- Department of Palynology and Climate Dynamics, Albrecht-von-Haller-Institute, University of Goettingen, Goettingen, Germany
In palaeoecology, multi-site macro-charcoal analyses provide information on climate–fire–vegetation linkages, their spatial and temporal extent, and the impact of prehistoric human practices. Our multi-site study comprises eight macro-charcoal records from two highly continental forest-steppe regions in western and north-central Mongolia, covering the Holocene. In addition to reviewing macro-charcoal influxes and comparing macro-charcoal morphotype results with arboreal/non-arboreal pollen ratios, our dataset provides the first fire frequency synthesis for two regions in Mongolia. During the early and mid-Holocene, the fire history in western and north-central Mongolia was controlled by the regional climate variability, whereas the fire intensities were higher in the Mongolian Altai. In general, fire frequencies are lower in northern Khangai. Increases in the fire frequency correlate with the potential beginning of a rise in the nomadic population of the Mongolian Altai after 1,100 cal yr BP. In both areas, individual macro-charcoal peaks, a marked site-to-site variability, and a generally very low impact on the forest-steppe distribution may mostly be explained by a strictly local nomadic disturbance.
1 Introduction
Mongolian forest-steppe ecosystems are particularly sensitive to natural and anthropogenic changes, mainly damage by fires, (over) grazing, and climate change. Especially, fires can cause serious damage to nature. In the specific case of one of our study areas in the northern Khangai Mountains, reconstructions of potential forest distribution suggest that 43% of the forested area was destroyed by fire over a comparatively short period from 1986 to 2017 (Klinge et al., 2021). Hence, an investigation of palaeofires, especially their driving factors and impact on the environment, may give a valuable perspective for future management and protection strategies of the species-rich ecosystem.
Charcoal accumulates during and following a fire event in natural and sedimentological archives. It is resistant to microbial activity and oxidation, making it an abundant resource persistent on a long timescale (Patterson et al., 1987; Scott, 2010). Depending on particle size, charcoal in sediments may even be recognized and interpreted by its characteristic morphological shape (wood, leaf, grass, and moss) (Umbanhowar and McGrath, 1998; Scott, 2010; Mustaphi and Pisaric, 2014). Charcoal can originate from both natural and human-induced fires; therefore, the interpretation of fire history from sedimentological archives depends upon understanding the processes controlling its production, dispersal, and accumulation (Patterson et al., 1987; Whitlock and Larsen, 2001; Higuera et al., 2009).
In recent years, the fire history of Mongolia and its adjacent regions has only been discussed sparsely. In the Mongolian Altai, several charcoal records have been investigated by Unkelbach et al. (2018), Unkelbach et al. (2019), Unkelbach et al. (2020), Unkelbach et al. (2021a), and Bruegger et al. (2018). There are also records from the Russian Altai (Eichler et al., 2011), the Chinese Altai (Li et al., 2019), and the northern Khangai (Unkelbach et al., 2021b). However, all records only present the fire history on a small scale. Umbanhowar et al. (2009) reconstructed modern and historical fires from a variety of lakes in grasslands in the Valley of Lakes. Their data suggest that fire has virtually been absent from the steppes in Northwestern Mongolia, whereas preliminary macro-charcoal results from the forest-steppes in the Altai Mountains and the northern Khangai Mountains show a higher fire activity over the mid-Holocene and late Holocene (Unkelbach et al., 2021a; 2021b). Merging the results of several charcoal records from an area allows a more comprehensive insight into the regional patterns and local differences in charcoal processes and fire impacts on the environment.
The aim of this study was to gain a holistic view on fire activity and macro-charcoal accumulation in the forest-steppe ecosystem. We combined the evidence of eight macro-charcoal records from two different forest-steppe regions in western and north-central Mongolia. All sediment cores were processed using the same methodology to ensure good comparability. In addition to reviewing macro-charcoal influxes and comparing macro-charcoal morphotype results with arboreal/non-arboreal pollen (AP/NAP) ratios, we also applied CharAnalysis software to calculate fire frequencies. The aim was to assess spatial and temporal patterns of charcoal deposition in different environments (steppe, forest, and high/low elevations), in different archives (lake and soil hollows), the impact of regional (climate) or local (landscape and humans) factors, and whether fires had a significant impact on the vegetation composition in both regions.
2 Study area
The first study area (A) is located in the Altai Tavan Bogd National Park (NP), Altai Mountains, in Northwestern Mongolia (Figure 1). The NP, established in 1996, is situated between 2,000 and 4,000 m a.s.l. (Lkhagvadorj et al., 2013). In most areas, traditional land use, e.g., animal husbandry and grazing by nomadic pastoralists and hunting are tolerated (Beket and Knapp, 2012).
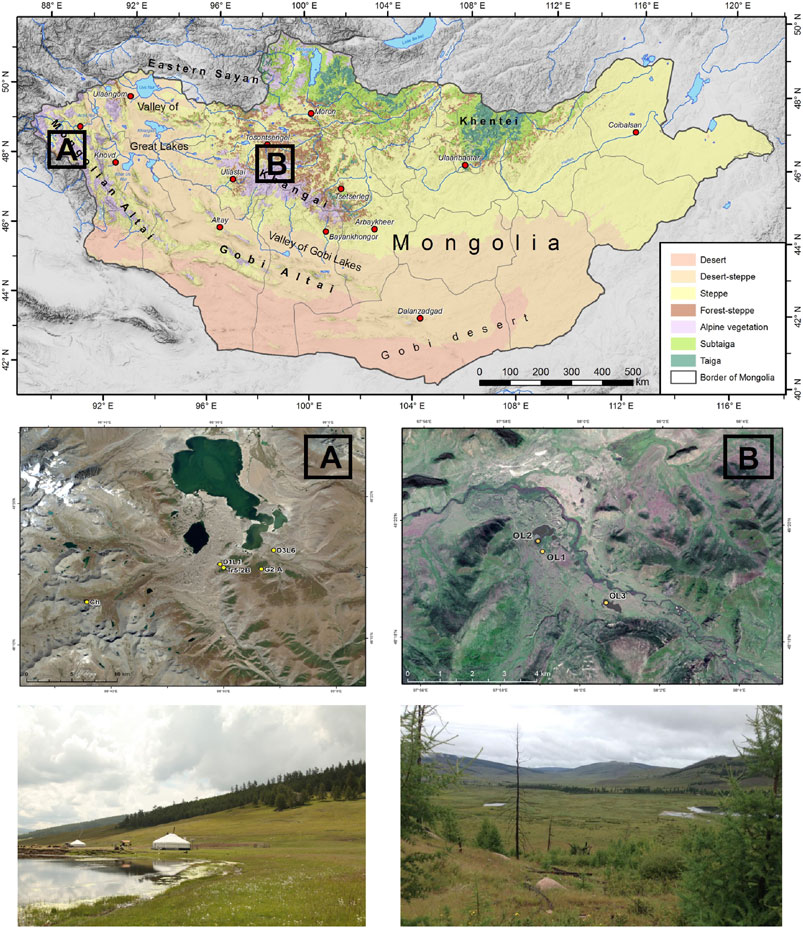
FIGURE 1. Overview map of Mongolia with locations of study areas Altai Tavan Bogd National Park (A) and Tarvagatai Nuruu National Park (B) (modified from Klinge et al., 2021), locations of studied sites within both study areas and photos of the landscape were taken by H. Behling in 2016 and 2018..
The second study area (B) is located in the Travagatai Nuruu NP, northern Khangai Mountains, in north-central Mongolia. The NP was established in 2000, and its summits reach up to 3,100–3,200 m a.s.l. (Ariuntsetseg et al., 2008). Infrastructure for nomadic herders is limited in this location. Thus, grazing occurs very sparsely nowadays.
The climate in both areas is highly continental due to their situation within Central Asia and their alpine conditions. Mean annual temperatures are −0.9°C (Ulgii, Mongolian Altai) and −5.9°C (Tosontsengel, northern Khangai), and the mean annual precipitation ranges from 150 to 500 mm, reaching its maximum in the short summer period (Schneider et al., 2021; AM Online Projects, 2022).
Located in the Central Asian forest-steppe zone, both areas show an altitudinal vegetation zonation controlled by elevation, precipitation, and solar radiation. Valleys below 2,200 m a.s.l. present grassland vegetation of sedges, grasses, forbs, and shrub communities, followed by dry steppe consisting of grasses and sedges, and above 2,800 m a.s.l. alpine tundra is dominant. Tree growth is limited to north-facing mountain slopes as a consequence of reduced evapotranspiration. Nowadays, forests and forest-steppes mainly consist of Siberian larch (Larix sibirica) and are usually present on the upper or central parts of the slopes (Hilbig, 1995; Gunin et al., 1999; Dulamsuren et al., 2014). In Tarvagatai Nuruu NP, around 80,000 ha of forest-steppe vegetation was destroyed by a severe fire in 2002 (Ariuntsetseg et al., 2008).
3 Materials and methods
For this study, we used eight sediment cores; five were sampled in Altai Tavan Bogd NP (A) in 2014 (Unkelbach et al., 2018; Unkelbach et al., 2021a) and 2016 (Unkelbach et al., 2019; Unkelbach et al., 2020), and three profiles were sampled in Tarvagatai Nuruu NP (B) in 2018 (Unkelbach et al., 2021b; this study). Profile locations and environmental information are given in Table 1 and Figure 2. Additional information on age-depth-models, pollen- and other multi-proxy data are available in the literature mentioned earlier.
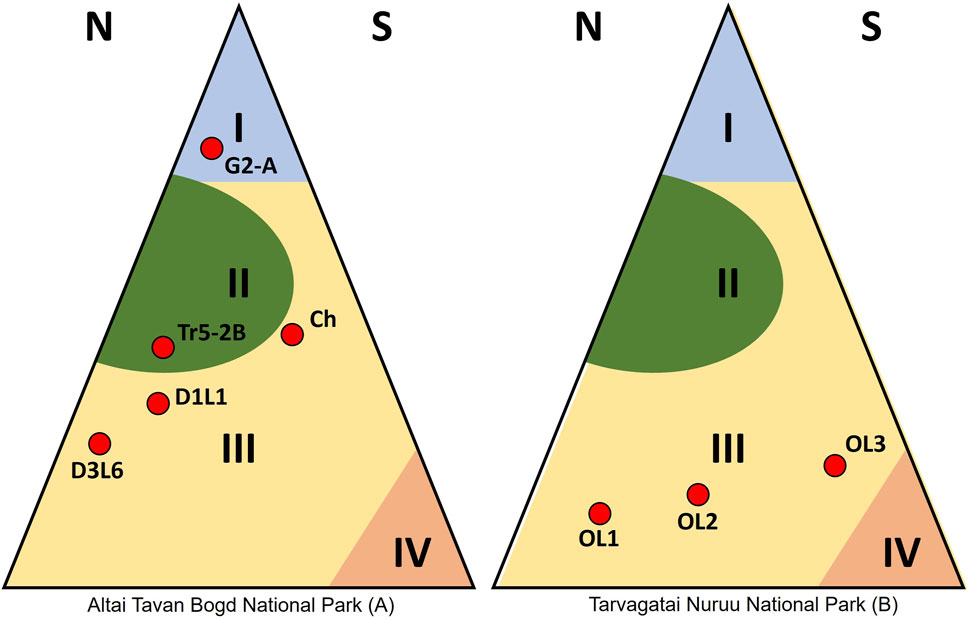
FIGURE 2. Locations of cores within vegetation zones, I: High-mountain zone (>2,100 m a.s.l.), II: Mountain forest zone (1,700–2,200 m a.s.l.), III: Mountain steppe zone (1,600–3,200 m a.s.l.), and IV: Desert zone (1,100–2,100 m a.s.l.); zonation after Beket and Knapp (2012).
For macro-charcoal analysis, all eight cores were sampled seamlessly in consistent intervals. Samples of 0.5 cm3 were processed according to the method established by Stevenson and Haberle (2005), and morphological types were identified and counted as described in Unkelbach et al. (2019). For each sample, influx values were calculated. Additionally, fire episodes were analyzed using CharAnalysis software (Higuera et al., 2009). The software separates background charcoal (CHARbackground) from charcoal peaks (CHARpeak). For background charcoal determination, a Lowess smoother robust to outliers over a 200-year smoothing interval was used. One CHARpeak visualizes an episode of one or more major local fire events. The CHARpeak magnitude hinges on the size and severity of the local fire (Whitlock et al., 2006; Ali et al., 2012; Xiao et al., 2017). For calculating the fire frequency, the time series of fire return intervals (FRI; time between CHARpeaks) was interpolated to annual resolution and again smoothed with the Lowess filter over 200 years (Higuera, 2009). Illustration of data was performed using TILIA and TILIAGRAPH software (Grimm, 1991).
4 Results
4.1 Mongolian Altai
4.1.1 Regional macro-charcoal influx over the last 4,310 cal yr BP
In the Altai Tavan Bogd NP, macro-charcoal influx varied widely over the last 4,310 cal yr BP (Figure 3). Average macro-charcoal influx ranges from 0.8 particles/cm2yr at site D3L6, 2.5 particles/cm2yr at site G2-A, 4.9 particles/cm2yr at site D1L1 and 9.7 particles/cm2yr at site Ch to 33.1 particles/cm2yr at site Tr5-2B. Average influxes vary with their surrounding vegetation (steppe or forest) but are not markedly correlated with other environmental factors such as elevation, latitude/longitude, or type of archive (lake or soil).
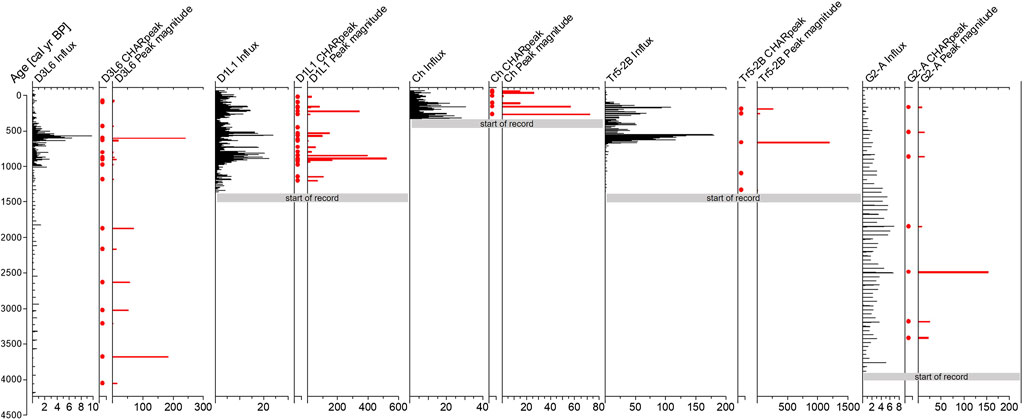
FIGURE 3. Altai Tavan Bogd NP macro-charcoal influxes (particles/cm2 yr), peaks, and respective peak magnitudes (particles/cm2 peak) based on CharAnalysis.
CharAnalysis software suggests several CHARpeaks with varying peak magnitudes for each site. More than twice as many CHARpeaks are reconstructed in the lake archives, whereas the average peak magnitude neither correlates with the type of archive nor with their geographic location. Average peak magnitudes are considerably higher at the forest site (Tr5-2B), with a maximum of 1,900 particles/cm2peak at around 600 cal yr BP.
Density and temporal resolution of samples impede the examination of fire synchronicity. Despite the remarkable variability among the CHARpeaks of the macro-charcoal records, over the last 1,100 years, there have been three fire events present simultaneously in three or more of the records. This applies to the fire events at around 200 cal yr BP, 600 cal yr BP, and 900 cal yr BP. Other connections between fire events among the sites are possible but not clearly reflected in the macro-charcoal data.
4.1.2 Fire frequency
In the Altai Tavan Bogd NP, the fire frequencies of the five sites (Figure 4A) differ in accordance with their varying peak magnitudes. A relative consistency between sites can only be observed from around 3,300 to 3,000 cal yr BP (sites D3L6 and G2-A), from around 1,100 to 400 cal yr BP (sites D3L6, D1L1, Tr5-2B, and G2-A) and over the last 100 years (D1L1, Ch, and Tr5-2B). At other times, reconstructed fire frequencies are more contradictory, especially at site Tr5-2B. Overall, the fire frequency at site D1L1 shows a higher range than at the four other sites.
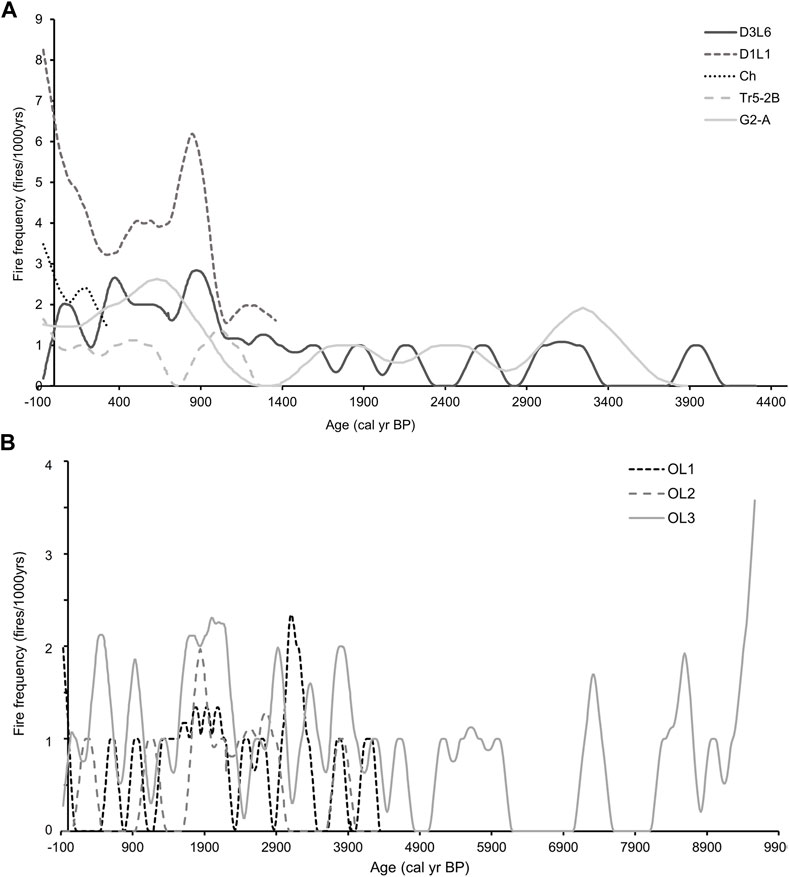
FIGURE 4. Fire frequency (number of fires per 200 years) for (A) Altai Tavan Bogd NP and (B) Tarvagatai Nuruu NP records based on CharAnalysis.
4.1.3 Site-specific macro-charcoal morphotype accumulation in the Mongolian Altai
The macro-charcoal compositions of three archives from the Altai Tavan Bogd NP (Figure 5; Table 2) are dominated by charred wood material. The wood macro-charcoal fraction is abundant at sites D1L1 (26.1%), Ch (36.2%), and Tr5-2B (20.5%). At sites D3L6 (24.2%) and G2-A (26.1%), the grass particle fraction is abundant.
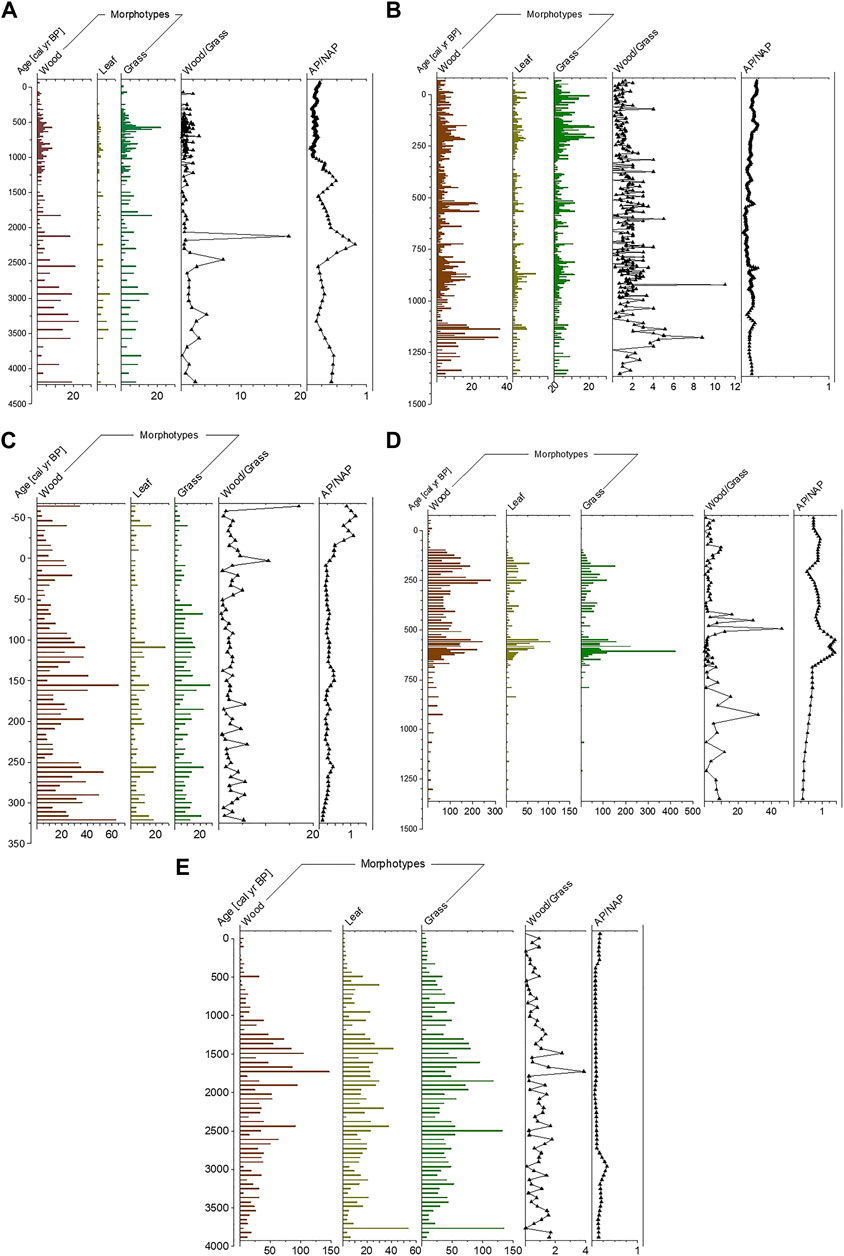
FIGURE 5. Macro-charcoal morphotypes (total counts per sample), wood/grass morphotype ratios, and AP/NAP ratios: (A) Site D3L6; charcoal and pollen data from Unkelbach et al. (2019), (B) site D1L1; charcoal and pollen data from Unkelbach et al. (2020), (C) site Ch; charcoal and pollen data from Unkelbach et al. (2021a), (D) site Tr5-2B; charcoal and pollen data from Unkelbach et al. (2021a), and (E) site G2-A; charcoal and pollen data from Unkelbach et al. (2018).
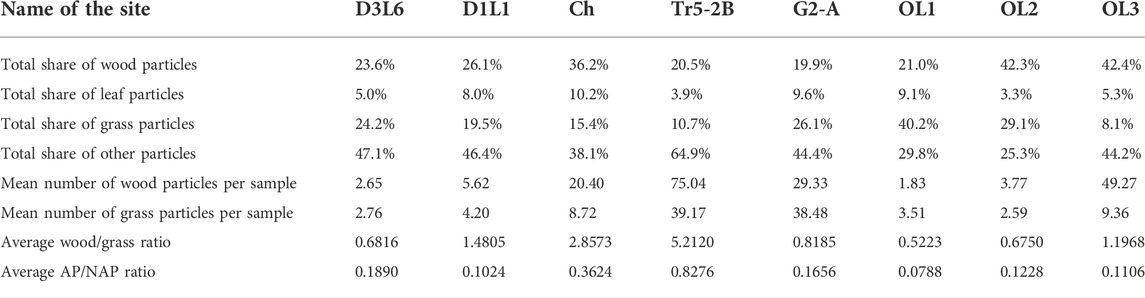
TABLE 2. Statistics for macro-charcoal morphotype results at the Altai Tavan Bogd NP (D3L6-G2A) and Tarvagatai Nuruu NP (OL1–OL3).
Macro-charcoal records from D1L1, Ch, and Tr5-2B all showed higher wood/grass ratios, whereas the highest average value (5.2120) is present at site Tr5-2B from within the forest. Wood/grass ratios at site D3L6 (0.6816) and at site G2-A (0.8185) are comparably low. At site D3L6 (Figure 5A), the wood/grass ratio shows some major changes, especially from 4,310 to around 2,000 cal yr BP. The ratio suggests three peaks of dominating wood macro-charcoal deposition from 3,750 to 3,250 cal yr BP, at around 2,500 cal yr BP and at around 2,100 cal yr BP. The wood/grass ratio at site D1L1 (Figure 5B) displays several peaks over the whole record. Two distinct peaks are illustrated from 1,250 to 1,100 cal yr BP and at around 920 cal yr BP. Overall, at site Ch (Figure 5C), the wood/grass ratio is rather stable, only with two maxima in recent times. These maxima are suggested for around 0 cal yr BP (=1950 AD) and the topmost sample from the year of coring. The wood/grass ratio at site Tr5-2B (Figure 5D) is also characterized by several major changes. The most distinct peaks are suggested for around 920 cal yr BP and an episode from 520 to 420 cal yr BP. At site G2-A (Figure 5E), the wood/grass ratio is more stable, varying mostly between 0 and 2. One peak episode is depicted from 1720 to 1,490 cal yr BP.
4.2 Northern Khangai
4.2.1 Regional macro-charcoal influx over the last 9,550 cal yr BP
Macro-charcoal influx is comparably low in the Tarvagatai Nuruu NP (Figure 6). In this area, macro-charcoal influx averages range from 0.6 particles/cm2yr at site OL1 and 0.7 particles/cm2yr at site OL2 to 5.8 particles/cm2yr at site OL3, whereas the massive charcoal accumulation at around 3,500 cal yr BP strongly influences the overall result of OL3. Average macro-charcoal influx seems to correlate with lake size. Other environmental factors are nearly identical at this location.
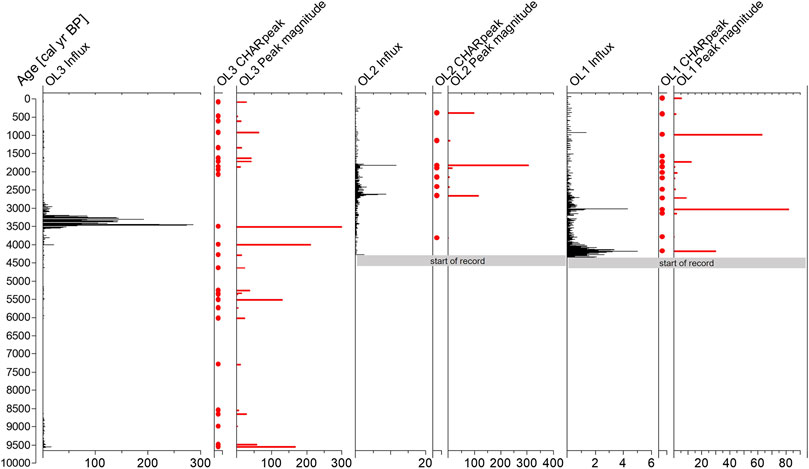
FIGURE 6. Tarvagatai Nuruu NP macro-charcoal influxes (particles/cm2yr), peaks, and respective peak magnitudes (particles/cm2peak) based on CharAnalysis.
CharAnalysis software suggests 14 CHARpeaks for OL1, eight CHARpeaks for OL2 and 26 CHARpeaks for OL3, each with varying peak magnitudes. However, it must be considered that core OL3 dates back more than 4,000 years further than OL1 and OL2. Average peak magnitudes vary from 15 particles/cm2peak (OL1) and 48 particles/cm2peak (OL3) to 69 particles/cm2peak (OL2).
Assuming that the age-depth-models are correct, there is no particular synchronicity among the reconstructed CHARpeaks of the three macro-charcoal records. However, the two fire events at around 3,500 cal yr BP and around 4,000 cal yr BP might potentially be related.
4.2.2 Fire frequency
Fire frequencies are generally lower at Tarvagatai Nuruu NP compared to the Mongolian Altai. Relative synchronicity between all three sites can be detected from around 2,400 to 1,500 cal yr BP. All other time periods are characterized by a higher variability. Discrepancies are especially high between the big lake OL3 and the two smaller lakes, OL1 and OL2. Overall, the range of reconstructed fire frequency is higher at site OL3.
4.2.3 Site-specific macro-charcoal morphotype accumulation
In the Tarvagatai Nuruu NP, the wood particle fraction is abundant in two macro-charcoal records: 42.3% at site OL2 and 42.2% at site OL3 (Table 2; Figure 7). At site OL1, charred grass material dominates the morphotype assemblage (40.2%).
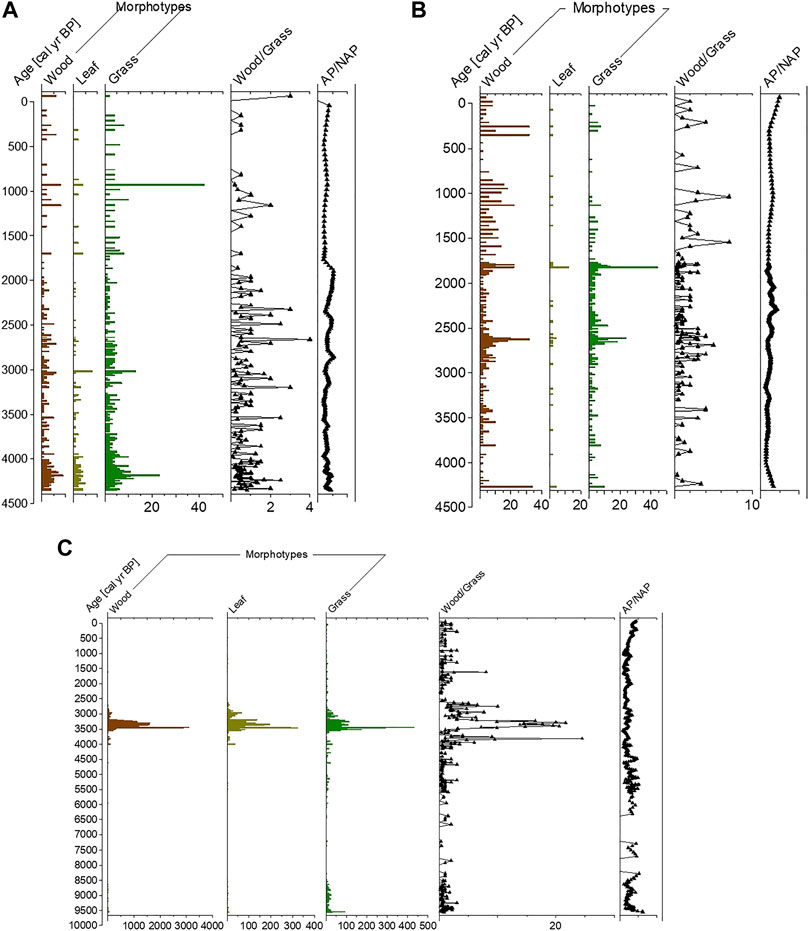
FIGURE 7. Macro-charcoal morphotypes (total counts per sample), wood/grass morphotype ratios, and AP/NAP ratios: (A) site OL1; pollen data from Li (unpublished), (B) site OL2; pollen data from Saindovdon (unpublished), and (C) site OL3; charcoal and pollen data from Unkelbach et al. (2021b).
In this area, only the macro-charcoal record at site OL3 shows an increased average wood/grass ratio of 1.1968. Comparably low average wood/grass ratios are present at sites OL1 (0.5223) and OL2 (0.6750). At site OL1 (Figure 7A), the wood/grass ratio shows a great number of changes over the whole macro-charcoal record. The most distinct peaks are suggested for 3,200 cal yr BP, 2,660 cal yr BP, 2,320 cal yr BP, 1,150 cal yr BP and the topmost sample from the year of coring. The wood/grass ratio at site OL2 (Figure 7B) is characterized by several variations, especially from 1800 cal yr BP to the present. The two most distinct maxima are displayed at around 1,540 cal yr BP and 1,040 cal yr BP. At site OL3 (Figure 7C), the wood/grass ratio shows the most prominent changes. Several peaks are suggested for the episode of increased fire activity from 3,550 to 2,640 cal yr BP and one distinct maximum at around 1,600 cal yr BP.
5 Discussion
5.1 Driving factors of fire in western and north-central Mongolia
In both study areas, the reconstructed fire histories over the Holocene are characterized by heterogenic patterns and shorter episodes of relative consistency (Figure 7). A rather homogenous fire reconstruction at all sites within one area suggests that regional factors, e.g., climate, are the main drivers of fire activity, whereas contrasting patterns indicate that local factors, e.g., topography, vegetation, human activity, or microclimate, superimpose regional signals (Whitlock et al., 2010).
In the Mongolian Altai, the composite macro-charcoal reconstructions (Figures 3, 4A) show a rather low fire activity from 4,310 to 1,100 cal yr BP. A marked increase in the fire regimes of all records is visible between 1,100 and 400 cal yr BP, followed by an episode of differing patterns and a maximum of fire activity over the last 100 years (in three of five records). Although radiocarbon-dated cereal grains from Tongtian Cave in the Chinese Altai indicate the presence of early settlers in the greater region from around 5,200 cal yr BP (Zhou et al., 2020), their impact on the fire activity in the area was presumably rather low. A comparison of our macro-charcoal data with available palynological records from western Mongolia (Rudaya et al., 2009; Sun et al., 2013; Huang et al., 2018; Zhang and Feng, 2018; Unkelbach et al., 2019) shows that rather humid climate conditions limited fire activity and fire regimes in the area. The pronounced increase in fire frequency at all sites starting around 1,100 cal yr BP coincides with abrupt decreases in the amount of biomass and plant diversity caused by a change to more arid and cold climate conditions (Sun et al., 2013; Unkelbach et al., 2019). Presumably, during this time, the temperature extremes between winter and summer also developed (Unkelbach et al., 2019). For all these reasons, more nomadic herders were forced to move on to unused pastureland in even more remote areas in the mountains, where they probably contributed to the destruction of forests, which increased the area of grasslands (Huang et al., 2018). Even more increased aridity and overgrazing favored lower aboveground biomass as fuel for fire, which may have resulted in less severe fires as expected from human activity (Umbanhowar et al., 2009). Additionally, in the forest-steppe of Mongolia, there is no tradition for burning grasslands on purpose as a tool for increasing pasture productivity because without enough precipitation following a fire event, burning even increases the risk of erosion and productivity loss during periods of drought (Drewa and Havstad, 2001; Germeraad and Enebisch, 1996; O`Dea and Guertin, 2003; Umbanhowar et al., 2009). Thus, smaller strictly local fires for producing heat and food for the nomadic herders, in combination with the local topography and vegetation, might have resulted in the individual fire histories after 1,100 cal yr BP. Over the last 100 years, climate warming, degradation of permafrost, changes in herding practices, and industrialization might have all affected the fire regimes (Fernández-Giménez, 1999; Lkhagvadorj et al., 2013; Unkelbach et al., 2020).
A similar trend can also be found in the fire history of the Tarvagatai Nuruu NP. Composite macro-charcoal reconstructions (Figures 4B, 6) indicate a high fire activity from 9,500 to 7,100 cal yr BP and an episode of low fire activity during the mid-Holocene. After 4,000 cal yr BP, fires become more frequent, but only a short period between 2,400 and 1,500 cal yr BP is characterized by a relative synchroneity. Compared to the Mongolian Altai, fire frequencies and fire regimes are lower except for the fire event in core OL3 at around 3,500 cal yr BP. Also pollen and coprophilous fungi data indicate a rather limited human impact in the area, even since the introduction (5,500–3,300 cal yr BP) and expansion (2,800–2,400 cal yr BP) of nomadic herding in Mongolia (Fernández-Giménez, 1999; Fernández-Giménez et al., 2017; Unkelbach et al., 2021b). Hence, our data suggest a mainly natural, climate-driven fire history for the northern Khangai. The variability between sites after 1,500 cal yr BP may still be attributed to rare human-induced fires or other local characteristics, e.g. microclimate or topographic features that limit/enhance the input of charcoal particles at a certain place. However, the small number of samples and the small total number of charcoal particles per sample must also be considered when interpreting our data from northern Khangai.
In other studies focusing on comparative charcoal analyses of several sites from the Pyrenees, France (Rius et al., 2011), the Carpathians, Romania (Florescu et al., 2018), and Northern Germany (Robin and Nelle, 2014), a clear distinction could be made between climate-induced wildfires during the early and mid-Holocene and human-made fires after permanently inhabiting the area. Especially during the last two millennia, human populations used fire frequently to open forests for agriculture or to promote grass growth for pastures. In very sparsely populated western and north-central Mongolia, these practices have been uncommon due to the history of nomadic herding and prohibitions of burning in the local fire management (Umbanhowar et al., 2009).
However, comparative studies from two remote areas in northwestern Russia (Kuosmanen et al., 2014) and northern Siberia (Novenko et al., 2022) yielded different interpretations for their varying reconstructed fire histories at the individual site. Kuosmanen et al. (2014) argued that in unmanaged boreal forests with patchy forest vegetation, the marked site-to-site variability is mainly controlled by topographic and microclimatic factors rather than human activity. In contrast, the findings from the transition zone from mixed Larix-forest to sub-Arctic tundra by Novenko et al. (2022) indicate a rather homogenous fire history between their five sites only following climate patterns.
5.2 Macro-charcoal accumulation in different environments
Our results show major discrepancies between the derived fire histories of all individual sites in both study areas (Figures 5, 7). It seems as if, in addition to the main drivers, namely, climate and human practices, basin type and surrounding vegetation also affect the macro-charcoal accumulation.
In the Mongolian Altai, macro-charcoal influx and peak magnitudes are markedly higher at site Tr5-2B within the forest belt (Figure 3) compared to the steppe sites, and the reconstructed fire frequency also shows the most contrasting trend (Figure 4A). This is substantiated by the fact that not only fire intensity but also the type, amount, and flammability of fuel influence the charcoal abundance in sediments (Pitkänen et al., 1999; Florescu et al., 2018). Hence, burning ground vegetation in steppes produces smaller amounts of charcoal residue than when L. sibirica trees are burnt (Pitkänen et al., 1999; Gavin et al., 2007). Consequently, (natural) chances in vegetation, e.g., shifts in the tree line, have an impact on fire occurrence and severity (Gavin et al., 2007). This was also observed in southern Scandinavian forests, where records from the hemiboreal region showed a greater temporal fire variability than in the temperate zone since temperate vegetation is regarded as less flammable than boreal vegetation (Rackham, 1986; Bradshaw et al., 2010). However, Bradshaw et al. (2010) discovered that compared to lake sediments, pollen accumulation rates are prone to be much higher in archives directly from forest basins, which most likely also applies to charcoal particles. Thus, our findings still need to be treated with caution. Unfortunately, having no information on the size of the “charcoal source area” of historical fires also complicates distinguishing between small and large fires and peak detection (Clark and Patterson, 1997; Gavin et al., 2007). Disparities between sites Ch, Tr5-2B and G2-A may also be explained by orographic and altitudinal effects, as also observed in the Harz Mountains, Germany (Robin and Nelle, 2014).
In the northern Khangai, general discrepancies in charcoal influx values (OL3 > OL2 > OL1; Figure 6) may be explained by differences in their catchment area. Lakes usually have a larger catchment than soils (Clark and Patterson, 1997); hence, the catchment may increase with lake size. Additionally, findings by Clark and Patterson (1997) of charcoal data from the United States suggested that clear charcoal peaks can only be detected in lake sediments when fires occurred close to the shore, but fires outside of the catchment resulted in extremely low values. The same seems to apply to most of our studied sites.
A similar comparative study from a lake and three peat bogs in the Pyrenees, France, by Rius et al. (2011) supports our heterogenic results; the study showed that charcoal accumulation differs significantly between the types of basins, but in that region, fire frequency calculations minimized the discrepancies. Thus, fire frequency variations still allow the detection of regional and local drivers of historical fire activity.
5.3 Impact of fires on the forest-steppe distribution in western and north-central Mongolia
Comparison of the wood/grass macro-charcoal ratio and the AP/NAP ratio of the same site allows a rough assessment of the influence of fire on the general distribution of forest and steppe in both study areas. There can be short-term (decennial to centennial scale) or long-term (millennial scale) changes in the vegetation composition following a fire event. In addition to a general change in fire frequency, the intensity, magnitude, and location of fire are equal factors when investigating its influence on the change of forest composition and structure (Ryan, 2002; Kuosmanen et al., 2014). However, interpretation results should be treated with caution because other natural influences, e.g., changing climate, also need to be considered while discussing the forest-steppe distribution of this sensitive ecosystem.
In the Mongolian Altai, a main finding of our site-specific results in this regard is that not all sites show linkages between the AP/NAP ratio and forest fires (Figure 5). At sites D1L1 and G2-A, the forest-steppe distribution seems to be entirely unaffected by fire activity. At site D3L6, high wood/grass ratios are reconstructed after declines in the AP/NAP ratios, at least before 2000 cal yr BP. This correlation is probably due to the higher proportion of dry wood after a possible climate-related decline of forest stands (Umbanhowar et al., 2009; Bruegger et al., 2018; Unkelbach et al., 2019). A similar relation can be observed at site Tr5-2B at around 600 cal yr BP, but after that marked fire event, the AP/NAP ratio was permanently lower at this site within the forest, which might indicate that the L. sibirica population remained smaller in the long-term. In contrast, at site Ch, an increase in the AP/NAP ratio can be observed after the high wood/grass ratio fire event around 70 years ago. This increase is caused by a significant spread of Salix shrubs (Unkelbach et al., 2021a) due to a more open forest character and moister soil conditions as a response to recent climate warming (permafrost degradation).
Unfortunately, for the northern Khangai, our site-specific macro-charcoal results suggest little to no linkages between the forest-steppe distribution and the occurrence of fire. Only a small relation between synchronously decreasing wood/grass and AP/NAP ratios can be detected at site OL1 after around 1,900 cal yr BP. However, it is difficult to determine a definite cause for this connection, but a climate-induced forest decline seems plausible.
Even though L. sibirica is classified as a pyrophytic species (Tsvetkov, 2004; Kharuk et al., 2019), increased fire activity over the last 1,100 cal yr BP (Mongolian Altai) and 4,000 cal yr BP (northern Khangai) does not provide evidence that it has increased the local Larix populations or altered the regional vegetation composition markedly. These findings are supported by the study by Kuosmanen et al. (2014) from an unmanaged boreal forest in northwestern Russia and by Bradshaw et al. (2010) from southern Scandinavian forest ecosystems.
6 Conclusion
Our high-resolution and multi-site macro-charcoal data from eight sites in western and north-central Mongolia allow a better understanding of the fire history in the forest-steppe ecosystem.
Based on our macro-charcoal data, we conclude that during the early and mid-Holocene, it seems most likely that the fire history in both our study areas is defined by the regional climate variability. This finding could be expected according to the syntheses of charcoal records from the global charcoal database (GCD) (Power et al., 2008; Daniau et al., 2012), although the oscillations are higher in the Mongolian Altai. Rises in the fire frequency correlate with the potential increase of the inhabitation of the Mongolian Altai by nomadic herders after 1,100 cal yr BP. Locally reconstructed macro-charcoal peaks and a very low impact on the forest-steppe distribution suggests that the marked site-to-site variability may mostly be explained by local nomadic disturbance, which is historically lower in the study region of northern Khangai. However, due to the extreme climatic conditions and the nomadic lifestyle (in contrast to conventional agriculture), an evaluation of our results in the global context is rather difficult.
Our study provides the first fire frequency synthesis for two regions in Mongolia and reveals the potential of multi-site macro-charcoal studies from different types of archives. Higher and more differentiated data availability, especially from the northern Khangai, would significantly improve the validity of our results.
Data availability statement
The raw data supporting the conclusion of this article will be made available by the authors, without undue reservation.
Author contributions
JU and HB developed the project idea. Fieldwork was carried out by HB. JU analyzed the data and prepared the manuscript. HB contributed to the discussion and read and approved the manuscript.
Funding
This study was supported by the German Science Foundation (DFG) research project BE 2116/28-2 and carried out at the University of Goettingen, Germany.
Acknowledgments
The authors are grateful to the team of Prof. G. Punsalpaamuu (*) and D. Saindovdon from the Mongolian State University of Education, Ulaanbaatar, Prof. U. Beket from Bayan-Ulgii, and Dr. Ch. Dulamsuren for their valuable help during field work. They thank A. Lemkul, A. Reinhardt, and U. Nüsse-Hahne for their assistance in laboratory preparation of pollen and charcoal samples. They are grateful to Dr. J. Li and D. Saindovdon for providing pollen data of cores OL1 and OL2. They also thank the editor Prof. Hong Yan and two reviewers for their valuable and comprehensive feedback on an earlier version of the manuscript.
Conflict of interest
The authors declare that the research was conducted in the absence of any commercial or financial relationships that could be construed as a potential conflict of interest.
Publisher’s note
All claims expressed in this article are solely those of the authors and do not necessarily represent those of their affiliated organizations, or those of the publisher, the editors, and the reviewers. Any product that may be evaluated in this article, or claim that may be made by its manufacturer, is not guaranteed or endorsed by the publisher.
Supplementary material
The Supplementary Material for this article can be found online at: https://www.frontiersin.org/articles/10.3389/feart.2022.959914/full#supplementary-material
References
Ali, A. A., Blarquez, O., Girardin, M. P., Hély, C., Tinquaut, F., El Guellab, A., et al. (2012). Control of the multimillennial wildfire size in boreal North America by spring climatic conditions. Proc. Natl. Acad. Sci. U.S.A. 109 (51), 20966–20970. doi:10.1073/pnas.1203467109
AM Online Projects (2022). Klima & wetter in ölgii. AvaliableAt: https://de.climate-data.org/location/29828.
Ariuntsetseg, L., Boldgiv, B., and Golde, A. (2008). Main habitats and floristic diversity in the Tarvagatai Nuruu national Park – general view. Mong. J. Biol. Sci. 6, 11–20. doi:10.22353/mjbs.2008.06.02
Beket, U., and Knapp, H. D. (2012). Protection of the natural and cultural heritage of the Mongolian Altai. Explor. into Biol. Resour. Mong. 12, 335–352.
Bradshaw, R. H., Lindbladh, M., and Hannon, G. E. (2010). The role of fire in southern Scandinavian forests during the late Holocene. Int. J. Wildland Fire 19 (8), 1040–1049. doi:10.1071/wf09108
Bruegger, S. O., Gobet, E., Sigl, M., Osmont, D., Papina, T., Rudaya, N., et al. (2018). Ice records provide new insights into climatic vulnerability of Central Asian forest and steppe communities. Glob. Planet. Change. 169, 188–201. doi:10.1016/j.gloplacha.2018.07.010
Clark, J. S., and Patterson, W. A. (1997). “Background and local charcoal in sediments: Scales of fire evidence in the paleorecord,” in Background and local charcoal in sediments: Scales of fire evidence in the paleorecord” in sediment records of biomass burning and global change. Editors J. S. Clark, H. Cachier, J. G. Goldammer, and B. J. Stocks (Berlin Heidelberg: Springer), 23–48. doi:10.1007/978-3-642-59171-6_3
Daniau, A. L., Bartlein, P. J., Harrison, S. P., Prentice, I. C., Brewer, S., Friedlingstein, P., et al. (2012). Predictability of biomass burning in response to climate changes. Glob. Biogeochem. Cycles 26 (4), GB4007. doi:10.1029/2011GB004249
Drewa, P. B., and Havstad, K. M. (2001). Effects of fire, grazing, and the presence of shrubs on Chihuahuan desert grasslands. J. Arid Environ. 48 (4), 429–443. doi:10.1006/jare.2000.0769
Dulamsuren, C., Khishigjargal, M., Leuschner, C., and Hauck, M. (2014). Response of tree-ring width to climate warming and selective logging in larch forests of the Mongolian Altai. J. Plant Ecol. 7, 24–38. doi:10.1093/jpe/rtt019
Eichler, A., Tinner, W., Brütsch, S., Olivier, S., Papina, T., and Schwikowski, M. (2011). An ice-core based history of Siberian forest fires since AD 1250. Quat. Sci. Rev. 30, 1027–1034. doi:10.1016/j.quascirev.2011.02.007
Fernández-Giménez, M. E. (1999). Sustaining the steppes: A geographical history of pastoral land use in Mongolia. Geogr. Rev. 89 (3), 315–342.
Fernández-Giménez, M. E., Venable, N. H., Angerer, J., Fassnacht, S. R., Reid, R. S., and Khishigbayar, J. (2017). Exploring linked ecological and cultural tipping points in Mongolia. Anthropocene 17, 46–69.
Florescu, G., Vannière, B., and Feurdean, A. (2018). Exploring the influence of local controls on fire activity using multiple charcoal records from northern Romanian Carpathians. Quat. Int. 488, 41–57. doi:10.1016/j.quaint.2018.03.042
Gavin, D. G., Hallett, D. J., Hu, F. S., Lertzman, K. P., Prichard, S. J., Brown, K. J., et al. (2007). Forest fire and climate change in Western north America: Insights from sediment charcoal records. Front. Ecol. Environ. 5 (9), 499–506. doi:10.1890/060161
Germeraad, P. W., and Enebisch, Z. (1996). The Mongolian landscape tradition: A key to progress. Nomadic traditions and their contemporary role in landscape planning and management in Mongolia. Netherlands: Rhoon.
Gunin, P. D., Vostokova, E. A., Dorofeyuk, N. I., Tarasov, P. E., and Black, C. C. (1999). Vegetation dynamics of Mongolia. Dordrecht: Kluwer Academic Publishers.
Higuera, P. E., Brubaker, L. B., Anderson, P. M., Hu, F. S., and Brown, T. A. (2009). Vegetation mediated the impacts of postglacial climate change on fire regimes in the south-central Brooks Range, Alaska. Ecol. Monogr. 79, 201–219. doi:10.1890/07-2019.1
Higuera, P. E. (2009). CharAnalysis 0.9: Diagnostic and analytical tools for sediment-charcoal analysis. AvaliableAt: https://github.com/phiguera/CharAnalysis.
Huang, X., Peng, W., Rudaya, N., Grimm, E. C., Chen, X., Cao, X., et al. (2018). Holocene vegetation and climate dynamics in the Altai mountains and surrounding areas. Geophys. Res. Lett. 45, 6628–6636. doi:10.1029/2018gl078028
Kharuk, V. I., Ranson, K. J., Petrov, I. A., Dvinskaya, M. L., Im, S. T., and Golyukov, A. S. (2019). Larch (Larix dahurica Turcz) growth response to climate change in the Siberian permafrost zone. Reg. Environ. Change. 19, 233–243. doi:10.1007/s10113-018-1401-z
Klinge, M., Dulamsuren, C., Schneider, F., Erasmi, S., Bayarsaikhan, U., Sauer, D., et al. (2021). Geoecological parameters indicate discrepancies between potential and actual forest area in the forest-steppe of Central Mongolia. For. Ecosyst. 8, 55. doi:10.1186/s40663-021-00333-9
Kuosmanen, N., Fang, K., Bradshaw, R. H., Clear, J. L., and Seppä, H. (2014). Role of forest fires in Holocene stand-scale dynamics in the unmanaged taiga forest of northwestern Russia. Holocene 24 (11), 1503–1514. doi:10.1177/0959683614544065
Li, Y., Zhang, Y., Wang, J., Wang, L., Li, Y., Chen, L., et al. (2019). Preliminary study on pollen, charcoal records and environmental evolution of Alahake Saline Lake in Xinjiang since 4,700 cal yr BP. Quat. Int. 513, 8–17. doi:10.1016/j.quaint.2019.01.014
Lkhagvadorj, D., Hauck, M., Dulamsuren, C., and Tsogtbaatar, J. (2013). Twenty years after decollectivization: Mobile livestock husbandry and its ecological impact in the Mongolian forest-steppe. Hum. Ecol. 41, 725–735. doi:10.1007/s10745-013-9599-3
Mustaphi, C. C., and Pisaric, M. F. J. (2014). A classification for macroscopic charcoal morphologies found in Holocene lacustrine sediments. Prog. Phys. Geogr. Earth Environ. 38 (6), 734–754. doi:10.1177/0309133314548886
Novenko, E. Y., Kupryanov, D. A., Mazei, N. G., Prokushkin, A., Phelps, L. N., Buri, A., et al. (2022). Evidence that modern fires may be unprecedented during the last 3400 years in permafrost zone of central Siberia, Russia. Environ. Res. Lett. 17, 025004. doi:10.1088/1748-9326/ac4b53
O'Dea, M. E., and Guertin, D. P. (2003). Prescribed fire effects on erosion parameters in a perennial grassland. J. Range Manag. 56 (1), 27–32. doi:10.2307/4003877
Patterson, W. A., Edwards, K. J., and Maguire, D. J. (1987). Microscopic charcoal as a fossil indicator of fire. Quat. Sci. Rev. 6, 3–23. doi:10.1016/0277-3791(87)90012-6
Pitkänen, A., Lehtonen, H., and Huttunen, P. (1999). Comparison of sedimentary microscopic charcoal particle records in a small lake with dendrochronological data: Evidence for the local origin of microscopic charcoal produced by forest fires of low intensity in eastern Finland. Holocene 9 (5), 559–567.
Power, M. J., Marlon, J., Ortiz, N., Bartlein, P. J., Harrison, S. P., Mayle, F. E., et al. (2008). Changes in fire regimes since the last glacial maximum: An assessment based on a global synthesis and analysis of charcoal data. Clim. Dyn. 30 (7), 887–907. doi:10.1007/s00382-007-0334-x
Rackham, O. (1986). “The history of the countryside: The classic history of britain’s landscape,” in Flora, and fauna (London: JM Dent).
Rius, D., Vannière, B., Galop, D., and Richard, H. (2011). Holocene fire regime changes from multiple-site sedimentary charcoal analyses in the Lourdes basin (Pyrenees, France). Quat. Sci. Rev. 30 (13-14), 1696–1709. doi:10.1016/j.quascirev.2011.03.014
Robin, V., and Nelle, O. (2014). Contribution to the reconstruction of central European fire history, based on the soil charcoal analysis of study sites in northern and central Germany. Veg. Hist. Archaeobotany 23 (1), 51–65. doi:10.1007/s00334-014-0438-2
Rudaya, N., Tarasov, P. E., Dorofeyuk, N. I., Solovieva, N., Kalugin, I., Andreev, A., et al. (2009). Holocene environments and climate in the Mongolian Altai reconstructed from the hoton-nur pollen and diatom records: A step towards better understanding climate dynamics in central Asia. Quat. Sci. Rev. 28, 540–554. doi:10.1016/j.quascirev.2008.10.013
Ryan, K. C. (2002). Dynamic interactions between forest structure and fire behavior in boreal ecosystems. Silva Fenn. 36 (1), 13–39. doi:10.14214/sf.548
Schneider, F., Klinge, M., Brodthuhn, J., Peplau, T., and Sauer, D. (2021). Hydrological soil properties control tree regrowth after forest disturbance in the forest steppe of central Mongolia. Soil 7, 563–584. doi:10.5194/soil-7-563-2021
Scott, A. C. (2010). Charcoal recognition, taphonomy and uses in palaeoenvironmental analysis. Palaeogeogr. Palaeoclimatol. Palaeoecol. 291, 11–39. doi:10.1016/j.palaeo.2009.12.012
Stevenson, J., and Haberle, S. (2005). Macro charcoal analysis: A modified technique used by the department of archaeology and natural history. Palaeoworks techn. Papers 5. Canberra: Australian National University.
Sun, A., Feng, Z., Ran, M., and Zhang, C. (2013). Pollen-recorded bioclimatic variations of the last ∼22,600 years retrieved from Achit Nuur core in the Western Mongolian Plateau. Quat. Int. 311, 36–43. doi:10.1016/j.quaint.2013.07.002
Tsvetkov, P. A. (2004). Pyrophytic properties of the larch Larix gmelinii in terms of life strategies. Russ. J. Ecol. 35 (4), 224–229. doi:10.1023/b:ruse.0000033790.50606.54
Umbanhowar, C. E., and Mcgrath, M. J. (1998). Experimental production and analysis of microscopic charcoal from wood, leaves and grasses. Holocene 8 (3), 341–346. doi:10.1191/095968398666496051
Umbanhowar, C. E., Shinneman, A. L. C., Tserenkhand, G., Hammon, E. R., Lor, P., and Nail, K. (2009). Regional fire history based on charcoal analysis of sediments from nine lakes in Western Mongolia. Holocene 19 (4), 611–624. doi:10.1177/0959683609104039
Unkelbach, J., Dulamsuren, Ch., and Behling, H. (2021a). Late Holocene climate and land-use history in the Mongolian Altai mountains: Combined evidence from palynological, macro-charcoal and tree-ring analyses. Trees, For. People 4, 100073. doi:10.1016/j.tfp.2021.100073
Unkelbach, J., Dulamsuren, C., Klinge, M., and Behling, H. (2021b). Holocene high-resolution forest-steppe and environmental dynamics in the Tarvagatai Mountains, north-central Mongolia, over the last 9570 cal yr BP. Quat. Sci. Rev. 266, 107076. doi:10.1016/j.quascirev.2021.107076
Unkelbach, J., Dulamsuren, C., Punsalpaamuu, G., Saindovdon, D., and Behling, H. (2018). Late Holocene vegetation, climate, human and fire history of the forest-steppe-ecosystem inferred from core G2-A in the 'Altai Tavan Bogd' conservation area in Mongolia. Veget Hist. Archaeobot 27 (5), 665–677. doi:10.1007/s00334-017-0664-5
Unkelbach, J., Kashima, K., Enters, D., Dulamsuren, Ch., Punsalpaamuu, G., and Behling, H. (2019). Late Holocene (Meghalayan) palaeoenvironmental evolution inferred from multi-proxy-studies of lacustrine sediments from the Dayan Nuur region of Mongolia. Palaeogeogr. Palaeoclimatol. Palaeoecol. 530, 1–14. doi:10.1016/j.palaeo.2019.05.021
Unkelbach, J., Kashima, K., Punsalpaamuu, D., Shumilovskikh, Ch., and Behling, H. (2020). Decadal high-resolution multi-proxy analysis to reconstruct natural and human-induced environmental changes over the last 1350 cal. yr BP in the Altai Tavan Bogd National Park, Western Mongolia. Holocene 30 (7), 1016–1028. doi:10.1177/0959683620908662
Whitlock, C., Bianchi, M. M., Bartlein, P. J., Markgraf, V., Marlon, J., Walsh, M., et al. (2006). Postglacial vegetation, climate, and fire history along the east side of the Andes (lat 41-42.5°S), Argentina. Quat. Res. 66, 187–201. doi:10.1016/j.yqres.2006.04.004
Whitlock, C., Higuera, P. E., McWethy, D. B., and Briles, C. E. (2010). Paleoecological perspectives on fire ecology: Revisiting the fire-regime concept. Open Ecol. J. 3 (1), 6–23. doi:10.2174/1874213001003020006
Whitlock, C., and Larsen, C. (2001). “Charcoal as a fire proxy,” in Tracking environmental change using lake sediments. Terrestrial, algal, and siliceous indicators. Editors J. P. Smol, H. J. B. Birks, and W. M. Last (Dordrecht: Kluwer), 3, 75–97.
Xiao, X., Haberle, S. G., Shen, J., Xue, B., Burrows, M., and Wang, S. (2017). Postglacial fire history and interactions with vegetation and climate in Southwestern Yunnan Province of China. Clim. Past. 13, 613–627. doi:10.5194/cp-13-613-2017
Zhang, D., and Feng, Z. (2018). Holocene climate variations in the Altai mountains and the surrounding areas: A synthesis of pollen records. Earth-Science Rev. 185, 847–869. doi:10.1016/j.earscirev.2018.08.007
Keywords: fire history, fire frequency, macro-charcoal, multi-site, Holocene, Mongolia, human impact
Citation: Unkelbach J and Behling H (2022) The reconstruction of Holocene northwestern Mongolian fire history based on high-resolution multi-site macro-charcoal analyses. Front. Earth Sci. 10:959914. doi: 10.3389/feart.2022.959914
Received: 02 June 2022; Accepted: 25 July 2022;
Published: 29 August 2022.
Edited by:
Hong Yan, Institute of Earth Environment (CAS), ChinaReviewed by:
Xiaozhong Huang, Lanzhou University, ChinaJianghu Lan, Institute of Earth Environment (CAS), China
Copyright © 2022 Unkelbach and Behling. This is an open-access article distributed under the terms of the Creative Commons Attribution License (CC BY). The use, distribution or reproduction in other forums is permitted, provided the original author(s) and the copyright owner(s) are credited and that the original publication in this journal is cited, in accordance with accepted academic practice. No use, distribution or reproduction is permitted which does not comply with these terms.
*Correspondence: Julia Unkelbach, anVsaWEudW5rZWxiYWNoQGJpb2xvZ2llLnVuaS1nb2V0dGluZ2VuLmRl