- 1State Key Laboratory of Shale Oil and Gas Enrichment Mechanisms and Effective Development, SINOPEC, Beijing, China
- 2School of Ocean and Earth Science, Tongji University, Shanghai, China
The variation in mineral composition will affect the rock brittleness, thus the change of mineral assemblages during diagenesis has a potential control on the brittleness of mudstones. In this study, thin section, X-ray diffraction (XRD), and Scanning Electron Microscope (SEM) analyses were used to investigate compositional and microscopic features of mudstones. With the enhancement of diagenesis, three mineral assemblages were divided due to the diagenetic evolution of minerals. Quartz, feldspar, dolomite, chlorite, and illite were regarded as brittle minerals and (quartz + feldspar + dolomite + illite + chlorite)/(detrital mineral + carbonate + clay mineral) was defined as the brittleness evaluation index The mudstone brittleness changed slightly during early diagenesis but increased gradually with enhancement of diagenesis in the late diagenesis stage. Quartz and feldspar were scattered above the clay matrix and the contact of grains was limited, therefore, the contribution of detrital minerals to the brittleness was affected by the properties of clay minerals. The diagenetic transformation of clay minerals resulted in the reduction of ductile components (smectite/I-Sm and kaolinite) and increase of brittle components (illite and chlorite), leading to the enhancement of integral rigidity of the mudstones. Meanwhile, the improved crystallization of carbonate in late diagenesis stage enlarged the carbonate grains which resulted in rigid contact between grains. These results highlighted the influence of diagenesis on mudstone brittleness. Therefore, for evaluation of mudstone brittleness, attention should be paid to the diagenesis process besides mineral composition.
1 Introduction
Detrital minerals, carbonates, and clay minerals are the main components of mudstones and the various properties of these components have significant effects on mudstone brittleness. The mudstone brittleness was always considered to be affected by the mineral composition (Jarvie et al., 2007). According to the mechanical properties, minerals in mudstone could be divided into brittle and ductile components. Brittle components consisted of quartz and carbonate (Jin et al., 2015; Rybacki et al., 2016; Ye et al., 2020). The methods of evaluating mudstone brittleness were usually based on the brittle mineral content. Jarvie et al. (2007) defined the quartz content as the main factor controlling the brittleness modulus of a shale, and quartz/(quartz + carbonate + clay minerals) was adopted to quantitatively calculate the brittleness (Jarvie et al., 2007). In addition to quartz, Nelson (1985) considered feldspar and dolomite were also the brittle components and another evaluation method was proposed. An increase in quartz, feldspar, and dolomite was believed to enhance mudstone brittleness and to promote the development of fractures (Rybacki et al., 2016; Iqbal et al., 2018). Conversely, the increase in the relatively ductile clay minerals would reduce the brittleness of mudstones (Rybacki et al., 2016; Wang, 2016). However, recent studies have shown that the content of brittle minerals was not linearly correlated to mudstone brittleness, and ascribed this phenomenon to the influence of diagenesis (Xiong et al., 2018). Therefore, investigating the effects of mineralogical composition on brittleness during progressive diagenesis may allow a better understanding of mudstone brittleness.
Minerals are not in chemical equilibrium with pore water at the time of deposition. During progressive burial, the increase of temperature and pressure lead to the diagenetic changes of minerals which allow them to approach equilibrium with the diagenetic environment (Bjørlykke and Jahren, 2012). Detrital minerals (e.g., quartz and feldspar) are chemically stable and barely change during diagenesis. Clay minerals, however, are chemically active and sensitive to the surrounding conditions, in this way, the clay mineral transformations resulted from the changes in the diagenetic environment are widespread. Kaolinite is stable at 70–100°C but will transform into illite at higher temperatures if there is a supply of potassium (Mantovani and Becerro, 2010). Meanwhile, smectite illitization and chloritization are important diagenetic processes of mudstones in most sedimentary basins around the world (Velde and Vasseur, 1992; Pelayo et al., 2016). The smectite and kaolinite can adsorb water in the surface or interlayer space, while illite and chlorite contain minor water molecules. The water content and activity have been proved to control the deformability of clay minerals (Fouché et al., 2004), which means that the higher water activity can result in poor mechanical properties (Wen et al., 2015). Therefore, mineral transformations can result in variations of physicochemical properties, such as the brittleness of the mudstones.
For carbonate in Dongying Depression, dissolution-recrystallization and dolomitization of calcite that are occurred during diagenesis could enlarge the grain size and promote the formation of calcite cement (Zeng et al., 2018). In this way, the cohesiveness between mineral grains is enhanced, resulting in transformation of mechanical properties of mudstones from plasticity to brittleness (Bjørlykke and Høeg, 1997). In addition, the diagenetic conditions (e.g., temperature, pressure, pH, ion types and concentration) are changing constantly during burial diagenesis. Since diagenetic transformation facilitates minerals approach equilibrium with diagenetic conditions, specific mineralogical assemblages are formed corresponding to the diagenetic environment (Du et al., 2019). The changes of mineralogical assemblages would inevitably lead to variations in mechanical properties of mudstones. Therefore, investigating the variations of mineralogical assemblages in different diagenetic stages is of great importance for understanding the control of diagenesis on mudstone brittleness.
In this study, mudstones collected from the subsurface of the Dongying Depression (Bohai Bay Basin, NE China) were studied by thin section, X-ray diffraction (XRD) and scanning electron microscopy (SEM) analyses. We investigated the effects of diagenesis on variations in mineralogical assemblages and established a brittleness evaluation index (BEI) with which to monitor changes in brittleness during progressive diagenesis. Correlations between mineralogical components and brittleness were established, and the influence of diagenesis and mineral assemblages on mudstone brittleness were discussed. The study will contribute to the design of mudstone fracture schemes and to the selection of appropriate injection fluids during shale oil or gas development projects.
2 Materials and Methods
A total of 168 mudstones from 10 wells (Table 1) in the Dongying Depression were collected (Figure 1). The samples were recovered from depths of 1,255.00–5,647.24 m and covered the Shahejie Formation (Es) and Kongdian Formation (Ek) (Table 1).
2.1 Thin Section
Samples from well N38 were selected for thin section analysis. The samples were embedded in epoxy resin and sectioned perpendicular to the lamination; the sections were polished using abrasive powder until they reached a thickness of 30 μm. The mineralogical structure and optical properties were then investigated using a ZEISS Axio Imager microscope-photometer under transmitted and reflected white light with magnification of ×50–500.
2.2 X-Ray Diffraction
A Dmax-RA diffractometer was used for XRD analysis which were conducted at 20 mA and 40 kV with CuKα radiation. The bulk mineralogy was analysed using randomly oriented powder samples which were scanned from 3 to 40°2θ. The clay mineral composition of the samples was analysed with oriented slides and multiple treatments: samples were air-dried, glycol-saturated, and heated to 550 °C. The oriented slides were scanned from 3 to 40°2θ at 2°2θ/min with a step width of 0.02°2θ.
2.3 Scanning Electron Microscopy
Samples from wells NY1 and N38 were selected for SEM analysis using a FEI Quanta 200 Scanning Electron Microscope. Prior to SEM analysis, samples were coated with carbon to improve their conductivity, and sample morphology was then analysed at various magnifications to determine the evolution of crystal shape during diagenesis.
3 Results
3.1 Mineral Composition
XRD analysis showed that the mudstones mainly consisted of detrital minerals, clay minerals, and carbonates (Figure 2A). Detrital minerals included quartz, K-feldspar, and plagioclase. The quartz content varied little (Figure 2C) with maximum and average values of 48 and 23%, respectively. The maximum value of the plagioclase content was 34% with an average of 10%. Only small amount of K-feldspar was present in the samples with an average of 2% (Figure 2C). The content of carbonates (calcite and dolomite) varied with depth (Figure 2C). The proportion of calcite (average 18%) fell gradually with increasing depth while dolomite (average 10%) increased progressively (Figure 2C). Clay minerals had an average content of 31%. Smectite/I-Sm (37%) and illite (53%) were the two main components of the clay minerals, accounting for 90% of the total clay content, while kaolinite and chlorite were present in small proportions (Figure 2B).
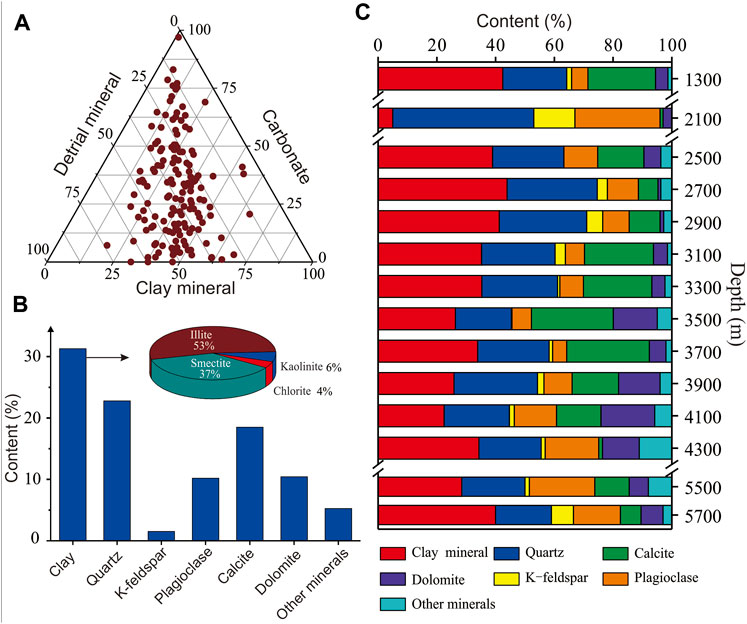
FIGURE 2. Mineralogical characteristics of mudstones in Dongying Depression. (A) Triangular chart of three components in bulk mineralogy; (B) Composition of bulk mineralogy and clay minerals; (C) Evolution of bulk mineralogy.
3.2 Microscopic Characteristics
The depositional fabric of mudstones was either massive or laminated microstructure. Detrital minerals (e.g., quartz and feldspar) were poorly rounded and dispersed within clay matrix (Figure 3A). Under SEM, except for some small quartz particles within pore spaces, no well-crystallized quartz and feldspar were observed (Figure 4A). In samples with a massive microstructure, clay minerals were present as matrix, on which the organic matter (OM) particles were distributed dispersedly (Figure 3A). In laminated mudstone, clay-mineral rich laminae alternated with OM- or detrital-rich laminae (Figures 3B,C). Illite showed a schistose morphology (Figure 4F) and chlorite typically took the form of rosettes or laminations (Figure 4H). As burial depths increased, the crystallinity of illite improved progressively (Figures 4F,G).
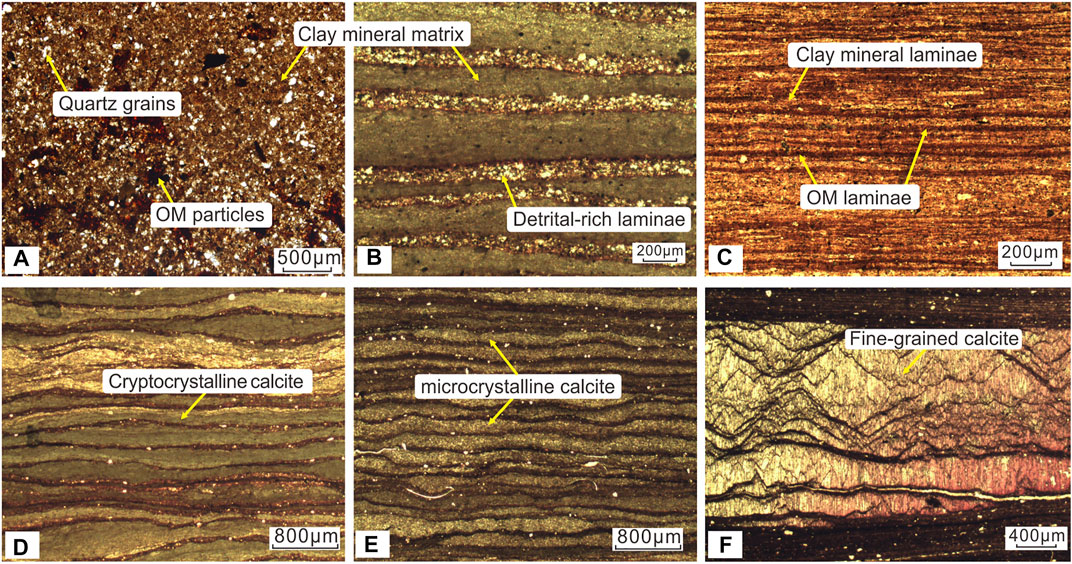
FIGURE 3. Photomicrographs of various microstructures in samples collected from Dongying Depression. (A) massive mudstone. (B) laminated mudstone with clay- and detrital-rich laminae. (C) laminated mudstone with clay- and OM-rich laminae. (D) cryptocrystalline calcite. (E) microcrystalline calcite. (F) fine-grained calcite.
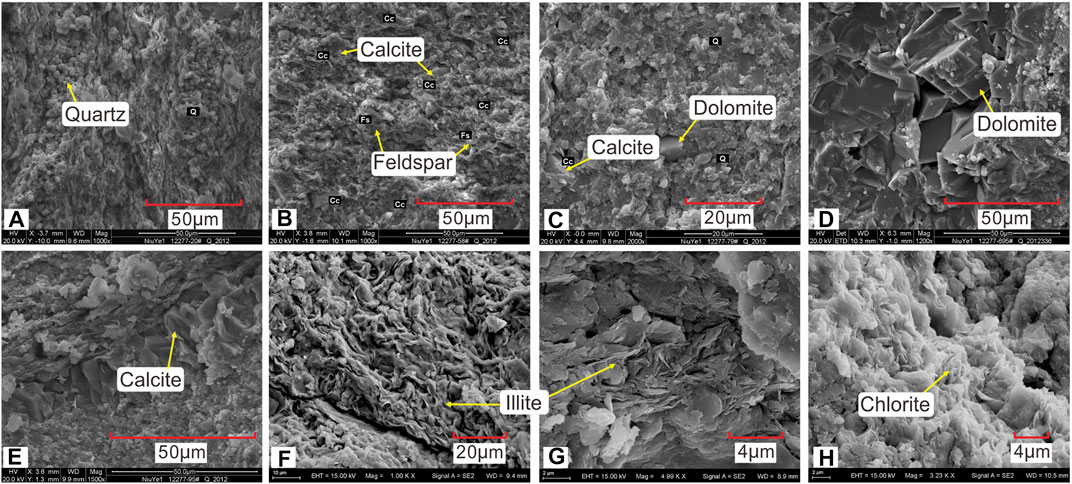
FIGURE 4. SEM Photographs of mudstone from Dongying Depression. Abbreviations for the minerals are labeled (Q: Quartz; Cc: Calcite; Fs: Feldspar; Ak: Ankerite; I: illite; Ch: Chlorite) (A) Quartz crystals fill in the pores (NY1 well, 3,297.03 m). (B) Calcite partial dissolves and feldspar exists in the pores (NY1 well, 3,306.62 m). (C) Calcite dissolves, authigenous dolomite and small quartz crystals distribute in the pores (NY1 well, 3,312.61 m). (D) Dolomite (NY1 well, 3,475.96 m); (E) Recrystallized calcite (NY1 well, 3,316.55 m). (F) Illite with schistose arrangement (S142 well, 2,677.64 m). (G) Illite with schistose arrangement (HK1 well, 3,969.69 m). (H) Chlorite is arranged as laminations (HK1 well, 4,019.16 m).
Two kinds of carbonate were recorded by microscope observations. The first was cryptocrystalline calcite which was distributed as discontinuously banded or lenticular structures. The particles were poorly crystallized and turbid with poor brightness (Figure 3D). SEM observations showed that this kind of calcite particles were partially dissolved and coexisted with feldspar particles (Figure 4B). The other was sparry carbonate (calcite and dolomite) with large and clear grains under polarizing microscope (Figure 3F), which showed typical crystal morphology and grew among cryptocrystalline calcites. With increasing depth, we could find the enlargement of crystal particles and formation of sparry calcite and dolomite (Figures 4C–E). Meanwhile, the morphology grew from small crystals into regular and large crystals with enhancement of diagenesis (Figures 4C–E).
4 Discussion
4.1 Diagenetic Stages and Mineralogical Assemblages
Detrital minerals in the mudstones were composed of quartz and feldspar with large grains. There was no evident relationship between the content of detrital minerals and burial depth (Figure 2C). Under SEM, only small quartz particles were observed in pores (Figure 4). Previous studies have shown that most of the detrital minerals in mudstones in the Dongying Depression were of terrigenous origin (Zeng et al., 2018). Due to their low chemical activity and relatively stable nature, quartz and feldspar were not significantly affected by the external environment during burial diagenesis.
Cryptocrystalline calcite was in general present at depths above 3,000 m. The mineral grains were micritic under optical microscope and no impurities were found in the calcite lamina (Figure 3E). Well-ordered crystals were hard to find by SEM (Figure 4B). The formation of this kind of calcite was generally considered to result from changes of the microenvironment lake by sedimentation of plankton during the high salinity period (Bennett et al., 2012). Cryptocrystalline calcite was little changed during diagenesis, but sparry calcite and dolomite began to appear at burial depths greater than 3,000 m and took the form of clean and bright grains. These grains distributed as lenses and veins within laminae that contained abundant carbonate (Figure 3F). Moreover, the dissolution of cryptocrystalline calcite was accompanied by the formation of calcite and dolomite crystals (Figures 4B–E). Previous studies have confirmed that primary depositional calcite could be partially dissolved by organic acids, and then recrystallized to form sparry calcite (Wang et al., 2005). In the presence of sufficient Fe and Mg, calcite might then be transformed into dolomite (Krajewski and Wozny, 2009).
Due to large adsorption capacity, clay minerals always associate and flocculate with OM, presenting laminated distributions. Therefore, clay minerals in mudstones initially came from the flocculation deposition during the deposition period (Zeng et al., 2018). Clay minerals were chemically active and prone to transformation, such as smectite illitization and chloritization (Velde and Vasseur, 1992; Pelayo et al., 2016). Further analyses of SEM and XRD revealed that the morphology of illite was mainly composed of schistose aggregates and the crystallinity improved gradually with increasing depth. Furthermore, the illite content was negatively correlated with that of smectite/I-Sm. Chlorite presented in the form of rosette aggregates that appeared in large amounts below 3,700 m. These phenomena proved the existence of transformations among different components of clay minerals.
Driven by thermal dynamics and kinetics, the dissolution-precipitation reaction of minerals would take place and form mineral assemblages that equilibrate with certain temperature and pressure (Bjørlykke and Jahren, 2012). In present study, clay minerals and carbonate had experienced an obvious diagenetic process, resulting in significant variation in content and mineral assemblages (Figure 5). For diagenetic minerals, the increase of content had a corresponding relationship with the improvement of grain size and crystallinity. Therefore, the variation of mineral assemblages could not only reflect the changes of mineral composition, but also characterized the diagenesis.
According to the evolution of mineral content and microscopic characteristics, three mineral assemblages were recognised:
1) Above 3,000 m, clay minerals mainly consisted of smectite and kaolinite, with a minor amount of illite and chlorite. Although the amount of carbonate was high, the thin section and SEM showed that the carbonate was mainly cryptocrystalline calcite, which had not undergone diagenetic transformation. The content of detrital minerals was high, forming a smectite-kaolinite-cryptocrystalline calcite-detrital mineral assemblage (Figure 4).
2) With increasing depth, transformation of smectite into illite led to a reduction of smectite/I-Sm and an increase of illite. Meanwhile, microcrystalline calcite and dolomite were formed through recrystallization and dolomitization of calcite. The diagenesis resulted in an illite-microcrystalline calcite-dolomite-detrital mineral assemblage.
3) Subsequently, a large amount of chlorite appeared due to chloritization of smectite, and clay minerals were dominated by illite and chlorite. The recrystallization of calcite further improved and fine-grained calcite and dolomite appeared. The mineral assemblage turned into illite-chlorite-fine grained calcite-dolomite-detrital mineral.
4.2 Control of Diagenesis on Mudstones Brittleness
4.2.1 The Influence of Mineral Properties on Brittleness
Quartz and feldspar were in general regarded as brittle minerals (Jarvie et al., 2007). Due to the high brittleness, quartz and feldspar were prone to produce cracks under external force, therefore previous researches hold that the higher content of detrital minerals, the better the brittleness of rock (Rybacki et al., 2016). Detrital minerals in mudstones in the Dongying Depression were mainly of terrigenous origin (Zeng et al., 2018). The grains were distributed upon argillaceous matrix and the contact between grains was limited (Figure 3A). Therefore, the effects of detrital minerals on brittleness were also controlled by cementation. In addition, Si that released from crystal structures of clay minerals during smectite illitization would crystallize into quartz which could act as cement to enhance the rock strength (Metwally and Chesnokov, 2012). Meanwhile, carbonate cementation also promoted the formation of solid rock framework. Therefore, the influence of detrital minerals on brittleness in different diagenetic stages required further analysis.
The clay minerals were traditional considered as plastic components mainly because they would expand and deform through hydration during hydraulic fracturing of mudstones or shale. However, the hydration ability of different clay minerals varies greatly. The expandable smectite which has high cation exchange capacity shows strong water adsorption ability and could adsorb water in interlayer and on external surface. Therefore, the smectite is liable to hydrate and deform plastically. Similarly, kaolinite also shows high hydration capacity and becomes plastic after hydration. However, although illite and smectite are both 2:1 phyllosilicate, illite is not expandable due to the high affinity of interlayer potassium to the structural layers. Therefore, illite does not readily hydrate and deform plastically. The same is true for chlorite. The interlayer space of chlorite is filled with a brucite-like sheet, which determines that chlorite is non-expandable and shows weak hydration. Therefore, smectite/I-Sm and kaolinite were considered as ductile components while illite and chlorite were brittle components. Thus, the varying proportions of different clay minerals would affect the mudstone brittleness. In addition, as clay minerals were matrix of mudstones, the properties of clay minerals would affect the contributions of quartz and feldspar to mudstone brittleness. Therefore, in order to evaluate the mudstone brittleness more accurately, the evolution of clay minerals should be taken into account when analysing the effects of clay minerals.
For carbonate, both calcite and dolomite belong to brittle minerals. However, the crystallinity varied considerably during diagenesis. When cryptocrystalline carbonate was dominated, the mineral grains were small and there was no carbonate cementation (Figure 3D), contributing little to the brittleness. While when carbonate presented as microcrystalline or fine-grained particles, the rigid contact between mineral grains was enhanced and the formation of carbonate cement enabled brittle minerals (e.g., quartz and feldspar) to form rock framework. Therefore, investigating the control of carbonate on brittleness required attention to the microscopic characteristics besides the composition.
4.2.2 Control of Mineral Assemblage and Diagenesis on Brittleness
The mineralogy and microscopic characteristics revealed that diagenesis altered the content and properties of minerals, forming different mineral assemblages corresponding to diagenetic environment. Therefore, the brittleness of mudstone was definitely affected by diagenesis and mineral assemblages. In order to investigate the control of diagenesis and mineral assemblages on mudstone brittleness, a BEI was established using brittle mineral content. Based on the mineral properties, quartz, feldspar, dolomite, illite, and chlorite were chosen as the brittle components to calculate the BEI. Therefore, a new expression of mudstone brittleness was proposed:
Where the WQFDIC is the weight of quartz, feldspar, dolomite, illite, and chlorite, WTot is the weight of total minerals. The illite and chlorite content has been converted into relative contents in bulk mineralogy (e.g., illite content = clay mineral content×illite proportion in clay). With the enhancement of diagenesis, the variation of brittleness showed two-stage characteristics (Figure 6). Brittleness was basically unchanged above 3,000 m, before increasing steadily below 3,000 m. This trend indicated that evolution of brittleness varied in different diagenetic stages, and the brittleness was enhanced significantly in late diagenetic stage.
In order to further determine the effects of diagenesis on brittleness, the different contribution of each mineral to brittleness in different diagenetic stages was compared by analysing the correlation between each mineral component and BEI. The results showed that although quartz was brittle, its contribution to brittleness varied in different stages (Figure 7A). In the early diagenesis stage, which was corresponding to smectite-kaolinite-cryptocrystalline calcite-detrital mineral assemblage, the BEI was reduced progressively although quartz content was high. Although the content of detrital minerals was relatively high, they were distributed in the argillaceous matrix and the grains were matrix-supported, resulting in viscous or plastic mechanical properties of rock. Furthermore, the argillaceous matrix was mainly composed of smectite/I-Sm and kaolinite, which were ductile. These two reasons became the main factors limiting the increase of brittleness and led to the negative correlation of quartz content and BEI. In the late diagenetic stages with illite-microcrystalline calcite-dolomite-detrital mineral and illite-chlorite-fine grained calcite-dolomite-detrital mineral assemblages, quartz content and BEI showed a significant positive correlation, indicating that diagenesis enhanced the contribution of quartz to brittleness. In this period, the Si that released from crystal structure of clay minerals during smectite illitization promoted the formation of quartz (Metwally and Chesnokov, 2012; Cai et al., 2018). The neo-formed quartz could act as cement to connect mineral grains, thus enhancing the brittleness of rock framework (Akinbinu, 2015). The correlation of feldspar with BEI was similar to that of quartz with BEI (Supplementary Figure S1).
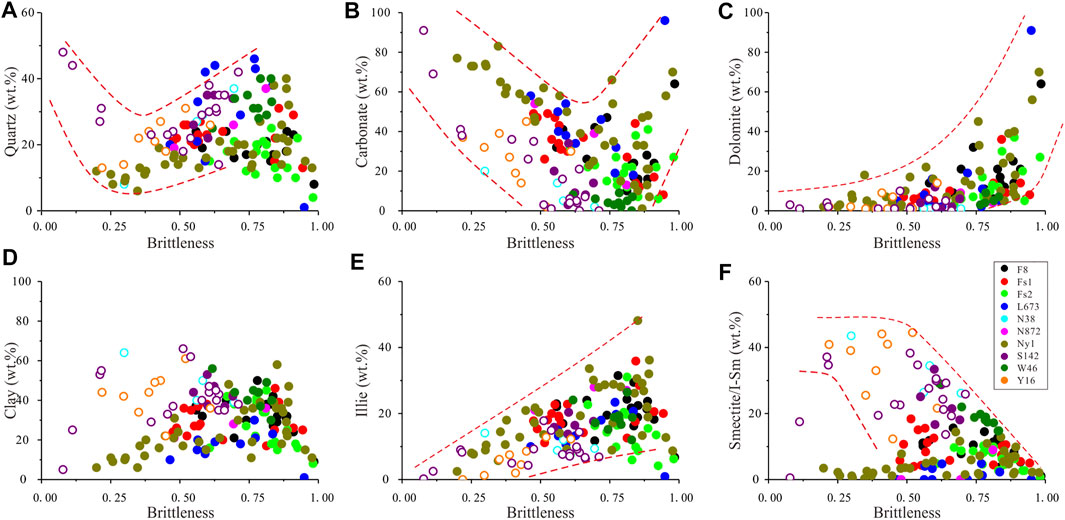
FIGURE 7. Correlations of BEI with quartz (A), carbonate (B), dolomite (C), clay (D), illite (E), smectite/I-Sm (F). (circle: samples in early diagenesis stage; solid circle: samples in late diagenesis stage).
Similar relationship also occurred between carbonate and BEI. Carbonate was negatively correlated with BEI in early diagenesis, followed by a positive correlation between them in late diagenesis (Figure 7B). However, different correlations between dolomite and brittleness were observed, in which weak and high positive correlations occurred in early and late diagenesis, respectively (Figure 7C). In the case of same mineral composition, the coarser the grains, the better the mudstone brittleness. In early diagenesis, carbonate was mainly composed of cryptocrystalline calcite with poor automorphism and tiny grains, exhibiting obvious plasticity. With the enhancement of diagenesis, cryptocrystalline calcite partially dissolved under the effects of organic acid, and then recrystallized to microcrystalline and fine-grained calcite or converted into dolomite in case of presence of Fe and Mg (Wang et al., 2005; Krajewski and Wozny, 2009). In this circumstance, crystallization was improved and mineral grains became larger (Figures 4C–E). Meanwhile, the carbonate cementation strengthened the cohesiveness between mineral grains, resulting in transformation of mechanical properties from plasticity to brittleness (Luan et al., 2014). Therefore, there was a two-stage correlation between carbonate and BEI. Dolomite appeared in large quantities and the crystallization improved gradually only in the late diagenesis, leading to the overall positive correlation with brittleness.
Previous studies considered that the increase of clay minerals would reduce the brittleness due to the plasticity of clay minerals (Rybacki et al., 2016; Iqbal et al., 2018). This study, however, found that clay minerals have no correlation with BEI in the whole diagenetic period (Figure 7D). This indicated that when using mineral components to analyse brittleness, clay minerals cannot be simply regarded as ductile components. Noteworthy was that illite was well positively correlated with BEI (Figure 7E). The illite content were low in early diagenesis stage but high in late diagenesis stage (Figure 7E), and the same is true of BEI (Figure 6). The positive correlation between illite and BEI highlighted the contribution of clay mineral transformation to the increase of mudstone brittleness. The negative correlation between smectite/I-Sm with BEI also confirmed that smectite illitization affected the brittleness of mudstones (Figure 7F). During diagenesis, conversions between clay mineral components took place due to variation of diagenetic environment (Li et al., 2016). Mudstones are mainly composed of clay minerals, which serve as the matrix and adhesion agent. The transformation of ductile components (e.g., smectite) into brittle components (e.g., illite) would enhance the integral rigidity of the mudstones. Therefore, the diagenesis of clay minerals should be taken into account when investigate the contribution of clay minerals to brittleness of mudstones.
4.3 Comparison of BEI Between Mudstones With Different Crude Output
Samples from three wells in Jiyang Depression with different crude output were collected to compare the BEI values. XYS9 well is a commercial oil flow well with daily crude output of 38.5 t/d, while L67 and L69 are stripper wells with daily oil production of 2.09 t/d and 0.19 t/d, respectively. Zhu et al. (2019) used the δTOC, which equalled to the difference of TOC between the raw and organic solvent extracted mudstones, to characterize the mobile oil content in mudstones. Here the δTOC of these three wells varied slightly (Figure 8A). Therefore, the amount of mobile oil content measured under laboratory conditions was not the main control for the different crude output in these three wells. The brittleness of the mudstones affects the hydraulic fracturing effect, which determined whether oil or gas could be successfully extracted. Therefore, brittleness of the mudstones from these three well was evaluated using the BEI parameter. The BEI varied obviously with the variation trend of BEIXYS9> BEIL67 > BEIL69 (Figure 8B). Further analysis of the relation between δTOC and the BEI showed different characteristics in these three wells (Figure 8C). A positive correlation between δTOC and BEI was found in well XYS9: δTOC was low when the brittleness of mudstones was low (BEI<0.5), while the brittle mudstones (BEI>0.5) corresponded to high δTOC. The match of high brittleness and oil content ensured the successful extraction of oil and the formation of industrial oil flow in XYS9 well. While in L67 and L69 wells, although δTOC also had high values, the low brittleness of the mudstones (BEI<0.5) may result in the poor fracturing effect, which inhibited the oil yield of these two wells. Therefore, the effective matching of oil content and brittleness was the key to determine the crude output in the productions process.
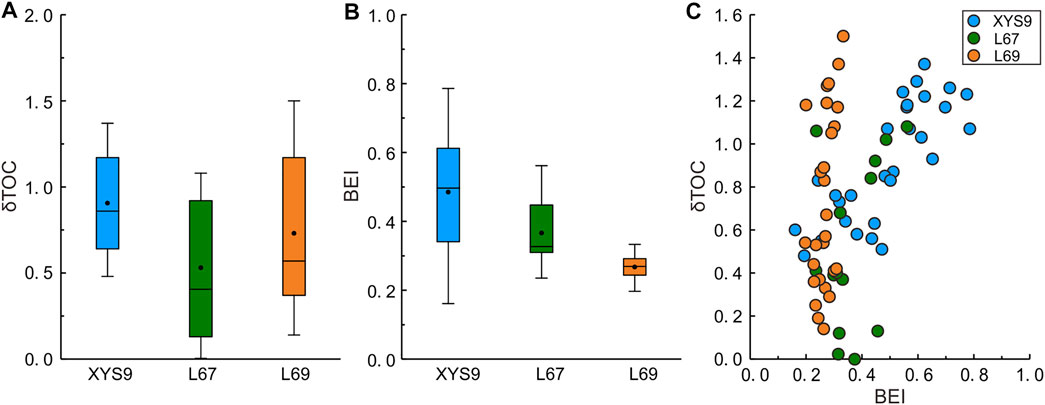
FIGURE 8. The variations of δTOC (A) and BEI (B), as well as their correlation in three wells in Jiyang Depression with different crude output (C).
5 Conclusion
Three mineral assemblages were recognised in mudstones during diagenesis due to the variations of diagenetic environment, thus two diagenetic stages were divided. Above 3,000 m, the early diagenesis corresponded to smectite-kaolinite-cryptocrystalline calcite-detrital mineral assemblage, while below 3,000 m was the late diagenesis, forming illite-microcrystalline calcite-dolomite-detrital mineral and illite-chlorite-fine grained calcite-dolomite-detrital mineral assemblages. Based on the variations of brittle components (e.g., quartz, feldspar, dolomite, illite, and chlorite), a BEI was established to evaluate the mudstone brittleness. According to BEI, the variation of mudstone brittleness showed two-staged characteristics. The mudstone brittleness changed slightly during early diagenesis but increased gradually with enhancement of diagenesis in the late diagenesis stage.
The contribution of brittle minerals to the mudstone brittleness was controlled by diagenesis to some extent. In early diagenesis, quartz and feldspar were scattered above the clay matrix which were mainly composed of ductile components (e.g., smectite/I-Sm and kaolinite). Meanwhile, carbonates were mainly cryptocrystalline, resulting in a failure to form a tightly compacted rock framework. In late diagenesis, clay minerals transformation was noteworthy. In this process, the replacement of brittle illite and chlorite for ductile components and the formation of quartz cement enhanced the integral rigidity of the mudstones. The recrystallization and dolomitization of cryptocrystalline calcite formed microcrystalline or sparry calcite and dolomite. The enhancement of crystallinity resulted in the rigid contact between carbonate grains.
Data Availability Statement
The original contributions presented in the study are included in the article/Supplementary Material, further inquiries can be directed to the corresponding author.
Author Contributions
JD: Methodology, Investigation, Writing - Original Draft, Formal analysis. JC: Conceptualization, Funding acquisition, Supervision, Writing - Original Draft. SL, BG, DF, and ZP: Resources, Investigation. XZ: Methodology, Investigation.
Funding
This work was financially supported by the National Natural Science Foundation of China (Grant Nos. 41672115; 41972126) and Ministry of Science and Technology, Sinopec (Grant No. P19017-6).
Conflict of Interest
Authors JD, SL, BG, DF, and ZP were employed by the company SINOPEC.
The remaining authors declare that the research was conducted in the absence of any commercial or financial relationships that could be construed as a potential conflict of interest.
The authors declare that this study received funding from State Key Laboratory of Shale Oil and Gas Enrichment Mechanisms and Effective Development, SINOPEC. The funder had the following involvement in the study: methodology, data curation, writing -review and editing, funding acquisition.
Publisher’s Note
All claims expressed in this article are solely those of the authors and do not necessarily represent those of their affiliated organizations, or those of the publisher, the editors, and the reviewers. Any product that may be evaluated in this article, or claim that may be made by its manufacturer, is not guaranteed or endorsed by the publisher.
Supplementary Material
The Supplementary Material for this article can be found online at: https://www.frontiersin.org/articles/10.3389/feart.2021.758046/full#supplementary-material
References
Akinbinu, V. A. (2015). Increasing Effect of Metamorphism on Rock Properties. Int. J. Mining Sci. Tech. 25, 205–211. doi:10.1016/j.ijmst.2015.02.007
Bennett, R. H., Bryant, W. R., and Hulbert, M. H. (2012). Microstructure of fine-grained Sediments: From Mud to Shale. New York: Springer.
Bjørlykke, K., and Høeg, K. (1997). Effects on Burial Diagenesis of Stress, Compaction and Fluid Flow in Sedimentary Basins. Mar. Petrol. Geol. 14, 267–276. doi:10.1016/S0264-8172(96)00051-7
Bjørlykke, K., and Jahren, J. (2012). Open or Closed Geochemical Systems during Diagenesis in Sedimentary Basins: Constraints on Mass Transfer during Diagenesis and the Prediction of Porosity in sandstone and Carbonate Reservoirs. Bulletin 96, 2193–2214. doi:10.1306/04301211139
Cai, J., Du, J., Chen, Z., Lei, T., and Zhu, X. (2018). Hydrothermal Experiments Reveal the Influence of Organic Matter on Smectite Illitization. Clays Clay Miner. 66 (1), 28–42. doi:10.1346/CCMN.2017.064083
Du, J., Cai, J., Wang, G., Zeng, X., Bao, Y., and Liu, F. (2019). The Effect of Diagenetic Environment on Hydrocarbon Generation Based on Diagenetic mineral Assemblage in Mudstone. Pet. Sci. Tech. 36, 2132–2142. doi:10.1080/10916466.2018.1528274
Fouché, O., Wright, H., Le Cléac'h, J.-M., and Pellenard, P. (2004). Fabric Control on Strain and Rupture of Heterogeneous Shale Samples by Using a Non-conventional Mechanical Test. Appl. Clay Sci. 26, 367–387. doi:10.1016/j.clay.2003.12.014
Iqbal, O., Ahmad, M., and Kadir, A. a. (2018). Effective Evaluation of Shale Gas Reservoirs by Means of an Integrated Approach to Petrophysics and Geomechanics for the Optimization of Hydraulic Fracturing: A Case Study of the Permian Roseneath and Murteree Shale Gas Reservoirs, Cooper Basin, Australia. J. Nat. Gas Sci. Eng. 58, 34–58. doi:10.1016/j.jngse.2018.07.017
Jarvie, D. M., Hill, R. J., Ruble, T. E., and Pollastro, R. M. (2007). Unconventional Shale-Gas Systems: The Mississippian Barnett Shale of north-central Texas as One Model for Thermogenic Shale-Gas Assessment. Bulletin 91, 475–499. doi:10.1306/12190606068
Jin, X., Shah, S. N., Roegiers, J.-C., and Zhang, B. (2015). An Integrated Petrophysics and Geomechanics Approach for Fracability Evaluation in Shale Reservoirs. J. Nat. Gas Sci. Eng. 20, 518–526. doi:10.2118/168589-pa
Krajewski, K., and Woźny, E. (2009). Origin of Dolomite−Ankerite Cement in the Bravaisberget Formation (Middle Triassic) in Spitsbergen, Svalbard. PPRes 30, 231–248. doi:10.4202/ppres.2009.11
Li, Y., Cai, J., Song, M., Ji, J., and Bao, Y. (2016). Influence of Organic Matter on Smectite Illitization: A Comparison between Red and Dark Mudstones from the Dongying Depression, China. Am. Mineral. 101, 134–145. doi:10.2138/am-2016-5263
Luan, X., Di, B., Wei, J., Li, X., Qian, K., Xie, J., et al. (2014). "Laboratory Measurements of Brittleness Anisotropy in Synthetic Shale with Different Cementation," in Proceedings of the 2014 SEG Annual Meeting. Denver: Society of Exploration Geophysicists, 3005–3009.
Mantovani, M., and Becerro, A. I. (2010). Illitization of Kaolinite: The Effect of Pressure on the Reaction Rate. Clays Clay Miner. 58, 766–771. doi:10.1346/CCMN.2010.0580604
Metwally, Y. M., and Chesnokov, E. M. (2012). Clay mineral Transformation as a Major Source for Authigenic Quartz in Thermo-Mature Gas Shale. Appl. Clay Sci. 55, 138–150. doi:10.1016/j.clay.2011.11.007
Nelson, R. A. (1985). Geologic Analysis of Naturally Fracture reservoirs:Contributions in Petroleum Geology and Engineering. Houston: Gulf Publishing Company.
Pelayo, M., García-Romero, E., Labajo, M. A., and Pérez del Villar, L. (2016). Evidence of montmorillonite/Fe-rich smectite transformation in the Morrón de Mateo bentonite deposit (Spain): Implications for the clayey barrier behaviour. Appl. Clay Sci. 131, 59–70. doi:10.1016/j.clay.2015.12.033
Rybacki, E., Meier, T., and Dresen, G. (2016). What Controls the Mechanical Properties of Shale Rocks? - Part II: Brittleness. J. Pet. Sci. Eng. 144, 39–58. doi:10.1016/j.petrol.2016.02.022
Velde, B., and Vasseur, G. (1992). Estimation of the Diagenetic Smectite to Illite Transformation in Time-Temperature Space. Am. Mineral. 77, 967–976.
Wang, G., Ren, Y., Zhong, J., Ma, Z., and Jiang, Z. (2005). Genetic Analysis on Lamellar Calcite Veins in Paleogene Black Shale of the Jiyang Depression. Acta Sedimentol. Sin. 79, 834–838. [in Chinese with English abstract].
Wang, H. (2016). Numerical Investigation of Fracture Spacing and Sequencing Effects on Multiple Hydraulic Fracture Interference and Coalescence in Brittle and Ductile Reservoir Rocks. Eng. Fracture Mech. 157, 107–124. doi:10.1016/j.engfracmech.2016.02.025
Wen, H., Chen, M., Jin, Y., Zhang, Y., Ge, W., Du, J., et al. (2015). Water Activity Characteristics of Deep Brittle Shale from Southwest China. Appl. Clay Sci. 108, 165–172. doi:10.1016/j.clay.2015.02.015
Xiong, Z., Cao, Y., Wang, G., Yang, Z., Shi, X., Zhang, J., et al. (2018). Controlling Effect of Lacustrine fine-grained Sedimentary Rocks Composition on Fracability. J. China Univ. Mining Tech. 47, 538–548. doi:10.13247/j.cnki.jcumt.000827
Ye, Y., Tang, S., and Xi, Z. (2020). Brittleness Evaluation in Shale Gas Reservoirs and its Influence on Fracability. Energies 13, 388–409. doi:10.3390/en13020388
Zeng, X., Cai, J., Dong, Z., Bian, L., and Li, Y. (2018). Relationship between Mineral and Organic Matter in Shales: The Case of Shahejie Formation, Dongying Sag, China. Minerals 8, 222–242. doi:10.3390/min8060222
Keywords: mudstone, diagenesis, mineralogical assemblage, brittleness evaluation index, Dongying depression
Citation: Du J, Cai J, Long S, Gao B, Feng D, Peng Z and Zeng X (2021) The Control of Diagenesis and Mineral Assemblages on Brittleness of Mudstones. Front. Earth Sci. 9:758046. doi: 10.3389/feart.2021.758046
Received: 13 August 2021; Accepted: 01 December 2021;
Published: 23 December 2021.
Edited by:
Jinbu Li, China University of Petroleum (East China), ChinaReviewed by:
Weichao Tian, Yangtze University, ChinaYingzhu Wang, Institute of Geology and Geophysics (CAS), China
Copyright © 2021 Du, Cai, Long, Gao, Feng, Peng and Zeng. This is an open-access article distributed under the terms of the Creative Commons Attribution License (CC BY). The use, distribution or reproduction in other forums is permitted, provided the original author(s) and the copyright owner(s) are credited and that the original publication in this journal is cited, in accordance with accepted academic practice. No use, distribution or reproduction is permitted which does not comply with these terms.
*Correspondence: Jingong Cai, amdjYWlAdG9uZ2ppLmVkdS5jbg==