- 1Jilin Changbaishan Volcano National Observation and Research Station, Institute of Geology, China Earthquake Administration, Beijing, China
- 2Institute of Volcanology, China Earthquake Administration, Changchun, China
- 3Changbai Mountain Volcano, The Academician Workstation of Jilin Province, Antu, China
- 4Jilin Earthquake Agency, Changchun, China
- 5School of Earth and Atmospheric Sciences, Georgia Institute of Technology, Atlanta, CO, United States
- 6School of Earth Sciences and Resources, China University of Geosciences, Beijing, China
- 7Institute of Surface-Earth System Science, School of Earth System Science, Tianjin University, Tianjin, China
One of the most active intraplate volcanoes in East Asia, Changbaishan volcano experienced unrest from July 2002 to July 2005. On 2002/06/28, the M 7.2 Wangqing deep-focus earthquake occurred ∼290 km northeast of Changbaishan volcano. While some studies have suggested a possible triggering relationship, the physical mechanism of such distant interaction is still not well understood. Using a template matching technique, which cross-correlates waveform of known events with continuous data, we perform systematic detection of microseismic events recorded by station CBS near Changbaishan volcano from July 1999 to July 2007. The detected earthquakes can be further categorized into three different types: volcano-tectonic (VT) events, long-period (LP) events and harmonic-spectra (HS) events. We detect 3763 VT events between July 2002 and July 2007. The intense VT earthquake swarm during the period from July 2002 to July 2005, along with recurring LPs and HSs and other geodetic/geochemical evidence, suggest magma movement during unrest. Compared with the hand-picked catalogue, the catalogue obtained by template matching technique reveals a delayed-triggering relationship between Wangqing deep-focus earthquake and unrest. The small magnitudes of the VT events and the limited numbers of LP and HS events suggest that the Wangqing mainshock likely triggered bubble excitation in the mid-crust magma system, resulting in overpressure and a small magma injection into the shallow magma chamber at a depth of ∼5 km, leading to the 3-years unrest.
Introduction
It is well known that large earthquakes are capable of triggering small to moderate-size earthquakes at long distances, especially in geothermal or volcanic regions (Hill and Prejean, 2015). A few studies have also documented significant increase of volcanic eruptions within a few days of large distant earthquakes (Linde and Sacks, 1998; Manga and Brodsky, 2006; Walter and Amelung, 2007; Sawi and Manga, 2018). At distance of more than a few hundred kilometers, static stress changes become negligible (King et al., 1994; King and Deves, 2015). Hence, mechanisms such as magma overpressure due to permanent volumetric expansions, or unclamping of dikes are not relevant (Walter and Amelung, 2007). Possible shaking related mechanisms due to dynamic seismic waves include gas bubble nucleation and growth in magma (Crews and Cooper, 2014), changes in permeability (Manga et al., 2018), or sloshing of a bubbly magma reservoir (Namiki et al., 2016). However, the physical mechanism involved in such remote interaction is still in debate. In addition, most remote earthquake-triggered eruptions occur along major plate boundaries and are associated with shallow subduction-zone earthquakes (e.g., Hill et al., 2002; Lara et al., 2004; Walter and Amelung, 2006, 2007; Sawi and Manga, 2018; Farías and Basualto, 2020). It is not clear whether deep-focus earthquakes are capable of triggering unrest and eruptions.
Most of the microseismicity around active volcanoes belongs to VT-type, which is likely caused by shear slip on a fault near a volcano, and produces clear P and S waves with sharp onset. In addition, there are some other types of seismic events associated with volcanoes. For example, long-period (LP) events exhibit emergent P waves, but no clear S waves, with dominant frequencies of 0.5–5 Hz (Chouet, 1996; McNutt, 2002). A harmonic-spectra (HS) event is another special type of fluid-related seismic event. Whose spectral signature is strikingly harmonic (Hough et al., 2000; Milluzzo et al., 2010). Both LP and HS events are associated with either magma or magma-derived fluid-controlled sources (Chouet, 1996; Hough et al., 2000; McNutt, 2002).
Changbaishan volcano (also known as Paektu/Baekdu in Korea) is one of the most active intraplate volcanoes in East Asia (Liu et al., 1998; Zhang et al., 2018) (Figure 1A). Its summit caldera is called Tianchi (Chinese for “Heaven Lake”), which situates at the border between China and North Korea. Continuous seismic monitoring of Changbaishan volcano has been in operation since the establishment of Changbaishan Volcano Observatory (CHVO) in July 1999. Volcanic unrest during the period from July 2002 to July 2005 has been reported by previous studies, which was accompanied by earthquake swarms, surface inflation, uplift of the cone, and geochemical anomalies in the gas emissions (Wu et al., 2005; Liu et al., 2011a; Liu et al., 2011b; Xu et al., 2012; Wei et al., 2013). Based on inversion of ground deformation measurements, this unrest is interpreted as resulting from a shallow intrusion of basaltic magma centered at 2–6 km beneath the volcano’s summit. During this “active period,” thousands of volcano-tectonic (VT) earthquakes were recorded. Most of them were small-magnitude events, and the largest event occurred in December 2004 had a local magnitude ML of 4.4.
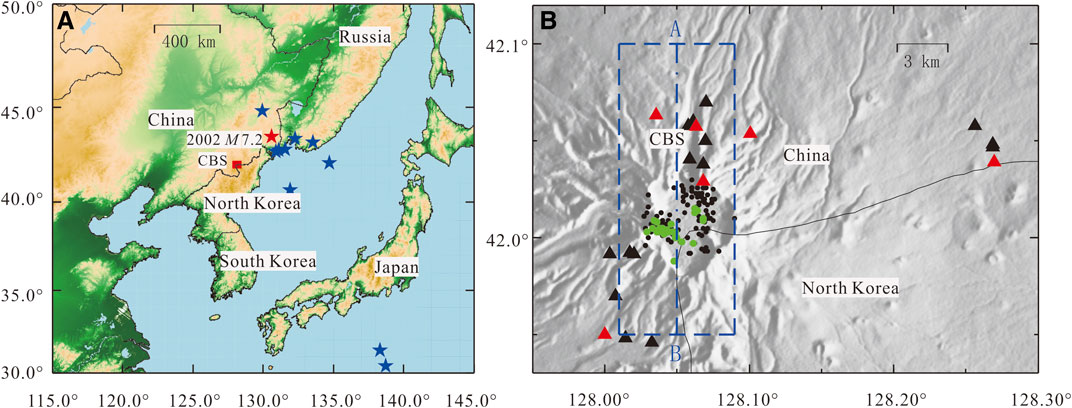
FIGURE 1. (A) Map showing the location of Changbaishan volcano in East Asia. The red star marks the location of the 2002 M 7.2 Wangqing earthquake. Blue stars mark other deep-focus earthquakes in this region. The blue triangles indicate the JEA catalogue stations, and the red box marks the location of the enlarged plot in (B). (B) Distribution of seismic stations and different types of seismic events during the volcanic unrest period. The red triangles mark stations used to build the CHVO catalogue, the black triangles indicate stations for the Wu catalogue, the black dots indicate VT events, and the green dots indicate HS events.
On June 28, 2002, an Mw 7.2 deep-focus earthquake occurred at a depth of 566 km approximately below the city of Wangqiang, which is about 290 km northeast of Changbaishan volcano (Figure 1A). This is the largest deep-focus earthquake occurred within 500 km (horizontal distance) of the volcano since 2000. A few previous studies have argued that the 2002 Wangqing earthquake may have triggered the volcanic unrest, which started in early July of 2002 (Lv et al., 2007; Liu et al., 2017). However, the available earthquake catalogue in this region did not show any clear increase of seismicity between the 2002 Wangqing earthquake and the onset of the volcanic unrest. Hence, the triggering relationship is still not clear.
Wu et al. (2005) deployed 15 temporary seismic stations to study volcanic seismic activities in Changbaishan volcano during the summer months from 2002 to 2007. They identified thousands of VT events with dominant frequency of 5–15 Hz, as well as 38 volcanic HS events with several harmonic frequency peaks. However, they failed to identify any clear LP events or volcanic tremor signals during their observations (Wu et al., 2005; Ming et al., 2007).
In this study, we obtain a complete and accurate VT catalogue based on a template matching technique (Shelly et al., 2007; Peng and Zhao, 2009; Zhang and Wen, 2015). We attempt to address two important issues that are crucial for understanding the physical processes involved in the 2002–2005 Changbaishan volcanic unrest. The first issue is related to the possible triggering relationship between the 2002 Wangqing deep-focus earthquake and the unrest. The second is whether any LP signals (either LP events or volcanic tremors) occurred within thousands of VT earthquakes during the 2002–2005 volcanic unrest period, which are typically used to infer magma or fluid movement (Chouet, 1996; McNutt, 2002).
Background
Changbaishan volcano has been widely recognized as an active volcano of global significance due to its Millennium eruption (VEI ≈ 7) in 946 CE (Xu et al., 2012; Oppenheimer et al., 2017; Hakozaki et al., 2018; Zhang et al., 2018). Seismic tomography (e.g., Zhao et al., 2009) has revealed that Changbaishan volcano is located above a big mantle wedge (BMW) defined by the subducting Pacific plate that is horizontally stagnant in the mantle transition zone (MTZ) and the overriding Eurasian plate. The BMW is thus widely considered as the first-order geodynamic feature responsible for origin of Changbaishan volcano (Kuritani et al., 2011; Zhao and Tian, 2013; Zhang et al., 2018). Recently, Tang et al. (2014) also performed seismic tomography in this region with dense temporary seismic network, and imaged a possible gap in the subducted slab that may be responsible for producing the decompression melting material that feeds Changbaishan and other active volcanoes in this region (e.g., Wudalianchi and Jingbohu volcanoes).
The magma plumbing system of Changbaishan volcano remains less understood at present. However, geophysical inversions indicate that there are at least three layers of magma reservoirs beneath Changbaishan volcano. The lowermost one is approximately located at Moho/lower crustal depths (∼30–40 km; Ri et al., 2016; Kim et al., 2017). The intermediate one is within mid-crustal depths (∼15–25 km; Tang et al., 2001), and the depths of the uppermost one is constrained to be within 10 km below the surface (Tang et al., 2001; Iacovino et al., 2016; Hammond et al., 2020). Geodetic inversions of inflation during the 2002–2005 unrest revealed a possible shallow magma chamber at the depth range of ∼5 km (Xu et al., 2012).
Lv et al. (2007) studied the relationship between Changbaishan volcanic earthquakes and intermediate-depth (60–300 km) and deep-focus (>300 km depth) earthquakes (including the 2002 Wangqing earthquake) that occurred along the subduction zone of the western Pacific Plate. Based on a comparison between the occurrence times of deep subduction-zone earthquakes and the VT earthquake rates, they suggested that earthquake swarms around Changbaishan volcano might be triggered by intermediate-depth and deep-focus subduction earthquakes (Lv et al., 2007). Based on historic documents in China and Korea, Li et al. (2012) showed that a magnitude greater than 8 deep-focus earthquake occurred in the “Hunchun-Wangqing deep-focus seismic zone” on October 6, 1597, and likely triggered numerous lake seiches in East China and a small-medium scale explosive eruption at Mt. Wangtian’e volcano (about 30 km away from Changbaishan volcano). These studies suggest a possible triggering relationship between deep earthquakes and volcanic eruptions in our study region.
Liu et al. (2017) investigated the triggering relationship between microearthquakes around Changbaishan volcano and five nuclear explosions in North Korea, as well as large shallow earthquakes at remote distances. They did not find any clear changes in microseismicity during the nuclear explosions with the maximum equivalent magnitude of 6.3. This is consistent with a recent numerical simulation indicating that a nuclear explosion of equivalent magnitude 7 is needed to produce dynamic stresses high enough to trigger eruptions of Changbaishan volcano (Hong et al., 2016). However, Liu et al. (2017) did identify a few large distant earthquakes (e.g., the 2004 Mw 9.2 Sumatra, the 2008 Mw 7.9 Wenchuan, and the 2011 Mw 9.1 Tohoku-Oki earthquakes) that triggered microearthquakes during their large-amplitude surface waves. These studies suggest that Changbaishan volcano may be sensitive to external stress perturbations due to seismic waves from shallow distant earthquakes.
Data and Methodology
During the volcanic unrest period of Changbaishan volcano (2002–2005), there were six seismic stations operated by CHVO (Figure 1B). Among these, station CBS was deployed on stable bedrock in an observation tunnel 3 km from Tianchi caldera and recorded continuously, while the other five seismic stations were deployed in the volcanic area. Due to harsh weather and other environmental conditions, these five stations only work intermittently during summer (June–October) every year, and the quality of their data is not as high as that from station CBS. To ensure the consistency of results for the entire time period, we use the catalogue produced by the single station CBS as a proxy of seismic activities of Changbaishan volcano.
In this study, we use the Match and Locate (M&L) method (Zhang and Wen, 2015) for earthquake detection. Similar to the standard matched-filter technique (e.g., Peng and Zhao, 2009), the M&L method first detects small events through continuous waveforms from stacked cross-correlation traces between template waveforms and potential events in the continuous waveforms over multiple stations and components. It then identifies the best-fitting locations of newly detected events by performing a grid search with differential travel times. In this study, we use three-component waveforms from the single station CBS, instead of multiple stations. Therefore, we do not perform any grid search for the newly detected event, but take the location of best-matching template event as that of newly detected events To improve the signal-to-noise ratio (SNR) of local seismic signals, we apply a bandpass filter of 2–8 Hz to both the template and continuous waveforms. We use a 25-s-long window starting 5 s before the hand-picked S arrival as template waveform. To confirm positive detections, we use two thresholds: a mean correlation coefficient (CC) value >0.78 and an SNR of the stacked cross-correlograms >2.0, following Zhang and Wen (2015). We test a large number of combinations of CC and SNR thresholds during the search process, and determine the optimum threshold of CC >0.78 and SNR >2.0 for this work. We choose a relatively high CC value because we have only one station recording with three components, which is subject to a higher false-detection rate than it would be the case for multiple stations. Once the mean CC and SNR values of a potential event exceed the pre-defined thresholds, we consider this as a positive detection (Figure 2).
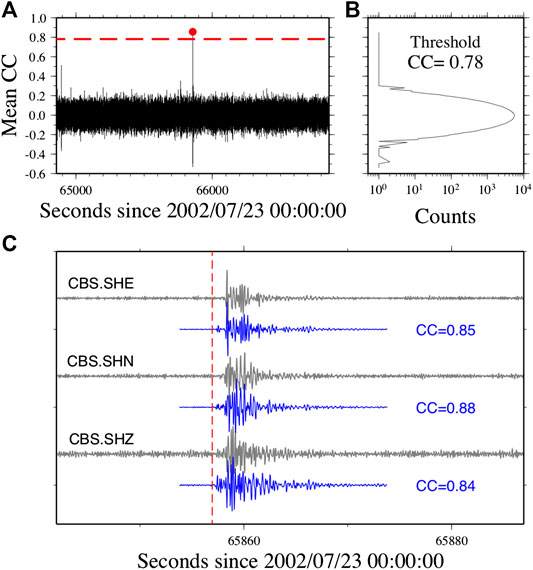
FIGURE 2. (A) Mean correlation coefficients between the template seismograms and the continuous waveforms in (C). The red dashed line indicates the mean CC threshold (0.78) for detection, the black line the mean CC value, and the red point the mean CC value of a positive detection. (B) Histogram of the mean CC in (A). (C) Template seismograms (blue traces) and continuous waveforms (gray traces). The red dashed line indicates the origin time of a positive detection and the template earthquake. The channel name and CC value are given on the left- and right-hand sides of each trace, respectively.
From July 1999 to July 2007, there are 3,427 seismic events in the CHVO manually picked catalogue (Supplementary Table S1). We visually examine waveforms of all events, and manually remove 1661 listed in the CHVO catalogue as possible false detections. These false detections are mainly of two types: interference signals and explosion-type signals from nearby industry activities (Supplementary Figure S1). To ensure the operational efficiency of the M&L program and to guarantee that all the remaining 1766 events can be recovered by the procedure, we first select 50 events with clear P and S waves and highly accurate locations as initial templates and scan through the time windows that include the remaining 1766 events. Any time an event is not detected by the M&L procedure, we manually stop the scanning and add this event to the templates, after which we restart the procedure. In this way, all of the 1766 remaining events can be detected by the new catalogue. In the end, the number of template events grows to 536 (Supplementary Table S2).
The locations of the 536 template events used in this study are obtained from three different catalogues. The first is the catalogue provided by the temporary seismic network (Wu et al., 2005), which only works during summer every year from 2002 to 2007. A total of 15 seismic stations close to Tianchi caldera, with a station spacing of 5 km provides reasonable station coverage. Here, we term this the Wu Catalogue. The second catalogue is that provided by the regional seismic network operated by the Jilin Earthquake Agency (the JEA catalogue), which consists of 10 regional seismic stations with an average distance of 100 km around Tianchi caldera. The third catalogue is that provided by the CHVO, which has been manually picked with continuous waveforms of the single station CBS (CHVO catalogue, Supplementary Table S1). The events are subsequently located if they are recorded by more than three stations in the CHVO. Among these three catalogues, the location accuracy of the Wu catalogue is the highest, and that of the CHVO catalogue is the lowest. Thus, if one event has been listed in two or three catalogues, we use the location listed in the catalogue with highest accuracy. For any new event detected by the M&L technique with the single station CBS, we simply assign the location of the template event with the highest mean CC value. As the combined template catalogue may not include all possible events with different locations or mechanisms, our final automatically detected catalogue is likely not complete, although it is more complete than any of manually picked catalogues.
To confirm the existence of potential triggering, we compare the seismicity changes before and after a specific event with the β-statistic value (Matthews and Reasenberg, 1988). A β-statistic value greater than 1.96 is generally considered as a statistically significant seismic increase with 95% confidence level (Gomberg et al., 2001; Hill and Prejean, 2007; Aron and Hardebeck, 2009; Peng and Gomberg, 2010; Liu et al., 2017). We use the following expression given by Aron and Hardebeck (2009):
where
Results
Using 536 templates, we detect 3,763 events through waveforms of station CBS from July 1999 to July 2007 (Figure 3B and Supplementary Table S3). The automatically detected catalogue not only includes 1776 events after visual inspection of the manually picked catalogue, but also includes 1987 newly detected events. Intensive seismicity started on July 5, 2002, and lasted for about three years. After the volcanic unrest (Figure 3A), the seismicity of Changbaishan volcano dropped slowly, and resumed to the background level around 2008. In order to provide a comprehensive view of the seismicity of Changbaishan volcano from 1999 to 2018, we use the joint earthquake catalogue, which include events produced by automatic detection from July 1999 to July 2007 and those listed in the CHVO catalogue after August 2007.
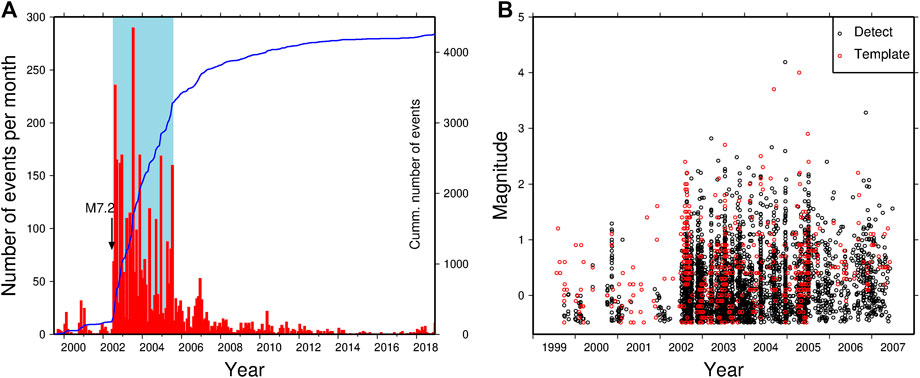
FIGURE 3. (A) Monthly rates of seismicity listed in the joint catalogue (red bars) from 1999 to 2018 around Changbaishan volcano. The joint catalogue is produced by merging two catalogues. The first is the automatically detected catalogue from Jul. 1999 to Jul. 2007 in this study, and the second one is manually picked catalogue from Aug. 2007 to Dec. 2018. The light blue shaded area indicates the time period of volcanic unrest. The blue curve represents the cumulative number of events. (B) Template events (red circles) and detected events (black circles) listed in the automatically detected catalogue.
Seismicity Changes Following the 2002 Wangqing Deep Earthquake
As mentioned before, on June 28, 2002 at 17:19:25 UTC, an Mw 7.2 earthquake at a depth of 566 km struck the city of Wangqing, which is about 290 km from Tianchi caldera (Figure 1A). The Wangqing mainshock was recorded well by five stations in the CHVO (Supplementary Figure S2). Before the Wangqing mainshock, the CHVO catalogue includes some false identifications, which have been removed by visual inspection (Supplementary Figure S3). After the mainshock, the automatically detected catalogue includes some newly detected events that were not listed in the CHVO catalogue (Figure 4 and Supplementary Figure S3). On July 5, 2002, seven days after the mainshock, the number of VT events increased significantly, marking the onset of the volcanic unrest (Figure 4).
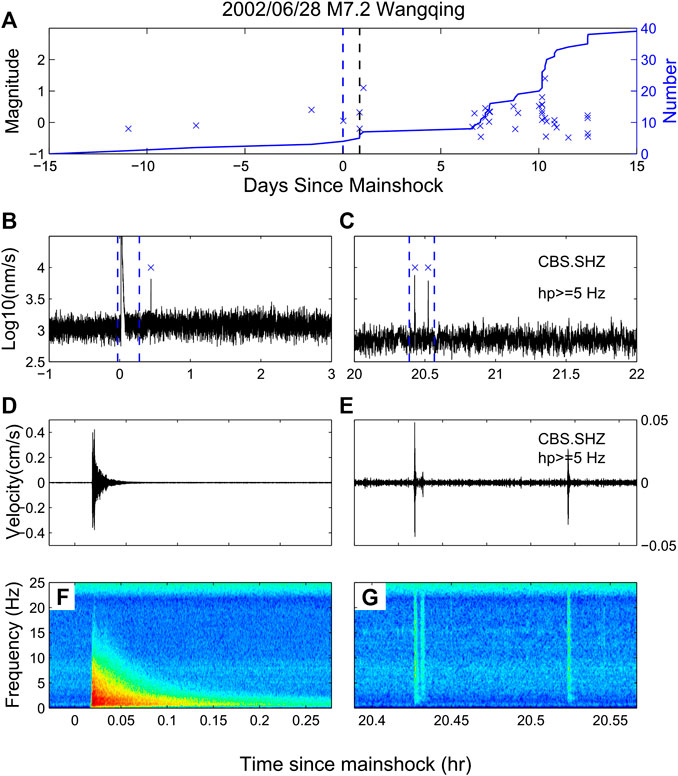
FIGURE 4. (A) Seismicity within 15 days before and after the 2002 M 7.2 Wangqing earthquake. The crosses mark events listed in the newly detected catalogue, the blue curve shows the cumulative number of earthquakes, and the vertical blue lines indicate the time window plotted in (B) and (C). (B,C) Envelope functions of the high-pass filtered 5 Hz waveform during and after the 2002 M 7.2 Wangqing earthquake. The newly detected earthquakes are marked as blue crosses. (D,E) Zoom-in plots of the areas between the blue dashed lines in (B) and (C). (F,G) Spectrograms of (D) and (E).
Using a 10-days time window before and after the Wangqing mainshock, the β-statistic values at July 5, 2002 for the newly detected and CHVO catalogues (βAutomatic and βManually picked) are calculated as 5.1 and 1.0, respectively (Figure 5), suggesting a possible triggering relationship. We note that βAutomatic is larger than βManually picked, mainly because several false detections in the manually picked catalogue were removed and additional events following the Wangqing mainshock were included during the detection process.
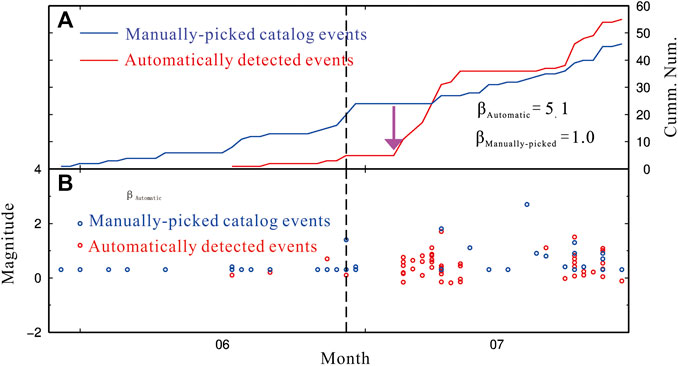
FIGURE 5. (A)β-statistic values of automatically detected (red curve) and manually picked (blue curve) catalogues. (B) Changbaishan volcanic earthquake activities before and after the 2002 Wangqing deep-focus earthquake. The purple arrow marks the onset of volcanic unrest (i.e., July 5, 2002).
Seismicity Changes Following Other Deep-Focus Earthquakes
During the study time period from 2000 to 2019, 67 deep-focus earthquakes (depth >300 km, magnitude >4) occurred within 1000 km around Tianchi caldera (Supplementary Table S4), as listed in the International Seismological Center (ISC) catalog. We measure the peak ground velocity (PGV) of each earthquake on the vertical component of station CBS after removing the instrument response and applying a low-pass filter of 5 Hz to avoid contamination by high-frequency local noise. The PGV of the Wangqing earthquake is 1.2 cm/s (Supplementary Table S4 and Figure 6), which is several times larger than that of other deep-focus earthquakes. Assuming a shear rigidity μ of 30 GPa, and a phase velocity Vph of 3.0 km/s, we can convert the PGV of the Wangqing earthquake (1.2 cm/s) into the dynamic stress σd 120 kPa with the following equation:
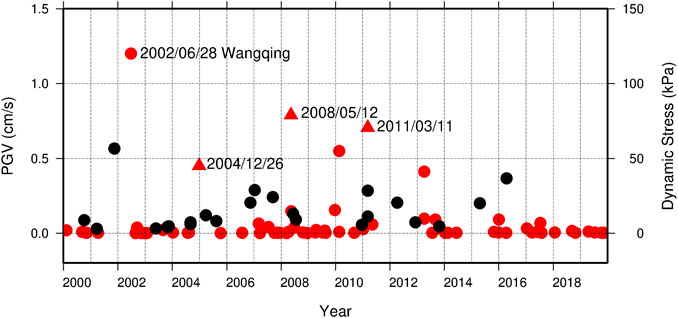
FIGURE 6. PGV and dynamic stress of deep-focus earthquakes and large distant earthquakes. Red circles represent deep-focus earthquakes within 500 km. Black circles represent large distant earthquakes beyond 1000 km. Three large distant earthquakes (2004 M 9.3 Sumatra, 2008 M 7.9 Wenchuan, and 2011 M 9.1 Tohoku-Oki earthquakes) that dynamically triggered micro-seismicity in Changbaishan volcano are marked by red triangles (Liu et al., 2017).
This is just an approximation since these values would likely be different at depth. But it provides a rough back-to-the-envelope estimation of the associated dynamic stress. Figure 6 also includes the PGVs of large distant earthquakes analyzed by Liu et al. (2017). The PGV of the Wangqing mainshock is slightly larger than those of large distant earthquakes that triggered microseismicity during their large-amplitude surface waves (e.g., the 2004 M 9.3 Sumatra, 2008 M 7.9 Wenchuan, and 2011 M 9.1 Tohoku-Oki earthquakes).
As mentioned before, VT earthquakes in Changbaishan volcano might be related to deep-focus earthquakes occurring in nearby regions (Lv et al., 2007). To check the relationship between other deep-focus events and local earthquakes, we plot the daily earthquake numbers and all deep-focus earthquakes with magnitude greater than 4 within 1000 km (Figure 7). In addition, we compute a running-window β-statistic time series (with T = 10 days, and a sliding window step of 1 day, Ta = 5 days) to help identify the triggering relationship. As expected, the β-statistic time series reflects the seismicity rate change quite well, with each peak in the β-value corresponding to a local earthquake swarm. In particular, the β-value on July 5, 2002 seven days after the June 28, 2002 Wangqing deep-focus earthquake, is as high as 5.1, setting the highest record since 1999. We argue that such a significant increase in seismicity suggests that the Wangqing earthquake might have triggered Changbaishan volcanic activity with a delayed response of seven days.
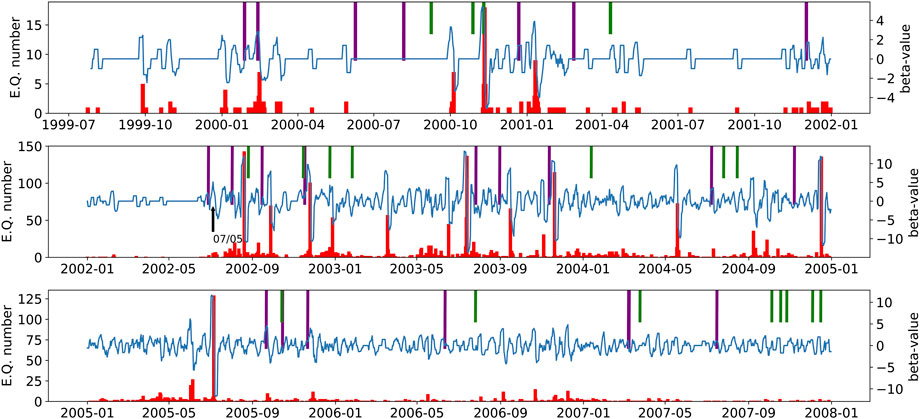
FIGURE 7. Daily earthquake numbers, β-statistic value from the detected catalog, and occurrence time of deep-focus earthquakes. The x-axis represents the date, the left y-axis the number of earthquakes per day, and the right y-axis the value of the β-statistic. The red bars indicate the number of earthquakes. The purple and green bars indicate deep-focus earthquakes greater than M 6 within 1000 km and earthquakes greater than M 4 within 500 km from Changbaishan volcano, respectively. The blue curves represent time series of the β-statistic, which has been computed with Ta = 5 days and a sliding window step of 20 days.
Out of the 22 selected deep-focus earthquakes with magnitude greater than M 6 that occurred within nine years (1999–2007), 12 (54.5%) were followed by increased seismicity (defined as β-statistic values >2 with T = 10 days and centering around the deep-focus earthquakes) (Figure 7). Using the same method, we analyze the triggering relationship between deep-focus earthquakes and Changbaishan seismic activities during 2008–2019 with the manually picked CHVO catalog (Supplementary Figure S4). Out of 26 selected deep-focus earthquakes during this time period, 16 (61.5%) were followed by increased seismicity. Our observations are generally consistent with previous studies, suggesting a moderate triggering relationship between deep-focus events and local seismicity at CBS (Lv et al., 2007; Liu et al., 2017).
Since July 1999, 67 (Supplementary Table S4) deep-focus earthquakes within 500 km (horizontal distance) occurred in this region; some are associated with increased seismicity in Changbaishan volcano, others are not. However, it is worth noting that the 2002 Wangqing mainshock is the largest deep-focus earthquakes occurred in this region in the past few decades. We also checked the local catalogue and waveforms at station CBS during other large deep-focus earthquakes at remote distances (e.g., 2013 M 8.3 Okhotsk Sea earthquake), and did not find any clear triggering evidence. Hence, it appears that only large deep-focus earthquakes occurring nearby (within 500 km in horizontal distance) are followed by unrest in this region.
Comparison Between Triggering by Deep-Focus Earthquakes and by Large Distant Earthquakes
In this section we compare the amplitude spectra of those distant shallow earthquakes that triggered seismicity in Changbaishan and deep-focus earthquakes with magnitude greater than 6 to identify potential differences in triggering behaviors between them (Figure 8). As expected, the amplitude spectra of deep earthquakes and those of large distant earthquakes show clearly different characteristics. For large distant earthquakes, the peaks appear in the low-frequency range (0.02–0.08 Hz, or 12.5–50 s), whereas for deep-focus ones, the peaks appear in the high-frequency range (0.4–2 Hz). Particularly for the 2002 Wangqing deep-focus earthquake, the high-frequency part (e.g., ∼1 Hz) of the amplitude spectrum is at least one order higher than those of large distant earthquakes.
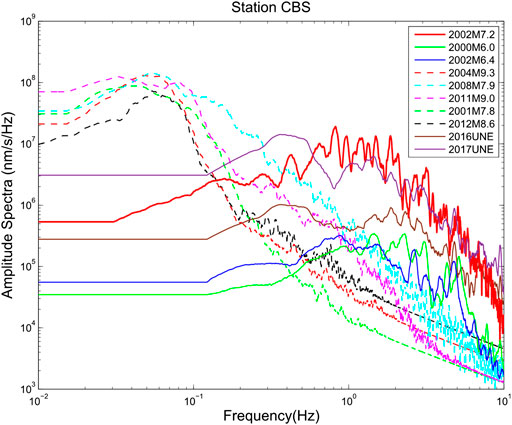
FIGURE 8. Amplitude spectra for the Wangqing earthquake and other deep-focus earthquakes and shallow distant earthquakes. Dashed curves correspond to shallow distant earthquakes, and solid lines to deep-focus earthquakes and the largest UNEs in North Korea. The linear-like spectra at high-frequency ranges (>1 Hz) for some events are due to small gap in the continuous waveform, resulting in some high-frequency artefact.
Although the Wangqing mainshock and three large distant earthquakes (2004 M 9.3 Sumatra, 2008 M 7.9 Wenchuan, and 2011 M 9.1 Tohoku-Oki earthquakes) triggered seismicity in Changbaishan volcano, the triggering seismic phases and the triggered seismicity are likely different. Large distant earthquakes dynamically trigger microseismicity through their large-amplitude surface wave trains, but are not followed by subsequent changes in seismic behaviors in Changbaishan volcano (Liu et al., 2017). In comparison, deep-focus earthquakes likely trigger microseismicity through their high-frequency body waves. We do not observe any instantaneous triggering during the Wangqing mainshock, but a time delay of 7 days is observed before the onset of the 2002–2005 earthquake swarm. Comparing with the spectrum of the Wangqing mainshock, the 2017 mb = 6.0 underground nuclear explosion (UNE) in North Korea has a slightly longer period of around 0.5 Hz, likely due to its surface waves (Supplementary Figure S5). But at higher frequency range of 1 Hz, the Wangqing earthquake spectrum is larger. The corresponding dynamic stress for the 2017 UNE is ∼63 kPa, smaller than the 120 kPa for the Wangqing earthquake.
Long-Period and Harmonic-Spectra Events
We re-examine those HS events (Figure 9) identified and located by Ming et al. (2007) and use the clear HS events as templates to scan the continuous waveforms recorded by the single station CBS to detect more potential HSs. In contrast to previous observations (Ming et al., 2007), waveforms of HS events recorded at station CBS span the entire volcanic unrest period, resulting in a total of 125 HSs from 1999 to 2007. The most common feature of these HSs is that they all have several (>2) clear harmonic peaks in their spectral plots (Figures 9A,B).
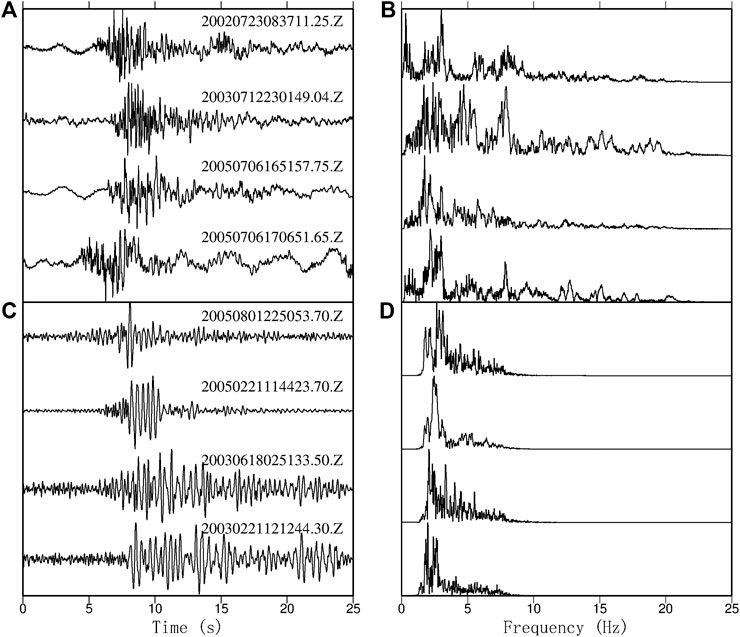
FIGURE 9. Some representative HS and LP events recorded by station CBS. All of these waveforms have been normalized before plotting. (A,B) Original waveforms and normalized spectral functions of vertical components, respectively, of HS events. (C,D) Original waveforms and normalized spectral functions of vertical components, respectively, of LP events. The numbers above the waveforms indicate the time of origin of the events.
By visually inspecting the waveforms originated by CHVO catalogue, we find that some waveforms show distinct low-frequency characteristics. We attribute these events with a dominant frequency of 2–3 Hz to LPs and put them into the templates. Eventually, 20 LPs have been detected in this study. Some of the representative LPs are shown in Figures 9C,D.
Discussion
Large earthquakes are capable of producing dynamic, static, and quasi-static stress changes (Freed and Andrew, 2005). Static stress change decays rapidly with distance, and becomes negligible beyond a few fault lengths (King et al., 1994; King and Deves, 2015). Given the large depth and horizontal distance between the Wangqing mainshock epicenter and Changbaishan volcano, the static stress change from the Wangqing mainshock does not seem the primary connecting agent. There are several physical models that connect the dynamic stresses disturbances from distant earthquakes with magma overpressure, thus triggering volcanic unrest/eruption and local seismicity. Most of them involve bubble excitation in idealized magmatic conditions but the physical processes are different. The rectified diffusion model involves volatiles in saturated fluids that are pumped into bubbles by dilatational phase of seismic waves from distant earthquakes (Sturtevant et al., 1996; Brodsky et al., 1998). In the advective overpressure model, bubbles are shaken from walls of the magma chamber (i.e., Pyle and Pyle, 1995; Hill and Prejean, 2015). In the sloshing model, the oscillatory motions of fluids in the magma chamber cause foam collapse and hence affect the presence and stability of bubbles (Namiki et al., 2016). Hill and Prejean (2015) also summarized a few additional models on bubble excitations by dynamic waves. In all these models, the ascending bubbles increase pressure in the shallow magma body and can cause volcanic unrest or eruption.
Brodsky and Prejean (2005) demonstrated that long-period (>30 s) waves generated by large distant earthquakes are more effective at triggering seismicity in Long Valley Caldera, California, and they proposed that fluid flow could play an important role as a low-pass filter and respond to long-period wave triggering. This is consistent with the recent observation of long-period surface waves triggering microseismicity in Changbaishan volcano (Liu et al., 2017). However, the triggering of the 2002–2005 volcanic unrest by the M 7.2 Wangqing deep-focus earthquake is quite different, both in terms of the triggering wave (relatively high-frequency body wave in the range of 0.4–2 Hz), and the triggered seismicity (delayed for 7 days and lasted for about 3 years). While we do not have additional evidence to distinguish between various models of bubble excitation due to dynamic shaking, our observations seem to suggest that this process is likely promoted by seismic waves in the relatively high-frequency range of 0.4 to 2 Hz, rather than at longer period. If we use the apparent lack of triggering due to the nearby UNE (Liu et al., 2017) as an additional constraint, then we could argue that the frequency range around 1 Hz is mostly relevant (see Figure 8).
Based on these arguments, we come up with the following scenario (Figure 10) to explain the apparent triggering between deep-focus earthquakes and volcanic unrest, as well as of different types of volcanic events. High-frequency body waves of the Wangqing deep-focus earthquake likely triggered some bubble growth and overpressure in the magma systems due to one of the aforementioned physical mechanisms. While it is not clear in which magma system had bubble growth, giving the apparent 7-days delay between the Wangqing mainshock and onset of volcanic unrest, we argue that it is likely that bubbles grew in the mid-crust magma system, resulting in overpressure and a small magma injection into the shallow magma chamber at a depth of ∼5 km (Figure 10B). This process readjusted the local stress state and triggered a delayed increase in VT seismicity at the top of the magma body (Figures 10B,C). The persistent appearance of VT events and the sporadic appearance of LP and HS events suggest transient and repetitive processes, respectively. The LP and HS events can be interpreted as resonances of the cracks filled by fluids above the magma system (Figures 10B,C). As mentioned before, the magmatic movement is supported by the independent observation of increasing volcanic gas emissions and ground deformations during the volcanic unrest period (Xu et al., 2012). However, because we did not have enough high-quality continuous recordings to relocate all the seismicity, we cannot provide additional evidence for magmatic or fluid movements such as spatio-temporal migration of aseismic slip and seismic swarms (e.g., Shelly et al., 2016).
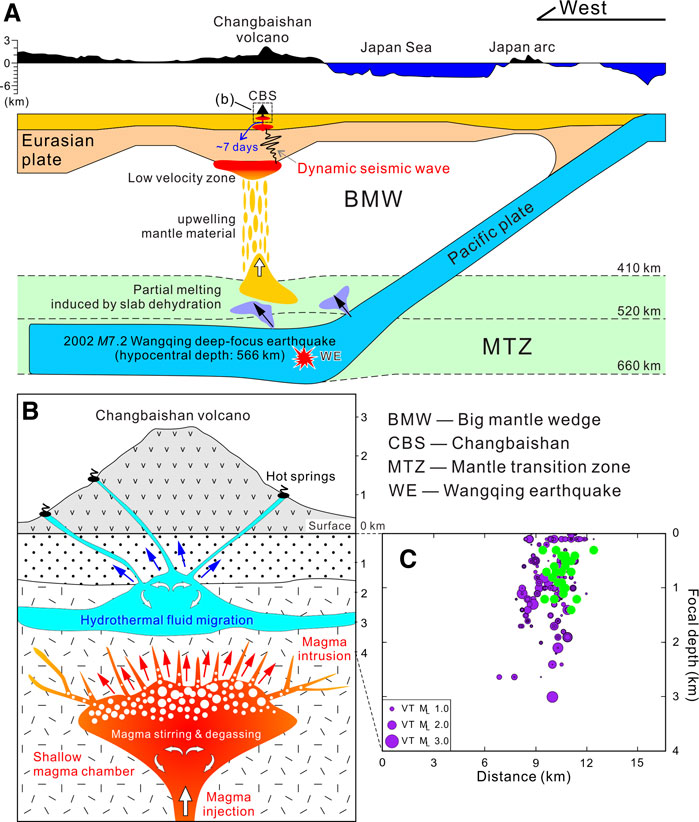
FIGURE 10. (A) A conceptual model showing magma migration from a deep source to a shallow reservoir of Changbaishan volcano in response to dynamic stress perturbations related to the Wangqing deep-focus earthquake in the stagnant Pacific Plate beneath NE Asia. An upwelling plume derived from the mantle transition zone is shown as the magma origin of Changbaishan volcano based on studies of seismic tomography (Zhao et al., 2009) and basalt geochemistry (Zhang et al., 2018). The 410, 520, and 660 km discontinuities are from Tian et al. (2016). (B) Hypothetical magmatic and hydrothermal processes corresponding to the 2002–2005 unrest of Changbaishan volcano. Injection of deep-sourced magmas into the shallow magma chamber led to anomalous magma stirring and degassing, which pressurized the shallow magma chamber and facilitated intrusion of gas-enriched magmas through cracks above the magma body. Hydrothermal fluid migration was influenced by crack intrusion of magmas. (C) A cross-sectional view of the study region along AB in Figure 1B. The green circles indicate HS events, and the purple circles with different scales indicate VT events with different magnitudes.
Hong et al. (2016) suggested that a hypothetical UNE in North Korea (about 130 km from Changbaishan volcano) with magnitude M = 7.0 can generate ∼67 kPa dynamic stress change on the volcano surface and ∼120 kPa in the magma chamber at depth, which are enough to disturb magma chambers and trigger volcanic eruptions. The dynamic stress of Wangqing earthquake is in the range of the hypothetical M 7 North Korea underground nuclear explosion. In addition, while the spectra of the 2017 mb = 6.0 UNE and the 2002 Wangqing mainshock do not completely overlap (Figure 8), it is expected that the spectrum of a hypothetical M = 7.0 UNE would be above that of the Wangqing mainshock at almost all frequency ranges. Hence, if other characteristics of the triggering waves (e.g., incident angles, back-azimuths, etc) do not play any important roles, we could argue that a magnitude 7 event (either nuclear explosion or deep-focus earthquake) nearby is capable of triggering an unrest or eruption at Changbaishan volcano.
Conclusion
In this study, we performed a systematic detection of seismic events around Changbaishan volcano to better understand the triggering relationship between the 2002 M 7.2 Wangqing deep-focus earthquake and the 2002–2005 unrest. We found clear evidence of LP events during the volcanic unrest process, and identified additional HS events. Thus, we identified three types of volcanic earthquakes (VT, LP and HS) during the unrest of Changbaishan volcano. The limited magnitudes of the VTs and small numbers of HSs and LPs suggest a small-scale magmatic process, likely indicating no major eruptive hazard in the foreseeable future.
With the new catalogue obtained by this study, we investigated the relationship between the 2002 Wangqing deep-focus earthquake and seismicity in Changbaishan volcano. The β-statistic value which has been calculated with a 5-days window at 7 days after the mainshock is as large as 5.1, suggesting a clear increase of seismicity at Changbaishan volcano. The onset of the seismic swarm and unrest on Changbaishan might have been dynamically triggered by the Wangqing earthquake with a delayed response of seven days. This apparent time delay between the deep-focus earthquake and the onset of swarm activity in Changbaishan volcano likely reflects the slow magmatic movements, which were accompanied by the 3-years earthquake swarm and volcanic unrest.
Data Availability Statement
The original contributions presented in the study are included in the article/Supplementary Material, and further inquiries can be directed to the corresponding author.
Author Contributions
All authors listed have made a substantial, direct, and intellectual contribution to the work and approved it for publication.
Funding
This study was funded by National Natural Science Foundation of China Grant 41572319 (GL), National Science Foundation Grants EAR-1543399 and EAR-1925965 (ZP, CL) and Jilin Changbaishan Volcano National Observation and Research Station (Project No. NORSCBS20-03).
Conflict of Interest
The authors declare that the research was conducted in the absence of any commercial or financial relationships that could be construed as a potential conflict of interest.
Supplementary Material
The Supplementary Material for this article can be found online at: https://www.frontiersin.org/articles/10.3389/feart.2020.599329/full#supplementary-material.
Acknowledgments
Seismic data utilized in this study were recorded by the Changbaishan Volcanic Observatory and can be requested from the Data Management Center of the China National Seismic network at the Institute of Geophysics, China Earthquake Administration (http://www.seisdmc.ac.cn/, in Chinese). We thank Zhikun Liu from the China University of Geoscience for comments on an early version of this paper. This manuscript benefits from useful comments by three anonymous reviewers.
References
Aron, A., and Hardebeck, J. L. (2009). Seismicity rate changes along the Central California coast due to stress changes from the 2003 M 6.5 San Simeon and 2004 M 6.0 Parkfield earthquakes. Bull. Seismol. Soc. Am. 99, 2280–2292. doi:10.1785/0120080239
Brodsky, E. E., and Prejean, S. G. (2005). New constrains on mechanisms of remotely triggered seismicity at Long Valley Caldera. J. Geophys. Res. 110, B04302. doi:10.1029/2004JB003211
Brodsky, E. E., Sturtevant, B., and Kanamori, H. (1998). Earthquakes, volcanoes, and rectified diffusion. J. Geophys. Res. 103 (B10), 23827–23838. doi:10.1029/98JB02130
Chouet, B. A. (1996). Long-period volcano seismicity: its source and use in eruption forecasting. Nature 380, 309–316.
Crews, J. B., and Cooper, C. A. (2014). Transient pore pressure response to confining stress excursions in Berea sandstone flooded with an aqueous solution of CO2. Water Resources Research. 50 (6), 4775–4786. doi:10.1002/2014WR015305
Farías, C., and Basualto, D. (2020). Reactivating and calming volcanoes: the 2015 MW 8.3 Illapel megathrust strike. Geophys. Res. Lett. 47 (16). doi:10.1029/2020GL087738
Freed, and Andrew, M. (2005). Earthquake triggering by static, dynamic, and postseismic stress transfer. Annual Review of Earth & Planetary Sciences, 33 (1), 335–365. doi:10.1146/annurev.earth.33.092203.122505
Gomberg, J., Reasenberg, P. A., Bodin, P., and Harris, R. A. (2001). Earthquake triggering by seismic waves following the Landers and Hector mine earthquakes. Nature 411, 462–466. doi:10.1038/35078053
Hakozaki, M., Miyake, F., Nakamura, T., Kimura, K., Masuda, K., and Okuno, M. (2018). Verification of the annual dating of the 10th Century Baitoushan volcano eruption based on an AD 774–775 radiocarbon spike. Radiocarbon. 60, 1–8. doi:10.1017/RDC.2017.75
Hammond, J. O. S., Wu, J.-P., Ri, K.-S., Wei, W., Yu, J.-N., and Oppenheimer, C. (2020). Distribution of partial melt beneath Changbaishan/Paektu volcano, China/Democratic People’s Republic of Korea. G-cubed. 21, e2019GC008461. doi:10.1029/2019GC008461
Hill, D. P., and Prejean, S. G. (2007). “Dynamic triggering in earthquake seismology,” in Treatise on Geophysics: earthquake seismology. Editor H. Kanamori (Amsterdam, Netherlands: Elsevier), 4, 257–291.
Hill, D. P., and Prejean, S. G. (2015). “Dynamic triggering,” in Treatise on geophysics: earthquake seismology. Editors H. Kanamori, and G. Schubert 2nd Edn (Oxford, United Kingdom: Elsevier), 4, 273–304.
Hill, D. P., Pollitz, F., and Newhall, C. (2002). Earthquake–volcano interactions. Phys. Today 55, 41–47. doi:10.1063/1.1535006
Hong, T., Choi, E., Park, S., and Shin, J. S. (2016). Prediction of ground motion and dynamic stress change in Baekdusan (Changbaishan) volcano caused by a North Korean nuclear explosion. Sci. Rep. 6, 21477. doi:10.1038/srep21477
Hough, S. E., Dollar, R. S., and Johnson, P. (2000). The 1998 earthquake sequence south of Long Valley Caldera, California: hints of magmatic involvement. Bull. Seismol. Soc. Am. 90, 752–763. doi:10.1785/0119990109
Iacovino, K., Ju-Song, K., Sisson, T., Lowenstern, J., Kuk-Hun, R., Jong-Nam, J., et al. (2016). Quantifying gas emissions from the “Millennium eruption” of Paektu volcano, democratic People’s Republic of Korea/China. Sci Adv. 2, e1600913. doi:10.1126/sciadv.1600913
Kim, S., Tkalčić, H., and Rhie, J. (2017). Seismic constraints on magma evolution beneath Mount Baekdu (Changbai) volcano from transdimensional Bayesian inversion of ambient noise data. J. Geophys. Res.: Solid Earth. 122, 5452–5473. doi:10.1002/2017JB014105
King, G. C. P., and Deves, M. H. (2015). Fault interaction, earthquake stress changes, and the evolution of seismicity. Treat. on Geophys. 4 (10), 243–271. doi:10.1016/B978-044452748-6.00069-9
King, G. C. P., Stein, R. S., and Lin, J. (1994). Static stress changes and the triggering of earthquakes. Bull. Seismol. Soc. Am. 84 (3), 935–953.
Kuritani, T., Ohtani, E., and Kimura, J.-I. (2011). Intensive hydration of the mantle transition zone beneath China caused by ancient slab stagnation. Nat. Geosci. 4, 713–716. doi:10.1038/ngeo1250
Lara, L. E., Naranjo, J. A., and Moreno, H. (2004). Rhyodacitic fissure eruption in Southern Andes (Cordón Caulle, 40.5°S) after the 1960 (Mw: 9.5) Chilean earthquake: a structural interpretation. J. Volcanol. Geoth. Res. 138, 127–138. doi:10.1016/j.jvolgeores.2004.06.009
Li, Y., Shi, Z., and Cao, X. (2012). The seiches and volcanic eruption triggered by the 6 October 1597 earthquake with M≥8 in the “Hunchun-Wangqing, Northeastern China, deep-focus seismic zone”. Acta Seismolo. Sinica. 34 (4), 557–570. doi:10.3969/j.issn.0253-3782.2012.04.012
Linde, A. T., and Sacks, I. S. (1998). Triggering of volcanic eruptions. Nature 395, 888–890. doi:10.1038/27650
Liu, R., Wei, H., and Li, J. (1998). The recent eruptions of Changbaishan Tianchi volcano. Beijing, China: Science Press.
Liu, G., Yang, J., Wang, L., and Sun, J. (2011a). Analysis of Tianchi volcano activity in Changbai Mountain, NE China. Glob. Geol. 14, 44–53. doi:10.3969/j.issn.1673-9736.2011.01.06
Liu, G., Sun, H., and Guo, F. (2011b). The newest monitoring information of Changbaishan volcano, NE China. Acta Petrol. Sin. 27, 2905–2911. CNKI:SUN:YSXB.0.2011-10-011
Liu, G., Li, C., Peng, Z., Li, X., and Wu, J. (2017). Detecting remotely triggered microseismicity around Changbaishan volcano following nuclear explosions in North Korea and large distant earthquakes around the world. Geophys. Res. Lett. 44, 4829–4838. doi:10.1002/2017gl072511
Lv, Z., Hong, H., and Wei, H. (2007). Research on the volcanic earthquake activity mechanism in Changbaishan Tianchi area. Seismol. Res. Northeast China 23, 40–48. doi:10.3969/j.issn.1674-8565.2007.01.007
Manga, M., and Brodsky, E. (2006). Seismic triggering of eruptions in the far field: volcanoes and geysers. Annu. Rev. Earth Planet Sci. 34, 263–291. doi:10.1146/annurev.earth.34.031405.125125
Manga, M., Fauria, K. E., Lin, C., Mitchell, S. J., Jones, M., Conway, C. E., Degruyter, W., Hosseini, B., Carey, R., and Cahalan, R. (2018). The pumice raft-forming 2012 havre submarine eruption was effusive. Earth & Planetary Science Letters, 489, 49–58. doi:10.1016/j.epsl.2018.02.025
Matthews, M. V., and Reasenberg, P. A. (1988). Statistical methods for investigating quiescence and other temporal seismicity patterns. Pure Appl. Geophys. 126, 357–372. doi:10.1007/BF00879003
McNutt, S. R. (2002). Volcano seismology and monitoring for eruptions. Int. Geophys. Ser. 81, 383–406.
Milluzzo, V., Cannata, A., Alparone, S., Gambino, S., Hellweb, M., Montalto, P., et al. (2010). Tornillos at Vulcano: clues to the dynamics of the hydrothermal system. J. Volcanol. Geoth. Res. 198, 377–393. doi:10.1016/j.jvolgeores.2010.09.022
Ming, Y., Wu, J., Su, W., Fang, L., and Wang, W. (2007). Harmonic-special event at Changbaishan Tianchi volcano. Seismol. Geol. 2, 492–501. [in Chinese with English abstract]. doi:10.3969/j.issn.0253-4967.2007.03.005
Namiki, A., Rivalta, E., Woith, H., and Walter, T. R. (2016). Sloshing of a bubbly magma reservoir as a mechanism of triggered eruptions. J. Volcanol. Geoth. Res. 320, 156–171. doi:10.1016/j.jvolgeores.2016.03.010
Oppenheimer, C., Wacker, L., Xu, J., Galván, J. D., and Büntgen, U. (2017). Multi-proxy dating the “Millennium Eruption”of changbaishan to late 946 CE. Quat. Sci. Rev. 158, 164–171. doi:10.1016/j.quascirev.2016.12.024
Peng, Z., and Gomberg, J. (2010). An integrative perspective of coupled seismic and aseismic slow slip phenomena. Nat. Geosci. 3, 599–607. doi:10.1038/ngeo940
Peng, Z., and Zhao, P. (2009). Migration of early aftershocks following the 2004 Parkfield earthquake. Nat. Geosci. 2, 877–881. doi:10.1038/ngeo697
Pyle, D. M., and Pyle, D. L. (1995). Bubble migration and the initiation of volcanic eruptions. J. Volcanol. Geoth. Res. 67 (4), 227–232. doi:10.1016/0377-0273(94)00111-S
Ri, K.-S., Hammond, J. O. S., Ko, C.-N., Kim, H., Yun, Y.-G., Pak, G.-J., et al. (2016). Evidence for partial melt in the crust beneath Mt. Paektu (Changbaishan), Democratic People’s Republic of Korea and China. Science Advances 2, e1501513. doi:10.17863/CAM.7009
Sawi, T. M., and Manga, M. (2018). Revisiting short-term earthquake triggered volcanism. Bulletin of Volcanology. 80: 57. doi:10.1007/s00445-018-1232-2
Shelly, D. R., Beroza, G. C., and Ide, S. (2007). Non-volcanic tremor and low-frequency earthquake swarms. Nature 446, 305–307. doi:10.1038/nature05666
Shelly, D. R., Ellsworth, W. L., and Hill, D. P. (2016). Fluid‐faulting evolution in high definition: connecting fault structure and frequency‐magnitude variations during the 2014 Long Valley Caldera, California, earthquake swarm. J. Geophys. Res.: Solid Earth. 121, 1776–1795. doi:10.1002/2015JB012719
Sturtevant, B., Kanamori, H., and Brodsky, E. E. (1996). Seismic triggering by rectified diffusion in geothermal systems. J. Geophys. Res. 1012 (B11), 25269–25282. doi:10.1029/96JB02654
Tang, J., Deng, Q., Zhao, G., Li, W., Xuan, F., Jin, G., et al. (2001). Electric conductivity and magma chamber at the Tianchi volcano area in Changbaishan Mountain. Seismol. Geol. 23, 191–200. [in Chinese with English abstract]. doi:10.3969/j.issn.0253-4967.2001.02.008
Tang, Y., Obayashi, M., Niu, F., Grand, S. P., Chen, Y. J., Kawakatsu, H., Tanaka, S., Ning, J., and Ni, J. F. (2014). Changbaishan volcanism in northeast china linked to subduction-induced mantle upwelling. Nature Geoscience, 7 (6), 470–475. doi:10.1038/ngeo2166
Tian, Y., Zhu, H., Zhao, D., Liu, C., Feng, X., Liu, T., et al. (2016). Mantle transition zone structure beneath the Changbai volcano: insight into deep slab dehydration and hot upwelling near the 410 km discontinuity. J. Geophys. Res. 121, 5794–5808. doi:10.1002/2016JB012959
Walter, T. R., and Amelung, F. (2006). Volcano–earthquake interaction at Mauna Loa volcano, Hawaii. J. Geophys. Res. 111, B05204. doi:10.1029/2005JB003861
Walter, T. R., and Amelung, F. (2007). Volcanic eruptions following M≥9 megathrust earthquakes: implications of the Sumatra-Andaman volcanos. Geology 35 (6), 539–542. doi:10.1130/G23429A.1
Wei, H., Liu, G., and Gill, J. (2013). Review of eruptive activity at Tianchi volcano, Changbaishan, northeast China: implications for possible future eruptions. Bull. Volcanol. 75, 706. doi:10.1007/s00445-013-0706-5
Wu, J., Ming, Y., and Zhang, H. (2005). Seismic activity at the Changbaishan Tianchi volcano in the summer of 2002. Chin. J. Geophys. 48, 621–628. [in Chinese with English abstract]. doi:10.1002/cjg2.701
Xu, J., Liu, G., Wu, J., Ming, Y., Wang, Q., Cui, D., et al. (2012). Recent unrest of Changbaishan volcano, northeast China: a precursor of a future eruption? Geophys. Res. Lett. 39, L16305. doi:10.1029/2012GL052600
Zhang, M., Guo, Z., Liu, J., Liu, G., Zhang, L., Lei, M., et al. (2018). The intraplate Changbaishan volcanic field (China/North Korea): a review on eruptive history, magma genesis, geodynamic significance, recent dynamics and potential hazards. Earth Sci. Rev. 187, 19–52. doi:10.1016/j.earscirev.2018.07.011
Zhao, D., and Tian, Y. (2013). Changbai intraplate volcanism and deep earthquakes in East Asia: a possible link? Geophys. J. Int. 195, 706–724. doi:10.1093/gji/ggt289
Zhang, M., and Wen, L. (2015). An effective method for small event detection: Match and locate (M&L). Geophys. J. Int. 200, 1523–1537. doi:10.1093/gji/ggu466
Keywords: Changbaishan volcano, volcanic tremor, deep-focus earthquake, matching template, long-period earthquake
Citation: Liu G, Li C, Peng Z, Liu Y, Zhang Y, Liu D, Zhang M and Pan B (2021) The 2002–2005 Changbaishan Volcanic Unrest Triggered by the 2002 M 7.2 Wangqing Deep Focus Earthquake. Front. Earth Sci. 8:599329. doi: 10.3389/feart.2020.599329
Received: 27 August 2020; Accepted: 08 December 2020;
Published: 09 February 2021.
Edited by:
Derek Keir, University of Southampton, United KingdomReviewed by:
Finnigan Illsley-Kemp, Victoria University of Wellington, New ZealandJames Oliver Scott Hammond, Birkbeck, University of London, United Kingdom
Diana Roman, Carnegie Institution for Science (CIS), United States
Copyright © 2021 Liu, Li, Peng, Liu, Zhang, Liu, Zhang and Pan. This is an open-access article distributed under the terms of the Creative Commons Attribution License (CC BY). The use, distribution or reproduction in other forums is permitted, provided the original author(s) and the copyright owner(s) are credited and that the original publication in this journal is cited, in accordance with accepted academic practice. No use, distribution or reproduction is permitted which does not comply with these terms.
*Correspondence: Chenyu Li, bGNoZW55dTE5OTJAZ21haWwuY29t