- 1GEOMAR Helmholtz-Centre for Ocean Research Kiel, Kiel, Germany
- 2Consultant, Nuglar, Switzerland
Volcanoes are sources of numerous threats including lava flows, pyroclastic flows, ash dispersal and landslides or sector collapses. In addition to these commonly known volcanic hazards, volcano-induced tsunamis can occur in the marine environment, introducing a major hazard that can affect populations located far away from the volcanoes. Existing tsunami warning systems generally do not account for volcano-generated tsunamis, due to the multiple source mechanisms that can cause such tsunamis, a limited understanding of precursory signals for these events, and the need for local detection rather than remote sensing. Among these source mechanisms of volcanic tsunamis, sector and lateral collapses are at the high risk-low frequency extreme of risk matrices. Marine volcanoes grow in specific environments, with factors like marine clays, constant full saturation, sediment transport and remobilization, interaction with ocean dynamics, and sea level changes that may impact edifice stability in distinct ways. The majority of historically documented marine volcano collapses occurred at erupting volcanoes, suggesting that eruptions could serve as a remotely detectable warning signal for collapses. However, careful examination of temporal sequences of these examples reveals that collapses do not always follow eruptions. Consequently, there is a need for identifying other, more robust precursors to volcano collapse, in particular in the marine environment, where the consequences of collapses may be widespread.
1 Introduction
Volcano collapses have the potential to generate massive and destructive landslides, as demonstrated by the 1980 collapse of Mount St. Helens, which involved a volume of ∼2.7 km3 (Moore et al., 1981; Voight et al., 1981). In marine environments, the sudden displacement of large amounts of material into the water can trigger tsunamis with far-reaching impacts on coastal areas, as seen in the 2018 collapse of Anak-Krakatau in Indonesia.
Historical records indicate that collapses of marine volcanoes have resulted in ∼15,000 fatalities over the past 400 years (Day, 2015; Grilli et al., 2019). There have been at least 10 documented cases of tsunamigenic volcano collapses (e.g., Day, 2015; Dufresne et al., 2021), and geological records provide evidence of giant collapses involving the mobilization of tens to thousands of cubic kilometers of material (Blahůt et al., 2019). Many Pleistocene and Holocene volcanoes (represented by black triangles in Figure 1) show signs of collapse or flank instability. Yet, in 2015, 10.6% (781 million) of the global population lived in Low Elevation Coastal Zones (LECZ), which make up only 2.1% of Earth’s land area and are vulnerable to marine hazards, including volcanogenic tsunamis (Supplementary Table S1). LECZ refers to connected coastal areas below 10 m of elevation (colored areas in Figure 1). Furthermore, international commerce and commodity supply are also vulnerable to tsunamis due to their reliance on global maritime traffic and coastal infrastructure, such as telecommunication cables, production platforms, and hydrocarbon pipelines (Clare et al., 2019). Projections indicate that exposure and vulnerability to tsunamis will increase. Neumann et al. (2015) predict a disproportionate population growth in LECZ by 2060, with 12.3% (1.4 billion) of the world’s population living in these areas. In Southeast Asia, home to nearly half of the known active volcanoes (∼48%), the population in LECZ is expected to increase from ∼590 million in 2015 to ∼950 million in 2060. Consequently, it is urgent to assess the hazards associated with volcano collapses and tsunamis and develop forecasting and early warning strategies.
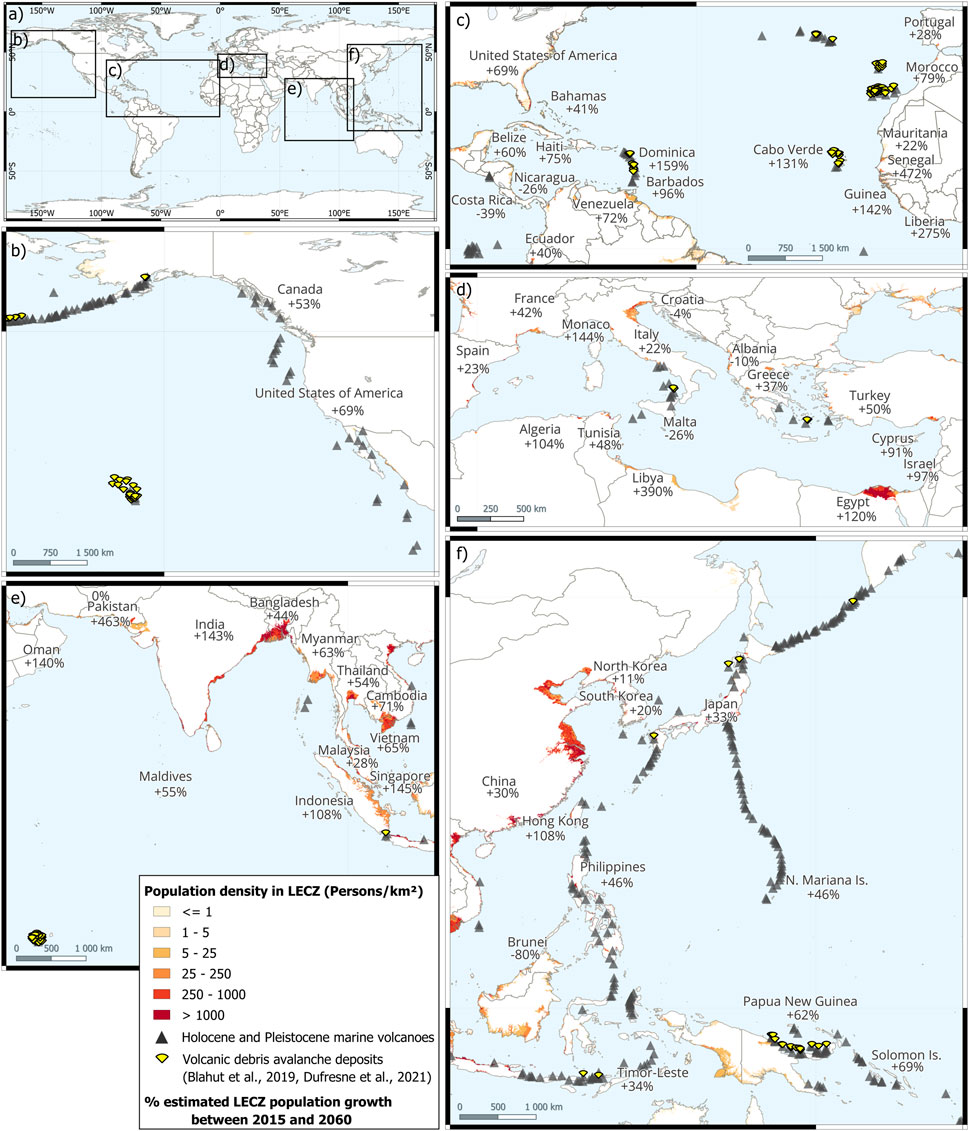
FIGURE 1. (A) Location of the close up views of (B) Northeast Pacific Rim, (C) Atlantic Ocean, (D) Mediterranean Sea, (E) Indian Ocean and (F) West Pacific Rim. Representation of the population density (color scale) in Low Elevation Coastal Zones (LECZ, colored area) in 2020 along with marine volcanoes reported to be active during the Pleistocene and the Holocene (black triangles, extracted from Global Volcanism Program, 2013). Population densities come from GPWv411 database (Center for International Earth Science Information Network - CIESIN - Columbia University, 2018) and elevation filter for LECZ estimate come from Center for International Earth Science Information Network - CIESIN - Columbia University and CUNY Institute for Demographic Research - CIDR - City University of New York (2021). The yellow inverted triangles indicate the location of Volcanic Debris Avalanche Deposits from Blahůt et al. (2019). Predictions of population growth in LECZ for 2060 are indicated as growth percentage (see scenario C from Neumann et al., 2015; Supplementary Table S1).
Scientists have long been investigating volcano collapses and their associated hazards. Giachetti et al. (2012) studied volcano-generated tsunamis triggered by a hypothetical collapse of Anak-Krakatau, highlighting the regional damage such an event would cause and emphasizing the need to include tsunami hazards in volcano monitoring. However, no warning was issued before the 2018 collapse of Anak-Krakatau (Zorn et al., 2023), which resulted in a tsunami and caused 437 deaths along nearby shores (Grilli et al., 2019; Walter et al., 2019). Despite a small earthquake with a local magnitude ML = 2–3 (Walter et al., 2019), there was no significant earthquake associated with the collapse that could trigger the warning system. In fact, most Tsunami Early Warning Systems (TEWS) do not currently consider volcanic tsunamis (Paris et al., 2014; Paris, 2015), including the Indonesian system (Ina-TEWS), which is designed for earthquake-generated tsunamis after the destructive 2004 tsunami caused by an earthquake. One exception is the TEWS at Stromboli Island, where Italian authorities established a monitoring system based on tide gauges surrounding the island after the 2002 tsunami triggered by submarine and subaerial landslides in the Sciara del Fuoco (Bellotti et al., 2009; De Girolamo et al., 2014). Although local TEWS can effectively mitigate hazards related to volcano collapse, these examples indicate that such warning systems are often established reactively following an event. To enable proactive measures before a volcano collapse, it is crucial to identify the highest-risk volcanoes and understand the precursor signals indicating an impending collapse.
Evidence of flank instability at La Palma, Canary Islands, was identified 2 decades ago (Day et al., 1999), with limited follow-up studies (González et al., 2010). It was not until the recent magmatic unrest at Cumbre Vieja volcano in 2021 that concerns about the possibility of volcano collapse at La Palma reawoke and sparked interest in the scientific community (González, 2022) and the public. The number of scientific publications (Periáñez, 2021; Fernández et al., 2022; González, 2022; Romero et al., 2022) and conference abstracts (Geersen et al., 2023; Miranda-Hardisson et al., 2023; Rodríguez-Losada et al., 2023; Walter et al., 2023) addressing flank instability or collapse at La Palma increased during the 19 months following the eruption. In contrast, only three references were published during the equivalent preceding period (Abadie et al., 2020; Arnaud et al., 2021; Fernández et al., 2021).
The increased interest in flank instability stems from the perception that marine volcano collapses are more prone to occur during or after an eruption, rather than during non-eruptive periods. However, to our knowledge, there is no evidence in the existing literature to support this link. It is crucial to understand the origin of this idea, as it may lead to the formation of a flawed paradigm with the potential for erroneous conclusions and devastating consequences, as demonstrated by the Tohoku-oki and Fukushima catastrophe (Lacassin and Lavelle, 2016). Therefore, the first objective of this perspective paper is to identify why erupting volcanoes may be perceived as more susceptible to collapse. The second objective is to examine whether collapses require an eruption and, consequently, whether eruptive activity could serve as an indicator of potential collapse at marine volcanoes. To achieve this, we conduct a comprehensive analysis of the available literature on historical marine volcano collapses, examining the sequence of eruptive activities and collapses, as well as additional factors influencing volcano stability. Additionally, we explore how uncertainties in historical and geological records, along with human risk perception, can challenge this apparent assumption.
2 Terminology and geological background
We hereafter use the generic terms “volcano collapse” or simply “collapse” to describe both sector or lateral collapses, that affect the core, conduit, or summit of the volcanic edifice and flank collapses that only affect a smaller portion of the flank. The term “marine volcano” incorporates both coastal and island volcanoes. Therefore, we refer to all tsunamis caused by slope instability at volcanoes as volcano collapse tsunamis.
Keating and McGuire (2000) have identified 23 preconditioning factors which promote edifice instability in marine environments. These factors can be divided into two categories: endogenetic (intra-edifice) and exogenetic (extra-edifice) factors. Endogenetic processes are associated with unstable foundations such as pelagic clays and rubble zones, decollement surfaces linked to intrusions, thermal alteration, edifice pore pressure and faulting (Figure 2). Exogenetic failures are associated with mechanically weak zones in the edifice (for example, caused by hydrothermal alteration), steep slopes, overloaded flanks, earthquakes, tilting, uplift, ice/snow coverage, rapid climate change and extreme climatic events, and weathering (Figure 2). While endogenetic failures are more significant during the constructive stages of volcanism, exogenetic failures continue throughout the history of volcanic islands (Keating and McGuire, 2000). The preconditioning state of marine volcanoes is vital, and a critical threshold is eventually reached when the driving force on the rock mass exceeds the resisting force of the zone of weakness, resulting in major slope failures.
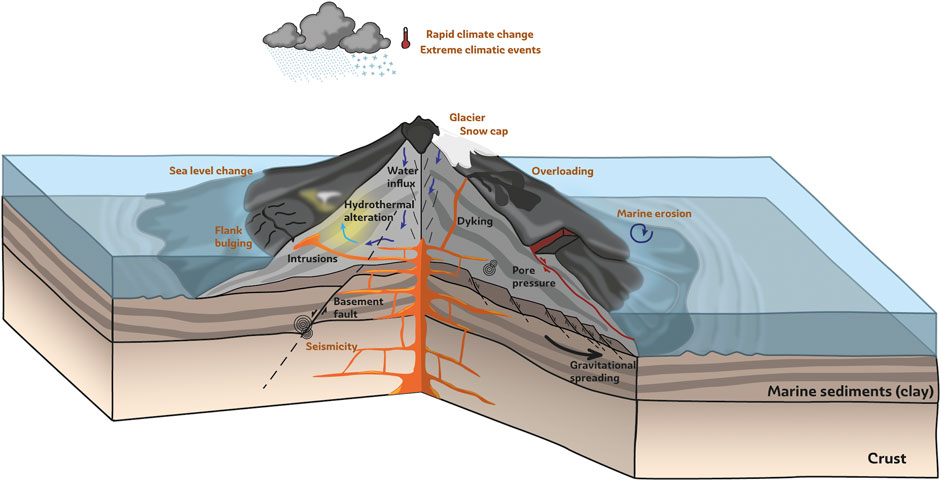
FIGURE 2. Cartoon (not to scale) illustrating the structures of oceanic volcanoes along with the different endogenetic (in black) and exogenetic (in brown) preconditioning factors of volcano collapse (based on McGuire, 1996; Keating and McGuire, 2000).
In most cases, a final trigger or a combination of triggers can also set the rock mass in motion, which may be related to or independent of the preconditioning factors. Triggers are typically short-lived dynamic events that suddenly cause a system in a static equilibrium to collapse (Voight and Elsworth, 1997). These triggers are either 1) rapid stress changes caused by new magma body propagating towards the surface (e.g., Belousova and Belousov, 1995; Siebert et al., 1995), or 2) an increase in ground accelerations induced by volcanic or tectonic earthquakes (e.g., Endo et al., 1989; Inoue, 2000; Schaefer et al., 2013). Capra (2006) proposed that climatic changes could also trigger volcano collapses, but due to the inherent uncertainties in deposit dating and climate fluctuations, establishing a direct cause-and-effect relationship is more challenging than demonstrating the potential role of climate as a destabilizing factor.
3 Data and results
We identified nine volcano collapses that occurred from 1600 onwards and generated tsunamis, by comparing Volcanic Debris Avalanche Deposits (VDAD, Day et al., 2015; Dufresne et al., 2021) and volcanic tsunami (Paris et al., 2014; Day, 2015; Paris, 2015) databases. Due to insufficient documentation, the volcano landslides from 1966 and 1971 at Tinakula volcano (in Solomon Island) were not included.
The earliest historical collapse occurred at Hokkaido Komagatake, an andesitic stratovolcano in northern Japan. Geological studies show that after 5,000 years of dormancy, new magma intruded into the volcanic edifice in 1640, followed by two sector collapses in different directions: one purely subaerial (Onuma lobe) with ∼0.3 km3 and one mobilizing both subaerial and submarine parts of the volcano (Shikabe lobe) with volumes ranging from 1.42 to 1.70 km3. The second collapse was accompanied by a blast and a tsunami (maximum local wave run-up ∼8 m), followed by a plinian eruption (Yoshimoto and Ui, 1998; Yoshimoto et al., 2007; Furukawa et al., 2008).
At Oshima-Oshima, a volcanic island southwest of Hokkaido in the Japan Sea, geological studies and historical documents indicate that after 1,500 years of dormancy, the volcano experienced activity between 1741 and 1790 (Katsui and Yamamoto, 1981). A few days after the eruption started on 18 August 1741 the most violent phase of the eruption was associated with a sector collapse of the north flank on August 29 (total of 2.4 km3 from subaerial and submarine part of the volcano) triggering a destructive tsunami (run-up
The deadliest recorded volcano collapse tsunami (Auker et al., 2013) occurred on 21 May 1792, when the southern lava dome (subaerial, 0.34 km3) of Mayu-yama volcano, part of the Unzen volcanic complex, collapsed (Ozeki et al., 2005). The subaerial volcanic debris avalanche entered the sea, and generated a tsunami that struck nearby areas (maximum local run-up
Augustine volcano in the Aleutians is a complex of overlapping summit lava domes with repeated major edifice collapses through geological times, which have produced large volcanic debris avalanches and related tsunamis (Siebert et al., 1995). The most recent one took place in August 1883 with few precursors indicating the ongoing phreatic eruption. While the duration of the 1883 eruption is uncertain, the paroxysmal phase was marked by a collapse of the upper edifice on 6 October 1883 mobilizing a volume of 0.3 km3 from the subaerial part of the volcano. It generated a tsunami with a run-up of 7–9 m at nearby Port-Graham (Siebert et al., 1995).
In Papua New Guinea, Ritter Island stratovolcano was known to be frequently active when on 13 March 1888 a collapse disintegrated about 2.4 km3 of its cone, initiating a tsunami with run-up waves
Paluweh (Rokatenda) volcano in the Lesser Sunda Islands is formed by overlapping craters and several lava domes. On 4 August 1928, an eruption occurred after almost 300 years of quiescence, which was accompanied by a significant landslide (unconstrained volume) that triggered a tsunami (run-up ranging from 5 to 10 m, Latter, 1981; Day et al., 2015). However, there is no evidence indicating whether the eruption or the collapse occurred first.
On 8 January 1933 a collapse (0.5 km3) occurred at Kharimkotan (Harimkotan) stratovolcano in the northern Kuriles, followed by a strong explosive eruption (Belousova and Belousov, 1995). Following the collapse, a tsunami was triggered with a maximum local run-up reaching 20 m. Belousov et al. (2007) has suggested that the magma was deeper at the moment of collapse, such that no lateral blasts were generated.
In 1979, the southern flank (subaerial) of Iliwerung volcano collapsed (0.05 km3), although the volcano was not erupting. This collapse resulted in tsunami waves with a maximum run-up of less than 10 m (Lassa, 2009; Paris et al., 2014). Yudhicara et al. (2015) have shown that hydrothermal alteration of the rocks and soil in the geothermal environment was probably the trigger of this collapse (Figure 2).
Stromboli volcano in the Tyrrhenian Sea, Italy, is a highly active stratovolcano, displaying continuous Strombolian explosive activity for 2,000–5,000 years. This activity involves periodic ejection of bombs and lapilli from the volcanic vents, occasionally punctuated by significant or paroxysmal explosions (Calvari et al., 2021). In the Holocene, Stromboli volcano has experienced several collapses, with some reaching volumes of up to 1–2 km3 (Tibaldi, 2001). Despite involving only 0.02 km3 of material (aerial and submarine), the recent collapses in 2002 at Stromboli generated a tsunami with a maximum run-up of 10 m. These collapses occurred 2 days after the onset of an effusive eruption, following a period of extraordinary strombolian activity (Brusca et al., 2004; Acocella, 2021). The aerial collapse was directly observed, including pre-collapse helicopter surveys that helped understand the collapse mechanism. Di Traglia et al. (2023) determined that the collapse was triggered by a lateral magma intrusion, which exerted significant thrust at high altitude, resulting in the destabilization of the entire slope.
The most recent event occurred at Anak-Krakatau, a stratovolcano in the Sunda strait in Indonesia. Following an intense yet ordinary eruptive period of several months (Cutler et al., 2022), its southwestern flank -including the summit area-collapsed on 22 December 2018 (∼ 0.18–0.31 km3) generating a maximum tsunami run-up of 13 m. While it was unclear whether the magmatic activity was directly leading to the collapse (Cutler et al., 2022), long-term deformation records indicated lateral motion for over a decade before the event (Zorn et al., 2023).
4 Discussion
4.1 Why are erupting marine volcanoes perceived as more prone to collapse?
Among historical volcano collapses attributed to flank instabilities, four occurred on erupting volcanoes (Oshima-Oshima, Augustine, Stromboli and Anak-Krakatau), three took place during periods of quiescence that later led to renewed eruptions (Hokkaido Komagatake, Ritter Island and Kharimkotan), one occurred on a non-erupting volcano with potential eruptions at neighboring volcanoes (Mayu-yama), and one collapse occurred without an eruption (Iliwerung). The Paluweh volcano event lacks sufficient data for a definitive classification, but it is likely to align with either the first or second category. Overall, most historical collapses were accompanied by eruptions (9 out of 10, including eruptions at neighboring volcanoes), either preceding or following the collapse. Without considering the exact sequence of events, this observation could lead to the interpretation that collapses of marine volcanoes predominantly occur on actively erupting ones.
Our human comprehension of risk, which to some extent, relies on images and associations (Slovic et al., 2004), might contribute to perceiving erupting volcanoes as more prone to collapse. These images and associations are often influenced by media coverage, which tends to focus more on erupting volcanoes compared to non-erupting ones, potentially shaping our perception of the associated threat. Even experts may be vulnerable to various heuristics and biases, especially when data or knowledge limitations come into play and intuition or subjective assumptions are involved (e.g., Tversky and Kahneman, 1973; 1974; Kahneman et al., 1982).
We suggest that both historical events and human perception contribute to the closer consideration of actively erupting marine volcanoes. However, such observations and perceptions should not be used as the sole scientific evidence to draw conclusions, particularly regarding precursory signals or mitigation strategies.
4.2 Are eruptions reliable precursors to marine volcano collapses?
The historical collapses identified in this study emphasize the significant role of eruptions in destabilizing volcanoes. However, the fact that eruptions followed collapses in four (possibly five) cases indicates the influence of other factors in the initial destabilization process. In some cases, eruptions may even be a consequence rather than the trigger of the collapse. Indeed, the sudden pressure relief resulting from a collapse and the interaction of hot magma with water can promote intense explosive eruptions. This was likely the case at Tenerife, where at least three major caldera-forming eruptions were induced by volcano collapse (Hunt et al., 2018). Additionally, terrestrial records provide evidence of collapses and deformations unrelated to eruptions. For example, collapses at Mombacho and Socompa volcanoes have been linked to gravitational spreading facilitated by weak hydrothermally altered or pre-existing structural features (van Wyk de Vries and Francis, 1997; van Wyk de Vries et al., 2001). Similarly, the recent signals observed at the dormant Damavand volcano in northern Iran has been attributed to long-term, slow, gravity-driven deformation, potentially indicating gravitational spreading (Shirzaei et al., 2011). Therefore, eruptive activity alone is not a robust precursor for forecasting collapses at volcanoes.
4.3 Limitations
While existing historical collapses provide valuable insights into the role of eruptions and other contributing factors in destabilizing volcanoes, a lack of comprehensive data limits our complete understanding of these phenomena. Although it is possible to rely on human testimony for historic events, reconstructing the exact sequence of events for historical marine volcano collapses can be challenging due to scarcity or incompleteness of accounts, especially when collapses occur on uninhabited or remote volcanic islands such as Oshima-Oshima, Ritter Island, Augustine, and Kharimkotan. Only the collapse of Stromboli was extensively documented in near real-time (Di Traglia et al., 2023). Furthermore, identifying and accurately characterizing small events (
5 Conclusion
Investigations of historical marine volcano collapses, geological examples, and conceptual models of preconditioning factors and triggers reveal that collapses can occur on both erupting and non-erupting volcanoes, and at various stages before, during, or after eruptions. Nevertheless, limiting our view to historic events of collapses and associated tsunamis, which all (except one) occurred on apparently erupting volcanoes, and combine it with our human perception, which relies on images and associations, may lead us to perceive erupting marine volcanoes as more prone to collapse as non-erupting ones. This finding is significant as it cautions against adopting a paradigm that exclusively focuses on collapse hazards in actively erupting marine volcanoes. Such a paradigm could lead to inaccurate hazard assessments, as observed in the case of the Tohoku-oki earthquake and tsunami (Lacassin and Lavelle, 2016). Prior to that event, it was commonly believed that segmented subduction zones like the one in Japan could not generate Mw 9+ earthquakes due to the absence of previous observations. Consequently, earthquake and tsunami mitigation measures in Japan were designed accordingly, disregarding evidence of similarly sized tsunamis in geological records. The Tohoku-oki earthquake shattered this paradigm.
Given the growing population living in vulnerable areas and our increasing reliance on seafloor infrastructures we need to develop strategies for assessing marine volcano stability and identify robust precursors. These tasks require the integration of a diverse and multidisciplinary community to tackle the geomechanical, geometrical, and magmatic aspects of preconditioning factors and triggers of marine volcano collapses. While some precursors may be generalized across volcanoes (or possibly types of volcanoes), assessing the stability conditions requires case-by-case analyses. Successfully meeting these challenges requires a united and collaborative effort from the scientific community. This is possible and timely considering rapid advances in monitoring technologies and capabilities in modeling and data analyses.
Data availability statement
The dataset for LECZ delineations and population distribution in LECZ in 2015 can be found at https://doi.org/10.7927/d1x1-d702. Population density can be located at https://doi.org/10.7927/H49C6VHWßor the population predictions in 2060, dataset come from Neumann et al. (2015) and are available at https://doi.org/10.1371/journal.pone.0118571.s004. Volcanic debris avalanches deposits come from Blahůt et al. (2019) and are available at https://www.irsm.cas.cz/ext/giantlandslides.
Author contributions
MU conceptualized the study and acquired the funding. SF led the writing of the original draft and designed the figures with the support of EK and CB. All authors contributed to manuscript revision, read, and approved the submitted version.
Funding
This project has received funding from the European Research Council (ERC) under the European Union’s Horizon 2020 research and innovation program (grant agreement No. 948797).
Acknowledgments
We thank the PRE-COLLAPSE team for engaging and productive discussions. We would like to extend a special thanks to Jens Karstens for his valuable comments and suggestions and Emma Hadré for her support in reviewing the literature for Cumbre Vieja volcano. Additionally, we thank Sylvain Mayolle for his drawing skills and advise and Malcolm Sanders for his careful proofreading of this paper. Eventually, we would like to thank Chief Editor Valerio Acocella, Associate Editor Susanna Jenkins, and Alessandro Tibaldi for their comprehensive reviews, which greatly contributed to improving the manuscript. The figures in this manuscript were created using the Free and Open Source QGIS, as well as Adobe Illustrator.
Conflict of interest
The authors declare that the research was conducted in the absence of any commercial or financial relationships that could be construed as a potential conflict of interest.
Publisher’s note
All claims expressed in this article are solely those of the authors and do not necessarily represent those of their affiliated organizations, or those of the publisher, the editors and the reviewers. Any product that may be evaluated in this article, or claim that may be made by its manufacturer, is not guaranteed or endorsed by the publisher.
Supplementary material
The Supplementary Material for this article can be found online at: https://www.frontiersin.org/articles/10.3389/feart.2023.1130561/full#supplementary-material
References
Abadie, S., Paris, A., Ata, R., Le Roy, S., Arnaud, G., Poupardin, A., et al. (2020). La Palma landslide tsunami: Calibrated wave source and assessment of impact on French territories. Nat. Hazards Earth Syst. Sci. 20, 3019–3038. doi:10.5194/nhess-20-3019-2020
Acocella, V. (2021). Advances in Volcanology. Cham: Springer International Publishing. doi:10.1007/978-3-030-65968-4Volcano-tectonic processes
Arnaud, G. E., Krien, Y., Abadie, S., Zahibo, N., and Dudon, B. (2021). How would the potential collapse of the Cumbre Vieja volcano in La Palma canary islands impact the Guadeloupe islands? Insights into the consequences of climate change. Geosciences 11, 56. doi:10.3390/geosciences11020056
Auker, M. R., Sparks, R. S. J., Siebert, L., Crosweller, H. S., and Ewert, J. (2013). A statistical analysis of the global historical volcanic fatalities record. J. Appl. Volcanol. 2, 2. doi:10.1186/2191-5040-2-2
Bellotti, G., Di Risio, M., and De Girolamo, P. (2009). Feasibility of tsunami early warning systems for small volcanic islands. Nat. Hazards Earth Syst. Sci. 9, 1911–1919. doi:10.5194/nhess-9-1911-2009
Belousov, A., Voight, B., and Belousova, M. (2007). Directed blasts and blast-generated pyroclastic density currents: A comparison of the bezymianny 1956, Mount St helens 1980, and soufrière hills, Montserrat 1997 eruptions and deposits. Bull. Volcanol. 69, 701. doi:10.1007/s00445-006-0109-y
Belousova, M. G., and Belousov, A. B. (1995). Prehistoric and 1933 debris avalanches and associated eruptions of harimkotan volcano (kurile islands). Per Mineral. LXIV, 99–100.
Blahůt, J., Balek, J., Klimeš, J., Rowberry, M., Kusák, M., and Kalina, J. (2019). A comprehensive global database of giant landslides on volcanic islands. Landslides 16, 2045–2052. doi:10.1007/s10346-019-01275-8
Brusca, L., Inguaggiato, S., Longo, M., Madonia, P., and Maugeri, R. (2004). The 2002-2003 eruption of stromboli (Italy): Evaluation of the volcanic activity by means of continuous monitoring of soil temperature, CO 2 flux, and meteorological parameters: 2002-2003 eruption of stromboli (Italy). Geochem. Geophys. Geosystems 5. doi:10.1029/2004GC000732
Calvari, S., Giudicepietro, F., Di Traglia, F., Bonaccorso, A., Macedonio, G., and Casagli, N. (2021). Variable magnitude and intensity of strombolian explosions: Focus on the eruptive processes for a first classification scheme for Stromboli volcano (Italy). Remote Sens. 13, 944. doi:10.3390/rs13050944
Capra, L. (2006). Abrupt climatic changes as triggering mechanisms of massive volcanic collapses. J. Volcanol. Geotherm. Res. 155, 329–333. doi:10.1016/j.jvolgeores.2006.04.009
Center for International Earth Science Information Network - CIESIN - Columbia University and CUNY Institute for Demographic Research - CIDR - City University of New York (2021). Low elevation coastal zone (LECZ) urban-rural population and land area estimates.Version 3.
Center for International Earth Science Information Network - CIESIN - Columbia University (2018). Gridded population of the world, version 4 (GPWv4): Population density, revision 11.
Clare, M., Chaytor, J., Dabson, O., Gamboa, D., Georgiopoulou, A., Eady, H., et al. (2019). A consistent global approach for the morphometric characterization of subaqueous landslides. Geol. Soc. Lond. Spec. Publ. 477, 455–477. doi:10.1144/SP477.15
Cutler, K. S., Watt, S. F., Cassidy, M., Madden-Nadeau, A. L., Engwell, S. L., Abdurrachman, M., et al. (2022). Downward-propagating eruption following vent unloading implies no direct magmatic trigger for the 2018 lateral collapse of Anak Krakatau. Earth Planet. Sci. Lett. 578, 117332. doi:10.1016/j.epsl.2021.117332
Day, S., Carracedo, J., Guillou, H., and Gravestock, P. (1999). Recent structural evolution of the Cumbre Vieja volcano, La Palma, Canary Islands: Volcanic rift zone reconfiguration as a precursor to volcano flank instability?J. Volcanol. Geotherm. Res. 94, 135–167. doi:10.1016/S0377-0273(99)00101-8
Day, S. J. (2015). “Volcanic tsunamis,” in The encyclopedia of volcanoes (Elsevier), 993–1009. doi:10.1016/B978-0-12-385938-9.00058-4
Day, S., Llanes, P., Silver, E., Hoffmann, G., Ward, S., and Driscoll, N. (2015). Submarine landslide deposits of the historical lateral collapse of Ritter Island, Papua New Guinea. Mar. Petroleum Geol. 67, 419–438. doi:10.1016/j.marpetgeo.2015.05.017
De Girolamo, P., Di Risio, M., Romano, A., and Molfetta, M. (2014). Landslide tsunami: Physical modeling for the implementation of tsunami early warning systems in the Mediterranean Sea. Procedia Eng. 70, 429–438. doi:10.1016/j.proeng.2014.02.048
Di Traglia, F., Calvari, S., Borselli, L., Cassanego, L., Giudicepietro, F., Macedonio, G., et al. (2023). Assessing flank instability of Stromboli volcano (Italy) by reappraising the 30 December 2002 tsunamigenic landslides. Landslides. doi:10.1007/s10346-023-02043-5
Dufresne, A., Siebert, L., and Bernard, B. (2021). “Distribution and geometric parameters of volcanic debris avalanche deposits,” in Volcanic debris avalanches: From collapse to hazard. Editors M. Roverato, A. Dufresne, and J. Procter (Cham: Springer International Publishing), 75–90. Advances in Volcanology. doi:10.1007/978-3-030-57411-6_4
Endo, K., Sumita, M., Machida, M., and Furuichi, M. (1989). “The 1984 collapse and debris avalanche deposits of ontake volcano, central Japan,” in Volcanic hazards. Editor J. H. Latter (Berlin, Heidelberg: Springer Berlin Heidelberg), 1, 210–229. doi:10.1007/978-3-642-73759-6_14
Fernández, J., Escayo, J., Camacho, A. G., Palano, M., Prieto, J. F., Hu, Z., et al. (2022). Shallow magmatic intrusion evolution below La Palma before and during the 2021 eruption. Sci. Rep. 12, 20257. doi:10.1038/s41598-022-23998-w
Fernández, J., Escayo, J., Hu, Z., Camacho, A. G., Samsonov, S. V., Prieto, J. F., et al. (2021). Detection of volcanic unrest onset in La Palma, Canary Islands, evolution and implications. Sci. Rep. 11, 2540. doi:10.1038/s41598-021-82292-3
Furukawa, R., Nanayama, F., and Yoshimoto, M. (2008). “Volcanic debris-avalanche as a cause of a historic tsunami: The A.D. 1640 eruption of the hokkaido-komagatake volcano, northern Japan,” in Solutions to coastal disasters 2008 (Turtle Bay, Oahu, Hawaii, United States: American Society of Civil Engineers), 235–247. doi:10.1061/40978(313)22
Geersen, J., Gross, F., Krastel, S., Böttner, C., Sánchez-Guillamón, O., Vázquez, J.-T., et al. (2023). Volcano-tectonic deformation of the submarine flank of Cumbre Vieja volcano, La Palma, Canary Islands. Vienna, Austria: EGU General Assembly 2023, EGU23-8954. doi:10.5194/egusphere-egu23-8954
Giachetti, T., Paris, R., Kelfoun, K., and Ontowirjo, B. (2012). Tsunami hazard related to a flank collapse of Anak krakatau volcano, Sunda strait, Indonesia. Geol. Soc. Lond. Spec. Publ. 361, 79–90. doi:10.1144/SP361.7
González, P. J., Tiampo, K. F., Camacho, A. G., and Fernández, J. (2010). Shallow flank deformation at Cumbre Vieja volcano (Canary Islands): Implications on the stability of steep-sided volcano flanks at oceanic islands. Earth Planet. Sci. Lett. 297, 545–557. doi:10.1016/j.epsl.2010.07.006
González, P. J. (2022). Volcano-Tectonic control of Cumbre Vieja. Science 375, 1348–1349. doi:10.1126/science.abn5148
Grilli, S. T., Tappin, D. R., Carey, S., Watt, S. F. L., Ward, S. N., Grilli, A. R., et al. (2019). Modelling of the tsunami from the december 22, 2018 lateral collapse of Anak krakatau volcano in the Sunda straits, Indonesia. Sci. Rep. 9, 11946. doi:10.1038/s41598-019-48327-6
Hunt, J. E., Cassidy, M., and Talling, P. J. (2018). Multi-stage volcanic island flank collapses with coeval explosive caldera-forming eruptions. Sci. Rep. 8, 1146. doi:10.1038/s41598-018-19285-2
Inoue, K. (2000). Shimabara-Shigatusaku earthquake and topographic changes by Shimabara catastrophe in 1792. Geogr. Rep. Tokyo Metropolitan Univ. 35, 59–69. doi:10.11475/sabo1973.52.4_45
D. Kahneman, P. Slovic, and A. Tversky (Editors) (1982). Judgment under uncertainty: Heuristics and biases (Cambridge: Cambridge University Press). doi:10.1017/CBO9780511809477
Karstens, J., Berndt, C., Urlaub, M., Watt, S. F., Micallef, A., Ray, M., et al. (2019). From gradual spreading to catastrophic collapse – reconstruction of the 1888 Ritter Island volcanic sector collapse from high-resolution 3D seismic data. Earth Planet. Sci. Lett. 517, 1–13. doi:10.1016/j.epsl.2019.04.009
Katayama, N. (1974). “Old records of natural phenomena concerning the “Shimabara Catastrophe”,” in Sci Rep Shimabara Volcano Obs Kyushu Univ Facul Sci. 9, 1–45.
Katsui, Y., and Yamamoto, M. (1981). The 1741-1742 activity of oshima-oshima volcano, north Japan. Jour. Fac. Sci. 19, 527–536.
Keating, B. H., and McGuire, W. (2000). Island edifice failures and associated tsunami hazards. Pure Appl. Geophys. 157, 899–955. doi:10.1007/s000240050011
Lacassin, R., and Lavelle, S. (2016). The crisis of a paradigm. A methodological interpretation of Tohoku and Fukushima catastrophe. Earth-Science Rev. 155, 49–59. doi:10.1016/j.earscirev.2016.01.013
Lassa, J. (2009). Bencana Yang Terlupakan? Mengingat Kembali Bencana Larantuka dan Lembata 1979–2009 (The Forgotten Disasters? Remembering The Larantuka and Lembata Disaster 1979–2009). J. NTT Stud. 1 (2), 159–184.
Latter, J. H. (1981). Tsunamis of volcanic origin: Summary of causes, with particular reference to Krakatoa, 1883. Bull. Volcanol. 44, 467–490. doi:10.1007/BF02600578
McGuire, W. J. (1996). Volcano instability: A review of contemporary themes. Geol. Soc. Lond. Spec. Publ. 110, 1–23. doi:10.1144/GSL.SP.1996.110.01.01
Miranda-Hardisson, A., González de Vallejo, L., Rodríguez-Losada, J. A., Hernández-Gutiérrez, L., de Villepreux, E., Álvarez-Hernández, A., et al. (2023). Stability analysis of the Tajogaite volcano slopes and lahar hazards for reconstruction and land planning in the affected areas, La Palma, Canary Islands. Vienna, Austria: EGU General Assembly 2023, EGU23-6926. doi:10.5194/egusphere-egu23-6926
Moore, J., Albee, W., Lipman, P., and Mullineaux, D. (1981). “Topographic and structural changes, march–july 1980—Photogrammetric data,” in The 1980 eruptions of Mount St. Helens (Washington). 1250, 123–134.
Neumann, B., Vafeidis, A. T., Zimmermann, J., and Nicholls, R. J. (2015). Future coastal population growth and exposure to sea-level rise and coastal flooding - a global assessment. PLOS ONE 10, e0118571. doi:10.1371/journal.pone.0118571
Ozeki, N., Okuno, M., and Kobayashi, T. (2005). Growth history of mayuyama, unzen volcano, kyushu, southwest Japan. Bull. Volcanol. Soc. Jpn. 50, 441–454. doi:10.18940/kazan.50.6_441
Paris, R. (2015). Source mechanisms of volcanic tsunamis. Philosophical Trans. R. Soc. A Math. Phys. Eng. Sci. 373, 20140380. doi:10.1098/rsta.2014.0380
Paris, R., Switzer, A. D., Belousova, M., Belousov, A., Ontowirjo, B., Whelley, P. L., et al. (2014). Volcanic tsunami: A review of source mechanisms, past events and hazards in southeast Asia (Indonesia, Philippines, Papua New Guinea). Nat. Hazards 70, 447–470. doi:10.1007/s11069-013-0822-8
Periáñez, R. (2021). Numerical simulation of the tsunami generated by a potential submarine landslide in La Palma (canary islands) after the september 2021 Cumbre Vieja eruption. Geosciences 11, 497. doi:10.3390/geosciences11120497
Rodríguez-Losada, J. A., Hernández-Gutiérrez, L. E., Miranda-Hardisson, A., González de Vallejo, L. I., Cervigón-Tomico, G., Díaz, H. d. l. R., et al. (2023). Evaluation of the slopes stability in the lava field of the 2021 Tajogaite eruption (La Palma, Canary Islands) in order to recover the territory for the development of new infrastructures. Vienna, Austria: EGU General Assembly 2023, EGU23-13809. doi:10.5194/egusphere-egu23-13809
Romero, J. E., Burton, M., Cáceres, F., Taddeucci, J., Civico, R., Ricci, T., et al. (2022). The initial phase of the 2021 Cumbre Vieja ridge eruption (Canary Islands): Products and dynamics controlling edifice growth and collapse. J. Volcanol. Geotherm. Res. 431, 107642. doi:10.1016/j.jvolgeores.2022.107642
Satake, K., and Kato, Y. (2001). The 1741 Oshima-Oshima Eruption: Extent and volume of submarine debris avalanche. Geophys. Res. Lett. 28, 427–430. doi:10.1029/2000GL012175
Schaefer, L. N., Oommen, T., Corazzato, C., Tibaldi, A., Escobar-Wolf, R., and Rose, W. I. (2013). An integrated field-numerical approach to assess slope stability hazards at volcanoes: The example of Pacaya, Guatemala. Bull. Volcanol. 75, 720. doi:10.1007/s00445-013-0720-7
Shirzaei, M., Walter, T., Nankali, H., and Holohan, E. (2011). Gravity-driven deformation of Damavand volcano, Iran, detected through InSAR time series. Geology 39, 251–254. doi:10.1130/G31779.1
Siebert, L., Begét, J. E., and Glicken, H. (1995). The 1883 and late-prehistoric eruptions of Augustine volcano, Alaska. J. Volcanol. Geotherm. Res. 66, 367–395. doi:10.1016/0377-0273(94)00069-S
Siebert, L., Glicken, H., and Ui, T. (1987). Volcanic hazards from bezymianny- and bandai-type eruptions. Bull. Volcanol. 49, 435–459. doi:10.1007/BF01046635
Slovic, P., Finucane, M. L., Peters, E., and MacGregor, D. G. (2004). Risk as analysis and risk as feelings: Some thoughts about affect, reason, risk, and rationality. Risk Anal. 24, 311–322. doi:10.1111/j.0272-4332.2004.00433.x
Tibaldi, A. (2001). Multiple sector collapses at stromboli volcano, Italy: How they work. Bull. Volcanol. 63, 112–125. doi:10.1007/s004450100129
Tversky, A., and Kahneman, D. (1973). Availability: A heuristic for judging frequency and probability. Cogn. Psychol. 5, 207–232. doi:10.1016/0010-0285(73)90033-9
Tversky, A., and Kahneman, D. (1974). Judgment under uncertainty: Heuristics and biases. Science 185, 1124–1131. doi:10.1126/science.185.4157.1124
van Wyk de Vries, B., and Francis, P. (1997). Catastrophic collapse at stratovolcanoes induced by gradual volcano spreading. Nature 387, 387–390. doi:10.1038/387387a0
van Wyk de Vries, B., Self, S., Francis, P., and Keszthelyi, L. (2001). A gravitational spreading origin for the Socompa debris avalanche. J. Volcanol. Geotherm. Res. 105, 225–247. doi:10.1016/S0377-0273(00)00252-3
Voight, B., and Elsworth, D. (1997). Failure of volcano slopes. Géotechnique 47, 1–31. doi:10.1680/geot.1997.47.1.1
Voight, B., Glicken, H., Janda, R., and Douglass, M. (1981). Catastrophic rockslide avalanche of may 18 (Mount St. Helens). U.S. Geol. Surv. Prof. Pap. 1250, 347–377.
Walter, T. R., Haghshenas Haghighi, M., Schneider, F. M., Coppola, D., Motagh, M., Saul, J., et al. (2019). Complex hazard cascade culminating in the Anak Krakatau sector collapse. Nat. Commun. 10, 4339. doi:10.1038/s41467-019-12284-5
Walter, T. R., Zorn, E., Gonzalez, P., Sansosti, E., Munoz, V., Shevchenko, A., et al. (2023). “Late complex tensile fracturing interacts with topography at Cumbre Vieja, La Palma,” in EGU 2023 (copernicus meetings). doi:10.5194/egusphere-egu23-3344
Watt, S. F., Karstens, J., Micallef, A., Berndt, C., Urlaub, M., Ray, M., et al. (2019). From catastrophic collapse to multi-phase deposition: Flow transformation, seafloor interaction and triggered eruption following a volcanic-island landslide. Earth Planet. Sci. Lett. 517, 135–147. doi:10.1016/j.epsl.2019.04.024
Yoshimoto, M., Takarada, S., and Takahashi, R. (2007). Eruptive history of Hokkaido-Komagatake volcano, northern Japan. J. Geol. Soc. Jpn. 113, S81–S92. doi:10.5575/geosoc.113.S81
Yoshimoto, M., and Ui, T. (1998). The 1640 sector collapse of Hokkaido Komagatake volcano, northern Japan. Bull. Volcanol. Soc. Jpn. 43, 137–148. doi:10.18940/kazan.43.4_137
Yudhicara, Y., Bani, P., and Darmawan, A. (2015). Geothermal system as the cause of the 1979 landslide tsunami in lembata island, Indonesia. Indonesian J. Geoscience 2, 91–99. doi:10.17014/ijog.2.2.91-99
Keywords: coastal and oceanic volcanoes, volcanic unrest, hazards, warning system, volcano collapse
Citation: Furst S, Urlaub M, Klein E and Bonanati C (2023) Are eruptions reliable precursors to marine volcano collapses?. Front. Earth Sci. 11:1130561. doi: 10.3389/feart.2023.1130561
Received: 23 December 2022; Accepted: 12 July 2023;
Published: 03 August 2023.
Edited by:
Susanna Jenkins, Nanyang Technological University, SingaporeReviewed by:
Alessandro Tibaldi, University of Milano-Bicocca, ItalyCopyright © 2023 Furst, Urlaub, Klein and Bonanati. This is an open-access article distributed under the terms of the Creative Commons Attribution License (CC BY). The use, distribution or reproduction in other forums is permitted, provided the original author(s) and the copyright owner(s) are credited and that the original publication in this journal is cited, in accordance with accepted academic practice. No use, distribution or reproduction is permitted which does not comply with these terms.
*Correspondence: Séverine Furst, c2Z1cnN0QGdlb21hci5kZQ==