The True Identity of Putative Tooth Alveoli in a Cenozoic Crown Bird, the Gastornithid Omorhamphus
- 1Univ Lyon, UCBL, ENSL, UJM, CNRS, LGL-TPE, University of Lyon, Villeurbanne, France
- 2Department of Geology and Geophysics, Peabody Museum of Natural History, Yale University, New Haven, CT, United States
- 3Department of Earth Sciences, University of Cambridge, Cambridge, United Kingdom
All extant birds are toothless, and recent molecular evidence suggests that edentulism in extant birds is the product of a single evolutionary transition to toothlessness on the line to crown birds in the Cretaceous. However, a fossil crown bird premaxilla from the Palaeogene of North America (assigned to the gastornithid Omorhamphus storchii) has been interpreted as bearing alveoli for teeth, an observation that would cast doubt on a single loss of teeth preceding the extant avian radiation. However, the identity of these putative alveoli has never been reinvestigated in detail. Here, we re-examine this problematic juvenile specimen, using non-invasive x-ray microtomography, enabling the assessment of the true identity of the large, alveolus-like pits on the ventral side of this premaxilla. Although superficially alveolus-like, we illustrate that these pits represent openings of large neurovascular canals communicating with both the medullary cavity as well as other canals opening along the dorsal and lateral surfaces of the upper jaw, and that none of these openings appear to represent tooth alveoli. Further, we demonstrate that claims of an adult gastornithid specimen (Gastornis parisiensis) exhibiting tooth alveoli are similarly unfounded. By rejecting the hypothesis of dentition in these gastornithids, we eliminate any lingering uncertainty regarding the persistence of teeth within the avian crown group. We illustrate the presence of similar large vascular openings along the ventral surface of the beak of juvenile Gastornis russelli/parisiensis, and smaller versions in the juvenile premaxillae of Sylviornis neocaledoniae. We suggest that the large vascular canals in gastornithid specimens such as O. storchii are a feature associated with rapid growth of the juvenile beak, allowing the attainment of a large and dorsoventrally deep beak early in ontogeny. This may have enabled young gastornithids to become autonomous early, consistent with a presumably precocial developmental strategy.
Introduction
Virtually all known representatives of the avian crown group, extant and extinct, are edentulous, with estimates suggesting a single transition to toothlessness in the ancestors of crown birds between 116 and 88 Ma (Louchart and Viriot, 2011; Meredith et al., 2014; Brocklehurst and Field, 2021). The only hitherto unchallenged example of a crown bird exhibiting tooth alveoli is a juvenile jaw specimen from the early Eocene of Wyoming (Sinclair, 1928; Lambrecht, 1930). This enigmatic specimen, referred to Omorhamphus storchii, therefore has important potential to inform our understanding of the evolutionary dynamics of avian dentition. However, since its initial descriptions the identity of these pits has not been revisited. The juvenile premaxilla, which bears distinctive pits in the jaw tomia interpreted as tooth alveoli, is associated with several other skeletal elements, and the specimen was assigned to Gastornithidae (previously known as Diatrymidae; Sinclair, 1928; Lambrecht, 1930). Hence, these holes were purported evidence that at least juveniles of O. storchii possessed teeth (Lambrecht, 1930), an interpretation that has not been critically evaluated.
Tooth alveoli in Omorhamphus storchii would represent the only known evidence of teeth in a crown bird, and would challenge current interpretations of the evolutionary history of avian tooth reduction (Dumont et al., 2016; Brocklehurst and Field, 2021). However, the O. storchii specimen in question appears to be skeletally immature, which might suggest the presence of teeth only in juveniles, as is the case in the extant platypus (Ornithorhynchus anatinus), a monotreme mammal in which adults are toothless (Grant and Fanning, 2007), or the ceratosaurian theropod dinosaur Limusaurus, in which the dentition was reduced throughout ontogeny leading to a toothless adult (Wang et al., 2017). Given the striking degree of homoplasy in the pattern of tooth reduction along the avian stem lineage throughout the Mesozoic (e.g., Louchart and Viriot, 2011; Brocklehurst and Field, 2021), the possibility of such homoplasy extending into the avian crown group during the Cenozoic demands investigation. Here, we redescribe the fossil jaw element of O. storchii in question, which represents the rostral portion of the premaxilla. We use high-resolution X-ray microtomography (μCT) to non-destructively investigate the internal structure of the bone, and re-evaluate previous interpretations of this specimen, which suggested that the holes in the premaxillary tomia might represent (Sinclair, 1928), or surely did represent (Lambrecht, 1930), tooth alveoli. These arguments were based only on external shape similarity between some of the pits in the O. storchii tomia with the tooth alveoli of typical thecodont vertebrates, and their similar position along the tomia (Sinclair, 1928; Lambrecht, 1930). Therefore, our application of μCT scanning allows us to evaluate the internal morphology of this specimen for the first time.
Materials and Methods
YPM 13106, a rostral portion of the premaxilla of Omorhamphus storchii, from the Lower Gray Bull Formation, early Eocene, Bighorn Basin, Wyoming. The fragment is ca. 51 mm long rostrocaudally, 22 mm at its widest point from right lateral edge to left lateral edge, and 36 mm at its deepest point dorsoventrally. We conservatively apply the binomen Omorhamphus storchii throughout this manuscript, rather than accepting its proposed synonymization with Gastornis giganteus (Andors, 1992), since there is no unambiguous evidence that the associated juvenile bones referred to O. storchii represent the same species as G. giganteus, which is known only from adult specimens, with no known associations of the two taxa.
We also examined the external morphology of juvenile upper beaks attributed to a gastornithid (MNHN-R2583; Gastornis russelli/parisiensis; early Eocene, France), and a sylviornithid (MNHN-NCP-220, 242, 263 and 290; Sylviornis neocaledoniae; Holocene, New Caledonia), as well as adult beaks of Gastornis laurenti (MHNT.PAL.2013.15.1; early Eocene, France) and Sylviornis neocaledoniae (NCP-242 and a partial unnumbered specimen; Holocene, New Caledonia).
X-ray microtomography was performed at the University of Texas High-Resolution X-ray CT Facility. Three-dimensional reconstructions of the premaxilla were generated from 16-bit TIFF stacks from a scan of the complete fossil. Scan parameters were as follows: NSI scanner. Feinfocus source, high power, 200 kV, 0.14 mA, 1 aluminum filter, Perkin Elmer detector, 0.25 pF gain, 1 fps (999.911 ms integration time), no binning, no flip, source to object 218.58 mm, source to detector 1316.527 mm, continuous CT scan, no frames averaged, 0 skip frames, 3600 projections, 6 gain calibrations, 5 mm calibration phantom, data range [−10, 100] (grayscale range adjusted from NSI defaults), beam-hardening correction = 0.3. Voxel size = 0.0302 mm. Total slices = 1813. Then, three-dimensional reconstructions were generated from 16-bit stacks from a central region of the fossil, in order to reach better resolution parameters. Scan parameters were as follows: NSI scanner, Feinfocus source, high power, 210 kV, 0.12 mA, 1 aluminum filter, Perkin Elmer detector, 0.25 pF gain, 1 fps (999.911 ms integration time), no binning, no flip, source to object 133.754 mm, source to detector 1316.527 mm, continuous CT scan, no frames averaged, 0 skip frames, 5400 projections, 6 gain calibrations, 0.762 mm calibration phantom, data range [−10, 600] (grayscale range adjusted from NSI defaults), beam-hardening correction = 0.25, voxel size = 0.0109 mm, total slices = 1683.
Results
Examination of the μCT reconstruction and the actual Omorhamphus specimen reveals that the pits in the premaxillary tomia originally interpreted as tooth alveoli exhibit different shapes in ventral view (Figure 1). The more rostrally positioned pits on the ventral surface of the premaxilla are rostrocaudally elongated ellipses. This apparent elongation appears to be the product of canals with circular cross-sections contacting the ventral surface of the premaxilla obliquely, whereas more caudally positioned pits are nearly orthogonal to the palatal surface, resulting in circular openings. The pits are situated on the palatal surface of the premaxilla, just medial to the tomia, which are eroded. The four principal pits visible on the left side, from rostral-most to caudal-most, comprise (Figure 1C): (a) a large pit (2.5 mm in mediolateral diameter) with an oblong opening; (b) a smaller pit (2 mm mediolateral diameter) with a circular opening which is also the lateral-most pit; (c) a ∼2 mm mediolateral diameter pit, with a slightly elongated opening; and (d) a ∼2 mm mediolateral diameter pit, with a more rounded opening. The three visible pits on the right side, from rostral-most to caudal-most, comprise: (e) a large pit (2.5 mm mediolateral diameter) with the most rostrocaudally elongated opening (10 mm), with its associated internal canal positioned at the most acute angle to the palatal surface; (f) and (g) two larger pits (∼2.5 mm in mediolateral diameter) with rounder openings (the more caudally situated pit’s palatal opening is incomplete due to specimen damage). An additional large opening is also situated far more medially, slightly left of the midline of the palatal surface (h). All of the pits are obturated by sediment, except for the large, oblique pit on the right side (e).
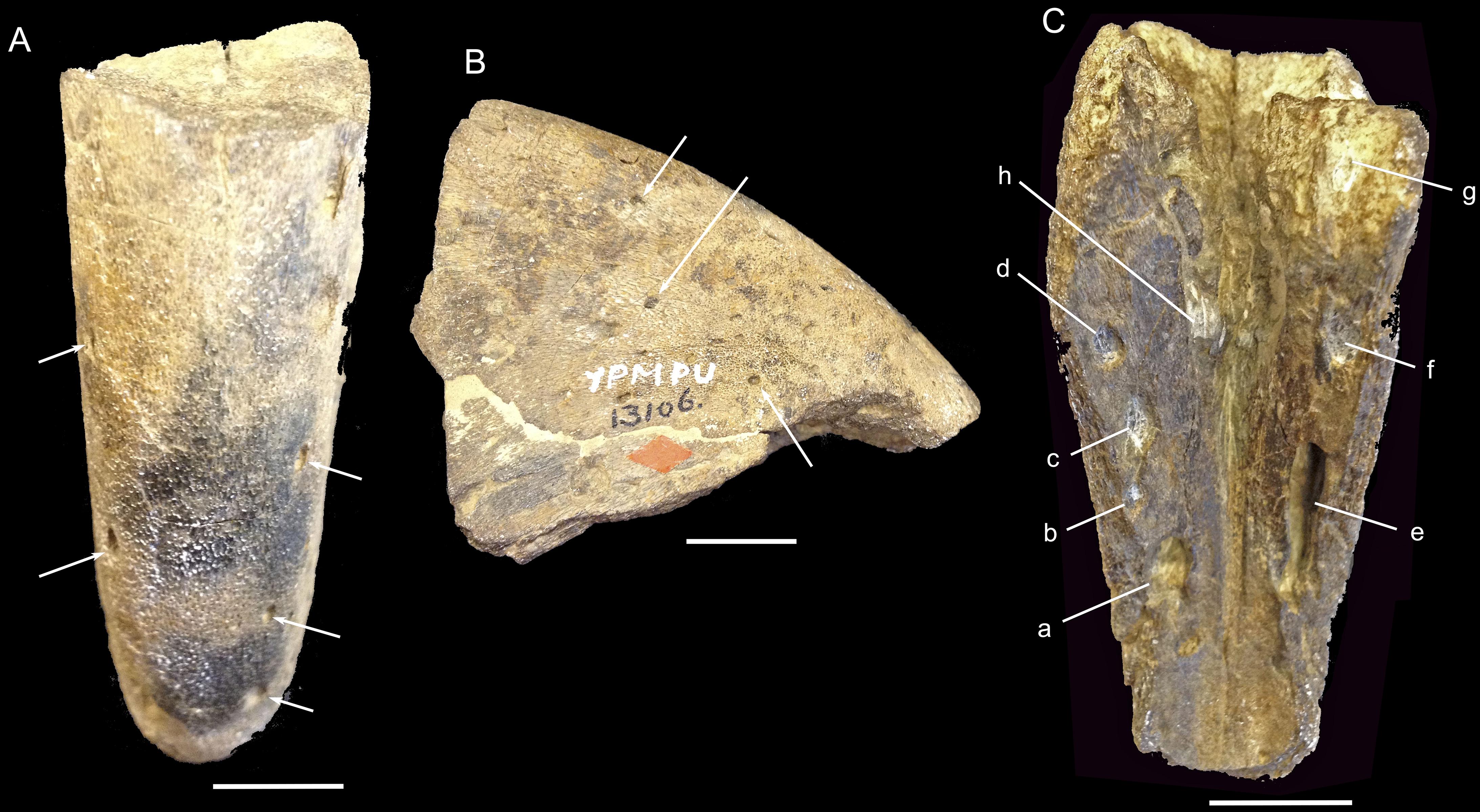
Figure 1. External views of Omorhamphus storchii YPM 13106 (rostral part of juvenile premaxilla). (A) Dorsal view. (B) Right lateral view. (a–g) Large openings visible in ventral view (C) on lateral sides of the palate just medial to the eroded tomia (a–d, left side; e–g, right side; h, median-left). The ventral pits are obturated by sediment, except the large oblique pit (labeled e). Several medium-sized foramina are visible (white arrows) on the dorsal and lateral sides (A,B). Scale bars, 10 mm.
X-ray μCT reveals that, despite the superficially alveolus-like appearance of some of these pits, all represent the openings of deep canals that communicate with other neurovascular canals and medullary cavities within the premaxilla (Figures 2, 3, Supplementary 3D File and Supplementary Figure 1). Among the vascular canals that communicate with the seven large pits studied here are medium-sized canals that open on the dorsal surface of the premaxilla, some dorsal openings being as large as the ventral “alveolus-like” pits (Figures 1A,B, Supplementary 3D File and Supplementary Figure 1). Based on digital examination of the internal structure of the bone, no visible differences in bone structure are apparent between the areas surrounding the seven canals and the rest of the jaw bone (Figure 4).
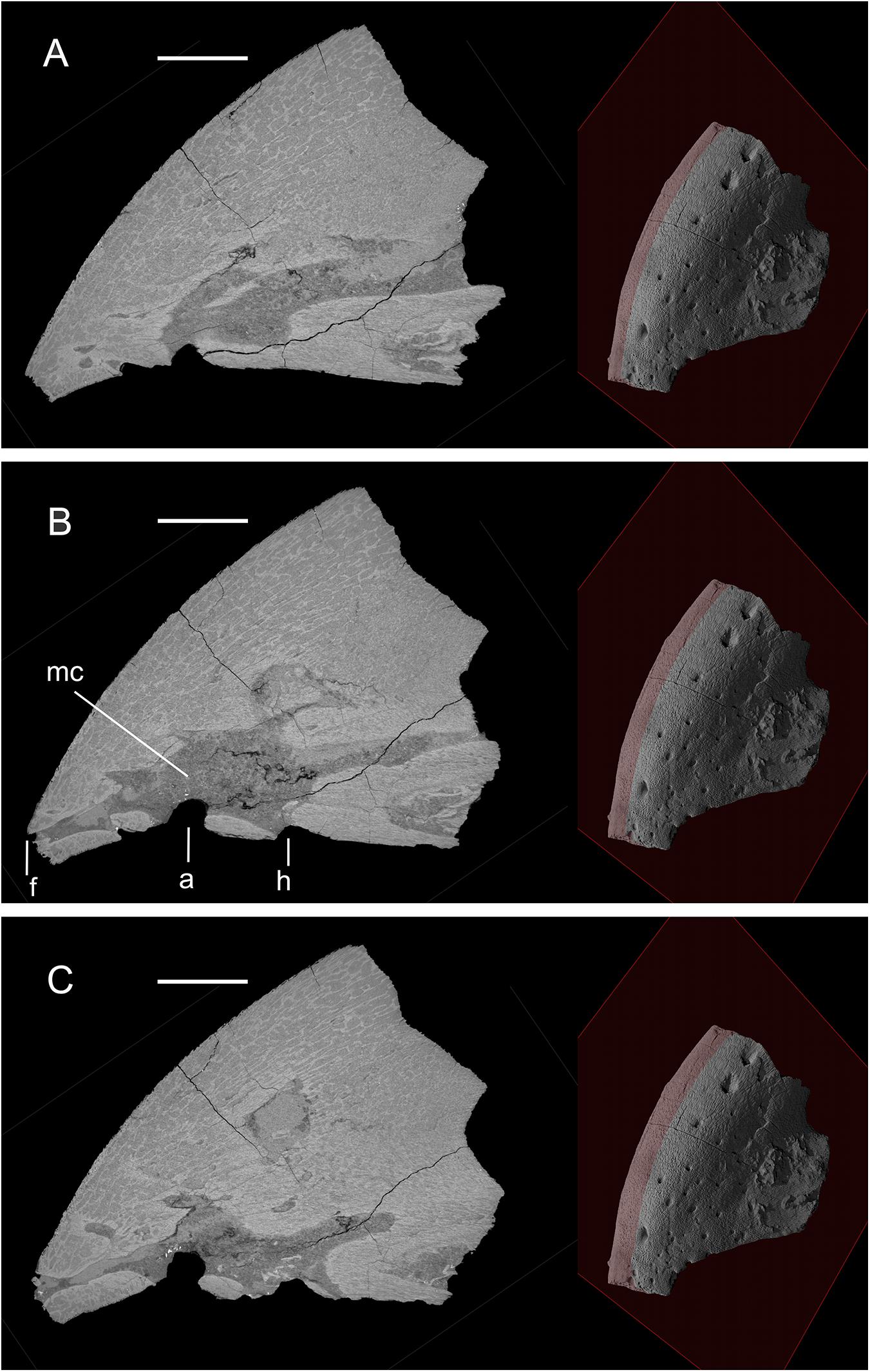
Figure 2. Three parasagittal sections through the premaxilla of YPM 13106, showing communications between canals and with the medullary cavity. Plane of parasagittal section moves toward the left from (A) through (B) and (C). The position of the plane is shown to the right of each section. Two of the large ventral pits (a, h) (see Figure 1) appear to communicate with the medullary cavity (mc), and a dorsal distal foramen (f). Scale bars, 10 mm.
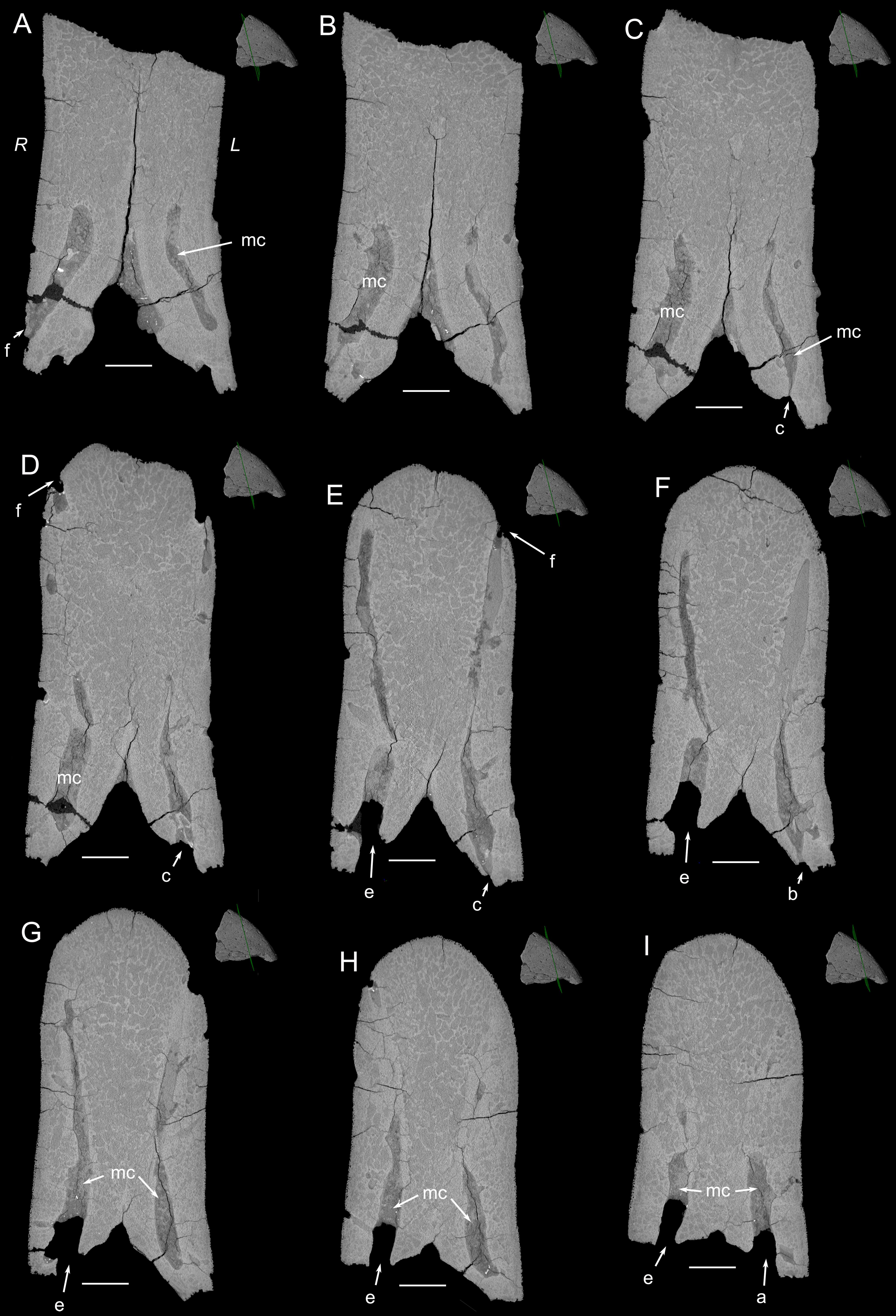
Figure 3. Nine parallel transverse sections through the premaxilla of YPM 13106. The planes of section are shown on the upper right corner of each virtual section. (A–I) The sections proceed from more caudal to more rostral. R, right side of a section. L, left side of a section. The succession of section planes (separated by ca. 2.2 mm) reveal connections between the large ventral openings (here a, b, c, e; see Figure 1), the medullary cavity (mc) and vascular canal openings, or foramina (f) to the dorsal and lateral sides of the premaxilla. Scale bars, 5 mm.
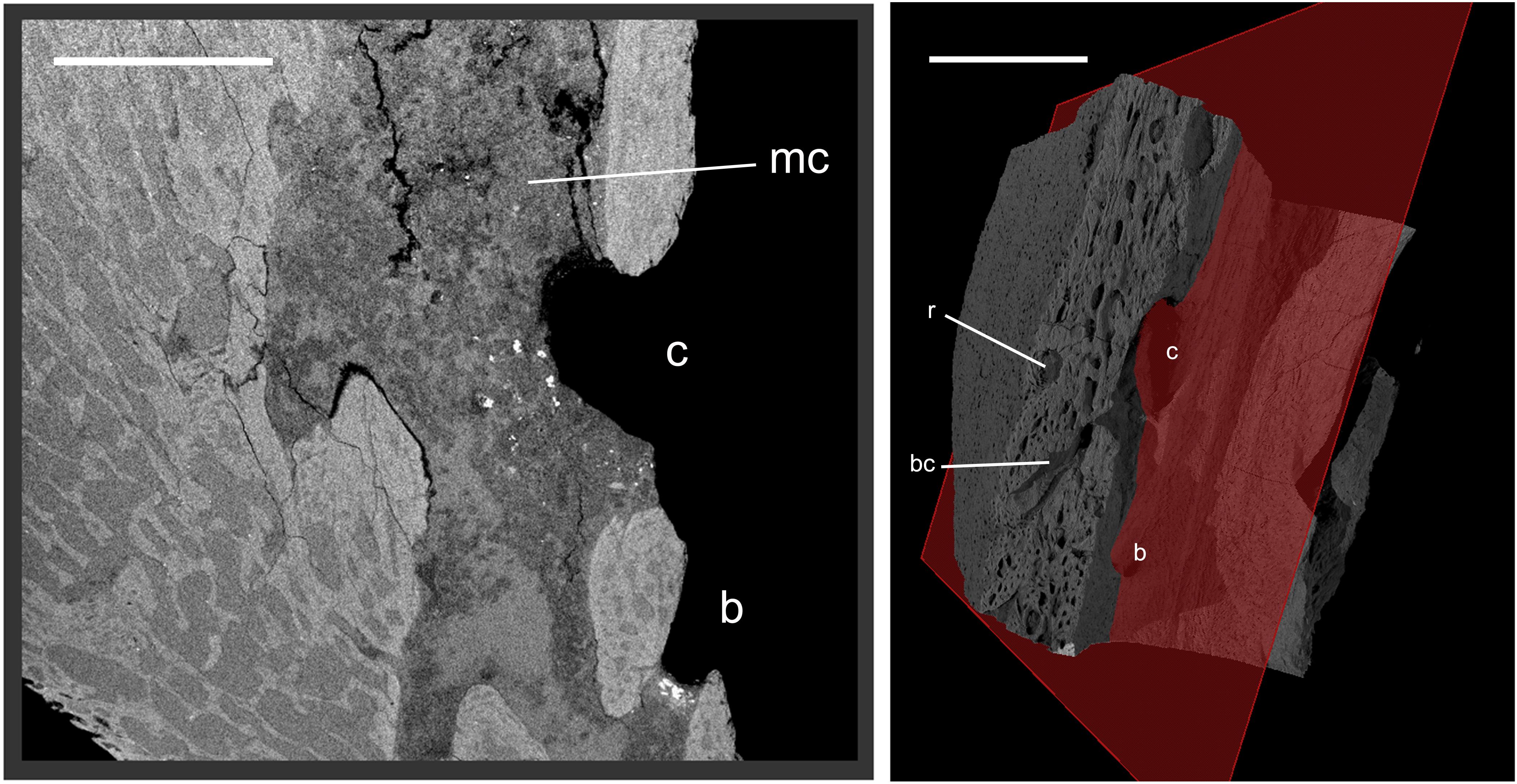
Figure 4. Closeup view in parasagittal section through the premaxilla of YPM 13106. The position of the plane of section is indicated on the right panel (ventro-lateral left view in volume). The regions surrounding the canals (and the cavity with which they communicate), including at openings on the palatal surface (b, c) (see Figure 1), show no difference in structure with the rest of the bone. The numerous smallest cavities visible in section and all over the surface (left side of specimen on right panel) are osteocyte lacunae. bc, branched canal; mc, medullary cavity; r, resorption pit corresponding to the site of a possible future secondary osteon. Scale bars, 5 mm.
Peripherally, the bone of the premaxilla comprises vascular canals with no apparent preferential orientation within the cortex, merging together and into a medulla toward the center of the premaxilla with a rather spongy structure composed of trabeculae. Elongated cavities lacking trabeculae are located near the tomial edges of the premaxilla, not far from the occlusal surface and oriented parallel to the tomia. The vascular canals, and cavities within the spongy part of the bone, communicate both with these elongated cavities and with the surface of the cortex (Supplementary 3D File and Supplementary Figure 1). The cortical bone structure is consistent with juvenile bone in an active growth phase, and as such the canals are likely to represent simple primary canals (and some possible primary osteons) (see Francillon-Vieillot et al., 1990; Starck and Chinsamy, 2002). The bone is highly vascularized, secondary osteons have not started to colonize the bone thickness, and no fundamental external system (lamellar or avascular bone with parallel fibers) exists at the periphery; instead, numerous osteocyte lacunae are visible on the surface (periphery) (Figure 4). All of these observations are consistent with a juvenile stage of premaxillary bone development, concordant with the highly “fibrous” external texture of the element.
Discussion
Our μCT data reveal that all seven of the more tomially positioned pits in the palatal surface of the premaxilla are neurovascular canals (not merely the rostralmost pit on the right side as suggested by Sinclair, 1928), with six of these canals now obturated by sediment. In addition, an eighth large pit positioned toward the midline of the palate is similar to the others, and appears to represent another vascular canal opening. The position of this opening far from the tomia adds evidence for the absence of any association between these pits and the purported presence of dentition. These vascular canals are large in diameter throughout their length, without abrupt constrictions, and communicate with the medullary cavities as well as smaller vascular canals within the bone. Tooth alveoli do communicate with neurovascular canals, but these are markedly narrower than the alveoli themselves (see, e.g., Ferreira-Cardoso et al., 2019). If these pits corresponded to tooth alveoli, the following structures would be expected to be observed surrounding the pits in birds (Dumont et al., 2016), or in non-avian archosaurs or mammals (LeBlanc et al., 2017; Funston et al., 2019): differentiated alveolar bone forming the internal wall of every cavity, traces of tooth buds of replacement teeth, and traces of Sharpey’s fibers. None of these features are discernible in the premaxilla of Omorhamphus. Moreover, alveoli (or remnants thereof) would also be expected to exhibit less irregular shapes and sizes than the pits observed in Omorhamphus. The absence of visible differentiation in structure between the bone surrounding the seven pits and the rest of the jaw bone is concordant with the identification of these structures as particularly large vascular canals. Finally, the location of these large canals differs from those of typical tooth alveoli, given that they are positioned with an irregular alignment mesial to the jaw tomia instead of regularly alongside the tomia. Hence, none of these pits can reasonably be interpreted as tooth alveoli, contrary to the heretofore unchallenged suggestion by Sinclair (1928) and assertion by Lambrecht (1930). The recognition that these putative gastornithid tooth alveoli have remained misidentified for nearly a century is reminiscent of the recent observation that bony ridges surrounding the lingual groove on the mandibles of caenagnathid theropods also do not represent vestiges of tooth-bearing structures (Funston et al., 2019).
Purported tooth alveoli in “Gastornis parisiensis” (formerly Gastornis edwardsii) were additionally noted by Lambrecht (1930). Sinclair (1928) had already mentioned that the beak margins of this species, of which all known specimens comprise adults described by Lemoine (1881), exhibit perforations similar to what he observed in O. storchii. But Lambrecht (1930) went further and was affirmative that the G. parisiensis premaxilla and mandible exhibit distinct alveoli for teeth along the tomia, based on figures from two plates in Lemoine (1881: plates 9, 10). On the other hand, concerning the premaxilla, Lemoine (1881) himself interpreted these holes as the openings of large vascular canals, and not as tooth alveoli. Since then, however, Martin (1992) demonstrated that the rostral and mandibular components concerned were non-avian in origin (and were instead probably attributable to turtles), along with numerous other elements in Lemoine’s reconstruction.
In his re-evaluation of Lemoine’s gastornithid description and reconstruction, Martin (1992) considered a tarsometatarsus to represent a new species, G. russelli. We examined the beak tentatively assigned to this species (specimen R2583, Reims collection) (Figures 5A,C). Supporting the suggestion by Bourdon et al. (2016), it appears to be attributable to a juvenile on the basis of the fibrous nature of its bone surface, which was not mentioned by Martin (1992). However, it appears to be more ontogenetically advanced than the beak of O. storchii, since the surface aspect is less fibrous and smoother than in the latter. In addition, the Omorhamphus beak, although fragmentary, is obviously smaller and less compressed laterally than R2583, in line with a markedly less advanced growth stage. The juvenile status of R2583 specimen may account for its relatively small beak, compared with known adult Gastornis beaks, suggesting that it could belong to a larger species such as G. parisiensis. Incidentally, although this was also the suggestion of Bourdon et al. (2016), recent recognition that the holotype tarsometatarsus of G. russelli is actually much larger than stated by Martin (1992) emphasizes the potential compatibility of the juvenile maxilla R2583 with G. russelli (Mourer-Chauviré and Bourdon, 2020). Mesial to the tomia of this juvenile Gastornis beak from Reims are several large vascular foramina (Figure 5C), similar to those of Omorhamphus storchii described above and found at similar locations along the jaw. The identical aspect and irregular size and position of the openings in R2583 indicate that they are of the same nature as those of O. storchii. The presence of very large vascular canals in juvenile beaks may therefore be constant in gastornithids, from both sides of the Atlantic, whereas it is undocumented in other birds.
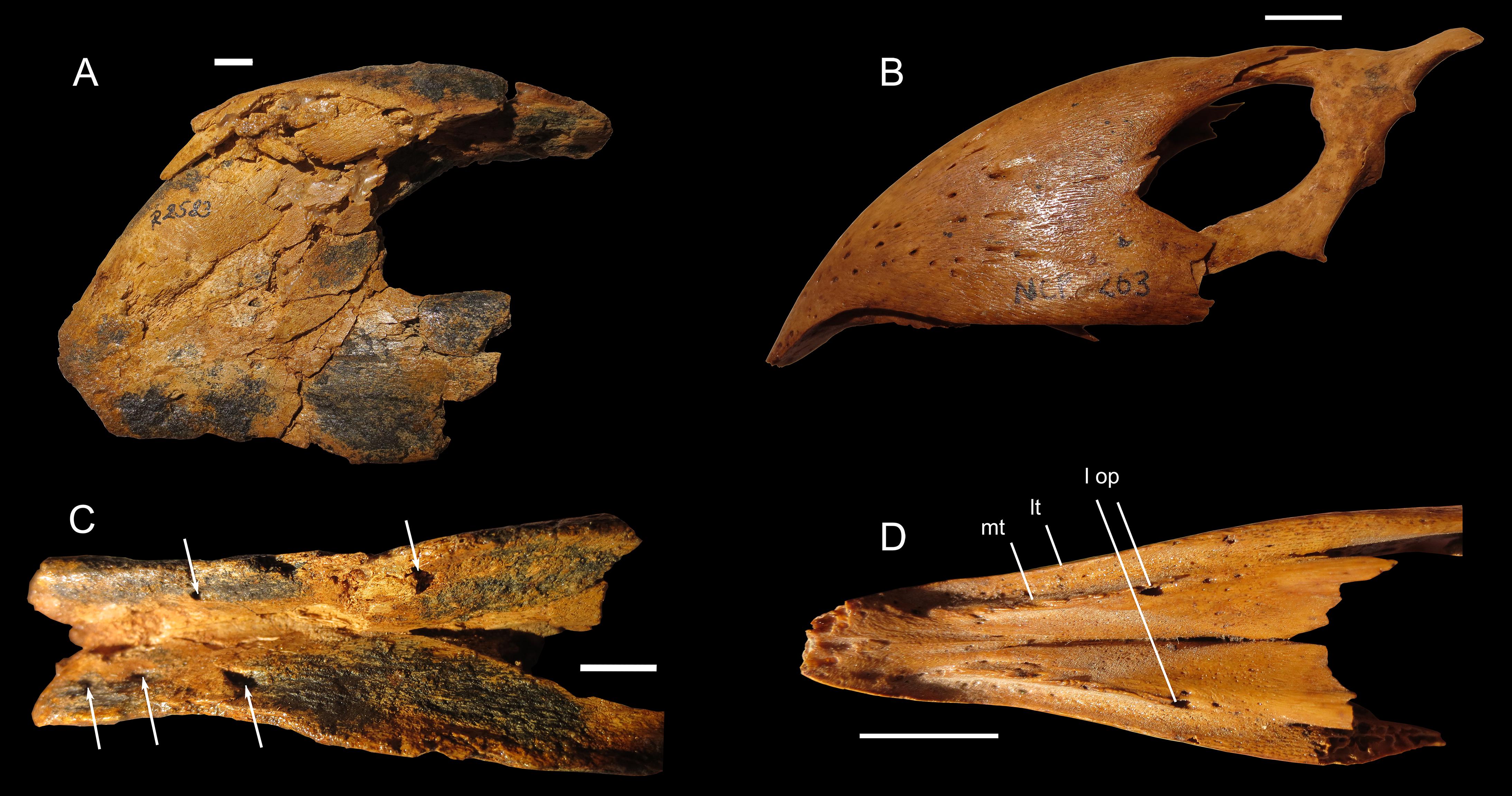
Figure 5. Juvenile upper beaks of Gastornis russelli/parisiensis R2583 (A,C) and Sylviornis neocaledoniae NCP-263 (B,D). (A,B) Left lateral views. (C,D) Ventral views. White arrows in (C) indicate large pits similar to those in Omorhamphus storchii, which are also vascular openings. In (D): lt, lateral tomial crest; mt, medial tomial crest; l op, large openings of vascular canals. Scale bars, 10 mm.
In sum, this work affirms that there is no evidence for the occurrence of teeth in any crown birds, living or extinct, and thus that avian dentition does not appear to have persisted into the Cenozoic. This supports the hypothesis of a single transition to neornithine toothlessness among the close ancestors of crown birds, pre-dating the Cretaceous-Paleogene transition as corroborated by the earliest-known probable crown bird fossils (Chatterjee, 2000; Louchart and Viriot, 2011; Meredith et al., 2014; Field et al., 2020; Brocklehurst and Field, 2021). An apparently reminiscent example is that of rather irregular large canals opening on the occlusal side of the mandibles of adult anteaters (Mammalia: Vermilingua), interpreted as evolutionary remnants of tooth alveoli in these edentulous mammals. These canals may be associated with a sensory role in conjunction with a mandibular keratinous pad (Ferreira-Cardoso et al., 2019). In such case, canals convey nerves as well as blood vessels, and are therefore truly neurovascular. Nevertheless, this example differs from that of gastornithids in that it is found in adult anteaters, whereas in gastornithids these pits are only evident in juveniles. Moreover, if these pits were associated with a sensory role in gastornithids, the restriction of these structures to juveniles would be puzzling, and without known extant analogs. Therefore, although we cannot entirely exclude the possibility that these canals housed nerves in addition to vasculature, we propose an alternative hypothesis whereby these large canals in juvenile gastornithid premaxillae were more explicitly associated with blood vessels.
Compared with non-gastornithid Neornithes, the juvenile gastornithid premaxillae examined here exhibit unusually large vascular canals on the sides of the palatal surface, both in terms of their absolute size and in relation to the dimensions of the beak. These may reasonably be explained by rapid growth of the extremely large beaks of gastornithids. Gastornithids were large, flightless birds, and have been interpreted as plant-eating relatives of Anseriformes or Galliformes (Andors, 1992; Angst et al., 2014; Worthy et al., 2017). These birds exhibited some of the largest-known beaks in avian evolutionary history, together with some phorusrhacids and dromornithids. Gastornithid beaks were relatively short, laterally compressed, and dorsoventrally deep, in some ways resembling much larger versions of the beak of the extant New Zealand Takahe (Porphyrio mantelli; Gruiformes: Rallidae) (Figure 6). Another extinct fossil galloanseran showing a deep, laterally compressed and curved beak is the large, flightless galliform Sylviornis neocaledoniae, of New Caledonia. Comparisons between Gastornis and Sylviornis are therefore interesting, and made possible by the existence of beaks deriving from juvenile specimens of Sylviornis. Most of the vascular foramina in juvenile Sylviornis beaks in dorsal view are small, becoming more densely spaced toward the rostral end, as in Omorhamphus. In palatal view, juvenile S. neocaledoniae exhibit smaller vascular openings than do O. storchii and juvenile Gastornis (Figure 5), both in terms of absolute size and relative to beak dimensions. Unlike O. storchii and Gastornis, however, S. neocaledoniae exhibits double bony tomia (lateral tomia and mesial tomia) in both juvenile and adult specimens (Mourer-Chauviré and Balouet, 2005). The largest vascular openings in juvenile specimens of Sylviornis are positioned at the caudal end of the mesial tomia (Figure 5D). The vascular openings of adult S. neocaledoniae beaks in palatal view are much reduced, and of comparable proportions to those of many extant birds with particularly large beaks (e.g., large macaws, storks, shoebill, large birds of prey, hornbills, and toucans; orig. obs.). The two largest foramina of juveniles are no longer visible in the beaks of adults. In juvenile S. neocaledoniae the vascular openings are of relatively small size, and the number of larger vascular openings is reduced compared with Gastornis sp. of similar ontogenetic stage (and the even younger material attributed to Omorhamphus storchii). This suggests reduced vascularization during beak growth in S. neocaledoniae relative to O. storchii and Gastornis, which may be related to the smaller absolute and relative size of the beak in adult Sylviornis.
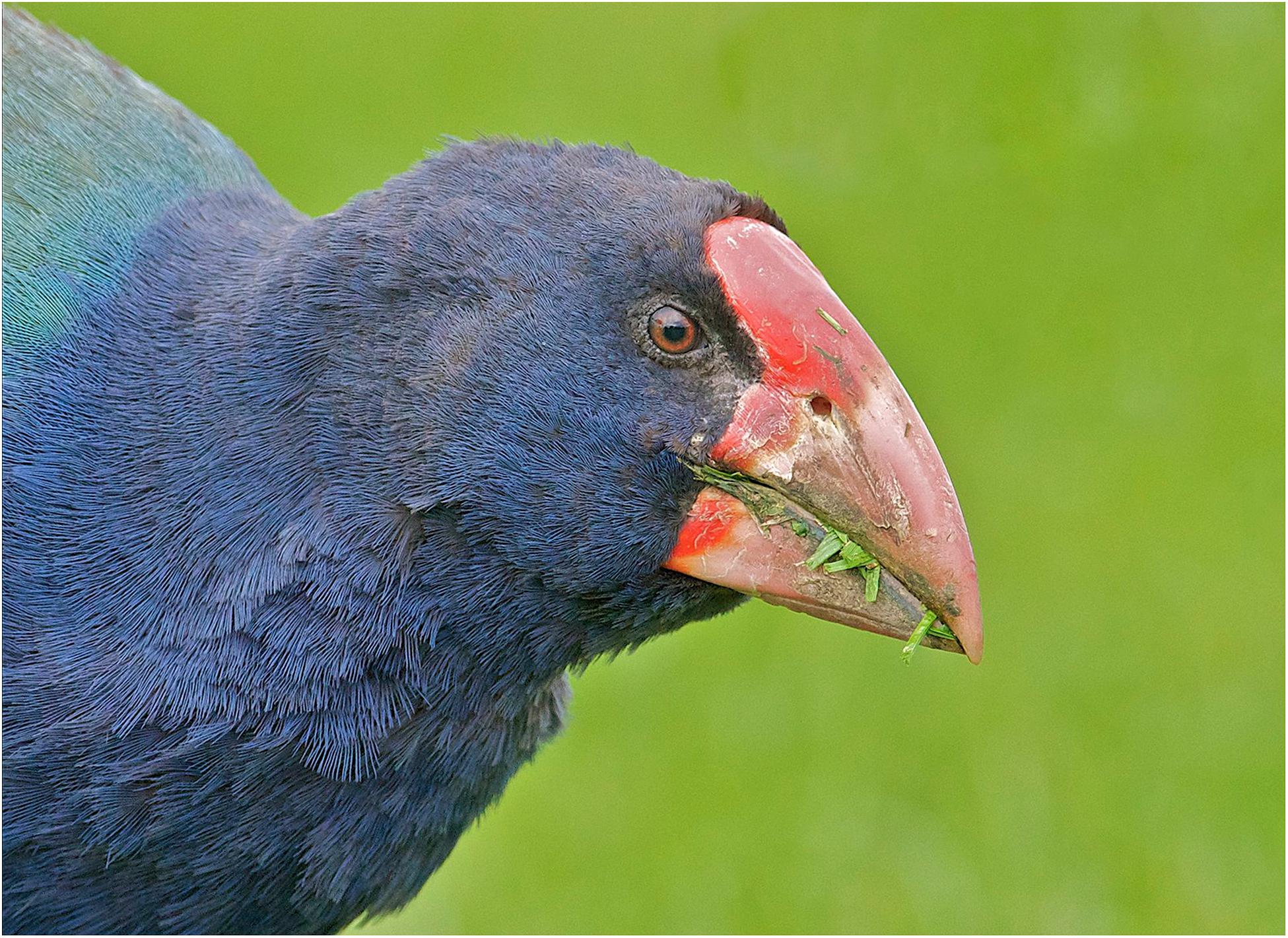
Figure 6. Closeup of the head of the extant New Zealand Takahe (Porphyrio mantelli). The general proportions of the bill are comparable to those of the vastly larger-bodied extinct neornithine clade Gastornithidae. Photo by DF.
A precocial developmental strategy and early behavioral autonomy can be hypothesized for gastornithids based on their hypothesized phylogenetic placement as stem group Anseriformes (Andors, 1992) or stem group Galliformes (see Worthy et al., 2017). Extant representatives of Anseriformes, and its extant sister clade Galliformes, exhibit developmental strategies ranging from precocial to superprecocial (the latter characterizing Megapodiidae, the sister clade to all other extant galliforms; Starck and Ricklefs, 1998; Botelho and Faunes, 2015; Prum et al., 2015). Hatchlings of extant precocial bird taxa encompass a range of feeding behaviors, including complete feeding autonomy, parental guidance, and short-term direct parental feeding (Starck and Ricklefs, 1998; Botelho and Faunes, 2015). Although presumably precocial, young gastornithids may not have been fully autonomous feeders, and a period of parental feeding assistance may have preceded the acquisition of full feeding autonomy, associated with the attainment of a mature beak. Among precocial birds the intermediate phase between hatching and full feeding autonomy tends to be short (Starck and Ricklefs, 1998). The large relative and absolute dimensions of adult gastornithid beaks (maximum depth of the premaxilla may reach 18 cm in adult Gastornis giganteus) may therefore have been attained fairly rapidly following hatching. This may have been achieved through rapid growth rates early in ontogeny, allowing adult-like, autonomous feeding in relatively young birds. Hence, the beaks of juvenile gastornithids may have required substantial vascularization in order to yield sufficient blood flux for rapid bone accretion. A general relationship between vascularization and growth rate exists (see Francillon-Vieillot et al., 1990; Starck and Chinsamy, 2002), which could suggest that the particularly large localized canals in O. storchii and R2583 (both juveniles) are related to rapid growth rates. In bird bones, the intensity of remodeling by Haversian bone tissue in adults is correlated with the intensity of blood flow and size of vascular canals (Allan et al., 2014). A similar relationship between intensity of primary bone accretion and the size of vascular canals during juvenile growth is therefore to be expected.
Upon reaching adult size, the larger vascular canals observed in juvenile beaks of gastornithids (and to a lesser extent in Sylviornis) presumably decreased in size with the obturation of primary osteons and simple canals through internal bone apposition, reducing their diameter and the dimensions of openings to the cortical surface. This mechanism is often observed in vertebrates (Francillon-Vieillot et al., 1990; Polig and Jee, 1990; Starck and Chinsamy, 2002) though data on bird beak growth series in general are scarce.
In adult beaks of Gastornis (G. giganteus, G. laurenti), rather small dorsal pits are still present in lateral view, but only toward the rostral end of the beak, while deep grooves are present over most of the dorsal surface of the beak (Bourdon et al., 2016); such grooves are absent in juvenile specimens (Omorhamphus and R2583). The latter grooves also characterize adult Sylviornis beaks (Mourer-Chauviré and Balouet, 2005) as opposed to juvenile ones (Figure 5B). The remnant pits on the rostral part of adult beaks can be compared with those visible in several extant birds, including those of some ratites which have been interpreted as being related to a sensory bill tip organ (Crole and Soley, 2017; du Toit et al., 2020). The deep grooves in adult Gastornis and Sylviornis are vascular grooves on the surface of the bone that have been interpreted as necessary to maintain and nourish a thick rhamphotheca (Mourer-Chauviré and Balouet, 2005) and their exclusive presence in adults presumably indicates that their formation took place after the cessation of primary bone growth. Therefore, it may be that a thickening of the beak rhamphotheca concomitantly occurred only after beak growth was completed. Beak rhamphotheca protects the underlying facial bones from damage, and is continuously replaced starting from its living base – becoming keratinized with growth toward the outer surface in order to prevent wear. The thickening of gastornithid beak rhamphotheca in adults may therefore have been related to a diet that incorporated hard or abrasive food items.
Data Availability Statement
The datasets presented in this study can be found in online repositories. The names of the repository/repositories and accession number(s) can be found below: The scan data for Omorhamphus storchii generated for this study, as well as three dimensional volumetric reconstructions, are deposited online and available on Zenodo (doi: 10.5281/zenodo.4670739).
Author Contributions
AL, B-AB, and DF designed the research. AL wrote the original manuscript and prepared figures with inputs from B-AB, SR, and DF. SR segmented the canal system. All authors conducted the analyses, collected the data, and reviewed and edited the manuscript.
Funding
DF acknowledges support from the UKRI Future Leaders Fellowship MR/S032177/1 and a Royal Society Research Grant (RGS/R2/192390).
Conflict of Interest
The authors declare that the research was conducted in the absence of any commercial or financial relationships that could be construed as a potential conflict of interest.
Acknowledgments
We thank Matthew Colbert for scanningthe specimen, Cécile Mourer-Chauviré for discussions and access to Gastornis material under study by herself and Estelle Bourdon, Ronan Allain, and Claire Sagne for access to Gastornis and Sylviornis material in the MNHN (Paris), comments on bone microstructure from Vivian de Buffrénil, and helpful comments from ARL, EP, and AHL, which improved the manuscript.
Supplementary Material
The Supplementary Material for this article can be found online at: https://www.frontiersin.org/articles/10.3389/feart.2021.661699/full#supplementary-material
References
Allan, G. H., Cassey, P., Snelling, E. P., Maloney, S. K., and Seymour, R. S. (2014). Blood flow for bone remodelling correlates with locomotion in living and extinct birds. J. Exp. Biol. 217, 2956–2962. doi: 10.1242/jeb.102889
Andors, A. V. (1992). Reappraisal of the Eocene groundbird Diatryma (Aves: Anserimorphae). Nat. Hist. Mus. Los Angeles Cty. Sci. Ser. 36, 109–125.
Angst, D., Lécuyer, C., Amiot, R., Buffetaut, E., Fourel, F., Martineau, F., et al. (2014). Isotopic and anatomical evidence of an herbivorous diet in the early Tertiary giant bird Gastornis, implications for the structure of Paleocene terrestrial ecosystems. Naturwissenschaften 101, 313–322. doi: 10.1007/s00114-014-1158-2
Botelho, J. F., and Faunes, M. (2015). The evolution of developmental modes in the new Avian phylogenetic tree. Evol. Dev. 17, 221–223. doi: 10.1111/ede.12126
Bourdon, E., Mourer-Chauviré, C., and Laurent, Y. (2016). Early Eocene birds from La Borie, southern France. Acta Palaeontol. Polon. 61, 175–190.
Brocklehurst, N., and Field, D. J. (2021). Macroevolutionary dynamics of dentition in Mesozoic birds reveal no long-term selection towards tooth loss. iScience 24, 102243. doi: 10.1016/j.isci.2021.102243
Chatterjee, S. (2000). “The morphology and systematics of Polarornis, a Cretaceous loon (Aves: Gaviidae) from Antarctica,” in Proceedings of the 5th Symposium of the Society of Avian Paleontology and Evolution, Vol. 1, eds Z. Zhou and F. Zhang (Beijing: Science Press), 125–155.
Crole, M. R., and Soley, J. T. (2017). Bony pits in the Ostrich (Struthio camelus) and Emu (Dromaius novaehollandiae) bill tip. Anat. Rec. 300, 1705–1715. doi: 10.1002/ar.23594
du Toit, C. J., Chinsamy, A., and Cunningham, S. J. (2020). Cretaceous origins of the vibrotactile bill-tip organ in birds. Proc. R. Soc. B 287, 20202322. doi: 10.1098/rspb.2020.2322
Dumont, M., Tafforeau, P., Bertin, T., Bhullar, B. A., Field, D., Schulp, A., et al. (2016). Synchrotron imaging of dentition provides insights into the biology of Hesperornis and Ichthyornis, the “last” toothed birds. BMC Evol. Biol. 16:178. doi: 10.1186/s12862-016-0753-6
Ferreira-Cardoso, S., Delsuc, F., and Hautier, L. (2019). Evolutionary tinkering of the mandibular canal linked to convergent regression of teeth in placental mammals. Curr. Biol. 29, 468–475. doi: 10.1016/j.cub.2018.12.023
Field, D. J., Benito, J., Chen, A., Jagt, J. W. M., and Ksepka, D. T. (2020). Late Cretaceous neornithine from Europe illuminates the origins of crown birds. Nature 579, 397–401. doi: 10.1038/s41586-020-2096-0
Francillon-Vieillot, H., de Buffrénil, V., Castanet, J., Géraudie, J., Meunier, F. J., Sire, J. Y., et al. (1990). “Microstructure and mineralization of vertebrate skeletal tissues,” in Skeletal Biomineralization: Patterns, Processes and Evolutionary Trends, Vol. 1, ed. J. G. Carter (New York, NY: Van Nostrand Reinhold), 471–530. doi: 10.1007/978-1-4899-5740-5_20
Funston, G. F., Wilkinson, R. D., Simon, D. J., LeBlanc, A. R. H., Wosik, M., and Currie, P. J. (2019). Histology of caenagnathid (Theropoda, Oviraptorosauria) dentaries and implications for development, ontogenetic edentulism, and taxonomy. Anat. Rec. 303, 918–934. doi: 10.1002/ar.24205
Lambrecht, K. (1930). Studien über fossile Riesenvögel. Geol. Hung. Ser. Palaeontol. 7, 1–37. doi: 10.1159/000397953
LeBlanc, A. R. H., Brink, K. S., Cullen, T. M., and Reisz, R. R. (2017). Evolutionary implications of tooth attachment versus tooth implantation: a case study using dinosaur, crocodilian, and mammal teeth. J. Vertebr. Paleontol. 37:e1354006. doi: 10.1080/02724634.2017.1354006
Lemoine, V. (1881). Recherches sur les Oiseaux Fossiles des Terrains Tertiaires Inférieurs des Environs de Reims, Part II. Reims: F. Keller, 75–170.
Louchart, A., and Viriot, L. (2011). From snout to beak: the loss of teeth in birds. Trends Ecol. Evol. 26, 663–673. doi: 10.1016/j.tree.2011.09.004
Martin, L. D. (1992). The status of the late Paleocene birds Gastornis and Remiornis. Nat. Hist. Mus. Los Angeles Cty. Sci. Ser. 36, 97–108.
Meredith, R. W., Zhang, G., Gilbert, M. T. P., Jarvis, E. D., and Springer, M. S. (2014). Evidence for a single loss of mineralized teeth in the common avian ancestor. Science 346, 1254390. doi: 10.1126/science.1254390
Mourer-Chauviré, C., and Balouet, J. C. (2005). Description of the skull of the genus Sylviornis Poplin, 1980 (Aves, Galliformes, Sylviornithidae new family), a giant extinct bird from the Holocene of New Caledonia. Monogr. Soc. Hist. Nat. Balears 12, 205–218.
Mourer-Chauviré, C., and Bourdon, E. (2020). Description of a new species of Gastornis (Aves, Gastornithiformes) from the Early Eocene of La Borie, Southwestern France. Geobios 63, 39–46. doi: 10.1016/j.geobios.2020.10.002
Polig, E., and Jee, W. S. S. (1990). A model of osteon closure in cortical bone. Calcif. Tissue Int. 47, 261–269. doi: 10.1007/bf02555907
Prum, R. O., Berv, J. S., Dornburg, A., Field, D. J., Townsend, J. P., Lemmon, E. M., et al. (2015). A comprehensive phylogeny of birds (Aves) using targeted next generation DNA sequencing. Nature 526, 569–573. doi: 10.1038/nature15697
Sinclair, W. J. (1928). Omorhamphus, a new flightless bird from the lower Eocene of Wyoming. Proc. Amer. Phil. Soc. 67, 51–65.
Starck, J. M., and Chinsamy, A. (2002). Bone microstructure and developmental plasticity in birds and other dinosaurs. J. Morphol. 254, 232–246. doi: 10.1002/jmor.10029
Starck, J. M., and Ricklefs, R. E. (1998). “Patterns of development: the altricial-precocial spectrum,” in Avian Growth and Development: Evolution within the Altricial-Precocial Spectrum, eds J. M. Starck and R. E. Ricklefs (Oxford: Oxford University Press), 3–30. doi: 10.1002/zoo.1430110103
Wang, S., Stiegler, J., Amiot, R., Wang, X., Du, G. H., Clark, J. M., et al. (2017). Extreme ontogenetic changes in a ceratosaurian theropod. Curr. Biol. 27, 144–148. doi: 10.1016/j.cub.2016.10.043
Keywords: Aves, beak, bone, Gastornithidae, Neornithes, ontogeny, Sylviornis, x-ray microtomography
Citation: Louchart A, Bhullar B-A, Riamon S and Field DJ (2021) The True Identity of Putative Tooth Alveoli in a Cenozoic Crown Bird, the Gastornithid Omorhamphus. Front. Earth Sci. 9:661699. doi: 10.3389/feart.2021.661699
Received: 31 January 2021; Accepted: 16 April 2021;
Published: 13 May 2021.
Edited by:
Fabien Knoll, Fundacion Agencia Aragonesa para la Investigacion y el Desarrollo, SpainReviewed by:
Aaron R. H. LeBlanc, University of Alberta, CanadaAndrew H. Lee, Midwestern University, United States
Edina Prondvai, University of Birmingham, United Kingdom
Copyright © 2021 Louchart, Bhullar, Riamon and Field. This is an open-access article distributed under the terms of the Creative Commons Attribution License (CC BY). The use, distribution or reproduction in other forums is permitted, provided the original author(s) and the copyright owner(s) are credited and that the original publication in this journal is cited, in accordance with accepted academic practice. No use, distribution or reproduction is permitted which does not comply with these terms.
*Correspondence: Antoine Louchart, antoine.louchart@ens-lyon.fr; Daniel J. Field, djf70@cam.ac.uk