- 1Key Laboratory of Western China’s Environmental System (Ministry of Education), College of Earth and Environmental Sciences, Lanzhou University, Lanzhou, China
- 2British Geological Survey, Nottingham, United Kingdom
- 3Northwest Institute of Eco-Environmental and Resources Research, Chinese Academy of Sciences, Lanzhou, China
Regional humidity is important for terrestrial ecosystem development, while it differs from region to region in inland Asia, knowledge of past moisture changes in the lower basin of northern Xinjiang remains largely unclear. Based on a pollen record from Jili Lake, the Artemisia/(Amaranthaceae + Ephedra) (Ar/(Am + E)) ratio, as an index of regional humidity, has recorded four relatively dry phases: 1) 400 BCE to 1 CE, 2) the Roman Warm Period (RWP; c. 1–400 CE), 3) the Medieval Warm Period (MWP; c. 850–1200 CE) and 4) the Current Warm Period (CWP; since 1850 CE). In contrast, the Dark Age Cold Period (DACP; c. 400–850 CE) and the Little Ice Age (LIA; c. 1200–1850 CE) were relatively wet. Lower lake levels in a relatively humid climate background indicated by higher aquatic pollen (Typha and Sparganium) after c. 1700 CE are likely the result of intensified irrigation for agriculture in the catchment as documented in historical records. The pollen Ar/(Am + E) ratio also recorded a millennial-scale wetting trend from 1 CE to 1550 CE which is concomitant with a long-term cooling recorded in the Northern Hemisphere.
Introduction
During the Holocene, the “westerlies-dominated climatic regime” (WDCR) is characterized by warm-dry and cold-wet phases in inland Asia on different timescales (Chen et al., 2016; Chen et al., 2019). On millennial timescales, the moisture in the WDCR gradually increased, with the wettest period occurring in the late Holocene, while in arid central Asia (ACA), the wettest phase occurred in the mid-to late Holocene (Chen et al., 2016; Chen et al., 2019). At centennial timescales, the WDCR was generally dry during the Medieval Warm Period (WMP) and relatively wet during the Little Ice Age (LIA), but there were some exceptions in Xinjiang (Chen J. et al., 2015), an important part of the WDCR. It has been suggested that the moisture balance has changed from warm-dry to warm-wet over the last few decades (Shi et al., 2006; Wang et al., 2007). Paleoclimate records from the region that span the late Holocene are mostly located at high altitudes (Huang et al., 2018; Lan et al., 2018; Yang et al., 2019); the lack of paleoclimatic records from low altitude areas, where most people are living, hampers understanding of regional climate change both in the past and into the future.
Recent global warming has resulted in the expansion of drylands around the world, and seriously threatens freshwater resources (Huang et al., 2015; Fan et al., 2020). Many studies suggest a major impact of climate change on regional human activities, with evidence that climate change plays an important role in the abandonment of cities (e.g., Bhattacharya et al., 2015; An et al., 2017; Yao Y.-F. et al., 2020; Jenny et al., 2020). In the Xinjiang area, early cultivation of crops began around 3000 BCE (Li Y., 2020), and an increase in population started at c. 2000 BCE. There is evidence for a widespread prehistoric culture in Xinjiang area, associated with a relatively wetter climate during the late Holocene (An et al., 2019). However, abrupt climate and environment changes might have led to the collapse of some civilizations, such as Xiaohe Culture (Zhang et al., 2017) and Loulan civilization (Fontana et al., 2019; Hao et al., 2019). A diatom record from Bosten Lake indicated that changes in hydro-climate was the main reason for the collapse of the Loulan Kingdom (Fontana et al., 2019). The record of RIK37 index from Sayram Lake suggested that humid conditions might have been conducive to the spread of the Mongol Empire across the ACA area during 1206–1260 CE (Yao Y. et al., 2020). Pollen records from Tian’E Lake in the Qilian Mountains suggested that continuous droughts were an important driving force of the abandonment of several archaeological sites and ancient cities along the Silk Road (Zhang J. et al., 2018). However, there are few humid records from the Northern Xinjiang area that span the last 2,000 years, which can be used to understand the relationship between human activities and climate change.
Lake sediments are an excellent archive of past climate change (Birks et al., 2012), and pollen analysis from lacustrine sediments can help to understand the regional climatic changes through time (Bartlein et al., 1986; Wen et al., 2010). Changes in the relative abundance of fossil pollen preserved in lake sediment archives reflect changes in vegetation in response to various driving force (e.g., precipitation, temperature, human impact), and pollen assemblages from lakes with a larger catchment reflect regional, not just local, changes in climate and vegetation (Sugita, 1994; Nielsen and Sugita, 2005; Xu et al., 2016). Here, we present the results of pollen analysis from Jili Lake (174.0 km2) located in the northern Xinjiang area to infer the history of climate and vegetation change over the last c. 2400 years. By combining these data with other evidence, for example, charcoal and historical documents (e.g., regional population and cultivation history), we explore the relationship between environment and human activities in this study area.
Study Area
Jili lake (46°51′–47°00′ N, 87°20′–87°32′ E, 483 m a.s.l.) is a large and shallow lake in the inland area of the Eurasian continent, located in the Junggar Basin between the Tianshan Mountains and the Altai Mountains (Figures 1A, B). The study area sits within a mid-latitude temperate continental climate. As recorded at the nearby Fuhai meteorological station (47°06′ N, 87°21′ E, Figure 1C), the mean annual temperature in the region is 4.7°C and the average annual precipitation is 131 mm (Figure 1D). Jili Lake receives water mainly from the Ulungur River from the Altai Mountains to the north, and it outflows to Ulungur Lake (Figures 1B,C). In 2015, the lake covered a surface area of c. 170 km2, and had an average depth of c. 10 m (Wang and Dou, 1998; Liu et al., 2018).
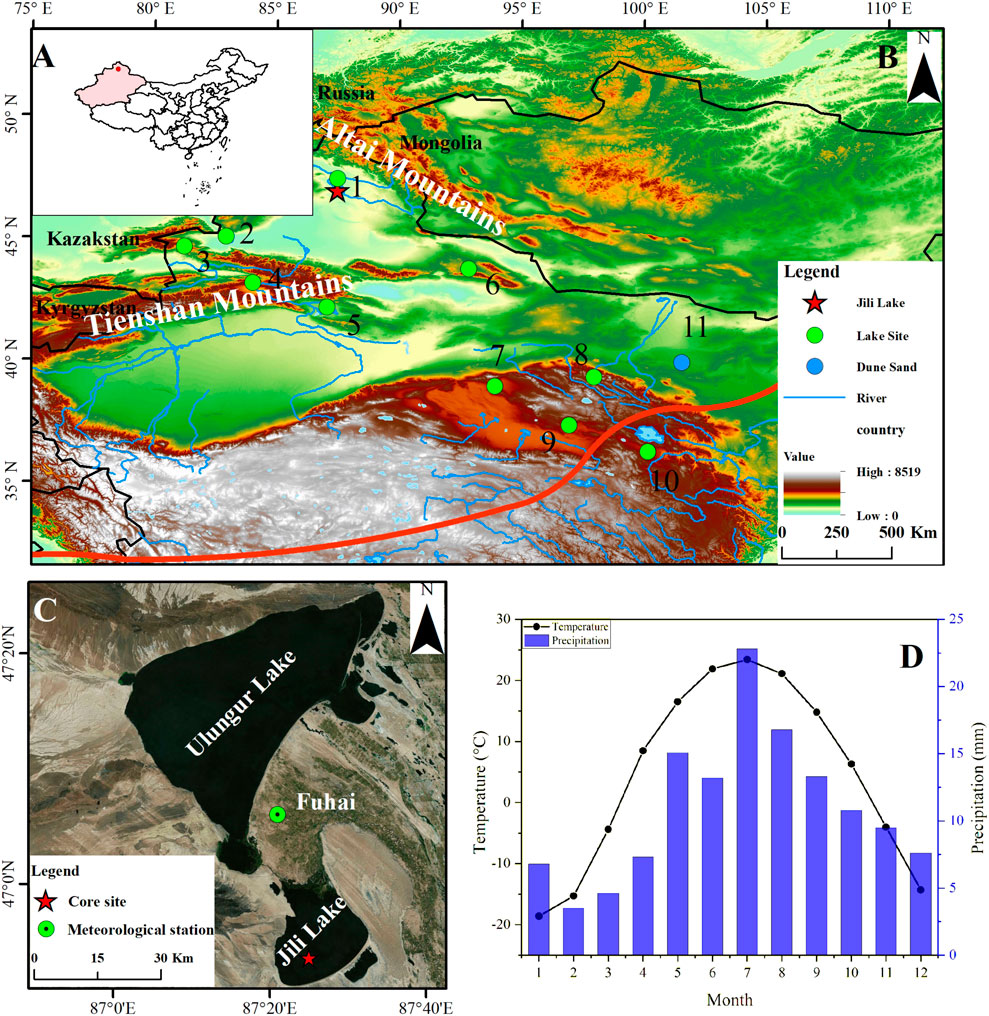
FIGURE 1. Environmental setting of Jili Lake. (A) The study area; (B) Location of Jili Lake and other lake sites mentioned in this study: 1 Ulungur Lake; 2 Ebinur Lake; 3 Sayram Lake; 4 Harnur Lake; 5 Bosten Lake; 6 Balikun Lake; 7 Sugan Lake; 8 Tian’E Lake; 9 Hurleg Lake; 10 Genggahai Lake; and 11 BYBS profile. The orange line is the modern limit of the Summer Monsoon (Chen et al., 2008). (C) Google Earth image of Jili Lake and Ulungur Lake area, and (D) Monthly temperature and precipitation from Fuhai meteorological station (elevation 500 m a.s.l.) for the years 1981–2010 CE.
In recent decades the surface area of Jili Lake has expanded, and its main water supply comes from the Ulungur river; the water level in Jili Lake has been affected by dam construction and intensified water exploitation (Li et al., 2015; Cheng et al., 2016). Desert vegetation in the vicinity of Jili Lake comprises mostly Amaranthaceae, but the northern part of the catchment mainly has many shrub-coppice covered dunes. The Gobi Desert area lies to the west, and a shrub-coppice dune chain to the south (Lang, 2020). In the Jili Lake area, Amaranthaceae and Artemisia accounted for more than 60% of the surface pollen sum, except for Ephedra, Tamarix, Jujube, Nitraria, Poaceae and Allium (Yan and Xu, 1989). The aquatic plants mainly consist of Phragmites, Myriophyllum spicatum and Potamogeton pectinatus, which are distributed in shallow water areas along the lake shore (Wang et al., 1981; Wang and Dou, 1998).
Materials and Methods
Core Sampling and Age-Depth Model
In February 2018 a sediment core, 253 cm in length (JL18-02-A), was collected from the southwestern area of Jili Lake using a Piston corer. The core was frozen and transported to Lanzhou University, sampled at a 1-cm intervals, and freeze-dried. The lithology of the c. 120 cm of the core can be divided into three intervals: 0–6 cm - cyan mud with loose sediments; 6–34 cm - the color of sediments is brown; 34–123 cm - pale silty clay with relatively compact sediments.
We focused on the upper 120 cm of the core because there was a hiatus in the sediment below this depth based on 7 radiocarbon dates (Lang, 2020). For the age-depth model of the upper 120 cm, three bulk organic samples from the core were processed for accelerator mass spectrometry (AMS) radiocarbon dating at the Beta Analytic Radiocarbon Dating Laboratory in Miami, Florida, United States (Table 1) (Lang, 2020). The 14C specific activity values of the lake-water DIC sample and the samples from the upper most layers of cores largely approximate to the pMC (percent modern carbon) values of the modern atmosphere (105.4 ± 1.0 pMC in 2007; Fellner and Rechberger, 2009), indicating that the CO2 exchange between the lake and atmosphere tends to be balanced, reflecting that the “reservoir effect” of the lake water is low. The age-depth model for Jili Lake (Figure 2) was calculated using “Clam” version 2.2 (Blaauw, 2010) and the IntCal13 calibration curve (Reimer et al., 2013). The core depth-chronology has a linear relationship and it provides a record of sediment deposition over the last 2400 years.
Pollen and Charcoal Analyses
A total of 96 samples were taken from the core for pollen analysis. Pollen grains were extracted from 1–3 g of dried sample, and preparation used HCl (10%) and HF (40%) to remove carbonates and silicates (Fægri and Iversen, 1989). Samples were sieved through a 10-μm mesh to remove small clay-sized particles, and clean samples were mounted in glycerin on glass slides. Pollen grains and charcoal particles were identified and counted using a Nikon ECLIPSE 80i optical microscope at ×400 magnification. More than 500 pollen grains and 300 charcoal particles were counted for each sample, and charcoal particles were grouped by long axis length: >100, 50–100, and <50 μm. To calculate pollen and charcoal concentration, one Lycopodium tablet (27,637 grains) was added to each sample prior to chemical pre-treatment (Maher, 1981). The percentages of pollen were calculated based on the sum of all counted terrestrial pollen grains, and the aquatic pollen percentages were calculated based on the sum of all counted pollen grains. The pollen diagrams were plotted using Tilia software (Grimm, 2011), and the pollen zones were divided by stratigraphically constrained cluster analysis using CONISS (Grimm, 1987).
Indicators of Climatic Humidity and Human Activities
Based on the investigations of modern surface pollen in northern Xinjiang, it has shown that Artemisia and Amaranthaceae (old name is Chenopodiaceae) (APG, 1998), are dominant species in desert-steppe and desert areas (Yu et al., 1998; Luo et al., 2009; Li et al., 2017). A lot of modern pollen investigations have shown that the Artemisia and Chenopodiaceae ratio (A/C ratio) is a valid indicator/proxy of humidity in desert and desert-steppe areas (e.g., El-Moslimany, 1990; Sun et al., 1994; Huang et al., 2009; Zhao et al., 2009; Li et al., 2010; Zhao et al., 2012; Zhang D. et al., 2018). As Ephedra is also one of the main taxa in desert areas like Amaranthaceae (Huang et al., 2009; Huang et al., 2018), here we use the sum of Amaranthaceae and Ephedra (Am + E) to indicate a relatively dry condition, and use the ratio of Artemisia (Ar) and (Am + E) as a new indicator of climatic humidity like A/C ratio.
The signal of regional human activity can be indicated by some specific pollen types and charcoal abundance. For example, Poaceae pollen grains among the 35–50 μm size range were considered to be cereal-type Poaceae (mostly might be wheat pollen), which can be used as an indicator of agricultural activity (Li et al., 2008; Li et al., 2012). Charcoal is a particularly useful proxy for recording the disturbance of vegetation by humans, in which macro-charcoal (>100 μm) could indicate changes in local fire occurrence in the past (Whitlock and Larsen, 2001; Li et al., 2008).
Results
A total of 62 pollen taxa and spore types were identified and 53477 pollen grains were counted, with an average of 557 pollen grains per sample. The main herbaceous taxa were Artemisia, Amaranthaceae, Poaceae and Asteraceae. Across the three zones as identified by CONISS, the percentages of Artemisia and Amaranthaceae exceeded 80% of the terrestrial pollen sum. The arboreal taxa with lower percentages were mainly Betula and Pinus. The percentage abundance of fern spores was very low, and aquatic pollen types were mainly Sparganium and Typha. The assemblage characteristics of each pollen zone are briefly described as follows (Figure 3).
Zone Ⅰ (119–87 cm, c. 380 BCE-400 CE)
The major pollen types included Asteraceae (0.2–3.6%, mean 2.3%), Ephedra (0.6–3.09%, mean 2%), Polygonaceae (0.2–3.6%, mean 2.74%), Rosaceae (1.0–5.8%, mean 2.1%), and Typha (1.0–2.8%, mean 1.60%). The sum of tree pollen types is c. 5.1% and including Pinus, Picea, Betula and Salix. The abundance of Poaceae (0.3–2.5%, mean 1.4%) and Cyperaceae (0–0.9%, mean 0.3%) was low, and the Ar/(Am + E) ratio (0.2–0.7, mean 0.4) had the lowest value in the whole core sequence. Overall, this zone had the lowest pollen concentration (c. 18873 grains/g). The pollen assemblages in this zone, can be further divided into two sub-zones. Sub-zone Ⅰ-1 (119–106 cm, c. 380–70 BCE) can be readily distinguished from sub-zone Ⅰ-2 (106–87 cm, c. 70 BCE-400 CE) by the decreasing percentage of Poaceae pollen.
Zone Ⅱ (87–40 cm, c. 400–1350 CE)
This zone was typified by an increase in Poaceae (0.5–13.2%, mean 6.5%) and Cyperaceae (0.2–1.5%, mean 0.8%) at the expense of Artemisia (mean 34%), Asteraceae (0.5–2.6%, mean 1.4%), and Rosaceae (0–2.4%, mean 0.5%) whose percentages decreased. This zone also sees the first appearance of cereal-type Poaceae (0–0.6%, mean 0.3%).
This zone can be further divided into two sub-zones. Sub-zone Ⅱ-2 (52–40 cm, c. 1160–1350 CE) can be readily distinguished from Sub-zone Ⅱ-1 (86–52 cm, c. 400–1160 CE) by the following features: further increases in Artemisia (32.6–41.7%, mean 36.8%), Poaceae (5.6–13.2%, mean 9%) percentages and Ar/(Am + E) ratio (0.6–1.1, mean 0.8) and the presence of cereal-type Poaceae/Poaceae, Amaranthaceae (37.3–52.3%, mean 43%), and a decrease in pollen concentration (now with a mean 82166 grains/g).
Zone Ⅲ (40–0 cm, c. 1350 CE to Present)
There were no obvious changes in the percentage of Poaceae pollen (3.1–9.8%, mean 6.7%) but there was a higher abundance of Cyperaceae pollen (0.4–2.2%, mean 1.1%) compared to the previous zone. In Zone Ⅲ, there was an obvious increase in aquatic pollen (Typha and Sporangium) percentages (0.4–6.5%, mean 2.3%), tree pollen percentages (0.8–9.6%, mean 3.9%) and Cereal-type Poaceae/Poaceae (0–33.3%, mean 10.6%). The Ar/(Am + E) ratio was relatively higher (0.6–1.1, mean 0.8), but was interrupted by a brief period (c. 1550–1700 CE) with lower values (0.7–0.8, mean 0.7).
Sub-zone Ⅲ-2 (23–0 cm, c. 1650 CE to present) is distinguished from sub-zone Ⅲ-1 (40–23 cm, c. 1350–1650 CE) by an apparent increase in Artemisia (30.7–42.3%, mean 32.3%), Salix (0.2–2.7%, mean 0.9%), Sparganium (1.8–6.5%, mean 2.6%), Rosaceae (0–2.3%, mean 1.0%), and Cereal-type Poaceae/Poaceae (0–33.3%, mean 12.1%) and a slight decrease in Poaceae (3.1–9.8%, mean 6.1%).
Discussion
Pollen Source Area and a Test of Pollen Humidity Indicator of Jili Lake
The size of pollen source area depends on the size of the depositional basin, characteristics of pollen types and spatial distribution of the plant species (Jackson, 1990; Sugita, 1994). Sugita (1994) suggests that pollen assemblages of sedimentary basins with a radius larger than 750 m is mainly influenced by regional pollen rather than local pollen, and hence we considered the pollen assemblages in Jili Lake (∼174 km2) can reflect regional vegetation variations. Because of strong aeolian activity in Northern Xinjiang, we suggested pollen assemblages are a mixed signal to the regional vegetation in the basin. As modern vegetation types show there are less forest around Jili Lake except some specific planted poplar trees to protect farmlands (Xinjiang Comprehensive Investigation Team, Chinese Academy of Sciences, 1978), the tree pollen types in the lake could have been transported by wind and/or river from the southern slopes of the Altai Mountains.
The Sparganium is adapted to growing in wet conditions, and the high content of Sparganium might indicate a low lake level (Davis, 1999). The aquatic plants in JL18-02-A core from Jili Lake were mainly Typha and Sparganium, both of which are emergent plants. A previous study showed the higher content of aquatic pollen and lower total pollen concentration may indicate a shallower lake environment (Huang et al., 2010).
To verify the reliability of Ar/(Am + E) ratio as an indicator of climatic humidity, we compared it to the relative humidity records from meteorological stations (Figure 4). It was demonstrated that the Ar/(Am + E) ratio fluctuations of JL18-02-A generally follow the relative humidity records from the Fuhai station, both showing an increasing trend in humidity during the 1950–2018 CE (Figure 4).Therefore, the Ar/(Am + E) ratio was a reliable indicator of humidity in this region, which is similar to A/C ratio used by many other previous studies (e.g., Huang et al., 2009; Zhang J. et al., 2018).
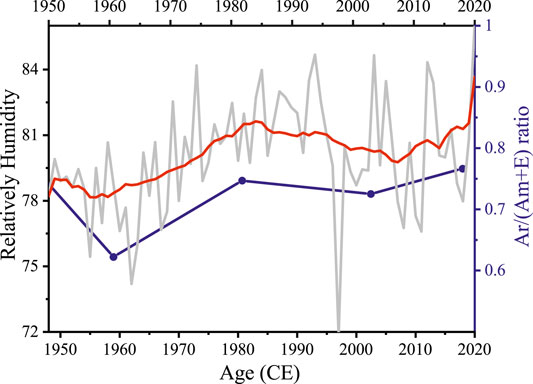
FIGURE 4. Comparison of Ar/(Am + E) for the core JL18-02-A with various Relative Humidity records from the Fuhai station (data from https://psl.noaa.gov/data/index.html): grey line: relative humidity; red line: relative humidity result of 25-point smoothing; blue dot line: Ar/(Am + E) ratio.
Comparison of Regional Moisture Changes in the Late Holocene
The moisture variations inferred from the Ar/(Am + E) ratio from Jili Lake (Figure 5A) over the last 2400 years is consistent with other regional records (e.g., Sayram Lake (Figure 5C) and Ebinur Lake (Figure 5D), and suggested that an increase in regional moisture, which is consistent with an increase in the average moisture index of Xinjiang towards the present (Wang et al., 2013). The nearby Lake Ulungur (Figure 5B) also showed an increase in moisture prior to 1450 CE, but then decreased sharply (Liu et al., 2008).
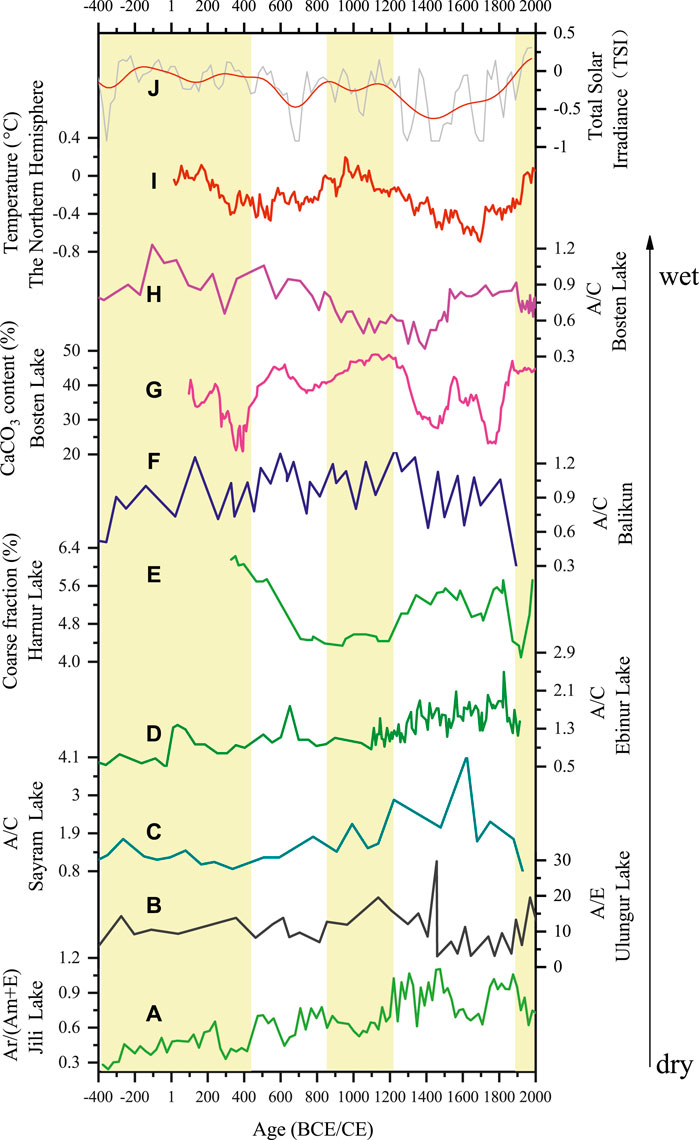
FIGURE 5. Comparison of Ar/(Am + E) for the core JL18-02-A with various paleoclimatic records: (A) Ar/(Am + E) ratio from Jili Lake (this study); (B) A/E ratio from Ulungur Lake (Liu et al., 2008); (C) A/C ratio from Sayram Lake (Jiang et al., 2013); (D) A/C ratio from Ebinur Lake (Wang et al., 2013); (E) Coarse fraction from Harnur Lake (Lan et al., 2018); (F) A/C ratio from Balikun Lake (Tao et al., 2010); (G) CaCO3 content from Bosten Lake (Fontana et al., 2019); (H) A/C ratio from Bosten Lake (Huang et al., 2009); (I) Temperature in the Northern Hemisphere (Ljungqvist, 2016); (J) Reconstructed Total Solar Irradiance (TSI) (Steinhilber et al., 2012).
From 400 BCE-400 CE, there is lower water level and lower effective moisture recorded in Jili Lake, which is also seen in other records. For instance, climate conditions were drier at Sayram Lake (Figure 5C) and Ebinur Lake (Figure 5D) during this period (as interpreted from a low A/C ratio). This period of drying is coincident with strong solar activity (Figure 5J), and the known warming associated with the Roman Warm Period (RWP, 0–400 CE) (Biintgen et al., 2011). In low-elevation areas in northern Xinjiang, there are many sites that suggest a dry climate during the RWP (Feng et al., 2017). In the Aral Sea area, there was a period of low lake level from 1 CE to 425 CE (Sorrel et al., 2006), and at Bosten Lake, a period of high salinity and lower lake level occurred from 280 to 480 CE (Fontana et al., 2019; Li et al., 2021). Historical documents show that in the 4th Century, the city of the Loulan Kingdom experienced a severe drought (Li et al., 1991), which might also have been recorded in Jili Lake, as indicated by a lower pollen humidity index (PHI) at 300–400 CE. The results of lithological and grain-size analyses from Jili Lake suggest the lake was shallower before 400 CE, hence the hydrodynamics caused by wave action on the lake was relatively strong (Lang, 2020). We interpret the low pollen concentration as evidence of strong hydrodynamics and/or drier conditions. There are also differences in the records of humidity at different altitudes in the Xinjiang region. From 400 BCE to 400 CE, a decrease in moisture occurred in high altitude areas [e.g., Tielishahan Peat (Zhang et al., 2016) and Narenxia Peat (Zhang D. et al., 2018), Yushenkule Peat (Yang et al., 2019), and Sayram Lake (Lan et al., 2020)], while a slight increase in moisture occurred in low altitude areas [e.g., Jili Lake (Figure 5A), Ulungur Lake (Figure 5B) and Ebinur Lake (Figure 5D)]. In addition, during this period moisture records from Bosten Lake in the southern Tienshan Mountains (Figure 5H; Huang et al., 2009) showed a slight decreasing in moisture during this period, which is inconsistent with low latitude lakes in northern Xinjiang.
From 400 CE to 850 CE [Dark Ages Cold Period (DACP)], the PHI indicates an increase in regional moisture, overlain by fluctuations in wet and dry phases at a centennial scale. The water level in Jili Lake began to increase, likely reaching its present-day level after c. 400 CE (Lang, 2020). Similarly, the moisture record from Ebinur Lake (Figure 5D) shows an increase in regional moisture, and pollen records from Kanas Lake suggests an increase in effective humidity from 550–1050 CE (Huang et al., 2018).
During the MWP (850–1200 CE), the climate was warm and dry. Although warm climatic conditions prevailed in the Northern Hemisphere (Figures 5I, J), the PHI suggests relatively dry conditions in the Jili Lake region. These drier conditions may be related to a period of higher evaporation at Bosten Lake, and also coincided with a glacial retreat event recorded at Karakuli Lake (Liu et al., 2014). During this period, there is a higher content of coarse grain sizes (40–200 μm) from Jili Lake suggests an intensification of storm activity at Jili Lake (Lang, 2020). Likewise, the increased coarse fraction in the record from Harnur Lake (Figure 5E) and decreased A/C ratios at Ebinur Lake (Figure 5D) corroborate this interpretation.
During the Little Ice Age (LIA: 1200–1850 CE), there was an increase in moisture from 1200 CE (Figure 5A), consistent with the A/C ratios of Sayram Lake (Figure 5C), Ebinur Lake (Figure 5D) and Harnur Lake (Figure 5E). Cold climate also recorded in Manas Lake (Song et al., 2015) and Bosten Lake (Figure 5G) during this period. This period is not characterized by uniform wet conditions. For example, the period 1560–1700 CE was relatively dry with a lower PHI, though the regional climate was still wetter than the period prior to 1200 CE (Figure 5A). At Jili Lake the abundance of aquatic pollen (Typha and Sparganium) peaked at 1665 CE, with a brief interval of lower water level at 1600–1855 CE. Other regional records also attest to relatively dry conditions during 1560–1700 CE: Sichanghu peatland, Hutubi River Basin, Manas Lake, Kesang Cave and Dalong Pond (Chen F. et al., 2015; Song et al., 2015; Cai et al., 2017; Lan et al., 2019; Ren et al., 2019). At Bosten Lake, the carbonate content (Figure 5G) suggests an interval of high evaporation during 1550–1700 CE; higher temperatures are recorded at the same times [e.g., at Manas Lake (Song et al., 2015) and Belukha glacier (Eichler et al., 2011)], suggesting that temperature was the main driving force of decreased moisture during this period. After 1850 CE, the PHI derived from the Jili Lake record suggests a drier climate, which is consistent with the records of other lakes in northern Xinjiang (Huang et al., 2009; Feng et al., 2016; Li et al., 2017; Yang et al., 2019; Yang et al., 2020).
Previous studies from northern Xinjiang showed that the region alternated between “cold-wet” and “warm-dry” during the late Holocene (Feng et al., 2006). Combining the Northern Hemisphere temperature (Figure 5I) with the PHI derived from the Jili Lake record, there is support for the climate model of “warm-dry” and “cold-wet” (Chen et al., 2019), overlain by a millennial timescale wetting trend from 1 CE to 1550 CE.
By comparison of regional moisture changes with records in monsoon marginal region (Figure 6), it was found that there was an increasing trend in moisture at Jili Lake (Figure 6A) during 400 BCE-2000 CE, which is in contrast to records from Hurleg Lake (Figure 6E) and Genggahai Lake (Figure 6F). The reason for these differences may be the climate of Hurleg Lake and Genggahai Lake being mainly driven by the Asia Summer Monsoon (Liu et al., 2016; Zhao et al., 2010). Records from Tian’E Lake (in the Qilian Mountains) and BYBS profile (in the western part of the Badain Jaran Desert) show a decrease in moisture during 400 BCE-1100 CE and an increase in moisture from 1100 BCE to 1800 CE, which was in antiphase to the moisture record at Sugan Lake (Figures 6B–D). An increase in moisture during 1100–1800 CE at Tian’E Lake and BYBS profile may be due to the weakening of the Asian Summer Monsoon caused by the reducing solar radiation (Steinhilber et al., 2012; Gao et al., 2020). In general, a wetting record from Jili Lake over 2400 years (Figure 6A) is obviously different from that in the monsoon marginal region.
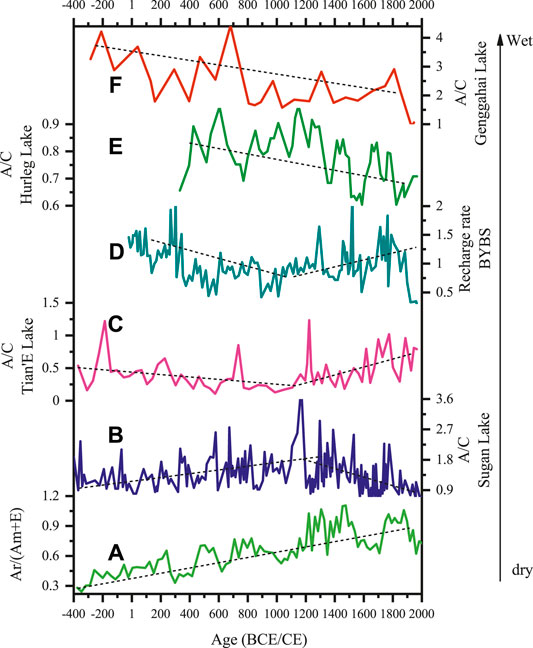
FIGURE 6. Comparison of Ar/(Am + E) ratio for the core JL18-02-A with various paleoclimatic records in the monsoon marginal region: (A) Ar/(Am + E) ratio from Jili Lake (this study); (B) A/C ratio from Sugan Lake (Li, 2020); (C) A/C ratio from Tian’E Lake (Zhang J. et al, 2018); (D) Recharge rate from BYBS profile (Ma et al., 2009); (E) A/C ratio from Hurleg Lake (Zhao et al., 2010); (F) A/C ratio from Genggahai Lake (Liu et al., 2016).
The water vapor in ACA originates from the North Atlantic Ocean, the Mediterranean Sea, Caspian Sea, and regional water recycling within inland Asia, and is transported from west to east by the westerlies (Aizen et al., 2006; Chen et al., 2008). The southward shift of the westerly jet stream facilitates the infiltration of water vapor from the Indian Ocean to ACA and leads to more rainfall over northern Xinjiang (Yang and Zhang., 2008; Zhao et al., 2014). Solar activity plays an important role in atmospheric circulation (Reid, 1991; Steinhilber et al., 2012). Yan et al. (2019) suggesting that changes in solar activity and the intensity and location of the westerly jet stream are the dominant control on hydroclimatic variations in ACA. The stronger westerly and the southern migration of the westerly jet stream, which corresponds to lower total solar irradiance (TSI) and colder conditions, could favor more water vapor transport to ACA, and vice versa (Yan et al., 2019). During 400 BCE-400 CE and the MWP, the Jili Lake region had a warm-dry climate when the solar activity was higher (Figures 5A, J). During the LIA, there was a period of higher evaporation (1560–1700 CE) and an associated low moisture index.
Human Activity in the Region and Its Impact on the Lake Level of Jili Lake
Since 1700 CE, there were higher concentrations of macro-charcoal (>100 μm) (Figure 7C), accompanied by an increase in the Ce/Po ratio (Figure 7E). These indicators suggest that a period of intensified human activity played an important role in influencing the vegetation in the region, and this can be matched with local and state historical documents. The population of Xinjiang gradually increased during 1626–1760 CE (Figure 7F). Irrigation for agriculture in northern Xinjiang began in the early Qing Dynasty (from 1716 CE) (Zhao and Xie, 1988) with a rapid phase of development during 1749–1840 CE (Fang, 1989). After 1755 CE, there was an upsurge in urban construction in northern Xinjiang, driven by political, economic and military factors, as well as expended cultivation and trade development (Yang, 2018). Likewise, during the 1600–1850 CE, Jili Lake had a lower lake level as indicated by the higher percentage of aquatic pollen (Typha and Sparganium) (Figure 7B) and the lower pollen concentration (Figure 7D). Notably, the PHI derived from the Jili Lake sediments was relatively higher during 1700–1850 CE and indicated relatively wet conditions (Figure 7A), which is in contrast to the lower lake level. Therefore, the wetter climate during 1700–1850 can’t explain the lower lake level and we infer that the lower water level in Jili Lake during 1600–1850 CE may have been caused by human activities or the more extensive exploitation of water resources. Similarly, the decline of water level in Ulungur Lake was likely caused by the greatly increased regional population and the associated development of oasis irrigation agriculture along the rivers in the lower basin during the Ming and Qing Dynasties (Liu et al., 2008; Tuerhong, 2011; Ni et al., 2021). Consequently, lower lake level may be mainly influenced by stronger irrigation agricultural activities during 1700–1850 CE.
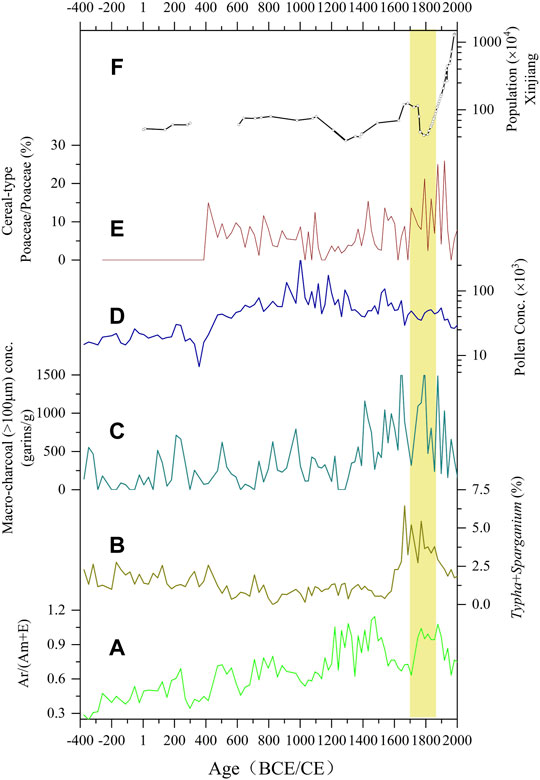
FIGURE 7. Comparison of Ar/(Am + E) ratio for the core JL18-02-A with population in Xinjiang: (A) Ar/(Am + E) ratio from Jili Lake (this study); (B) The percentage of Typha and Sparganium from Jili Lake (this study); (C) Macro-charcoal (>100 μm) concentration from Jili Lake (this study); (D) Pollen concentration form Jili Lake (this study, Y-axis is a log scale); (E) Cereal-type Poaceae/Poaceae (Ce/Po) from Jili Lake (this study); (F) Population in Xinjiang, Y-axis is a log scale (Zhao and Xie, 1988). The orange area indicates a population growth period.
Conclusion
The high-resolution pollen record from core JL18-02-A reveals the evolution of vegetation in Jili Lake and its surrounding area over the last 2400 years in response to climate drivers and human impact. The comparison of moisture availability, as inferred by the Ar/(Am + E) ratios to other regional records suggest that the moisture in the northern Xinjiang region study area shows an increase of humidity from 1 CE to 1550 CE, corresponding to a long-term cooling in the Northern Hemisphere. Pollen assemblages indicated that regional vegetation dominated by desert gradually shifted into a desert steppe. The moisture was characterized by the “warm-dry” periods of RWP (c. 1 to c. 400 CE), MWP (c. 850 to c. 1200 CE) and CWP (since 1850 CE), and the “cold-wet” periods of DACP (c. 400 to c. 850 CE) and LIA (c. 1200 to c. 1850 CE). Over the last 2400 years, the monsoon had little influence on moisture changes in the Jili Lake basin. Notably, during 1700–1850 CE, the increase of the percentages in aquatic pollen (Typha and Sparganium) and cereal-type Poaceae pollen reflecting anthropogonic impacts, and the rise of macro-charcoal and population may result from the intensified irrigation for agriculture in the catchment.
Data Availability Statement
The raw data supporting the conclusions of this article will be made available by the authors, without undue reservation.
Author Contributions
XH conceived this study. YX and LX identified the pollen. YX, LX, and XH wrote and revised the manuscript. KM, JZ, YL, and XC discussed the data. KM improved the language.
Funding
This study was funded by the National Key Research and Development Program of China (Grant No. 2017YFA0603403), Sub-project “Climatic and environmental evolution in the QTP for the past 40,000 years” (Project No. XDA2009000003) and National Natural Science Foundation of China (award 41991251).
Conflict of Interest
The authors declare that the research was conducted in the absence of any commercial or financial relationships that could be construed as a potential conflict of interest.
The handling editor declared a shared affiliation with the authors (JZ, YX, LX, and XH) at time of review.
Publisher’s Note
All claims expressed in this article are solely those of the authors and do not necessarily represent those of their affiliated organizations, or those of the publisher, the editors and the reviewers. Any product that may be evaluated in this article, or claim that may be made by its manufacturer, is not guaranteed or endorsed by the publisher.
Acknowledgments
We thank Prof. Mingrui Qiang from South China Normal University for coring the samples. We gratefully acknowledge the editor Prof. Zhuolun Li, and the two reviewers Prof. Chun Mei Ma and Prof. John Dodson for extensive comments and helpful revisions.
References
Aizen, V. B., Aizen, E. M., Joswiak, D. R., Fujita, K., Takeuchi, N., and Nikitin, S. A. (2006). Climatic and Atmospheric Circulation Pattern Variability from Ice-Core Isotope/geochemistry Records (Altai, Tien Shan and Tibet). Ann. Glaciol. 43, 49–60. doi:10.3189/172756406781812078
An, C., Wang, W., Duan, F., Haung, W., and Chen, F. (2017). Environmental Changes and Cultural Exchange between East and West along the Silk Road in Arid Central Asia (In Chinese). Acta Geogr. Sin. 72, 875–891. doi:10.11821/dlxb201705009
An, C., Zhang, M., Wang, W., Liu, Y., Duan, F., and Dong, W. (2019). The Pattern of Xinjiang Physical Geography and its Relationship with the Temporal-Spatial Distribution of Agriculture and Husbandry (in Chinese). Sci. Sin.-Terrae 50, 295–304. doi:10.1360/SSTe-2019-0050
APG (1998). An Ordinal Classification for the Families of Flowering Plants. Ann. Mo. Bot. Gard. 85, 531–553. doi:10.2307/2844848
Bartlein, P. J., Prentice, I. C., and Webb, T. (1986). Climatic Response Surfaces from Pollen Data for Some Eastern North American Taxa. J. Biogeogr. 13, 35–37. doi:10.2307/2844848
Bhattacharya, T., Byrne, R., Böhnel, H., Wogau, K., Kienel, U., Ingram, B. L., et al. (2015). Cultural Implications of Late Holocene Climate Change in the Cuenca Oriental, Mexico. Proc. Natl. Acad. Sci. USA 112, 1693–1698. doi:10.1073/pnas.1405653112
Buntgen, U., Tegel, W., Nicolussi, K., Mccormick, M., Frank, D., Trouet, V., et al. (2011). 2500 Years of European Climate Variability and Human Susceptibility. Science 331, 578–582. doi:10.1126/science.1197175
Birks, H. J. B., Lotter, A. e. F., and Smol, S. J. J. P. (2012). Tracking Environmental Change Using Lake Sediments: Data Handling and Numerical Techniques. Dordrecht: Springer. doi:10.1111/j.1365-2427.2004.01211.x
Blaauw, M. (2010). Methods and Code for 'classical' Age-Modelling of Radiocarbon Sequences. Quat. Geochronol. 5 (5), 512–518. doi:10.1016/j.quageo.2010.01.002
Cai, Y., Chiang, J. C. H., Breitenbach, S. F. M., Tan, L., Cheng, H., Edwards, R. L., et al. (2017). Holocene Moisture Changes in Western China, Central Asia, Inferred from Stalagmites. Quat. Sci. Rev. 158, 15–28. doi:10.1016/j.quascirev.2016.12.014
Chen, F., Yu, Z., Yang, M., Ito, E., Wang, S., Madsen, D. B., et al. (2008). Holocene Moisture Evolution in Arid central Asia and its Out-of-phase Relationship with Asian Monsoon History. Quat. Sci. Rev. 27, 351–364. doi:10.1016/j.quascirev.2007.10.017
Chen, F., Yuan, Y.-j., Wei, W.-s., Yu, S.-l., Zhang, T.-w., Shang, H.-m., et al. (2015a). Tree-ring Recorded Hydroclimatic Change in Tienshan Mountains during the Past 500 Years. Quat. Int. 358, 35–41. doi:10.1016/j.quaint.2014.09.057
Chen, J., Chen, F., Feng, S., Huang, W., Liu, J., and Zhou, A. (2015b). Hydroclimatic Changes in China and Surroundings during the Medieval Climate Anomaly and Little Ice Age: Spatial Patterns and Possible Mechanisms. Quat. Sci. Rev. 107, 98–111. doi:10.1016/j.quascirev.2014.10.012
Chen, F., Jia, J., Chen, J., Li, G., Zhang, X., Xie, H., et al. (2016). A Persistent Holocene Wetting Trend in Arid central Asia, with Wettest Conditions in the Late Holocene, Revealed by Multi-Proxy Analyses of Loess-Paleosol Sequences in Xinjiang, China. Quat. Sci. Rev. 146, 134–146. doi:10.1016/j.quascirev.2016.06.002
Chen, F., Chen, J., Huang, W., Chen, S., Huang, X., Jin, L., et al. (2019). Westerlies Asia and Monsoonal Asia: Spatiotemporal Differences in Climate Change and Possible Mechanisms on Decadal to Sub-orbital Timescales. Earth-Science Rev. 192, 337–354. doi:10.1016/j.earscirev.2019.03.005
Cheng, Y., Li, S., and Mengubieke, E. (2016). Changes of Water and Salt Characteristic of Ulungur Lake and the Corresponding Cause Analysis (In Chinese with English Abstract). Environ. Protect Xinjiang 38, 1–7. doi:10.3969/j.issn.1008-2301.2016.01.001
Davis, O. K. (1999). Pollen Analysis of Tulare Lake, California: Great Basin-like Vegetation in Central California during the Full-Glacial and Early Holocene. Rev. Palaeobotany Palynol. 107, 249–257. doi:10.1016/S0034-6667(99)00020-2
Eichler, A., Tinner, W., Brütsch, S., Olivier, S., Papina, T., and Schwikowski, M. (2011). An Ice-Core Based History of Siberian forest Fires since AD 1250. Quat. Sci. Rev. 30, 1027–1034. doi:10.1016/j.quascirev.2011.02.007
El-Moslimany, A. P. (1990). Ecological Significance of Common Nonarboreal Pollen: Examples from Drylands of the Middle East. Rev. Palaeobotany Palynol. 64, 343–350. doi:10.1016/0034-6667(90)90150-H
Fægri, K., and Iversen, J. (1989). Textbook of Pollen Analysis. 4th Edition. London, England: John Wiley & Sons.
Fan, M., Xu, J., Chen, Y., Li, D., and Tian, S. (2020). How to Sustainably Use Water Resources-A Case Study for Decision Support on the Water Utilization of Xinjiang, China. Water 12, 3564. doi:10.3390/w12123564
Fang, Y. (1989). The History of Xinjiang Culticating. Xinjiang Uygur Autonomous Region: Xinjiang Juvenile Publishing House. (in Chinese).
Fellner, J., and Rechberger, H. (2009). Abundance of 14C in Biomass Fractions of Wastes and Solid Recovered Fuels. Waste Manag. 29 (5), 1495–1503. doi:10.1016/j.wasman.2008.11.023
Feng, X., Ni, J., Yan, S., Kong, Z., and Yang, Z. (2006). Lake fluctuation and environmental change in the late Holocene recorded by plain lakes in northern Xinjiang (in Chinese). Chin. Sci. Bull. 51, 49–55.
Feng, Z., Sun, A., Abdusalih, N., Ran, M., Kurban, A., Lan, B., et al. (2016). Vegetation Changes and Associated Climatic Changes in the Southern Altai Mountains within China during the Holocene. Holocene 27, 683–693. doi:10.1177/0959683616670469
Feng, Z., Zhang, T., and Ran, M. (2017). Climatic and Hydrological Changes in Northern Xinjiang and Surrounding Areas in the Past Ten Thousand Years. Lanzhou: Lanzhou University Press. in Chinese.
Fontana, L., Sun, M., Huang, X., and Xiang, L. (2019). The Impact of Climate Change and Human Activity on the Ecological Status of Bosten Lake, NW China, Revealed by a Diatom Record for the Last 2000 Years. Holocene 29, 1871–1884. doi:10.1177/0959683619865586
Gao, Y., Li, Z., Zhu, R., and Wang, N. a. (2020). Quantitative Reconstruction of Holocene Millennial-Scale Precipitation in the Asian Monsoon Margin of Northwest China, Revealed by Phytolith Assemblages from Calcareous Root Tubes in the Tengger Desert. Clim. Dyn. 55, 755–770. doi:10.1007/s00382-020-05293-4
Grimm, E. C. (1987). CONISS: A FORTRAN 77 Program for Stratigraphically Constrained Cluster Analysis by the Method of Incremental Sum of Squares. Comput. Geosci. 13 (1), 13–35. doi:10.1016/0098-3004(87)90022-7
Grimm, E. C. (2011). Tilia 1.7.16 Software. Springfield, IL: Illinois State Museum, Research and Collection Center.
Hao, Z., Zheng, J., Yu, Y., Xiong, D., Liu, Y., and Ge, Q. (2019). Climatic Changes during the Past Two Millennia along the Ancient Silk Road. Prog. Phys. Geogr. Earth Environ. 44, 605–623. doi:10.1177/0309133319893919
Huang, X. Z., Chen, F. H., Fan, Y. X., and Yang, M. L. (2009). Dry Late-Glacial and Early Holocene Climate in Arid central Asia Indicated by Lithological and Palynological Evidence from Bosten Lake, China. Quat. Int. 194, 19–27. doi:10.1016/j.quaint.2007.10.002
Huang, X., Zhou, G., Ma, Y., Xu, Q., and Chen, F. (2010). Pollen Distribution in Large Freshwater lake of Arid Region: a Case Study on the Surface Sediments from Bosten Lake, Xinjiang, China. Front. Earth Sci. China 4, 174–180. doi:10.1007/s11707-009-0060-2
Huang, J., Yu, H., Guan, X., Wang, G., and Guo, R. (2015). Accelerated Dryland Expansion under Climate Change. Nat. Clim Change 6, 166–171. doi:10.1038/NCLIMATE2837
Huang, X., Peng, W., Rudaya, N., Grimm, E. C., Chen, X., Cao, X., et al. (2018). Holocene Vegetation and Climate Dynamics in the Altai Mountains and Surrounding Areas. Geophys. Res. Lett. 45, 6628–6636. doi:10.1029/2018GL078028
Jackson, S. T. (1990). Pollen Source Area and Representation in Small Lakes of the Northeastern United States. Rev. Palaeobotany Palynol. 63, 53–76. doi:10.1016/0034-6667(90)90006-5
Jenny, J.-P., Koirala, S., Gregory-Eaves, I., Francus, P., Ahrens, B., Brovkin, V., et al. (2020). Reply to Li et al.: Human societies began to play a significant role in global sediment transfer 4,000 years ago. Proc. Natl. Acad. Sci. USA 117, 5571–5572. doi:10.1073/pnas.1922723117
Jiang, Q., Ji, J., Shen, J., Matsumoto, R., Tong, G., Qian, P., et al. (2013). Holocene Vegetational and Climatic Variation in westerly-dominated Areas of Central Asia Inferred from the Sayram Lake in Northern Xinjiang, China. Sci. China Earth Sci. 56, 339–353. doi:10.1007/s11430-012-4550-9
Lan, J., Xu, H., Sheng, E., Yu, K., Wu, H., Zhou, K., et al. (2018). Climate Changes Reconstructed from a Glacial lake in High Central Asia over the Past Two Millennia. Quat. Int. 487, 43–53. doi:10.1016/j.quaint.2017.10.035
Lan, B., Zhang, D., Yang, Y., He, L., Zhang, X., and Zhong, R. (2019). Diatom-based Reconstructions of Hydrological Variations and the Underlying Mechanisms during the Past ∼520 Years in the central Tianshan Mountains. J. Hydrol. 575, 945–954. doi:10.1016/j.jhydrol.2019.06.001
Lan, J., Zhang, J., Cheng, P., Ma, X., Ai, L., Chawchai, S., et al. (2020). Late Holocene Hydroclimatic Variation in central Asia and its Response to Mid-latitude Westerlies and Solar Irradiance. Quat. Sci. Rev. 238, 106330. doi:10.1016/j.quascirev.2020.106330
Lang, W. (2020). The History of Sandstorm Activity Recorded by the Sedimentation of Jili Lake in Northern Xinjiang during the Last 1600 Years the Master’s Thesis of. Lanzhou University. (in Chinese). doi:10.27204/d.cnki.glzhu.2020.000173
Li, J., Sang, X., Ji, Y., and Chen, R. (1991). The Climate in Xinjiang. Beijing: Meteorology Press. (in Chinese).
Li, Y., Zhou, L., and Cui, H. (2008). Pollen Indicators of Human Activity. Sci. Bull. 53, 1281–1293. doi:10.1007/s11434-008-0181-0
Li, F., Sun, J., Zhao, Y., Guo, X., Zhao, W., and Zhang, K. (2010). Ecological Significance of Common Pollen Ratios: A Review. Front. Earth Sci. China 4, 253–258. doi:10.1007/s11707-010-0112-7
Li, M., Li, Y., Xu, Q., Pang, R., Ding, W., Zhang, S., et al. (2012). Surface Pollen Assemblages of Human-Disturbed Vegetation and Their Relationship with Vegetation and Climate in Northeast China. Chin. Sci. Bull. 57, 535–547. doi:10.1007/s11434-011-4853-9
Li, Q., Lu, L., Wang, C., Li, Y., Sui, Y., and Guo, H. (2015). MODIS-derived Spatiotemporal Changes of Major Lake Surface Areas in Arid Xinjiang, China, 2000-2014. Water. 7, (10). 5731–5751. doi:10.1007/s11430-019-9550-710.3390/w7105731
Li, Y., Qiang, M., Zhang, J., Huang, X., Zhou, A., Chen, J., et al. (2017). Hydroclimatic Changes over the Past 900 Years Documented by the Sediments of Tiewaike Lake, Altai Mountains, Northwestern China. Quat. Int. 452, 91–101. doi:10.1016/j.quaint.2016.07.053
Li, Y., Hu, L., Zhao, Y., Wang, H., Huang, X., Chen, G., et al. (2021). Meltwater‐Driven Water‐Level Fluctuations of Bosten Lake in Arid China over the Past 2,000 Years. Geophys. Res. Lett. 48, 2020GL090988. doi:10.1029/2020GL090988
Li, Q. (2020). Solar Forcing of Desert Vegetation and Drought Frequency during the Last 2700 Years in the interior Qaidam Basin, Northeastern Tibetan Plateau. Sci. China Earth Sci. 63, 561–574. doi:10.1007/s11430-019-9550-7
Li, Y. (2020). Agriculture and Palaeoeconomy in Prehistoric Xinjiang, China (3000-200 Bc). Veget Hist. Archaeobot 30, 287–303. doi:10.1007/s00334-020-00774-2
Liu, X., Herzschuh, U., Shen, J., Jiang, Q., and Xiao, X. (2008). Holocene Environmental and Climatic Changes Inferred from Wulungu Lake in Northern Xinjiang, China. Quat. Res. 70, 412–425. doi:10.1016/j.yqres.2008.06.005
Liu, X., Herzschuh, U., Wang, Y., Kuhn, G., and Yu, Z. (2014). Glacier Fluctuations of Muztagh Ata and Temperature Changes during the Late Holocene in Westernmost Tibetan Plateau, Based on Glaciolacustrine Sediment Records. Geophys. Res. Lett. 41, 6265–6273. doi:10.1002/2014GL060444
Liu, S., Huang, X., Qiang, M., Lin, X., Bai, Z., and Peng, W. (2016). Vegetation and Climate Change during the Mid-late-holocene Reflected by the Pollen Record from Lake Genggahai, Northeastern Tibetan Plateau. Quat. Sci. 36 (2), 247–256. (in Chinese). doi:10.11928/j.issn.1001-7410.2016.02.01
Liu, Y., Yang, J., Chen, Y., Fang, G., and Li, W. (2018). The Temporal and Spatial Variations in Lake Surface Areas in Xinjiang, China. Water 10, 431. doi:10.3390/w10040431
Ljungqvist, F. C. (2016). A New Reconstruction of Temperature Variability in the Extra‐tropical Northern Hemisphere during the Last Two Millennia. Geografiska Annaler: Ser. A, Phys. Geogr. 92, 339–351. doi:10.1111/j.1468-0459.2010.00399.x
Luo, C., Zheng, Z., Tarasov, P., Pan, A., Huang, K., Beaudouin, C., et al. (2009). Characteristics of the Modern Pollen Distribution and Their Relationship to Vegetation in the Xinjiang Region, Northwestern China. Rev. Palaeobotany Palynol. 153, 282–295. doi:10.1016/j.revpalbo.2008.08.007
Ma, J., Edmunds, W. M., He, J., and Jia, B. (2009). A 2000 Year Geochemical Record of Palaeoclimate and Hydrology Derived from Dune Sand Moisture. Palaeogeogr. Palaeoclimatol. Palaeoecol. 276, 38–46. doi:10.1016/j.palaeo.2009.02.028
Maher, L. J. (1981). Statistics for Microfossil Concentration Measurements Employing Samples Spiked with Marker Grains. Rev. Palaeobotany Palynol. 32, 153–191. doi:10.1016/0034-6667(81)90002-6
Ni, Z., Zhang, E., Sun, W., Meng, X., Ning, D., Jiang, Q., et al. (2021). Response of the Chironomid Community to Late Holocene Climate Change and Anthropogenic Impacts at Lake Ulungur, Arid Central Asia. Quat. Int. doi:10.1016/j.quaint.2021.08.007
Nielsen, A. B., and Sugita, S. (2005). Estimating Relevant Source Area of Pollen for Small Danish Lakes Around AD 1800. The Holocene 15, 1006–1020. doi:10.1191/0959683605hl874ra
Reid, G. C. (1991). Solar Total Irradiance Variations and the Global Sea Surface Temperature Record. J. Geophys. Res. 96, 2835. doi:10.1029/90JD02274
Reimer, P. J., Bard, E., Bayliss, A., Beck, J. W., Blackwell, P. G., Ramsey, C. B., et al. (2013). Intcal13 and Marine13 Radiocarbon Age Calibration Curves 0-50,000 Years Cal BP. Radiocarbon 55 (4), 1869–1887. doi:10.1017/S003382220004886410.2458/azu_js_rc.55.16947
Ren, W., Zhao, Y., Li, Q., and Chen, J. (2019). Changes in vegetation and moisture in the northern Tianshan of China over the past 450 years. Front. Earth Sci. 14, 479–491. doi:10.1007/s11707-019-0788-2
Shi, Y., Shen, Y., Kang, E., Li, D., Ding, Y., Zhang, G., et al. (2006). Recent and Future Climate Change in Northwest China. Clim. Change 80, 379–393. doi:10.1007/s10584-006-9121-7
Song, M., Zhou, A., Zhang, X., Zhao, C., He, Y., Yang, W., et al. (2015). Solar Imprints on Asian Inland Moisture Fluctuations over the Last Millennium. Holocene 25, 1935–1943. doi:10.1177/0959683615596839
Sorrel, P., Popescu, S.-M., Head, M. J., Suc, J. P., Klotz, S., and Oberhänsli, H. (2006). Hydrographic Development of the Aral Sea during the Last 2000 Years Based on a Quantitative Analysis of Dinoflagellate Cysts. Palaeogeogr. Palaeoclimatol. Palaeoecol. 234, 304–327. doi:10.1016/j.palaeo.2005.10.012
Steinhilber, F., Abreu, J. A., Beer, J., Brunner, I., Christl, M., Fischer, H., et al. (2012). 9,400 Years of Cosmic Radiation and Solar Activity from Ice Cores and Tree Rings. Proc. Natl. Acad. Sci. 109, 5967–5971. doi:10.1073/pnas.1118965109/-/DCSupplemental
Sugita, S. (1994). Pollen Representation of Vegetation in Quaternary Sediments: Theory and Method in Patchy Vegetation. J. Ecol. 82, 881–897. doi:10.2307/2261452
Sun, X., Du, N., Weng, C., Lin, R., and Wei, K. (1994). Paleovegetation and paleoenvironment of Manas Lake, Xinjiang, N. W. China during the last 14000 years (in Chinese). Quat. Sci. 239–248.
Tao, S., An, C., Chen, F., Tang, L., Wang, Z., Lü, Y., et al. (2010). Pollen-inferred Vegetation and Environmental Changes since 16.7 Ka BP at Balikun Lake, Xinjiang. Chin. Sci. Bull. 55, 2449–2457. doi:10.1007/s11434-010-3174-8
Tuerhong, M. (2011). A Brief Account of Chinese Ancient Thought and Literatures about Stationing Troops to Garrison the Frontiers. J. Libr. Inf. Sci. 21, 64–66. doi:10.3969/j.issn.1005-6033.2011.12.029 (in Chinese with English abstract).
Wang, S., and Dou, H. (1998). Chinese Journal of Lakes. Beijing: Science Publishing House. (in Chinese).
Wang, X., Wang, H., Zhang, K., Lian, G., Zhang, L., Zhou, W., et al. (1981). A Preliminary Study on the Natural Conditions of Wulungu Lake in Xinjiang and its Fishery Biology Basis(in Chinese). Trans. Oceanol. Limnol. 4, 19–28. doi:10.13984/j.cnki.cn37-1141.1981.04.004
Wang, J., Li, J., Chen, F., Gou, X., Peng, J., and Liu, P. (2007). Variation of the Dryness in the Recent 200 a Derived from Tree-Rings Width Records in the East Tianshan Mountains. J. Glaciol. Geocryol. 29, 209–216. (in Chinese).
Wang, W., Feng, Z., Ran, M., and Zhang, C. (2013). Holocene Climate and Vegetation Changes Inferred from Pollen Records of Lake Aibi, Northern Xinjiang, China: A Potential Contribution to Understanding of Holocene Climate Pattern in East-central Asia. Quat. Int. 311, 54–62. doi:10.1016/j.quaint.2013.07.034
Wen, R., Xiao, J., Chang, Z., Zhai, D., Xu, Q., Li, Y., et al. (2010). Holocene Precipitation and Temperature Variations in the East Asian Monsoonal Margin from Pollen Data from Hulun Lake in Northeastern Inner Mongolia, China. Boreas 39, 262–272.
Whitlock, C., and Larsen, C. V. (2001). “Charcoal as a Fire Proxy,” in Tracking Environmental Changes Using Lake Sediments,Terrestrial, Algal, and Siliceous Indicators. Editors J.P. Smol, H.J.B. Birks, and W.M. Last (Dordrecht: Kluwer Academic Publishers), Vol. 3, 75e96.
Xu, Q., Chen, F., Zhang, S., Cao, X., Li, J., Li, Y., et al. (2016). Vegetation Succession and East Asian Summer Monsoon Changes since the Last Deglaciation Inferred from High-Resolution Pollen Record in Gonghai Lake, Shanxi Province, China. Holocene 27, 835–846. doi:10.1177/0959683616675941
Yan, S., and Xu, Y. (1989). Spore-pollen Association in Surface-Soil in Altay(in Chinese), Xinjiang. Arid Zone Res. 26, 26–33. doi:10.13866/j.azr.1989.01.005
Yan, D., Xu, H., Lan, J., Zhou, K., Ye, Y., Zhang, J., et al. (2019). Solar Activity and the Westerlies Dominate Decadal Hydroclimatic Changes over Arid Central Asia. Glob. Planet. Change 173, 53–60. doi:10.1016/j.gloplacha.2018.12.006
Yang, L., and Zhang, Q. (2008). Interannual Variation of Summer Precipitation in Xinjiang and Asian Subtropical Westerly Jet Stream (In Chinese). J. Appl. Meteorol. Sci. 19, 171–179. doi:10.3724/SP.J.1011.2008.00323
Yang, Y., Zhang, D., Lan, B., Abdusalih, N., and Feng, Z. (2019). Peat δ13Ccelluose-signified Moisture Variations over the Past ∼2200 Years in the Southern Altai Mountains, Northwestern China. J. Asian Earth Sci. 174, 59–67. doi:10.1177/0959683619854510.1016/j.jseaes.2018.11.019
Yang, Y., Ran, M., and Sun, A. (2020). Pollen‐recorded Bioclimatic Variations of the Last ∼2000 Years Retrieved from Bayan Nuur in the Western Mongolian Plateau. Boreas 49, 350–362. doi:10.1111/bor.12423
Yang, J. (2018). Study on the Reasons of the Rise of Northern Xinjiang during the Reign of Qianlong(in Chinese). J. Kashi Univ. 39, 33–40. doi:10.13933/j.cnki.2096-2134.2018.04.007
Yao, Y., Lan, J., Zhao, J., Vachula, R. S., Xu, H., Cai, Y., et al. (2020a). Abrupt Freshening since the Early Little Ice Age in Lake Sayram of Arid Central Asia Inferred from an Alkenone Isomer Proxy. Geophys. Res. Lett. 47. doi:10.1029/2020GL089257
Yao, Y.-F., Wang, X., Guo, W., Jiang, H.-E., Li, M., Ferguson, D. K., et al. (2020b). Archaeobotanical Evidence Reveals the Human-Environment Interactions during the 9th-13th Centuries at Turpan, Xinjiang on the Ancient Silk Road. Veget Hist. Archaeobot 29, 539–552. doi:10.1007/s00334-019-00764-z
Yu, G., Prentice, I. C., Harrison, S. P., and Sun, X. (1998). Pollen‐based Biome Reconstructions for China at 0 and 6000 Years. J. Biogeogr. 25, 1055–1069. doi:10.1046/j.1365-2699.1998.00237.x
Zhang, D., and Feng, Z. (2018a). Holocene Climate Variations in the Altai Mountains and the Surrounding Areas: A Synthesis of Pollen Records. Earth-Science Rev. 185, 847–869. doi:10.1016/j.earscirev.2018.08.007
Zhang, Y., Meyers, P. A., Liu, X., Wang, G., Ma, X., Li, X., et al. (2016). Holocene Climate Changes in the central Asia Mountain Region Inferred from a Peat Sequence from the Altai Mountains, Xinjiang, Northwestern China. Quat. Sci. Rev. 152, 19–30. doi:10.1016/j.quascirev.2016.09.016
Zhang, Y., Mo, D., Hu, K., Bao, W., Li, W., Abuduresule, I., et al. (2017). Holocene Environmental Changes Around Xiaohe Cemetery and its Effects on Human Occupation, Xinjiang, China. J. Geogr. Sci. 27, 752–768. doi:10.1007/s11442-017-1404-6
Zhang, D., Feng, Z., Yang, Y., Lan, B., Ran, M., and Mu, G. (2018b). Peat δ13Ccelluose-recorded Wetting Trend during the Past 8000 Years in the Southern Altai Mountains, Northern Xinjiang, NW China. J. Asian Earth Sci. 156, 174–179. doi:10.1016/j.jseaes.2018.01.029
Zhang, J., Huang, X., Wang, Z., Yan, T., and Zhang, E. y. (2018c). A Late-Holocene Pollen Record from the Western Qilian Mountains and its Implications for Climate Change and Human Activity along the Silk Road, Northwestern China. Holocene 28, 1141–1150. doi:10.1177/0959683618761548
Zhao, W., and Xie, S. (1988). Population History of China. Beijing: People's Publishing House, 1–645. in Chinese.
Zhao, Y., Xu, Q., Huang, X., Guo, X., and Tao, S. (2009). Differences of Modern Pollen Assemblages from lake Sediments and Surface Soils in Arid and Semi-arid China and Their Significance for Pollen-Based Quantitative Climate Reconstruction. Rev. Palaeobotany Palynol. 156, 519–524. doi:10.1016/j.revpalbo.2009.05.001
Zhao, Y., Yu, Z., Liu, X., Zhao, C., Chen, F., and Zhang, K. (2010). Late Holocene Vegetation and Climate Oscillations in the Qaidam Basin of the Northeastern Tibetan Plateau. Quat. Res. 73, 59–69. doi:10.1016/j.yqres.2008.11.007
Zhao, Y., Li, F., Hou, Y., Sun, J., Zhao, W., Tang, Y., et al. (2012). Surface Pollen and its Relationships with Modern Vegetation and Climate on the Loess Plateau and Surrounding Deserts in China. Rev. Palaeobotany Palynol. 181, 47–53. doi:10.1016/j.revpalbo.2012.05.007
Keywords: moisture change, pollen records, the historical period, climate change, arid central asia
Citation: Xiao Y, Xiang L, Huang X, Mills K, Zhang J, Chen X and Li Y (2021) Moisture Changes in the Northern Xinjiang Basin Over the Past 2400 years as Documented in Pollen Records of Jili Lake. Front. Earth Sci. 9:741992. doi: 10.3389/feart.2021.741992
Received: 15 July 2021; Accepted: 16 September 2021;
Published: 30 September 2021.
Edited by:
Zhuolun Li, Lanzhou University, ChinaReviewed by:
John Dodson, Institute of Earth Environment, Chinese Academy of Sciences, ChinaChun Mei Ma, Nanjing University, China
Copyright © 2021 Xiao, Xiang, Huang, Mills, Zhang, Chen and Li. This is an open-access article distributed under the terms of the Creative Commons Attribution License (CC BY). The use, distribution or reproduction in other forums is permitted, provided the original author(s) and the copyright owner(s) are credited and that the original publication in this journal is cited, in accordance with accepted academic practice. No use, distribution or reproduction is permitted which does not comply with these terms.
*Correspondence: Xiaozhong Huang, eHpodWFuZ0BsenUuZWR1LmNu
†These authors have contributed equally to this work