- 1Geological Survey of Canada, Natural Resources Canada, Calgary, AB, Canada
- 2GNS Science, Lower Hutt, New Zealand
- 3Department of Geoscience, University of Calgary, Calgary, AB, Canada
For the first time, polar organic compounds in extracted bitumen from the Lower Triassic Montney Formation have been analyzed. This stratigraphic unit is one of the most prolific low-permeability reservoirs in Western Canada. However, its organic geochemical characterization is a challenge due to low biomarker concentration in the liquids and frequent mixing of gas/condensate hydrocarbons. Since typical biomarkers were not available, this study focused on another group of molecules, polar oxygen-compounds, which were derivatized and subsequently resolved using chromatographic techniques. In the polar fractions, based on their contrasting molecular distributions, n-fatty acids and alkan-2-ones do not seem to share a common origin and do not have an apparent association with the n-alkanes. This study is also the first report of 1,13-, 1,14- and 1,15-diols in fossil organic matter; and in carbon number ranges of C15-C29, C16-C29 and C17-C29 respectively. The similar distributions of 1,14- 1,15-diols suggests a common origin for these compound classes, whereas theand 1,13-diols seem to derive from a different source or mechanism. A series of alkan-3-ols has also been identified in the C12-C28 range, sharing a common distribution pattern with the n-fatty acids. The large variability detected in the molecular distribution of oxygen-containing aliphatic compounds introduces the question whether they may record a geochemical signature that precedes thermal degradation and hydrocarbon migration events within Montney reservoirs.
1 Introduction
In the Western Canadian Sedimentary Basin, a significant amount of petroleum resources is currently produced from the Lower Triassic Montney Formation (unconventional estimated reserves at 12,719 billion m3 of natural gas, 14,521 million barrels of natural-gas-liquids, and 1,125 million barrels of oil, BC Oil and Gas Commission, 2012; Rokosh et al., 2012). Hydrocarbons in the Montney Formation are thought to originate from thermal degradation of migrated oil. This is based on the fact that the organic matter in Montney mostly consists of pore-filling solid bitumen (Sanei et al., 2015; Wood and Sanei, 2016; Wood et al., 2018). Maximum burial and thermal maturity reached in the Late Cretaceous/Early Paleogene caused thermal cracking of accumulated hydrocarbons which led to the petroleum accumulations we know today (Wood and Sanei, 2016; Ducros et al., 2017; Euzen et al., 2021). Primary organic matter has also been identified within Montney (Ardakani et al., 2022). However, the relative contribution from in-situ kerogen to the petroleum accumulations remains unknown. Source rock candidates include the Middle Triassic Doig Formation, the Lower Jurassic Gordondale Member of the Fernie Formation, and the Montney Formation (Alan and Creaney, 1991; Ejezie, 2007; Euzen et al., 2021). Nonetheless, fluid to source correlations to date (e.g., based on biomarkers) continue to be rather inconclusive.
Recent studies on gas hydrocarbons have facilitated the definition of hydrocarbon play boundaries within Montney (e.g., Cesar et al., 2020b; Cesar et al., 2021), as well as potential gas migration pathways (Wood and Sanei, 2016; Euzen et al., 2021; Wood et al., 2022) using a molecular approach. The overall geochemical signature of produced gases from these fields seems to be controlled by thermal maturity effects and fluid mixing due to migration (Cesar et al., 2020a; Cesar et al., 2021; Cesar et al., 2022; Wood et al., 2022). As a result, gas geochemical tools alone have not been successful discriminators for the identification of the source(s) of hydrocarbons.
In the liquid phase, geochemical assessment has been limited by the low abundance of biomarkers (e.g., hopanes and steranes) in the produced condensates and light oils. In the Montney Formation, little has been studied regarding the geochemistry of light hydrocarbons, which often cannot be detected in rock extracts due to volatility loss during sample handling/preparation, limiting fluid to source correlation. Research on condensate samples has suggested the possibility of mixtures of migrated hydrocarbons with hydrocarbons derived from original kerogen of the Montney Formation (Cesar et al., 2020a).
In contrast to molecules in the gas-condensate range, there is a group of compounds that have not been analyzed yet, these are polar compounds in the resin fraction of soluble bitumen. It is known that resins are scarce (or absent) in the produced condensates and light oils. However, they are preserved in the solid bitumen residue within the reservoir, and potentially record a fingerprint of the original source of migrated hydrocarbon that charged Montney productive intervals. At the same time, these polar compounds can be a source of gas/condensate, particularly when thermal alteration (cracking) takes place (e.g., Michels et al., 2000) as is the case of the Montney Formation.
In this study, and for the first time, we characterize polar compounds of extracted bitumen from the Montney Formation in order to 1) identify the main oxygen-aliphatic compound classes, 2) investigate associations between them, and 3) discuss their potential as source discriminators.
2 Geological setting
The Montney Formation (Figure 1A) deposited in the northwestern margin of the North American craton during the Lower Triassic (Davies, 1997; Davies et al., 2018; Zonneveld and Moslow, 2018) in a mixed siliciclastic-carbonate depositional environment that accumulated shoreface to offshore, and turbidite sediments (Davies, 1997; Davies et al., 2018; Zonneveld and Moslow, 2018). The lithology includes a complex succession of dolomitic siltstone, minor component of very fine-to fine-grained sandstone with local bioclastic carbonate rocks and minor shale intervals (Davies, 1997; Davies et al., 2018; Zonneveld and Moslow, 2018).
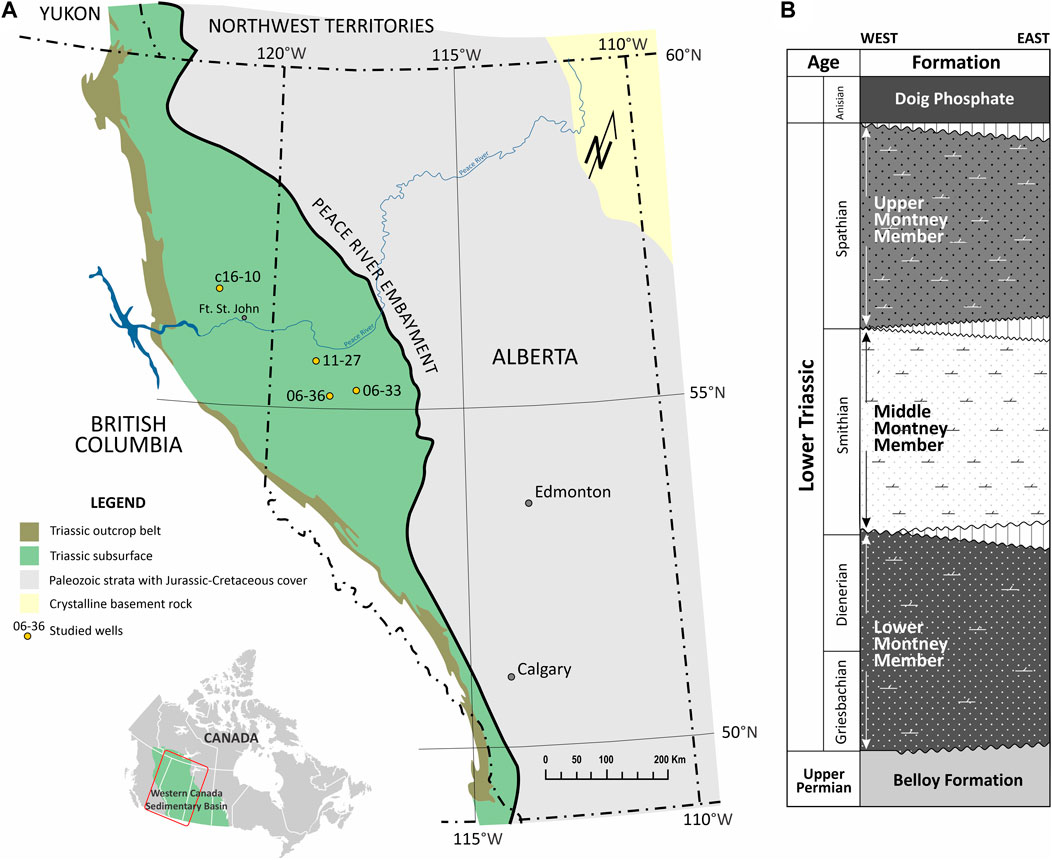
FIGURE 1. Geological context indicating (A) the Montney Formation and location of studied wells (modified after Ardakani et al., 2022), and (B) a summarized stratigraphic column (after Zonneveld and Moslow, 2018).
The Montney Formation is divided into three main members (Davies, 1997; Davies et al., 2018; Zonneveld and Moslow, 2018). The Lower Member corresponds to multi-cyclic very fine-grained sandstone and dolomitic siltstone. It overlies the Triassic unconformity on Permian and/or older strata, and it is overlain by the base of the Middle Member in the east (where present), and by the Upper Member in the west. The Middle Member consists of a thick succession of bituminous dolomitic siltstone with interbedded very-fine grained sandstone. It overlies the Lower Member and is marked by a boundary of reworked clasts of the underlying units. The Upper Member corresponds to multicyclic, coarsening-up siltstones and very fine sandstones dominated by storm-related fabrics, with local dolomitized coquina facies; and it is overlain by the Doig Formation. The Upper Member is primarily seen in the British Columbia section (Supplementary Figure S1). In addition, the Montney stratigraphic succession deeps towards the southwest, thus the sections in British Columbia are often more mature than those from Alberta (BC Oil and Gas Commission, 2012; Rokosh et al., 2012). The general schematic stratigraphy is illustrated in Figure 1B.
3 Materials and methods
3.1 Sample preparation
A total of 15 powdered and homogenized rock samples were solvent-extracted using dichloromethane (DCM) in a Soxhlet apparatus for 24 h. The general characteristics of the samples are listed in Table 1. The samples selected for this study correspond to core intervals from the different stratigraphic Members (Lower, Middle, and Upper), different locations, and different thermal maturity levels as indicated by rock pyrolysis (Tmax) and aromatic ratios (Table 1).
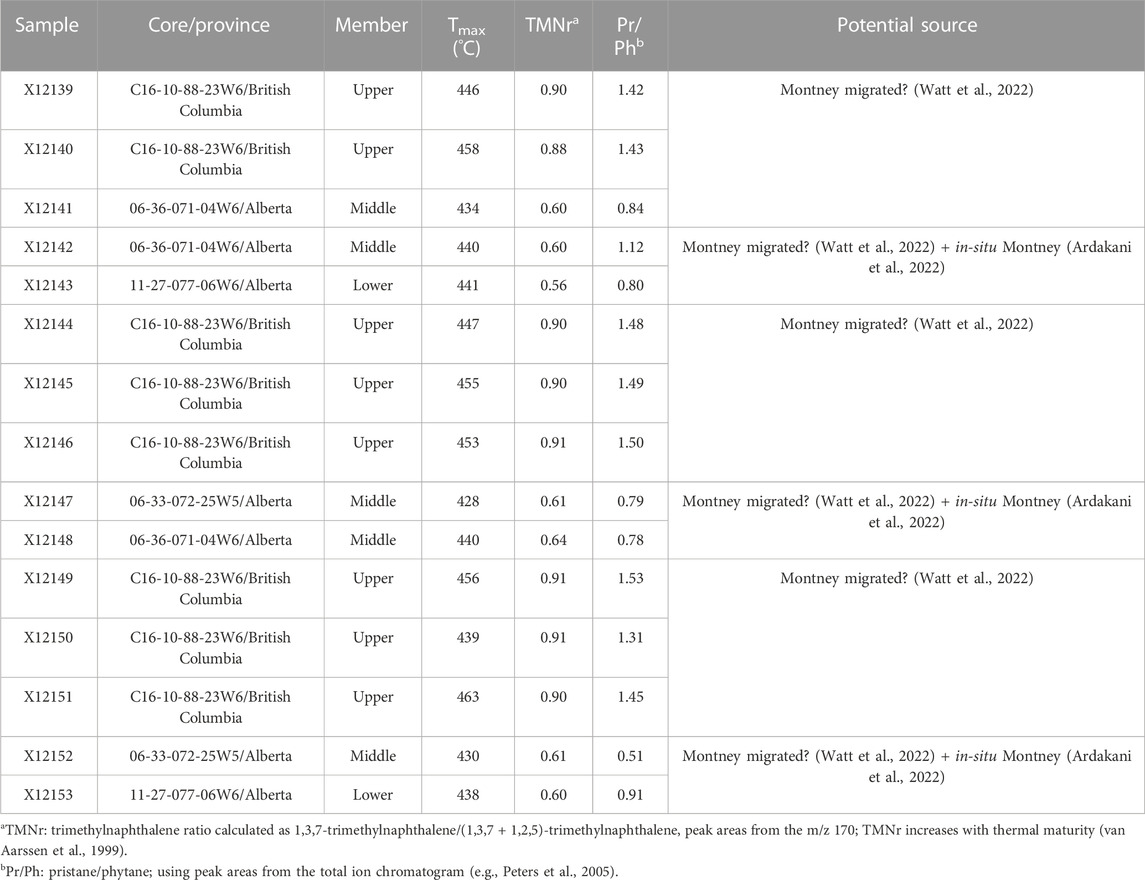
TABLE 1. Montney Formation core rock samples included in this study. Core locations shown in Figure 1A.
Polar fractions were obtained from the extracted bitumen using column chromatography by elution with methanol once saturate and aromatic hydrocarbon fractions had been eluted with n-pentane and DCM:n-pentane, respectively. Aliquots of the polar fractions (0.5–1 mg) were derivatized in 200 μL of pyridine and 300 μL of N,O-Bis(trimethylsilyl) trifluoroacetamide (BSTFA) at 70°C for 1 h. Then, the derivatized fractions were dried under a gentle stream of nitrogen at 35°C and re-dissolved in n-hexane for molecular analysis as explained below. Procedural blanks were prepared and analyzed to monitor for any contamination during sample preparation, and instrument blanks and standards were run to check for instrument carry over or any other instrumental anomalies. Nonadecanol was used as internal standard.
3.2 Molecular analysis
Derivatized fractions were analyzed via gas chromatography time-of-flight mass spectrometry (GC–QTOF-MS) on an Agilent 7890BGC - 7200 QTOF mass spectrometer equipped with a DB5-ms column (60 m × 0.25 μm i.d. × 0.25 mm f.t.), using splitless injection and helium as carrier gas (1.3 mL/min). The temperature program of the GC oven started at 80°C for 1 min, then ramped at 5°C/min to 325°C and held at the final temperature for 30 min. Compound identification was carried out using relative retention times and characteristic mass spectra.
The distribution of n-alkanes in the saturate fractions were determined using GC with flame ionization detection (FID) analysis of the saturated hydrocarbon fractions on an Agilent 7890B GC-FID. A 30 m × 0.25 mm × 0.25 μm DB-1 fused silica capillary column was used, with helium as carrier gas. The samples were injected using split injection with the injector temperature set at 300°C. The temperature program of the GC oven started at 60°C, then ramped at 6°C/min to 300°C for 30 min. The FID temperature was maintained at 300°C.
4 Results
4.1 Fatty acids
C15-C30 n-fatty acids (carboxylic acids) are present in all samples (Figure 2A, m/z 117) and typically dominated by C18-C20 (Figure 2A; Figure 3A). However, samples X12140 and X12143 have bimodal distributions with maximum abundance at C18-C19 and C22-C24 (Figures 3B, D). Samples X12141 and X12148 display unimodal distributions that are to some extent flat tops between C19 and C23 (Figure 3C).
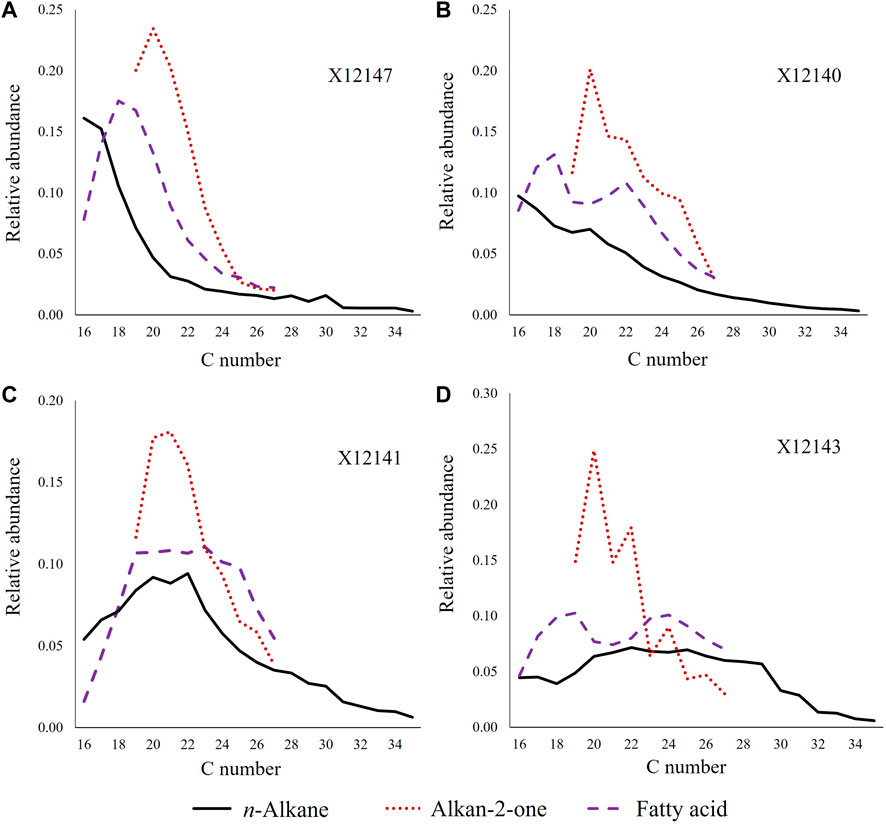
FIGURE 3. Main distribution patterns observed in the n-fatty acids: (A) unimodal distribution, (B,D) bimodal distribution, (C) flatten top; all compared with the corresponding distribution of alkan-2-ones and n-alkanes.
4.2 Alkanones
Alkanones, or more specifically alkan-2-ones, were identified using the m/z 58 as shown in Figure 2B, usually in the C17-C27 carbon range. All the samples exhibit unimodal distributions with maximum abundance of C20 as illustrated in Figure 3. Predominance of even carbon numbered homologues was found in samples X12140, X12142, X12143, X12148, X12149, X12151, and X12152 (e.g., Figure 3D).
4.3 Diols
Figure 4 presents the diols detected in the studied samples, which include 1,13-diols (m/z 257), 1,14-diols (m/z 271), and 1,15-diols (m/z 285). These compound classes were identified in the ranges C15-C29, C16-C29, and C17-C29, respectively.
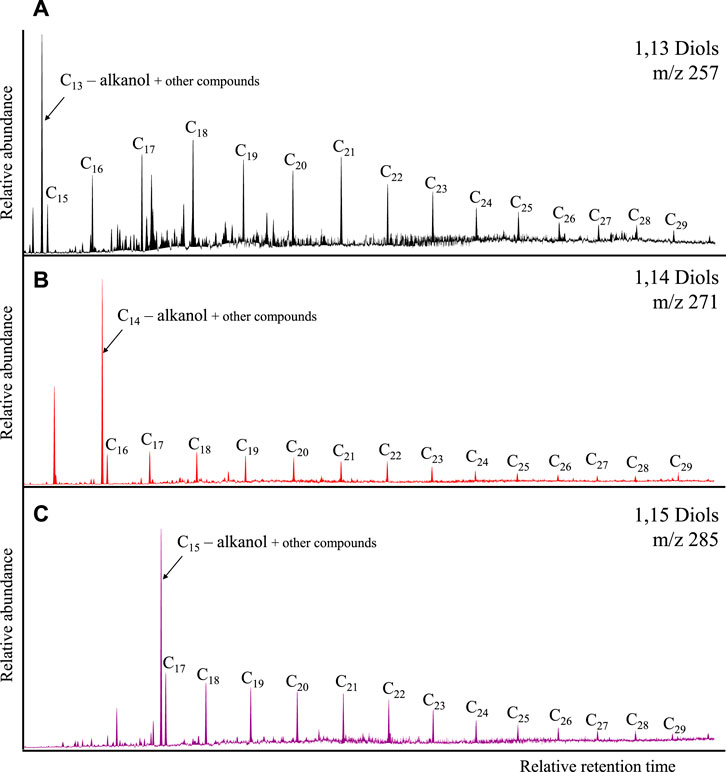
FIGURE 4. Diols identified in the samples (e.g., X12153): (A) 1,13-diols, (B) 1,14-diols, (C) 1,15-diols.
The main carbon number distribution patterns of the diols can be observed in Figure 5. The 1,14- and 1,15- diols (Figures 5B, C) generally decrease in abundance with increasing carbon number at variable slopes, except samples X12140 and X12143 which register higher abundances in the C20+ range. Another aspect to notice is the preference for odd carbon numbers in the 1,14-diols, particularly in the C20+ range (Figure 5B). The odd preference is less emphasized in the 1,15-diols yet observed in samples X12145, X12151 and X12152 (e.g., Figure 5C). The 1,13-diols exhibit a more variable molecular distribution though with a preference for C18 and odd carbon numbers after C20 (Figure 5A).
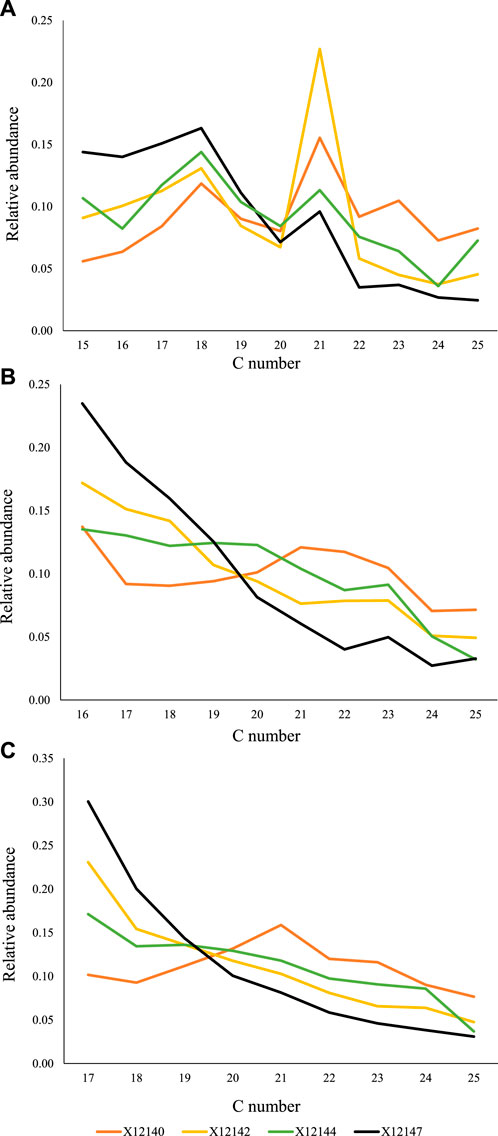
FIGURE 5. Selected samples to illustrate the main distribution patterns of (A) 1,13-diols, (B) 1,14-diols, and (C) 1,15-diols identified in the samples.
4.4 Alkan-3-ols
For this compound series (Figure 6A; Supplementary Figure S2), the chain length varied from C12 to C28 (sometimes C28+ visible). The distribution patterns were found to be very similar to those of the fatty acids (Figure 3, Figure 6B): 1) mixed/bimodal distribution (e.g., X12140, X12143), 2) flatten top (e.g., X12141, X12148), and 3) decreasing abundance with increasing carbon number after maximum peak (the rest of the samples). Certain preference for C20 and C22 was observed in samples X12140, X12142-X12146, and X12148-X12151.
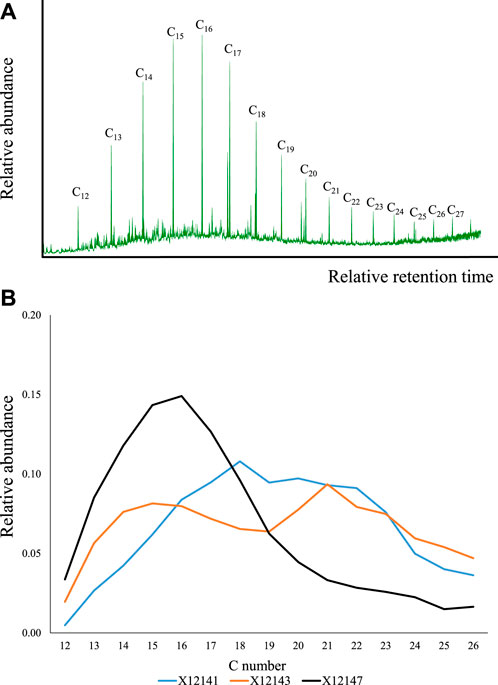
FIGURE 6. Alkan-3-ols identified in the samples. (A) The m/z 131 indicating carbon chain length, and (B) the main distribution patterns (see also, Supplementary Figure S2).
5 Discussion
5.1 The significance of fatty acids in fossil organic matter from the Montney Formation
In recent sedimentary organic matter, fatty acids have typically been attributed to algal and bacterial (C14-C20; Perry et al., 1979; Cranwell et al., 1987; Naeher et al., 2022), aquatic macrophytes (C22-C26; Cranwell et al., 1987; Volkman et al., 1999; Naeher et al., 2022) and terrestrial plant sources (C28-C32; Rieley et al., 1991; Freeman and Pancost, 2014; Naeher et al., 2022). It is also considered that terrestrial plants synthesize longer chain fatty acids preferably (Wang and Liu, 2012; Naafs et al., 2019). However, chain length alone is not always diagnostic of the source of these compounds, particularly in environments with aquatic and terrestrial organic matter inputs (Fang et al., 2014). As well, the molecular distribution of n-alkanes and n-fatty acids are expected to be similar when the n-alkanes derive from the decarboxylation of the acids during deposition/diagenesis (Barakat et al., 2000; Naafs et al., 2019).
In fossil organic matter (e.g., petroleum, bitumen), the evaluation of linear carboxylic acids is less common because of their degradation with thermal maturity and many other biomarkers being available for petroleum geochemistry studies (e.g., hopanes, steranes, aromatic markers, etc.). However, we must highlight the work by Jaffé and Gallardo (1990), Jaffé et al. (1992), Jaffé and Gallardo (1993), who suggested that most of the fatty acids identified in fossil organic matter are originally protected within more complex geo-polymer structures (later kerogen, bitumen) and released with increasing thermal maturity before the peak of oil generation. Free fatty acids, instead, would not be preserved but degrade to n-alkanes and/or other compounds during diagenesis. Therefore, opposite to recent environments, we may infer that in petroleum fluids and bitumen extracts from mature source rocks, the fatty acids should not be expected to have a distribution pattern similar to the n-alkanes as the latter would not necessarily be degradation products of the former (Jaffé and Gallardo, 1990; Jaffé et al., 1992; Jaffé and Gallardo, 1993).
This may explain why in our samples the distribution of n-fatty acids generally differs from the distribution of n-alkanes. For instance, samples X12140 and X12143, from completely different locations and stratigraphic intervals (Table 1; Figure 1), have a bimodal distribution pattern of fatty acids in common (Figures 2B, D). The same bimodality is not expressed in the n-alkanes distribution of these samples. It could be argued that a second group of fatty acids with highest peaks in the C22-C24 range (Figures 2B, D) represents an additional terrestrial organic matter input. However, additional evidence is required to confirm terrestrial contribution. As well, bimodal fatty acids distribution could simply result from hydrocarbon mixing before maximum burial of the Montney Formation. Post maximum burial migration events in Montney (e.g., during the Eocene uplift) consisted of gas/condensate which unlikely carried fatty acids due to insolubility of the latter.
It is important to mention that in sample X12143, primary organic matter was identified besides solid bitumen (Ardakani et al., 2022). Therefore, we cannot rule out the possibility of fatty acids bimodality resulting from the mixture of migrated hydrocarbons and in-situ organic matter.
Samples X12141 and X12148 are also peculiar because they showed a flatten-top distribution pattern of n-fatty acids, which is different to the n-alkanes from the same samples. These samples come from the same well (Table 1) and Middle Montney Member although 40 m apart from each other. At this stage, it is challenging to propose source variations attributed to a flatten profile, but these samples could also represent mixtures of multiple-sourced hydrocarbons.
In the rest of the samples, fatty acids abundances generally decrease with increasing carbon number after C18-C20 (Figure 2A; Figure 3A), similar to the n-alkanes. However, there is no obvious indication that the latter are degradation products of the fatty acids. For instance, if decarboxylation was taking place (loss of a COOH group), the highest peak in the n-alkane distribution should be displaced one carbon number less compared to the highest peak of the fatty acids, and that is not the case in any of the samples (see also Supplementary Table S4).
We must emphasize that comparisons between fatty acids and n-alkanes are also limited by the fact that the current distribution of n-alkanes in the Montney Formation has largely resulted from thermal cracking of migrated hydrocarbons during Montney’s maximum burial, and additional fluid migration/mixing during the Eocene uplift (Wood and Sanei, 2016; Cesar et al., 2020a; Wood et al., 2022).
It is also possible that the distribution of fatty acids is responding to localized organic facies variations. A larger number of samples and geochemical constraints will provide light on this matter. Likewise, biodegradation cannot be ruled out although thermal maturity might have overprinted the molecular distribution ultimately.
5.2 The significance of alkan-2-ones in fossil organic matter from the Montney Formation
Alkan-2-ones are commonly present in peat and peat-forming vegetation (C17-C35; Puttmann and Bracke, 1995; Zheng et al., 2007; Naafs et al., 2019). In addition, bacterial and algal sources have been found to generate these compounds in a variety of depositional settings (C14-C31; Grimalt et al., 1990; Lopez-Dias et al., 2013; Zhang and Volkman, 2020). Although extensively studied in recent environments, alkan-2-ones have been detected in fossil organic matter of different thermal maturities with a moderate (or none) odd over even predominance of carbon chain length (Grimalt et al., 1990; George and Jardine, 1994; Leif and Simoneit, 1995; Strelnikova and Serebrennikova, 2011; Jaraula et al., 2015). Similar to the case of fatty acids, the low concentration of ketones in mature petroleum source rocks compared to other biomarkers has probably reduced the interest in the analysis of these compounds in petroleum (Alhassan and Anderson, 2013).
Zhang and Volkman (2020) reported an even over odd predominance of alkan-2-ones in a torbanite from the Sydney Basin, Australia, similar to the C-number predominance in samples from our study. The Australian torbanite contains organic matter type I and has vitrinite reflectance of 0.4 %Ro. The same authors suggested that these compounds could have derived from cleavage of C-O bonds in the kerogen and indicated the presence of long-chain alkyl moieties in green algae (Derenne et al., 1997; Zhang and Volkman, 2017). Despite the difference in maturity, this example may explain the presence and marine origin of alkan-2-ones in Montney samples since there is no reported evidence of significant terrestrial organic matter input in the Montney Formation.
We rule out alkan-2-one generation via decarboxylation of fatty acids because of their different molecular distribution patterns. Decarboxylation would have led to alkan-2-ones with one less carbon atom, switching maximum peak heights towards lower carbon chains (Puttmann and Bracke, 1995; Lopez-Dias et al., 2013). Instead, the highest alkanone peak found in our samples corresponds to a carbon chain (C20) longer than the highest peaks in the fatty acid distribution (C18-C19) (Figure 2; Figure 3; Supplementary Tables S1, S2). In addition, oxidation of n-alkanes does not seem to be a potential generation pathway either because of the contrasting molecular distribution pattern of both compound classes (Figure 3, Supplementary Tables S2, S4).
5.3 The significance of alcohols in fossil organic matter from the Montney Formation
Contrary to the case of fatty acids and alkan-2-ones, to our knowledge, this is the first report ever of alkyl diols in fossil organic matter.
In recent environments, these lipids typically consist of even-numbered carbon chains with hydroxyl groups at C-13, C-14, or C-15, and 28 to 32 carbon atoms (e.g., Rodrigo-Gamiz et al., 2016; Rampen et al., 2021). In the studied samples, diol distributions are characterized by an odd-numbered carbon preference after C20, and shorter chain length (less than 30 carbon atoms). It is possible that the diols detected in this research are thermal breakdown products of the original long-chain diols, and the loss of a methyl group by thermal cleavage has switched the carbon length preference to odd numbers.
Generally, 1,14-diols are considered to be sourced by specific diatoms and are typically associated with upwelling zones (Koning et al., 2001; Wakeham et al., 2002; Damste et al., 2003), whereas 1,15-diols have been attributed to specific marine and freshwater algae (Volkman et al., 1992, 1999) in recent organic matter. However, in our sample set, 1,14- and 1,15- diols seem to share a common source (Figures 7A, B). This is interpreted based on their markedly similar distribution. In Figure 7A, we have plotted the ratios of the C16-C18 range to the C20-C22 range for both compound classes and they express an almost 1:1 correlation, with no significant thermal maturity overprint (Figure 7B). Their mutual association cannot be explained by their distribution in recent environments. It is possible that in the Mesozoic, 1,14- and 1,15- diols were synthesized by a common source/organism. The samples X12147 and X12152 stand out in Figure 7 with values [C16-C18]/[C20-C22] above three. They are the only two samples from the well 06-33-072-25W5 (Middle Montney, Table 1), and local organic matter type variations may be responsible for their uniqueness.
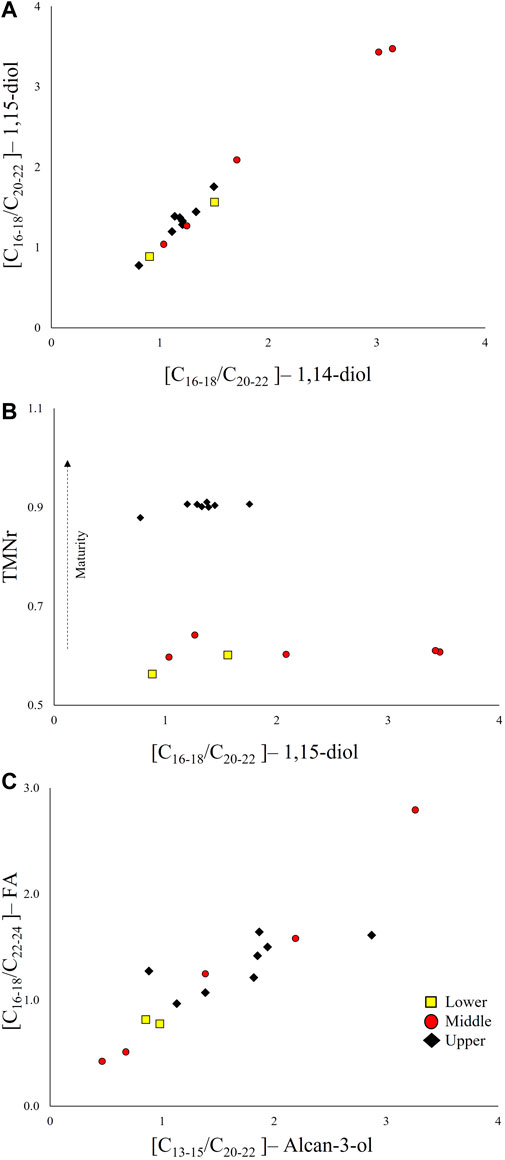
FIGURE 7. Relationship between selected alcohol parameters. (A) The [C16-18/C20-22]– 1,15-diol versus [C16-18/C20-22]– 1,14-diol, (B) TMNr versus [C16-18/C20-22]– 1,15-diol. (C) Relationship between alkan-3-ols and fatty acids expressed as the [C16-18/C22-24]–FA versus [C13-15/C20-22]–alkan-3-ol.
Contrary to 1,14-diols, 1,13-diols have been found to share a source with 1,15-diols in recent depositional environments (e.g., Rodrigo-Gamiz et al., 2016; Rampen et al., 2021). However, in this study, the distribution of 1,13-diols clearly differs from 1,14- and 1,15- diols (Figure 5A). Additionally, we noticed a marked preference for C211,13-diol with respect to C20 and C22 in most of the samples, and for C181,13-diol in sample X12152 (Figure 5A). Additional studies are necessary to investigate potential sources for the 1,13 homologues, but their origin most likely differ from the sources of 1,14- and 1,15- diols.
Another possibility is that the original distribution of alkyl-diols has simply been overprinted by other geochemical phenomena (e.g., mineral-catalyzed isomerization/cyclization, biodegradation), to which each compound class (1,13- versus 1,14- versus 1,15-) may have a different susceptibility. Under such condition, our interpretation of a common source for 1,14-diols and 1,15-diols would not necessarily be the case.
The presence of alkan-3-ols seems to be closely related to the fatty acids, probably sharing a common source since their molecular distributions are significantly similar (Figure 3; Figure 6; Figure 7C). To our knowledge, this research is also the first time that alkan-3-ols are reported in fossil organic matter. In recent environments, alkan-3-ols have only been identified in surface-wax washings of Primulaceae (Radulovic and Zivkovic Stosic, 2021) or are perhaps usually not reported in studies of recent organic matter. At this stage, an association with plant contribution is challenged by the limited evidence of terrestrial organic matter contribution to Montney hydrocarbons (e.g., Ardakani et al., 2022). If we attempt to find other potential precursors based on the molecular structure, a suitable candidate may be the 3-hydroxy-fatty acid compound class, which are widely distributed in aquatic environments. These acids are known to be part of cell membranes in bacterial communities (Cranwell et al., 1987; Wakeham, 1999; Wakeham et al., 2003), and have been used for temperature reconstruction due to the sensitivity of certain bacterial groups to climate change (Wang et al., 2016; Huguet et al., 2019; Yang et al., 2021). However, the potential degradation of 3-hydroxy-fatty acids to alkan-3-ols in the study samples yet lacks supporting evidence.
5.4 Potential associations with the general Montney Formation stratigraphy
To further investigate the applicability of oxygenated aliphatic compounds as source discriminators, we divided the data in Lower, Middle, and Upper members in Figure 7. The molecular characteristics of the samples do not seem to be specific of a particular Montney Member. The samples from the Upper Member in British Columbia are more mature (Figure 7B). The lack of differentiation among Montney Members might have resulted from hydrocarbons migrating from the same source or variable combinations of two or more sources.
Although defining source discriminators of Montney petroleum fluids remains a challenge, we have made evident that oxygenated aliphatic compounds are suitable candidates to achieve such goal, not only because they have been preserved, but also because of their variability in the samples. The characteristics of polar compounds are also important because they have very unlikely been overprinted by hydrocarbons that migrated during the Eocene, which mostly consist of gas/condensate (very low concentration of polar compounds). Therefore, the polar compounds from the extracted bitumen are a closer approximation to the hydrocarbons that originally migrated into Montney intervals before its maximum burial.
From a broader perspective, it is intriguing that the molecular distributions observed herein do not indicate clear association with the generation mechanisms currently known for the synthesis of these compounds in recent sediments. Another pathway for investigation is to evaluate whether the polar compounds studied were as sensitive to climate variations as they are known to be in recent depositional environments.
A final aspect that is important for low-permeability reservoirs in the region is that the thermal degradation of polar compounds might also be affecting other rock properties such as organic porosity, which plays a key role in hydrocarbon production.
6 Conclusion
Fatty acids, alkan-2-ones, diols and alkan-3-ols have been identified in extracted bitumen from the Montney Formation. This may represent the first time that 1,13-, 1,14- and 1,15- diols as well as alkan-3-ols are reported in fossil organic matter.
Regardless of thermal maturation overprint on the original signatures of the fatty acids merely based on their distribution, we infer marine organic matter (<C20 range dominant) source. Exceptions include samples X12140 and 12143 where a bimodal molecular distribution was observed with additional maximum peaks in C22 and C24 respectively. Further evidence is required to suggest a terrestrial input in these samples. Both samples correspond to different stratigraphic members and thermal maturity, thus the bimodality is probably a consequence of the mixing of contrasting hydrocarbon charges within those Montney sections before maximum burial.
Based on their distinctive distribution pattern, fatty acids have not been degraded to n-alkanes (e.g., via decarboxylation). Likewise, the alkan-2-ones do not represent oxidation products of the n-alkanes. Mechanisms to explain the preference for even-numbered carbon chain of alkan-2-ones remain unknown though even-chain ketones have been identified in Australian torbanites previously.
In contrast to recent organic matter, in Montney Formation the 1,14- and 1,15- seem to share a common source or generation mechanism as expressed by their very similar molecular distribution patterns. These and the 1,13-diols could be breakdown products of their longer-chain counterparts or have otherwise precursors that have not been identified to this date. The origin of 1,13-diols is not clearly associated to the other alkyl diols as interpreted based on their different molecular patterns.
The highly similar molecular distribution of alkan-3-ols and fatty acids suggest that they are biosynthetically related and/or share a common source. Their origin from the degradation of compounds with similar structure (e.g., 3-hydroxy-fatty acids) remains unclear.
Ultimately, our research opens a new path in geochemical screening of the unconventional Lower Triassic Montney Formation and conveys renovated interest in studying oxygenated compound classes in fossil organic matter that had scarcely been considered in recent years.
Data availability statement
The original contributions presented in the study are included in the article/Supplementary Material, further inquiries can be directed to the corresponding author.
Author contributions
JC conceptualized the study, wrote the first draft, and made Figures 2–7. SN helped conceptualizing the study and identifying compounds. JC, RR, and MM generated the data. RR and OA secured funding. OA elaborated Figure 1. All authors contributed to the analysis and interpretation of the data, and to writing and reviewing the manuscript.
Funding
This research was funded by Natural Resources Canada (NRCan) and its Geoscience for New Energy Supply (GNES) program, grant number 331403. The derivatization procedure was funded by the GEM-GeoNorth program in anticipation that the methodology can be applied to future program/project research.
Acknowledgments
The authors thank the editors for organizing the special issue and acknowledge the reviewers for their contribution to enhance the quality of this work.
Conflict of interest
The authors declare that the research was conducted in the absence of any commercial or financial relationships that could be construed as a potential conflict of interest.
Publisher’s note
All claims expressed in this article are solely those of the authors and do not necessarily represent those of their affiliated organizations, or those of the publisher, the editors and the reviewers. Any product that may be evaluated in this article, or claim that may be made by its manufacturer, is not guaranteed or endorsed by the publisher.
Supplementary material
The Supplementary Material for this article can be found online at: https://www.frontiersin.org/articles/10.3389/feart.2023.1137026/full#supplementary-material
References
Alhassan, A., and Anderson, J. T. (2013). Ketones in fossil materials a mass spectrometric Analysis of a crude oil and a coal tar. ENERG FUEL 27, 5770–5778. doi:10.1021/ef400961d
Allan, J., and Creaney, S. (1991). Oil families of the western Canada basin. Bull. Can. Petrol. Geol. 39, 107–122. doi:10.35767/gscpgbull.39.2.107
Ardakani, O. H., Cesar, J., Pedersen, P. K., Mackie, S. J., Reyes, J., and Wood, J. M. (2022). Occurrence and preservation of primary organic matter in a hybrid unconventional reservoir: Montney Formation, Western Canada Sedimentary Basin. Int. J. Coal Geol. 261, 104096. doi:10.1016/j.coal.2022.104096
Barakat, A. O., El-Gayar, M. Sh., and Mostafa, A. R. (2000). Geochemical significance of fatty acids in crude oils and related source rocks from Egypt. Pet. Sci. Technol. 18 (5–6), 635–655. doi:10.1080/10916460008949864
BC Oil and Gas Commission (2012). Montney Formation play atlas NEBC. Canada: Government of British Columbia, 36.
Cesar, J., Becker, V., and Mayer, B. (2020a). Organic and isotope geochemistry analysis of petroleum condensates from the unconventional portion of the Montney Formation, Western Canada. Fuel 282, 118879. doi:10.1016/j.fuel.2020.118879
Cesar, J., Nightingale, M., Becker, V., and Mayer, B. (2020b). Stable carbon isotope systematics of methane, ethane and propane from low-permeability hydrocarbon reservoirs. Chem. Geol. 558, 119907. doi:10.1016/j.chemgeo.2020.119907
Cesar, J., Mayer, B., Deblonde, C., Mort, A., and Ardakani, O. H. (2021). Alternative indicators to assess the distribution characteristics of methane, ethane, and propane derived from petroleum in the Montney Formation, Western Canada. Fuel 294, 120524. doi:10.1016/j.fuel.2021.120524
Cesar, J., Mayer, B., Becker, V., Nightingale, M., and Ardakani, O. H. (2022). Molecular and stable carbon isotope geochemistry of mud-gas-derived hydrocarbons and its application for the assessment of low-permeability reservoirs from the Montney Formation, Western Canada. Org. Geochem 163, 104328. doi:10.1016/j.orggeochem.2021.104328
Cranwell, P. A., Eglinton, G., and Robinson, N. (1987). Lipids of aquatic organisms as potential contributors to lacustrine sediments—II. Org. Geochem. 11 (6), 513–527. doi:10.1016/0146-6380(87)90007-6
Damste, J. S. S., Rampen, S., Irene, W., Rijpstra, C., Abbas, B., Muyzer, G., et al. (2003). A diatomaceous origin for long-chain diols and mid-chain hydroxy methyl alkanoates widely occurring in quaternary marine sediments: Indicators for high-nutrient conditions. Geochim. Cosmochim. Acta 67 (7), 1339–1348. doi:10.1016/s0016-7037(02)01225-5
Davies, G. R., Watson, N., Moslow, T. F., and Maceachern, J. A. (2018). Regional subdivisions, sequences, correlations and facies relationships of the lower triassic Montney Formation, west-central Alberta to northeastern British Columbia, Canada — With emphasis on role of paleo structure. Bull. Can. Petrol. Geol. 66, 23–92.
Davies, G. R. (1997). The triassic of the western Canada Sedimentary Basin: Tectonic and stratigraphic framework, paleogeography, paleoclimate and biota. Bull. Can. Petrol. Geol. 45, 434–460. doi:10.35767/gscpgbull.45.4.434
Derenne, S., Largeau, C., Hetényi, M., Brukner-Wein, A., Connan, J., and Lugardon, B. (1997). Chemical structure of the organic matter in a pliocene maar-type shale: Implicated botryococcus race strains and formation pathways. Geochim. Cosmochim. Acta. 61, 1879–1889. doi:10.1016/s0016-7037(97)00042-2
Ducros, M., Sassi, W., Vially, R., Euzen, T., and Crombez, V. (2017). “2-D basin modeling of the Western Canada sedimentary basin across the Montney-Doig system. implications for hydrocarbon migration pathways and unconventional resources potential,”. Petroleum systems analysis—case studies. Editors M. A. AbuAli, I. Moretti, and H. M. Nordgård Bolås Tulsa: (AAPG Memoir), 114, 117–134.
Ejezie, N. (2007). Triassic oil families and possible source rocks, peace river embayment area, Alberta, Canada. [dissertation/PhD thesis]. Calgary: University of Calgary.
Euzen, T., Watson, N., Fowler, M., Mort, A., and Moslow, T. F. (2021). Petroleum distribution in the Montney hybrid play: Source, carrier bed, and structural controls. AAPG Bull. 105, 1867–1892. doi:10.1306/12222020088
Fang, J., Wu, F., Xiong, Y., Li, F., Du, X., An, D., et al. (2014). Source characterization of sedimentary organic matter using molecular and stable carbon isotopic composition of n-alkanes and fatty acids in sediment core from Lake Dianchi, China. Sci. Total Environ. 473–474, 410–421. doi:10.1016/j.scitotenv.2013.10.066
Freeman, K. H., and Pancost, R. D. (2014). “Biomarkers for terrestrial plants and climate,” in Treatise on geochemistry. Editor K. K. Turekian (Oxford: Elsevier), 395–416.
George, S. C., and Jardine, D. R. (1994). Ketones in a Proterozoic dolerite sill. Org. Geochem. 21, 829–839. doi:10.1016/0146-6380(94)90042-6
Grimalt, J. O., Angulo, L., Lopez-Galindo, A., Comas, M. C., and Albaiges, J. (1990). Lipid and mineralogical composition of the cretaceous black shale deposits of the fardes formation (southern iberian paleomargin, betic cordillera, south Spain). Chem. Geol. 82, 341–363. doi:10.1016/0009-2541(90)90089-p
Huguet, A., Coffinet, S., Roussel, A., Gayraud, F., Anquetil, C., Bergonzini, L., et al. (2019). Evaluation of 3-hydroxy fatty acids as a pH and temperature proxy in soils from temperate and tropical altitudinal gradients. Org. Geochem. 129, 1–13. doi:10.1016/j.orggeochem.2019.01.002
Jaffé, R., and Gallardo, M. T. (1993). Application of carboxylic acid biomarkers as indicators of biodegradation and migration of crude oils from the Maracaibo Basin, Western Venezuela. West. Venezuela. Org. Geochem. 20 (7), 973–984. doi:10.1016/0146-6380(93)90107-m
Jaffé, R., and Gardinali, P. R. (1990). “Generation and maturation of carboxylic acids in ancient sediments of the Maracaibo Basin, Venezuela,”. Advances in organic geochemistry 1989. Editor B. Durand Exeter, UK: (F. Behar Org. Geochem), 16, 211–218.
Jaffé, R., Gardinali, P., and Wolf, G. A. (1992). Evolution of alkanes and carboxylic acids in ancient sediments from the Maracaibo Basin. Org. Geochem. 18 (2), 195–201. doi:10.1016/0146-6380(92)90130-p
Jaraula, C. M. B., Schwark, L., Moreau, X., Pickel, W., Bagas, L., and Grice, K. (2015). Radiolytic alteration of biopolymers in the Mulga Rock (Australia) uranium deposit. J. Appl. Geochem. 52, 97–108. doi:10.1016/j.apgeochem.2014.11.012
Koning, E., van Iperen, J. M., van Raaphorst, W., Brummer, G.-J. A., and van Weering, T. C. E. (2001). Selective preservation of upwelling-indicating diatoms in sediments off Somalia, NW Indian Ocean. Deep Sea Res. 1 Oceanogr. Res. Pap. 48, 2473–2495. doi:10.1016/s0967-0637(01)00019-x
Leif, R. N., and Simoneit, B. R. T. (1995). Ketones in hydrothermal petroleums and sediment extracts from Guaymas Basin, Gulf of California. Gulf Calif. Org. Geochem. 23, 889–904. doi:10.1016/0146-6380(95)00085-2
Lopez-Dias, V., Blanco, C. G., Bechtel, A., Puttmann, W., and Borrego, A. G. (2013). Different source of n-alkanes and n-alkan-2-ones in a 6000cal. yr BP Sphagnum-rich temperate peat bog (Ronanzas, N Spain). Org. Geochem. 57, 7–10. doi:10.1016/j.orggeochem.2013.01.006
Michels, R., Burkle, V., Mansuy, L., Langlois, E., Ruau, O., and Landais, P. (2000). Role of polar compounds as source of hydrocarbons and reactive medium during the artificial maturation of Mahakam Coal. ENERG FUEL 14, 1059–1071. doi:10.1021/ef000046d
Naafs, B. D. A., Inglis, G. N., Blewett, J., McClymont, E. L., Lauretano, V., Xie, S., et al. (2019). The potential of biomarker proxies to trace climate, vegetation, and biogeochemical processes in peat: A review. Glob. Planet Change 179, 57–79. doi:10.1016/j.gloplacha.2019.05.006
Naeher, S., Cui, X., and Summons, R. (2022). Biomarkers: Molecular tools to study life, environment, and climate. Elements 18 (2), 79–85. doi:10.2138/gselements.18.2.79
Perry, G. J., Volkman, J. K., Johns, R. B., and Bavor, H. (1979). Fatty acids of bacterial origin in contemporary marine sediments. Geochim. Cosmochim. Acta. 43, 1715–1725. doi:10.1016/0016-7037(79)90020-6
Peters, K. E., Walters, C. C., and Moldowan, J. M. (2005). The biomarker guide. Cambridge: Cambridge University Press.
Puttmann, W., and Bracke, R. (1995). Extractable organic compounds in the clay mineral sealing of a waste disposal site. Org. Geochem. 23 (1), 43–54. doi:10.1016/0146-6380(94)00106-b
Radulovic, N. S., and Zivkovic Stosic, M. Z. (2021). Long-chain syn-1-phenylalkane-1,3-diyl diacetates, related phenylalkane derivatives, and sec-alcohols, all possessing dominantly iso-branched chain termini, and 2/3-methyl-branched fatty acids from Primula veris L. (Primulaceae) wax. Phytochem 186, 112732. doi:10.1016/j.phytochem.2021.112732
Rampen, S. W., Thomas, F., Rybalka, N., and Thiel, V. (2021). The long chain diol index: A marine palaeotemperature proxy based on eustigmatophyte lipids that records the warmest seasons. PNAS 119 (16), e2116812119. doi:10.1073/pnas.2116812119
Rieley, G., Collier, R. J., Jones, D. M., and Eglinton, G. (1991). The biogeochemistry of ellesmere lake, U.K.—I: Source correlation of leaf wax inputs to the sedimentary lipid record. Org. Geochem. 17, 901–912. doi:10.1016/0146-6380(91)90031-e
Rodrigo-Gamiz, M., Rampen, S. W., Schouten , S., and Sinninghe Damste, J. S. (2016). The impact of oxic degradation on long chain alkyl diol distributions in Arabian Sea surface sediments. Org. Geochem. 100, 1–9. doi:10.1016/j.orggeochem.2016.07.003
Rokosh, C., Lyster, S., Anderson, A., Berhane, H., Brazzoni, T., Chen, D., et al. (2012). “Summary of Alberta’s shale- and siltstone-hosted hydrocarbon resource potential,” in Energy resources conservation board/alberta geological Survey, open file report 2012-06.
Sanei, H., Wood, J. M., Ardakani, O. H., Clarkson, C. R., and Jiang, C. (2015). Characterization of organic matter fractions in an unconventional tight gas siltstone reservoir. Int. J. Coal Geol. 150–151, 296–305. doi:10.1016/j.coal.2015.04.004
Strelnikova, E. B., and Serebrennikova, O. V. (2011). Ketones of Western siberian jurassic oils. Petrol. Chem. 51, 264–269. doi:10.1134/s0965544111030145
van Aarssen, B. G. K., Bastow, T. P., Alexander, R., and Kagi, R. I. (1999). Distributions of methylated naphthalenes in crude oils: Indicators of maturity, biodegradation and mixing. Org. Geochem. 30, 1213–1227. doi:10.1016/s0146-6380(99)00097-2
Volkman, J. K., Barrett, S. M., Dunstan, G. A., and Jeffrey, S. W. (1992). C30–C32 alkyl diols and unsaturated alcohols in microalgae of the class Eustigmatophyceae. Org. Geochem. 18, 131–138. doi:10.1016/0146-6380(92)90150-v
Volkman, J. K., S., Barrett, S. M., and Blackburn, S. I. (1999). Eustigmatophyte microalgae are potential sources of C29 sterols, C22–C28 n-alcohols and C28–C32 n-alkyl diols in freshwater environments. Org. Geochem. 30, 307–318. doi:10.1016/s0146-6380(99)00009-1
Wakeham, S. G., Peterson, M. L., Hedges, J. I., and Lee, C. (2002). Lipid biomarker fluxes in the arabian sea, with a comparison to the equatorial pacific ocean. Deep Sea Res. 2 Top. Stud. Oceanogr. 49, 2265–2301. doi:10.1016/s0967-0645(02)00037-1
Wakeham, S. G., Lewis, C. M., Hopmans, E. C., Schouten, S., and Damste, J. S. S. (2003). Archaea mediate anaerobic oxidation of methane in deep euxinic waters of the Black Sea. Geochim. Cosmochim. Acta 67 (7), 1359–1374. doi:10.1016/s0016-7037(02)01220-6
Wakeham, S. G. (1999). Monocarboxylic, dicarboxylic and hydroxy acids released by sequential treatments of suspended particles and sediments of the Black Sea. Org. Geochem. 30 (9), 1059–1074. doi:10.1016/s0146-6380(99)00084-4
Wang, Z., and Liu, W. G. (2012). Carbon chain length distribution in n-alkyl lipids: A process for evaluating source inputs to lake qinghai. Org. Geochem 50, 36–43. doi:10.1016/j.orggeochem.2012.06.015
Wang, C., Bendle, J., Yang, Y., Yang, H., Sun, H., Huang, J., et al. (2016). Impacts of pH and temperature on soil bacterial 3-hydroxy fatty acids: Development of novel terrestrial proxies. Org. Geochem. 94, 21–31. doi:10.1016/j.orggeochem.2016.01.010
Watt, E. A., Laycock, D. P., Michael, E., Toblin, R. C., Kelly, S., and Johnston, M. N. (2022). Hydrocarbon charge and petroleum system evolution of the Montney Formation: A multidisciplinary case study of the blueberry sub-play in northeast British Columbia, Canada. BCEG 69, 21–50. doi:10.35767/gscpgbull.69.1.021
Wood, J., and Sanei, H. (2016). Secondary migration and leakage of methane from a major tight-gas system. Nat. Commun. 7 (13614), 13614–13619. doi:10.1038/ncomms13614
Wood, J., Sanei, H., Ardakani, O. H., Curtis, M., and Akai, T. (2018). Organic petrography and scanning electron microscopy imaging of a thermal maturity series from the Montney tight-gas and hydrocarbon liquids fairway. Bull. Can. Pet. Geol. 66 (2), 499–515.
Wood, J. M., Cesar, J., Ardakani, O. H., Rudra, A., and Sanei, H. (2022). Geochemical evidence for the internal migration of gas condensate in a major unconventional tight petroleum system. Sci. Rep. 12, 7931. doi:10.1038/s41598-022-11963-6
Yang, Y., Wang, C., Bendle, J. A., Luo, Z., Dang, X., Xue, J., et al. (2021). Appraisal of paleoclimate indices based on bacterial 3-hydroxy fatty acids in 20 Chinese alkaline lakes. Org. Geochem. 160, 104277. doi:10.1016/j.orggeochem.2021.104277
Zhang, Z., and Volkman, J. K. (2017). Algaenan structure in the microalga Nannochloropsis oculate characterized from stepwise pyrolysis. Org. Geochem. 104, 1–7. doi:10.1016/j.orggeochem.2016.11.005
Zhang, Z., and Volkman, J. K. (2020). Isotopically enriched n-alkan-2-ones with even chain predominance in a torbanite from the Sydney Basin, Australia. Org. Geochem. 144, 104018. doi:10.1016/j.orggeochem.2020.104018
Zheng, Y., Zhou, W., Meyers, P. A., and Xie, S. (2007). Lipid biomarkers in the Zoige-Hongyuan peat deposit: Indicators of Holocene climate changes in West China. Org. Geochem. 38, 1927–1940. doi:10.1016/j.orggeochem.2007.06.012
Keywords: polar, diol, acid, ketone, low-permeability, Montney
Citation: Cesar J, Robinson R, Naeher S, Milovic M and H. Ardakani O (2023) Fatty acids, alkanones and alcohols from a major lower Triassic low-permeability petroleum reservoir. Front. Earth Sci. 11:1137026. doi: 10.3389/feart.2023.1137026
Received: 03 January 2023; Accepted: 24 April 2023;
Published: 09 May 2023.
Edited by:
Feng Yang, China University of Geosciences Wuhan, ChinaReviewed by:
Guangyou Zhu, Research Institute of Petroleum Exploration and Development (RIPED), ChinaZhixing Ru, SINOPEC Petroleum Exploration and Production Research Institute, China
Copyright © 2023 Cesar, Robinson, Naeher, Milovic and H. Ardakani. This is an open-access article distributed under the terms of the Creative Commons Attribution License (CC BY). The use, distribution or reproduction in other forums is permitted, provided the original author(s) and the copyright owner(s) are credited and that the original publication in this journal is cited, in accordance with accepted academic practice. No use, distribution or reproduction is permitted which does not comply with these terms.
*Correspondence: Jaime Cesar, amFpbWVyYWZhZWwuY2VzYXJjb2xAdWNhbGdhcnkuY2E=