- 1Key Laboratory of Mountain Hazards and Surface Processes, CAS/Institute of Mountain Hazards and Environment, CAS, Chengdu, China
- 2University of Chinese Academy of Sciences, Beijing, China
- 3CAS Center for Excellence in Tibetan Plateau Earth Sciences, Chinese Academy of Sciences (CAS), Beijing, China
The Tibetan Plateau suffers from various types of geohazards (collapses, landslides, and debris flows.) due to abrupt changes in complex topography and weather conditions. Global warming and frequent high-intensity earthquakes in recent years have exacerbated the situation. Collapses and landslides provide vast amount of soil and debris which are conveyed downstream by runoff caused by extreme rainfalls to form large-scale debris flows; then, the debris flows block rivers and finally form dam-break floods, that is, a hazard chain triggered by debris flows. Along the evolution direction of the hazard chain, the affected areas are constantly amplified. This study first summarizes the related research studies on river blockage, debris-flow dam failure, and the hazard chain triggered by debris flows and then points out the drawbacks of existing research studies. Overall, the research (including mechanism, risk assessment, key prevention, and control technologies) on the hazard chain triggered by debris flows is still in its infancy and is disconnected among single hazard types in the hazard chain; meanwhile, the understanding of the mechanism of debris flow blocking the river is not enough; the established model and discriminant have minimal application scope, and there is no empirical model and dynamic model of debris-flow dam failure. Finally, several key scientific issues of this field were raised: 1) it is necessary to elaborate the coupling mechanism of debris-flow dam formation and construct the discriminant and numerical model of debris flow blocking the river with high precision and a wide application range. 2) It is necessary to further study the failure mechanism of a debris-flow dam, construct the numerical model of the failure process of a debris-flow dam, and accurately simulate the outburst flood hydrograph. 3) It is necessary to clarify the critical transformation conditions and dynamic evolution process of the hazard chain caused by debris flows, complete the accurate quantitative simulation of the whole disaster chain process, then establish a complete risk assessment system of the hazard chain, and finally develop some key prevention and control technologies suitable for the hazard chain.
1 Introduction
In recent years, river blockages induced by debris flows occur more frequently due to the impact of climate change and earthquakes, especially in case of high-intensity earthquakes (Zhou et al., 2022), which are often accompanied by multiple collapses and landslides (Djalante 2019; Haque et al., 2019; Bai et al., 2020). Occurrence of collapses and landslides leads to change of geological and geomorphic conditions and increases the scale of loose materials in gullies, which is prone to large-scale debris flows when there are heavy rainfalls (Liu and Zhao 2016; Domènech et al., 2019; Zhou W. et al., 2019; Ciccarese et al., 2020; Liu et al., 2020; Zhang et al., 2020). River blockage occurs when abundant loose materials of debris flows are brought from tributaries into the main river in a short time, but the main river does not have enough capacity to carry these materials downstream. It often leads to inundation upstream and outburst flood induced by debris-flow dam failure. This is often referred to as the hazard chain induced by debris flows (Guo et al., 2004; Yin et al., 2009; Chang et al., 2011; Cui et al., 2011; Chen C. G. et al., 2012; Zhuang et al., 2012; Cui et al., 2013; Liu and Zhao 2016).
For example, on 9 April 2000, a huge avalanche-landslide-debris flow broke out in the Zhamu gully, a tributary of Tibet’s Polongzangbu River Basin, which completely blocked the Yigong Zangpo River. The peak discharge of the dam-break flow reached 124,000 m3/s when the debris-flow dam failed and smashed nearly a 30-km highway downstream. A total of 2.5 million residents were displaced with 94 casualties (Song 2015). On 7 August 2010, a large-scale debris flow was triggered by heavy rainfall in Luojiayu and Sanyanyu gullies, Zhouqu County, resulting in 1,364 deaths and 401 missing people. After the debris flow passed through the county of Zhouqu, the Bailong River was blocked and inundated half of the county (Supplementary Figure S1) (Zhang et al., 2007; Hu et al., 2010; Tang et al., 2011). At around 23:00 on 5 July 2016, a glacier lake (Cirenmacuo Lake) outburst flood transformed into debris flows in Zhangzangbu, Nyalam County. Soon after the river was blocked, dam breach occurred, flooded the Zhangmu port and hydropower facilities, and destructed road and houses in the downstream towns of Kodari and Tatopani in Nepal (Cook et al., 2018; Liu J. K. et al., 2019). On 17 October 2018, a large-scale debris flow broke out in the Sedongpu gully of Milin County, Tibet. The debris flow blocked the Palalung Zangbo River and formed a barrier dam (height 77–106 m, width 3,500 m, and volume 30 × 106 m3). The reservoir capacity of the barrier lake reached 3260 × 106 m3, and the flood peak flow reached 18,000 m3/s after 56 h, which caused important losses to the upstream and downstream (Liu C. z. et al., 2019) (Supplementary Figure S2).
The dynamic evolution process of the hazard chain induced by debris flows is shown in Figure 1. It mainly includes two kinds of secondary hazards: debris flow blocking the river and debris-flow dam failure.
Based on a brief review of the literature on single mountain hazard types (river blockage and debris-flow dam failure) in the hazard chain triggered by debris flows, this study points out the issues occurred in these studies. Then, research studies on the hazard chain of debris flows blocking the river and dam-break flood are discussed, and some unresolved key issues are put forward.
2 Mechanism of River Blockage Induced by Debris Flows
2.1 Influencing Factors
Debris flows are mass movement of viscous and highly concentrated fluid–solid mixture between hyper-concentrated flows and soil mass (Prochaska et al., 2008; Hu et al., 2012; Namgyun et al., 2019). When a debris flow is blocking a river, there is a complicated interaction between the debris flow and the main river, adding difficulty to the identification of related influencing factors. Selecting influencing factors is fairly important to establish a discriminant with accuracy while simplifying the mathematical model. Therefore, multiple research studies are carried out to identify influencing factors in river blockings induced by debris flows (Table 1).
Table 1 indicates the complexity and diversity of related influencing factors, including the total volume of debris flows, gravity, discharge and width of the main river, ratio of the main river and other discharges, and angle of intersection. The aforementioned influencing factors can be mainly summarized into three categories: main rivers, debris flows, and other comprehensive influencing factors. In addition, although significant progress has been made in studying these related influencing factors, it is worth noting that some of these results are only qualitative descriptions, limiting the applications to other cases. In particular, the process and mechanism of river blockage induced by debris flows under the coupling of multiple factors are not clear, which should be considered by means of experiments and numerical simulation studies in the future.
2.2 Main River Blocking Patterns
There are three river-blocking patterns induced by debris flows: complete blockage, partial blockage, and submerged dam blockage (Chen C. g. et al., 2012; Chen et al., 2013; Liu and Zhao 2016). Complete blockage (Figure 2A) refers to a debris flow flowing into the mainstream with vast amount of solid materials. The flow front rapidly reaches the opposite bank and completely blocks the main river. Partial blockage (Figure 2B) means that part of the flow front moves forward above the water surface. However, it cannot reach the opposite bank due to insufficient total mass or strong dynamic conditions of the main river. Blockage caused by submerged dam (Figure 2C) means that due to the small scale of the debris flows or the depth of the main river, the flow front can only move forward below the water surface and then form a submerged dam that partly blocks the river (Liu and Yao 2012).
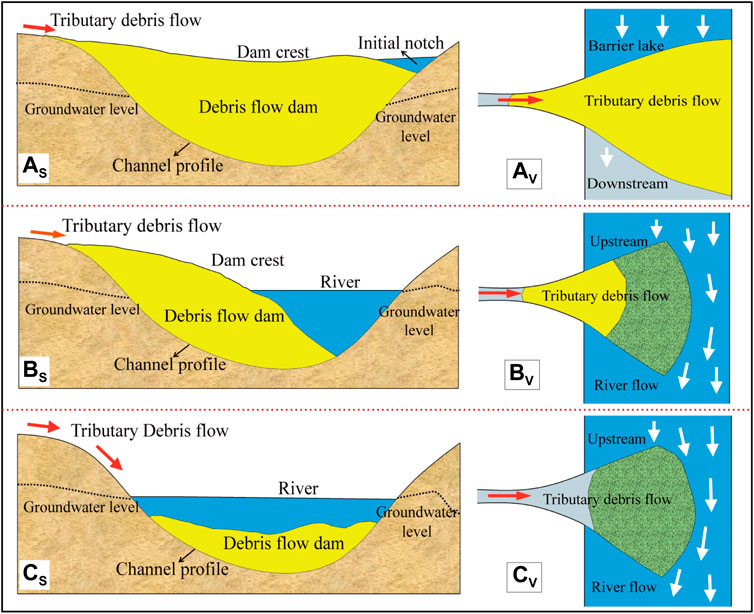
FIGURE 2. Three river-blocking patterns induced by debris flows: (A) Complete blockage, (B) partial blockage, and (C) submerged dam blockage. The subscript “s” in the figure number represents the “sectional view” and “v” represents the “vertical view.”
Compared with partial blockage and submerged dam blockage, complete blockage can heavily increase the upstream water level and result in serious damage. In order to predict river blockages and take effective hazard mitigation measures, many scholars select key influencing factors based on dimensionless analysis or specific parameter analysis to study the threshold of river blocking induced by debris flows. The judgment formula is shown in Table 2. In addition, it should be noted that although the risk of partial blockage patterns is lower than that of complete blockage patterns, it is easy to cause serious erosion of the opposite bank and cause secondary geological disasters, such as landslides and collapses (Figure 3). Therefore, all river-blocking patterns should be further deeply studied in the future.
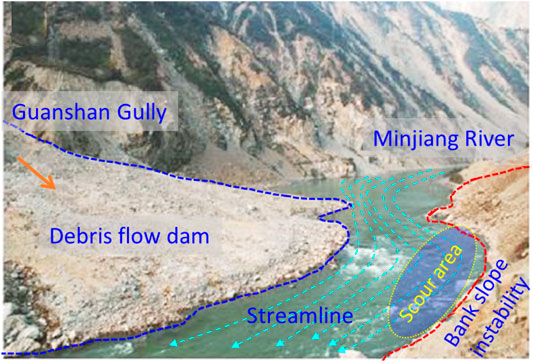
FIGURE 3. Partial blockage pattern of debris flow causes serious scouring on the opposite bank (In 2008, a debris flow occurred in Guanshan gully, Sichuan province, China, partially blocking the Minjiang River. After the sharp deflection of the river flow streamline, the bank slope was scoured, resulting in the instability and failure of the opposite bank slope).
Due to the complexity of the influencing factors and the mechanism in general, it is difficult to establish a discriminant with comprehensive influencing factors. The selection index is simple, and the application scope of the established discriminants is restricted to a certain extent. The main reason behind that is the limited understanding in the mechanism of river blockage. Therefore, the discriminants should be selected cautiously when it is applied, and a discriminant with a larger scope of application should be established considering multiple key influencing factors.
2.3 River Blocking Mechanism
2.3.1 Experimental Study of River Blocking
The process of river blocking induced by debris flows is essentially a complex process of interactions between Newtonian fluid (water) and non-Newtonian fluid (debris flows) (Chen et al., 2011; Maria et al., 2014; Stancanelli et al., 2015). Through flume experiments, we can have an intuitive preliminary understanding of the process of debris flows blocking the river. When the debris flows enter into the main river, most coarse particles such as boulders and cobbles deposit at the gully mouth, which changes the local boundary conditions of the confluence area (Maria et al., 2014). The debris flow head, driven by the high momentum, continues to advance to the opposite bank of the main river (Wang et al., 2017). Under the strong hydraulic condition of the main river, the fine particles from debris flows are carried away by the water flow. The sediment concentration and velocity distribution of the solid–liquid two-phase flow at the confluence are characterized as “small and fast in the upper part and large and slow in the lower part” (Chen et al., 2013). When the head of the debris flows reaches the opposite bank and a large volume continues to flow into the river, the main river can be completely blocked; thus, a dam is formed (Wang et al., 2017). In case of a confluence, viscous debris flows are prone to move and accumulate as a whole, while the dilute ones are easier to be carried away in the way of suspended and bed loads, respectively (Chen et al., 2013).
In addition, Chen et al. (2004b)carried out experiments to study the confluence process of the debris flows and water flows. It was found that the inflow angle and the velocity ratio of the main river and tributaries have a great impact on the position and shape of sediment accumulation. Based on the theory of density flow, the motion equation of the debris flow front in the main river was deduced, and the calculation formula of its movement distance was given by (Liu C. R. et al. 2013; Liu C. R. et al. 2014; Liu and Zhao 2016), but these results have not been verified by examples. Chen (2016) studied the intersection process of debris flows and the main river using the flume model test and calculated the width of the debris-flow dam plug by momentum conservation theory; however, these results also have not been verified by examples. At the same time, flume experiments are likely to mask many important experimental phenomena in the prototype. Therefore, it is necessary to further strengthen the verification of experimental results and fully consider the similarity between the model and the prototype, including geometric similarity, kinematic similarity, and dynamic similarity, so that the results of small-scale model experiments can be directly applied to the prototype. Furthermore, based on macroscale analysis of the basin, Lv et al. (2016) pointed out that the two-phase debris flows blocked the Nujiang River, forming a large number of dam Nick points, changing the river flow pattern, causing a large amount of sediment deposition in the lake, and limiting riverbed undercutting. Although the results of this study are qualitative, it can inspire us: in the study of the debris flow blocking the river process, in addition to the intersection process and mechanism of debris flows and the main river, we should also pay attention to the influence of the intersection process on the upstream and downstream of the intersection area, including the upstream and downstream hydraulic characteristics and sediment transport characteristics.
Although research on the threshold of river blocking based on empirical expressions has made some progress, there are few achievements in the quantitative description of its mathematical models by the view point of the hydrodynamic process of water and sediment.
2.3.2 Numerical Simulation of River Blocking
Compared with the traditional flume model test, application of numerical simulation methods to study and analyze the river-blocking mechanism induced by debris flow is more cost-effective, time-saving, and is easier to change parameters (Szczukiewicz et al., 2014; Celis et al., 2017).
Combining with particle-in-cell (PIC) and marker and cell (MAC) algorithms, Chen et al. (2013) proposed a two-field coupling model for the interaction between the debris flow and the main river. The main characteristic of the model is that the rheological parameters and sediment settling velocity in the calculation unit of a confluence may be expressed with the aid of the specific weight of mixed fluids. In addition, based on the weir flow theory, a numerical calculation method to figure out the main river depth and debris flow velocity after river blockage was established by Chen C. G. et al. (2012). However, the shock wave at the initial debris flow intersection was not fully considered in the model. Liu and Yao (2012) applied the finite volume method to simulate and analyze the flow field of the main river under different blocking coefficients of debris flows in the partial blockage pattern; however, in the model, the debris flow blockage body is generalized as circular, which is inconsistent with the actual fan-shaped accumulation body, and under the action of the main river flow, the fan edge will inevitably shift in the water flow direction (Figure 4). Some commonly used fluid mechanics simulation software applications have also been used to simulate the debris flow movement and accumulation process. For example, CFX software was used by Han et al. (2016) and Xu et al. (2019) to simulate debris flows in the Mozi gully in Wenchuan County and Shiwulong Gully in Rantang County, respectively; FLO-2D software was used by Wang et al. (2017) to simulate and analyze river blockage patterns of the Guangyuanbao gully debris flows under different rainfall intensities. Although these pieces of software have high accuracy in the simulation of debris flow movement, there are large errors in the simulation of the debris flow accumulation process because these pieces of software do not consider the interaction between the debris flow and the main river water flow in the simulation.
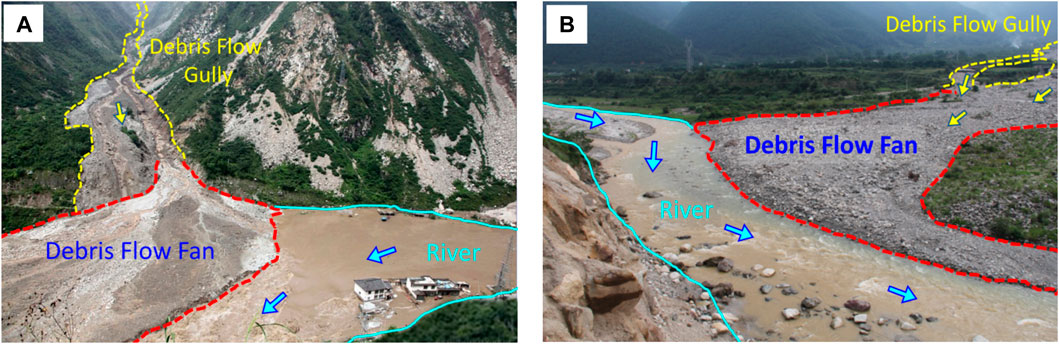
FIGURE 4. (A) On July 3, 2011, the debris flow in Gaojiagou Gully, Yinxing Township, Wenchuan County, Sichuan Province blocked the Minjiang River. (B) On June 26, 2020, a debris flow in Yihai Town, Mianning County, Sichuan Province blocked Caogu River..
Therefore, for the complex process involving sediment dynamics, hydrodynamics, and soil dynamics of the debris flow blocking the river, it is necessary to consider using other relatively novel numerical simulation methods, such as DDA (Fu et al., 2020), MPM (Du et al., 2021), and NMM (Yang et al., 2019, 2020; Zheng et al., 2019). Table 3 lists some new numerical simulation methods in recent years, which can be used to simulate the process of debris flows blocking the river.
All the aforementioned studies are aimed at analyzing river blockage induced by the viscous debris flow. The main reason is that diluted debris flows are accompanied by the sedimentation of large particles during the movement. The internal composition is highly heterogenous, and the related mechanism is far more complicated. Furthermore, the results of numerical simulation are quite different from reality since the numerical simulation parameters of the debris-flow dams are difficult to be calibrated, and the model’s boundary conditions and dynamic equations are oversimplified. Hence, numerical simulation studies on the process of river blocked by diluted debris flows are rare at present.
3 Mechanism of Debris-Flow Dam Failure
The internal composition of debris flows and the dynamic process of river blockage are complex. There are great differences between the dams induced by debris flows and the ones induced by the landslides (or maimora dam) (Wang et al., 2017; Wu et al., 2020). The former is difficult to maintain long-term stability and often bursts soon, and dam failure often occurs when there is an overtopping flow (Yan et al., 2009). The main differences between different types of barrier dams are shown in Table 4. These differences lead to the inevitable differences between the collapse process and the mechanism of the debris-flow dam and other dam types. For example, the former is difficult to maintain long-term stability and often bursts soon, and dam failure often occurs when there is an overtopping flow (Yan et al., 2009).
There are three failure modes of a barrier dam: overtopping, piping, and slope instability (Figure 5) (Peng and Zhang 2012; Shrestha and Nakagawa 2016; Dhunagan and Wang 2019; Kumar et al., 2019; Jiang et al., 2020; Ruan et al., 2021). However, because the debris-flow dams are mostly saturated, they have high clay content and strong water-retaining property; moreover, the height and slope of debris-flow dams are much smaller than other dams (landslide dam and moraine dam), and the seepage path is longer (Chen et al., 2011; Liao et al., 2012; Pan et al., 2013; Liu et al., 2015; Neupane et al., 2019; Sattar et al., 2019; Zou et al., 2020). Therefore, it is very difficult for the debris-flow dam to have piping or dam slope failure.
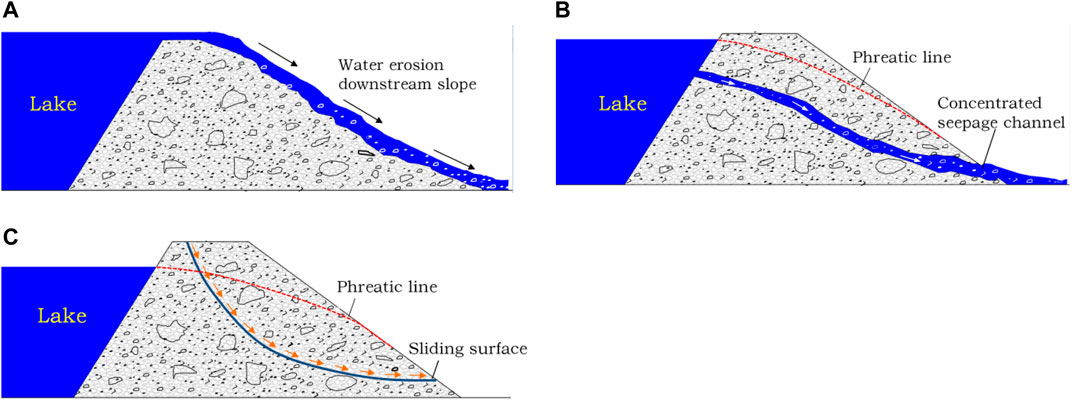
FIGURE 5. Failure modes of a debris-flow dam: (A) Overtopping, (B) piping, and (C) slope instability.
In the process of debris-flow dam failure, based on the flume model experiment, Ruan et al. (2021) drew on the idea of dividing landslide dams and divided the failure process of debris-flow dams into three stages according to the longitudinal evolution characteristics of the breach: the formation stage of the retrogressive scarp, the erosion stage of the retrogressive scarp, and the decline stage of the retrogressive scarp (Figure 6). Different from landslide dams, the debris-flow dam can form a very obvious retrogressive scarp in the process of failure. In addition, in a recent study, we found that the erosion rate and peak discharge of debris-flow dam failure were significantly higher than those of landslide dams under the same conditions, and the peak time of debris-flow dam failure was slower (Figure 7). Therefore, in the future, it is necessary to further compare and study different types of dams (the debris-flow dam, landslide dam, and moraine dam) and construct the theory and model of different types of dams.
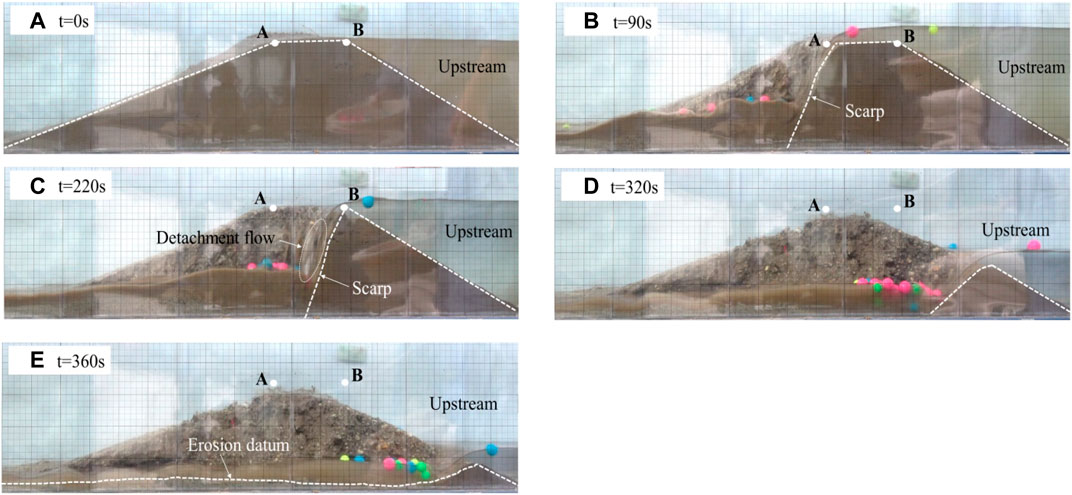
FIGURE 6. Longitudinal evolution process of the debris-flow dam breach. (A,B) Formation stage of the retrogressive scarp (0–90 s); (B–D) Erosion stage of the retrogressive scarp (90–320 s); and (D,E) Decline stage of the retrogressive scarp (320–360 s) (Ruan et al., 2021).
A reasonable description of the development process of the breach (the longitudinal downcutting process and cross widening process) is a prerequisite for accurate simulation of the outburst flood. At present, several models of the breach evolution of barrier dams have been proposed (Figure 8). The models of Chang and Zhang (2010), Zhong et al., (2017), and Zhou G. G. D. et al. (2019) are only applicable to landslide dams. When Chang and Zhang’s (2010) and Zhong et al’s., (2017) models are used to simulate the outburst flood, the cross-sectional shape of the breach is assumed to be trapezoidal (Figures 8A,B). Zhou G. G. D. et al’s. (2019) model does not have a calculation model for the cross widening of the breach and a complete calculation model for the longitudinal evolution of the breach (Figure 8C), which cannot be applied to numerical simulation. Ruan et al. (2021)obtained the longitudinal evolution model of a debris-flow dam through flume experiments (Figure 8D), but the bottom slope and velocity of the breach in the model are difficult to obtain in advance (Formula 1), and there is no cross expansion model of the breach, which cannot be applied to numerical simulation.
where E is the erosion rate (cm/s); μ and Dc are grain-size distribution parameters (Li et al., 2013; Li et al. 2015; Li et al. 2017); v is the flow velocity of the breach (m/s); and θ is the longitudinal slope of the breach (°).
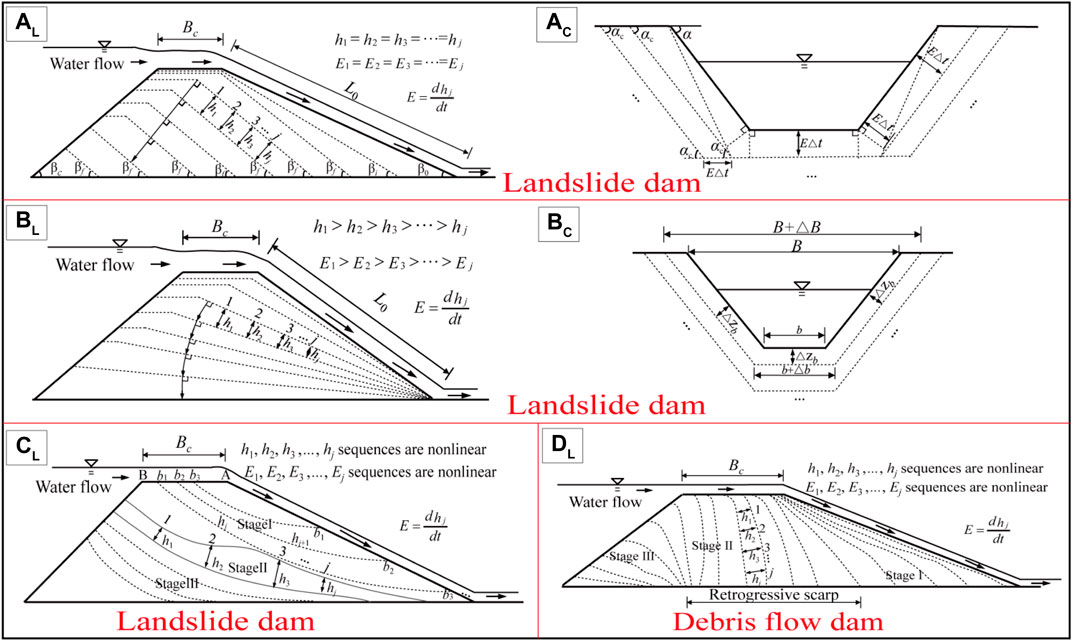
FIGURE 8. Evolution models of the breach of the barrier dam. (A) Evolution model of the breach of the landslide dam proposed by Chang and Zhang (2010) (modified). (B) Evolution model of the breach of the landslide dam proposed by Zhong et al. (2017) (modified). (C) Evolution model of the breach of the landslide dam proposed by Zhou G. G. D. et al. (2019) (modified). (D) Evolution model of the breach of the debris-flow dam proposed by Ruan et al. (2021) (modified). The subscript “L” in the figure number represents the “longitudinal evolution of the breach” and “V” represents the “cross evolution of the breach”.
The numerical simulation of debris-flow dam failure is still in the preliminary exploration stage. Depending on the gradual breach model (Zhang and Huang 2007; Huang 2008), Zhao et al. (2009) stimulated the flood discharge process and calculated the peak discharge of the debris-flow dam in the Kezi River, Xinjiang. In this model, it is assumed that the cross-sectional shape of the breach is trapezoidal. However, for high viscous debris-flow dams, the failure process is often accompanied by intermittent large-scale collapse of the negative angle breach slope (Ruan et al., 2021). Moreover, Liu Hd et al. (2013) calculated the stability of a debris-flow dam by FLAC3D software, which can be used to determine the failure possibility of the debris-flow dam. However, the stability of a debris-flow dam is not the focus of the study because the length of a debris-flow dam along the river is usually very long, and the upstream and downstream dam slopes are much slower than those of the landslide dam. It is difficult to understand the instability of the dam slope, and the overtopping failure is the focus of the study of the debris-flow dam. Table 5 summarizes several dam-break models based on dynamics proposed in recent years, which can simulate the evolution process of the break and the dam-break flood hydrograph, but they are only applicable to the simulation calculation of a landslide dam. In the future, these models can be improved according to the characteristics of debris-flow dams, and then, some dam-break models suitable for debris-flow dams can be obtained.
In summary, little research on the failure of debris-flow dams has been conducted until now. The existing research studies mainly focus on the failure mode and process of dam break. Research on the failure mechanism of a landslide dam, especially the evolution law of the debris-flow dam of the breach, including longitudinal and cross evolution laws of the breach, is relatively limited. In addition, there are no numerical models and theoretical calculation methods that can completely and reasonably describe the evolution law of the breach of the debris-flow dam (undercutting erosion and lateral collapse of the breach) and the flow discharge during the dam failure process. The dynamic model of the debris-flow dam break should be constructed in the future. Furthermore, from Table 6, it can be found that there is no empirical formula for the peak discharge of dam break that is applicable to debris-flow dams, which is also the focus of future studies.
4 Hazard Chain Triggered by River-Blocking Debris Flows
Mountain hazards often include collapses, landslides, debris flows, and glacial lake outburst floods (GLOF). (Zhong et al., 2017; Begam et al., 2018; Bhambri et al., 2019; Medwedeff et al., 2020; Shen P. et al., 2020). However, sometimes due to factors such as limited space, large-scale or extra large-scale mountain hazards often transform from one kind to another, forming hazard chains and causing severe damages (Shulmeister et al., 2009; Xu et al., 2014). There are many variants of mountain hazard chains, with different combinations of disaster types (Zhong et al., 2013). “The debris-flow river-blocking debris-flow dammed-lake dam-break flood” is only a common type of the mountain hazard chain. Due to the distinctions in material composition, structure, movement form, and enlargement scale, the dynamic process of river blocking and dam failure caused by debris flows is significantly different from that caused by collapse or landslides.
4.1 Basic Characteristics and Mechanism
Attention was paid to formation, basic characteristics, distribution laws, hazard risk analysis, and prevention measures of debris flows in the last couple of years. However, less attention was paid to the hazard chain caused by debris flows. Cui et al. (2008) pointed out that Wenchuan earthquake-induced debris flows→dammed lakes→burst floods and secondary disasters were distributed intensively along the fracture zone and its two sides from the perspective of regional spatial analysis, and it did not involve the transformation process and mechanism of disasters. Xu et al. (2012) analyzed the formation conditions and chain formation mechanism of four types of disasters chains and found that in all these disaster chains, each episode was caused by the previous episode. Moreover, the amount of mass movement decreased along the disaster chains. Zou et al. (2013)investigated and analyzed some typical hazard chains after the Wenchuan earthquake and pointed out that a complete hazard chain process includes four key stages: disaster formation, the triggering stage, damage stage, and chain-breaking stage; but these analyses were qualitative. Zhong et al. (2013)analyzed the factors causing a mountain hazard chain and pointed out that the formation of mountain hazard chains is the result of interaction, infiltration, transmission, and transformation of materials and energy of each disaster under specific conditions. The most remarkable characteristic of a disaster chain is the obvious amplification effect in the evolution process of the disaster chain. However, there are very few quantitative research studies on the amplification effect of disaster chains caused by debris flows, which is the focus of future research. He (2014) preliminarily simulated and calculated the river-blocking debris flow and dam-break hazard chains caused by the so-called “8.13 Hongchun gully large-scale debris flow” from three aspects: the impact force of the debris flow, peak discharge of dam breaks, and the evolution process of flood outburst; but these three parts are separated in the analysis process. Fan et al., (2020) first integrated the rock mass stability model (FLAC3D and RocPlane tools), landslide sliding MassFlow program, dam-break DABA program, and dam-break flood HEC-RAS program to simulate the whole process of river blocking and collapse hazard chains which might be caused by three potential landslides in the Baige landslide dam. Although each model has its limitations to some extent, the simulation process has error accumulation; the study only focuses on the river-blocking landslide break hazard chain, and it provides a new idea for the study of river-blocking debris flow-dam break hazard chains and other types of disaster chains (Figure 9). In addition to numerical simulation, experimental research can also be used as an important research method for future research on the disaster chain.
The transformation among disaster types is the key in forming a hazard chain, making the critical conditions of the transformation a prerequisite for the formation of a hazard chain. Although achievements have been made in studying critical conditions of river-blocking debris flows and the dam-break model, the two are studied separately. In order to get a better understanding of the transformations in the hazard chain and quantitative descriptions of the whole process, it is necessary to conduct a systematic and in-depth analysis of the whole hazard chain and the evolution process of each knot in the chain.
4.2 Risk Assessment and Key Prevention and Control Technologies
Risk assessment of a single mountain-hazard type has been relatively mature after years of development. Based on empirical methods and numerical simulation methods (Tacconi Stefanelli et al., 2020; Choi et al., 2021), a relatively mature mountain-hazard risk assessment system has been established. However, in the context of global warming, a single mountain-hazard type is often transformed to form a hazard chain with strong destructive power (Fan et al., 2020), which also brings many challenges to the risk assessment of the hazard chain. For example, the specific challenges of the risk assessment of the hazard chain caused by debris flows are as follows. 1) The hazard chain caused by debris flows mainly includes three single types of hazards (the debris flow, weir dam, and outburst flood). The three single types of hazards occur continuously and follow their respective physical laws and transformation mechanisms. Therefore, in the risk assessment, it is necessary to clarify the transformation mechanism of three single types of hazards and systematically evaluate the entire hazard chain. For example, the physical properties of the debris flow in the process of movement are changing at all times, and there are erosion and scale amplification effects along the movement direction (Chen et al., 2020). The characteristics of the dam after debris flow blocking the river and the evolution of the outburst flood need to be fully considered. 2) In the risk assessment, it is necessary to take full account of the hazard-bearing bodies within the impact range of the entire hazard chain, but the impact range is often hundreds of kilometers or even thousands of kilometers. The hazard-bearing bodies involved mainly include roads, railways, bridges, villages, and farmland. The workload of the investigation is very huge. Hence, the traditional risk assessment method of a single hazard type cannot meet the quantitative assessment requirements of many mountain hazard chains. On this basis, it is necessary to further consider the relationship between various single hazards to truly describe the risks brought by the evolution process of the mountain hazard chain.
At present, the key technologies for debris flow prevention and control have been studied in depth. A variety of debris flow prevention and control projects have been proposed, such as check dams (Kim and Kim 2021) and drainage channels (Wang et al., 2021), and have been widely applied to effectively reduce the risk of debris flow hazards. In terms of the debris-flow dam, there is no key technology for the prevention and control of the debris-flow dam, and the related prevention and control technology of the landslide dam can be used for reference in the future. Most importantly, for the large-scale hazard chains occurring in the alpine gorge area, such as the hazard chain caused by debris flows, the conventional key technologies for the prevention and control of single disaster types cannot meet the needs of hazard reduction. Therefore, on the basis of clarifying the evolution mechanism of hazard chains, we need to develop new materials and structural prevention and control projects suitable for strong earthquake, high altitude, extremely cold and large temperature difference working environments, explore new hazard risk prevention and control principles, and regulate the whole process of hazard chain material and energy. In addition, effective engineering measures are needed to control the key links of hazard chain and cut off the critical conditions of disaster chain transformation.
5 Conclusion and Prospects
Although some progress has been made in studying debris flows that block the main river, the investigations on hazard chains of river-blocking debris flows and dam-break floods are still at an initial stage. Some conclusions and several frontier scientific issues on the river-blocking mechanism by debris flows and dam failure hazard chains are drawn as follows:
1) There are three patterns of river-blocking debris flows: complete blockage, partial blockage, and submerged dam blockage. Although the river-blocking factors have already been considered comprehensively and varied discriminants were established, studies on river blocking are mostly restricted at laboratory scales (flume tests), which limit the applications of the established river-blocking discriminants because the characteristics of the debris flows, the shape of the main river, and its hydrogeological conditions are unique in real-world scenarios. In addition, studies on the mechanism of river blocking by debris flows mainly focus on describing the macro phenomena. There are fewer quantitative achievements in describing the mathematical model of the dynamic process in water–particle interactions, which is also the main reason for low accuracies in the simulation results. In this regard, it is necessary to elaborate on the coupling mechanism of debris-flow dam formation, and then, a discriminant and numerical simulation model of debris flow blocking the river with multiple influencing factors and larger application scope should be established in the future. In the meantime, a large-scale model test is worth considering in future studies.
2) At present, studies on the breach of debris-flow dams mainly focus on the breach mode and dynamic failure process by considering different particle size distribution, bulk density, and clay content of a debris-flow dam, not on the breach mechanism, especially the law of the breach development (undercut and side collapse of the breach). There is no numerical model or theoretical calculation method that can reasonably reflect the evolution law of the breach and the discharge hydrograph of the outburst flood. It is vital to estimate the peak discharges of the outburst flood and erosion rate of dam materials accurately in order to predict the dynamic failure process of the dam with higher precision. Hence, in the future, it is necessary to further strengthen the research on the mechanism of debris-flow dam failure, accurately describe the evolution process of the breach, establish the physical model of debris-flow dam failure based on dynamics, and establish a prediction model of the peak discharge of debris-flow dam failure.
3) The hazard chain of river-blocking debris flows and dam failure is a common type of mountain hazard chain, with continuous evolution and strong destructive power. Although researchers have made some progress in the blockage and breach models, the investigations are designed and conducted separately. Also, related studies are still lingering over the initial stage, mainly focusing on typical case studies of the hazard-inducing environments, inducing factors, basic characteristics, and prevention measures. In addition, the research study on the risk assessment and key prevention and control technology of the disaster chain caused by debris flows is still in the initial stage of exploration. The traditional risk assessment theoretical methods and key prevention and control technology for a single disaster type are no longer applicable to the disaster chain caused by debris flows, especially because the current understanding of the whole dynamic evolution process of the disaster chain is not enough. In this regard, it is worth noticing the critical conditions and dynamic evolution process of hazard type transformation, conducting in-depth and systematic investigations on the hazard chain, and completing a quantitative description of the whole process of the hazard chain. Finally, a complete hazard chain risk assessment system should be established, and some key prevention and control technologies suitable for the hazard chain should be developed as well, including new materials, prevention and control projects, and emergency plan measures.
Author Contributions
HC and HR finished the manuscript. XL and YY collected the data and references. JC, HC, and HR modified the manuscript. All authors contributed to the manuscript and approved the submitted version.
Funding
The research reported in this manuscript is funded by the National Key R&D Program of China (Grant No. 2018YFE0100100), the National Natural Science Foundation of China (Grant Nos. U20A20112 and 41771045), and the CAS Light of West China Program.
Conflict of Interest
The authors declare that the research was conducted in the absence of any commercial or financial relationships that could be construed as a potential conflict of interest.
Publisher’s Note
All claims expressed in this article are solely those of the authors and do not necessarily represent those of their affiliated organizations, or those of the publisher, the editors, and the reviewers. Any product that may be evaluated in this article, or claim that may be made by its manufacturer, is not guaranteed or endorsed by the publisher.
Supplementary Material
The Supplementary Material for this article can be found online at: https://www.frontiersin.org/articles/10.3389/feart.2022.830044/full#supplementary-material
References
Bai, S., Lu, P., and Thiebes, B. (2020). Comparing Characteristics of Rainfall- and Earthquake-Triggered Landslides in the Upper Minjiang Catchment, China. Eng. Geology. 268, 105518. doi:10.1016/j.enggeo.2020.105518
Begam, S., Sen, D., and Dey, S. (2018). Moraine Dam Breach and Glacial lake Outburst Flood Generation by Physical and Numerical Models. J. Hydrol. 563, 694–710. doi:10.1016/j.jhydrol.2018.06.038
Benn, D. I., and Owen, L. A. (2002). Himalayan Glacial Sedimentary Environments: A Framework for Reconstructing and Dating the Former Extent of Glaciers in High Mountains. Quat. Int. 97-98, 3–25. doi:10.1016/S1040-6182(02)00048-4
Bhambri, R., Hewitt, K., Kawishwar, P., Kumar, A., Verma, A., Snehmani, , et al. (2019). Ice-dams, Outburst Floods, and Movement Heterogeneity of Glaciers, Karakoram. Glob. Planet. Change 180, 100–116. doi:10.1016/j.gloplacha.2019.05.004
Celis, M. A. C., Wanderley, J. B. V., and Neves, M. A. S. (2017). Numerical Simulation of Dam Breaking and the Influence of Sloshing on the Transfer of Water between Compartments. Ocean Eng. 146, 125–139. doi:10.1016/j.oceaneng.2017.09.029
Chang, D. S., and Zhang, L. M. (2010). Simulation of the Erosion Process of Landslide Dams Due to Overtopping Considering Variations in Soil Erodibility along Depth. Nat. Hazards Earth Syst. Sci. 10, 933–946. doi:10.5194/nhess-10-933-2010
Chang, D. S., Zhang, L. M., Xu, Y., and Huang, R. Q. (2011). Field Testing of Erodibility of Two Landslide Dams Triggered by the 12 May Wenchuan Earthquake. Landslides 8, 321–332. doi:10.1007/s10346-011-0256-x
Chen Cg, C. G., Yao, L. K., Yang, Q. H., and Liu, C. R. (2012). Study of River-Blocking Due to Debris Flow Based on Weir Flow Theory. J. Nat. Disasters 21, 131–135. (In Chinese). doi:10.1088/1674-1056/21/10/106802
Chen, C. G., Yao, L. K., and Yang, Q. H. (2004b). Experimental Research on confluence between Debris Flow and Main River. Journal of Southwest Jiaotong University: Chengdu, 10–14. (In Chinese). doi:10.3969/j.issn.0258-2724.2004.01.003
Chen, C. G., Yao, L. K., Liu, C. R., and Du, C. (2013). Study on Conditions of River-Blocking Due to Debris Flow. J. Hydraulic Eng. 44, 648–656. (In Chinese). doi:10.13243/j.cnki.slxb.2013.06.002
Chen, D. M., Wang, Z. Y., and He, Y. (2002). Experimental Study on the Fluvial Process of Debris Flow Discharging into a River. J. Sediment Res. 213, 22–28. (In Chinese). doi:10.16239/j.cnki.0468-155x.2002.03.004
Chen, J., Wang, D., Zhao, W., Chen, H., Wang, T., Nepal, N., et al. (2020). Laboratory Study on the Characteristics of Large wood and Debris Flow Processes at Slit-Check Dams. Landslides 17, 1703–1711. doi:10.1007/s10346-020-01409-3
Chen, R., Liu, X., Cao, S., and Guo, Z. (2011). Numerical Simulation of deposit in confluence Zone of Debris Flow and Mainstream. Sci. China Technol. Sci. 54, 2618–2628. doi:10.1007/s11431-011-4510-1
Chen, Z., Ma, L., Yu, S., Chen, S., Zhou, X., Sun, P., et al. (2015). Back Analysis of the Draining Process of the Tangjiashan Barrier Lake. J. Hydraul. Eng. 141, 05014011. doi:10.1061/(ASCE)HY.1943-7900.0000965
Chen, Z. S. (2016). Study on the Characteristics of Water Mud Flow after Debris Flow Entering the Main River. Sichuan. Southwest Jiaotong University: Chengdu. (In Chinese).
Cheng, Z., Dang, C., Liu, J., and Gong, Y. (2007). Experiments of Debris Flow Damming in Southeast Tibet. Earth Sci. Front. 14, 181–185. doi:10.1016/s1872-5791(08)60010-x
Choi, G.-M., Lee, I.-W., and Yune, C.-Y. (2021). Risk Assessment of Debris Flow at Regional Scale Considering Catchment Area in Chuncheon, Korea. KSCE J. Civ Eng. 25, 1176–1190. doi:10.1007/s12205-021-0136-4
Ciccarese, G., Mulas, M., Alberoni, P. P., Truffelli, G., and Corsini, A. (2020). Debris Flows Rainfall Thresholds in the Apennines of Emilia-Romagna (Italy) Derived by the Analysis of Recent Severe Rainstorms Events and Regional Meteorological Data. Geomorphology 358, 107097. doi:10.1016/j.geomorph.2020.107097
Clague, J., and Evans, S. (2000). A Review of Catastrophic Drainage of Moraine-Dammed Lakes in British Columbia. Quat. Sci. Rev. 19, 1763–1783. doi:10.1016/S0277-3791(00)00090-1
Cook, K. L., Andermann, C., Gimbert, F., Adhikari, B. R., and Hovius, N. (2018). Glacial lake Outburst Floods as Drivers of Fluvial Erosion in the Himalaya. Science 362, 53–57. doi:10.1126/science.aat4981
Costa, J. E., and Schuster, R. L. (1991). Documented Historical Landslide Dams from Around the World. US Geological Survey Open-File Report: Vancouver, 91–239. doi:10.3133/ofr91239
Cui, P., Chen, X.-Q., Zhu, Y.-Y., Su, F.-H., Wei, F.-Q., Han, Y.-S., et al. (2011). The Wenchuan Earthquake (May 12, 2008), Sichuan Province, China, and Resulting Geohazards. Nat. Hazards 56, 19–36. doi:10.1007/s11069-009-9392-1
Cui, P., Wei, F. Q., Chen, X. Q., and He, S. M. (2008). Geo-hazards in Wenehuan Earthquake Area and Countermeasures for Disaster Reduetion. Bull. Chin. Acad. Sci. 42, 317–323. doi:10.16418/j.issn.1000-3045.2008.04.004
Cui, P., Zhou, G. G. D., Zhu, X. H., and Zhang, J. Q. (2013). Scale Amplification of Natural Debris Flows Caused by Cascading Landslide Dam Failures. Geomorphology 182, 173–189. doi:10.1016/j.geomorph.2012.11.009
Cui, P., Zhu, Y. Y., Han, Y. S., Chen, X. Q., and Zhuang, J. Q. (2009). The 12 May Wenchuan Earthquake-Induced Landslide Lakes: Distribution and Preliminary Risk Evaluation. Landslides 6, 209–223. doi:10.1007/s10346-009-0160-9
Dang, C., Cheng, Z. L., and Liu, J. J. (2009a). Conditions of Debris Flow Blocking the Main River. Mountain Res. 27, 557–563. (In Chinese). doi:10.3969/j.issn.1008-2786.2009.05.007
Dang, C., Cui, P., and Cheng, Z. L. (2009b). The Formation and Failure of Debris Flow-Dams, Background, Key Factors and Model Tests: Case Studies from China. Environ. Geol. 57, 1901–1910. doi:10.1007/s00254-008-1479-6
Dhungana, P., and Wang, F. (2019). The Relationship Among the Premonitory Factors of Landslide Dam Failure Caused by Seepage: an Experimental Study. Geoenviron Disasters 6. doi:10.1186/s40677-019-0135-7
Djalante, R. (2019). Key Assessments from the IPCC Special Report on Global Warming of 1.5 °C and the Implications for the Sendai Framework for Disaster Risk Reduction. Prog. Disaster Sci. 1, 100001. doi:10.1016/j.pdisas.2019.100001
Domènech, G., Fan, X., Scaringi, G., van Asch, T. W. J., Xu, Q., Huang, R., et al. (2019). Modelling the Role of Material Depletion, Grain Coarsening and Revegetation in Debris Flow Occurrences after the 2008 Wenchuan Earthquake. Eng. Geology. 250, 34–44. doi:10.1016/j.enggeo.2019.01.010
Du, C., Yao, L. K., Yang, Y. L., and Du, C. (2012). Criteria of Debris Flow Damming of Large River in Terms of Dynamical Process and Material Composition. J. Hydraulic Eng. 43, 128–132. (In Chinese). doi:10.13243/j.cnki.slxb.2012.s2.010
Du, W., Sheng, Q., Fu, X., Chen, J., and Zhou, Y. (2021). Extensions of the Two-phase Double-point Material point Method to Simulate the Landslide-Induced Surge Process. Eng. Anal. Boundary Elem. 133, 362–375. doi:10.1016/j.enganabound.2021.09.020
Evans, S. G., and Clague, J. J. (1994). Recent Climatic Change and Catastrophic Geomorphic Processes in Mountain Environments. Geomorphology 10, 107–128. doi:10.1016/0169-555X(94)90011-6
Fan, X., Yang, F., Siva Subramanian, S., Xu, Q., Feng, Z., Mavrouli, O., et al. (2020). Prediction of a Multi-hazard Chain by an Integrated Numerical Simulation Approach: the Baige Landslide, Jinsha River, China. Landslides 17, 147–164. doi:10.1007/s10346-019-01313-5
Froehlich, D. C., Froehlich, D. C., and Froehlich, D. (1995). Peak Outflow from Breached Embankment Dam. J. Water Resour. Plann. Manag. 121, 90–97. doi:10.1061/(ASCE)0733-9496(1995)121:1(90)
Fu, X., Sheng, Q., Li, G., Zhang, Z., Zhou, Y., and Du, Y. (2020). Analysis of Landslide Stability under Seismic Action and Subsequent Rainfall: a Case Study on the Ganjiazhai Giant Landslide along the Zhaotong-Qiaojia Road during the 2014 Ludian Earthquake, Yunnan, China. Bull. Eng. Geol. Environ. 79, 5229–5248. doi:10.1007/s10064-020-01890-z
Gregoretti, C., Maltauro, A., and Lanzoni, S. (2010). Laboratory Experiments on the Failure of Coarse Homogeneous Sediment Natural Dams on a Sloping Bed. J. Hydraul. Eng. 136, 868–879. doi:10.1061/(ASCE)HY.1943-7900.0000259
Guo, Z. X., Cao, S. Y., Liu, X. N., and Fang, D. (2004). Experimental Study on Parameters Affecting the River-Blocking Due to Debris Flow. J. Hydraulic Eng. 2004, 39–45. (In Chinese). doi:10.3321/j.issn:0559-9350.2004.11.007
Hakimzadeh, H., Nourani, V., and Amini, A. B. (2014). Genetic Programming Simulation of Dam Breach Hydrograph and Peak Outflow Discharge. J. Hydrol. Eng. 19, 757–768. doi:10.1061/(ASCE)HE.1943-5584.0000849
Han, M., Hu, T., Wang, Y., and Hong, M. L. (2016). Dynamics Character and River-Blocking Analysis of Narrowsteep Channels Debris Flowin WENchuan Earthquake Region—Illustrated with Case of Mozi Gully along Duwen Freeway. J. Eng. Geology. 24, 559–568. (In Chinese).
Haque, U., da Silva, P. F., Devoli, G., Pilz, J., Zhao, B., Khaloua, A., et al. (2019). The Human Cost of Global Warming: Deadly Landslides and Their Triggers (1995-2014). Sci. Total Environ. 682, 673–684. doi:10.1016/j.scitotenv.2019.03.415
He, Q. D. (2014). A Preliminary Study on the Disaster Chain Induced by the confluence of Large Debris Flow. Sichun: Southwest Petroleum University: Chengdu. (In Chinese).
He, Y. P. (2003). Influence of Debris Flow on River Bed Evolution in Mountainous Area. Beijing: University of Chinese Academy of Sciences. (In Chinese).
Herrmann, H., and Bucksch, H. (2014). “Moraine Dam,” in Dictionary Geotechnical Engineering/Wörterbuch GeoTechnik (Berlin, Heidelberg, Place: Springer), 889. doi:10.1007/978-3-642-41714-6_132713
Hu, K. H., Cui, P., Tian, M., and Yang, H. J. (2012). A Review of the Debris Flow Dynamic Models and Numerical Simulation. J. Hydraulic Eng. 43, 79–84. doi:10.3969/j.issn.0559-9350.2012.z2.015
Hu, K. H., Gangge, Y., Cui, P., Guo, X. J., and Yang, W. (2010). Preliminary Analysis of Extra-large-scale Debris Flow Disaster in Zhouqu County of Gansu Province. Mountain Res. 28, 628–634. (In Chinese). doi:10.3969/j.issn.1008-2786.2010.05.017
Huang, J. C. (2008). Numerical Modeling of Flow through Breach of Landslide Dams. J. Hydraulic Eng. 39, 1235–1240. (In Chinese).
Jiang, X., Wörman, A., Chen, P., Huang, Q., and Chen, H. (2020). Mechanism of the Progressive Failure of Non-cohesive Natural Dam Slopes. Geomorphology 363, 107198. doi:10.1016/j.geomorph.2020.107198
Kim, M.-I., and Kim, N. (2021). Analysis of Debris Flow Reduction Effect of Check Dam Types Considering the Mountain Stream Shape: A Case Study of 2016 Debris Flow Hazard in Ulleung-Do Island, South Korea. Adv. Civil Eng. 2021, 1–12. doi:10.1155/2021/8899368
Kim, N., Nakagawa, H., Kawaike, K., and Zhang, H. (2019). Estimation of Debris Flow Discharge Coefficient Considering Sediment Concentration. Int. J. Sediment Res. 34, 1–7. doi:10.1016/j.ijsrc.2018.05.003
Kirkpatrick, G. W. (1977). Evaluation Guidelines for Spillway Adequacy. American Society of Civil Engineers: Reston, 395–414.
Kumar, V., Gupta, V., Jamir, I., and Chattoraj, S. L. (2019). Evaluation of Potential Landslide Damming: Case Study of Urni Landslide, Kinnaur, Satluj valley, India. Geosci. Front. 10, 753–767. doi:10.1016/j.gsf.2018.05.004
Lai, P.-J., Chiu, C.-F., Hsu, S.-K., and Dong, J.-J. (2015). Longevity of Landslide Dams. Eng. Geology. Soc. Territory 2, 1105–1108. doi:10.1007/978-3-319-09057-3_195
Li, D.-y., Zheng, D.-f., Wu, H., Shen, Y.-q., and Nian, T.-k. (2021). Numerical Simulation on the Longitudinal Breach Process of Landslide Dams Using an Improved Coupled DEM-CFD Method. Front. Earth Sci. 9, 673249. doi:10.3389/feart.2021.673249
Li, M.-H., Sung, R.-T., Dong, J.-J., Lee, C.-T., and Chen, C.-C. (2011). The Formation and Breaching of a Short-Lived Landslide Dam at Hsiaolin Village, Taiwan - Part II: Simulation of Debris Flow with Landslide Dam Breach. Eng. Geology. 123, 60–71. doi:10.1016/j.enggeo.2011.05.002
Li, Y., Liu, J., Su, F., Xie, J., and Wang, B. (2015). Relationship between Grain Composition and Debris Flow Characteristics: a Case Study of the Jiangjia Gully in China. Landslides 12, 19–28. doi:10.1007/s10346-014-0475-z
Liao, M., Huang, R. Q., Gan, J. J., Zhong, W., and Li, Q. Y. (2012). Study of Formation Mechanism and Blocking in Giant Debris Flow at Hongchun Gully Yingxiu Town Wenchuan County. Amr 610-613, 3267–3276. doi:10.4028/www.scientific.net/AMR.610-613.3267
Liu Cr, C. R., Yao, L. K., Chen, C. G., and Du, C. (2014). A Distinguishing Method for the River Blocking from Submerged Dam Based on the Theory of Gravity Current. J. Nat. Disasters 23, 113–120. (In Chinese). doi:10.1088/1674-1056/23/7/077308
Liu Cr, C. R., Yao, L. K., Du, C., and Yang, Q. H. (2013). Experimental Research on Criteria and Disaster-Forming Patterns of Damming Large River by Debris Flow in Earthquake Disaster Areas. China Civil Eng. J. 46, 146–152. (In Chinese). doi:10.15951/j.tmgcxb.2013.s1.008
Liu, C. R., and Yao, L. K. (2012). Study on Features of River-Blocked by Debris Flow and Criterion of Disaster at the Opposite River Bank. J. Sichuan University(Engineering Sci. Edition) 44, 93–100. (In Chinese).
Liu, C. R., and Zhao, S. G. (2016). Experimental Study on Mechanism of Large River Blocking by Debris Flow. J. Chongqing Jiaotong University(Natural Science) 35, 90–95. (In Chinese).
Liu Cz, C. Z., Lü, J. T., Tong, L. Q., Chen, H. Q., Liu, Q. Q., Xiao, R. H., et al. (2019). Research on Glacial/rock Fall-Landslide-Debris Flows in Sedongpu basin along Yarlung Zangbo River in Tibet. Geology. China 46, 219–234. (In Chinese). doi:10.12029/gc20190201
Liu Hd, H. D., Li, X. C., Liu, S., and Zhu, H. (2013). Zhouqu Catastrophic Mudslide Barrier Dam Stability Analysis. Yellow River 35, 116–119. (In Chinese).
Liu, J. J, Tang, C., and Cheng, Z.L. (2013). The Two Main Mechanisms of Glacier lake Outburst Flood in Tibet, China. J. Mt. Sci. 10, 239–248. doi:10.1007/s11629-013-2517-8
Liu, J. F., You, Y., Chen, X. Q., Liu, J. K., and Chen, X. Z. (2014). Characteristics and hazard Prediction of Large-Scale Debris Flow of Xiaojia Gully in Yingxiu Town, Sichuan Province, China. Eng. Geology. 180, 55–67. doi:10.1016/j.enggeo.2014.03.017
Liu, J. K., Zhang, J. J., Gao, B., Li, Y. L., Li, M. Y., Wu Jin, D. J., et al. (2019). An Overview of Glacial lake Outburst Flood in Tibet,China. J. Glaciology Geocryology 41, 1335–1347. (In Chinese).
Liu, J., You, Y., Chen, X., and Chen, X. (2015). Mitigation Planning Based on the Prediction of River Blocking by a Typical Large-Scale Debris Flow in the Wenchuan Earthquake Area. Landslides 13, 1231–1242. doi:10.1007/s10346-015-0615-0
Liu, S., Wei, L., and Hu, K. (2020). Topographical and Geological Variation of Effective Rainfall for Debris-Flow Occurrence from a Large-Scale Perspective. Geomorphology 358, 107134. doi:10.1016/j.geomorph.2020.107134
Liu, W. R., and Zhou, Z. Y. (2015). Discussion on the Types of River Blocking by Debris Flow in Wenchuan Earthquake Area. Pearl River 36, 113–116. (In Chinese).
Lv, L. Q., Wang, Z. Y., Xu, M. Z., Qi, L. J., and Zhang, C. D. (2016). Geomorphic Characters of Debris Flow Fans along Nu River and the River Blocking Mechanisms. J. Hydraulic Eng. 47, 1245–1252.
Macdonald, T. C., and Langridge‐Monopolis, J. (1984). Breaching Charateristics of Dam Failures. J. Hydraulic Eng. 110, 567–586. doi:10.1061/(asce)0733-9429(1984)110:5(567)
Medwedeff, W. G., Clark, M. K., Zekkos, D., and West, A. J. (2020). Characteristic Landslide Distributions: An Investigation of Landscape Controls on Landslide Size. Earth Planet. Sci. Lett. 539, 116203. doi:10.1016/j.epsl.2020.116203
Neupane, R., Chen, H., and Cao, C. (2019). Review of Moraine Dam Failure Mechanism. Geomatics, Nat. Hazards Risk 10, 1948–1966. doi:10.1080/19475705.2019.1652210
Osti, R., Bhattarai, T. N., and Miyake, K. (2011). Causes of Catastrophic Failure of Tam Pokhari Moraine Dam in the Mt. Everest Region. Nat. Hazards 58, 1209–1223. doi:10.1007/s11069-011-9723-x
Pan, H., Wang, R., Huang, J., and Ou, G. (2013). Study on the Ultimate Depth of Scour Pit Downstream of Debris Flow Sabo Dam Based on the Energy Method. Eng. Geology. 160, 103–109. doi:10.1016/j.enggeo.2013.03.026
Peng, M., and Zhang, L. M. (2012). Breaching Parameters of Landslide Dams. Landslides 9, 13–31. doi:10.1007/s10346-011-0271-y
Pierce, M. W., Thornton, C. I., and Abt, S. R. (2010). Predicting Peak Outflow from Breached Embankment Dams. J. Hydrol. Eng. 15, 338–349. doi:10.1061/(ASCE)HE.1943-5584.0000197
Prochaska, A. B., Santi, P. M., Higgins, J. D., and Cannon, S. H. (2008). A Study of Methods to Estimate Debris Flow Velocity. Landslides 5, 431–444. doi:10.1007/s10346-008-0137-0
Ruan, H., Chen, H., Wang, T., Chen, J., and Li, H. (2021). Modeling Flood Peak Discharge Caused by Overtopping Failure of a Landslide Dam. Water 13, 921. doi:10.3390/w13070921
Sattar, A., Goswami, A., and Kulkarni, A. V. (2019). Hydrodynamic Moraine-Breach Modeling and Outburst Flood Routing - A hazard Assessment of the South Lhonak lake, Sikkim. Sci. Total Environ. 668, 362–378. doi:10.1016/j.scitotenv.2019.02.388
Shen D, D., Shi, Z., Peng, M., Zhang, L., and Jiang, M. (2020). Longevity Analysis of Landslide Dams. Landslides 17, 1797–1821. doi:10.1007/s10346-020-01386-7
Shen P, P., Zhang, L., Wong, H. F., Peng, D., Zhou, S., Zhang, S., et al. (2020). Debris Flow Enlargement from Entrainment: A Case Study for Comparison of Three Entrainment Models. Eng. Geology. 270, 105581. doi:10.1016/j.enggeo.2020.105581
Shrestha, B. B., and Nakagawa, H. (2016). Hazard Assessment of the Formation and Failure of the Sunkoshi Landslide Dam in Nepal. Nat. Hazards 82, 2029–2049. doi:10.1007/s11069-016-2283-3
Shulmeister, J., Davies, T. R., Evans, D. J. A., Hyatt, O. M., and Tovar, D. S. D. (2009). Catastrophic Landslides, Glacier Behaviour and Moraine Formation - A View from an Active Plate Margin. Quat. Sci. Rev. 28, 1085–1096. doi:10.1016/j.quascirev.2008.11.015
Singh, K. P., and Snorrason, A. (1984). Sensitivity of Outflow Peaks and Flood Stages to the Selection of Dam Breach Parameters and Simulation Models. J. Hydrol. 68, 295–310. doi:10.1016/0022-1694(84)90217-8
Song, Z. (2015). Progress in the Research of the Critical Conditions of River Blocking by Debris Flows. Sediment. Geology. Tethyan Geology. 35, 109–112. (In Chinese). doi:10.3969/j.issn.1009-3850.2015.03.016
Stancanelli, L. M., Lanzoni, S., and Foti, E. (2014). Mutual Interference of Two Debris Flow Deposits Delivered in a Downstream River Reach. J. Mt. Sci. 11, 1385–1395. doi:10.1007/s11629-014-3051-z
Stancanelli, L. M., Lanzoni, S., and Foti, E. (2015). Propagation and Deposition of Stony Debris Flows at Channel Confluences. Water Resour. Res. 51, 5100–5116. doi:10.1002/2015WR017116
Szczukiewicz, S., Magnini, M., and Thome, J. R. (2014). Proposed Models, Ongoing Experiments, and Latest Numerical Simulations of Microchannel Two-phase Flow Boiling. Int. J. Multiphase Flow 59, 84–101. doi:10.1016/j.ijmultiphaseflow.2013.10.014
Tacconi Stefanelli, C., Casagli, N., and Catani, F. (2020). Landslide Damming hazard Susceptibility Maps: a New GIS-Based Procedure for Risk Management. Landslides 17, 1635–1648. doi:10.1007/s10346-020-01395-6
Tang, C., Huang, R. Q., Huang, D., and Zhang, W. F. (2006). Impacts of Debris Flows on the Reservoir of a Hydropower Station in the Meigu River of Jinshajiang. J. Eng. Geology. 2006, 145–151. (In Chinese).
Tang, C., Rengers, N., Van Asch, T. W. J., Yang, Y. H., and Wang, G. F. (2011). Triggering Conditions and Depositional Characteristics of a Disastrous Debris Flow Event in Zhouqu City, Gansu Province, Northwestern China. Nat. Hazards Earth Syst. Sci. 11, 2903–2912. doi:10.5194/nhess-11-2903-2011
Walder, J. S., and O'Connor, J. E. (1997). Methods for Predicting Peak Discharge of Floods Caused by Failure of Natural and Constructed Earthen Dams. Water Resour. Res. 33, 2337–2348. doi:10.1029/97WR01616
Wang, F., Wang, J., Chen, X., and Chen, J. (2021). Experimental Study on Debris-Flow Velocity Control Mechanism with Baffles in a Drainage Channel. Bull. Eng. Geol. Environ. 80, 5203–5217. doi:10.1007/s10064-020-02086-1
Wang, L., Chang, M., Dou, X., Ma, G., and Yang, C. (2017). Analysis of River Blocking Induced by a Debris Flow. Geofluids 2017, 1–8. doi:10.1155/2017/1268135
Wang, Y., Tan, R., Jan, C., and Tian, B. (2009). Gravel Accumulation in Deposits of Viscous Debris Flows with Hyper-Concentration. J. Mt. Sci. 6, 88–95. doi:10.1007/s11629-009-0120-9
Webby, M. G. (1996). Discussion of “Peak Outflow from Breached Embankment Dam” by David C. Froehlich. J. Water Resour. Plann. Manag. 122, 316–317. doi:10.1061/(asce)0733-9496(1996)122:4(316)
Wei, F. Q., Hu, K. H., Cui, P., Chen, J., and He, Y. P. (2002). Characteristics and Origing of Debris Flow of Jiangjiagou Valley Blocking. J. Soil Water conservation 4, 71–75. (In Chinese). doi:10.13870/j.cnki.stbcxb.2002.06.021
Wu, C., Hu, K., Liu, W., Wang, H., Hu, X., and Zhang, X. (2020). Morpho-sedimentary and Stratigraphic Characteristics of the 2000 Yigong River Landslide Dam Outburst Flood Deposits, Eastern Tibetan Plateau. Geomorphology 367, 107293. doi:10.1016/j.geomorph.2020.107293
Wu, J. S., Cheng, Z. L., and Geng, X. Y. (2005). Formation of Dam from Debris Flow in the Southeast Tibet. Mountain Res. 2, 4399–4405. (In Chinese). doi:10.3969/j.issn.1008-2786.2005.04.003
Xu, L., Meng, X., and Xu, X. (2014). Natural hazard Chain Research in China: A Review. Nat. Hazards 70, 1631–1659. doi:10.1007/s11069-013-0881-x
Xu, M., Wang, Z., Qi, L., Liu, L., and Zhang, K. (2012). Disaster Chains Initiated by the Wenchuan Earthquake. Environ. Earth Sci. 65, 975–985. doi:10.1007/s12665-011-0905-3
Xu, X. J., Hu, X. W., Xu, Z. P., and Han, Y. F. (2019). Incipient Shiwalong Gully, Motion Condition and River-Blocking Possibility of Debris Flow, Rangtang County, Sichuan Province. Chin. J. Geol. Hazard Control. 30, 44–53. (In Chinese).
Xu, Y. N., Kuang, S. F., Huang, Y. J., and Wang, L. (2002). The Risk index of Debris Flow into the confluence. J. Nat. Disasters 2002, 33–38. (In Chinese).
Yan, Z. W., Wei, Y. Q., and Cai, H. (2009). Formation Mechanism and Stability Analysis of Barrier Dam. Chin. J. Geol. Hazard Control. 20, 55–59. (In Chinese). doi:10.3969/j.issn.1003-8035.2009.04.012
Yang, Y., Sun, G., Zheng, H., and Qi, Y. (2019). Investigation of the Sequential Excavation of a Soil-Rock-Mixture Slope Using the Numerical Manifold Method. Eng. Geology. 256, 93–109. doi:10.1016/j.enggeo.2019.05.005
Yang, Y., Xu, D., Liu, F., and Zheng, H. (2020). Modeling the Entire Progressive Failure Process of Rock Slopes Using a Strength-Based Criterion. Comput. Geotechnics 126, 103726. doi:10.1016/j.compgeo.2020.103726
Yin, Y., Wang, F., and Sun, P. (2009). Landslide Hazards Triggered by the 2008 Wenchuan Earthquake, Sichuan, China. Landslides 6, 139–152. doi:10.1007/s10346-009-0148-5
Yong, L., Chengmin, H., Baoliang, W., Xiafei, T., and Jingjing, L. (2017). A Unified Expression for Grain Size Distribution of Soils. Geoderma 288, 105–119. doi:10.1016/j.geoderma.2016.11.011
Yong, L., Xiaojun, Z., Pengcheng, S., Yingde, K., and Jingjing, L. (2013). A Scaling Distribution for Grain Composition of Debris Flow. Geomorphology 192, 30–42. doi:10.1016/j.geomorph.2013.03.015
Zhang, B. W., and Huang, J. C. (2007). Study on Gradual Breach Mode of Overtopping of Embankment Dam. China Flood Drought Manag. 2007, 28–31. (In Chinese).
Zhang, J. S., Shen, X. J., and Xie, H. (2007). Study on Factors Affecting the River-Blocking Due to Debirs-Flow in the Upper Reaches of Minjiang River. J. catastrophology 2007, 82–86. (In Chinese). doi:10.3969/j.issn.1000-811X.2007.02.019
Zhang, J. S., and Xie, H. (2008). Empirical Formula Discrimination of the Possibility of River Blocking by Debris Flow in the Upper Reaches of Minjiang River. Resour. Environ. Yangtze Basin 2008, 651–655. (In Chinese).
Zhang, L., Peng, M., Chang, D., and Xu, Y. (2016). Dam Failure Mechanisms and Risk Assessment. John Wiley & Sons: Singapore, 307–321. doi:10.1002/9781118558522.ch12
Zhang, L., Xiao, T., He, J., and Chen, C. (2019). Erosion-based Analysis of Breaching of Baige Landslide Dams on the Jinsha River, China, in 2018. Landslides 16, 1965–1979. doi:10.1007/s10346-019-01247-y
Zhang, S. J., Xu, C. X., Wei, F. Q., Hu, K. H., Xu, H., Zhao, L. Q., et al. (2020). A Physics-Based Model to Derive Rainfall Intensity-Duration Threshold for Debris Flow. Geomorphology 351, 106930. doi:10.1016/j.geomorph.2019.106930
Zhao, X., Gao, Q., and Tang, X. J. (2009). Simulation Analysis on Broken Dam of Debris Flow in Kezi River of Xinjiang. J. Zhejiang Water Conservancy Hydropower Coll. 23, 24–27. (In Chinese).
Zheng, H., Shi, Z., Shen, D., Peng, M., Hanley, K. J., Ma, C., et al. (2021). Recent Advances in Stability and Failure Mechanisms of Landslide Dams. Front. Earth Sci. 9, 659935. doi:10.3389/feart.2021.659935
Zheng, H., Yang, Y., and Shi, G. (2019). Reformulation of Dynamic Crack Propagation Using the Numerical Manifold Method. Eng. Anal. Boundary Elem. 105, 279–295. doi:10.1016/j.enganabound.2019.04.023
Zhong, D. L., Xie, H., Wei, F. Q., Liu, H. J., and Tang, J. F. (2013). On Mountain Disaster Chain. J. Mountain Sci. 31, 314–326. (In Chinese). doi:10.1200/jco.2013.50.4639
Zhong, Q. M., Chen, S. S., Mei, S. A., and Cao, W. (2017). Numerical Simulation of Landslide Dam Breaching Due to Overtopping. Landslides 15, 1183–1192. doi:10.1007/s10346-017-0935-3
Zhou Ggd, G. G. D., Zhou, M., Shrestha, M. S., Song, D., Choi, C. E., Cui, K. F. E., et al. (2019). Experimental Investigation on the Longitudinal Evolution of Landslide Dam Breaching and Outburst Floods. Geomorphology 334, 29–43. doi:10.1016/j.geomorph.2019.02.035
Zhou W, W., Fang, J., Tang, C., and Yang, G. (2019). Empirical Relationships for the Estimation of Debris Flow Runout Distances on Depositional Fans in the Wenchuan Earthquake Zone. J. Hydrol. 577, 123932. doi:10.1016/j.jhydrol.2019.123932
Zhou, Y., Sheng, Q., Li, N., and Fu, X. (2022). The Dynamic Mechanical Properties of a Hard Rock under True Triaxial Damage-Controlled Dynamic Cyclic Loading with Different Loading Rates: a Case Study. Rock Mech. Rock Eng. 2022, 214. doi:10.1007/s00603-021-02756-w
Zhu, D. P., Qin, L. K., and He, Q. D. (2016). Effect of Debris Flow Converging into River on the Basis of Physical Simulation Experiments. Chin. J. Rock Mech. Eng. 35, 3680–3688. (In Chinese).
Zhu, P. Y., Cheng, Z. L., and You, Y. (2000). Researeh on Causes of River Blocking by Sediment Delivery of Peilong Gully Debris Flow in the Sichuan-Xizang Highway. J. Nat. Disasters 2000, 80–83. (In Chinese).
Zhuang, J. Q., Ge, Y. G., and Chen, X. (2012). Assessment of River Block Probability by Debris Flow along Dujiangyan-Wenchun Highway after Wenchun Earthquake. J. Eng. Geology. 20, 195–203. (In Chinese) CNKI:SUN:GCDZ.0.2012-02-008.
Zou, Q., Cui, P., Jiang, H., Wang, J., Li, C., and Zhou, B. (2020). Analysis of Regional River Blocking by Debris Flows in Response to Climate Change. Sci. Total Environ. 741, 140262. doi:10.1016/j.scitotenv.2020.140262
Keywords: debris flow dam, river-blocking mechanism, dam failure, hazard chain, floods
Citation: Chen H, Ruan H, Chen J, Li X and Yu Y (2022) Review of Investigations on Hazard Chains Triggered by River-Blocking Debris Flows and Dam-Break Floods. Front. Earth Sci. 10:830044. doi: 10.3389/feart.2022.830044
Received: 06 December 2021; Accepted: 25 March 2022;
Published: 10 May 2022.
Edited by:
Yongtao Yang, Institute of Rock and Soil Mechanics (CAS), ChinaReviewed by:
Xuewei Liu, Institute of Rock and Soil Mechanics (CAS), ChinaXiaodong Fu, Institute of Rock and Soil Mechanics (CAS), China
Copyright © 2022 Chen, Ruan, Chen, Li and Yu. This is an open-access article distributed under the terms of the Creative Commons Attribution License (CC BY). The use, distribution or reproduction in other forums is permitted, provided the original author(s) and the copyright owner(s) are credited and that the original publication in this journal is cited, in accordance with accepted academic practice. No use, distribution or reproduction is permitted which does not comply with these terms.
*Correspondence: Jiangang Chen, chenjg@imde.ac.cn