- 1Physical Science and Engineering Division, King Abdullah University of Science and Technology (KAUST), Thuwal, Saudi Arabia
- 2Department of Earth Sciences, University of Geneva, Geneva, Switzerland
- 3School of Ocean and Earth Science, University of Southampton, Southampton, United Kingdom
- 4International Seismological Centre, Thatcham, United Kingdom
- 5Department of Earth Sciences, Eritrea Institute of Technology, Asmara, Eritrea
Earthquake swarms occur sporadically at divergent plate boundaries but their recurrence over multiple decades and relation to magmatic spreading activity remain poorly understood. Here we study more than 100 earthquake swarms over a 60-year period in the southern Red Sea, Afar, and Gulf of Aden region. We first compiled an earthquake-swarm catalogue by integrating reexamined global and local earthquake catalogues from 1960 to 2017. This yielded 134 earthquake swarms that mainly cluster in 19 different areas in the study region, showing that in most cases swarms recur every few decades in the same area. The swarms exhibit a range of earthquake magnitudes and often include multiple M3 to M5 events with some swarms having occasional larger earthquakes over M6, primarily in southern Afar. Many of the earthquake swarms were clearly associated with rifting events, consisting of magmatic intrusions, surface faulting, and in some cases volcanic eruptions. Together, the swarms suggest that extension at these divergent plate boundaries occurs episodically along <100 km long segments, some of which were previously unrecognized. Within the study region, the Gulf of Aden shows the most frequent swarm activity, followed by Afar and then the southern Red Sea. The results show that the three areas were subject to an increase of earthquake-swarm activity from 2003 to 2013 in the form of three rifting episodes and at least seven volcanic eruptions. We interpret that the most likely controls on temporal variations in earthquake swarm activity are either temporal variations in magma supply, or rifting-induced stress change that trigger clusters of swarms.
Introduction
Earthquake swarms are observed worldwide at divergent plate boundaries. Most of these swarms occur along oceanic ridges and along transform zones (Bird et al., 2002; Boettcher and Jordan, 2004; Roland and McGuire, 2009; Passarelli et al., 2018b). Earthquake swarms are seismic sequences that cluster in space and time with the majority of moment release occurring after the start of the sequence (Roland and McGuire, 2009; Chen and Shearer, 2011). Seismicity rate during swarm-like sequences fluctuates in time with acceleration and deceleration phases that cannot be described with the simple Omori law (e.g., Sykes, 1970; Dziak et al., 2006). They often lack a distinctive mainshock and most of the seismic moment is released through multiple earthquakes of comparable magnitude. They have characteristic durations of days to months that do not scale with the total moment released during the sequence (Passarelli et al., 2018b), which indicates that their temporal evolution is likely modulated by transient processes (e.g., fluid migration, magmatic intrusions, or aseismic slip) acting on top of the long-term tectonic load (Vidale and Shearer, 2006; Passarelli et al., 2015, 2018b). Therefore, no governing law has yet been discovered for swarm seismicity which makes it difficult to use their physical and statistical characteristics for their detection and to understand their spatial and temporal evolution. However, a thorough investigation of the patterns and evolution of the earthquake activity can give insight into both the driving mechanism and seismo-tectonic conditions leading to earthquake swarms in both tectonic and volcanic regions. Here we focus on earthquake swarms at divergent plate boundaries, which often occur contiguously with tectonic and magmatic activity.
Earthquake swarms at divergent plate boundaries have been identified in global catalogues, e.g., in the Atlantic Ocean, in some cases related to magmatic intrusions along spreading ridges (Sykes, 1970; Bergman and Solomon, 1990), at the Gakkel ridge (Arctic Ocean) with the emplacement of a new lava field (Edwards et al., 2001) and at the Indian Ocean ridge (Läderach et al., 2012; Schlindwein, 2012). Local seismic data (derived from hydrophone instruments) in the northeast Pacific ocean (Juan De Fuca, Axial Seamount, Gorda Ridge and East Pacific Rise) show earthquake swarms associated with magmatic intrusions, with detailed studies of magma propagation direction and speed (Dziak et al., 2006; Dziak et al., 2007; Dziak et al. 2011). Such sequences have been repeatedly observed inland along rift zones in Iceland (e.g., Ágústsdóttir et al., 2016; Einarsson and Brandsdottir, 2021) and also at the Kilauea volcano in Hawaii during lateral magma propagation along rift zones (e.g., Neal et al., 2019). Tectonic earthquake swarms have also been described north of Iceland, within a transform fault zone (Passarelli et al., 2018b). As of yet, global catalogues have not been extensively exploited to identify earthquake swarms, despite the significant increase of seismic instrument deployments and of available seismic data during the last 30 years, e.g., in the International Seismological Centre, 2010 (ISC, www.isc.ac.uk) catalogue that integrates local seismic data from contributing agencies all over the world.
The southern Red Sea, Afar, and Gulf of Aden (SAGA) region has been continuously affected by earthquake swarms at least since early earthquake location studies began in the 1950s (Fairhead and Girdler, 1970; Gouin, 1979 and references therein) and is thus an ideal region to study earthquake swarm activity over several decades. In the Afar region (Ethiopia; Figure 1A), several major earthquake sequences have occurred, such as at Serdo (1969; Abdallah et al., 1979; Kebede et al., 1969), Asal Ghoubbet (1979; Abbate et al., 1995), Dobi (Jacques et al., 1989; Jacques et al., 1996, 2011), Dabbahu (2005–2010; Wright et al., 2006; Ayele et al., 2005; Ebinger et al., 2008; Grandin et al., 2005; Hamling et al., 2010; Barnie et al., 2016), Dallol (2004; Nobile et al., 2012) and during the Nabro eruption in 2010 (Hamlyn et al., 2014; Goitom et al., 2015). In addition to these earthquake swarms in Afar, notable swarms have occurred in the southern Red Sea in the Zubair Archipelago (Jónsson and Xu, 2015; Xu et al., 2015; Eyles et al., 2018) and in the Gulf of Aden (2010–2011; Leroy et al., 2012; Shuler and Nettles, 2012; Ahmed et al., 2016; Figure 1A).
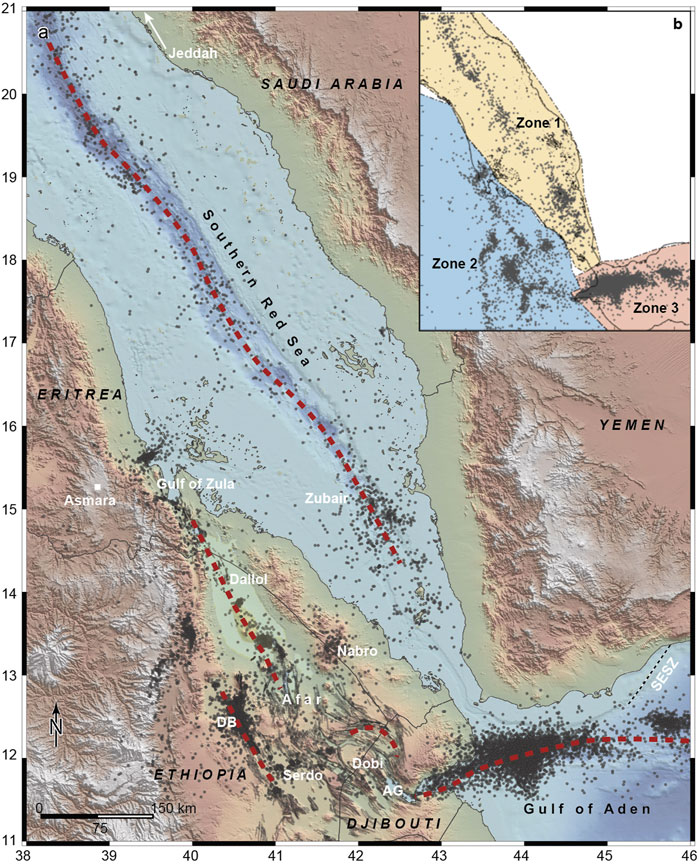
FIGURE 1. Map of the southern Red Sea, Afar, and Gulf of Aden (SAGA) region. (A) Earthquake locations from the ISC catalogue are shown as black dots, country borders as thin black lines and plate boundaries in red dashed lines. (B) The earthquake swarm analysis was carried out separately for three different zones (zone 1: southern Red Sea, zone 2: Afar, and zone 3: Gulf of Aden).
Relating seismicity and earthquake swarms to magma intrusions can be difficult (Peterson et al., 2020), especially for swarms occurring offshore (e.g., Passarelli et al., 2018a) and for older inland swarms from the time before regular geodetic observations (i.e., prior to the 1990s, which corresponds to the beginning of InSAR and GPS). However, most inland swarms that have occurred in the SAGA region in the past 30 years have shown unambiguous relationship with magmatic intrusions. Moreover, the presence of numerous seafloor volcanic vents, in areas where offshore swarms have occurred, shows that multiple magmatic intrusions must have taken place in the past. In this paper, we compile the first regional appraisal of the earthquake swarms and related volcanic activity for the time period from 1960 to 2020. Integrating our results with historical records back to the early 19th century (1838), based on the book of Gouin (1979), provides an overview of earthquake swarms and volcanic activity in the region for the last 180 years. We also discuss temporal variations in magma supply to the SAGA region, which is one of the most volcanically and seismically active divergent plate boundaries on Earth (Hofstetter and Beyth, 2003). This study also aims to better understand long-term, multi-decadal behavior of divergent plate boundaries and to identify areas prone to recurrent strain accommodation during rifting cycles, which is of significant importance for seismic and volcanic hazard assessments in the region.
Earthquake Swarm Detection
We used the ISC (International Seismological Center) Bulletin (2020) to extract a catalogue of earthquakes for the SAGA region and to identify and analyze earthquake swarms. The ISC bulletin is assembled from reported hypocenters and associated parametric data (station arrival times, amplitude, magnitudes, moment tensors, etc.) from over 150 contributors around the world [for details, see e.g., Section 3 of International Seismological Centre (2020)]. Within about 24–30 months, ISC analysts review monthly batches of reports and the data are revised (in a broad sense, e.g., phases, hypocenters, magnitudes). During this stage, some earthquakes may be banished, merged, or split into more events. The largest earthquakes (usually of magnitude above 3.5, and some smaller ones that are reported by multiple agencies) are relocated by the ISC using the algorithm by Bondár and Storchak (2011). All phases with a valid travel-time prediction in the 1D ak135 model (Kennett et al., 1995) are used in the location determination, along with elevation, ellipticity (Kennett and Gudmundsson, 1996; Engdah et al., 1998), and depth-phase bounce point corrections (Engdah et al., 1998). Body- and surface-wave magnitudes are also re-computed. As such, the ISC Bulletin contains both locations and magnitudes from contributing agencies (at local and global scales) beside the ISC’s own re-computations (if an earthquake is relocated). While not manually reviewed by the ISC, small earthquakes, which are normally reported by only one contributor, still undergo automatic checks to prevent (given the information available) faulty or inconsistent solutions to be released in the ISC Bulletin.
The earthquake catalogue we extracted for the SAGA region consists of over 15,200 earthquakes from 1960 to 2017. The earthquake locations are a mixture of solutions from contributing agencies and the ISC. The largest provider is UREES (http://www.isc.ac.uk/cgi-bin/agency-get?agency=UREES), with earthquake bulletins from Ebinger et al. (2008), Keir et al. (2009), and Belachew et al. (2011), followed by DHMR (http://www.isc.ac.uk/cgi-bin/agency-get?agency=DHMR), the ISC, and other agencies (Supplementary Figure S1). The earthquake magnitude (if any) is listed following the criteria by Di Giacomo and Storchak (2016). The catalogue is limited to earthquakes of magnitude 4 and above from 1960 until the 1990s, as only earthquakes recorded teleseismically were reported. However, in recent decades, the detection of smaller earthquakes (down to magnitude 2) improved thanks to better national seismic networks as well as temporary network deployments in the SAGA region (Supplementary Figures S2, S3). Reported earthquake locations extracted from the ISC Bulletin are usually constrained by data from more than five seismic stations (Supplementary Figures S4A,B) and the location uncertainty is within 30 km for about 86% of the events in the extracted catalogue (Supplementary Figures S3C,D). While the extracted catalogue is inhomogeneous in both space and time and almost half of the earthquakes (∼7,400) are without a determined magnitude, making statistical analysis of the catalogue challenging, this catalogue compilation still resulted in the most extensive and updated information on the seismicity in the SAGA region to date.
In order to efficiently detect swarms, we developed a two-step methodology based on the earthquake occurrence rate and spatial location. In the first step we identified every short time window during which the earthquake rate was higher than the background rate over a broader region. In the second step, for each selected time window, we then marked the location where the seismicity clustered in space through a density-based clustering algorithm (see details below). We divided the ∼1,000 km × 1,200 km large SAGA region into three zones (Figures 1A,B), based on key tectonic features, and applied this swarm detection methodology separately to the seismicity of the three zones, i.e., the southern Red Sea, Afar and Gulf of Aden. Zone 1 corresponds to the southern Red Sea, from 21°N in the northwest to Bab-el-Mandeb (11°N) in the southeast, zone 2 broadly corresponds to the Afar region (from Massawa 15.6°N in the northwest to 11.5°N in the southeast), and zone 3 is the Gulf of Aden, from the Gulf of Tadjoura (40°W; Djibouti) to ∼100 km east of the Shukra El Sheik fracture Zone at (46°W; Yemen; Figure 1A).
We want to stress that this two-step time-space approach is suitable when working with large seismically active regions. An increase in seismicity rate, with respect to high baseline activity in a large region, can either be due to clustered seismicity in space (i.e., single mainshock-aftershock sequences or earthquake swarms) or derive from a simultaneous increase in earthquake rate in more than one area. Our swarm detection algorithm is suitable for both scenarios.
In the first step, we used the classical β-statistics approach to identify time windows during which the observed seismicity rate was higher than the determined long-term background rate (Matthews and Reasenberg, 1988). The β-statistics is the standardized distribution of the number of events with respect to the long-term expected number of earthquakes and defined as β=(n(t,Δt)—ne(t,Δt))/σ(t,Δt), where n(t,Δt) is the number of observed events in a time window (t, t+Δt) and ne(t,Δt) and σ(t,Δt) are the average and standard deviation of the expected number of window events given the observed background seismicity rate r (Reasenberg and Simpson, 1992). Anomalously high seismicity rate in a time window (t, t+Δt) is identified when the β-statistics values is greater than a threshold value indicating how many standard deviations the window rate is higher than the expected long-term rate. Negative β-statistics values result when the number of window events is below the number expected from the background rate. The background seismicity rate r is usually calculated on declustered catalogues. However, while space-time-magnitude methods for declustering based on aftershock rate (e.g., Reasenberg, 1985) have proven effective in detecting swarm-like seismicity (Passarelli et al., 2018b), here such methods cannot be applied due to the lack of magnitude determination for nearly half of the events. We coped with this issue by instead calculating the background rate r with a time-dependent approach, i.e., by determining the background rate for each year from 1960 to 2017 (see gray horizontal bars in Figure 2A for Gulf of Aden catalogue), which roughly can account for both the improved catalogue completeness over time (cumulative earthquake number plot in Figure 2A) and for jumps in reported earthquake rate due to local and temporary seismic deployments. For the β-statistics calculation on non-overlapping windows of Δt = 30 days, we calculated the observed number of events n(t,Δt), the expected one ne(t,Δt), and the standard deviation σ(t,Δt)= ne(t,Δt)−1/2 from the yearly background rate r as defined above on non-overlapping windows of Δt = 30 days (Matthews and Reasenberg, 1988). The results of the β-statistics are reported in Figure 2B, as well as the time periods during which we detected an increase in seismicity rate (β-statistics>3). If more than one consecutive 30-days-long time windows met the criteria of detectability, we grouped these windows into a longer one.
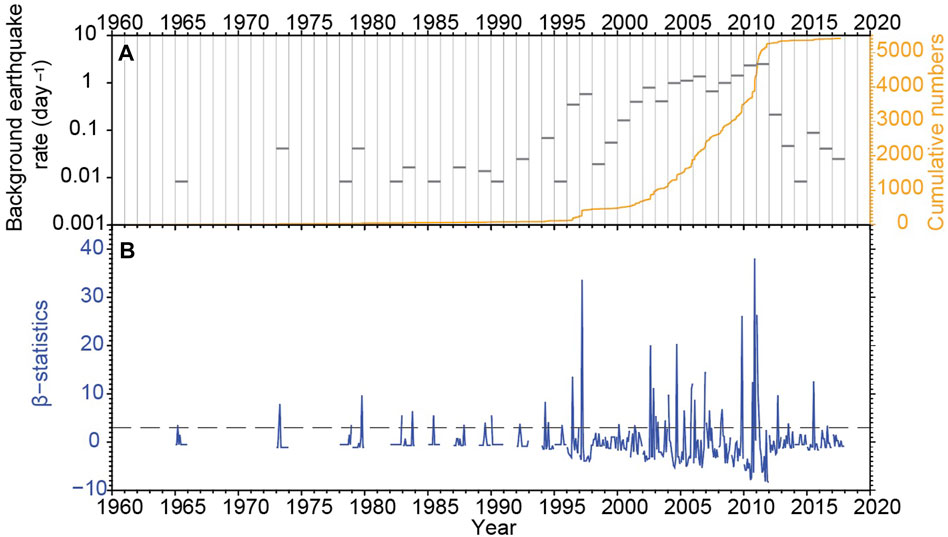
FIGURE 2. Background seismicity rate and earthquake swarm detection for Gulf of Aden. (A) Horizontal bars indicate the estimated background seismicity rate for each year, with gaps representing years with no earthquakes in the catalogue. The cumulative number of earthquakes is shown by the orange curve. (B) The beta statistics calculated for non-overlapping windows of 30 days is shown in dark blue, with gaps reflecting lack of earthquakes in the catalogue. The horizontal dashed line represents the swarm-detection threshold value of 3 standard deviations. Note that the improved monitoring is likely accounting for some of the seismicity increase (see Supplementary Figure S2 for more information).
Once time windows of elevated seismicity rate had been identified in the first step, we used a density-based clustering algorithm in the second step to mark the spatial extent of the seismicity increase. For this we applied the widely-used DBscan algorithm (Ester et al., 1996), using the built-in function dbscan in Matlab, to search for neighboring points in a Euclidean space. We set the neighborhood parameter to ε = 25 km and the minimum number of events in a cluster to Nmin= 5 after a trial-and-error procedure (Cesca, 2020).
To assess the variability of the number of swarm detections in the β-statistics calculation, we tested an alternative detection scheme in which we accounted for a time-dependent earthquake background rate, rather than keeping it constant for each calendar year (Figure 2). The time-dependent background rate was calculated on overlapping time windows ΔTi = 360 days sliding every 30 days, so that ΔTi is centered on the shorter time window Δti= 30 days used for the β-statistics calculation. The alternative algorithm produced minor changes in the number of swarms in the three study regions. In the Southern Red Sea, two additional swarms were identified, but for the Gulf of Aden the swarm number stayed the same, while in the Afar region nine additional swarms were detected (Supplementary Table S1). All these additional swarms occurred in swarm areas (i.e., clusters) that were already identified with the previous approach. In the Afar region, a few small seismic swarms occurred at the periphery of dike intrusions during the Dabbahu rifting episode (2005–2010) and went undetected by the previous approach. Although including a time-dependent earthquake background rate increases slightly the temporal resolution of our scanning algorithm, the few additional swarms do not change the overall picture of spatially distributed clusters.
We applied this swarm detection algorithm separately to all of the detected time windows in the three regions of the southern Red Sea, Afar and Gulf of Aden. We fully rely on this automatic detection procedure for earthquake swarms in the Afar and Gulf of Aden regions, as there were dozens of spatially and temporally overlapping swarms (Supplementary Figure S5). For the southern Red Sea, however, we also visually inspected the dataset as this region has had fewer earthquake swarms that are spatially more distributed. We looked for series of earthquakes that occur repeatedly over time (several events per day and/or hours) in the dataset. These series are typically preceded and followed by periods of no earthquake activity. Then, we looked at the earthquake mean coordinates and magnitudes, and retained them if they were all located in the vicinity (<50 km) of the ridge axis and/or in areas where other swarms have been detected automatically. We found that 25 earthquake swarms that we visually identified in the southern Red Sea were rejected by the automatic detection procedure, despite having all the characteristics of earthquake swarms, mainly due to poor earthquake locations in this region. To account for this problem, we complemented the set of automatically detected earthquake swarms with several manually picked swarms. To the earthquake swarm catalogue described above, we added five earthquake swarms in the Afar area that are not in the ISC catalogue and we also replaced one ISC swarm by a relocated one (Table 1). Furthermore, we pay special attention to the 1993 Bada swarm sequence (modified from Ogubazghi et al., 2004), as it fills a spatial seismicity gap between the Erta Ale Range in Afar and Alid volcano (South of the Gulf of Zula). Finally, we completed the earthquake swarm dataset with 11 historical events (i.e., six strong earthquake swarms and five volcanic eruptions) going back to 1838 in the Afar and Eritrea regions.
The identified earthquake swarms do not show a typical mainshock-aftershock decay, but rather contain multiple earthquakes of comparable magnitudes throughout the duration of each swarm (Figures 3A–D). In total for the 1960–2017 time period, we found that over half (∼8,700 earthquakes) of the earthquakes extracted from the ISC catalogue for the SAGA region fall within 134 earthquake swarm sequences (145 when considering the historical events) located in 19 separate swarm areas (or clusters, i.e., distinct areas where earthquake swarms tend to reoccur).
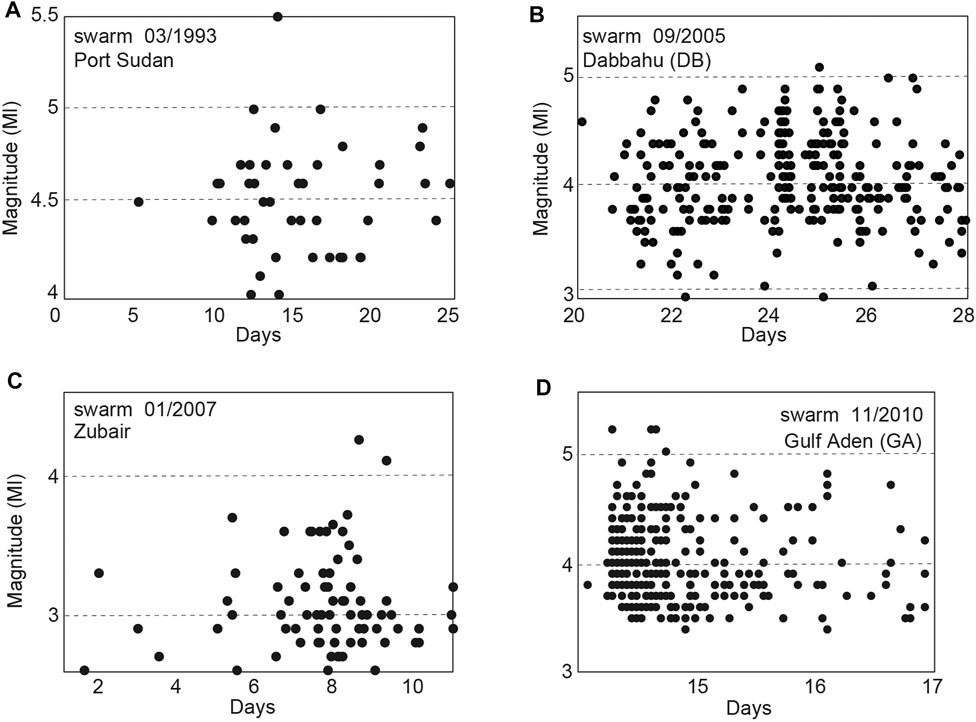
FIGURE 3. Examples of typical earthquake swarm sequences in the SAGA region from (A) Port Sudan (March 1993), (B) Dabbahu (September 2005), (C) Zubair Archipelago (January 2007), and (D) Gulf of Aden (November 2010).
Results
In the following, we describe the earthquake swarm activity separately for the three different zones and then discuss the results in a regional context. We start with an overview of the activity from 1960 to 2020 from north to south for the southern Red Sea and the Afar regions, and from west to east for the Gulf of Aden. We first describe the different swarm cluster areas that were detected, we then detail the different earthquake swarms that were identified in each cluster area, and finally we present the spatio-temporal distribution of the earthquake swarms. We complement the results with historical information going back to 1838, corresponding to the first large earthquake swarm described in Massawa, yielding a period of ∼180 years of earthquake swarms for the Afar region.
Southern Red Sea Earthquake Swarms From 1960 to 2017
In the southern Red Sea, we identified seven clusters, along the southern Red Sea rift axis that together hosted a total of 45 earthquake swarms (of which 25 were detected manually). The seven distinct clusters are separated by areas that are relatively aseismic (Figures 4A,B). All the seven cluster areas have been prone to recurrent swarms during the study period (Figure 4C).
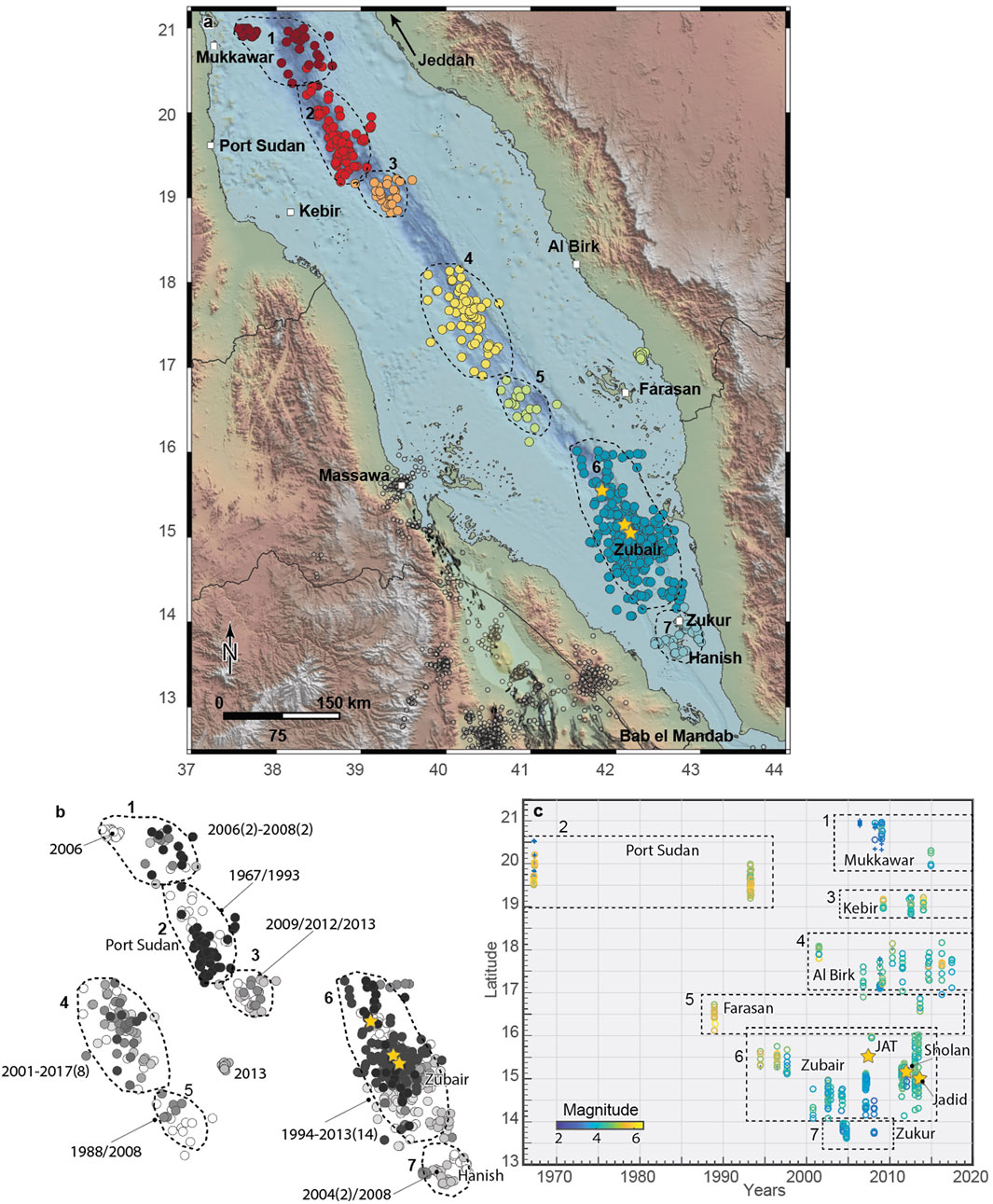
FIGURE 4. Earthquake swarms in the southern Red Sea from 1960 to 2017. (A) Seven different clusters were identified along the southern Red Sea rift axis. (B) Each cluster contains several earthquake swarms whose timing is indicated by the grey gradient from white (older events) to black (younger events). The larger swarms are marked by the year of occurrence with the number of swarms for the noted period in brackets. (C) Swarm latitude as a function of time for the different clusters, with colors showing event magnitudes, blue stars earthquakes with no magnitudes, and yellow stars volcanic eruptions.
The northernmost cluster, east of Mukkawar Island and southwest of Jeddah (cluster 1, 21°N) had an earthquake swarm in 2006 and four swarms from February to December 2008, with magnitudes ranging from 2.2 to 3.9. In cluster 2 (150 km East of Port Sudan, 19.7°N; Figure 4A, a strong swarm of 29 detected earthquakes occurred in March 1967, with five earthquakes > M5. Another swarm took place in this cluster in March 1993 with 46 earthquakes of magnitudes ranging from 4 to 5.6, with six earthquakes larger than magnitude 5. This is the strongest earthquake swarm recorded in the southern Red Sea during the study period. A small swarm occurred in this cluster in 2014. Offshore of Kebir Island in Sudan (cluster 3, 19°N; Figure 4A), we identified four swarms from 2002 to 2013 with earthquake magnitude ranging from 2.3 to 5.1. In cluster 4 (17.5°N), located about 120 km further southeast along the rift axis and offshore of the town of Al Birk (Saudi Arabia), we extracted 10 earthquake swarms from 1975 to 2017. Although these are swarms with limited number of events, their magnitudes range from 3 to 5 and are closely related in time (i.e., several earthquakes per day). In 1988 and 2013, two swarms occurred at a similar latitude northeast of the Farasan Islands (cluster 5, 16.8°S). The November 1988 swarm was among the strongest in the southern Red Sea during the study period with 12 earthquakes ranging from M4.1 to M5.6. The Zubair Archipelago area (cluster 6, ∼15°N; Figures 4A,B) was subject to four swarms from 1994 to 1997 with magnitudes ranging from 3 to 5.1, followed by intense activity from 2007 to 2013, consisting of eight discrete earthquake swarms and three volcanic eruptions. The first of the three eruptions occurred in 2007 on Jebel at Tair island (north of Zubair; Figure 4A), killing four people (Jónsson and Xu, 2015). The later two eruptions gave birth to two volcanic islands, Sholan in 2011–2012, and Jadid in 2015 (Xu et al., 2015). Seven earthquake swarms occurred between 2002 and 2007, 70 km to the south of the Zubair Archipelago. For simplicity we included these swarms in the Zubair cluster (cluster 6) as they partly spatially overlap with the swarms of that cluster. Finally, the southernmost earthquake swarm cluster of the southern Red Sea (cluster 7, 13.8°N), located ∼100 km to the southeast of Zubair islands, had two earthquake swarms in April and September 2004 in the Hanish-Zukur volcanic islands.
Afar and Eritrea Earthquake Swarms From 1960 to 2017
We found seven different clusters in Afar with a total of 46 swarms, located from the town of Massawa in Eritrea in the northwest to the Asal Ghoubbet rift in Djibouti about 550 km to the southeast (Figure 5A). The earthquake swarms took place along the entire length of Afar and, for the most part, were associated with the different active magmatic segments. A few earthquake swarms have also occurred at the rift escarpment (Abala region, Figure 5A) and at the complex structures of the central to eastern Afar, from Serdo to Asal Ghoubbet (Figure 5A).
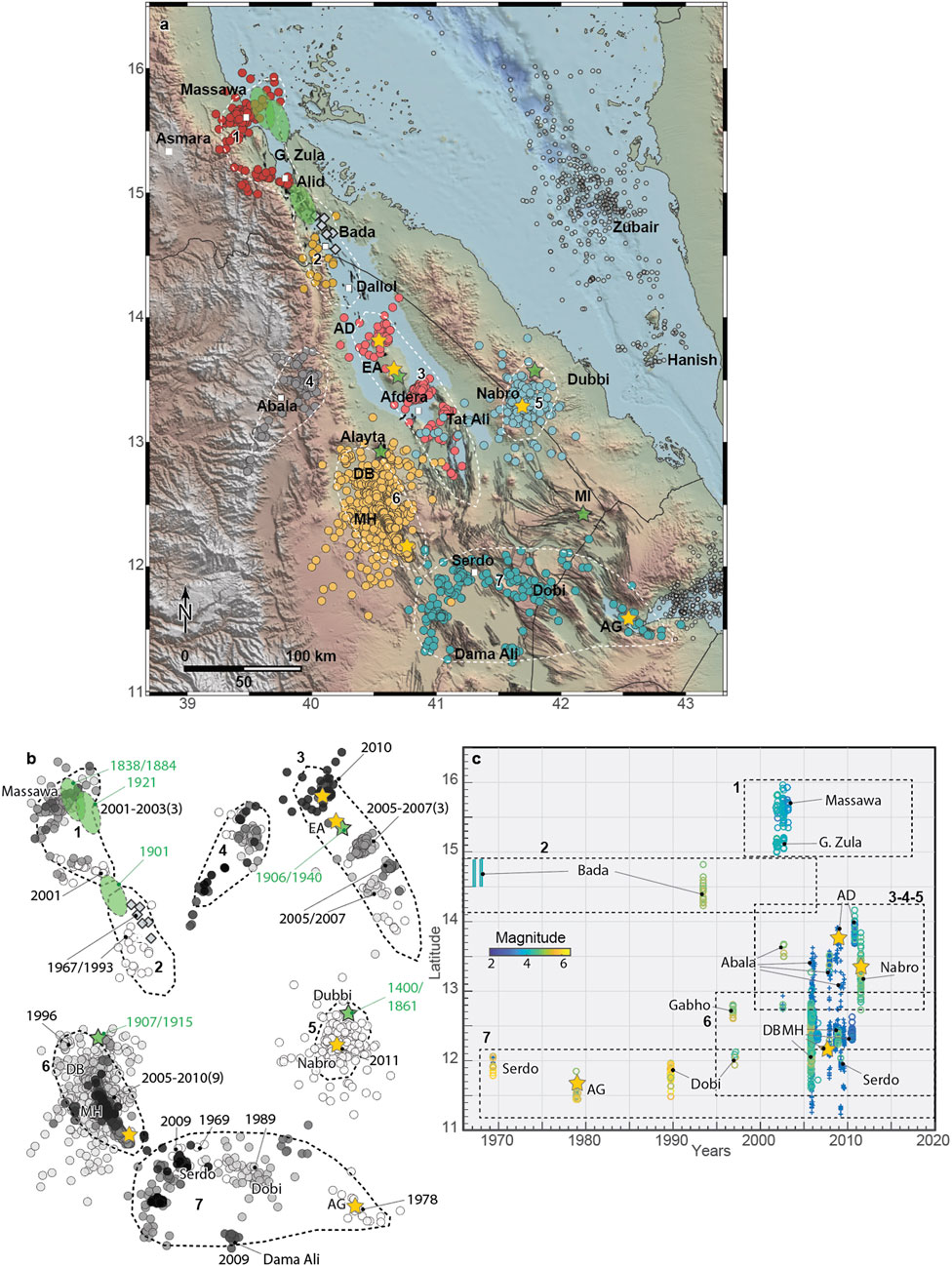
FIGURE 5. Earthquake swarms in the Afar region from 1960 to 2017. (A) Seven different clusters were identified in the Afar region from Massawa (Eritrea) to Asal Ghoubbet (Djibouti). AD is for Alu Dalafilla, EA Erta Ale, MH Manda Hararo, MI Manda Inakir, AG Asal Ghoubbet. (B) Each cluster consists of several earthquake swarms whose timing is indicated by the grey gradient from white (older events) to black (younger events). The larger swarms are marked by the year of occurrence with the number of swarms (in brackets) for the noted period. Green polygons and green stars represent historical swarms and eruptions, respectively. (C) Swarm latitude as a function of time for the different clusters, with colors showing event magnitudes and blue stars representing earthquakes with no magnitudes. Yellow stars show volcanic eruptions.
In November 2001, a small earthquake swarm (35 earthquakes with an mean magnitude of 3.2) occurred in the vicinity of Massawa (cluster 1, 15.6°N). This was followed in 2002, by a year-long swarm with low to moderate magnitude earthquakes (1 < M < 4) (Figures 5A,B). The data was collected by the Eritrean seismic survey (259 earthquakes) and the determined event locations show a clear WSW-ENE oriented pattern (Figures 5A,B). This orientation differs by almost 70° from the roughly NNW-SSE oriented northern Afar rift axis in the Gulf of Zula region, ∼100 km to the south of Massawa. In October-November 2001, another swarm occurred to the west of the Gulf of Zula (Figures 5A–C).
In May 1967 and in May 1968, two earthquake sequences occurred in northern Afar, between the Alid and Bada areas (cluster 2, 14.5°N), south of the Gulf of Zula (Gouin, 1979). In the May 1967 swarm, 79 earthquakes were reported, with magnitudes between 3.1 and 5.1 (Dakin, 1975). Then, following some activity in mid-November 1967, another earthquake swarm occurred in May 1968 with ∼80 earthquakes. These two sequences are not in the ISC catalogue and we show here a few of the related earthquakes reported by Gouin (1979). The Bada region (Eritrea, north of the Ethiopian border; Figure 5A) was subject to another swarm in May 1993 that lasted 25 days and included 17 earthquakes of magnitudes ranging from 4 to 5.2 (see further analysis in Figure 6).
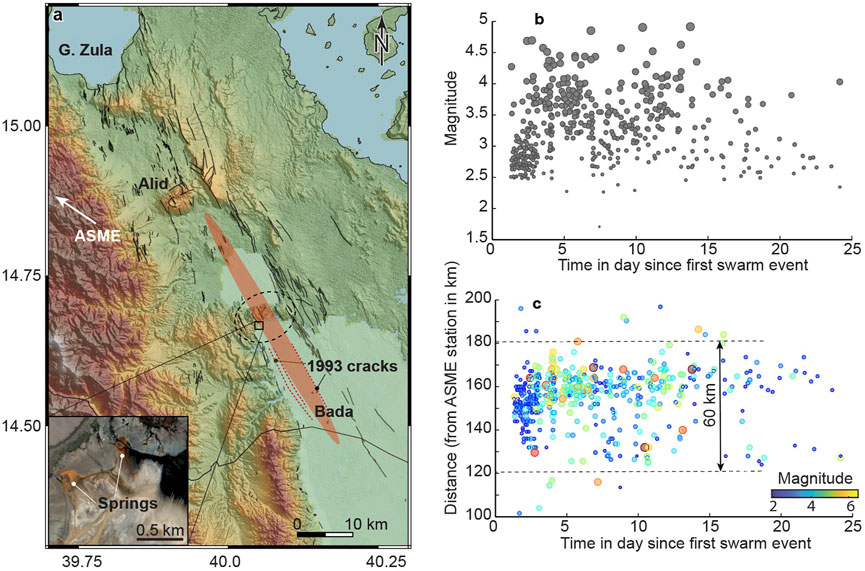
FIGURE 6. The May 1993 Bada earthquake swarm in Eritrea. (A) Map of the Bada region, northern Afar, with the transparent elongated red ellipse showing the approximate extension of the 1993 Bada swarm along a 60-km-long rift segment that was activated during this swarm. Inset shows the location of ferruginous springs at the periphery of a doming area (the dashed circle). (B) Earthquake magnitudes with time show a typical swarm pattern with multiple max-magnitude events during the swarm and absence of a mainshock at the beginning of the sequence. (C) Distance of the swarm earthquakes from the ASME seismic station (see white arrow for the ASME direction located in Asmara; see Figure 1 for location) versus time for the 25 days the swarm lasted.
Cluster 3 extends from Alu-Dalafilla (14°N) down to 12.5°N, along the Erta Ale range, containing the Tat Ali and the Afdera volcanoes (Figure 5A). In 2004, an earthquake swarm occurred in the Dallol area, accompanied by a magnitude 5 earthquake, and marked by a dike intrusion (Nobile et al., 2012). The seismic swarm induced by the intruding dike was recorded at just one station (FURI near Addis Ababa) and hence no locations are reported here. In November 2008, an eruption occurred at Alu-Dalafilla volcanic center, likely fed by a shallow sill reservoir (Pagli et al., 2012). There is again no record of swarm activity in the ISC catalogue accompanying this eruption. Three swarms occurred north of the town of Afdera in 2005, 2006 and 2007 (no magnitudes reported). This seismic activity has been interpreted as related to a tectonic rift linkage connecting the Tat Ali and the Erta Ale volcanic range systems (Illsley-Kemp et al., 2018; La Rosa et al., 2019; Pagli et al., 2019). In October 2005, yet another swarm occurred at the Afdera volcano (no magnitudes have been reported, apart from a single M3 earthquake).
The cluster 4 is offset from the main rift axis and is located near the rift border (13.5°N), partly in the marginal grabens of the western Afar margin in the Abala region (Figure 5A; see Zwaan et al., 2020). A total of 164 swarm earthquakes were detected in this cluster (150 earthquakes have no magnitudes reported), within 9 separate swarms. The strongest swarm occurred in November 2007, 30 km northeast of the town of Abala.
Cluster 5 is the location of the Nabro volcano (13.38°N). The period was marked by the Nabro eruption in June 2011 (east of the Afar rift zone, Figure 5A), the largest eruptions in the region for the last 150 years (since the Dubi eruption in 1861; Figures 5A,B). A total of 142 swarm earthquakes with magnitudes ranging from 3.2 to 5.6 (five larger than magnitude 5) occurred associated with the Nabro eruption.
The first recorded swarm in cluster 6 (from 12 to 13°N) occurred at Gab’ho volcano in 1996 with 10 earthquakes of magnitude from 3.2 to 5. Then, about a decade later, this area was hit by the start of the Dabbahu rifting episode that lasted for 5 years, from May 2005 to June 2010 in the Dabbahu and Manda Hararo magmatic segments (Ebinger et al., 2010). This episode consisted of 14 discrete dike intrusions. Our catalogue contains eight swarms from September 2005 to May 2010 that we describe below. Most of the swarm events have no reported magnitudes (∼3,500 events). The first swarm in the ISC catalogue started in September 2005 and lasted until December 2005. We extracted 1709 earthquakes, out of which 362 have magnitudes between 2.8 and 5.5, with 18 earthquakes larger than magnitude 5. From January to March 2006, a swarm composed of 340 earthquakes (no magnitudes recorded) occurred in the central part of the Dabbahu magmatic segment. From October to November 2007, 440 earthquakes (without magnitudes) were reported. The earthquakes took place over 50 km from Dabbahu volcano south to the Manda Hararo magmatic segment. Another earthquake swarm with a similar pattern occurred from 29 June to September 28, 2008 with 272 earthquakes (no magnitudes reported). Three swarms occurred in roughly the same area in August 2008 (889 earthquakes), February 2009 (215 earthquakes) and May 2009 (48 earthquakes). No magnitudes have been reported for the earthquakes of these three swarms. The last swarm of the Dabbahu rifting episode took place in May 2010 with 104 earthquakes of magnitudes from 0.5 to 4.3.
Cluster 7 extends from Semera to Asal Ghoubbet (from 41 to 42.7°E; Figure 5A). In 1969, the strongest swarm of this area occurred near the town of Serdo in central Afar (Figure 5A). Of the 420 inhabitants, around 40 people died and 160 were wounded by the collapse of the constructions (Gouin, 1979). Ground cracks and subsidence were observed and measured, with faults showing vertical offsets of up to 95 cm and lateral offset of up to 65 cm (Gouin, 1979). Over 250 earthquakes of magnitude above 3 were recorded, with 11 of them in the magnitude range of 4.9–6.2. This area experienced a destructive earthquake swarm (6 months duration) in 1631 in the Aussa region (southeastern Afar), killing 50 people in the town of Waraba (Gouin, 1979). The 1969 activity took place within a broad depression bordered by normal faults (NE of the Tendaho graben), but the earthquake locations are widespread due to poor location determination, although they appear oriented NW-SE over distance of ∼45 km, parallel to the basin direction.
In November 1978, a strong swarm occurred in the Asal Ghoubbet area (Figure 5A), which led to the Ardukôba eruption in December 1978. The swarm, with 16 earthquakes of magnitude ranging from 4.2 to 5.3 occurred in a rift zone that is oriented NW-SE (∼40 km long), extending from offshore to inland. The Asal Ghoubbet rifting event was the first to be surveyed by geodesy in the region (Le Dain et al., 1979; Lépine and Hirn, 1992; Jacques et al., 1996; Doubre et al., 2007a, 2007b).
In August 1989, the NW-SE oriented and ∼40-km-long Dobi graben (cluster 7; Figures 5A,B) experienced a powerful seismic swarm that generated widespread subsidence and normal faulting. For this earthquake swarm, we used relocated events by Jacques et al. (2011). The swarm included 25 recorded earthquakes with 11 earthquakes larger than magnitude 5 and five earthquakes exceeding 6. In 1997, another swarm occurred in the Dobi area with magnitudes from 3.5 to 4.9. From September to December 2005 (contemporary to the first 2005 Dabbahu intrusion event) a swarm composed of 35 recorded earthquakes (no magnitude reported) occurred in the Semera region (cluster 7; Figure 5A). In October 2005, eight earthquakes with magnitudes ranging from 3.4 to 4.7 were detected NW of the Asal region. In November 2007, a small earthquake swarm occurred in the Semera region, somewhat spatially overlapping with the October 2005 swarm, consisting of nine recorded earthquakes (no magnitudes reported). This swarm occurred at the same time as the November 2007 swarm of the Dabbahu rifting episode. In February 2009, an earthquake sequence occurred at the Dama Ali volcano, southeast of Semera (cluster 7; Figures 5A,B). During the same month, another earthquake sequence was detected 10 km west of Serdo (56 earthquakes, no magnitudes). In June 2009, a sequence (35 earthquakes, no magnitudes) occurred 15 km southwest of Semera.
In addition to the data from the ISC catalogue (Figures 5A,B) and to complement the swarm analysis in the poorly studied northern Afar region, we analyzed data from the Eritrean Seismic Survey to infer earthquake magnitudes and locations in the Bada area (651 earthquakes) in May—June 1993. The data are from the ASMA seismic station, located in Asmara city (Eritrea; see Figure 1 for location), whose azimuth from Bada is roughly co-linear with the most likely orientation of the earthquake swarm locations, based on observed fresh ground cracks and graben subsidence. We therefore use the time difference between P and S-wave arrival times at the station to estimate the distance of each earthquake from the station and relative along-rift position within the swarm (Figure 6A). The swarm shows earthquakes with similar magnitudes throughout the 25-days duration of the activity (Figure 6B). Locally, the likely earthquake swarm area corresponds to a topographic high hosting hydrothermal activity (Figure 6A).
Afar and Eritrea Historical Earthquake Swarms From 1838 to 1960
Historical documents contain several earthquake sequences [often referred as tremors by Gouin (1979)] that were witnessed in the SAGA region prior to 1960. For example, repeated earthquake swarms were reported in northern Afar, Gulf of Zula and Massawa (Red Sea coastal area of Eritrea, N15.5) with six major earthquake swarms from the early 19th century (Gouin, 1979) to 1921. From 25 February to the end of March 1838, strong swarms were felt in Massawa (15.6°N), followed by several other smaller sequences in 1844 and 1848 (Figures 5A,B). From July to October 1884, the coastal region close to Massawa was devastated by a sequence of earthquakes and a small tidal wave. The sequences likely occurred in between the Dahlak archipelago and the town of Massawa. From November 11, 1901 to March 1902, tremors were felt near Alid volcano (Figure 5A), south of the Gulf of Zula and in the city of Massawa. Then, during the whole year of 1921, a strong earthquake sequence almost entirely destroyed the city of Massawa. It was so intense that Eritrean folklore remembers it as “Zemene delekeleke”, the era of earthquakes (Gouin, 1979). In addition to these sequences, the Dubbi volcano (13.5°N, 41.8°E) on the Red Sea coast of Eritrea erupted in May 1861 and was associated with strong earthquakes that were felt during 4 months and destroyed two villages, killing 106 people and livestock. This eruption was preceded by another eruption in 1,400. In central Afar, five older volcano-tectonic events have been reported in the Erta Ale (1906, 1940) and Alayta volcanic range (1907, 1915; https://volcano.si.edu/) and in 1928, when an eruption occurred in the Manda Inakir rift in Djibouti (Audin et al., 1990).
Gulf of Aden Earthquake Swarms From 1960 to 2017
The Gulf of Aden shows by far the strongest swarm activity of the three regions, back to the 1970s. We identified five clusters in the area and 43 swarms distributed from the Gulf of Tadjoura in the west (Figure 7A) and then along the Aden ridge for over 250 km to the east (Figures 7A–C). The Aden ridge has been divided in three structural domains, with western, central and eastern domains based on three distinct lithosphere types (Hébert et al., 2001; Leroy et al., 2012). The eastern and central domains have oceanic crust with thickness that changes from 8 to 12 km (Hébert et al., 2001), with the western domain likely being transitional crust between oceanic and continental (Dauteuil et al., 2001). In the following description, clusters 2, 3 and 4 correspond broadly to the western, central and eastern domains, respectively (see Ahmed et al., 2016). As we defined the cluster areas based on swarm event locations, we decided to merge clusters 1, 2, 3 and 4, as they appear to spatially overlap and contain relatively poor event locations, and we then describe them chronologically. From west (42.7°E) to east (45°E) the centers of the four overlapping clusters are located in the Gulf of Tadjoura area (cluster 1, 43°E), offshore of Obock town (cluster 2, 43.5°E; Figure 7A), south of Khor Umera (cluster 3, 44°E; south coast of Yemen; Figure 7A), the cluster containing most of the swarms, and southwest of the city of Aden (cluster 4, 44.7°E; Figure 7). These four cluster areas form an almost structural continuum made of a series of oblique rifts that are well visible in bathymetric data (Ahmed et al., 2016).
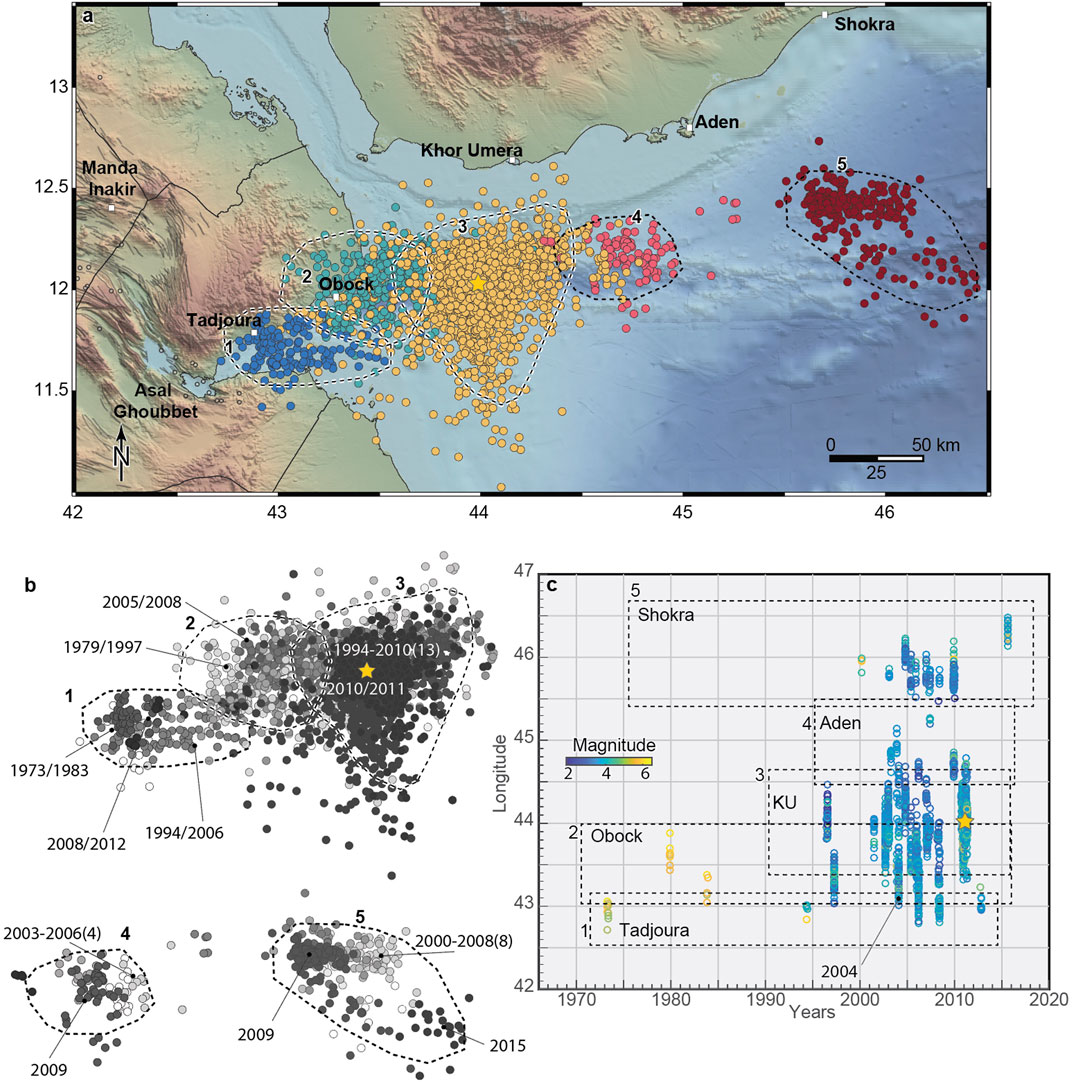
FIGURE 7. Earthquake swarms in the Gulf of Aden from 1960 to 2017. (A) Five different swarm areas (clusters) were identified in the Gulf of Aden, from the Gulf of Tadjoura to the west of the town of Shokra (Yemen). (B) Each cluster consists of several earthquake swarms whose timing is indicated by the grey gradient from white (older events) to black (younger events). The larger earthquake swarms are marked by the year of occurrence with the number of swarms (in brackets) for the noted period. The yellow star shows the location of the 2010 submarine eruption. (C) Longitude location of the swarms as a function of time with colors indicating event magnitudes.
Clusters 1 and 2 contain 24 earthquake swarms from 1973 to 2011. In 1973, a year after the Asal Ghoubbet rifting event, a powerful swarm occurred offshore of Obock (Figures 7A,B), comprising seven earthquakes of magnitudes between 5.0 and 5.7. Six swarms occurred from 1993 to 1997 and they are relatively well located on the rift axis. From 2001 to 2003, 5 swarms occurred; two strong swarms in August and November 2002 with 188 earthquakes and magnitudes ranging from 3 to 5.1, of which 15 earthquakes were larger than magnitude 4. In January 2004, another strong swarm made of 83 earthquakes occurred along the entire Aden ridge (i.e., clusters 1, 2, 3 and 4), contrasting earlier swarms that mostly occurred within only single portions of the ridge. In this large swarm, the earthquakes were well distributed from Tadjoura (43°E) to 44.7°E, with magnitudes ranging from 2.8 to 5.3. Then, from 31 August to September 26, 2010, a strong swarm occurred in the area, hosting the largest rifting episode during the period of observation in the Gulf of Aden. From October 2010 to November 25, 2010, 379 earthquakes were recorded (ISC catalogue) with magnitudes ranging from 2.7 to 5.5, with 24 earthquakes larger than five (see Schuler and Nettles, 2012 and Ahmed et al., 2016). This was followed by another powerful swarm from January to March 2011, which included 581 earthquakes of magnitudes ranging from 2.3 to 5.2, with two earthquakes larger than 5. Although our automatic swarm detection methodology selected these earthquakes as being within a single swarm, this swarm has been split in two by Ahmed et al. (2016).
Another cluster area was found further to the east (cluster 5, 46°E; Figures 7A–C). We identified a total of 10 earthquake swarms within this cluster from February 2000 to November 2015 that contained some 316 earthquakes of magnitudes ranging from 2.2 to 5.6, with eight earthquakes above magnitude 5. This cluster is offset from the main ridge axis; however, volcanic vents have been observed at this location (Dauteuil et al., 2001).
Discussion
Recurrent Swarm Activity Highlights Spreading Centers in the SAGA Region
Our results show that many areas in the SAGA region have been subject to recurrent earthquake swarm activity since 1960. Good examples include the swarm areas (or clusters) offshore of Port Sudan (with two strong swarms occurring in 1967 and 1993), Kebir, and Al Birk, and in the Zubair archipelago (see Figure 4A). These clusters in the southern Red Sea likely relate to active spreading centers on the Red Sea rift axis.
The Bada region of northern Afar (Figure 6A) was hit by three strong swarms in 1967, 1968 and 1993, and the area north of Bada was affected recurrently by earthquake swarms in 1838, 1884, and 1920–21, destroying parts of Massawa city. This area at the northern end of the northern Afar axis has been seismically active with repeating swarms over the past two centuries, leading to questions on how the rift axis extends northward through the Gulf of Zula (Viltres et al., 2020) and connects to a transform zone from Massawa region to the central rift trough in the southern Red Sea. The 2002 Massawa earthquake swarm shows a clear WSW-ENE orientation of the activity that differs from the NNE-SSW rift orientation south of the Gulf of Zula. Two large-magnitude earthquakes (M6.6 and M6.1) occurred just west of the Red Sea trough, respectively in 1977 and 1980, suggesting a possible transform fault, an interpretation supported by the strike-slip focal mechanisms of these earthquakes (Figure 9). About 340 km to southwest, on the rift escarpment, the cluster 4 shows also recurrent swarms in the Abala region with a strong event in November 2007. Then, the Dabbahu-Manda Hararo magmatic segments have been subjected to intrusions of 14 discrete dikes from 2005 to 2010 during the Dabbahu rifting episode.
The Gulf of Aden has probably produced the region’s best example of recurrent swarm sequences along a spreading ridge that then hosted a rifting episode in 2010–2011 (cluster 1 to 3, Figure 7A). The same area had 23 other earthquake swarms along this 250 km spreading area from 1973 to 2011. Clusters 4 and 5 in the Gulf of Aden have also shown recurrent swarm activity that likely relates to other spreading centers (Figure 7).
More broadly, the spreading centers concentrate the strain release during magmatic and tectonic events and therefore, their locations are important for a better understanding of the overall evolution of plate motions in the SAGA region. Earthquake swarm activity in the triple junction reveals overlapping rift segments. The Southern Red Sea and the Afar ridges are located on both sides of the Danakil Alps and both spreading ridges overlap for ∼150 km (Figure 8). In central Afar, en-echelon, partly overlapping rifts segments (Asal Ghoubbet- Dobi- Serdo- Manda Hararo) are also highlighted with both swarm activity and rift structures (Figure 8). These multiscale overlapping segments suggest a complex interaction between tectonics and magmatism in the SAGA region influenced by the regional geodynamics. While the earthquake swarms since 1960 highlight most of divergent plate boundaries in the SAGA region, a few zones did not show any swarm activity during the study period. For example, a 100-km-long segment between clusters 3 and 4 in the southern Red Sea (Figure 8A) remained quiescent. This does not necessarily mean that such quiet ridge segments are completely inactive, as the study period includes only the past 60 years. In Iceland, time intervals of ∼200 years between rifting episodes appear not atypical, e.g., between periods of activity at Krafla (1724–1729 and 1975–1984) and at Holuhraun during Bárðarbunga rifting events (1797 and 2014–2015), with the inter-rifting duration characterized by relative seismic quiescence (Ruch et al., 2016).
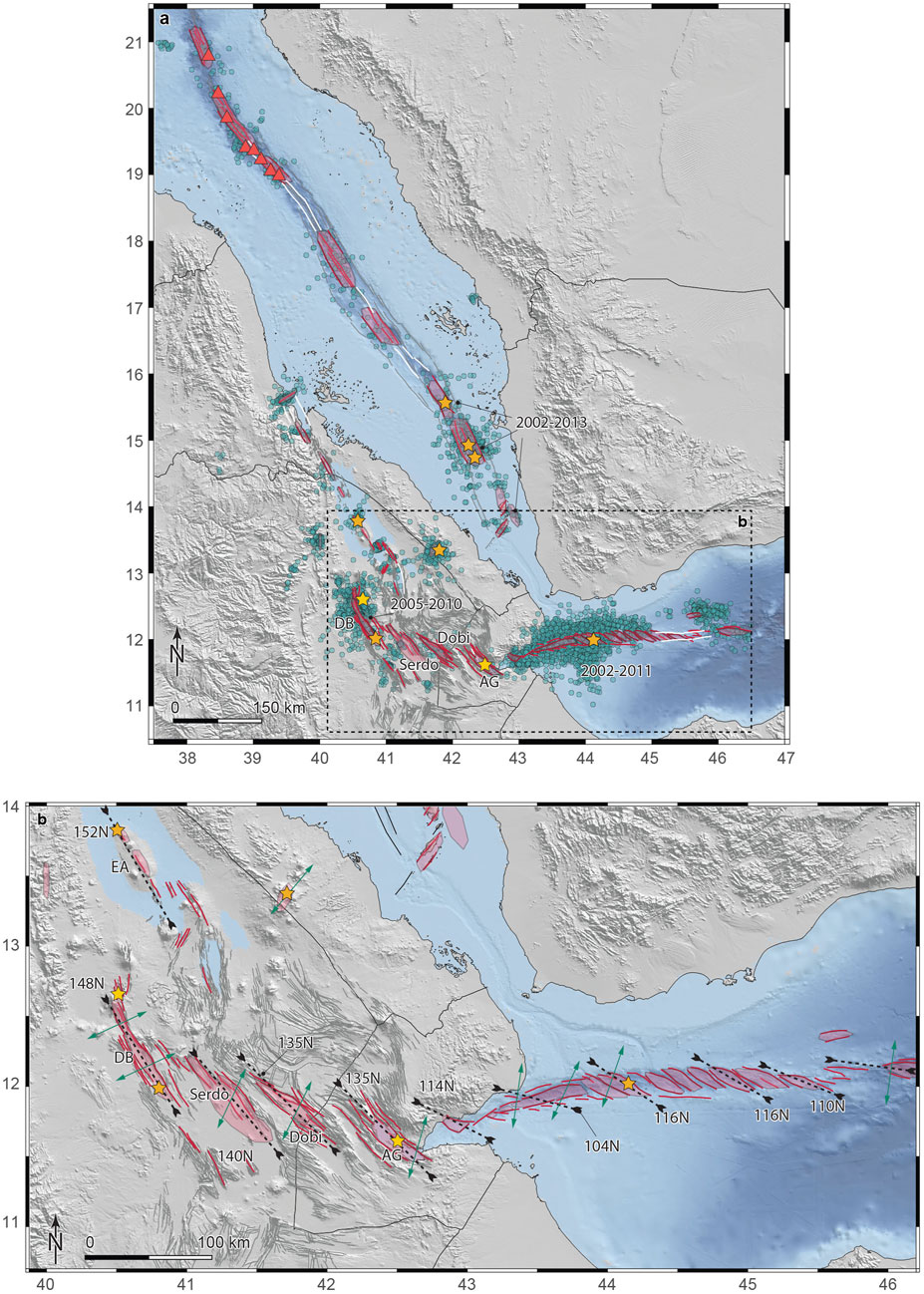
FIGURE 8. Earthquake swarms and tectonic activity for the last 60 years in the SAGA region. (A) Swarm location from 1960 to 2017 (green dots). Red lines and semi-transparent pink polygons show structures and portion of the active rift zones where swarms have been observed from 1960 to 2017. White lines show areas where no swarms have been detected from 1960, along known plate boundaries. Yellow stars are volcanic eruption locations. Red triangles show volcanic vents and volcanic domes at sea bottom (after Augustin et al., 2016). (B) Close up view on the structural continuum from Gulf of Aden (after Ahmed et al., 2016), Asal Ghoubbet (AG), Dobi, Serdo and the Dabbahu magmatic segment (DB) characterized by oblique spreading and en-echelon extensional basins. Dashed lines with opposite arrows show the corresponding basin’s mean orientation. Green double arrows show the mean extension direction, extracted from focal mechanisms analysis. Erta Ale (EA) has a similar orientation that Dabbahu magmatic segment. Grey lines are first order lineaments of the area; thin black lines show the coast line and the borders of the countries.
On the Origin of Earthquake Swarms
Our results show that apart from a few quiescent segments, most of the length of the plate boundaries separating the Nubian, Arabian and Somalian plates have been activated by seismic swarms and volcanic activity since 1960 (Figure 8A). Focal mechanisms also show that most of the swarms are characterized by normal faulting, indicative of the extensional tectonics that dominates in the region (Figure 9).
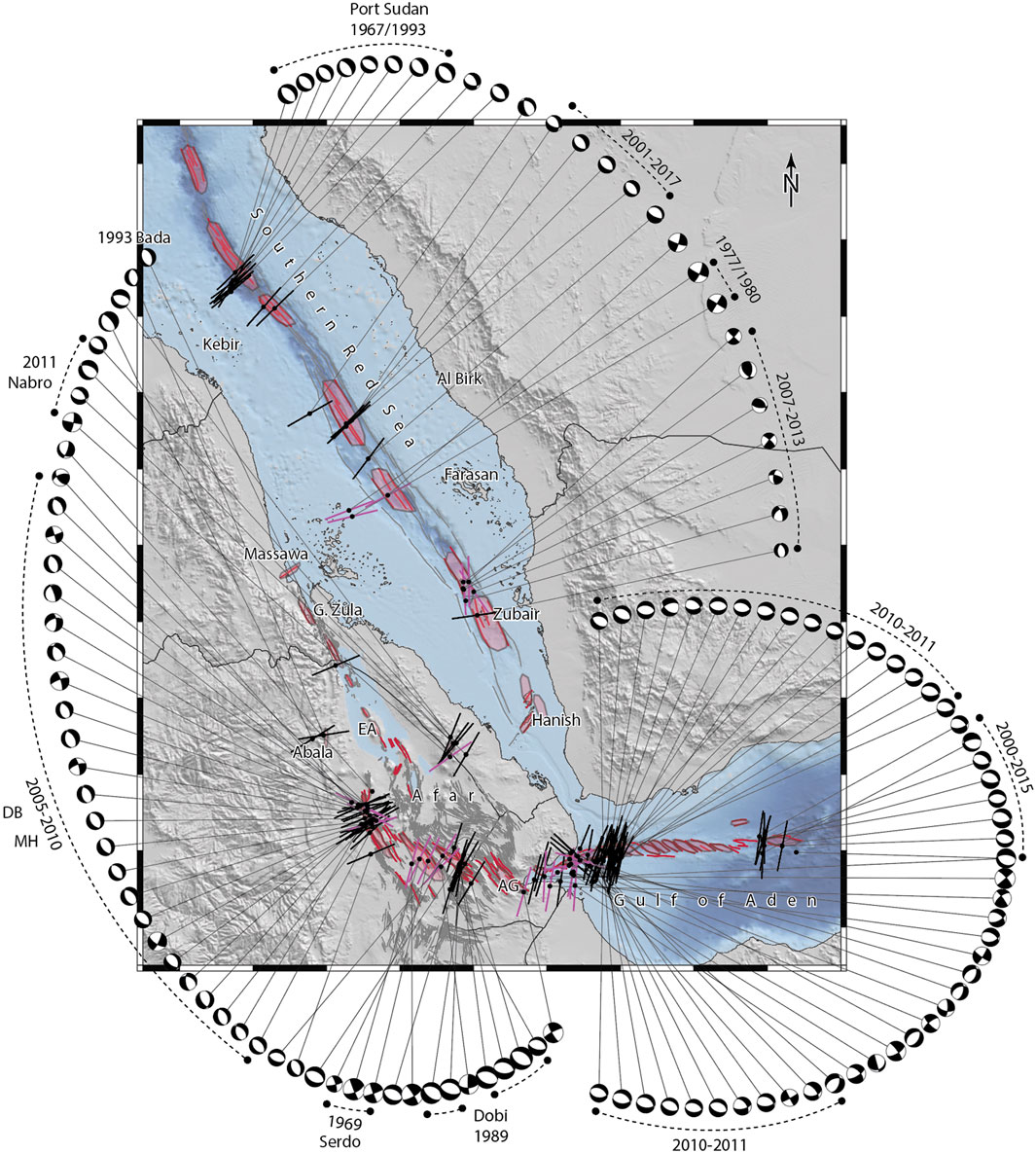
FIGURE 9. Focal mechanisms (from Hofstetter and Beyth, 2003, and Global Centroid Moment Tensor project, GCMT, www.globalcmt.org, Dziewonski et al., 1981; Ekström et al., 2012) related to swarms in the SAGA region from 1960 to 2017 as sourced from the ISC Bulletin. Extension axes are shown by black segments (normal faulting) and pink segments (strike-slip faulting). Selected strong swarms are indicated by dates and area names (see main text for details). DB and MH for Dabbahu and Manda Hararo magmatic segments, respectively. Pink polygons and red lines represent the areas that have been affected by swarm activity (see Figure 8 for details).
The central question on the origin of these earthquake swarms is whether they are of magmatic or tectonic origin. Some of the swarms were clearly magmatic, as they were accompanied by observed volcanic activity. However, for the majority of the swarms detected in the SAGA region, the link to magma is less clear. Resolving this has important implications, as swarms are responsible for key morphological processes observed in active rift zones and often precede volcanic eruptions. The central axis of the Southern Red Sea trough hosts numerous volcanic vents and thus the earthquake swarms in this region are likely associated with magma activity. Bathymetric data of cluster areas 2 and 3 (e.g., offshore of Port Sudan, see Figure 4A for location) exhibit several volcanic vents and fresh lava flows (Figure 8A; Augustin et al., 2016), showing the contribution of magma in shaping the rift zone and pointing to a likely origin of the observed swarms. Further south, cluster 6 (Zubair Archipelago) had intense activity in 14 earthquake swarms between 1994 and 2013. Three volcanic eruptions occurred during this time period, which demonstrates a magmatic origin of the earthquake swarms, at least in these three cases. Then, most of the earthquake swarms observed inland in the SAGA region and after regular InSAR observations started (after 1990s) show unambiguous contribution of magma through dike injections (e.g., Gab’ho 1996, Dabbahu, 2005–2010, Dallol, 2004). A few other swarms relate to rift linkage and are likely of a tectonic origin (Afdera swarm, 2005–2007, Illsley-Kemp et al., 2018; La Rosa et al., 2019).
Earthquake swarms that preceded the era of InSAR ground deformation analysis (i.e. from before the early 1990s) and did not trigger volcanic eruptions have typically been associated to tectonic events, rather than to magmatic intrusions, e.g., the swarms at Serdo (1969), Dobi (1989) (e.g. Ebinger and Wijk, 2013; Keir et al., 2013; Pagli et al., 2019) and in Bada (1993). However, for these events there is no unambiguous way to attribute the swarm activity to either magmatic or tectonic processes. In Bada, rift parallel ground cracks, vertical offsets and rockfalls resulted in a formation of a graben (Ghebreab and Solomon, 1994; Ogubazghi et al., 2004). Note that 17 earthquakes larger than M4 happened after the field campaign of Ghebreab and Solomon (1994) so that final ground measurements were never completed. These surface effects, as well as the space-time evolution of the swarm with event migration away from a segment centered volcanic system and the largest earthquakes at the migration fronts, are typical of dike-induced seismicity (Figure 6C), e.g., as observed during the Dabbahu rifting episode (e.g., Barnie et al., 2016). The repeated swarm activity in the Bada area points to the presence of an immature spreading center, such as the one in Dallol, located about 50 km to the southeast.
Although the large-magnitude earthquakes observed during the Serdo and the Dobi earthquake sequences relate to meter-scale fault slip at the surface, the swarm patterns of the two sequences are characterized by an absence of main shock-aftershock sequences. The observed ground ruptures (graben formation, normal faulting and open fractures) are also typically observed during rifting events so that magma contribution cannot be excluded for these two sequences. Moreover, recent dike intrusions have been associated with earthquakes of similarly large magnitudes, such as the Miyakejima dike intrusion (Passarelli et al., 2015) where large dike-induced stresses both produced large earthquakes on likely newly formed normal faults and on pre-existing tectonic strike-slip structures that were favorably oriented. The Serdo area includes a few volcanic edifices (e.g., Dama Ali volcano; erupted in 1631 CE, Figure 5A), suggesting that magma reached the surface in the region. Reed et al. (2014) documented high Vp/Vs ratios within the lower crust beneath the Dobi graben, which implies the presence of melt (Demissie et al., 2018). Finally, the structural framework of the region is composed of a set of left-stepping en-echelon basin structures from the Aden ridge oriented ∼110°N, Asal Ghoubbet (135°N), Dobi (135°N), Serdo (140°N) and the Manda Hararo magmatic segment (148°N) forming a structural continuum (Figure 8B). The tensional axes derived from the focal mechanisms of swarms are all oblique with respect to the mean orientation of the basins (Figures 8B, 9). These en-echelon basins are affected at both edges by magmatic intrusions (i.e., Gulf of Aden ridge and the Manda Hararo-Dabbahu magmatic segments) and the central portion (Dobi and Serdo) might originate from a mixed source of deformation where magmatic and tectonic processes may interact. Therefore, while the origin of the older swarms in this region cannot be unambiguously related to either tectonic or magmatic activity, for some of these swarms both tectonic and magmatic processes were likely at work.
Swarm and Volcanic Activity Increase During the mid 2000s to mid 2010s
Supplementary Figure S2 broadly shows a progressive increase in annual number of earthquakes reported by the ISC since the early 1990s compared to the preceding period. Much of this increase is consistent with improvements in regional seismic station density. To address this, we also plotted annual numbers of events of M4.5 and larger in order to reveal temporal changes in earthquake activity above the magnitude of completeness through the entire time period (Figure S2). This shows noticeable lobes of increased number of M4.5 and larger earthquakes in roughly decadal timeframes such as in the late 1970s, and the late 1980s to mid 1990s. We test this hypothesis of increase of earthquake rate for the class of M4.5 and larger earthquakes over decadal timeframes against a stationary earthquake occurrence described by a Poisson model with constant rate (Zhuang et al., 2012). In a Poissonian stationary model, the times between two consecutive earthquakes (i.e., inter-event times IETs) follow an exponential distribution with first moment equal to the long-term seismicity rate λ. The fit of seismicity rate to the observed earthquakes restitutes λ = 0.026 with 95% confidence interval λCI= 0.024–0.029. We simulate 100 synthetic catalogs of IETs from the exponential distribution with randomly varying λCI. We then compared the observed and each of the simulated distributions of IETs through a Kolmogorov-Smirnov (KS) two-sided goodness-of-fit test (Massey, 1951) under the null hypothesis that both are independent sample from the same underlying distribution. We rejected the null hypothesis 100% of times at significance level of α = 0.01 for the 100 simulations. We applied the same test to only events with M5 and larger and obtained the same results. We conclude that at first order the observed catalog does not follow a Poissonian stationary process and the earthquake lobes over decadal timeframe are a feature in the SAGA catalog not likely caused by the aftershock activity and thus relates to temporal increase of the swarm activity.
The most recent increase occurs during 2003–2013 in which there is an increase of the swarm activity in all the three rift branches of the southern Red Sea, Afar and the Gulf of Aden (SAGA region; Figure 2B and Figure 10). During the same time period, a total of seven volcanic eruptions occurred in all the three areas (Figure 8A). The three different rift branches were subject to three roughly synchronous rifting episodes, with Dabbahu (2005–2010; Wright et al., 2006), Gulf of Aden (2003–2011; Ahmed et al., 2016) and the southern Red Sea (2003–2013; Xu et al., 2015). The swarms in the Zubair Archipelago were temporally related to magmatic intrusions and eruptions (Jebel at Tair, Sholan and Jadid islands), while no volcanic activity was reported in association with two swarms further south near the Zukur and Hanish islands in 2004. Our dataset shows that the Gulf of Aden had been subject to strong swarms already from 2002 to 2004, indicating an increase of swarm activity 8 years before the main rifting activity in late 2010. This overall active 2003–2013 time period was followed by a seismically quiet period from 2013 to 2020 without any noticeable swarm activity.
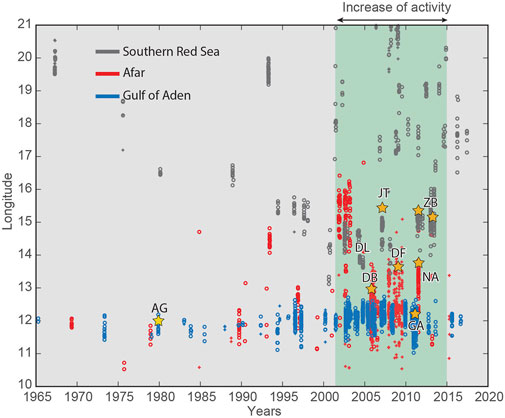
FIGURE 10. Increase of swarm and volcanic activity observed from 2003 to 2013 in the SAGA region, preceded and followed by lower rates of swarm activity and the absence of volcanic eruptions. Yellow stars are eruptions; AG: Asal Ghoubbet; DL: Dallol; DB: Dabbahu; JT: Jebel at Tair; DF: Alu Dalafilla; GA: Gulf of Aden; NA: Nabro; ZB: Zubair Archipelago.
These synchronous rifting episodes are focused in Afar and in the immediately adjacent parts of the southernmost Red Sea and westernmost Gulf of Aden. They suggest that this sector of the SAGA region experiences a regional rifting cycle, where the plate extension may have generated enough strain deficit at the plate boundaries to be released during a five to 10 years co-rifting period after an inter-rifting period of tectonic loading. This could suggest a common source of deformation for all three rift branches. Since rifting episodes are modulated by the interplay between magma supply and tectonic stress (e.g. Buck, 2006), we also propose that the regional increase in activity could have been triggered by an overall increase of the magma supply rate from the underlying mantle in the SAGA region. Alternatively, the synchronous increase in activity across multiple segments of the rift might also be explained by regional stress triggering in which positive Coulomb stress changes induced by magma intrusion at one rift branch and associated earthquake swarms trigger magmatic and tectonic activity at the other rift branches that were near failure. However, there is little evidence for static stress triggering between distant (∼350 km) rifting events and episodes in the region (Viltres et al., 2018). Such stress triggering has been observed at smaller scales within Afar, i.e., where several small swarms have been detected at the periphery of large dike intrusions in Afar in 2005, 2007 and 2009, synchronously with the Dabbahu rifting episode. These swarms could thus relate to static stress changes triggered by the dike intrusions inducing an increase of the seismicity at the dike periphery.
Conclusion
Observations of earthquake swarm activity over multiple decades can provide new information about areas prone to swarm activity (clusters), on swarm reoccurrence, and on regional magmatic and tectonic activity in general. In this study, we have generated a new earthquake swarm catalogue for the southern Red Sea, Afar and Gulf of Aden (the SAGA region) by integrating the ISC catalogue with additional local datasets, producing the first multi-decadal overview of the earthquake swarm activity of the SAGA region. The new swarm catalogue is composed of 19 clusters and 134 swarms from 1960 to 2017. Adding 11 volcano-tectonic events (six earthquake swarms and five volcanic eruptions) that occurred from 1838 to 1960, the catalogue includes a total of 145 events in the last 180 years. Most of the clusters show recurrent swarm activity over decades, supporting the presence of unnoticed active spreading centers in the SAGA region. Most of these swarms have likely a magmatic origin, as supported by eruption or ground deformation study, although we cannot rule out an unambiguous magmatic origin for all these swarms. The three rift branches show an increase of the swarm and volcanic activity between 2003 and 2013 suggesting the presence of a rifting cycle affecting part of the SAGA region.
Data Availability Statement
The original contributions presented in the study are publicly available and the ISC catalogue for the period of observation in the SAGA region, the swarm results and the focal mechanisms are all available in a repository and can be found here: https://doi.org/10.31905/TKSACJMM. Further questions can be directed to the authors.
Author Contributions
JR design the research, analyze the data, designed the Figures and wrote the paper; DK participated to the design of the research, analyzed the data and wrote the paper; LP analyzed the earthquake swarm data and wrote dedicated section of the paper; DG provided the revisited ISC catalogue and wrote dedicated section of the paper; GO provided and analyzed the data for the Bada sequence; SJ participated to the design of the research and wrote the paper.
Funding
This research was supported by King Abdullah University of Science and Technology (KAUST), under award number OSR-2015-CRG4-2643, and by the Swiss National Science Foundation, grant PP00P2_176869 JR. In addition, DK was supported by NERC grant NE/L013932 and by MiUR through PRIN grant 2017P9AT72 and LP was supported by NEWTON-g project, Grant Agreement No 801221.
Conflict of Interest
The authors declare that the research was conducted in the absence of any commercial or financial relationships that could be construed as a potential conflict of interest.
Publisher’s Note
All claims expressed in this article are solely those of the authors and do not necessarily represent those of their affiliated organizations, or those of the publisher, the editors and the reviewers. Any product that may be evaluated in this article, or claim that may be made by its manufacturer, is not guaranteed or endorsed by the publisher.
Acknowledgments
We thank SO and SH for their in-depth and very constructive comments that contribute to ameliorate the quality of the paper. We also thank the Editor LL and the Field Chief Editor Valerio Acocella for the careful handling of the paper.
Supplementary Material
The Supplementary Material for this article can be found online at: https://www.frontiersin.org/articles/10.3389/feart.2021.664673/full#supplementary-material
Footnotes
†Present Address: Luigi Passarelli, Department of Earth Sciences, University of Geneva, Geneva, Switzerland
References
Abbate, E., Passerini, P., and Zan, L. (1995). Strike-slip Faults in a Rift Area: a Transect in the Afar Triangle, East Africa. Tectonophysics 241, 67–97. doi:10.1016/0040-1951(94)00136-w
Abdallah, A., Courtillot, V., Kasser, M., Le Dain, A.-Y., Lépine, J.-C., Robineau, B., et al. (1979). Relevance of Afar Seismicity and Volcanism to the Mechanics of Accreting Plate Boundaries. Nature 282, 17–23. doi:10.1038/282017a0
Ágústsdóttir, T., Woods, J., Greenfield, T., Green, R. G., White, R. S., Winder, T., et al. (2016). Strike-slip Faulting during the 2014 Bárðarbunga-Holuhraun dike Intrusion, central Iceland, Geophys. Res. Lett. 6, 1–402. doi:10.1002/2015GL067423
Ahmed, A., Doubre, C., Leroy, S., Kassim, M., Keir, D., Abayazid, A., et al. (2016). Seafloor Spreading Event in Western Gulf of Aden during the November 2010-March 2011 Period Captured by Regional Seismic Networks: Evidence for Diking Events and Interactions with a Nascent Transform Zone. Geophysical Journal International. 205, 1244–1266. doi:10.1093/gji/ggw068
Audin, J., Vellutini, P. J., Coulon, C., Piguet, P., and Vincent, J. (1990). The 1928?1929 Eruption of Kammourta Volcano ? Evidence of Tectono-Magmatic Activity in the Manda-Inakir Rift and Comparison with the Asal Rift, Afar Depression, Republic of Djibuti. Bull. Volcanol. 52, 551–561. doi:10.1007/BF00301536
Augustin, N., van der Zwan, F. M., Devey, C. W., Ligi, M., Kwasnitschka, T., Feldens, P., et al. (2016). Geomorphology of the central Red Sea Rift: Determining Spreading Processes. Geomorphology 274, 162–179. doi:10.1016/j.geomorph.2016.08.028
Ayele, A., Jacques, E., Kassim, M., Kidane, T., Omar, A., Tait, S., et al. (2007). The Volcano-Seismic Crisis in Afar, Ethiopia, Starting September 2005. Earth and Planetary Science Letters 255, 177–187. doi:10.1016/j.epsl.2006.12.014
Barnie, T. D., Keir, D., Hamling, I., Hofmann, B., Belachew, M., Carn, S., et al. (2016). A Multidisciplinary Study of the Final Episode of the Manda Hararo Dyke Sequence, Ethiopia, and Implications for Trends in Volcanism during the Rifting cycleGeological Society London Special Publications. Geol. Soc. Lond. Spec. Publications 420, 149–163. doi:10.1144/sp420.6
Belachew, M., Ebinger, C., Cote, D., Keir, D., Rowland, J. V., Hammond, J. O. S., et al. (2011). Comparison of dike Intrusions in an Incipient Seafloor-Spreading Segment in Afar, Ethiopia: Seismicity Perspectives: Journal of Geophysical Research. Solid Earth. 116, 1–23. doi:10.1029/2010jb007908
Bergman, E. A., and Solomon, S. C. (1990). Earthquake Swarms on the Mid-Atlantic Ridge: Products of Magmatism or Extensional Tectonics? J. Geophys. Res. 95, 4943–4965. doi:10.1029/jb095ib04p04943
Bird, P., Kagan, Y. Y., and Jackson, D. D. (2002). Plate Tectonics and Earthquake Potential of Spreading Ridges and Oceanic Transform Faults: Geodynamics Ser. 30, 203–218. doi:10.1029/gd030p0203
Boettcher, M. S., and Jordan, T. H. (2004). Earthquake Scaling Relations for Mid-ocean ridge Transform Faults: Journal of Geophysical Research. Solid Earth. 109, 1–21. doi:10.1029/2004jb003110
Bondár, I., and Storchak, D. (2011). Improved Location Procedures at the International Seismological Centre. Geophysical Journal International 186, 1220–1244. doi:10.1111/j.1365-246x.2011.05107.x
Buck, W. R. (2006). The Role of Magma in the Development of the Afro-Arabian Rift System. Geol. Soc. Lond. Spec. Publications 259, 43–54. doi:10.1144/gsl.sp.2006.259.01.05
Cesca, S. (2020). Seiscloud, a Tool for Density-Based Seismicity Clustering and Visualization. J. Seismol 24, 443–457. doi:10.1007/s10950-020-09921-8
Chen, X., and Shearer, P. M. (2011). Comprehensive Analysis of Earthquake Source Spectra and Swarms in the Salton Trough, California: Journal of Geophysical Research. Solid Earth. 116, 1–17. doi:10.1029/2011jb008263
Dakin, F. M. (1975). A Preliminary Survey of the Distribution and Characteristics of Earthquake Sequences in Ethiopia and T.F.A.I. Bull. Geophys. Obs. Ethiopia 15, 51–70.
Dauteuil, O., Huchon, P., Quemeneur, F., and Souriot, T. (2001). Propagation of an Oblique Spreading centre: the Western Gulf of Aden. Tectonophysics 332, 423–442. doi:10.1016/s0040-1951(00)00295-x
Demissie, Z., Mickus, K., Bridges, D., Abdelsalam, M. G., and Atekwana, E. (2018). Upper Lithospheric Structure of the Dobi Graben, Afar Depression from Magnetics and Gravity Data. J. Afr. Earth Sci. 147, 136–151. doi:10.1016/j.jafrearsci.2018.06.012
Di Giacomo, D., and Storchak, D. A. (2016). A Scheme to Set Preferred Magnitudes in the ISC Bulletin. J. Seismol 20, 555–567. doi:10.1007/s10950-015-9543-7
Doubre, C., Manighetti, I., Dorbath, C., Dorbath, L., Jacques, E., and Delmond, J. C. (2007a). Crustal Structure and Magmato-Tectonic Processes in an Active Rift (Asal-Ghoubbet, Afar, East Africa): 1. Insights from a 5-month Seismological experiment: Journal of Geophysical Research. Solid Earth 112, 1–22. doi:10.1029/2005jb003940
Doubre, C., Manighetti, I., Dorbath, C., Dorbath, L., Jacques, E., and Delmond, J. C. (2007b). Crustal Structure and Magmato-Tectonic Processes in an Active Rift (Asal-Ghoubbet, Afar, East Africa): 1. Insights from a 5-month Seismological experiment: Journal of Geophysical Research. Solid Earth 112, 1–32. doi:10.1029/2005jb003940
Dziak, R. P., Bohnenstiehl, D. R., Cowen, J. P., Baker, E. T., Rubin, K. H., Haxel, J. H., et al. (2007). Rapid dike Emplacement Leads to Eruptions and Hydrothermal Plume Release during Seafloor Spreading Events. Geol. 35, 579–582. doi:10.1130/g23476a.1
Dziak, R. P., Bohnenstiehl, D. R., Matsumoto, H., Fowler, M. J., Haxel, J. H., Tolstoy, M., et al. (2006). Seafloor-spreading Event at 9°50′N, East Pacific Rise: Ridge dike Intrusion and Transform Fault Interactions from Regional Hydroacoustic Data, January 2009 Seafloor-Spreading Event at 9°50N, East Pacific Rise: Ridge dike Intrusion and Transform Fault Interactions from Regional Hydroacoustic Data: Geochemistry, Geophysics. Geochem. Geophys. Geosyst. 10, a–n. January. doi:10.1029/2009GC002388
Dziak, R. P., Haxel, J. H., Bohnenstiehl, D. R., Chadwick, W. W., Nooner, S. L., Fowler, M. J., et al. (2011). 2012, Seismic Precursors and Magma Ascent before Theeruption at Axial Seamount. Nature Geoscience. 5, 478–482. doi:10.1038/ngeo1490
Dziewonski, A. M., Chou, T.-A., and Woodhouse, J. H. (1981). Determination of Earthquake Source Parameters from Waveform Data for Studies of Global and Regional Seismicity. J. Geophys. Res. 86, 2825–2852. doi:10.1029/JB086iB04p02825
Ebinger, C., Ayele, A., Keir, D., Rowland, J., Yirgu, G., Wright, T., et al. (2010). Length and Timescales of Rift Faulting and Magma Intrusion: The Afar Rifting Cycle from 2005 to Present. Annu. Rev. Earth Planet. Sci. 38, 439–466. doi:10.1146/annurev-earth-040809-152333
Ebinger, C. J., Keir, D., Ayele, A., Calais, E., Wright, T. J., Belachew, M., et al. (2008). Capturing Magma Intrusion and Faulting Processes during continental Rupture. Seismicity of the Dabbahu (Afar) Rift. 174, 1138–1152. doi:10.1111/j.1365-246x.2008.03877.x
Ebinger, C., Wijk, J. V, and Keir, D. (2013). The Time Scales of continental Rifting: Implications for Global Processes. Geol. Soc. Americav. 2500, 1–26. doi:10.1130/2013.2500(11)
Edwards, M. H., Kurras, G. J., Tolstoy, M., Bohnenstiehl, D. R., Coakley, B. J., and Cochran, J. R. (2001). Evidence of Recent Volcanic Activity on the Ultraslow-Spreading Gakkel ridge. Nature 409, 808–812. doi:10.1038/35057258
Einarsson, P., and Brandsdóttir, B. (2021). Seismicity of the Northern Volcanic Zone of Iceland. Front. Earth Sci. 9. doi:10.3389/feart.2021.628967
Ekström, G., Nettles, M., and Dziewoński, A. M. (2012). The Global CMT Project 2004-2010: Centroid-Moment Tensors for 13,017 Earthquakes. Phys. Earth Planet. Interiors 200-201, 1–9. doi:10.1016/j.pepi.2012.04.002
Engdah, R., Hilst, E., Van., Der, R., and Buland, R. (1998). Global Teleseismic Earthquake Relocation with Improved Travel Times and Procedures for Depth Determination. Bulletin of the Seismological Society of America. 88, 722–743.
Ester, M., Kriegel, H. P., Sander, J., and Xu, X. (1996). A Density-Based Algorithm for Discovering Clusters in Large Spatial Database with Noise:. KDD Proc., 226–231. doi:10.1016/B978-044452701-1.00067-3
Eyles, J. H. W., Illsley-Kemp, F., Keir, D., Ruch, J., and Jónsson, S. (2018). Seismicity Associated with the Formation of a New Island in the Southern Red Sea. Frontiers in Earth Science. 6, 1–10. doi:10.3389/feart.2018.00141
Fairhead, J. D., and Girdler, R. W. (1970). The Seismicity of the Red Sea, Gulf of Aden and Afar Triangle: Philosophical Transactions of the Royal Society A. Mathematical, Physical and Engineering Sciences. 267, 49–74. doi:10.1098/rsta.1970.0023
Ghebreab, W., and Solomon, S. (1994). Formation of a Recent Asymmetric Graben-In-Graben Structure at Bada, the Danakil Depression of Eritrea. Geoscience and Development. 1, 16–18.
Goitom, B., Oppenheimer, C., Hammond, J. O. S., Grandin, R., Barnie, T., Donovan, A., et al. (2015). First Recorded Eruption of Nabro Volcano, Eritrea, 2011. Bull. Volcanol 77. doi:10.1007/s00445-015-0966-3
Gouin, P. (1979). Earthquake History of Ethiopia and the Horn of Africa (International Development Research Centre. Ottawa: Canada.258
Grandin, R., Socquet, A., Binet, R., Klinger, Y., Jacques, E., de Chabalier, J.-B., et al. (2005). September 2005 Manda Hararo-Dabbahu Rifting Event, Afar (Ethiopia): Constraints provided by Geodetic Data. J. Geophys. Res. 114. doi:10.1029/2008JB005843
Hamling, I. J., Wright, T. J., Calais, E., Bennati, L., and Lewi, E. (2010). Erratum: Stress Transfer between Thirteen Successive Dyke Intrusions in Ethiopia. Nat. Geosci 3, 806. doi:10.1038/ngeo993
Hamlyn, J. E., Keir, D., Wright, T. J., Neuberg, J. W., Goitom, B., Hammond, J. O. S., et al. (2014). Seismicity and Subsidence Following the 2011 Nabro Eruption, Eritrea: Insights into the Plumbing System of an Off-Rift Volcano. J. Geophys. Res. Solid Earth 119, 8267–8282. doi:10.1002/2014jb011395
Hébert, H., Deplus, C., Huchon, P., Khanbari, K., and Audin, L. (2001). Lithospheric Structure of a Nascent Spreading ridge Inferred from Gravity Data: The Western Gulf of Aden. J. Geophys. Res. 106, 26345–26363. doi:10.1029/2000jb900391
Hofstetter, R., and Beyth, M. (2003). The Afar Depression: Interpretation of the 1960-2000. Earthquakes. 155, 715–732. doi:10.1046/j.1365-246x.2003.02080.x
Illsley-Kemp, F., Bull, J. M., Keir, D., Gerya, T., Pagli, C., Gernon, T., et al. (2018). Initiation of a Proto-Transform Fault Prior to Seafloor Spreading, Geochemistry, Geophysics, Geosystems. 19, 4744–4756. doi:10.1029/2018GC007947
International Seismological Centre (2010). Summary of the Bulletin of the International Seismological Centre, 2013. January -June.
Jacques, E., Kidane, T., Tapponnier, P., Manighetti, I., Gaudemer, Y., Meyer, B., et al. (1989). 2011, Normal Faulting during Theearthquakes in central Afar. Sequential Triggering and Propagation of Rupture along the Dobi Graben. Bulletin of the Seismological Society of America. 101, 994–1023. doi:10.1785/0120080317
Jacques, E., King, G. C. P., Tapponnier, P., Ruegg, J. C., and Manighetti, I. (1996). Seismic Activity Triggered by Stress Changes after the 1978 Events in the Asal Rift, Djibouti. Geophys. Res. Lett., 23. Djibouti, 2481–2484. Geophysical Research Letters. doi:10.1029/96gl02261
Jacques, E., Ruegg, J. C., Lépine, J. C., Tapponnier, P., King, G. C. P., and Omar, A. (1999). Relocation ofM ≥ 2 Events of the 1989 Dôbi Seismic Sequence in Afar: Evidence for Earthquake Migration. Geophys. J. Int. 138, 447–469. doi:10.1046/j.1365-246x.1999.00881.x
Jónsson, S., and Xu, W., 2015, Volcanic Eruptions in the Southern Red Sea during 2007-2013, The Red Sea, Springer Earth System Sciences, 175–186. doi:10.1007/978-3-662-45201-1_10
Kebede, F., Kim, W.-Y., and Kuhlanek, O. (1969). Serdo Earthquake Sequence in Central Afar, Ethiopia, Deduced from Teleseismic Body Waves: V. 1989, Dynamic Source Parameters of the 94, 5603–5614.
Keir, D., Bastow, I. D., Pagli, C., and Chambers, E. L. (2013). The Development of Extension and Magmatism in the Red Sea Rift of Afar. Tectonophysics 607, 98–114. doi:10.1016/j.tecto.2012.10.015
Keir, D., Hamling, I. J., Ayele, A., Calais, E., Ebinger, C., Wright, T. J., et al. (2009). Evidence for Focused Magmatic Accretion at Segment Centers from Lateral dike Injections Captured beneath the Red Sea Rift in Afar. Geology. 37, 59–62. doi:10.1130/g25147a.1
Kennett, B. L. N., Engdahl, E. R., and Buland, R. (1995). Constraints on Seismic Velocities in the Earth from Traveltimes. Geophysical Journal International 122, 108–124. doi:10.1111/j.1365-246x.1995.tb03540.x
Kennett, B. L. N., and Gudmundsson, O. (1996). Ellipticity Corrections for Seismic Phases. Geophysical Journal International 127, 40–48. doi:10.1111/j.1365-246x.1996.tb01533.x
La Rosa, A., Pagli, C., Keir, D., Sani, F., Corti, G., Wang, H., et al. (2019). Observing Oblique Slip during Rift Linkage in Northern Afar. Geophys. Res. Lett. 46, 10782–10790. doi:10.1029/2019gl084801
Läderach, C., Korger, E. I. M., Schlindwein, V., Müller, C., and Eckstaller, A. (2012). Characteristics of Tectonomagmatic Earthquake Swarms at the Southwest Indian Ridge between 16°E and 25°E. Geophysical Journal International 190, 429–441. doi:10.1111/j.1365-246x.2012.05480.x
Le Dain, A. Y., Robineau, B., and Tapponnier, P. (1979). Les effects tectoniques de l’evenement sismique et volcanique de novembre 1978 dans le rift d’Asal-Ghoubbet. Bull. Soc. Geol. France. 7, 817–822. doi:10.2113/gssgfbull.s7-xxii.6.817
Lépine, J.-C., and Hirn, A. (1992). Seismotectonics in the Republic of Djibouti, Linking the Afar Depression and the Gulf of Aden. Tectonophysics 209, 65–86. doi:10.1016/0040-1951(92)90011-t
Leroy, S., Razin, P., Autin, J., Bache, F., d’Acremont, E., Watremez, L., et al. (2012). From Rifting to Oceanic Spreading in the Gulf of Aden: a Synthesis. Arab J. Geosci. 5, 859–901. doi:10.1007/s12517-011-0475-4
Massey, F. J. (1951). The Kolmogorov-Smirnov Test for Goodness of Fit.,. J. Am. Stat. Assoc. 46 (No. 253), 68–78. doi:10.1080/01621459.1951.10500769
Matthews, M. V., and Reasenberg, P. A. (1988). Statistical Methods for Investigating Quiescence and Other Temporal Seismicity Patterns. Pageoph 126, 357–372. doi:10.1007/bf00879003
Neal, C. A., Brantley, S. R., Antolik, L., Babb, J. L., Burgess, M., Calles, K., et al. (2019). The 2018 Rift Eruption and summit Collapse of Kīlauea Volcano. Science 363, 367–374. doi:10.1126/science.aav7046
Nobile, A., Pagli, C., Keir, D., Wright, T. J., Ayele, A., Ruch, J., et al. (2012). Dike-fault interaction during 2004 Dallol intrusion North. edge Erta Ale Ridge (Afar, Ethiopia). Geophys. Res. Lett. 39. doi:10.1029/2012gl053152
Ogubazghi, G., Ghebreab, W., and Havskov, J. (2004). Some Features of the 1993 Bada Earthquake Swarm of southeastern Eritrea. J. Afr. Earth Sci. 38, 135–143. doi:10.1016/j.jafrearsci.2003.12.002
Pagli, C., Wright, T. J., Ebinger, C. J., Yun, S.-H., Cann, J. R., Barnie, T., et al. (2012). Shallow Axial Magma Chamber at the Slow-Spreading Erta Ale Ridge. Nat. Geosci 5, 284–288. doi:10.1038/ngeo1414
Pagli, C., Yun, S.-H., Ebinger, C., Keir, D., and Wang, H. (2019). Strike-slip Tectonics during Rift Linkage. v 47, 31–34. doi:10.1130/g45345.1
Passarelli, L., Heryandoko, N., Cesca, S., Rivalta, E., Rasmid, S., Dahm, T., et al. (2018a). Magmatic or Not Magmatic? the 2015–2016 Seismic Swarm at the Long-Dormant Jailolo Volcano, West Halmahera, Indonesia. Frontiers in Earth Science. 6, 1–17. doi:10.3389/feart.2018.00079
Passarelli, L., Rivalta, E., Cesca, S., and Aoki, Y. (2015). Stress Changes, Focal Mechanisms, and Earthquake Scaling Laws for the 2000 dike at Miyakejima (Japan). J. Geophys. Res. Solid Earth 120, 4130–4145. doi:10.1002/2014jb011504
Passarelli, L., Rivalta, E., Jónsson, S., Hensch, M., Metzger, S., Jakobsdóttir, S. S., et al. (2018b). Scaling and Spatial Complementarity of Tectonic Earthquake Swarms. Earth Planet. Sci. Lett. 482, 62–70. doi:10.1016/j.epsl.2017.10.052
Peterson, D. E., Garibaldi, N., Keranen, K., Tikoff, B., Miller, C., Lara, L. E., et al. (2020). Active Normal Faulting, Diking, and Doming Above the Rapidly Inflating Laguna del Maule Volcanic Field, Chile, Imaged With CHIRP, Magnetic, and Focal Mechanism Data. J. Geophys. Res. Solid Earth 125 (8), e2019JB019329. doi:10.1029/2019jb019329
Reasenberg, P. A., and Simpson, R. W. (1992). Response of Regional Seismicity to the Static Stress Change Produced by the Loma Prieta EarthquakeAdvancement of Science. Science 255, 1687–1690. doi:10.1126/science.255.5052.1687 http://www.jstor.org/stable/2876633.
Reasenberg, P. (1985). Second-order Moment of central California Seismicity, 1969-1982. J. Geophys. Res. 90 (B7), 5479–5495. doi:10.1029/JB090iB07p05479
Reed, C. A., Almadani, S., Gao, S., Elsheikh, A., Cherie, S., Abdelsalam, M., et al. ., 2014, Receiver Function Constraints on Crustal Seismic Velocities and Partial Melting beneath the Red Sea Rift and Adjacent Regions, Afar Depression. Journal of Geophysical Research: Solid Earth119(3), doi:10.1002/2013JB010719
Roland, E., and McGuire, J. J. (2009). Earthquake Swarms on Transform Faults. Geophysical Journal International 178, 1677–1690. doi:10.1111/j.1365-246x.2009.04214.x
Ruch, J., Wang, T., Xu, W., Hensch, M., and Jónsson, S. (2016). Oblique Rift Opening Revealed by Reoccurring Magma Injection in central Iceland. Nat. Commun. 7. doi:10.1038/ncomms12352
Schlindwein, V. (2012). Teleseismic Earthquake Swarms at Ultraslow Spreading Ridges. Indicator for Dyke Intrusions? 190, 442–456. doi:10.1111/j.1365-246x.2012.05502.x
Shuler, A., and Nettles, M. (2012). Earthquake Source Parameters for the 2010 Western Gulf of Aden Rifting Episode. Geophysical Journal International 190, 1111–1122. doi:10.1111/j.1365-246x.2012.05529.x
Sykes, L. R. (1970). Earthquake Swarms and Sea-Floor Spreading. J. Geophys. Res. 75, 6598–6611. doi:10.1029/jb075i032p06598
Vidale, J. E., and Shearer, P. M. (2006). A Survey of 71 Earthquake Bursts across Southern California: Exploring the Role of Pore Fluid Pressure Fluctuations and Aseismic Slip as Drivers: Journal of Geophysical Research. Solid Earth,. 111, 1–12. doi:10.1029/2005jb004034
Viltres, R., Jónsson, S., Ruch, J., Doubre, C., Reilinger, R., Floyd, M., et al. (2018). Spatio-temporal Stress Changes Caused by Rifting Episodes in the southernRed Sea Region. Geophys. Res. Abstract 20, EGU2018–17821. 2018EGU General Assembly 2018. doi:10.1093/gji/ggaa109
Viltres, R., Jónsson, S., Ruch, J., Doubre, C., Reilinger, R., Floyd, M., et al. (2020). Kinematics and Deformation of the Southern Red Sea Region from GPS Observations. Geophysical Journal International 221, 2143–2154. doi:10.1093/gji/ggaa109
Wright, T. J., Ebinger, C., Biggs, J., Ayele, A., Yirgu, G., Keir, D., et al. (2006). Magma-maintained Rift Segmentation at continental Rupture in the 2005 Afar Dyking Episode. Nature 442, 291–294. doi:10.1038/nature04978
Xu, W., Ruch, J., and Jónsson, S. (2015). Birth of Two Volcanic Islands in the Southern Red Sea. Nature Communications. 6, 7104,. doi:10.1038/ncomms8104
Zhuang, J., Harte, D., Werner, M. J., Hainzl, S., and Zhou, S., 2012, Basic Models of Seismicity: Temporal Models, Community Online Resource for Statistical Seismicity Analysis, doi:10.5078/corssa-79905851 Available at http://www.corssa.org.
Keywords: earthquake swarm, Afar depression, seismicity analysis, Red Sea—Gulf of Aden, rifting and breakup, volcanism, tectonics
Citation: Ruch J, Keir D, Passarelli L, Di Giacomo D, Ogubazghi G and Jónsson S (2021) Revealing 60 years of Earthquake Swarms in the Southern Red Sea, Afar and the Gulf of Aden. Front. Earth Sci. 9:664673. doi: 10.3389/feart.2021.664673
Received: 05 February 2021; Accepted: 29 July 2021;
Published: 16 August 2021.
Edited by:
Luis E. Lara, Servicio Nacional de Geología y Minería de Chile (SERNAGEOMIN), ChileReviewed by:
Sarah Jaye Oliva, University of British Columbia, Vancouver, CanadaSigrún Hreinsdóttir, GNS Science, New Zealand
Copyright © 2021 Ruch, Keir, Passarelli, Di Giacomo, Ogubazghi and Jónsson. This is an open-access article distributed under the terms of the Creative Commons Attribution License (CC BY). The use, distribution or reproduction in other forums is permitted, provided the original author(s) and the copyright owner(s) are credited and that the original publication in this journal is cited, in accordance with accepted academic practice. No use, distribution or reproduction is permitted which does not comply with these terms.
*Correspondence: Joël Ruch, am9lbC5ydWNoQHVuaWdlLmNo