- 1Institute of Archaeology, Centre for Applied Archaeology, Nicolaus Copernicus University in Toruń, Toruń, Poland
- 2Department of Archaeology, Copper Museum in Legnica, Legnica, Poland
- 3Department of Historical Studies, University of Gothenburg, Gothenburg, Sweden
- 4Institute of Archaeology, University of Wrocław, Wrocław, Poland
- 5Polish Geological Institute - National Research Institute, Warszawa, Poland
- 6School of Geography, Earth and Atmospheric Sciences, University of Melbourne, Parkville, VIC, Australia
- 7Faculty of Chemistry, Biological and Chemical Research Centre, University of Warsaw, Warsaw, Poland
Despite rich deposits of copper in SW Poland, their exploitation in prehistory has not been confirmed, and in the literature it is usually argued that raw materials processed in the Bronze Age were imported. This is despite the same area providing abundant evidence of prehistoric metallurgy including casting moulds, tuyeres or crucibles. The concentration of prehistoric sites in parts of the Sudety Mountains (in particular a region called Pogórze Kaczawskie) rich in copper ores and far from arable land may also indicate prehistoric prospection activities in this area in the search for raw materials. In this paper, we discuss the issue of the provenance of the metal used in SW Poland, an area where metallurgy-related items dated to the Urnfield period (ca. 1300–500/450 BC) are most numerous. Our study utilises historical evidence of pre-modern mining, GIS analysis of prehistoric sites, and lead isotopic analyses conducted on bronze (n=35) and lead (n=1) artefacts found near the copper outcrops in Silesia, and compared with European lead isotope databases. The lead isotopic data for the Zechstein Limestone (Ca1) ores from a local mine and modern black copper from blast furnaces at Leszczyna and Chełmiec in SW Poland were also analysed as reference data (n=6). Our research demonstrates that metal objects in SW Poland were made of copper obtained from various parts of Europe confirming intense contacts of the Urnfield communities but the lead ornaments were sourced from material of local origin providing the first evidence for the provenance of Polish lead.
1 Introduction
The acquisition of the metal raw materials used for casting production and an attempt to identify sources of ores are critical questions in prehistoric metallurgy. In Europe, copper ore mines, located on the Iberian Peninsula, the British Isles or in the eastern Alps, have operated since the Eneolithic (O’Brien, 2015). The oldest copper mining in Europe is attested for the Balkans (Borić, 2009). In addition to these deposits, the use of which has been confirmed by archaeological research, however, there are many other potential mining sites in Europe that could have been used in prehistory, but for which we do not have clear evidence of local exploitation. In these mining regions, this activity may have been of a small-scale, and traces have not yet been identified (cf. e.g., Harding, 2000, 207-210) or they could have been destroyed by later exploitation (Tylecote, 1987).
The prehistoric exploitation of copper ore deposits in Poland has not been confirmed so far and in the literature it is argued that all metal processed in Poland was imported (e.g., Kostrzewski, 1953, 194; Gardawski, 1979, 273; Gedl, 1982, 39). However, the possibility of exploiting raw materials such as copper or tin in SW Poland (historical land of Lower Silesia) in prehistory was noted in the archaeological literature even before World War II. Witter (1938) argued that it was possible to exploit native copper and indicated several locations in Silesia, where native copper occurred. He suggested that oxidized copper ores, such as azurite or malachite, were used and the metal was obtained by simple reduction. Witter also discusses oxidized copper ores related to the so-called Silesian copper-bearing shales as a possible source of the copper in the Bronze Age. He suggested that the extraction of oxidized ore from shallow shale deposits was possible in prehistoric times (Witter, 1938, 202).
This paper has become the basic source for later considerations on the prehistoric extraction of copper ores in Lower Silesia (Jamka, 1950, 28; Kostrzewski, 1953, 194; Gediga, 1967, 229-230; Gediga, 1982, 112; Gediga, 1988, 11; Gedl, 1988, 29; Stolarczyk, 2014a, 496-497). Gedl suggested the possibility of obtaining copper ores in the Kaczawskie Foothills (Polish: Pogórze Kaczawskie) as early as in the Montelius period II (ca. 1600/1500–1300 BC), with exploitation developed until the Early Iron Age (ca. 800/750–500/450 BC; Gedl, 1988, 34). However, this hypothesis has not yet been confirmed in any way. In these literature studies future research perspectives were provided, highlighting the need to conduct field work and to perform metal provenance analyses aimed at confirming or excluding the possibility of prehistoric use of local metallic raw materials (Witter, 1938; Gediga, 1988). We note that almost a century has passed from the moment Wilhelm Witter first indicated in 1938 the locations of possible exploitation to the first research work of this kind undertaken by us in 2019.
The present paper concerns the issue of the provenance of the metal used for production of the metal artefacts in south-western Poland, i.e., the area where.
- the copper deposits are located;
- numerous Bronze and Early Iron Age metal artefacts occur;
- metallurgy-related items, settlements with traces of metallurgical activities and graves furnished with casting tools are the most numerous.
We applied a number of methods to answer the following questions.
1. What is the provenance of the metal used in southern Poland?
2. Can lead isotope studies of local copper ore deposits and metal artefacts discovered in their immediate vicinity confirm local exploitation?
3. Were the local copper ore deposits accessible for Bronze Age and Early Iron Age society and could they have been exploited in prehistory?
Our research also provides a summary of previous research on the origin of the metallic raw materials in south-western Poland in the (Urnfield) Bronze Age and the Early Iron Age.
2 Geological background
The European Kupferschiefer ore deposits are sediment-hosted strata-bound copper deposits associated with the Upper Permian geologic period and extending over a large area of Europe from England through the Netherlands and Germany to Poland (Vaughan et al, 1989; Wedepohl, 1994; Kucha, Pawlikowski, 2010; Borg et al, 2012; Figure 1A).
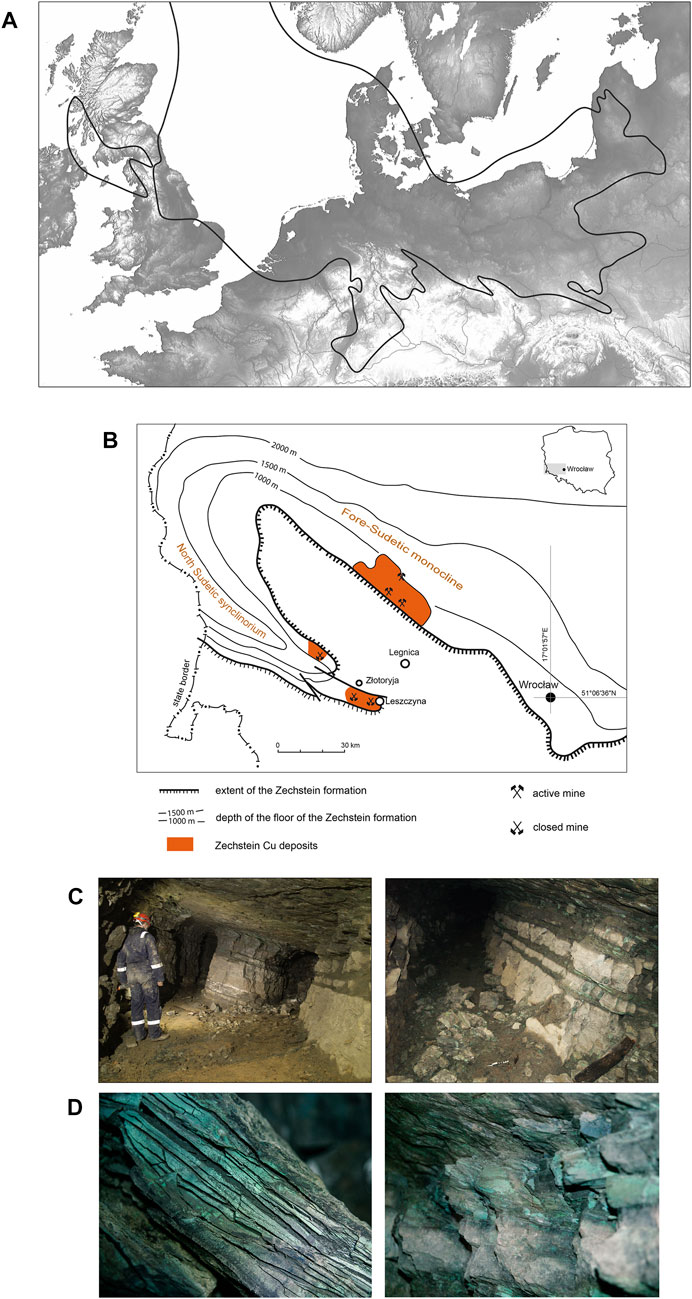
FIGURE 1. Range of the Zechstein sea in Europe. Source: OpenStreetMap and Voughan et al, 1989, with modifications (A); Zechstein deposits in SW Poland. Source: Stolarczyk et al, 2017, with modifications (B); The Stilles Glück mine in Leszczyna: a bed of copper-bearing marl in on the lower level of the excavations (C), photo by A. Kostka, T. Stolarczyk; secondary malachite mineralization within the copper-bearing marl bed (D), photo by D. Zapał.
The study area is located in the southeastern part of the North Sudetic Basin (NSB), next to the NE edge of the Bohemian Massif in SW Poland. The specific geological unit is named the Leszczyna Syncline (Figure 1B). Both the Leszczyna Syncline and the NSB form a NW-SE oriented, elongated structure that appeared as a result of large-scale folding and faulting, mainly during Cretaceous compression (Solecki, 2011). The NSB is bordered by faults and adjoins the Kaczawa Metamorphic Complex from the north and east. Situated on the Variscan metamorphic basement, the volcanic and sedimentary cover of the basin is dated to Paleozoic—Carboniferous and Permian (Zechstein and Rotliegend formations) and Mesozoic—Triassic and Cretaceous (Solecki, 2011). Neogene basaltic intrusions are spread around the unit.
Zechstein sediments were deposited when the area was located in the marginal part of the Zechstein Sea Basin which over time changed its extent because of periodical transgressions and regressions. Environmental changes resulted in the deposition of an alternating succession of marls and slates with limestones, referred to as cyclothems (Raczyński, 1997). Copper ores are present within the Lower Zechstein sequence (Zechstein Limestone Ca1), and also in Triassic and Cretaceous rocks. Stratigraphically, the exact position of the NSB is not recognized precisely (Fijałkowska-Mader et al, 2018) but it is commonly accepted that it is located between the Kupferschiefer T1, and Lower Anhydrite A1d, all of which were sedimented within the Permian PZ1 cycle (first one out of three sedimentation cycles recognized within Polish Zechstein basinal succession). Copper-bearing series include limestones, marls, dolomites, shales, anhydrides, and locally sandstones (Solecki, 2011), however, shales, marls, and limestones constitute the dominant Cu-ores containing approx. 0.35 to 1 wt% Cu (Maciejak, Maciejak, 2016). Chalcocite and bornite dominate within sulfides but generally, the Zechstein Cu-bearing series in the NSB is characterized as an oxidation zone where Cu-carbonates (namely, malachite and azurite) constitute the main Cu-carriers.
Although Cu-enriched rocks are present around the whole NSB unit, their depth changes with different locations. Surface outcrops are present near Leszczyna village (SE outline of the NSB; Figure 1B). The thickness of the deposit ranges from approx. 2, up to approx. 3.5 m, and the deposit declines at a slight angle of av. 6° to N and NW, reaching over 800 m near the Konrad Mine in Iwiny, about 25 km NW from the research area. That site lies within the Grodziec Syncline where Cu was also mined in the past (Kowalski et al, 2017). The entire area was named the “Old Copper Basin” in contrast to the “New Copper Basin” in the Fore-Sudetic Monocline where the ores are currently exploited (Derkowska et al, 2021).
The Zechstein is divided into lower, middle and upper units. The cuprous marl, which is the Lower Zechstein formation, is a heterogeneous rock, consisting of numerous layers of marly slate and limestone arranged alternately, as shown in Figure 1C. In Western Europe, deposits of a similar geological origin were exploited, among others in the area of Mansfeld, Eisleben and Hettstedt (Saxony-Anhalt, district Mansfeld-Südharz; Voughan et al, 1989; Borg et al, 2012). These were rather poor deposits, but their presence in the form of a relatively regular bed facilitated the exploitation and made it profitable in modern times.
3 Historical mining activity in SW Poland
Some indirect data pertaining to the penetration of this area in prehistory was obtained by a surface survey in 1982–1984 (Gedl, 1986). Gedl argues that the area has been intensely explored for various raw materials since the Neolithic, first for high-quality lithic material then for copper and gold ores. This possibility may be indicated by the distribution of the Bronze and Early Iron Age locations marked by pottery shards and some objects made of bronze and gold which correspond with easy-accessible copper and ore deposits. Also, a relatively high number of finds far from arable land also suggest the prehistoric prospection of this area in search of raw materials (Figure 2A).
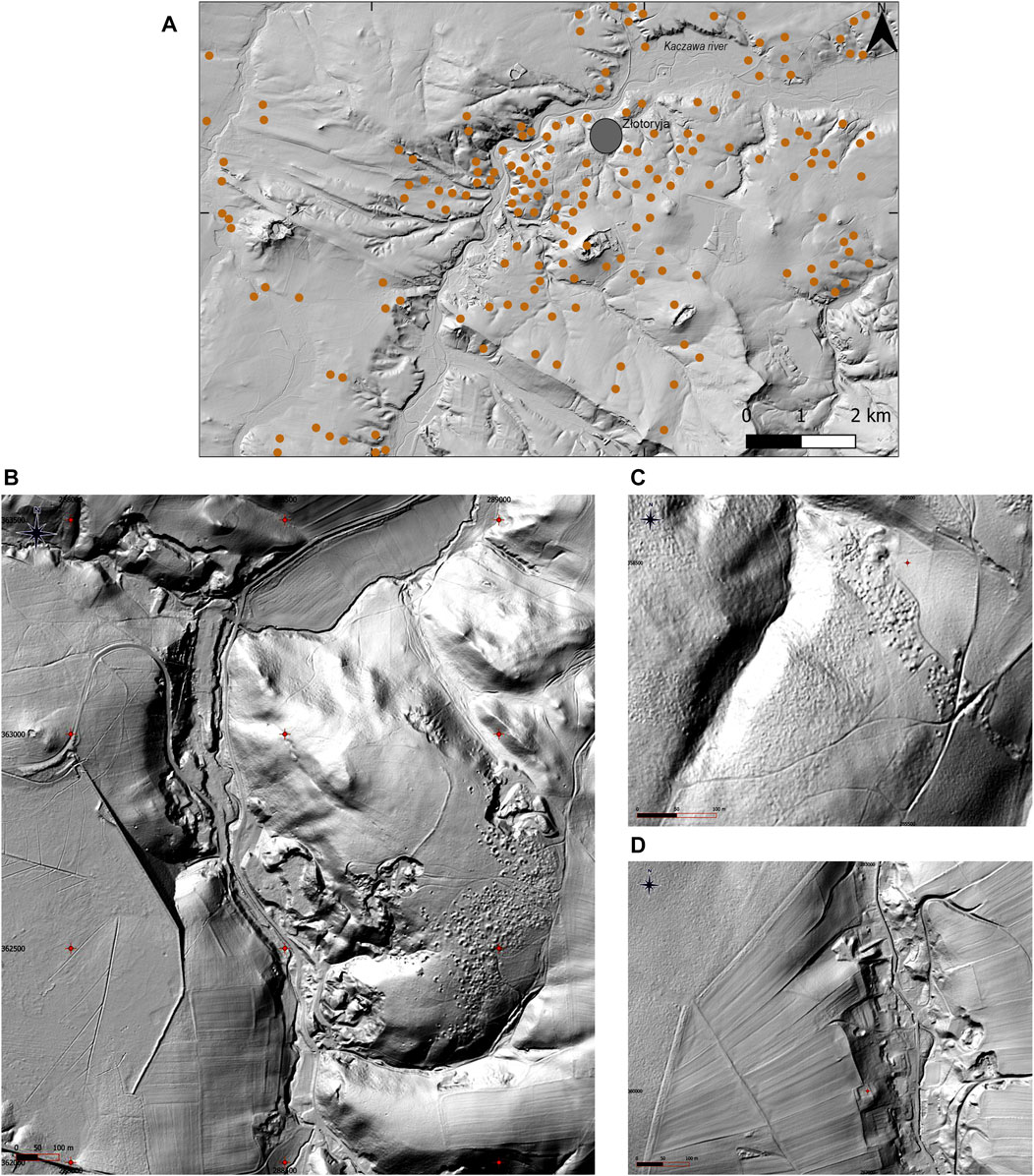
FIGURE 2. Distribution of prehistoric and early medieval sites in the Kaczawskie Foothills based on surface surveys. Source: Gedl 1986; geoportal.gov.pl, with modifications (A); post-mining structures—exploitation fields near Leszczyna, Kondratów and Biegoszów. Accumulation of shafts (Pingen) of various morphology (B–D). Source: geoportal.gov.pl.
Extensive research on metallic ore deposits and their exploitation in Lower Silesia has been carried out mostly in the context of younger periods, i.e., the Middle Ages onwards (Stolarczyk, 2011; 2012a; 2012b; Maciejak, Maciejak, 2013; Maciejak et al, 2016). The results of these studies will be briefly discussed in order to characterize the problems related to the exploitation of local deposits. They form the basis for further considerations related to the possible extraction of metallic ores in prehistory.
Stolarczyk identifies 20 mining regions (2011, Figure 1) that existed in Lower Silesia between the 13th and 17th centuries that were associated with the extraction of gold, silver, copper, lead and tin. Mining activity in this area was intense at the beginning of the 13th century, which is confirmed by historical sources and the results of archaeological field surveys (Stolarczyk, 2014). The most intense mining activity related to the extraction of silver, copper and lead in Lower Silesia took place in the mining area of Miedzianka (Miedzianka-Ciechanowice-Janowice). The main metallic mineral found in the polymetallic deposits of this region was chalcopyrite, and apart from it, also identified, are chalcocite, bornite, covellite, tetrahedrite, galena, pyrite, and magnetite. The metal content in the ore ranged from 0%–35% Cu, 0.3%–2.5% Zn, 16%–28% Fe (Stolarczyk, 2012b, 229). Smaller mining regions associated with the exploitation of copper include the region of Leszczyna—Kondratów—Biegoszów—Nowy Kościół, located in the Kaczawskie Foothills (see Figure 1B). This is one of the regions indicated by Witter in 1938. Due to the relatively shallow level of ores, we will focus in detail on this region.
The use of local polymetallic ores in the Leszczyna region is confirmed by written sources and archaeological research from the beginning of the 15th century (Stolarczyk, 2011, 211). The copper ore deposits occurring here belong to both vein and bed deposits. They are associated with the deposits of the Zechstein sea (late Permian).
In the Leszczyna ore deposit, the predominant ores are bornite (Cu5FeS4: 63.3% Cu, 11.2% Fe, 25.5% S), chalcocite (Cu2S: 79.8% Cu, 20.2% S), and chalcopyrite (CuFeS2: 34.54% Cu, 30.54% Fe, 34.9% S) occurring mostly in the top parts of the spotted marl and the bottom parts of the lead marl. Pyrite, galena and native silver are also found in the ore. Oxidized minerals—azurite (Cu3(CO3)2(OH)2; up to 57% Cu) and malachite (Cu2CO3(OH)2; up to 55% Cu) occur in fault zones and fissures, as well as in the vicinity of outcrops. Also, covellite (CuS) and native copper are recorded in the deposit (Stolarczyk et al, 2017, 19). A general view of the copper and silver content in marl is shown by the output from the years 1865–1882 in the Stilles Glück mine. According to archival data, 85000 tons of copper-bearing marl were extracted at that time. On average, 13 kg of copper and 40 g of silver were obtained from one ton of marl (Stolarczyk et al, 2017).
Relief forms related to mining activities carried out in the Leszczyna region are numerous relicts of the shafts used to exploit the copper-bearing bed (Figure 2B–D). Small-scale deformations of the land surface (German Pingen) are among the oldest and smallest post-mining forms of land relief in the area of the Old Copper Basin (Kowalski et al, 2017, 187). These are hollows in the form of a funnel, formed in the place of collapsed vertical mining shafts. The exact date of their origin is unknown, although it is believed that they originate in the 13th century. Next to the shafts there are usually heaps of waste rock, most often in a circular or semicircular form. Most of these types of objects occur in the vicinity of Leszczyna, at the highest level of the workings associated with the modern Character Mine. Pingen are also present but less numerous in the vicinity of Nowy Kościół and Biegoszów, and Kondratów (Kowalski et al, 2017, 189).
The exact characterization of Pingen in the vicinity of Leszczyna was presented using a LIDAR digital terrain model (DTM) by Kowalski and co-authors (2017, 189). According to this study, shaft hollows have various forms, most often they are cavities with a maximum diameter not exceeding 20 m and a depth of 4 m. Next to them are smaller Pingen (up to 2 m in diameter). In the eastern part of the study area of Kowalski et al, they are shallow, irregularly spaced and overlapping shafts (2017, Figure 9). The lack of accurate dating of most of the ‘Pingen fields’, as well as their different sizes, may indicate different chronologies. It cannot be excluded that some of them are the results of prehistoric exploration as it is observed elsewhere that long linear series of Pingen were recorded (e.g., in Mitterberg; Stöllner, 2015, 180).
4 Archaeological evidence of prehistoric metallurgy in SW Poland
The beginnings of copper processing in modern Poland start in the Neolithic, in the early fourth millennium BC (Dziekoński, 1962; Wojciechowski, 1973; Kulczycka-Leciejewiczowa, 1979, 145; Wiślański, 1979, 237; Gedl, 1982, 37). Evidence of this early metallurgy is small funnel-shaped and perforated objects which probably were tuyeres or casting crucibles (por. np. Gedl, 1985, 32–33; Bátora, 2003). They are however very rare and in most cases where metal objects are found it is not sure if they were manufactured locally (Kadrow, 2017, 84–86).
The local metallurgy of copper and its alloys also dates back to the Early Bronze Age (ca. 2400–1600 BC). The archaeological evidence, more abundant that in the case of the Neolithic, comes mostly from western and northern Poland (Richthofen, 1924, 61, Pl. 1-2; Lasak, 1991, 39; Figures 5D–H; Butent-Stefaniak, 1997, 89; Bruszczewo, 2004; Jaeger, Czebreszuk, 2010; Jaeger et al, 2015; Paruzel, 2011, 249, 250; Kowalski et al, 2021).
The territory of Poland during the Bronze Age and the Early Iron Age (ca. 2400–500/450 BC) was characterized by a varied level of metallurgical activity. This is based only on the distribution of items usually interpreted as remains of metallurgy: we assume, they reflect the intensity of local metallurgy. The distribution of finds directly related to the metal casting, such as casting moulds, nozzles, crucibles, and casting cores, reflect local metallurgical alloying activity (e.g., Jockenhövel, 1986; Bachmann et al, 2003).
An intense development of metallurgy occurred in the subsequent stages of the Bronze Age, in particular in the so-called Urnfield period (1300–500/450 BC). It must be noted here, that in Polish literature, unlike in other parts of Europe, the Urnfield period does not refer to the Late Bronze Age only but starts earlier in what is called Middle Bronze Age or Reinecke Hallstatt A (Kaczmarek, 2017, 277). It also stretches to periods usually labeled as Reinecke Hallstatt C and D (ibidem). To avoid misunderstanding, we add detailed labels when presenting the chronological groups of analysed artefacts. Having in mind the local Polish chronology and its labels, the peak of metallurgical development is therefore dated to Late Bronze Age (Reinecke HB2-B3, ca. 900–800 BC) and Early Iron Age (Reinecke HC-D, 800/750–500/450 BC; Figure 3A). This is reflected both in quantity and quality of finds related to this activity. In Lower Silesia, 30 sites with casting tools have been identified so far and most of them were found in the middle Oder basin. They come from settlements and grave contexts, as well as stray finds. Polish authors pointed to the exceptional convergence of such a large number of finds of casting tools in the vicinity of copper deposits (e.g., Jamka, 1950). They tried to combine the increase in casting production in this area with the exploitation of local copper deposits or the collection of native copper, that is, access to a raw material that was not available on such a scale before.
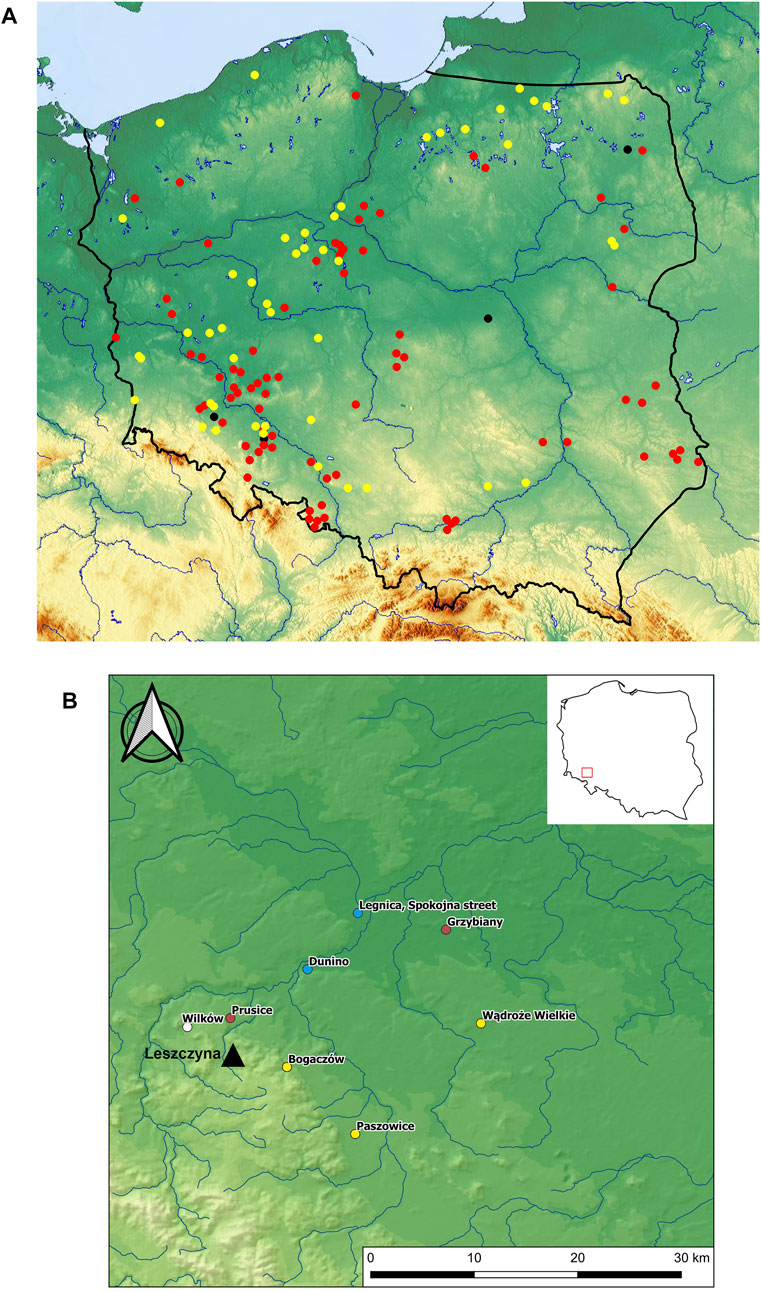
FIGURE 3. Distribution of finds related to metallurgy. Black dots—Montelius per. III, red—Montelius IV-V, yellow—Early Iron Age. Source: OpenStreetMap and Nowak 2020 (A); locations mentioned in the text. Source: google maps, map compilation by P. Dulęba (B).
Casting moulds made of stone, metal, and clay are the most frequently discovered finds in southwestern Poland, coming from 47 sites (with 103 two- and three-piece casting moulds and thousands of disposable casting moulds used in lost wax technique). Other categories of artefacts—nozzles, and crucibles—are much less common. Raw material in the form of lumps of plano-convex ingots (‘casting cakes’) is very rare. We do not have copper ore finds for the studied area.
5 Materials studied
5.1 Metal artefacts
To provide data on composition and possible material provenance, we sampled 35 metal objects and residues from one stone and two ceramic casting moulds (Table 1). All the objects were found close to copper ore outcrops in Pogórze Kaczawskie which might have been exploited in prehistory (Figure 3B). We selected a variety of types including tools, ornaments, weapons and pieces of raw material. The chronology of the collection covers the so-called Urnfield period starting ca. 1300 BC and lasting to the end of the Early Iron Age, i.e. 500-450 BC. We divided the collection into four main chronological groups labelled after Reinecke’s chronological system to trace diachronic dynamics in casting technology and raw material supply (Table 1). The most numerous group is dated to the late Bronze and Early Iron Age, i.e. 900-500/450 BC.
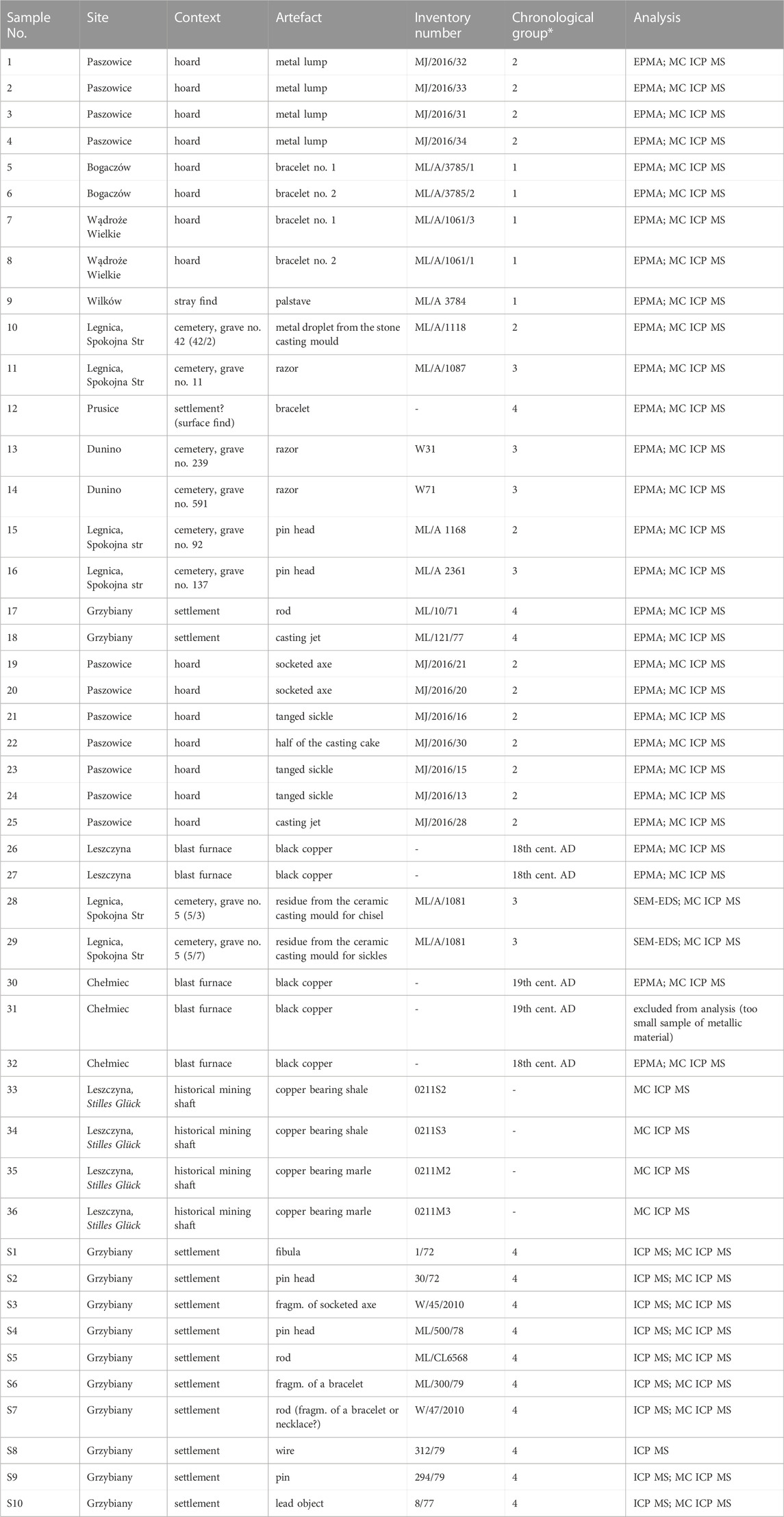
TABLE 1. list of samples * group 1: Reinecke HA1-A2, ca. 1200–1000 BC, group 2: Reinecke HB1, ca. 1000–900 BC, group 3: Reinecke HB2-B3, ca. 900–800 BC; group 4: Reinecke HC-D, ca. 800–450 BC.
The objects come from various contexts including three hoards (Bogaczów, Wądroże Wielkie, Paszowice), two urnfields (Legnica Spokojna str., Dunino), two settlements (Grzybiany, Prusice) and a stray find from Wilków (Figure 4, 5).
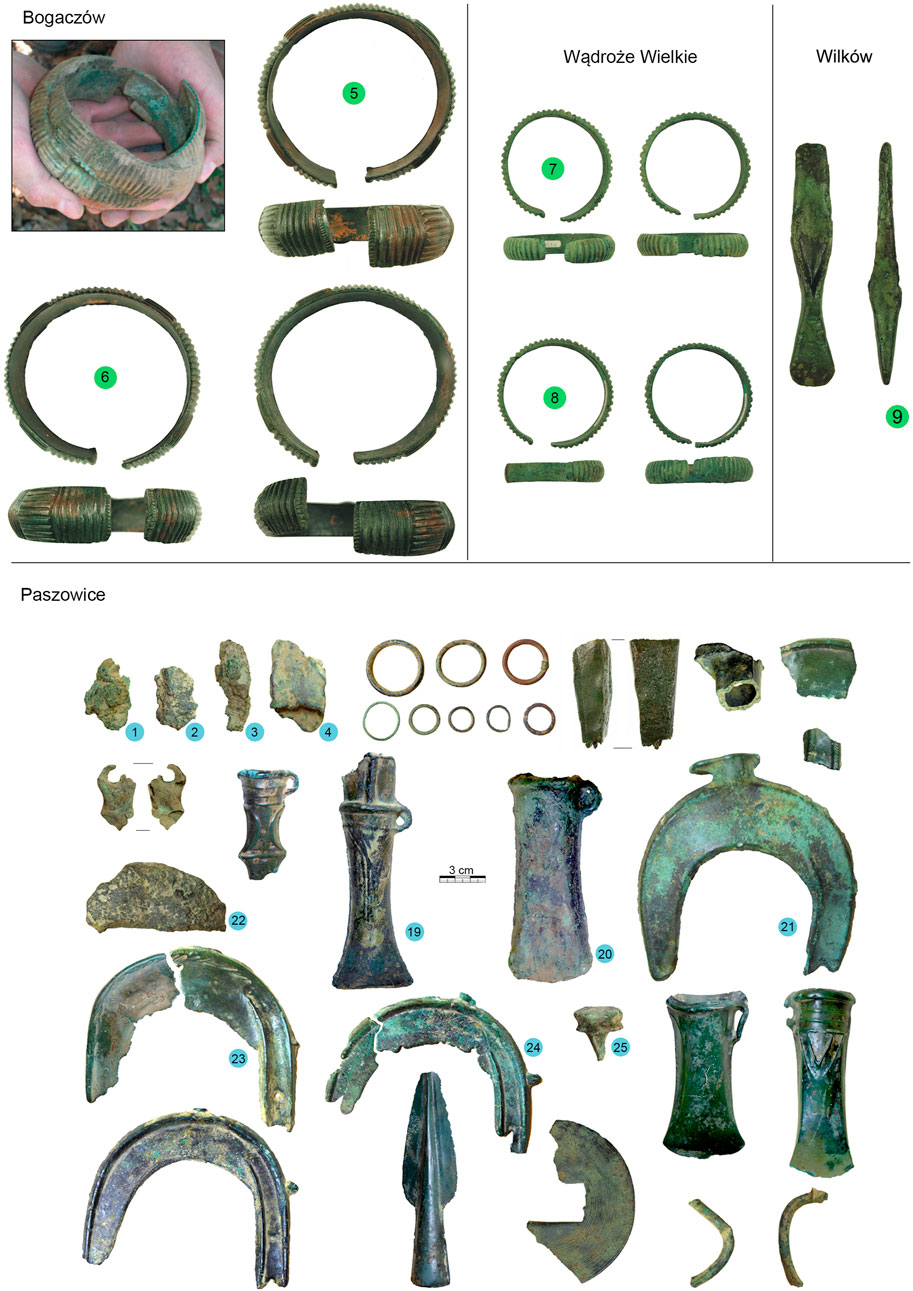
FIGURE 4. Sampled objects from hoards from Bogaczów, Wądroże Wielkie, Paszowice, and a stray find from Wilków. Dots contain sample numbers, colours mark the chronological groups (green—group 1, blue—group 2).
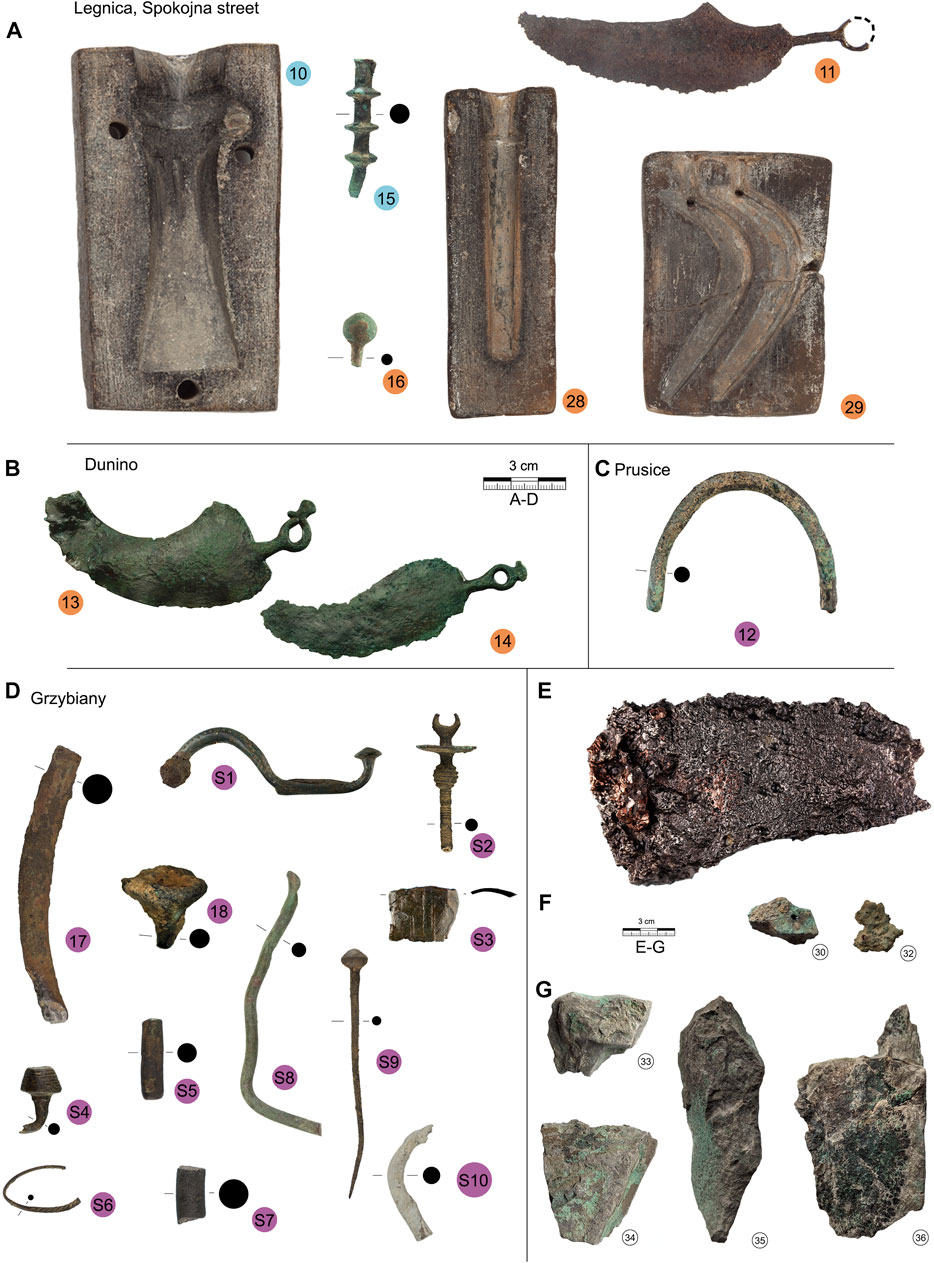
FIGURE 5. Sampled objects from cemeteries in Legnica, Spokojna str. and Dunino, settlements in Prusice and Grzybiany. Dots contain sample numbers, colours mark the chronological groups (blue—group 2, orange—group 3, purple—group 4) (A–D); reference material sampled for the study: an example of black copper from local smelter processing local ores (marls and shales) from the Stilles Glück Mine and the ores from this mine. Dots contain sample numbers (E–G).
The first chronological group includes two bracelets from Bogaczów, two from Wądroże Wielkie, and a palstave of the Bohemian type from Wilków. They are the oldest artefacts (Reinecke HA1-A2, ca. 1200–1000 BC) from Lower Silesia sampled for lead isotopes (Figure 4; Table 1).
The next group (Reinecke HB1, ca. 1000–900 BC) includes 11 objects from the Paszowice hoard (Nowak et al, 2019; 2022a; 2023b), one pin and a metal sample from stone casting mould found at the cemetery at Legnica Spokojna str. (Nowak, Stolarczyk, 2016). The collection from Paszowice consists of two socketed axes, three sickles, a casting jet, 1/2 of the casting cake and four lumps (Figure 4).
The third group (Reinecke HB2-B3, ca. 900–800 BC) includes six objects (Figure 5). Four come from the younger phase of the cemetery at Legnica Spokojna str., they are a crescent razor, pin head and two samples of residues from ceramic casting moulds. Two other razors come from the cemetery in Dunino. The crescent razors have traditionally been considered as imports from north Italy (Łuka, 1959, 35), but the casting mould for such razors discovered together with the matching cast from the cemetery in Legnica indicate their local production (Gedl, 1981, 34).
The last group covers the Early Iron Age (Reinecke HC-D, ca. 800–450 BC) and includes thirteen objects from two sites (Figure 5). Twelve come from a large metallurgical workshop at the settlement in Grzybiany (Stolarczyk, Baron, 2014). They are a casting jet, metal rods, fragment of socketed axe, fibula of the Certosa type and a profiled-head pin. Also one object made of lead (probably fragmented ornament) was sampled. A fragment of an Early Iron Age bracelet found during a surface survey at Prusice was sampled as well.
5.2 Ores and black copper
Reference data were obtained from two types of material: black copper and copper ores.
Four samples of black copper from metallurgical heaps around Leszczyna and Chełmiec were tested as reference material (sample nos. 26–27, 30, 32; Table 1; Figure 5). Blast furnaces worked here in the 18th and 19th century. They used material from local mines located in Leszczyna, Kondratów and Chełmiec.
We also obtained samples of copper-bearing shales and marls from layers related to the Zechstein deposits (samples nos. 33–36; Table 1 and Figure 5). For this purpose, we went to the Stilles Glück Mine in Leszczyna and collected samples in situ.
Ore samples chosen for Pb isotope analyses consist of marls and shales collected from the historical mining shaft located in Leszczyna village, in the interior the of historical mining center (Figure 1B). The material represents historically mined ores with minimal weathering impact, since the temperature and humidity conditions are stable, especially compared to samples found on the surface.We collected 15 samples from the rock profile (Figure 1C) of alternating lithologies starting from the ceiling to the floor of the outcrop. They were shales and marls alternately. Layer thicknesses range between 3 and 20 cm, with marl layers being wider (5–20 cm) compared to shales (3–10). We selected four samples for further isotopic analyses, i.e., two shales (0211S2, 0211S3) and two marls (0211M2, 0211M3) collected from the upper part of the profile (Figure 5G).
The ore samples are associated with the Zechstein Limestone formation (Ca1) and represent oxidized Cu mineralization. Secondary phases (malachite) are noted on the rock surface, confirming high Cu concentration. The top part of the profile adjoins the lead-bearing marls with specific red spots suggesting the presence of ore approx. 6–8 m deep in the local rock profile (Biernacka et al, 2005). Given the depth of the adit, where samples were collected, the depth of local ore reaches approx. 5–7 m below ground surface. The ore, however, wedges west, towards the historical smelting center (Kowalski et al, 2017; Kądziołka et al, 2020), suggesting that in the past, the ore was nearer the surface and more available for mining.
6 Methods applied
To answer the questions about the provenance of the raw material we applied a variety of methods including analysis of historical evidence of pre-modern mining and excavations at the mining sites, and comparison of lead isotopic analyses determined for artefacts found near the copper outcrops in Silesia with European lead isotope databases. The lead isotopic data for copper ores (the Zechstein Limestone Ca1 mineralization belonging to the Zechstein Copper-bearing Series) from a local mine and modern black copper from local blast furnaces were analysed as reference data. The data were produced under several research projects and partly published (Nowak et al, 2022b; 2023a; 2023b) but have never been interpreted in a complete and coherent way.
6.1 Archaeological excavations and field survey
Based on the information available in the literature, DTM LIDAR analyses and field surveys, surface prospection with metal detectors, we conducted preliminary archaeological excavation in the Leszczyna historical mining region in the Kaczawskie Foothills. The excavations were aimed at verifying the chronology of the mining shafts and an attempt to determine the beginning of copper exploitation from copper-bearing marls in this region of Lower Silesia. It is assumed that the mining began in the Middle Ages, although, there are no appropriate data from the field works.
6.2 Chemical analysis
6.2.1 Electron probe microanalysis (EPMA)
The chemical composition of 28 samples (nos. 1–26, 30, 32) was determined at the Polish Geological Institute—National Research Institute in Warsaw using a Cameca SX-100 electron microprobe (EPMA). The amount of metallic material of sample no. 31 was too small for testing, therefore it was excluded from further analyses. Metal samples were obtained using a micro-drill and HSS drills with a diameter of 1–2 mm. Drilling chips or small pieces up to 1.0 mm in length were mounted in epoxy resin in 1-inch discs. Samples were then polished with diamond paste and carbon coated to obtain electrical conductivity. Detailed photographs were taken, using both optical and scanning electron microscopes. Analytical conditions of the EPMA were set to 15 kV of accelerating voltage and 20 nA beam current. The diameter of the electron beam (spot size) was set to 50 µm (samples 1, 3, 4, 5, 18, 19, 20, 24, 25) and 10 µm (2, 6, 7–17, 21, 22, 23, 26, 30, 32), depending on the available homogeneous area of the samples. Due to a variable composition at the µm-scale, approximately 20 areas (15–22) were analysed per each sample.
The following standards used and corresponding X-ray lines were used: Si Kα wollastonite, Al Kα orthoclase, S Kα pyrite, Ca Kα wollastonite, Ag Lα hessite, Sb Lα InSb, Sn Lα cassiterite, Cl Kα vanadinite, Bi Mβ Bi2Se3, Pb Mβ galena, Zn Kα ZnS, Se Lβ ZnSe, As Lβ FeAsS, Cu Kα CuFeS2, Ni Kα Ni metal, Co Kα Co metal, Fe Kα pyrite, Mg Kα diopside, Au Mα Au metal, Hg Mβ cinnabar.
6.2.2 Scanning electron microscopy with energy dispersive X-ray spectroscopy (SEM-EDS)
One sample of black copper from the modern blast furnace from Leszczyna (no. 27) and two samples (nos. 28–29) from the residues found inside the mould from grave no. 5 in the cemetery in Legnica, Spokojna str., were prepared and several analyses using SEM-EDS were undertaken. Tests of samples were carried out with an EDS spectrometer (Oxford Instruments) coupled with a Hitachi Tabletop TM4000 Scanning Electron Microscope, using 15 kV excitation energy and selecting test sites using from × 30 to ×300 magnification of the samples. The research was carried out in the Laboratory of Archaeometry and Conservation of Archaeological Artefacts of the Institute of Archaeology at the University of Wrocław.
6.2.3 Inductively coupled plasma mass spectrometry (ICP-MS)
Samples S1-S10 were analysed at the University of Warsaw Biological and Chemical Research Centre to determine their elemental composition. They were weighed and treated with an appropriate mixture of acids (4.0 mL 65% HNO3, Merck Suprapur®, 0.5 mL 40% HF, Merck Suprapur®). Next, the samples were digested in a closed microwave system (Milestone Ethos Up, 25 min ramping to 230°C, 15 min hold at 230°C). The resulting solutions were diluted by weight to about 50 g and subjected to elemental analysis. All solutions were measured by ICP MS (Perkin Elmer NexION300D). External calibration (Merck ICP Standard VIII, Merck Sn, Sb and Ti monoelemental ICP standards) with internal standard (Rh) correction was used. The accuracy of the calibration has been verified using reference materials SPS SW1 and SPS SW2 (Spectrapure Standards, Norway).
Supplementary Table S1 shows the selected measurement parameters, along with a list of monitored isotopes.
6.2.4 Inductively coupled plasma optical emission spectrometry (ICP-OES) and inductively coupled plasma mass spectrometry (ICP-MS) for marl and shale samples
Major and minor elements as well as total C and S of the marl and shale samples were measured in the Bureau Veritas Mineral Laboratories (BVM—ACME Lab, Canada) for another study using this rock material for another purpose (Derkowska et al, 2023). A previously prepared subsample was subjected to crushing and pulverizing followed by lithium borate (LiBO2/Li2B4O7) fusion and nitric acid dissolution. For major and minor elements, inductively coupled plasma optical emission spectrometry (ICP-OES) and inductively coupled plasma mass spectrometry (ICP-MS) were applied, respectively. Carbon and sulfur concentrations were measured via LECO combustion analyses.
For accuracy estimation, laboratory certified reference samples were used as well as replicate analyses for reproducibility calculations. The analytical reproducibility (2σ) was counted based on one replicate analysis and estimated at 0.4% for major and minor elements. Analytical accuracy (2σ) was estimated based on six certified standards: STD GS311-1, STD GS910-4, STD DS11, STD OREAS262, STD SO-19 and reached 2.6% for major elements, 6.7% for minor elements and 4% for C and S. Additionally, mineral compositions were determined with petrographic observations and SEM-EDS analyses conducted in the Polish Geological Institute-National Research Institute.
6.3 Pb isotope compositions
Lead isotope compositions for metal samples 1 to 30 and 32 (31 was excluded) were determined using material prepared as described in Section 6.2.1.; samples 33–36 (marls, shales) were submitted as small chips. The samples were analysed by multicollector-inductively coupled plasma mass spectrometry (MC-ICP-MS) at the University of Melbourne, Australia. Samples (1–241 mg) were weighed into Savillex PFA beakers and reacted with 7M HNO3 (2 mL, 120°C, 15 h). Cu-rich samples formed blue solutions with minor residue while marl/shale samples produced abundant residue. After drying, the samples were re-dissolved in 0.6M HBr, followed by Pb extraction on small (15 × 0.4 mm) beds of AG1-X8 (100–200 mesh) anion resin, using the HBr-HCl technique. Pb isotope ratios were measured on a Nu Plasma MC-ICP-MS with sample introduction via a low-uptake PFA nebuliser and Aridus desolvator. Data were collected using total Pb signal of 10–12 V, and instrumental mass bias was corrected using thallium doping (Woodhead, 2002). The thallium doping technique is expected to yield iPb/204Pb results with an external precision of 0.04%–0.08% (2std dev). This is confirmed by the results for the SRM 981 Pb standard, analysed as unknowns in the same sessions. Seven analyses of Pb extracted from the BCR-2 reference basalt show greater variability, but the average agrees with the GeoREM-preferred composition (http://georem.mpch-mainz.gwdg.de) and is consistent with the long-term laboratory average and with TIMS reference data.
Isotopic data for samples S1-S10 were acquired at the University of Warsaw Biological and Chemical Research Centre. MC-ICP-MS Operating Parameters for this are in Supplementary Table S2. Samples were digested as described in Section 6.3. Sample 8 could not be analysed because the small amount of sample available has only 3 ppm Pb. Isotopic data were obtained on a Nu Plasma 3 MC-ICP-MS equipped with an Aridus-3 desolvator and a plasma shield. The Pb-rich samples (>880 ppm) were analysed without Pb purification and aspirated into the desolvator after dilution to ∼30 ppb Pb with 2% HNO3 run solution doped with SRM997 thallium (∼15 ppb TL).
7 Results
7.1 Archaeological excavations and field survey
The area of research was selected through a field survey conducted within the site and an earlier analysis of DTM LIDAR data available at www.geoportal.gov.pl. On the basis of the conducted queries, it was assumed that the objects in the form of Pingen, located in the eastern part of the site, above the shafts area of the Character Mine, may be the oldest remnants of mining works carried out here. Shafts and mining heaps located in the northern part of the site were selected for research (Figure 6A). We assumed that, in the case of prehistoric mining activities in this region, older mining structures could have been largely destroyed by younger activities as suggested in the literature (comp. Gedl, 1986).
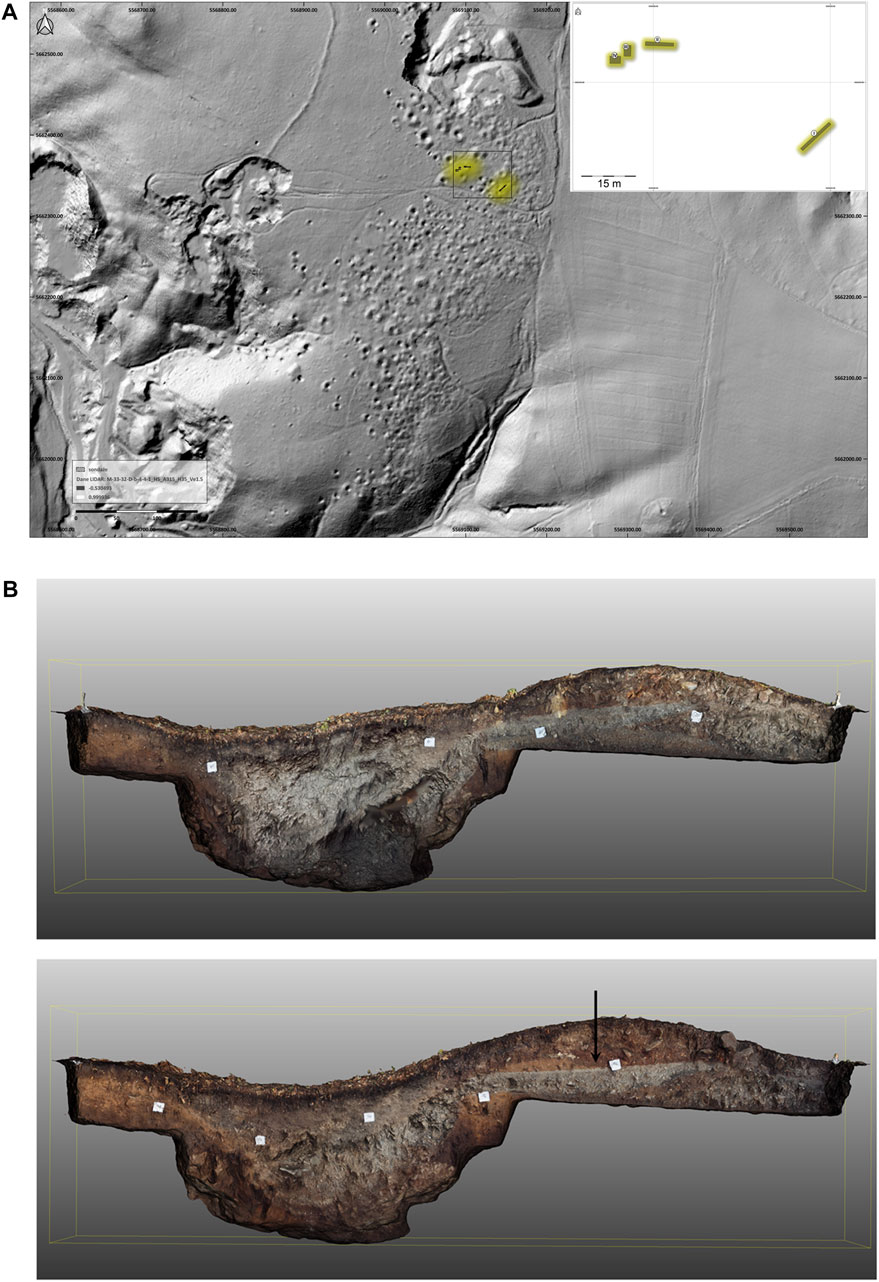
FIGURE 6. DTM LIDAR map of the Leszczyna minig area. The trenches of archaeological excavations from 2019 are marked in yellow. Map compilation by P. Noszczyński and T. Stolarczyk (A); Leszczyna mining area, the trench no. I/2019: 3D model of the explored surface with sections and profiles. A horizontal construction marked with an arrow. Compilation by P. Noszczyński and T. Stolarczyk (B).
In 2019 we opened four trenches of total area 50 m2 on three Pingen and surrounding heaps (Figure 6A). Among fragmented rocks in one trench 11 pottery shards were found. The composition of the ceramic paste and decoration is typical for sites dated 15th and early 16th centuries (Pankiewicz, Rodak, 2016, 333-334; Łesiuk, 2019). Accordingly, in two trenches, a layer of finely fragmented marl suggests a presence of a horizontal construction (Figure 6B), which we assume to be of younger date.
Another three trenches, although shown similar stratigraphy, did not produce any artefacts therefore the question of their chronology remains open.
7.2 Chemical composition
7.2.1 Electron probe microanalysis (EPMA)
Chemical compositions of analysed samples (Table 2) show significant variations, both in major (Cu, Sn) and trace elements. Copper, being an obvious major element, constitutes from 85%–86% (bronze artefacts from the chronological group 4) up to 96%–99% (copper artefacts from group 2). Tin content in bronze artefacts varies within the range 3.3%–10.8%. Sulfur content is generally below 1%, with the exception of some copper samples from Group 2 and two single samples from other groups (higher content of impurities). Lead content is mostly below 0.1%, with only samples 12, 18 (Group 4) and 32 (modern) containing more than 1%.
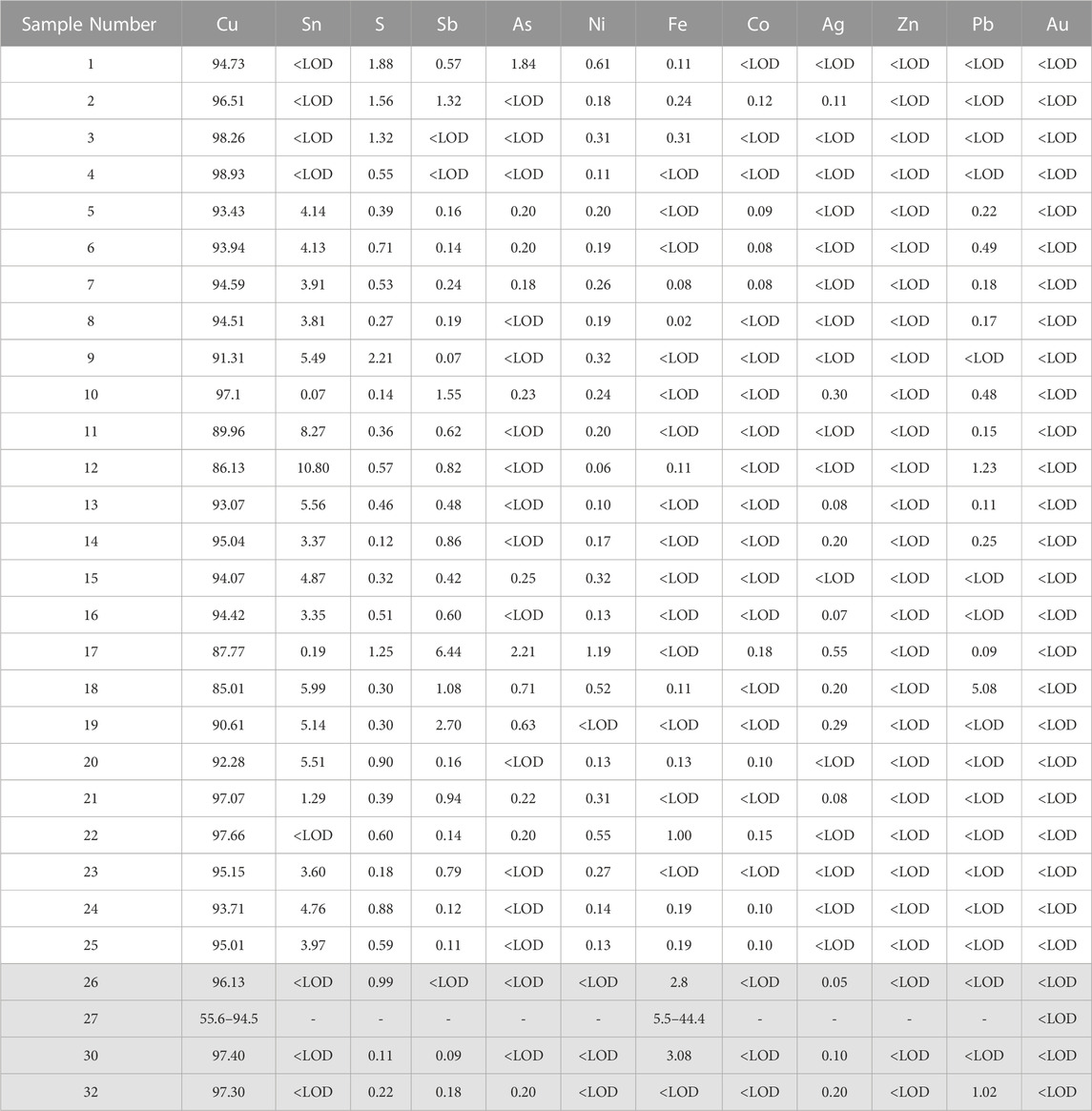
TABLE 2. Chemical composition (EPMA). Results are in weight %, averaged from ∼20 point analyses per sample. Sample no. 27 was analysed using SEM-EDS at the Institute of Archaeology University of Wrocław without mounting in epoxy resin and polishing. The modern black copper samples are marked (in grey).
Trace elements content was considered in order to distinguish possible groups which could correspond with chronology or a possible common raw material provenance. Sb, Ag and Ni contents were plotted (Figure 7) relative to the corresponding chronological groups. Plots with As were excluded as its distribution appears to be random. Chronological group 2 covers a wide area on all diagrams, showing varying composition, with other groups (1, 3 4) fitting mostly inside of this area. Samples from Group 1 are very close to each other, providing a strong clue to their common origin. Groups 3 and 4 both are more widespread, but it should be noted that their average trace element content (especially Sb) is distinctly higher than that of other groups. It is clearly visible that samples from modern times stand out from the main trend; while most archaeological samples have relatively constant Ag/Sb ratio, modern samples stand out clearly.
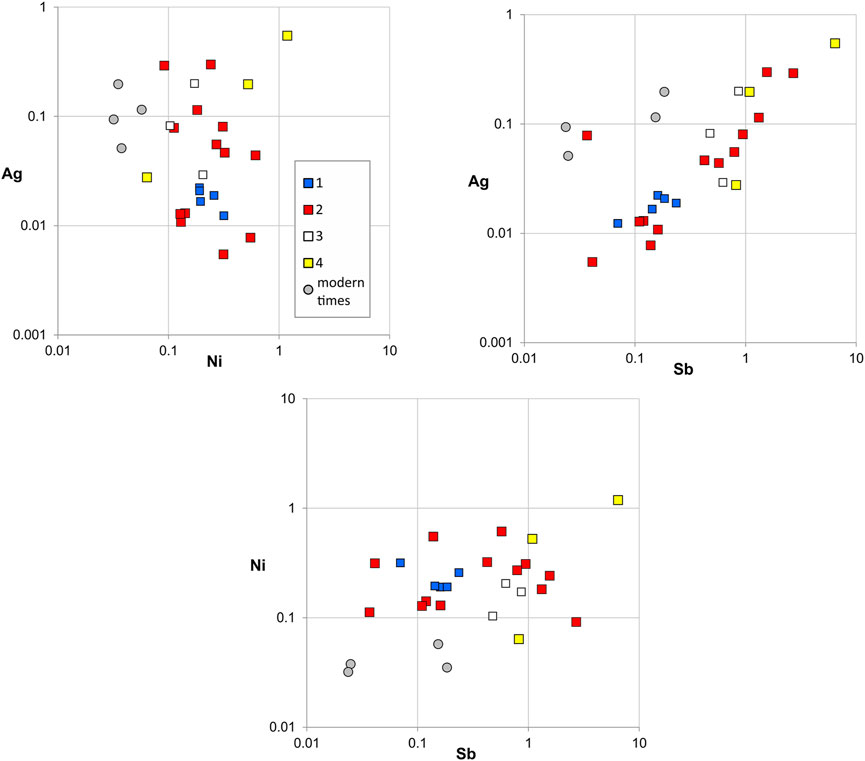
FIGURE 7. Diagrams showing relations between trace elements (Ag, Sb, Ni) of chronologically different groups of artefacts, in logarithmic scale; values are in weight %.
A variety of textures were observed in SEM-BSE images, showing not only different impurities and/or melt droplet in bulk material, but also a heterogeneous composition of the main bronze alloy (effects of imperfect technology). Detailed analysis of these features was not a purpose of this study, but some observations are worth mentioning. Samples 1, 2 and 19 (chronological group 2) contain very similar impurities—up to 50 um in size, probably pieces of unmelted ore. Samples from Group 1 are very similar, with homogenous texture and impurities of constant compositions, which fits well with their trace elements composition. Samples 17 and 18 (Group 4) have significantly higher content of impurities than other samples, these impurities are also of varied composition. Samples 10, 11, 14, 19, 20, 21, 23, 24 and 25 show a visible heterogeneous texture of more or less parallel bands/streaks. Modern samples (black copper, slags) have very variable composition depending on which small piece was analysed, so it should be noted that the adopted method (EPMA) seems to be inadequate in their case.
7.2.2 Scanning electron microscopy with energy dispersive X-ray spectroscopy (SEM-EDS)
The analyses were aimed at determining the composition of the layers inside the casting moulds for sickles and chisels (nos. 28 and 29). The analytical results are semi-quantitative. The small size of the samples obtained from the mould cavities prevented their suitable positioning in the resin block in order to obtain uniformly flat surfaces. The results are presented in Supplementary Table S3. The lead contents in the samples taken for analyses from scrapings from the moulds was confirmed by the results obtained at the University of Melbourne, when dissolving the aliquots for lead isotope analyses (Nowak et al, 2022b, 10).
7.2.3 Inductively coupled plasma mass spectrometry (ICP-MS)
Table 3 presents the results of determining the total content of selected elements (samples S1-S10). The results for lead were calculated as an average of results obtained by monitoring 208Pb, 207Pb and 206Pb signals. For the other elements, the table shows which isotope was used to calculate the total content. The results are expressed in mg/kg dry weight. They take into account all dilutions and apply only to the objects submitted to the laboratory.
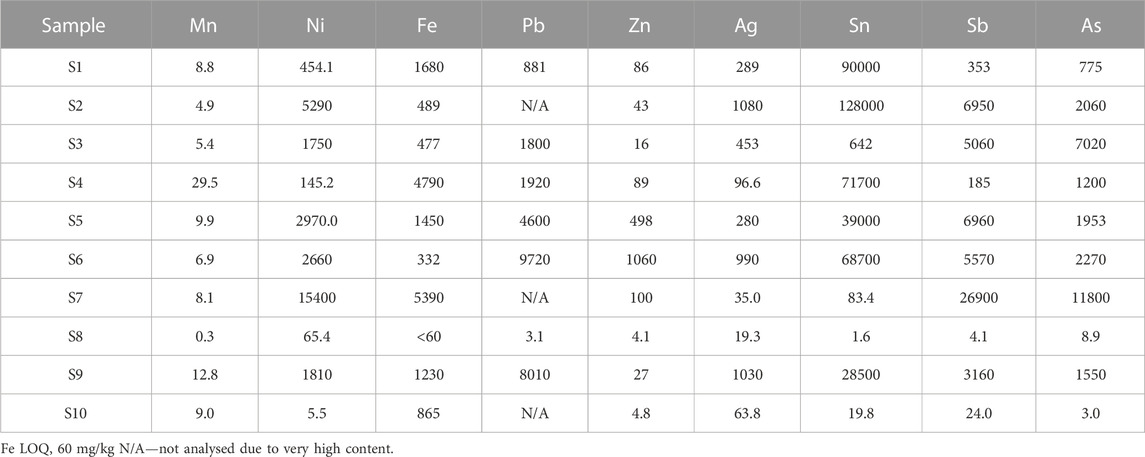
TABLE 3. The table shows the total contents of selected elements. The results are presented in mg/kg. They refer to the dry weight of the samples and take into account all dilutions. Cu was not analysed as it is a the main component of the investigated objects (Cu and Pb content is available in Garbacz-Klempka, Rzadkosz, 2014, Tabela 1).
7.2.4 Results of chemical composition of marl and shale samples (ICP-OES and ICP-MS)
Analysed marls and shales differ significantly in chemical composition (Supplementary Table S4). Compared to marls consisting of 0.17–0.73 wt% Cu, shales are more Cu-enriched with 1.3–2.3 wt% Cu. Other ore elements, lead included, reach significantly lower concentrations, usually below 100 mg/kg. The concentrations of such elements as Zn, Ni, As and Ag is much higher in shales than marls. Sb, Bi and Au do not significantly differ between two types of rocks, but there is slightly higher concentration in shales (in the case of Bi more significant than in Sb and Au).
7.3 Lead isotope analysis
In Table 4 we present the results of isotopic measurements by multicollector ICP MS for samples of archaeological artefacts, modern black copper and samples of marls and copper-bearing shales from the Stilles Glück Mine in Leszczyna. The table contains the mass of the sample available for investigation as well as the measured total lead content.
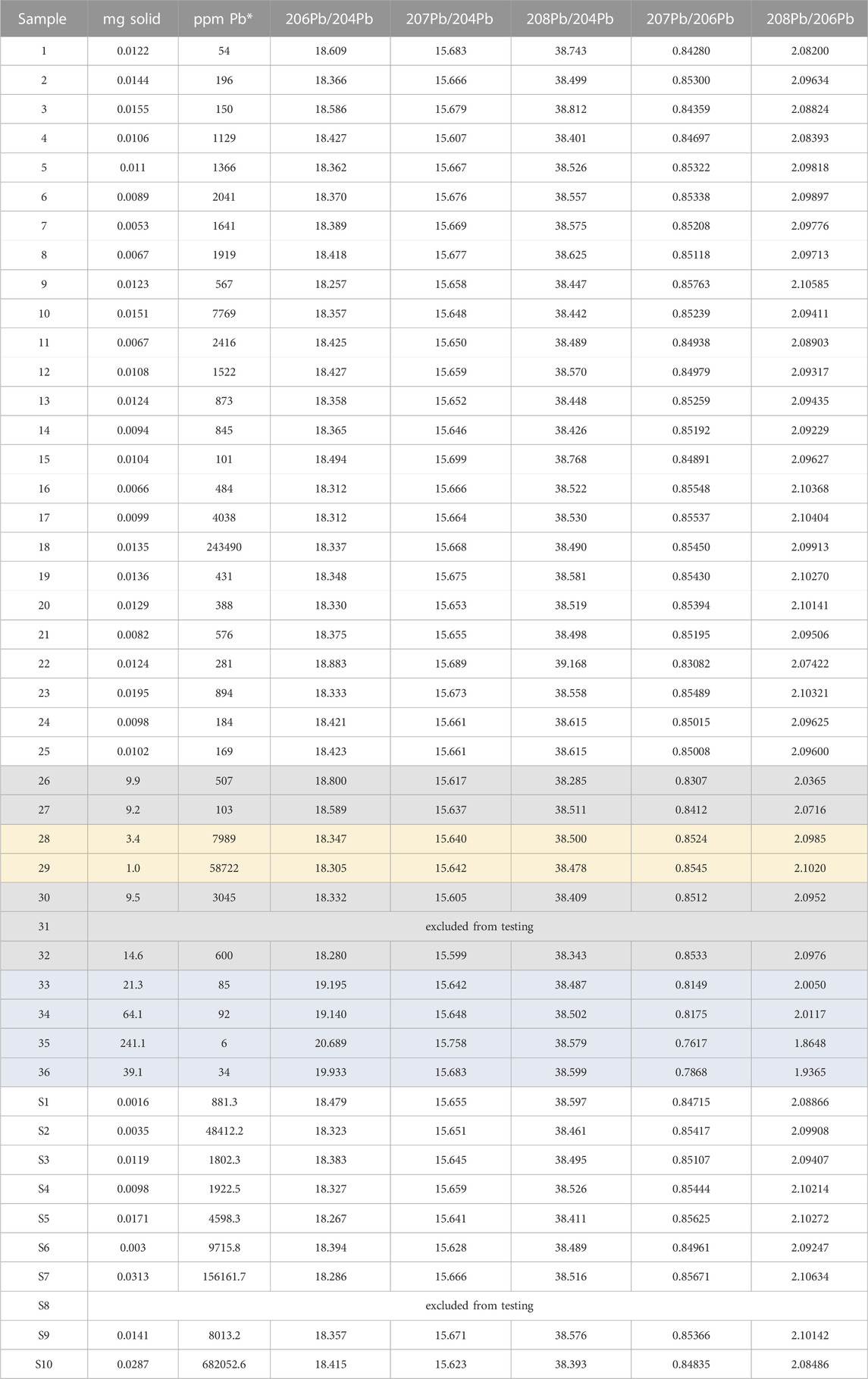
TABLE 4. Lead isotope analysis for artefacts and black copper (in grey), residues from the casting moulds (in orange) and marls and shales from the Stilles Glück Mine in Leszczyna (in blue). ‘mg solid’ is the total sample weight; ‘ppm Pb*‘ is a concentration estimate for Pb released from these samples during nitric acid treatment (based on signal size during Pb isotope analysis, total dilution, and the dry sample weight).
8 Discussion
The preliminary field research of the three shafts (Pingen) and their vicinity that we conducted did not prove their prehistoric origin, although the discovery ceramics dated to 15–16th centuries BC in the spoil heap of shaft no. I/2019 generally confirms their early date. We must emphasize that only a small area was studied, and that future research should attempt to study a much larger range. It may then be possible to recognise stratigraphical relations between objects, as well as possible identification of the background related to the preliminary preparation of ore.
For the discussion of the possible provenance of the raw material, the analysed artefacts are considered in four broad chronological groups. The IBERLID and OXALID databases (http://oxalid.ox.ac.uk; https://www.ehu.eus/ibercron/iberlid; Garcia de Madinabeitia et al, 2021) and other sources were used to interpret the lead isotope results.
The provenance of metal from the first chronological group (1200–1000 BC).
In this group there are four bracelets from Bogaczów and Wądroże, and a palstave from Wilków that is of a Bohemian type. The bracelets from Bogaczów (nos. 5 and 6) have identical chemical and lead isotope compositions, which is also the case for the pair of bracelets from Wądroże Wielkie (nos. 7 and 8). All four bracelets have geochemical characteristics consistent with the ores from the Italian southeastern Alps in the region of Trentino-Bolzano (Artioli et al, 2016). The axe from Wilków also seems consistent with the ores from this region (Nowak et al, 2023a), but its lead isotope composition is also consistent with the ores from the very rich and extensively mined region of Jaen in south east Spain (Lillo, 1992; Stos-Gale et al, 1995; Marcoux, 1998; Santos Zaldeuegui et al, 2004; Tornos and Chiaradia, 2004; Klein et al, 2009). The lead isotope compositions of the ores and these artefacts are compared on Figures 8A,B. The presence of copper from the Italian southeastern Alps and probably from the southeastern Iberia in western Poland at the end of the second millennium BC is not so surprising, as already discussed in our previous papers (Stos-Gale, 2019; Nowak et al, 2023b).Tin bronze with copper from the mines in the Italian Alps and, possibly, from southern Spain, was widely used in the second half of the second millennium BC (Artioli et al, 2020; Nørgaard et al, 2021). Bronze with these geochemical characteristics was most common among the finds dated to this period in Scandinavia (Melheim et al, 2018; Ling et al, 2019), but was also found in Serbia (Molloy, 2019; Gavranović et al, 2022) and in Bulgaria (Varbitsa hoard of sickles: Nowak et al, 2023b). Therefore, it is possible that bronze of western Mediterranean origin could find its way to the sites not far east from the river Odra, either from Scandinavia, or from the south, from the Balkans.
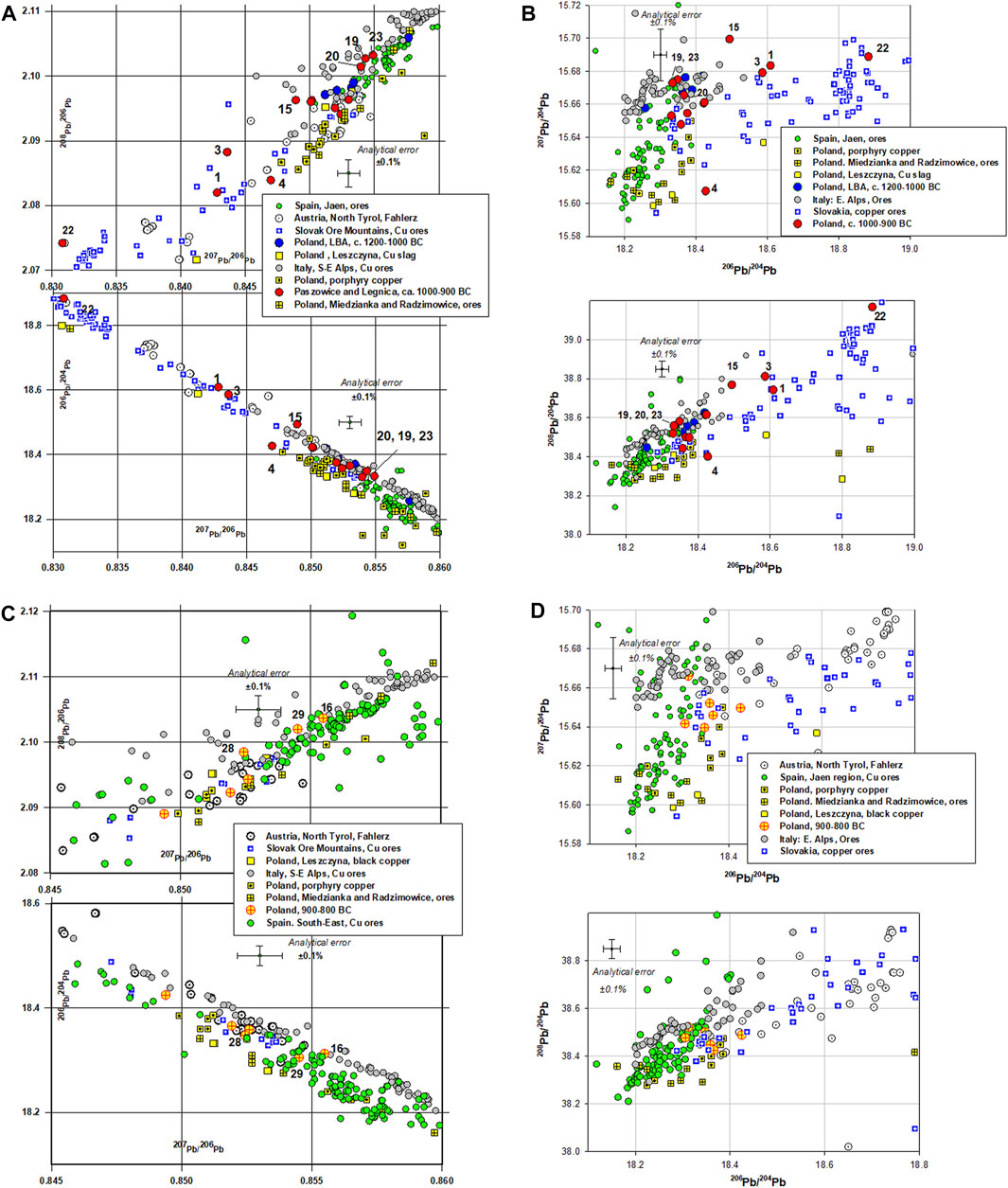
FIGURE 8. Comparison of lead isotope ratios to 206Pb of the copper-based metals from the sites in south-west Poland dating to 1200–900 BC with the ore deposits consistent with their geochemistry. The data for the copper ores from Poland includes data published by Church et al (1996) for porphyry copper from Myszków in Silesia (south-west Poland) that is currently regarded as an important copper mine. However, the copper from this deposit most likely could not have been exploited in Prehistoric times, because the ore is only found at a considerable depth (A); Comparison of the same samples of ores and artefacts as shown on A but in respect to the non-radiogenic isotope 204Pb. This aspect of the 3-dimensional lead isotope characteristics of these samples confirms the consistency of the analysed copper-based metals dated to 1200–900 BC with the ores from Slovak Ore Mountains and South Eastern Italian Alps from the mining sites in the region of Trentino-Bolzano. On this figure the difference between the copper ores from south-west Poland and the prehistoric metals is clearly visible. No. 4 seem to have lead isotope ratios close to the ores from Poland, but on A its lead isotope ratios are not consistent with these ores, but with the ores from the Slovak Ore Mountains (B); The comparison of lead isotope ratios of the copper-based metals dated to 900–800 BC with the ores from south-east Spain shows the consistency of two of them with the ores from South-east Spain in the region of the town in Jaen and further in the mountains of Los Pedroches and in the Alcudia Valley. These copper-lead and silver ore deposits have been exploited throughout the Bronze and Iron Ages and then during the Phoenician and Roman periods, as proven by extensive research in the last 40 years (C, D). Lead isotope data after: Wedepohl et al, 1978; Bielicki, Tischendorf 1991; Church, Vaughn 1992; De Vleeschouwer et al, 2009; Nimis et al, 2012; some data for Kupferschiefer as private communication from E. Pernicka. References for ores from Tyrol, Slovak Ore Mountains, Italy, S-E Alps (Trentino-Bolzano) and Spain are in the text.
Figure 8A,B show also the lead isotope ratios measured for the copper ores from occurrences in south west Poland which have been exploited in the last few hundred years, and samples of modern blister copper from the smelter in Leszczyna (18th century). Based upon the lead isotope and chemical evidence these copper minerals could not have been used as a source of copper for any of the artefacts analysed for this project.
The provenance of metal from the second chronological group (1000–900 BC).
The largest group of metal artefacts found in Poland dated to this period comes from the metal hoard found in Paszowice in the extreme south-west of the country. Twelve artefacts from this hoard have been analysed for their lead isotope and chemical compositions and the possible origin of the metal from this hoard has been discussed in detail in our previous paper (Nowak et al, 2023b). The analytical results show a large diversity in the origin of these bronzes and fragments of copper ingots, as can be seen of Figure 8A,B. Pure copper fragments (nos. 1, 3, 4 and 22) are isotopically and chemically consistent with the an origin from the Slovak Ore Mountains (nos. 1 and 4) (Schreiner, 2007), Eastern Alps (no. 22) (Pernicka et al, 2016; Tables 3, 4), and Sardinia (no. 3) (Boni and Koeppel, 1985; Boni et al, 1992; Stos-Gale et al, 1995; Begemann et al, 2001). The sickle (no. 24) and the casting jet (no. 25) have metal compositions consistent with the Sardinian ores, while the sickle no. 21 is consistent with ores from North Tyrol, Austria (Höppner et al, 2005) or Slovak ore Mountains, as is the copper lump no. 2. The socketed axes nos. 19 and 20, and the tanged sickle no. 23 have the geochemical characteristics of the ores from the region of Jaen in south-east Spain (Lillo, 1992; Stos-Gale et al, 1995; Klein et al, 2009; Santos Zaldeuegui et al, 2004a; Marcoux, 1998; Tornos and Chiaradia, 2004). On Figure 8A and Figure 8B the comparison of the lead isotope data for these three bronzes (19, 20 and 23) looks superficially very similar to the data for the ores from Trentino-Bolzano in the Italian Alps. However, their lead isotope compositions are within 1 analytical error for all ratios from the ores from the region of Jaen in southern Spain, not with the ores from Italy. Additionally, two of these artefacts contain quite significant amounts of antimony (19%—2.7% Sb, 23%—0.79% Sb). According to several of the publications of the research group from Padova lead by Gilberto Artioli, antimony is not commonly found in this quantity in the ores in the copper mines in this region. On the other hand according to Galan Huertos and Mirete Mayo (1979, 171) ores containing antimony appear frequently with chalcopyrite and malachite in the Spanish province of Jaen. Therefore in this case we propose that the geochemical characteristics of these three artefacts are consistent with the ores from this province.
Additionally two small metal finds from Legnica, Spokojna street, are included in this group: a metal droplet from a casting mould (no. 10) and a pin head (no. 15). Both of these finds are consistent chemically and isotopically with the ores from the deposits in North Tyrol, Austria, but also with possibly with the ores in Slovakia and Erzgebrige.
On Figure 8B the plot of ratios 207Pb/204Pb/ and 206Pb/204Pb show quite clearly that none of the metals dated to the period 1200–900 BC analysed for this research are consistent with the geochemistry of the Polish copper ores.
The provenance of metal from the third chronological group (900–800 BC).
The group of analysed bronze artefacts dated to Ha B2-Ha B3 (900–800 BC) consists of one razor, residues from two casting moulds and a pin head from the graves excavated at Spokojna Street in Legnica (nos. 11, 16 and nos. 28–29), and two razors from the cemetery in Dunino (nos. 13 and 14). The six samples from these artefacts are tin-copper alloys with antimony content above 0.5% and significant lead content in case of the samples from the moulds. The lead isotope compositions of these metals are varied and indicate that not just one source of copper was used in this region at this period (Figures 8C,D). The lead in the corroded metal remains in the sickle mould no. 29 and the low lead tin bronze pin head no. 16 are isotopically consistent with the ores in the region of Linares in south-east Spain. The lead in the other mould in a shape of a chisel (no. 28) has a different lead isotope compositions, indicating the origin of copper from younger ores (on Figure 8C 207Pb/206Pb <0.853). This metal, and the other four artefacts belonging to this chronological group, all have lead isotope compositions falling in the range of the ores from North Tyrol, Austria and the ores from the Slovak Ore Mountains. The presence of antimony in all these bronzes is also consistent with the geochemistry of the copper ores in both these regions. On Figure 8C it seems that some of the artefacts have similar lead isotope ratios to the ores and black copper from Leszczyna, but again, on Figure 8D these similarities disappear, additionally, analyses of these copper ores and black copper found antimony in quantities well below 0.1%.
The metals and residues from casting moulds from the cemetery on Spokojna Street in Legnica have been analysed some time ago and a paper discussing their possible origin has been published (Nowak et al, 2022b). In this paper we concluded that four finds from this site (nos. 10, 11, 28 and 29) are possibly consistent with the ores from the Erzgebirge in south-east Germany and Western Czechia. As it can be seen on Figure 9A,B their lead isotope ratios are not unambiguous: they indeed fall within the range of the lead and copper ores from the Erzgebirge (Freiberg, Kirchberg and Olovi), but also with the ores from the Inn Valley in North Tyrol, Austria and with the ores from the Slovak Ore Mountains. These last two mineralisations contain also minerals with a high antimony, which is also present in high quantity in the residues from the moulds 28 and 29. Lead in these two residues is in the range 3%–5%, so the lead isotope ratios indicate perhaps copper ores that occur with galena, or was added to copper and in this case the lead isotope ratios only indicate the origin of lead. But the tin content is very high, in excess of 14%. In the Erzgebirge there are occurrences of cassiterite that might have been exploited in the Bronze Age as suggested by Mason et al (2020). In this paper they state that: ‘. composition artifacts that occur north of the Middle Danube in Vojvodina, Transylvania, and Central Europe are likely associated primarily with ores from the West Pluton of the Erzgebirge.’ The cassiterite in the Erzgebirge is not associated with copper and lead ores (Baumann et al, 1986, 315–319), but if the copper alloy was imported from the south-east (Slovakia) or from the Austrian Alps, it is possible that the high tin content indicates that local tin from the Erzgebirge was available. This statement is controversial, since there is no direct evidence of Bronze Age mining of any of the ores from the Erzgebirge in the Bronze Age. However, this hypothesis should be taken into account and awaiting further research.
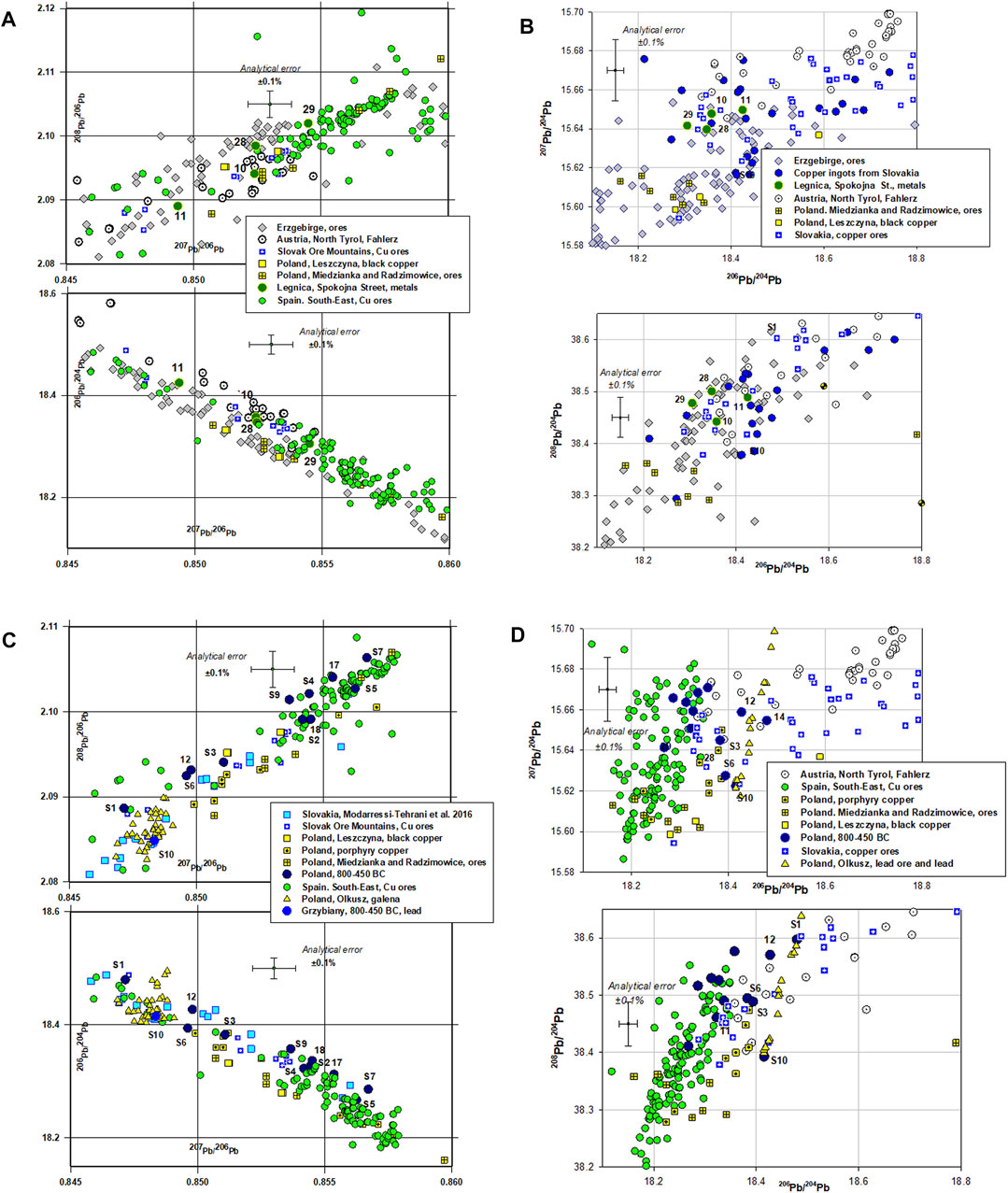
FIGURE 9. The comparison of lead isotope ratios of the copper-based artefacts from Legnica, Spokojna Street, that have geochemical characteristics consistent with the lead and tin ores from the Erzgebirge (A, B); The comparison of lead isotope ratios of the copper-based artefacts from Legnica, Spokojna Street, that have geochemical characteristics consistent with the lead and tin ores from the Erzgebirge. Comparisons of the lead isotope ratios of the copper-based metals dated to 800–450 BC from south-west Poland with the ores from the Slovak Ore Mountains and south-east Spain show the wide distribution of the lead isotope ratios of these metals and the isotopic consistency of some of them with the copper bars found in the Slovak Ore Mountains. The piece of lead found in Grzybiany is fully consistent with lead from the mine in Olkusz in Silesia (C, D). Lead isotope data after: Wedepohl et al, 1978; Bielicki, Tischendorf 1991; Church, Vaughn 1992; Niederschlag et al, 2003; De Vleeschouwer et al, 2009; Nimis et al, 2012; unpublished Isotrace, Oxford data for Olkusz; some data for Kupferschiefer as private communication from E. Pernicka. References for ores from Tyrol, Slovak Ore Mountains, Italy, S-E Alps (Trentino-Bolzano) and Spain are in the text.
The metal residue from the mould no. 29 show more convincing consistency with the ores from S-W Spain.
The provenance of metal from the fourth chronological group (800–450 BC).
The group of analysed metals dating to the period 800–450 BC (Early Iron Age) consists of 11 fragments from the settlement of Grzybiany near Legnica and a surface find of a bracelet from the settlement of Prusice near Złotoryja. All these artefacts have quite varied elemental compositions. The bracelet from Prusice (no. 12) is made of a good tin bronze, with over 10% of tin with about 1% of antimony and 1% of lead. Another bracelet, from Grzybiany (no. S6) is also made of bronze with about 7% of tin, 0.5% of antimony, 0.2% of nickel and arsenic and nearly 1% of lead. These two bracelets have lead isotope compositions very similar to two copper bars found in the Slovak Ore Mountains near the Špania Dolina Piesky and published by Modarressi-Tehrani (et al, 2016): they are no. 947 (10% Sb, 0.5% Pb) and no. 951 (4.59 Sb and 0.3% Pb, but only 65% Cu). So it is possible that the metal for these artefacts came to Grzybiany from Slovakia (Figures 9C,D).
Three metal rods (nos. 17, S5 and S7) have similar lead isotope compositions characteristic of Precambrian ores, but quite varied chemistry. No. 17 has only 0.2% of tin, but over 6% of antimony, 2% of arsenic, 1% of nickel and 0.5% of silver—clearly this represents copper smelted from tetrahedrite mineral. The rod no. S5 is of low tin bronze (c. 4% of tin), with 0.7% of antimony, 0.2% of arsenic and a similar amount of nickel, 0.5% of cobalt and 0.5% of lead. All other elements are in quantities below 0.1%. The rod no. S7 is described as perhaps a fragment of a necklace or a bracelet. It was made of copper with nearly 3% of antimony, 1%–1.5% of arsenic and nickel and 15% of lead. This chemical composition represents copper smelted from a tetrahedrite ore, either mixed with galena, or with lead added to the copper when casting the artefact. In this case the lead isotope ratios can only be interpreted as to the origin of the lead component. The lead isotope ratios and geochemistry of these metals are consistent with the copper-lead ores in only one region that is known to have produced much of copper, lead and silver in the first millennium BC, the deposits along the Guadalquivir river and in the region of Jaen in south-east Spain (Tornos, Chiaradia, 2004; Saez et al, 2021). The variety of the chemical compositions of copper ores used in pre-Roman times in Spain is evident in the body of analyses published in a large volume some years ago, making clear that some ores containing antimony, silver and lead have been used for smelting copper in the final BA and the Iron Age (Rovira Llorens et al, 1997, 193, 201, 254–265).
The casting jet no. 18 is of 5% tin bronze and contains about 1% of antimony, and about 0.5% of nickel, but the 5% of lead make it only possible to say that this lead is fully consistent isotopically with the ores from the region of Jaen in south Spain. Very similar isotopically and chemically (12% Sn, 0.7% Sb, 0.5% Ni and 5% of Pb) is the pin no. S2. The other two pin heads (nos. S4 and S9) have also broadly similar chemistry and lead isotope compositions. So it seems that the pins and the casting jet contain lead consistent with the ores from southern Spain (Figures 9C,D).
The remaining two copper-based artefacts contain geochronologically younger lead. The fibula no. S1 is made of copper of high purity with 9% of tin and its lead isotope composition seems consistent with the ores from the mine of Calabona on Sardinia (Stos-Gale et al, 1995), or also from the Spanish mines in the region of Los Pedroches. The fragment of the socketed axe no. S3 is not tin bronze, but copper with about 0.5% of antimony and arsenic and 0.2% of lead. Its lead isotope and chemical composition is consistent with the ores from the Slovak Ore Mountains (Figures 9C,D).
Finally the piece of lead no. S10 has lead isotope composition fully consistent with the galena from the silver/lead mines in Olkusz, west of Krakow in southern Poland. So far this seems the earliest fragment of lead possibly originating from this mine found in the archaeological excavation. Later examples of lead from Olkusz include several pieces found in the second century AD site of Jakuszowice (Stos-Gale, 1993) and in the 15th c AD context in Krakow main market square (Stos-Gale et al, 2012). The lead isotope and chemical compositions of other analysed artefacts dated to the 800–450 BC do not indicate that lead from this mine was added to copper-based metal to make artefacts.
9 Conclusion
Our research shows, that the shale and marl samples from the local Stilles Glück Mine in Leszczyna (nos. 33–36) contain very little lead (between about 10 and 90 ppm; Table 4) and have radiogenic isotope ratios. The black copper fragments analysed as reference have a higher lead content than the analysed minerals (about 100–3000 ppm; Table 4), but this may be the result of the concentration of lead in the copper during the smelting process. Isotope ratios of samples from the Stilles Glück Mine and black copper smelted from local ores are not consistent with the isotope results obtained for archaeological artefacts discovered in the vicinity of the Zechstein deposits.
Also, excavations in Pingen fields indicated as a potential area of prehistoric mining did not provide evidence of local prehistoric mining. Early modern period pottery fragments found in one of the deep shafts indicate their younger chronology. As we have already pointed out above, in order to verify and confirm these findings, it is necessary to conduct further broad-based research.
Our research allowed us to identify the sources of the raw material which was used to produce objects discovered in the vicinity of the copper outcrops (Table 5; Figure 10). The lead isotope analysis showed that the raw material reached southwestern Poland in the Urnfields period from many directions. However, certain tendencies are visible for four selected chronological groups.
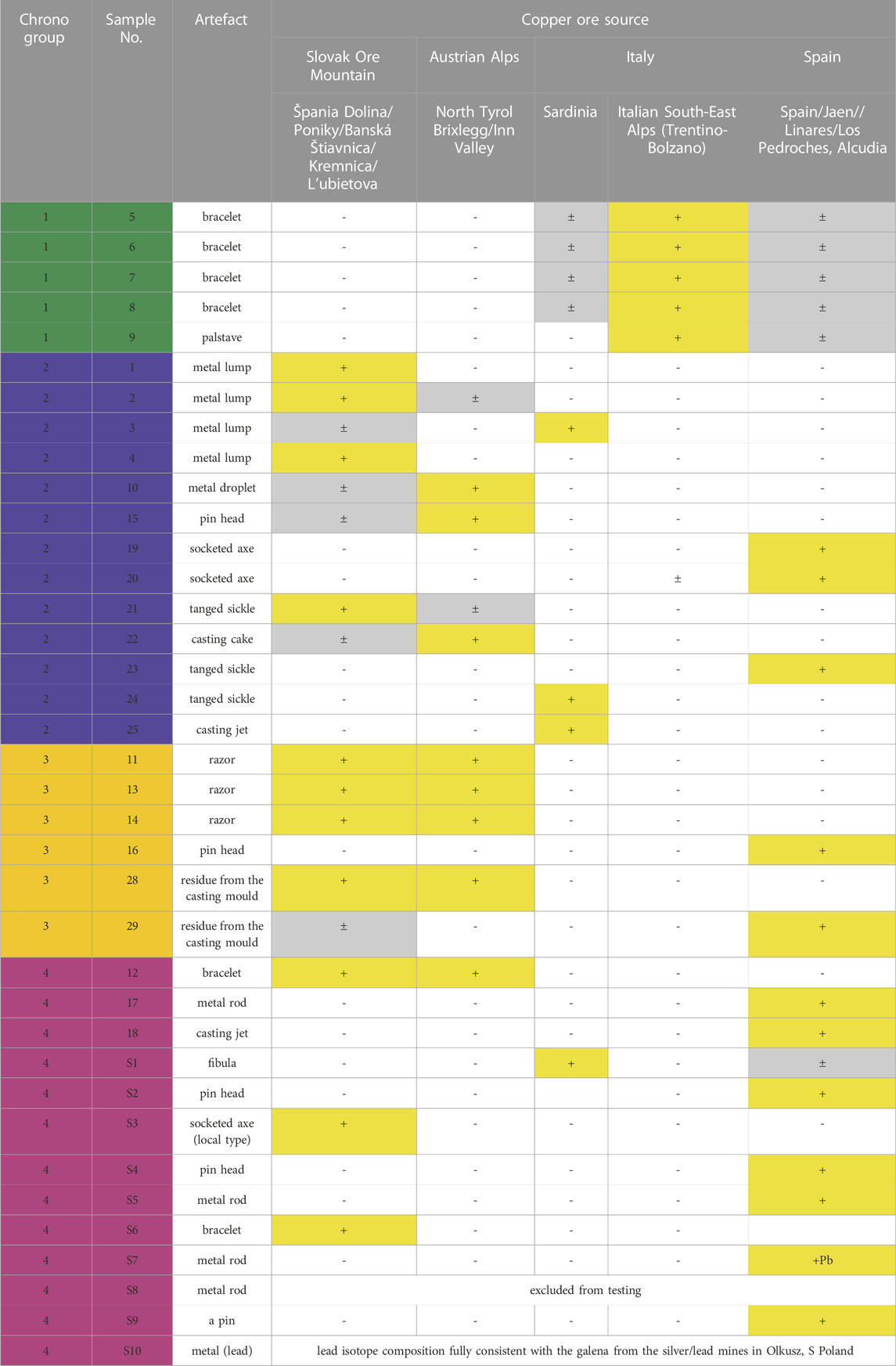
TABLE 5. A list of identified possible sources of copper and lead used to produce artefacts found in SW Poland. Yellow colour +—entirely consistent with the lead isotope and chemistry of the ores; grey colour +– - marginally consistent. Chronological groups are marked with different colours (according to the rest of the text).
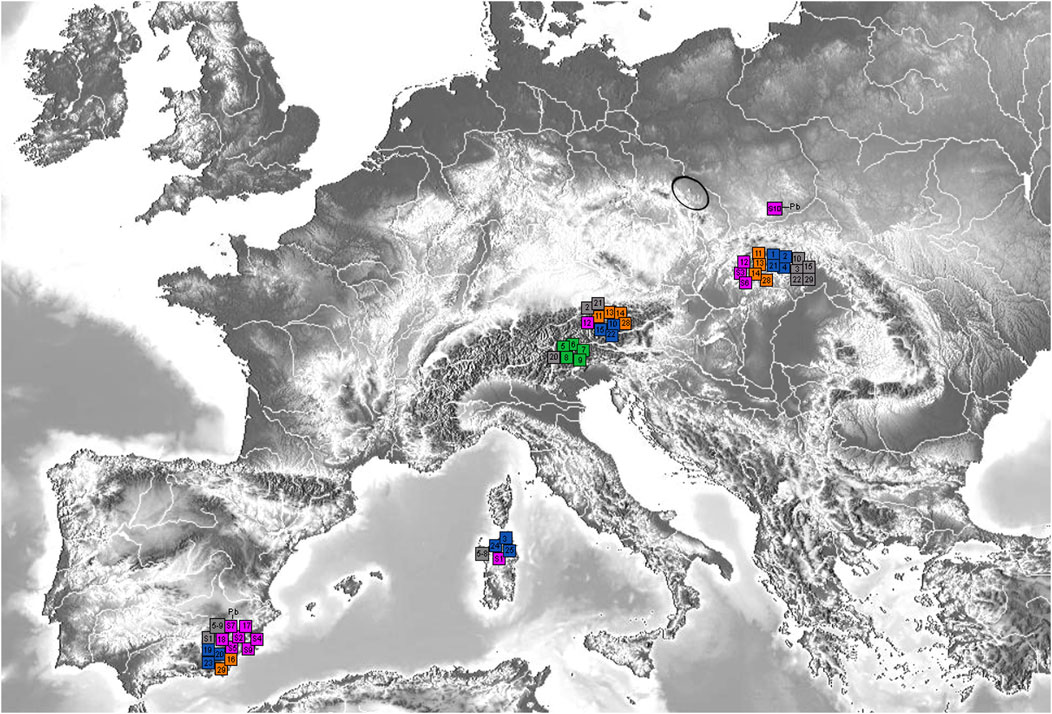
FIGURE 10. The map shows the identified sources of origin of the metal raw material in specific chronological groups consistent and marginally consistent (grey colour) with the lead isotope and chemistry of the ores. The colours of the squares (green, blue, orange and violet) indicate chronological groups and are consistent with the rest of the text. The black circle shows the area of the research.
In the first chronological group (1200–1000 BC), artefacts from three different contexts form a compact group regarding provenance determined based on lead isotopic composition. The metal used for producing, most likely, all the bracelets from the hoards from Wądroże Wielkie and Bogaczów and the axe from Wilków was obtained from the Italian Southeastern Alps (Trentino-Bolzano) ore deposits. There is a possibility that metal from the Alps reached SW Poland through intermediaries as demonstrated by the recently published by results of isotopic analyses of black copper deposited in the form of plano-convex ingots in the hoard from Staré Hodějovice (Kmosek et al, 2020). Local metallurgists in southern Bohemia may have been importing raw contaminated copper, refining it on the spot, and distributing it further north at the beginning of the Urnfields.
The second chronological group (1000–900 BC) is characterized by a significant diversification of the sources of the raw material used. At that time, based on the observations of lead isotopes, we confirmed the significant involvement of the local Lusatian Urnfield culture community in obtaining metal from various sources. The metal was hardly obtained directly from such distant sources as those from the Iberian Peninsula or Sardinia. This type of ‘exotic’ metal confirms the participation of Lusatian Urnfield communities in long-distance trade, even if they were only one of the subsequent contractors of this exchange.
In the third chronological group (900–800 BC), we see some trends again, manifested by more significant involvement in sourcing metal from closer areas. Two isotopically and chemically similar metal sources were determined for four samples. The direction from the Austrian Alps and the Slovak Ore Mountains are a convenient and close source supplying the surrounding areas and distributing metal north of the Carpathians and the Sudetes. For two objects—a pin head and metal used in the casting mould—metal from other areas was also identified. The metal from the Iberian Peninsula may indicate the constant participation of local communities in the long-distance exchange route, which we identify for artefacts from the second group.
The fourth of our chronological groups (800–450 BC) is mainly related to studying metal artefacts discovered in the Grzybiany settlement with confirmed intensive metallurgical activity in the Early Iron Age. Most of the examined artefacts are isotopically convergent with the deposits from the Iberian Peninsula. Based on our research, this is the main source of the metal influx in this period to SW Poland and supplying the local workshop in the settlement in Grzybiany. One of the rods, with high lead content, was also identified as originating from the Spain area. For the Certosa fibulae, the origin of the metal was found in Sardinia. It was likely imported from northern Italy, as the literature indicates. Metal from the nearby Slovak Ore Mountains was used to produce a local type of socketed axes, similar to a bracelet decorated with twisting and a bracelet discovered in the settlement in Prusice.
Results of lead isotope studies of a fragment of lead discovered in the settlement in Grzybiany are very interesting. This fragment has a lead isotope composition entirely consistent with galena from the mines in Olkusz in southern Poland. So far, it is the earliest lead fragment possibly originating from this mine found in archaeological excavations. It is also the first time that local exploitation of deposits of metallic raw materials in Poland can be confirmed.
Our research provides a whole new picture of the contacts of the Urnfield community in order to obtain metal. As our preliminary research indicates, the copper was not sourced locally.
Data availability statement
The original contributions presented in the study are included in the article/Supplementary Material, further inquiries can be directed to the corresponding author.
Author contributions
KN: Conceptualisation, Project administration, Funding acquisition, Investigation, Writing, Visualization. TS: Investigation, Visualisation. ZASG: Data curation, Writing, Visualisation. JB: Conceptualisation, Writing. KD, PD, BM, JW, JK and RM: Formal analysis, Investigation, Data curation, Writing. All authors contributed to the article and approved the submitted version.
Funding
This work received financial support from the National Science Centre, Poland (projects nos. 2017/27/N/HS3/01097 and 2021/40/C/HS3/00097). The work was also supported by Nicolaus Copernicus University in Toruń under the University Centre of Excellence programme.
Acknowledgments
We want to express our gratitude to the Director of the Regional Museum in Jawor, Arkadiusz Muła, and the Director of the Copper Museum in Legnica, Marcin Makuch, for making the artefacts available for testing. We would also like to thank Szymon Kostka, Michał Jóźków and Robert Nowakowski, which made it possible to descend to the Stilles Glück Mine in Leszczyna and collect samples. We would also like to thank Mariusz Łesiuk for his participation in field and exploratory research, as well as the members of the Historical and Exploratory Association of the Duchy of Legnica (Stowarzyszenie Historyczno-Eksploracyjne Księstwa Legnickiego) metal detecting group. We would also like to express our gratitude to the anonymous Reviewers for all valuable comments that allowed us to improve the manuscript.
Conflict of interest
The authors declare that the research was conducted in the absence of any commercial or financial relationships that could be construed as a potential conflict of interest.
Publisher’s note
All claims expressed in this article are solely those of the authors and do not necessarily represent those of their affiliated organizations, or those of the publisher, the editors and the reviewers. Any product that may be evaluated in this article, or claim that may be made by its manufacturer, is not guaranteed or endorsed by the publisher.
Supplementary material
The Supplementary Material for this article can be found online at: https://www.frontiersin.org/articles/10.3389/feart.2023.1184949/full#supplementary-material
References
Artioli, G., Angelini, I., Nimis, P., and Villa, I. M. (2016). A lead isotope database of copper ores from the southeastern Alps: A tool for the investigation of prehistoric copper metallurgy. J. Archaeol. Sci. 75, 27–39. doi:10.1016/j.jas.2016.09.005
Artioli, G., Canovaro, C., Nimis, P., and Angelini, I. (2020). LIA of prehistoric metals in the central mediterranean area: A review. Archaeometry 62, 53–85. Suppl. 1. doi:10.1111/arcm.12542
Bachmann, H.-G., Jockenhövel, A., Spichal, U., and Wolf, G. (2003). Zur bronzezeitlichen Metallversorgung im mittleren Westdeutschland: Von der Lagerstätte zum Endprodukt. Berichte der Kommission für Archäologische Landesforschung Hessen 7, 67–120. 2002/2003.
Bátora, J. (2003). Contribution to the problem of craftsmen graves at the end of Aeneolithic and in the Early Bronze Age in Central, Western and Eastern Europe. Slov. Archeol. 50 (2002), 179–228.
Baumann, L., Kolbel, B., Kraft, M., Lachelt, S., Rentzsch, J., and Schmidt, K. (1986). “German democratic republic,” in Mineral Deposits of Europe. Editors F. W. Dunning, and A. M. Evans (London: The Mineralogical Society and The Institution of Mining and Metallurgy) 3, 303–329. Central Europe.
Begemann, F., Schmitt-Strecker, S., Pernicka, E., and Lo Schiavo, F. (2001). Chemical composition and lead isotopy of copper and bronze from nuragic Sardinia. Eur. J. Archaeol. 4 (1), 43–85. doi:10.1179/eja.2001.4.1.43
Bielicki, K.-H., and Tischendorf, G. (1991). Lead isotope and Pb- Pb model age determinations of ores from Central Europe and their metallogenetic interpretation. Contributions Mineralogy Petrology 106, 440–461. doi:10.1007/bf00321987
Biernacka, J., Borysiuk, K., and Raczyński, P. (2005). Zechstein (Ca1) limestone-marl alternations from the North-Sudetic Basin, Poland: Depositional or diagenetic rhythms? Geol. Quart. 49 (1), 1–14. Warszawa.
Boni, M., Innace, A., Köppel, V., Früh-Green, G., and Hansmann, W. (1992). Late to post-hercynian hydrothermal activity and mineralization in southwest Sardinia (Italy). Econ. Geol. 87, 2113–2137. doi:10.2113/gsecongeo.87.8.2113
Boni, M., and Koeppel, V. (1985). Ore-lead isotope pattern from the Iglesiente-Sulcis Area (SW Sardinia) and the problem of remobilization of metals. Mineral. Deposits 20, 185–193. doi:10.1007/bf00204563
Borg, G., Piestrzyński, A., Bachmann, G. H., Püttmann, W., Walther, S., and Fiedler, M. (2012). “An overview of the European kupferschiefer deposits,” in Geology and genesis of major copper deposits and districts of the World: A tribute to R.H. Sillitoe. Editors J. W. Hedenquist, M. Harris, and F. Camus (Colorado: Special Publications of the Society of Economic Geologists), 16, 455–486. doi:10.5382/SP.16.18
Borić, D. (2009). “Absolute dating of metallurgical innovations in the vinča culture of the Balkans,” in Metals and society. Studies in honour of Barbara S. Ottaway. Universitätsforschungen zur prähistorischen Archäologie. Editors T, L. Kienlin, and B. Roberts (Bonn: Dr. Rudolf Habelt), 169, 191–246.
Bruszczewo, I. (2004). “Ausgrabungen und Forschungen in einer prähistorischen Siedlungskammer Großpolens,” in Forschungsstand – erste Ergebnisse – das östliche Feuchtbodenareal Studien zur Archäologie in Ostmitteleuropa 2, Poznań, Kiel. Editors J. Czebreszuk, and J. Müller (Rahden/Westfalen: Marie Leitorf) 2.
Butent-Stefaniak, B. (1997). Z badań nad stosunkami kulturowymi w dorzeczu górnej i środkowej Odry we wczesnym okresie epoki brązu. Wrocław, Poland: Zakład Narodowy im. Ossolińskich.
Church, S. E., and Vaugh, R. B. (1992). Lead-isotopic characteristics of the Cracow-Silesia Zn-Pb ores. report 92–393 (southern Poland: Us Department of Interior, US Geological Survey Open-File), 92–393.
Church, S. E., Vaughn, R. B., Gent, C. A., and Hopkins, R. T. (1996). Lead-isotopic, sulphur-isotopic, and trace-element studies of galena from the Silesian-Cracow Zn-Pb ores, polymetallic veins from the Gory Swietokrzyskie MTS, and the Myszkow porphyry copper deposit, Poland. Warszawa: Prace Panstwowego Instytutu Geologicznego.
De Vleeschouwer, F., Fagel, N., Cheburkin, A., Pazdur, A., Sikorski, J., Matielli, N., et al. (2009). Anthropogenic impacts in North Poland over the last 1300 years — a record of Pb, Zn, Cu, Ni and S in an ombrotrophic peat bog. Sci. total Environmnet 407/21, 5674–5684. doi:10.1016/j.scitotenv.2009.07.020
Derkowska, K., Kierczak, J., Potysz, A., Pietranik, A., Pędziwiatr, A., Ettler, V., et al. (2023). Combined approach for assessing metal(loid)s leaching, mobility and accumulation in a specific near-neutral (pH) environment of a former Cu-smelting area in the Old Copper Basin, Poland. Appl. Geochem. 154, 105670. doi:10.1016/j.apgeochem.2023.105670
Derkowska, K., Świerk, M., and Nowak, K. (2021). Reconstruction of copper smelting technology based on 18–20th-century slag remains from the Old Copper Basin, Poland. Minerals 11 (9), 926. doi:10.3390/min11090926
Dziekoński, T. (1962). Metalurgia miedzi w osadzie kultury ceramiki wstęgowej malowanej w Złotej, pow. Sandomierz, oraz próba ustalenia pochodzenia przerabianego tam surowca. Stud. z dziejów górnictwa i Hut. 7, 7–125.
Fijałkowska-Mader, A., Durkowski, K., and Sokalski, D. (2018). Palynological and lithofacial implications for Zechstein stratigraphy in the marginal part of the North-Sudetic Synclinorium (Lower Silesia, southwestern Poland). Z. Dtsch. Ges. für Geowiss. 169 (4), 495–515. doi:10.1127/zdgg/2018/0142
Galan Huertos, E., and Mirete Mayo, S. (1979). Introduccion a los minerales de Espana. Madrid: Servicio de publicaciones Ministerio de Industria y Energia. Instituto Geologico y Minero de Espana. ISBN 84-74 74 -076-2.
Garbacz-Klempka, A., and Rzadkosz, S. (2014). “Charakterystyka składu chemicznego i struktury zabytków metalowych z osady kultury łużyckiej w Grzybianach,” in Osada kultury pól popielnicowych w Grzybianach koło Legnicy. Editors T. Stolarczyk, and J. Baron (Legnica-Wrocław: Muzeum Miedzi w Legnicy), 507–538.
Garcia de Madinabeitia, S., Gil Ibarguchi, J. L., and Santos Zalduegui, J. F. (2021). Iberlid: A lead isotope database and tool for metal provenance and ore deposits research. Ore Geol. Rev. 137, 104279. doi:10.1016/j.oregeorev.2021.104279
Gardawski, A. (1979). “Zagadnienia wytwórczości,” in Prahistoria Ziem Polskich 4. Od środkowej epoki brązu do środkowego okresu lateńskiego. Editors J. Dąbrowski, and Z. Rajewski (Wrocław-Warszawa-Kraków-Gdańsk, Poland: Zakład Narodowy im. Ossolińskich), 257–278.
Gavranović, M., Mehofer, M., Kapura, A., Koledin, J., Mitrović, J., Papazovska, A., et al. (2022). Emergence of monopoly, copper exchange networks during the late bronze age in the western and central Balkans. PLoS ONE 17 (3), e0263823. doi:10.1371/journal.pone.0263823
Gediga, B. (1988)Surowce mineralne w pradziejach i we wczesnym średniowieczu Europy Środkowej. Badania nad źródłami i eksploatacją surowców mineralnych w pradziejach i we wczesnym średniowieczu na Śląsku. Wrocław-Warszawa-Kraków-Gdańsk-Łódź: The National Institute of the Ossolińskis, 7–18.
Gediga, B. (1982). Metalurgia brązu w kulturze łużyckiej na Śląsku. Pamiętnik Muz. Miedzi 1, 109–130.
Gediga, B. (1967). Plemiona kultury łużyckiej w epoce brązu na Śląsku środkowym. Wrocław-Warszawa-Kraków: Narodowy Instytut im. Ossolińskich.
Gedl, M. (1985). Archeologia pierwotna i wczesnośredniowieczna. Część III. Epoka brązu i wczesna epoka żelaza w Europie (wyd. 3. poprawione). Kraków: Nakł. Uniwersytetu Jagiellońskiego.
Gedl, M. (1986). Badania poszukiwawcze na Pogórzu Kaczawskim. Acta Archaeol. Carpathica 25, 207–218.
Gedl, M. (1988). Uwagi na temat możliwości wykorzystywania miejscowych złóż miedzi w Polsce w epoce brązu i we wczesnej epoce żelaza. Surowce mineralne w pradziejach i we wczesnym średniowieczu Europy Środkowej. Kraków: Wrocław-Warszawa-Kraków-Gdańsk-Łódź, 29–39.
Gedl, M. (1982). Zarys dziejów metalurgii miedzi i brązu na ziemiach polskich do początków epoki żelaza. Pamiętnik Muz. Miedzi 1, 33–65. Legnica.
Harding, A. F. (2000). European societies in the bronze Age, cambridge. Cambridge: Cambridge University Press.
Höppner, B., Bartelheim, M., Hujsmans, M., Krauss, R., Martinek, K.-P., Pernicka, E., et al. (2005). Prehistoric copper production in the InnValley (Austria) and the earliest copper in Central Europe. Archaeometry 47 (2), 293–315. doi:10.1111/j.1475-4754.2005.00203.x
Iberlid, (2023). Ibercron. Available at: https://www.ehu.eus/ibercron/iberlid This archive is accessible at: http://www.ehu.eus/ibercron/data/IBERLID.xlsx Accessed in February 2023 geoportal.gov.pl.
Jaeger, M., and Czebreszuk, J. (2010). Does a periphery look like that? The cultural landscape of the unetice culture’s kościan group. Universitätsforschungen zur Prähistorischen Archäologie, 191. Bonn: Dr. Rudolf Habelt, 217–235.
Jaeger, M., Czebreszuk, J., Müller, J., and Kneisel, J. (2015). “Metal finds,” in Bruszczewo IV. Natural resources and economic activities of the Bronze Age people. Editors J. Czebreszuk, J. Müller, M. Jaeger, and J. Kneisel (Bonn: Dr. Rudolf Habelt), 227–234.
Jamka, R. (1950). Prehistoryczne i wczesnodziejowe ośrodki produkcji górniczej i rzemieślniczej na Śląsku. Przegląd Hist. 41, 21–69.
Jockenhövel, A. (1986). Struktur und Organisation der Metallverarbeitung in urnenfelderzeitlichen Siedlungen Süddeutschlands. Veröffentlichungen Museums für Ur- Frühgeschichte Potsdam 20, 213–234.
Kaczmarek, M. (2017). “The snares of ostensible homogeneity. Lusatian culture or lusatian urnfields?,” in The past societies 3 (2000-500 BC). Editor U. Bugaj (Warszawa: Institute of Archaeology and Ethnology, Polish Academy of Sciences), 263–293.
Kadrow, S. (2017). “The Danubian world and the dawn of the metal ages,” in The Past Societies 2 (5500-2000 BC). Editors P. Włodarczak (Warszawa: Institute of Archaeology and Ethnology, Polish Academy of Sciences), 63–106. 5500-2000 BC.
Kądziołka, K., Pietranik, A., Kierczak, J., Potysz, A., and Stolarczyk, T. (2020). Towards better reconstruction of smelting temperatures: Methodological review and the case of historical K-rich Cu-slags from the Old Copper Basin, Poland. J. Archaeol. Sci. 118, 105142. doi:10.1016/j.jas.2020.105142
Klein, S., Domergue, C., Lahaye, Y., Brey, G. P., and von Kaenel, H-M. (2009). The lead and copper isotopic composition of copper ores from the Sierra Morena (Spain). J. Iber. Geol. 35 (1), 59–68.
Kmosek, J., Erban Kochergina, Y. V., Chvojka, O., and Fikrle, M. (2020). Tracking Alpine copper—analysis of Late Bronze Age copper ingot hoard from South Bohemia. Archaeol. Anthr. Sci. 12, 234. doi:10.1007/s12520-020-01186-z
Kostrzewski, J. (1953). Wytwórczość metalurgiczna w Polsce od neolitu do wczesnego okresu żelaznego. Przegląd Archeol. 9, 177–211.
Kowalski, A., Maciejak, K., Wojewoda, J., Kozłowski, A., and Raczyński, P. (2017). Anthropogenic changes of the “Old Copper Basin” area landscape (North-Sudetic Synclinorium) in the light of LiDAR-based geomorphometric analysis and archival data. Biul. Państw. Inst. Geol. 469, 0–200. doi:10.5604/01.3001.0010.0080
Kowalski, Ł., Adamczak, K., Bokiniec, A., Bielińska-Majewska, B., Karczmarek, Ł., Krzyszowski, A., et al. (2021). New data for research on the early bronze age in northern Poland (2350-1600 BC). Archäologisches Korresp. 51/1, 473–499. doi:10.11588/ak.2021.4.93266
Kucha, H., and Pawlikowski, M. (2010). Badania genezy cechsztyńskich złóż miedzi w Polsce/Genetic investigations of copper Zechstein deposits, Poland. Geologia 36/4, 513–538.
Kulczycka-Leciejewiczowa, A. (1979). “Pierwsze społeczeństwa rolnicze na ziemiach polskich. Kultury kręgu naddunajskiego,” in Prahistoria ziem polskich 2. Neolit. Editor W. Hensel (Wrocław-Warszawa-Kraków-Gdańsk: Zakład Narodowy im. Ossolińskich), 19–164.
Lasak, I. (1991). Uwagi o pozostałościach konstrukcji drewniano-ziemnych na osadzie wczesnobrązowej (stan 22) w Radłowicach. Stud. Archeol. 20, 23–45.
Łesiuk, M. (2019). “Zabytki ruchome odkryte w trakcie badań wykopaliskowych w 2019 r. Ceramika naczyniowa,” in Opracowanie wyników badań zrealizowanych w 2019 r. w miejscowości Leszczyna, gm. Editor T. Stolarczyk (Złotoryja. unpublished report.
Lillo, J. (1992). Vein-type base-metal ores in Linares-La carolina (Spain): Ore-lead isotopic constrains. Euroean J. Mineralogy 4, 337–344. doi:10.1127/ejm/4/2/0337
Ling, J., Hjarthner-Holdar, E., Grandin, L., Stos-Gale, Z., Kristiansen, K., Melheim, A. L., et al. (2019). Moving metals IV: Swords, metal sources and trade networks in bronze age Europe. J. Archaeol. Sci. Rep. 26, 101837. doi:10.1016/j.jasrep.2019.05.002
Łuka, L. J. (1959). Importy italskie i wschodnioalpejskie oraz ich naśladownictwa na obszarze kultury “łużyckiej” okresu halsztackiego w Polsce. Slavia Antiq. 6, 34–36.
Maciejak, K., and Maciejak, M. (2013). Górnictwo miedzi w niecce złotoryjskiej od średniowiecza do końca XIX w. Dzieje górnictwa – Elem. Eur. dziedzictwa kult. 5, 209–227.
Maciejak, K., and Maciejak, M. (2016). Zakłady górnicze „Nowy Kościół. Hered. Minariorum 3, 83–108. doi:10.5277/hm150205
Marcoux, E. (1998). Lead isotope systematics of the giant massive sulphide deposits in the Iberian Pyrite Belt. Min. Depos. 33, 45–58. doi:10.1007/s001260050132
Mason, A., Powell, W., Bankoff, A., Mathur, R., Price, M., Bukatović, A., et al. (2020). Provenance of tin in the Late Bronze Age Balkans based on probabilistic and spatial analysis of Sn isotopes. J. Archaeol. Sci. 122 (6), 105181. doi:10.1016/j.jas.2020.105181
Melheim, L., Grandin, L., Persson, P.-O., Billström, K., Stos-Gale, Z., Ling, J., et al. (2018). Moving metals III: Possible origins for copper in bronze Age Denmark based on lead isotopes and geochemistry. J. Archaeol. Sci. 96, 85–105. doi:10.1016/j.jas.2018.04.003
Modarressi-Tehrani, D., Garner, J., and Kvietok, M. (2016). “Copper production in the Slovak ore Mountains,” in From bright ores to shiny metals, Festschrift for Andreas Hauptmann. Der Anschnitt, Beiheft 29. Editors G. Körlin, M. Prange, T. Stöllner, and U. Yalcin (Rahden/Westfalen: Marie Leidorf), 109–123.
Molloy, B. (2019). “A warrior’s journey? Some recent taxonomic, trace element and lead isotope analyses of mediterranean later bronze age metalwork in the central western Balkans,” in Papers in honour of frastko vacsić 80th birthday. Editors V. Filipović, A. Bulatović, and A. Kapuran (Belgrade: Institute of Archaeology), 143–154.
Niederschlag, E., Pernicka, E., Seifert, T., and Bartelheim, M. (2003). The determination of lead isotope ratios by multiple collector ICP-MS: A case study of early bronze age artefacts and their possible relation with ore deposits of the Erzgebirge. Archaeometry 45, 61–100. doi:10.1111/1475-4754.00097
Nimis, P., Omenetto, P., Giunti, I., Artioli, G., and Angelini, I. (2012). Lead isotope systematics in hydrothermal sulphide deposits from the central-eastern Southalpine (northern Italy). Eur. J. Mineralogy 24, 23–37. doi:10.1127/0935-1221/2012/0024-2163
Nørgaard, H. W., Pernicka, E., and Vandkilde, H. (2021). Shifting networks and mixing metals: Changing metal trade routes to Scandinavia correlate with Neolithic and Bronze Age transformations. PLoS ONE 16 (6), e0252376. doi:10.1371/journal.pone.0252376
Nowak, K., Baron, J., Puziewicz, J., and Ziobro, M. (2019). Multi-faceted analysis of metal sickles from the late Bronze Age scrap deposit found in Paszowice, SW Poland. Geochemistry 79 (3), 446–452. doi:10.1016/j.chemer.2019.05.006
Nowak, K. (2020). Organizacja produkcji metalurgicznej na wybranym obszarze Europy Środkowej w czasach pól popielnicowych. Wrocław: University of Wrocław. unpublished doctoral dissertation.
Nowak, K., Sielicka, K., and Miazga, B. (2022a). Skarb z Paszowic, woj. dolnośląskie. Analiza śladów produkcji i (nie)używania (Hoard from Paszowice, Lower Silesia. Analysis of (non)use-wear and manufacture traces). Archeol. Pol. 67, 81–115. doi:10.23858/APol67.2022.004
K. Nowak, and T. Stolarczyk (Editors) (2016). Metalurdzy znad Kaczawy. Cmentarzysko ciałopalne z epoki brązu odkryte w Legnicy przy ul. Spokojnej (Legnica-Wrocław: Muzeum Miedzi w Legnicy).
Nowak, K., Stolarczyk, T., Stos-Gale, Z. A., and Miazga, B. (2022b). The Late Bronze Age 'metallurgists' graves’ in south-Western Poland. Tracing the provenance of the metal raw material using casting moulds. J. Archaeol. Sci. Rep. 42, 103393. doi:10.1016/j.jasrep.2022.103393
Nowak, K., Stos-Gale, Z., Derkowski, P., and Stolarczyk, T. (2023a). “Interpretation of the lead isotope and chemical analyses of the copper-based artefacts from Lower Silesia,” in Oriental and European Archaeology, UK-gespräche “bronze Age metallurgy production – consumption – exchange“ and 20th anniversary archaeometallurgical laboratory VIAS. Editors M. Gavranović, and M. Mehofer, 23–24. in review.
Nowak, K., Tarbay, J. G., Stos-Gale, Z. A., Derkowski, P., and Sielicka, K. (2023b). A complex case of trade in metals: The origin of copper used for artefacts found in one hoard from a Late Bronze Age Lusatian Urnfield Culture in Poland. J. Archaeol. Sci. Rep. 49, 103970. doi:10.1016/j.jasrep.2023.103970
Oxalid, (2023). Oxalid. Available at: http://oxalid.ox.ac.uk (This archive is accessible at: https://oxalid.arch.ox.ac.uk/The%20Database/TheDatabase.htm Accessed in February 2023.
Pankiewicz, A., and Rodak, S. (2016). “Śląska ceramika jako źródło do poznania garncarstwa okresu wczesnonowożytnego,” in Ceramika i szkło w archeologii i konserwacji Wrocław. Editors S. Siemianowska, P. Rzeźnik, and K. Chrzan(Wrocław: Wydawnictwo Instytutu Archeologii i Etnologii Polskiej Akademii Nauk), 331–356.
Paruzel, P. (2011). Stanowisko Budziszów Wielki 20, gm Wądroże Wielkie, pow. jaworski, w świetle badań archeologicznych prowadzonych w związku z modernizacją autostrady A-4. Raport 241-273, 2005–2006. ISBN 978-83-63260-00-2.
Pernicka, E., Lutz, J., and Stöllner, T. (2016). Bronze Age copper produced at Mitterberg, Austria, and its distribution. Archaeol. Austriaca 100, 19–56. doi:10.1553/archaeologia100s19
Raczyński, P. (1997). Warunki sedymentacji osadów cechsztynu w niecce północnosudeckiej. Przegląd Geol. 45 (7), 693–699.
Richthofen, B. v. (1924). Auf den spuren alter siedlungen. 1. Altschlesien: Gegend von Mertschütz Kreis Liegnitz 1 (2), 57–65.
Rovira Llorens, S., Montero Ruiz, I., and Consuegra Rodriguez, S. (1997). Las primeras etapas metalúrgicas en la Península Ibérica: I Análisis de materiales. Madrid: Instituto Universitario Ortega y Gasset.
Saez, R., Nocete, F., Gil Ibarguchi, J. I., Rodriguez-Bayona, M., Inacio, N., Quispe, D., et al. (2021). A lead isotope database for copper mineralization along the Guadalquivir River Valley and surrounding areas. J. Iber. Geol. 47, 411–427. doi:10.1007/s41513-020-00151-y
Santos Zalduegui, J. F., Garcia de Madinabeitia, S., Gil Ibarguchi, J. I., and Palero, F. (2004). A lead isotope database: The Los Pedroches Alcudia area (Spain); implications for archaeometallurgical connections across south Western and south eastern Iberia. Archaeometry 46 (4), 625–634. doi:10.1111/j.1475-4754.2004.00178.x
Schreiner, M. (2007). Erzlagerstätten im Hrontal, Slowakei. Genese und Prähistorische Nutzung. Forschungen zur Archäometrie und Altertumswissenschaft 3. Rahden/Westfalien: Marie Leidorf.
Solecki, (2011). “Structural development of the epi-Variscan cover in the North Sudetic Synclinorium area,” in Mezozoik I kenozoik dolnego sląska. Editors A. Żelaźniewicz, J. Wojewoda, and W. Cieżkowski, 19–36. (In Polish).
T. Stolarczyk, and J. Baron (Editors) (2014). Osada kultury pól popielnicowych w Grzybianach koło Legnicy. (Legnica, Poland: Muzeum Miedzi w Legnicy)
Stolarczyk, T. (2014a). Der Bergbau in Niederschlesien in 13.–14. Jahrhundert – der archäologische Forschungsstand. Praehistorica 31/2 (2013), 655–678.
Stolarczyk, T. (2011). “Der Buntmetallbergbau in Niederschlesien vom 13. bis zum Anfang des 17. Jahrhunderts,” in Aufbruch unter Tage. Stand und Aufgaben der montanarchäologischen Forschungen in Sachsen. Arbeits-und Forschungsberichte zur sächsischen Bodendenkmalpflege. Editor R. Smolnik (Dresden: Landesamt für Archäologie), 200–214.
Stolarczyk, T., Kobylańska, M., Kierczak, J., Madziarz, M., and Garbacz-Klempka, A. (2017). Leszczyna. Monografia ośrodka górnictwa i metalurgii rud miedzi. Radziechów: Fundacja Archeologiczna Archeo.
Stolarczyk, T. (2014a). “Pracownia brązownicza z osady w Grzybianach – zagadnienia wstępne,” in Osada kultury pól popielnicowych w Grzybianach koło Legnicy. Editors T. Stolarczyk, and J. Baron (Legnica-Wrocław: CEON), 493–505.
Stolarczyk, T. (2012a). The mining of the polimetalic ore in Dolni Slezko in 13th-17th century. Acta rerum Nat. 12, 81–78.
Stolarczyk, T. (2012b). Wyniki badań archeologicznych nad problematyką dawnego górnictwa i hutnictwa miedzi na terenie Pogórza Kaczawskiego oraz Gór Kaczawskich i Rudaw Janowickich w 2012 r. Szkice Legn. 33, 207–238.
Stöllner, T. (2015). “Der Mitterberg als Großproduzent für Kupfer in der Bronzezeit,” in Bergauf bergab 10000 jahre bergbau in den ostalpen. Editors T. Stöllner, and K. Oeggl (Bochum: Marie Leidorf), 175–185.
Stos-Gale, Z. A., Degryse, P., and de Muynck, D. (2012). “Metale z wykopalisk na rynku krakowskim: Pochodzenie geologiczne metali na podstawie pomiarów izotopów ołowiu,” in Nawarstwienia historyczne miast (Kraków, Poland: Wydawnictwa Akademii Górniczo-Hutniczej im. S. Staszica w Krakowie), 295–311.
Stos-Gale, Z. A., Gale, N. H., Houghton, J., and Speakman, R. (1995). Lead isotope data from the Isotrace laboratory, Oxford: Archaeometry data base 1, ores from the Western mediterranean. Archaeometry 37 (2), 407–415. doi:10.1111/j.1475-4754.1995.tb00753.x
Stos-Gale, Z. A. (2019). “Lead isotopes and the origin of bronze artefacts,” in The origins of the Bronze Age in Warmia and Masuria revealed by scientific analyses. Editor J. Sobieraj (Olsztyn: Muzeum Warmii i Mazur), 83–118.
Stos-Gale, Z. A. (1993). The origin of metals from the roman-period levels of a site in southern Poland. J. Eur. Archaeol. 1/2, 101–131. doi:10.1179/096576693800719365
Tornos, F., and Chiaradia, M. (2004). Plumbotectonic evolution of the Ossa Morena zone, Iberian Peninsula: Tracing the influence of mantle-crust interaction in ore-forming processes. Econ. Geol. 99, 965–985. doi:10.2113/gsecongeo.99.5.965
Vaughan, D., Sweeney, M., Friedrich, G., Diedel, R., and Haranczyk, C. (1989). The kupferschiefer: An overview with an appraisal of the different types of mineralization. Econ. Geol. 84, 1003–1027. doi:10.2113/gsecongeo.84.5.1003
Wedepohl, K. H., Delavaux, M. H., and Doe, B. R. (1978). The potential source of lead in the permian kupferschiefer bed of Europe and some selected paleozoic mineral deposits in the federal republic of Germany. Contributions Mineralogy Petrology 65, 273–281. doi:10.1007/bf00375513
Wiślański, T. (1979). “Kształtowanie się miejscowych kultur rolniczo-hodowlanych plemion kultury pucharów lejkowatych,” in Prahistoria ziem polskich 2. Neolit. Editor W. Hensel (Wrocław-Warszawa-Kraków-Gdańsk: Zakład Narodowy im. Ossolińskich), 165–260.
Witter, W. (1938). Über vorgeschichtliche die Kupfergewinnung in Schlesien. Altschlesien 7, 201–213.
Keywords: copper, exploitation, ores, metal provenance, Urnfield period, Bronze Age, metalwork, Zechstein limestone (Ca1)
Citation: Nowak K, Stolarczyk T, Stos-Gale Z, Baron J, Derkowska K, Derkowski P, Miazga B, Woodhead J, Karasiński J and Maas R (2023) Question of local exploitation of copper ore deposits in the Urnfield time in Poland. Front. Earth Sci. 11:1184949. doi: 10.3389/feart.2023.1184949
Received: 12 March 2023; Accepted: 19 June 2023;
Published: 21 July 2023.
Edited by:
Heide Wrobel Nørgaard, Moesgaard Museum, DenmarkReviewed by:
Mario Gavranovic, Austrian Academy of Sciences (OeAW), AustriaErnst Pernicka, Curt Engelhorn Centre Archaeometry, Germany
Copyright © 2023 Nowak, Stolarczyk, Stos-Gale, Baron, Derkowska, Derkowski, Miazga, Woodhead, Karasiński and Maas. This is an open-access article distributed under the terms of the Creative Commons Attribution License (CC BY). The use, distribution or reproduction in other forums is permitted, provided the original author(s) and the copyright owner(s) are credited and that the original publication in this journal is cited, in accordance with accepted academic practice. No use, distribution or reproduction is permitted which does not comply with these terms.
*Correspondence: K. Nowak, a2FtaWwubm93YWtAdW1rLnBs