 1988). The sample was split in two and submitted to two laboratories, Paris and Zurich, which yielded different ages: 105 ± 70 BP (Paris) and 1,020 ± 65 BP (University of Zurich) did not resolve the debate (Orombelli and Porter, 1988). An additional sample taken from the same location in 1986 was analyzed by a 14C laboratory in Rome and resulted in 2,320 ± 150 BP. Such a spread of ages pointed to a problem of 14C dating peat at that location. Therefore the conclusions of Aeschlimann (1983), mostly based on 14C age, were not supported. As Orombelli and Porter (1988) stressed, the importance of accurate interpretation of the geomorphological data cannot be underestimated, considering the geological hazard in the highly popular resort region of Mont Blanc.
In a further attempt to resolve the dispute, Deline and Kirkbride (2009) revisited the site. They proposed a smaller extent of the 1717 CE rock avalanche deposit in the valley with possibly, mixed deposits of an earlier rock avalanche that partly covered older moraines (Deline and Kirkbride, 2009). To describe the deposits' complex geomorphology, they divided the Val Ferret into distal, central, and proximal sectors. The proximal one, Arp Nouva, has also been divided into three subsectors, with granitic boulders dispersed across the valley. A new sampling at the Arp Nuova peat bog developed onto granitic rock avalanche deposit and radiocarbon dating at 14C laboratory in Lyon provided ages ranging from 1,030 ± BP to modern (Table 1 in Deline and Kirkbride (2009). Deline and Kirkbride (2009) suggested that the valley's deposits have a dual origin: Late-glacial moraines and two ca. 1,000 and 1717 CE rock avalanches for which they downscaled by ca. 50% of the volume estimate of rock debris proposed by Porter and Orombelli (1980). The differentiation between the deposition of the boulders is now possible with the cosmogenic nuclide exposure dating. The atoms of 10Be produced and accumulated in the rock exposed to cosmic rays give a measure of exposure time. In their study, Akçar et al. (2012) and Akçar et al. (2014) sampled 19 granitic boulders (1.3–6 m high) located in the upper Ferret Valley and measured the 10Be concentration. 17 of the boulders resulted in apparent ages close to the 18th century. They strengthened the Porter and Orombelli scenario of the 1717 CE rock avalanche deposit covering the whole valley floor, without Lateglacial moraines. The remaining two boulders that delivered apparent ages of ca 10–11 ka were sampled from Lateglacial deposits of the Ferret glacier.
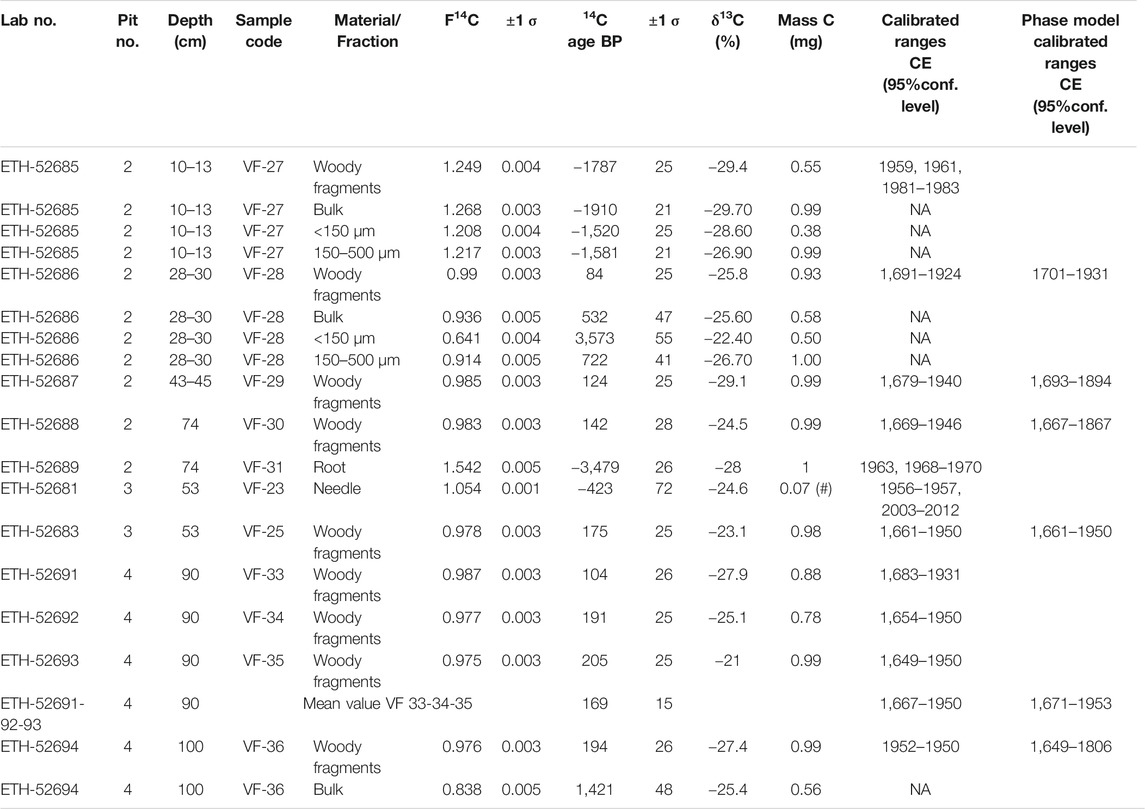
TABLE 1. Results of the AMS analysis obtained on samples selected from organic rich layers and on Total Organic Carbon TOC of various fractions of sediments. F14C is the concentration measured in the sample, corrected for fractionation and normalized to the 1950 value, and the corresponding 14C age. The δ13C values was measured on the graphite. The mass C is the final carbon content of the sample. The first sample contained only 70 µg of C (#) and was analyzed as CO2 using gas ion source (GIS). Calendar ages corresponding to the measured F14C concentration. Calibration and a phase model was performed using the OxCal calibration with INTCAL13 calibration curve (Reimer et al., 2013). The postmodern ages were calibrated using the CALIBomb and with the data for the Northern Hemisphere (Hua et al., 2013; Levin et al., 2013).
Although the analysis of 10Be delivered conclusive evidence for the rock avalanche being the source of the boulders, the discrepancy observed in radiocarbon ages of peat samples from the Arp Nouva peat bog remained unresolved. Therefore, another sampling campaign was planned in 2013 and completed as part of a master thesis. Various fractions of sediment were 14C dated, and wood was considered to be the best choice. Our results illustrate the importance of a clear selection and description of the carbon source used for radiocarbon dating.
Study Site
The Arp Nouva in the upper Ferret Valley is located on the southern, Italian flank of the Mont Blanc massif, on the border with Switzerland and France (Figure 1). The Ferret Valley is one of the Aosta Valley tributaries, with Triolet and Pré de Bar glaciers at its north-east. The Triolet glacier is now a partly debris-covered cirque glacier surrounded by steep rock walls with peaks exceeding 3,500 m a.s.l., from which rockfalls detach. The upper Ferret Valley floor is characterized by chaotic boulder accumulations with several ridges on the plain of Greuvetta and the Biche forest, and the sizable morainic complex of the Triolet glacier upstream (Figure 2; Deline and Kirkbride, 2009; Akçar et al., 2012).
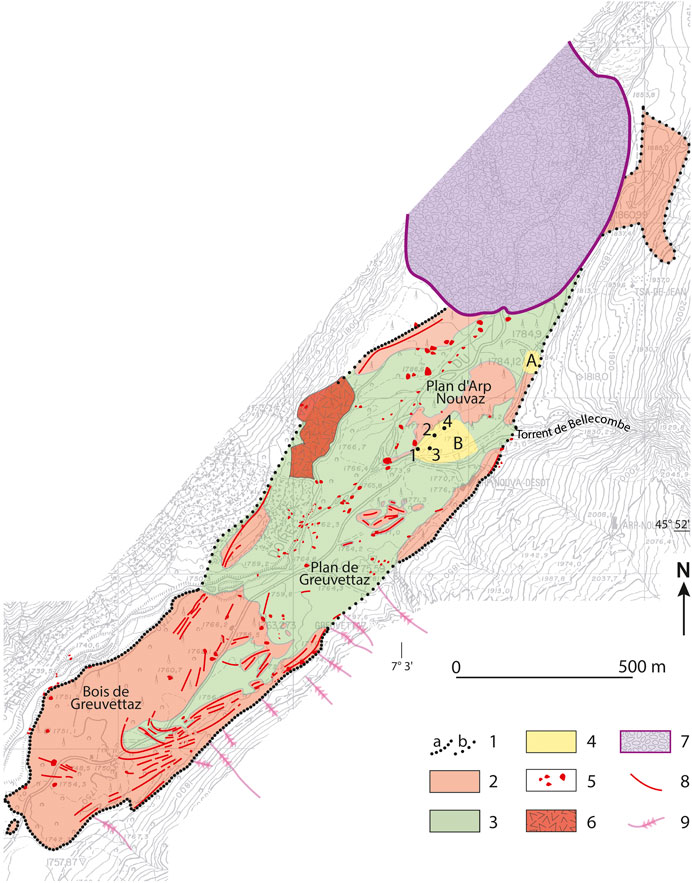
FIGURE 2. Geomorphological map of the rock avalanche deposit of 1717 CE. 1: Limit of the deposit [(A): recognized; (B): inferred]; 2: chaotic block deposit; 3: alluvial deposit; 4: peat bog; 5: megablocks; 6: local rockfall deposit; 7: Holocene moraine complex of Triolet Glacier; 8: granitic ridges; 9: snow avalanche couloir; (A): peat bog sampled by Aeschlimann (1983), (B): peat bog sampled by Orombelli and Porter (1988), and Deline and Kirkbride (2009); 1–4: location of pits from this study (Topographic map: 1:10,000 with 10 m contour interval. Archivi topocartografici della Regione Autonomo Valle d’Aosta—permit no. 52–18/08/1999).
Materials and Methods
Sampling and Sample Selection
Four spatially distributed pits were dug on Arp Nouva near the Bellecombe torrent (Figure 3 and Supplementary Figure S1). The depth in the sections varied between 60–100 cm (Figures 4A–C). Overall, 36 samples were collected from undisturbed, enriched organic layers. Our study's focus was on material deposited deeper than 30 cm, which would most likely contain the oldest deposit. The sample sections showed a succession of greyish laminated fine sediment, peat layers, and gray silty gravel (Figures 4A–C).
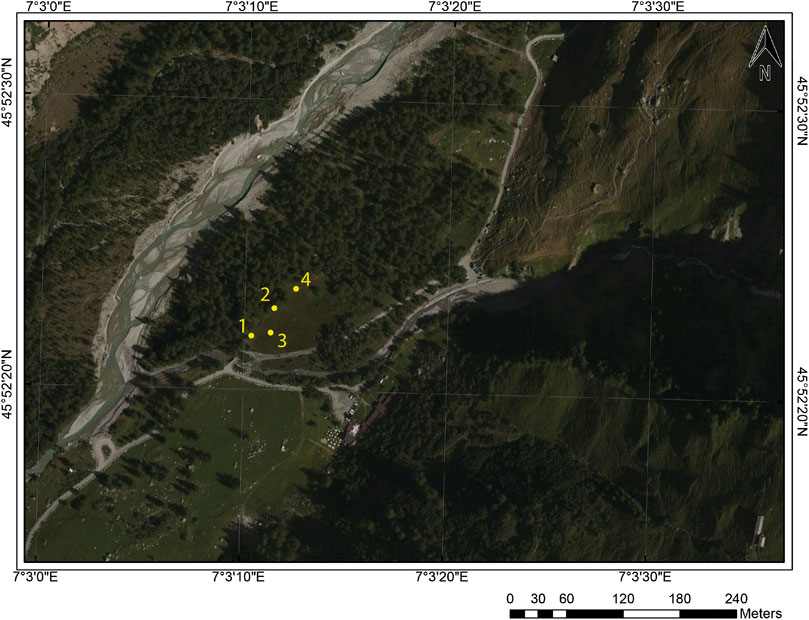
FIGURE 3. Sample location of the four pits (Arp Nouva). A boulder was found at the bottom of the pit Arp Nouva -3. The woods around the pits constitutes of larch trees.
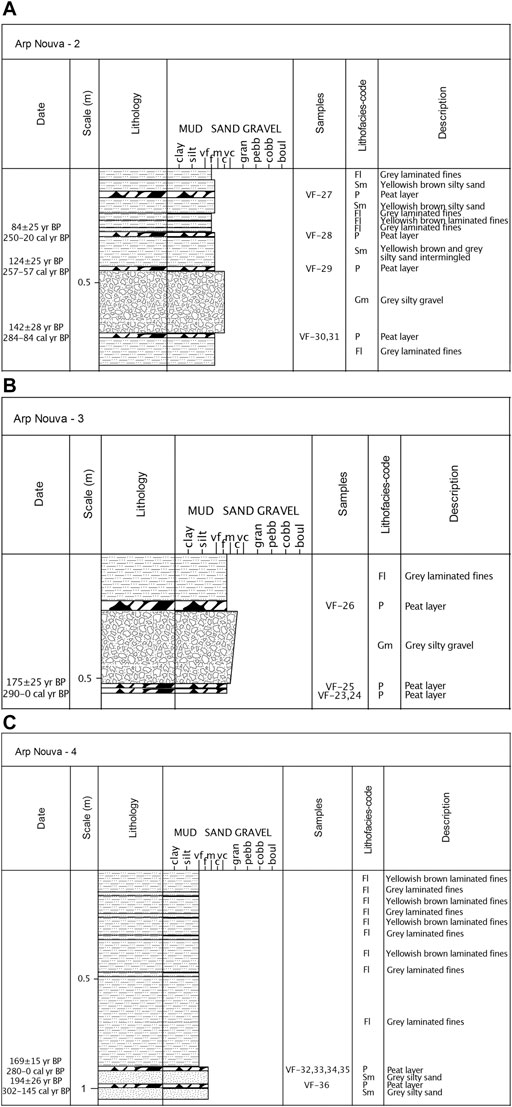
FIGURE 4. Samples and the description of soil profiles from the pits: (A) Arp Nouva-2, (B) Arp Nouva-3 and (C) Arp Nouva-4.
Radiocarbon Dating
In the ETH laboratory, samples were frozen to prevent microbial activity and the building of mold and fungus. Eight samples from three profiles were chosen for the analysis (for details, see Supplementary Figure S2). Most of the samples contained many visible roots; thus, the selection of wood fragments was essential. They were placed in glass beakers filled with DW and left for at least 24 h to disintegrate. Occasionally, the beakers were placed for 15 min in an ultrasonic bath to speed up the process. The sieving of samples was performed using sieves of mesh 500 and 125 µm. Pieces of wood were picked from a fraction of >500 µm. In one (V-23), a very small plant fragment (needle) was found and selected as a separate sample. One unidentified sample (V-27) of wood/root and one clearly identified as a large root (V-31) were picked for analysis (Supplementary Figures S3–S5). The fine fraction was saved for potential analysis. The wood fragments and one macrofossil were treated with Acid-Base-Acid to remove carbonates and humic acids, which might contaminate with old and young carbon (Hajdas, 2008). The clean material was weighed (ca. 2 mg of wood = ca. 1 mg·C) and wrapped in Sn cups for combustion in an Elemental Analyzer and a subsequent graphitization in the AGE graphitization system (Nemec et al., 2010). A set of standards (oxalic acid OXA II) and background material (phthalic anhydride) were graphitized to accompany the unknown samples’ AMS analysis. The resulting graphite samples were pressed into the aluminum cathodes for the AMS isotopic analysis. The very small sample (V-23) was analyzed as CO2 using the gas ion source (Ruff et al., 2010). The 14C/12C and 13C/12C ratio was measured on graphite samples using the dedicated 14C AMS instrument MICADAS (Synal et al., 2007).
Results
Table 1 summarizes the results of the AMS 14C analysis. The F14C is a concentration of 14C measured in the samples normalized and corrected for fractionation (δ13C). Conventional radiocarbon ages were calculated using Libby's half-life for 14C (Reimer et al., 2004; Stuiver and Polach, 1977). The δ13C values used for correction of F14C (see Reimer et al., 2004) were measured on graphite samples. Radiocarbon ages were calibrated (Table 1) using the OxCal software (Ramsey and Lee, 2013) and the INTCAL13 calibration curve (Reimer et al., 2013). In addition to simple calibration, a Bayesian model of OxCal was used to calibrate all the samples with F14C < 1 and positive 14C ages (Figures 5 and Supplementary Figure S6). The samples with F14C > 1 indicate the post-1950 source of carbon (modern). The corresponding calendar ages were obtained using the Bomb Peak 14C data (Hua et al., 2013; Levin et al., 2013) and the online calibration software http://calib.org/CALIBomb/.
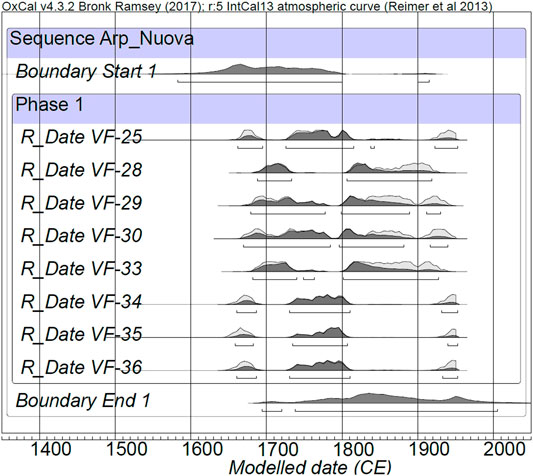
FIGURE 5. Calendar age intervals as a result of calibration. 14C ages of wood fragments from Arp Nova pits calibrated using the phase model of OxCal. Dark gray areas show posterior probability distributions resulting from the Bayesian phase model of OxCal. The light gray areas show the standard (unmodelled) probability distributions.
Discussion
The results of 14C dating obtained for various fractions selected from the bulk samples indicate the different carbon pools present in the profiles. The top 30 cm show consistently modern ages, i.e., F14C > 1 for all the subsamples of VF27 (10–13 cm). The very close agreement between sub-samples 14C signature suggests no sources of old reworked carbon deposited in the top 10 cm layer. This is not the case for samples deeper than 30 cm as in all profiles, bulk and fine fraction show presence of old carbon (V28, V29, and V36). However, the newly obtained radiocarbon ages on woody fragments selected from the peat layers in Arp Nouva show a bimodal distribution. The very small sample V-23 (tiny needle) and the V-27 and V-31 wood/root samples resulted in negative radiocarbon ages (F14C > 1). The remaining samples had radiocarbon ages between 84 ± 25 BP and 205 ± 25 BP. The negative radiocarbon ages of the root V-31 and the wood/root fragment V-27 are not surprising as the valley's meadows are overgrown with trees (Supplementary Figure S1). The root V-31 was chosen on purpose to demonstrate the presence of modern carbon deep in the soil, the fact that explains the modern (negative 14C age) date obtained by Deline and Kirkbride (2009). The presence of roots of all sizes and probably ages is indisputable. The second negative age was obtained on a much smaller woody fragment (V-27), which could also be a fragment of the large root, however unrecognizable using binocular investigation. The needle V-23 was selected in the hope that it might date the deposit. However, the calendar age of 1956–1957 or 2003–2012 indicates that this small macrofossil was modern. It was probably contamination introduced to the sample during the digging of the pit in 2013. The sample V-27, which was challenging to identify as either wood or root, has the post-1950 14C concentration, which would support identification as root. However, no radiocarbon ages older than 230 BP were observed (205 ± 25 BP), and the oldest age of the wood is limited to the late 17th century, at the earliest. Although this limit slightly predates the 1717 CE rock avalanche, such an effect can be expected when wood fragments are dated. The small wood fragments found in the organic-rich sections were deposited over the years, but their origin might be the blast in 1717 CE that killed the trees. One could expect older ages of the wood if the trees grew for some time before the event. The fact that we have not yet found much older ages of wood does not exclude such a possibility. However, radiocarbon ages as old as 885 ± 60 BP (Aeschlimann, 1983) or older (Orombelli and Porter, 1988) cannot be explained by the old wood effect. Such discrepancy must have another reason, and the most probable explanation is the presence of old carbon in sediments deposited along with fresh organic matter.
Similar to Akçar et al. (2012) and Akçar et al. (2014), our results support the conclusion of Porter and Orombelli (1980) and Orombelli and Porter (1988) that the entirety of the blocky deposits was left during the 1717 CE event. The peat layers deposited in the Ferret Valley near the locations, which were investigated in the past, are now consistently dated to maximum 370 years (1,652–1950 CE; 95.4% confidence level). The radiocarbon ages on fragments of the wood date the deposit to the late 17th/early 18th century. More precise dating is not possible due to the wiggly nature of the calibration curve, which results in multiple calendar intervals corresponding to the measured 14C ages of wood (Supplementary Figure S5). Sometimes, Bayesian models of OxCal might help to obtain a more precise chronology (Ramsey and Lee, 2013; Ramsey, 2017). However, due to the limited number of samples, the effect is not so apparent; a bigger pool of ages could improve the fit (Figure 5).
Nevertheless, our results allow us to explain the discrepancies in ages observed by Porter and Orombelli (1980), Aeschlimann (1983) and Orombelli and Porter (1988). After being able to investigate the composition of the samples, we are convinced that the scatter in previously published 14C ages is most probably due to the material chosen for dating performed in the past. From this perspective, it is admirable to read the efforts of scientists involved in the dispute. At that time, AMS facilities were scarce, and most 14C dating has been performed using conventional counting techniques. This method required at least a gram of carbon, therefore substantially larger bulk samples.
Nowadays, we can measure samples as small as 70 µg of carbon (VF-23), which allows us an in-depth analysis of the sources of carbon. The capacity and potential of the new AMS facilities allows for detailed 14C dating of multiple profiles. Independent of the sites' location, the atmospheric 14C signal corresponding to the dated event must be unraveled from the dated record. Wood and macro remains of terrestrial plants are the material of choice. Nevertheless, the application of multiple chronological methods is the best solution in dating deposits of unknown date. Our study supports and is supported by the results obtained by cosmogenic “in-situ” method applied to the boulders (Akçar et al., 2012; Akçar et al., 2014). Moreover, both dating methods are consistent with the dendrochronological investigations of Porter and Orombelli (1980), showing the potential of a multi-disciplinary approach.
Conclusion
The Ferret Valley, which is the site of the historic 1717 CE rock avalanche deposit, was revisited in 2013 and new samples were collected in the hope of resolving the controversy surrounding past inconsistent radiocarbon ages. Only woody fragments were chosen for the latest analysis. Results of radiocarbon dating of such well-defined material do not show radiocarbon ages older than 250 BP. Thus, the old radiocarbon ages (885–2,320 BP) can be explained by the choice of material. Our study highlights the importance of sample selection and illustrates the potential of the radiocarbon dating method.
Data Availability Statement
The raw data supporting the conclusions of this article will be made available by the authors, without undue reservation.
Ethics Statement
Written informed consent was obtained from the individuals for the publication of any potentially identifiable images or data included in this article.
Author Contributions
All authors listed have made a substantial, direct, and intellectual contribution to the work and approved it for publication.
Conflict of Interest
The authors declare that the research was conducted in the absence of any commercial or financial relationships that could be construed as a potential conflict of interest.
Acknowledgments
Participants of the field trip and helped to dig the pits: Olivia Steinemann Anne Claude, Loren Eggenschwiler, Regina Reber, Serdar Yesilyurt, André Roveyaz, Fabian Christener, and Melaine le Roy. Colleagues at the Laboratory of Ion Beam Physics, ETH for support with laboratory preparation and AMS analysis.
Supplementary Material
The Supplementary Material for this article can be found online at: https://www.frontiersin.org/articles/10.3389/feart.2020.580293/full#supplementary-material.
References
Aeschlimann, H. (1983). Zur Gletschergeschichte des italienischen Mont Blanc Gebietes Val Veni–Val Ferret–Ruitor. Switzerland: Zurich University
Agassiz, L. (1845). “Les glaciers et le terrain erratique du revers meridional du Mont‐Blanc,” in Nouvelles excursions et séjours dans les glaciers et les hautes regions des Alpes de M. Agassiz et de ses compagnons de voyage. Editor E. Desor (Neuchatel: Kissling)
Akçar, N., Deline, P., Ivy-Ochs, S., Alfimov, V., Hajdas, I., Kubik, P., et al. (2012). The AD 1717 rock avalanche deposits in the upper Ferret Valley (Italy): a dating approach with cosmogenic Be-10. J. Quat. Sci. 27, 383–392. doi:10.1002/jqs.1558
Akçar, N., Ivy-Ochs, S., Deline, P., Alfimov, V., Kubik, P. W., Christl, M., et al. (2014). Minor inheritance inhibits the calibration of the Be-10 production rate from the AD 1717 val ferret rock avalanche, European Alps. J. Quat. Sci. 29, 318–328. doi:10.1002/jqs.2706
Deevey, E. S., and Stuiver, M. (1964). Distribution of natural isotopes of carbon in linsley-pond and other new-England lakes. Limnol. Oceanogr. 9 (1), 1–11
Deline, P., and Kirkbride, M. P. (2009). Rock avalanches on a glacier and morainic complex in haut val Ferret (Mont Blanc massif, Italy). Geomorphology 103, 80–92. doi:10.1016/j.geomorph.2007.10.020
Hajdas, I. (2008). The Radiocarbon dating method and its applications in Quaternary studies. E&G Quat. Sci. J. 57, 2–24. doi:10.3285/eg.57.1-2.1
Hua, Q., Barbetti, M., and Rakowski, A. Z. (2013). Atmospheric radiocarbon for the period 1950–2010. Radiocarbon 55, 2059–2072. doi:10.2458/azu_js_rc.v55i2.16177
Levin, I., Kromer, B., and Hammer, S. (2013). Atmospheric Delta (CO2)-C-14 trend in Western European background air from 2000 to 2012. Tellus Ser. B Chem. Phys. Meteorol. 65 (1), 20092. doi:10.3402/tellusb.v65i0.20092
Mayr, F. (1969). Die postglazialen Gletscherschwankungen des Mont Blanc-Gebietes. Zeitschrift für Geomorphology 8 (Suppl.), 31–57
Nemec, M., Wacker, L., and Gaggeler, H. (2010). Optimization of the graphitization process at age-1. Radiocarbon 52, 1380–1393. doi:10.1017/s0033822200046464
Orombelli, G., and Porter, S. C. (1988). Boulder deposit of upper val Ferret (courmayeur, Aosta Valley) - deposit of a historic giant rockfall and debris avalanche or a late-glacial moraine. Eclogae Geol. Helv. 81, 365–371
Porter, S. C., and Orombelli, G. (1980). Catastrophic rockfall of september 12, 1717 on the Italian flank of the Mont Blanc massif. Zeitschrift für Geomorphologie Stuttgart 24, 200–218
Ramsey, C. B., and Lee, S. (2013). Recent and planned developments of the program oxcal. Radiocarbon. 55, 720–730. doi:10.1017/s0033822200057878
Ramsey, C. B. (2017). Methods for summarizing radiocarbon datasets. Radiocarbon 59, 1809–1833. doi:10.1017/rdc.2017.108
Reimer, P., Brown, T., and Reimer, R. (2004). Discussion: reporting and calibration of post-bomb C-14 data. Radiocarbon 46, 1299–1304. doi:10.1017/s0033822200033154
Reimer, P. J., Bard, E., Bayliss, A., Beck, J. W., Blackwell, P. G., Ramsey, C. B., et al. (2013). Selection and treatment of data for radiocarbon calibration: an update to the international calibration (IntCal) criteria. Radiocarbon 55, 1923–1945. doi:10.2458/azu_js_rc.55.16955
Ruff, M., Szidat, S., Gaggeler, H. W., Suter, M., Synal, H. A., and Wacker, L. (2010). Gaseous radiocarbon measurements of small samples. Nucl. Instrum. Methods Phys. Res., Sect. B. 268, 790–794. doi:10.1016/j.nimb.2009.10.032
Sacco, F. (1918). I ghiacciai italiani del gruppo del Monte Bianco. Bolletino del Comitato Glaciologico Italiano, 3: 21–102.
Saussure, H.-B. (1786). Voyages dans les Alpes précédés d’un essai sur l’histoire naturelle des environs de Genève. Tome second. Barde, Manguet & Compagnie, Genéve, XVI-641 p.
Stuiver, M., and Polach, H. A. (1977). Reporting of C-14 data - discussion. Radiocarbon. 19, 355–363
Synal, H. A., Stocker, M., and Suter, M. (2007). MICADAS: a new compact radiocarbon AMS system. Nucl. Instrum. Methods Phys. Res. Sect. B Beam Interact. Mater. Atoms 259, 7–13. doi:10.1016/j.nimb.2007.01.138
Virgilio, F. (1883). Sui recenti studi circa le variazione periodiche dei ghiacciai. Bolletino del Club Alpino Italiano. 50, 50–70
Keywords: radiocarbon dating, peat, rock avalanche, Mont Blanc massif, Val Ferret, Triolet
Citation: Hajdas I, Sojc U, Ivy-Ochs S, Akçar N and Deline P (2021) Radiocarbon Dating for the Reconstruction of the 1717 CE Triolet Rock Avalanche in the Mont Blanc Massif, Italy. Front. Earth Sci. 8:580293. doi: 10.3389/feart.2020.580293
Received: 06 July 2020; Accepted: 23 December 2020;
Published: 29 January 2021.
Edited by:
Steven L. Forman, Baylor University, United StatesReviewed by:
Vincenzo Amato, University of Molise, ItalyCristina Maria Pinto Gama, University of Evora, Portugal
Copyright © 2021 Hajdas, Sojc, Ivy-Ochs, Akçar and Deline. This is an open-access article distributed under the terms of the Creative Commons Attribution License (CC BY). The use, distribution or reproduction in other forums is permitted, provided the original author(s) and the copyright owner(s) are credited and that the original publication in this journal is cited, in accordance with accepted academic practice. No use, distribution or reproduction is permitted which does not comply with these terms.
*Correspondence: Irka Hajdas, aGFqZGFzQHBoeXMuZXRoei5jaA==