- 1Department of Environmental Engineering, School of Environmental Science and Engineering, Sun Yat-Sen University, Guangzhou, China
- 2Guangdong Provincial Key Laboratory of Environmental Pollution Control and Remediation Technology, Sun Yat-sen University, Guangzhou, China
- 3Department of Mechanical Engineering, The University of Hong Kong, Hong Kong, Hong Kong
As air pollution poses a great challenge around the globe, it is essential to fashion out a way of efficiently degrading the air pollutants. Vacuum Ultraviolet (VUV)-based processes are an emerging and promising technology for environmental remediation such as air cleaning, wastewater treatment, and air/water disinfection. When VUV irradiation, photolysis, photocatalysis, and ozone-assisted oxidation are involved at the same time, it results in the fast degradation of air pollutants because of their strong oxidizing capacity. The mechanisms of how the oxidants are produced and how they react are discussed in this review. This paper focuses mainly on the three VUV-based oxidation processes including VUV photolysis, VUV combined with ozone-assisted oxidation, and VUV-PCO with emphasis on their mechanisms and applications. Also, the outlook of these processes are proposed in this paper.
Introduction
Common Air Pollutions
With the rapid development of industry and increasing population, air pollutions presently poses a more serious challenge. Solving the problem of air pollution is one of the most difficult challenges faced by a number of governments around the world (Spigno et al., 2003). Air pollutants, including biological and gaseous substances exist in ambient as well as in indoor environments and require simultaneous removal for better air quality (Yu et al., 2009). Gaseous pollutants can be classified into organic and inorganic, and among them organic compounds take a majority of the emission category. Inorganic pollutants mainly refer to sulfur dioxide, nitric oxide, hydrogen sulfide, and so on, while organic pollutants mainly consist of Volatile Organic Compounds (VOCs) with boiling points between room temperature and 260°C (Huang et al., 2015b) including alkanes, aromatic hydrocarbons, aromatics, olefins, alcohols, aldehydes, ketones, halogenated hydrocarbon, and so on. Among the list of 25 toxic gaseous pollutants listed by U.S. EPA, 18 of them are organic compounds (Lee, 1991). The generation, transportation and storage processes of the raw chemicals and industries, such as pharmaceutical chemicals, printing, and petrochemical industries produce most of the VOCs (Li et al., 2009; Brito et al., 2015). VOCs cause great harm to the human health. Long time exposure to VOCs may cause health problems, such as Sick Building Syndromes (SBS) (Järnström et al., 2006), cancer, genetic mutation, and so on. It can also cause air pollution like PM2.5, photochemical smog, ozone, and global warming (McGwin et al., 2010; Wu et al., 2012).
In the past, most of the studies focused on the degradation of single pollutant but not on multiple air pollutants (Thiruvenkatachari et al., 2008). VUV-based processes are advanced oxidation processes (AOPs) without selectivity, which is of a great advantage for the degradation of multiple air pollutants.
Common Air Purification Methods
Air purification process can be generally divided into physical, chemical, and biological methods.
Physical Methods
In physical methods, pollutants can be separated from waste gas due to the different physical properties like boiling point and solubility. It can be divided into absorption, adsorption, membrane separation, and condensation. Moretti et al. (Moretti, 2002) tried to absorb the organic gas by Nonpolar mineral oil such as light diesel and engine oil with a removal efficiency of 90%. Noll (Standeker et al., 2009), Moura (Moura et al., 2011), Daifullah (Daifullah and Girgis, 2003), and Kwong (Kwong and Chao, 2010) adopted the silica aerosol, alumina, activated carbon, and fly-ash in order to adsorb VOCs. High removal rate of VOCs can be achieved under membrane separation (Xing et al., 2000). Baker et al. (1994) recycled VOCS from waste gas by compression, condensation, and membrane separation.
However, there are some disadvantages that tend to limit the application of physical methods. For example, most of the VOCs are insoluble in water which reduces the absorption efficiency. In addition, the solution after absorption needs further disposal. Although the adsorption process is simple and it can also recycle the useful compounds of the waste gas, secondary pollution will occur during the desorption process which may increase the cost. The high cost also greatly restricts the application of membrane separation.
Biological Methods
In biological treatment, pollutants are generally decomposed into carbon dioxide, water, and cytoplasm by microbial metabolism (Deshusses and Johnson, 2000). Biological degradation has many operational and cost advantages over the conventional physico-chemical methods (Aizpuru et al., 2001). Niu et al. (2014) discovered that a bio-tricking filter can be used to remove inorganic pollutants such as NO from air stream using bacteria extracted from waste water sludge. Lu et al. (2000) used bio-trickling to destruct BTEX vapor and they found that more than 90% BTEX could be achieved. Rene et al. (2009) discovered that removal efficiencies of benzene is higher than 90% in a laboratory scale bio-filtration. However, this system needs a large area and long start-up time (Wang et al., 2008).
Chemical Methods
Chemical methods can remove pollutants by a series of reactions like neutralization and redox reactions. The processes include incineration and catalytic combustion (Barbero et al., 2008), plasma treatment (Daniels, 2002), and acid-base spray (Dulin and Rosar, 1975). Harmful substances can be degraded completely into non-toxic substances by chemical methods. However, the cost is higher than physical and biological methods as special chemicals are needed in the processes, thus, it is highly necessary to reduce their cost. The required temperature of VOCs catalytic combustion can be greatly decreased by developing efficient catalysts, thereby reducing the energy consumption. Wang et al. (Wang, 2004) adopted γ-Al2O3 supported transition-metal oxide catalysts in order to oxidize methylbenzene which can be degraded completely under a temperature of 360°C. Wang et al. (2006) also used CuO/CeO2 for catalytic oxidation of toluene which can be completely degraded at temperature lower than 240°C.
As shown above, the application of traditional technology is limited by some disadvantages such as byproducts, cost, and degradation capacity. Therefore, it is essential to develop a cost-effective and environmental-friendly treatment method under increasingly complicated air pollution problems and stringent air quality standards. As such AOPs have been studied for air pollution control because of its high efficiency and mild reaction condition (Zhu et al., 2015). Among them, VUV-based processes are emerging processes for the degradation of air pollutants that can provide an efficient solution for air pollution control.
Introduction of UV and VUV Irradiation
UV irradiation refers to the electromagnetic radiation with wavelength from 1 to 380 nm. It can be categorized into UV-A (380–315 nm), UV-B (315–280 nm), UV-C (280–200 nm), vacuum-UV (VUV, 200–100 nm), and extreme UV (100–1 nm) (Oppenländer, 2003). The main method used to produce UV radiation is through the discharge from low pressure mercury lamp. Medium pressure mercury lamp can also be used to produce UV-A, UV-B, and UV-C. However, UV-B and UV-C can be absorbed by the glass tube and it needs special material like quartz to avoid loss of UV light (Thiruvenkatachari et al., 2008). As for the VUV lamp, it required a quartz with high purity in order to prevent the absorption of the shorter wavelength's UV irradiation. Therefore, barrier discharge (Masschelein and Rice, 2002) and Xe-excimer radiators (Wang et al., 2010) are generally used to produce VUV irradiation.
Recently, among the different AOPs, VUV-based processes have attracted much attention in the degradation of air pollutants. Yang (Yang et al., 2007), Huang (Huang et al., 2011), and Jiang (Jiang et al., 2015) have tried to eliminate the air pollutants with VUV photolysis. Compared with UV, VUV can degrade air pollutants more efficiently due to the following reasons: (1) VUV can directly destruct the compounds due to its energetic photons; (2) With certain humidity, VUV irradiation can interact with water vapor and produce hydroxyl radicals that can degrade compounds; (3) Oxygen species like O(1D), O(3P), and O3 can be formed from the reaction between VUV irradiation and oxygen (Bergonzo and Boyd, 1993; Hashem et al., 1997; Fu et al., 2011). When degradation capacity and cost are put into consideration, the application of VUV becomes more attractive. However, it also has some disadvantages such as the formation of residual ozone that will cause secondary pollution.
Generation of the Oxidants
General VUV-based processes include the VUV photolysis (Alapi and Dombi, 2007a), VUV–PCO (Huang et al., 2011), VUV combined with Ozone-assisted Catalytic Oxidation (VUV–OZCO) (Huang, 2015). The sections below will focus on the oxidants generated from these three processes.
Hydroxyl Radicals Formation
Photolysis
As the wavelength of VUV spectral domain (100–200 nm, mainly at 185 nm) is shorter than that of normal UV (Gonzalez et al., 2004), it can produce photons with higher energy level. Therefore, many studies have been carried out to achieve photo-degradation of pollutants by VUV photolysis alone (Ye et al., 2014). Energetic photons play an important role in the degradation process because they can result in direct degradation of the pollutants and in the formation of oxidizing species like hydroxyl radical and ozone that can oxidize pollutants (Bergonzo and Boyd, 1993; Kutschera et al., 2009; Zoschke et al., 2014). Hydroxyl radical is highly reactive for the oxidation of the pollutants in gaseous or aqueous phase with no selectivity.
In the VUV photolysis of aqueous reaction systems, photolysis of water is the main pathway to produce hydroxyl radical (Gonzalez et al., 2004; Zoschke et al., 2014). Water absorbs light strongly at wavelengths lower than 190 nm. However, due to the high absorption rate, the VUV irradiation is absorbed within a narrow layer around the lamp (approx. 300 mm at 185 nm) (Kutschera et al., 2009). The ionization of water occur via the homolysis (Equation 1) and photochemical (Equation 2; Zoschke et al., 2014) reactions. The oxidants formed are mainly hydroxyl radicals, hydrogen atoms, and solvated electrons all of which initiate manifolds of reduction and oxidation reactions (Oppenländer, 2003). The quantum yields (φ) of the hydroxyl radical and the solvated electrons are 0.33 and 0.045, respectively (Lopez et al., 2000; Kutschera et al., 2009).
In the same way, the photolysis of moisture gas takes place in gaseous phase and hydroxyl radicals are generated with the VUV irradiation. In addition, another way to form · OH is in a humidified O2 stream which can open up another pathway for the decomposition of pollutants. With wavelengths under 220 nm, oxygen can be dissociated into two O atoms (Equation 3; Atkinson et al., 1996). The O atom does not only interact with water to produce hydroxyl radicals (Equation 4), it also reacts with oxygen to produce ozone (Equation 5; Alapi and Dombi, 2007b). Therefore, there is a competition between the generation of hydroxyl radicals and ozone. It was reported that humidity inhibits O3 formation in an O2 stream and ·OH will be formed due to the reaction of O atom and water (Atkinson et al., 1996). Further reactions in the system will lead to the formation of hydrogen peroxide (Ye et al., 2013; Equations 7–9). Also, the generated H2O2 will also be decomposed into hydroxyl radicals (Equation 10) under the irradiation of 185 and 254 nm UV light (Atkinson et al., 2004).
Overall, hydroxyl radicals are generated in gaseous phase via four pathways: (1) homolysis of water, (2) photochemical ionization of water, (3) reactions between O atom and water, and (4) photolysis of hydrogen peroxide.
Photocatalysis of Semiconductor
Hydroxyl radicals produced by photolysis are mainly attributed to the 185 nm UV irradiation. However, VUV lamp can only emit < 10% 185 nm irradiation while the majority 254 nm UV irradiation has not been fully utilized in photolysis (Alapi and Dombi, 2007a). Therefore, semiconductor like TiO2 was used to produce more hydroxyl radicals via photocatalysis. The mechanism of photocatalytic oxidation (PCO) is similar to that of UV/TiO2, which is shown in Figure 1.
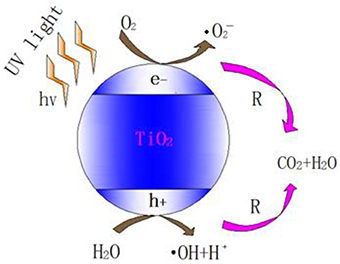
Figure 1. Scheme of photocatalysis of TiO2 (Tompkins et al., 2005).
As one of the semiconductor catalysts, TiO2 photocatalytic material has special electronic structure. Unlike metals which have a continuum of electronic states, semiconductors possess a void energy region where low energy levels are available to promote recombination of electrons and holes produced by photo-activation within the material. The void region which extends from the top of the filled valence band to the bottom of the vacant conduction band is called the band gap (Linsebigler et al., 1995). With the irradiation of UV light, TiO2 absorbs the energy of photon. When the energy of photon is higher than the width of the semiconductor's void band, the electron of valence band will transfer to the conduction band. Therefore, the pairs of electron-holes are created in the semiconductor, and the charge will be transferred between electron-hole pairs and adsorbed species (reactants) on the semiconductor surface, photo-oxidation will then occur (Zhao and Yang, 2003).
The photo-generated electron (e−) has strong redox potential and is able to reduce the electron acceptor of the semiconductor's surface. The photo-generated hole (h+) generated by activation is a strong oxidant. It reacts with absorbed water (H2O) or surface hydroxyl ion chemically (OH−) and produces hydroxyl radical with strong oxidizing property (Schwitzgebel et al., 1995).
O2 and H2O will interact with the electrons and photo-generated holes in humid air. The overall reaction is as follows (Konstantinou and Albanis, 2004): First, O2 and H2O are absorbed on the surface of the TiO2 and then form adsorbed oxygen and adsorbed water, respectively (Equations 11, 12). Subsequently, the surface of the TiO2 produces photo-generated electron (e−) and photo-generated hole (h+) under UV irradiation (Equation 13).


Afterwards, the photo-generated hole is captured by the surface of adsorbed water and hydroxyl ion, producing hydroxyl radicals (Equations 14, 15).
Furthermore, the addition of O2 leads to an increase in the upward band bending, and therefore, suppresses the electron-hole recombination process, leading to a more efficient photo-activity (Linsebigler et al., 1995). Meanwhile, further reaction will continuously produce hydroxyl radical (Equations 16–19).
Generation of Ozone
The VUV irradiation at 185 nm can be used for the generation of ozone using oxygen. As shown in Equations (3) and (5), O atom will be generated by the photolysis of oxygen when absorbing VUV irradiation and then reacted with an oxygen molecule, forming ozone. This reaction takes place in the presence of a molecule M which absorbs the excessive kinetic energy (Bolton and Denkewicz, 2008). Meanwhile, ozone can be decomposed by 254 nm UV irradiation, forming an O atom and an oxygen molecule, as shown in Equation 20.
The yield of ozone from VUV irradiation is very low. About 9 g/kWh and 7.2 g/kWh of ozone can be produced from oxygen and air, respectively (Hashem et al., 1997). Two reasons might be responsible for the low ozone generation. First, the emission intensity at 185 nm constitutes only 8% of all UV irradiation. Also, partial ozone could be decomposed by 254 nm UV light. In addition, VUV irradiation can be easily absorbed by the water vapor. The generated O3 increases with the decreasing water content. Ye et al. (2013) studied the effect of the operating parameters on ozone formation. It was found that H2O and O2 contents, as well as flow rate, could significantly affect O3 production. The increased H2O content led to the decreased O3 production, whereas O2 content had an opposite effect.
Despite the low ozone yields of low-pressure mercury vapor lamps, ozone generation and photolysis of pollutants can be simultaneously achieved by a single irradiation source (Hashem et al., 1997). The VUV systems with internal ozone generation has been used for the disinfection of micro-pollutants (Bolton and Denkewicz, 2008; Zoschke et al., 2012), as shown in Figure 2. The air or oxygen go through the annular space between the UV lamp and the quartz sleeve which can absorb the VUV radiation at 185 nm to generate ozone. The gas containing ozone is injected into the water on the other side of the quartz sleeve. In addition, the UV/VUV radiation passes directly through the quartz sleeve into water to achieve the photolysis of an aqueous phase.
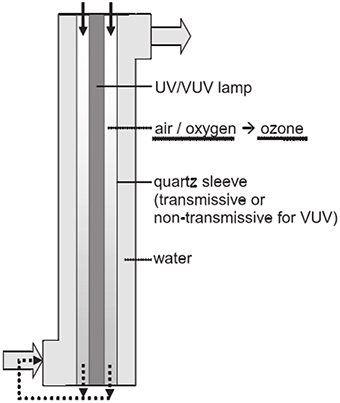
Figure 2. Schematic cross-section of a VUV reactor with internal ozone generation (Zoschke et al., 2012).
Ozone is a strong oxidant and has a great potential to be utilized for the degradation of pollutants. It was reported that high efficiency of degradation of benzene (Einaga and Futamura, 2004a; Einaga et al., 2009), ethanol (Shayan and Vahedpour, 2013), and acetone (Reed et al., 2006) can be achieved by ozone catalytic oxidation treatment.
The key of ozone catalytic oxidation technology is the preparation of catalysts with high CO2 selectivity, stability, and removal efficiency. Ozone decomposition catalysts consist of precious metals (such as palladium, silver) and transition metal oxides, and so on. The application of precious metals is greatly limited by its high cost and the difficulty of regeneration. In recent years, transition metal oxides have attracted much attention. Among the transition metal oxides, the supported catalysts are more attractive because of its lower cost and better catalytic stability. The supports include Al2O3 (Einaga and Futamura, 2004b, 2005; Konova et al., 2006), TiO2 (Radhakrishnan and Oyama, 2001), SiO2, and molecular sieve (Einaga et al., 2013). Einaga (Einaga and Ogata, 2009) investigated the performance of ozone catalytic oxidation of benzene over Mn catalysts with different carriers such as SiO2, Al2O3, TiO2, and ZrO2. The results showed that the increased specific surface area of the support can enhance benzene degradation. The high specific surface area does not only facilitate the absorption of pollutants but also prolongs the reaction time between ozone and pollutants.
Transition metals including Mn, Fe, Co, Cu, Ni, and Ag were generally used as active components.Among different Al2O3 supported transition metals, Mn has excellent catalytic activity toward both ozone decomposition and benzene degradation (Einaga and Ogata, 2010). Ebrahim et al. (Rezaeia et al., 2013) studied the effect of Mn loading on ozone catalytic degradation of toluene and found that high Mn loading is detrimental for ozone decomposition and benzene degradation. Despite its potential for efficient pollutant degradation, ozone catalytic oxidation technology is still faced with some problems like the deactivation of catalyst, emission of residual ozone, and generation of byproducts.
VUV-Based Processes for Air Pollutants Degradation
Direct VUV Photolysis
Direct photolysis by Ultraviolet (UV) and Vacuum Ultraviolet (VUV) have been intensively studied for removing both organic and inorganic compounds from water or air. VUV lamp has its main emission at 254 nm with a small (around 6%) intensity of irradiation at 185 nm, while UV lamp has its output only at 254 nm (Alapi and Dombi, 2007a). VUV photolysis is known for better performance on the removal of pollutants based on the following reasons: (1) the high-energy photons generated by 185 nm irradiation can degrade organic compounds; (2) in the presence of water, 185 nm irradiation can produce strong oxidants such as hydroxyl radical (•OH); (3) 185 nm irradiation can generate ozone in the presence of O2 (Yang et al., 2007). Oxygen species [for example, O(1D), O(3P), and O3] and hydroxyl radicals (•OH) are generated by the dissociation of oxygen and water molecules under VUV with high-energy photon according to the following reactions (Bergonzo and Boyd, 1993; Hashem et al., 1997; Fu, 2011; Equations 21–25):
These energetic oxidants can be utilized to improve removal efficiencies of the pollutants. It has been proven that VUV photolysis is a more efficient and economical process than the UV photolysis (Huang et al., 2013).
VUV photolysis has also been used in the treatment of wastewater. It has proven to be effective for the treatment of certain types of wastewater pollutants such as fluorescence of phenol, nitrobenzene, SDBS surfactants, Methylene Blue (MB), and other pollutants (Imoberdorf and Mohseni, 2011a,b; Zoschke et al., 2014). Alapi et al. (Alapi and Dombi, 2007a) compared the UV and VUV photolysis of phenol and revealed that the rate of degradation of phenol in the VUV process is about 2 times faster than that in the UV-irradiated process, as shown in Figure 3. Phenol decomposition is initiated by direct photolysis via a biphotonic process in the UV-irradiated solutions, while it can also take place by ·OH-based reactions in VUV-irradiated solutions (Alapi and Dombi, 2007a).
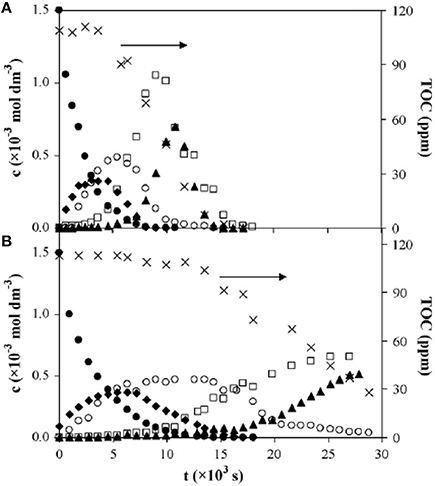
Figure 3. Time dependences of concentrations of phenol (∙), the overall concentrations of 1,2- and 1,4-dihydroxybenzene (♦), the overall concentrations of malic, maleic, and tartaric acids (°); concentrations of oxalic acid (▴), the overall concentrations of the hydroperoxide (□) and total organic carbon (TOC) concentrations (×) in (A) UV/VUV, and (B) UV-irradiated solutions saturated with oxygen at 1.5 × 10−3mol·dm−3 initial concentration (Alapi and Dombi, 2007a).
Recently, much attempt was made using VUV to degrade air pollutants, such as benzene (Huang H. B. et al., 2014), methylbenzene (Jeong et al., 2006), chlorinated methanes (Alapi and Dombi, 2007b), gaseous α-pinene (Chen et al., 2010). Cheng (Cheng, 2011) investigated the photo-degradation of gaseous α-pinene by a VUV light. It was discoveredthat most efficient conversion was obtained under the moderate humidity condition. As shown in Figure 4, nearly 100% conversion efficiency of α-pinene was achieved in about 70 s under moderate humidity condition.
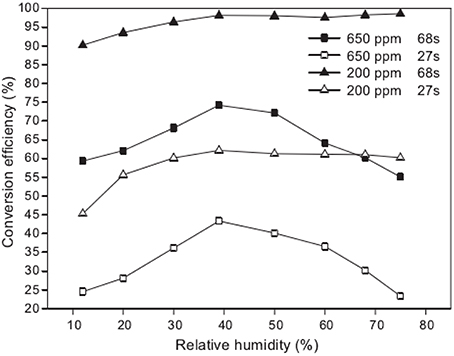
Figure 4. Effect of different humidity on the conversion efficiencies of α-pinene photo-degraded in the air (Cheng, 2011).
Meanwhile, Alapi et al. (Alapi and Dombi, 2007b) investigated the effect of relative humidity and pollutant concentration on the degradation of chlorinated methanes and their mixtures in an oxygen stream under direct VUV photolysis. Chen et al. (2010) used VUV photo-degradation to improve the removal capacity of gaseous α-pinene which is hydrophobic and poorly biodegradable. ? investigated the effect of relative humidity, residence time, initial benzene concentration, and reaction temperature on photo-oxidation of gaseous benzene by 185 nm VUV irradiation. It was discovered that benzene removal efficiency can be greatly improved in the presence of vapor and the formation of hydroxyl radicals is mainly responsible for the degradation. The abovementioned experiments indicated that VUV photolysis is a promising approach to degrade air pollutants.
However, VUV photolysis alone is not effective enough for the total oxidation of organic compounds because of the limited efficiencies that may lead to the formation of organic intermediates (Zhao and Yang, 2003; Alapi and Dombi, 2007a). More importantly, VUV photolysis will generate much toxic O3 (Alapi and Dombi, 2007b; Yang et al., 2007), which is harmful to human health as it causes headaches, throat dryness and damage to mucus membranes at levels as low as 0.1–1 ppm (Huang et al., 2011). Thus, the residual O3 will cause secondary pollution if not removed before emission.
VUV Photolysis-Ozone Catalytic Oxidation (VUV-OZCO)
Apart from the VUV photolysis, there is another process in the VUV-based processes which has great potential to deal with air pollution. Ozone used for ozone-assisted catalytic oxidation was generally generated from high voltage discharge. The cost of this is high and the system is relatively complex. In addition, the degradation efficiency of VUV photolysis alone is low and the residual ozone brings new pollution (Bergonzo and Boyd, 1993; Hashem et al., 1997; Fu, 2011). To avoid the above problems, VUV photolysis can be combined with ozone-assisted catalytic oxidation to make use of the residual ozone and eliminate it. Such a novel process is called VUV-OZCO, in which VOCs would be initially destructed by the VUV photolysis and then be further oxidized by the OZCO using the residual O3 from the VUV photolysis. VUV-OZCO would not only save the cost but also improve the pollutants removal efficiency and eliminate the problem of residual ozone. A possible system of the abovementioned process is shown in Figure 5.
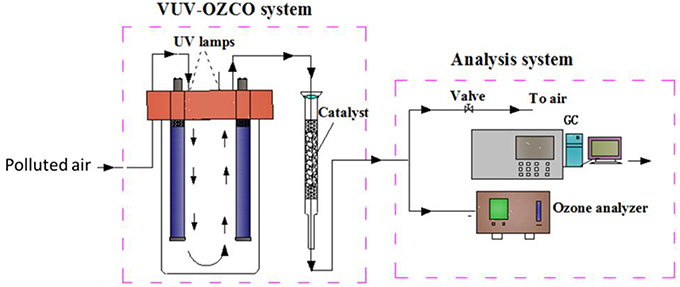
Figure 5. The schematic diagram of VUV-OZCO system (Huang, 2015).
As mentioned above, VUV-OZCO degrades pollutant via two path ways: (1) VUV photolysis; (2) Ozone-assisted catalytic oxidation. The mechanism of VUV photolysis has been described above. The mechanism of catalytic oxidation of ozone varies under different reaction conditions. Under low temperature and low humidity condition, catalytic decomposition of ozone involves two irreversible reactions, that is, the adsorption of ozone and desorption of oxygen atom on the surface of catalyst. The specific processes are as follow (Dhandapani and Oyama, 1997; Li and Oyama, 1998; Equations 26–28):
where * is the symbol of the catalyst surface active site.
Under high humidity, the catalyst surface will form a layer of liquid membrane and generate active site by the interaction between metal ions and adsorption liquid membrane. Besides, the liquid film will react with intermediate O* to generate hydroxyl radicals, which can be used to degrade ozone and pollutants. Some studies have shown that the increased humidity is beneficial to improve the removal efficiency of ozone decomposition and air pollutants. Specific processes are shown in Equations (29–34) (Dhandapani and Oyama, 1997; Einaga and Futamura, 2004b):
Under certain humidity, ozone catalytic oxidation uses highly reactive intermediates O*, and ·OH to degrade organic pollutants (Equations 35–37).
where R represents the pollutant to be degraded.
Huang et al. (Huang, 2015) firstly developed this system to degrade VOCs and studied its performance and mechanism. The results show that both benzene and O3are completely removed by VUV-OZCO with Mn/ZSM-5 catalysts. As a new technology, few studies have been carried out using VUV-OZCO for air pollution control. However, it provides an efficient and promising process for the degradation of VOCs. This VUV-OZCO process would not only improve the degradation and the mineralization rate, but it will also remove residual ozone.
Photocatalytic Oxidation under VUV Irradiation (VUV-PCO)
VUV-PCO without Ozone Oxidation
Besides the emission of by-products such as ozone, VUV photolysis alone will cause wastage of the UV energy since only 185 nm VUV irradiation can be directly used to destruct the air pollutants while the 254 nm UV irradiation, which is the main emission of the VUV lamps, is not utilized. To fully make use of the UV irradiation, photocatalysts were introduced into the VUV photolysis process. Such process is called VUV-PCO process. A possible installation of the VUV-PCO system is shown in Figure 6.
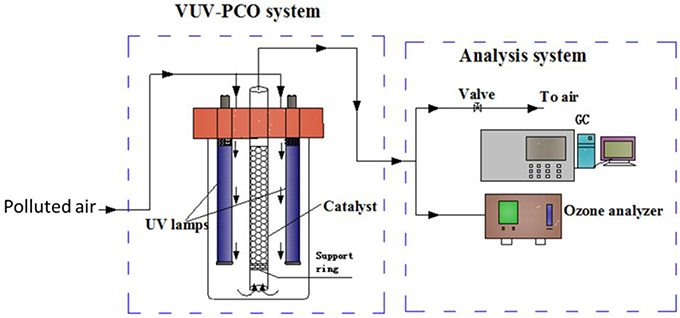
Figure 6. Schematic diagram of a VUV-PCO system (Huang et al., 2015a).
It is well known that conventional PCO has some disadvantages, such as catalyst deactivation (Peral and Ollis, 1997; Cao et al., 2000), low degradation rate (Mo et al., 2009), formation of toxic byproducts (Huang and Li, 2011), recombination of electron-hole pair, and low efficiency (Mo et al., 2009; Huang and Li, 2011). It has proven that VUV-PCO can obviously improve the efficiency and stability performance of PCO of pollutants and reduce the generation of intermediates (Zhang and Liu, 2004; Yu and Lee, 2007; Huang et al., 2009; Huang and Li, 2011). Huang et al. (2011) compared the VUV, VUV-PCO, UV-PCO processes for toluene destruction. Results showed that the toluene removal efficiency in the VUV-PCO process was 7 times more than that in the UV-PCO process, and no obvious deactivation was observed in the former, as shown in Figure 7.
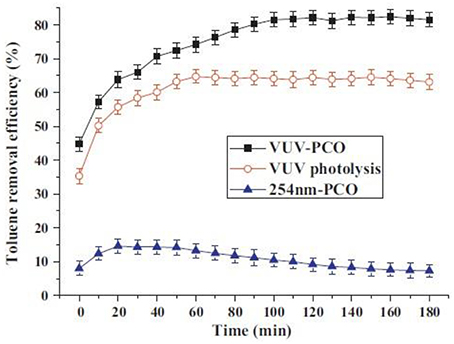
Figure 7. Toluene removal efficiency in different processes (Huang et al., 2011).
Compared with VUV photolysis, toluene removal was greatly improved. Meanwhile, both the organic compounds and ozone were significantly reduced. In contrast with the UV-PCO, the VUV-PCO can destruct pollutants with more pathways, besides UV-PCO and UV photolysis (Huang et al., 2011, 2015a). Ozone has a strong electro-negativity, which improves the ability to capture the photon-generated electrons to produce hydroxyl radicals (Huang et al., 2009; Huang and Li, 2011). Meanwhile, ozone can inhibit the combination between electronics and electronic acupuncture point to improve the photoluminescence efficiency (Huang et al., 2009; Huang and Li, 2011). O3 is a byproduct from the VUV lamp, which is also a strong oxidizing agent. Although ozone alone cannot directly oxidize refractory VOCs such as benzene and toluene, it can be decomposed into highly active oxygen through catalytic decomposition for VOCs oxidation (Huang et al., 2015a).
VUV-PCO with Ozone Oxidation
Despite the fact that ozone can help to decompose the VOCs, it can only be partially decomposed and utilized since most of the photocatalysts developed have limited activity toward ozone decomposition (Jeong et al., 2004). The residual ozone still posesa great challenge to achieve a good solution of free secondary pollution. Recently, modified photocatalysts were studied to further eliminate O3 efficiently while enhancing the removal performance of VOCs.
In the study of Fu (2014), Pt modified TiO2 was used to degrade gaseous formaldehyde and O3 byproduct under the UV, UV+O3, and VUV-PCO processes. The FE-SEM image of TiO2 film modified with Pt nanoparticles is shown in Figure 8. It was discovered that ~4–8 Pt nanoparticles (NPs) were deposited onto each TiO2 particle and this uniform dispersion of Pt(NPs) was beneficial for increasing the amount of reactive sites and metal-TiO2 contact area, which result in better performance of HCHO degradation. The above experiments showed that the degradation rate of HCHO decreases in the order of VUV>O3+UV254 nm>UV254 nm for both photocatalysts studied, as indicated in Figure 9. In addition, in the VUV-PCO process, O3 is utilized to strengthen the efficiency of PCO of VOCs.
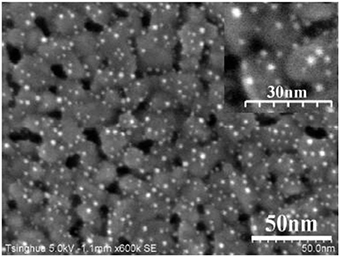
Figure 8. Ultra high-resolution FESEM image of TiO2 film modified with Pt nanoparticles (Fu, 2014).
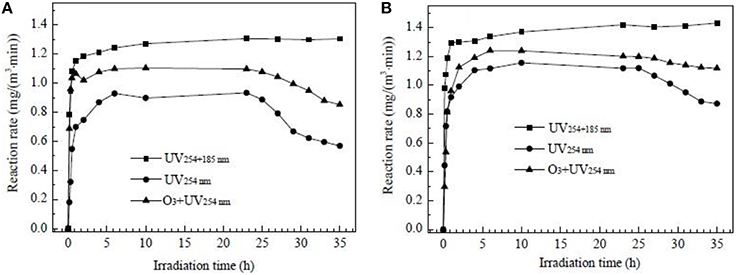
Figure 9. Degradation rate of formaldehyde as a function of irradiation time under UV254, O3+UV254, and UV254+185 nm irradiation over pure TiO2 (A) and Pt-TiO2 films (B) (Fu, 2014).
Meanwhile, Kim (Kim et al., 2014) deposited palladium nanoparticles onto TiO2 film for simultaneous removal of toluene and ozone byproduct. Compared with the direct VUV photolysis and the TiO2/VUV photocatalytic processes, Pd–TiO2/VUV process exhibited a better performance toward toluene degradation and 90% of the ozone generated by the 185 nm VUV photolysis was simultaneously degraded, as shown in Figure 10. Immersion time reflects to the time that he immersed the TiO2 film into a palladium colloid solution in order to deposit palladium nanoparticles onto the TiO2 film.
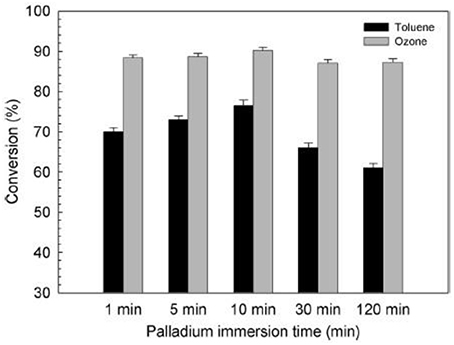
Figure 10. Effect of the immersion time on the activity of Pd–TiO2 films to degrade toluene and ozone under VUV irradiation (Kim et al., 2014).
Huang et al. (2015a) prepared TiO2 modified by transition metals (Mn, Co, Cu, Ni, and Fe) for studying the PCO of gaseous benzene under VUV irradiation. They found that the PCO efficiency of benzene under VUV irradiation reached 58%, which is over 20 times higher than that under 254 nm UV irradiation, as shown in Table 1. In addition, ozone can be completely eliminated by MnO2/TiO2.
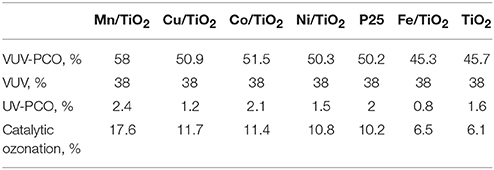
Table 1. Contribution of various sub-processes to benzene removal (Huang et al., 2015a).
Compared to UV-PCO and VUV, VUV-PCO showed higher performance, less byproducts and better stability during VOCs oxidation. Among the multiple pathways in VUV-PCO, photolysis, and catalytic ozonation played a dominant role in VOCs destruction while the contribution of UV-PCO is relatively low, as shown in Table 1 (Huang et al., 2015a). More efforts should be made to develop efficient materials with excellent photocatalytic activity and superior capacity for ozone decomposition. Although the stability of VUV-PCO was greatly increased compared to UV-PCO, VUV-PCO still faces the challenge of catalytic deactivation due to the accumulation of organic intermediates and water vapor on the surface (Quici et al., 2010). Thus, how to inhibit catalyst deactivation and increase the stability poses another challenge in the VUV-PCO process.
The porous support may be a way to solve the deactivation problem and increase the efficiency of degradation. Yuan et al. (2013) have used H-ZSM-5 as the photocatalyst support to generate the TiO2/M-ZSM-5 catalyst (M = Zn, Cu, Mn). The prepared samples were used as catalyst for degradation of gaseous acetaldehyde in the presence of UV light irradiation, O3 or UV–O3. TiO2/Mn-ZSM-5 presents the highest activity with acetaldehyde degradation rate of 78.9%, as shown in Figure 11. The superior performance results from the coupling effect of photocatalytic and ozone oxidation.
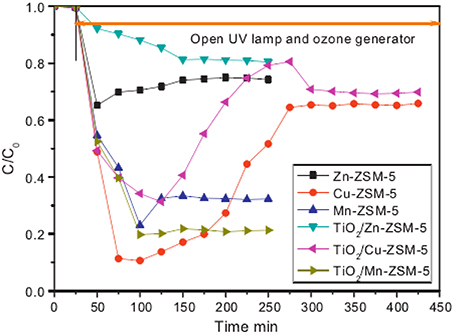
Figure 11. Degradation of acetaldehyde on M-ZSM-5 and TiO2/M-ZSM-5 under UV–ozone (UV main wavelength, 254 nm; inlet concentration of CH3CHO, 269 ppm; space velocity, 67,000 h−1; inlet concentration of ozone, 131 ppm) (Yuan et al., 2013).
Porous material like ZSM-5 can adsorb the pollutants so that it can prolong the reaction time of photocatalysis and ozone catalytic oxidation thereby improving the efficiency of degradation of pollutants. With its intensive oxidation performance, it will accumulate less intermediates thereby solving the problem of deactivation. Therefore, further research on VUV-based process in the future should adopt this catalyst and find out its potential.
Outlooks in VUV-Based Processes for Air Pollutants Degradation
To increase the utilization of VUV irradiation and the residual ozone, VUV-OZCO, and VUV-PCO with ozone oxidation should be focused on, in further study. More attention should be paid on:
(1) Catalytic deactivation. Catalyst deactivation is mainly caused by water deposition and the accumulation of intermediates on the surface of catalysts (Gandhi et al., 2012). It may be solved by hydrophobic catalysts (Zhao and Lu, 1998; Kuwahara et al., 2009) and by metal doping on the catalysts to improve the rate of mineralization and oxidation ability (Qi and Yang, 2004; Wang et al., 2012; Yang et al., 2014).
(2) Mechanism study. VUV-PCO is an emerging process for VOCs degradation and few works were carried out. The mechanism of it is still unclear, which prevent making the best use of it for air pollution control. Therefore, further study should be continued to find out the relationship between VUV photolysis, photocatalysis, and ozone oxidation.
(3) Immobilization of the catalysts (Lei et al., 2009). Most of the catalysts developed are powder or particle catalysts. They cannot be widely used in the industry since it is hard to be reused and regenerated. It is necessary to develop supported catalysts such as honeycomb.
Summary
VUV-based process is an emerging technology. It is very efficient for pollutants degradation as compared with conventional process. The development of VUV-based processes has provided new direction for the generation of reactive hydroxyl radicals for pollution degradation (Chih-Ming et al., 2011).
The technology mainly involves three processes that can efficiently degrade organic compounds: direct photolysis, photocatalysis, and ozone oxidation produced by the by-product ozone. In this paper, the generation of the active groups and the effect of active group are reviewed. By producing hydroxyl radicals, these three kinds of effects can all result in fast degradation of organic matter, despite they act alone or together. The combination of the three processes enhances the oxidation and offers many opportunities in the air pollution treatment.
Although, only a few practical applications of VUV-based processes have so far been carried out this technology has great potential for air pollution control. Unlike water treatment, there is no need to worry about the low penetration of the VUV light, which is the main technical limitation on waste-water treatment. VUV irradiation will not be strongly limited by the pollutant molecules and the particles compared with the wastewater treatment. Therefore, it can improve the efficiency of the VUV lamp. In addition, existing problems of the VUV-based processes include the catalyst deactivation and the unclear degradation mechanism and the immobilization of catalyst. It is anticipated that these problems can be solved in future for efficient utilization of the process in the industry.
Author Contributions
Haibao Huang was in the charge of writing the review paper. HL and LW were in the charge of VUV-based processes for air pollutants degradation. Huiling Huang and JZ were in the charge of introduction and generation of the oxidants. DL was in the charge of outlooks and summary.
Conflict of Interest Statement
The authors declare that the research was conducted in the absence of any commercial or financial relationships that could be construed as a potential conflict of interest.
Acknowledgments
The authors gratefully acknowledge the financial supports from National Nature Science Foundation of China (No. 51208207), Doctoral Program of Higher Education of China (No. 20120172120039), Key Science and Technology Project in Guangzhou (No. 2014Y2-00094) and Guangdong (No. 2015B020236004), NSFC-RGC (No. 51561165015, No. N_HKU718/15), and the Key Fundamental Research Funds for the Central Universities (15lgjc07).
References
Aizpuru, A., Malhautier, L., Roux, J.-C., and Fanlo, J.-L. (2001). Biofiltration of a mixture of volatile organic emissions. Waste Manag. Assoc. 51, 1662–1670. doi: 10.1080/10473289.2001.10464388
Alapi, T., and Dombi, A. (2007a). Comparative study of the UV and UV/VUV-induced photolysis of phenol in aqueous solution. J. Photochem. Photobiol. A 188, 409–418. doi: 10.1016/j.jphotochem.2007.01.002
Alapi, T., and Dombi, A. (2007b). Direct VUV photolysis of chlorinated methanes and their mixtures in an oxygen stream using an ozone producing low-pressure mercury vapour lamp. Chemosphere 67, 693–701. doi: 10.1016/j.chemosphere.2006.10.066
Atkinson, R., Baulch, D. L., Cox, R. A., Crowley, J. N., Hampson, R. F., Hynes, R. G., et al. (2004). Evaluated Kinetic and Photochemical Data for Atmospheric Chemistry: Volume I - Gas Phase Reactions of Ox, HOx, NOx and SOx Species. Copernicus GmbH.
Atkinson, R., Baulich, D. L., Cox, R. A., Crowley, J. N., Hampson, R. F., Kerr, J. A., et al. (1996). Summary of evaluated kinetic and photochemical data for atmospheric chemistry iupac subcommittee on gas kinetic data evaluation for atmospheric chemistry. Atmos. Environ. 30, 3903–3904. doi: 10.1016/1352-2310(96)87633-3
Baker, R. W., Simmons, V. L., Kaschemekat, J., and Wijmans, J. G. (1994). Membrane systems for VOC recovery from air streams. Separation 31, 231–235. doi: 10.1016/0015-1882(94)80376-5
Barbero, B. P., Costa-Almeida, L., Sanz, O., Morales, M. R., Cadus, L. E., and Montes, M. (2008). Washcoating of metallic monoliths with a MnCu catalyst for catalytic combustion of volatile organic compounds. Chem. Eng. J. 139, 430–435. doi: 10.1016/j.cej.2007.12.033
Bergonzo, P., and Boyd, I. W. (1993). Low pressure photodeposition of silicon nitride films using a xenon excimer lamp. Appl. Phys. Lett. 63, 1757–1759. doi: 10.1063/1.110705
Bolton, J. R., and Denkewicz, R. (2008). Synergistic Disinfection of Drinking Water Using Ultraviolet and Ozone Co-generated from the Same UV Lamp. World Water Congress on Ozone and Ultraviolet Technologies.
Brito, J., Wurm, F., Yanez-Serrano, A. M., de Assuncao, J. V., Godoy, J. M., and Artaxo, P. (2015). Vehicular emission ratios of VOCs in a megacity impacted by extensive ethanol use: results of ambient measurements in Sao Paulo, Brazil. Environ. Sci. Technol. 49, 11381–11387. doi: 10.1021/acs.est.5b03281
Cao, L. X., Gao, Z., and Suib, S. L. (2000). Photocatalytic oxidation of toluene on nanoscale TiO2 catalysts: studies of deactivation and regeneration. Am. Chem. Soc. 220, 269. doi: 10.1006/jcat.2000.3050
Chen, J. M., Cheng, Z. W., Jiang, Y. F., and Zhang, L. L. (2010). Direct VUV photodegradation of gaseous alpha-pinene in a spiral quartz reactor: intermediates, mechanism, and toxicity/biodegradability assessment. Chemosphere 81, 1053–1060. doi: 10.1016/j.chemosphere.2010.09.060
Cheng, Z.-W. (2011). Conversion characteristics and kinetic analysis of gaseous α-pinene degraded by a VUV light in various reaction media. Sep. Purif. Technol. 77, 26–32. doi: 10.1016/j.seppur.2010.11.014
Chih-Ming, M., Gui-Bing, H., Hua-Wei, C., Nguyen-Thi, H., and Yung-Shuen, S. (2011). Photooxidation contribution study on the decomposition of Azo dyes in aqueous solutions by VUV-based AOPs. Int. J. Photoenergy 2011:156456. doi: 10.1155/2011/156456
Daifullah, A. A. M., and Girgis, B. S. (2003). Impact of surface characteristics of activated carbon on adsorption of BTEX. Colloids Surf. A 214, 181–193. doi: 10.1016/S0927-7757(02)00392-8
Daniels, S. L. (2002). “On the ionization of air for removal of noxious effluvia” (Air ionization of indoor environments for control of volatile and particulate contaminants with nonthermal plasmas generated by dielectric-barrier discharge). IEEE Trans. Plasma Sci. 30, 1471–1481. doi: 10.1109/TPS.2002.804211
Deshusses, M. A., and Johnson, C. T. (2000). Development and validation of a simple protocol to rapidly determine the performance of biofilters for VOC treatment. Technology 34, 461–467. doi: 10.1021/es9909172
Dhandapani, B., and Oyama, S. T. (1997). Gas phase ozone decomposition catalysts. Appl. Catal. B 11, 129–166. doi: 10.1016/S0926-3373(96)00044-6
Dulin, J. M., and Rosar, E. C. (1975). Air Pollution Control Process for Glass Manufacture (Chicago, IL: Industrial Resources, Inc.).
Einaga, H., and Futamura, S. (2004a). Comparative study on the catalytic activities of alumina-supported metal oxides for oxidation of benzene and cyclohexane with ozone. React. Kinet. Catal. Lett. 81, 121–128. doi: 10.1023/B:REAC.0000016525.91158.c5
Einaga, H., and Futamura, S. (2004b). Catalytic oxidation of benzene with ozone over alumina-supported manganese oxides. J. Catal. 227, 304–312. doi: 10.1016/j.jcat.2004.07.029
Einaga, H., and Futamura, S. (2005). Oxidation behavior of cyclohexane on alumina-supported manganese oxides with ozone. Appl. Catal. B 60, 49–55. doi: 10.1016/j.apcatb.2005.02.017
Einaga, H., Harada, M., and Ogata, A. (2009). Relationship between the structure of manganese oxides on alumina and catalytic activities for benzene oxidation with ozone. Catal. Lett. 129, 422–427. doi: 10.1007/s10562-008-9814-9
Einaga, H., and Ogata, A. (2009). Benzene oxidation with ozone over supported manganese oxide catalysts: effect of catalyst support and reaction conditions. J. Hazard. Mater. 164, 1236–1241. doi: 10.1016/j.jhazmat.2008.09.032
Einaga, H., and Ogata, A. (2010). Catalytic oxidation of benzene in the gas phase over alumina-supported silver catalysts. Environ. Sci. Technol. 44, 2612–2617. doi: 10.1021/es903095j
Einaga, H., Teraoka, Y., and Ogata, A. (2013). Catalytic oxidation of benzene by ozone over manganese oxides supported on USY zeolite. J. Catal. 305, 227–237. doi: 10.1016/j.jcat.2013.05.016
Fu, P. (2011). Photocatalytic degradation of low concentration formaldehyde and simultaneous elimination of ozone by-product using palladium modified TiO2 films under UV254+185nm irradiation. Appl. Catal. B 105, 220–228. doi: 10.1016/j.apcatb.2011.04.021
Fu, P. (2014). Characterization of Pt-TiO2 film used in three formaldehyde photocatalytic degradation systems UV254 nm, O3+UV254nm and UV254+185nm via X-ray photoelectron spectroscopy. Chin. J. Catal. 35, 210–218. doi: 10.1016/S1872-2067(12)60740-2
Fu, P., Zhang, P., and Li, J. (2011). Photocatalytic degradation of low concentration formaldehyde and simultaneous elimination of ozone by-product using palladium modified TiO2 films under UV254+185nm irradiation. Appl. Catal. B 105, 220–228. doi: 10.1016/j.apcatb.2011.04.021
Gandhi, V. G., Mishra, M. K., and Joshi, P. A. (2012). A study on deactivation and regeneration of titanium dioxide during photocatalytic degradation of phthalic acid. J. Ind. Eng. Chem. 18, 1902–1907. doi: 10.1016/j.jiec.2012.05.001
Gonzalez, M., Oliveros, E., Worner, M., and Braun, A. (2004). Vacuum-ultraviolet photolysis of aqueous reaction systems. J. Photochem. Photobiol. C 5, 225–246. doi: 10.1016/j.jphotochemrev.2004.10.002
Hashem, T., Zirlewagen, M., and Braun, A. (1997). Simultaneous photochemical generation of ozone in the gas phase and photolysis of aqueous reaction systems using one VUV light source. Water Sci. Technol. 35, 41–48. doi: 10.1016/S0273-1223(97)00007-3
Huang, H. (2015). “Efficient degradation of gaseous benzene by VUV photolysis combined with ozone-assisted catalytic oxidation: performance and mechanism,” in The 7th China- Japan Workshop on Environmental Catalysis and Eco-Materials (Guangzhou).
Huang, H., Huang, H., Zhang, L., Hu, P., Xu, Y., Ye, X., et al. (2014). Photooxidation of gaseous benzene by 185 nm VUV irradiation. Environ. Eng. Sci. 31, 481–486. doi: 10.1089/ees.2014.0100
Huang, H., Huang, H., Zhang, L., Hu, P., Ye, X., and Leung, D. Y. C. (2015a). Enhanced degradation of gaseous benzene under vacuum ultraviolet (VUV) irradiation over TiO2 modified by transition metals. Chem. Eng. J. 259, 534–541. doi: 10.1016/j.cej.2014.08.057
Huang, H., Leung, D. Y. C., Kwong, P. C. W., Xiong, J., and Zhang, L. (2013). Enhanced photocatalytic degradation of methylene blue under vacuum ultraviolet irradiation. Catal. Today 201, 189–194. doi: 10.1016/j.cattod.2012.06.022
Huang, H., Leung, D. Y. C., Li, G., Leung, M. K. H., and Fu, X. (2011). Photocatalytic destruction of air pollutants with vacuum ultraviolet (VUV) irradiation. Catal. Today 175, 310–315. doi: 10.1016/j.cattod.2011.04.015
Huang, H., and Li, W. (2011). Destruction of toluene by ozone-enhanced photocatalysis: performance and mechanism. Appl. Catal. B 102, 449–453. doi: 10.1016/j.apcatb.2010.12.025
Huang, H., Xu, Y., Feng, Q., and Leung, D. Y. C. (2015b). Low temperature catalytic oxidation of volatile organic compounds: a review. Catal. Sci. Technol. 5, 2649–2669. doi: 10.1039/C4CY01733A
Huang, X., Yuan, J., Shi, J., and Shangguan, W. (2009). Ozone-assisted photocatalytic oxidation of gaseous acetaldehyde on TiO2/H-ZSM-5 catalysts. J. Hazard. Mater. 171, 827–832. doi: 10.1016/j.jhazmat.2009.06.070
Imoberdorf, G., and Mohseni, M. (2011a). Modeling and experimental evaluation of vacuum-UV photoreactors for water treatment. Chem. Eng. Sci. 66, 1159–1167. doi: 10.1016/j.ces.2010.12.020
Imoberdorf, G., and Mohseni, M. (2011b). Degradation of natural organic matter in surface water using vacuum-UV irradiation. J. Hazard. Mater. 186, 240–246. doi: 10.1016/j.jhazmat.2010.10.118
Järnström, H., Saarela, K., Kalliokoski, P., and Pasanen, L. A. (2006). Reference values for indoor air pollutant concentrations in new, residential buildings in Finland. Atmos. Environ. 40, 7178–7191. doi: 10.1016/j.atmosenv.2006.06.021
Jeong, J., Sekiguchi, K., and Sakamoto, K. (2004). Photochemical and photocatalytic degradation of gaseous toluene using short-wavelength UV irradiation with TiO2 catalyst: comparison of three UV sources. Chemosphere 57, 663–671. doi: 10.1016/j.chemosphere.2004.05.037
Jeong, J., Sekiguchi, K., Saito, M., Lee, Y., Kim, Y., and Sakamoto, K. (2006). Removal of gaseous pollutants with a UV-C254+185nm/TiO2 irradiation system coupled with an air washer. Chem. Eng. J. 118, 127–130. doi: 10.1016/j.cej.2005.11.020
Jiang, Z., Chen, M., Shi, J., Yuan, J., and Shangguan, W. (2015). Catalysis removal of indoor volatile organic compounds in room temperature:from photocatalysis to active species assistance catalysis. Catal. Surv. Asia 19, 1–16. doi: 10.1007/s10563-014-9177-8
Kim, J., Zhang, P., Li, J., Wang, J., and Fu, P. (2014). Photocatalytic degradation of gaseous toluene and ozone under UV254+185 nm irradiation using a Pd-deposited TiO2 film. Chem. Eng. J. 252, 337–345. doi: 10.1016/j.cej.2014.05.015
Konova, P., Stoyanova, M., Naydenov, A., Christoskova, S., and Mehandjiev, D. (2006). Catalytic oxidation of VOCs and CO by ozone over alumina supported cobalt oxide. Appl. Catal. A 298, 109–114. doi: 10.1016/j.apcata.2005.09.027
Konstantinou, I. K., and Albanis, T. A. (2004). TiO2-assisted photocatalytic degradation of azo dyes in aqueous solution: kinetic and mechanistic investigations. Appl. Catal. B 49, 1–14. doi: 10.1016/j.apcatb.2003.11.010
Kutschera, K., Bornick, H., and Worch, E. (2009). Photoinitiated oxidation of geosmin and 2-methylisoborneol by irradiation with 254 nm and 185 nm UV light. Water Res. 43, 2224–2232. doi: 10.1016/j.watres.2009.02.015
Kuwahara, Y., Maki, K., Matsumura, Y., Kamegawa, T., Mori, K., and Yamashita, H. (2009). Hydrophobic modification of a mesoporous silica surface using a fluorine-containing silylation agent and its application as an advantageous host material for the TiO2 photocatalyst. Ind. Eng. Chem. Res. 113, 1552–1559. doi: 10.1021/jp809191v
Kwong, C. W., and Chao, C. Y. H. (2010). Fly-ash products from biomass co-combustion for VOC control. Bioresour. Technol. 101, 1075–1081. doi: 10.1016/j.biortech.2009.09.008
Lee, B. (1991). Highlights of the clean air act amendments off 1990. Waste Manag. Assoc. 41, 16–19. doi: 10.1080/10473289.1991.10466820
Lei, Z., Liu, X., and Jia, M. (2009). Modeling of Selective Catalytic Reduction (SCR) for NO removal using monolithic honeycomb catalyst. Energy Fuels 23, 6146–6151. doi: 10.1021/ef900713y
Li, W., and Oyama, S. T. (1998). Mechanism of ozone decomposition on a manganese oxide catalyst. 2. Steady-state and transient kinetic studies. J. Am. Chem. Soc. 120, 9047–9052. doi: 10.1021/ja9814422
Li, W. B., Wang, J. X., and Gong, H. (2009). Catalytic combustion of VOCs on non-noble metal catalysts. Catal. Today 148, 81–87. doi: 10.1016/j.cattod.2009.03.007
Linsebigler, A. L., Lu, G., and Yates, J. T. (1995). Photocatalysis on TiO2 surfaces: principles, mechanisms, and selected results. Chem. Rev. 95, 735–758. doi: 10.1021/cr00035a013
Lopez, J. L., Einschlag, F. S. G., González, M. C., Capparellia, A. L., Oliveros, E., Hashemet, T. M., et al. (2000). Hydroxyl radical initiated photodegradation of 4-chloro-3,5-dinitrobenzoic acid in aqueous solution. J. Photochem. Photobiol. A 137, 177–184. doi: 10.1016/S1010-6030(00)00357-9
Lu, C., Chu, W., and Lin, M.-R. (2000). Removal of BTEX vapor from waste gases by a trickle bed biofilter. Waste Manag. Assoc. 50, 411–417. doi: 10.1080/10473289.2000.10464021
Masschelein, W. J., and Rice, R. G. (2002). Ultraviolet Light in Water and Wastewater Sanitation (Boca Raton, FL: CRC Press).
McGwin, G. Jr., Lienert, J., and Kennedy, J. I. Jr. (2010). Formaldehyde exposure and asthma in children: a systematic review. Environ. Health Perspect. 118, 313–317. doi: 10.1289/ehp.0901143
Mo, J., Zhang, Y., Xu, Q., Zhu, Y., Lamson, J. J., and Zhao, R. (2009). Determination and risk assessment of by-products resulting from photocatalytic oxidation of toluene. Appl. Catal. B 89, 570–576. doi: 10.1016/j.apcatb.2009.01.015
Moura, C. P., Vidal, C. B., Barros, A. L., Costa, L. S., Vasconcellos, L. C. G., Dias, F. S., et al. (2011). Adsorption of BTX (benzene, toluene, o-xylene, and p-xylene) from aqueous solutions by modified periodic mesoporous organosilica. J. Colloid Interface Sci. 363, 626–634. doi: 10.1016/j.jcis.2011.07.054
Niu, H., Leung, D. Y. C., Wong, C., Zhang, T., Chan, M., and Leung, F. C. C. (2014). Nitric oxide removal by wastewater bacteria in a biotrickling filter. J. Environ. Sci. 26, 555–565. doi: 10.1016/S1001-0742(13)60456-8
Peral, J., and Ollis, D. F. (1997). TiO2 photocatalyst deactivation by gas-phase oxidation of heteroatom organics. J. Mol. Catal. A 115, 347–354. doi: 10.1016/S1381-1169(96)00330-5
Qi, G., and Yang, R. T. (2004). Characterization and FTIR Studies of MnOx-CeO2 Catalyst for Low-Temperature Selective Catalytic Reduction of NO with NH3. J. Phys. Chem. 108, 15738–15747. doi: 10.1021/jp048431h
Quici, N., Vera, M. L., Choi, H., Puma, G. L., Dionysiou, D. D., Litter, M. I., et al. (2010). Effect of key parameters on the photocatalytic oxidation of toluene at low concentrations in air under 254 + 185 nm UV irradiation. Appl. Catal. B 95, 312–319. doi: 10.1016/j.apcatb.2010.01.009
Radhakrishnan, R., and Oyama, S. T. (2001). Ozone decomposition over manganese oxide supported on ZrO2 and TiO2: a kinetic study using in situ laser Raman spectroscopy. J. Catal. 199, 282–290. doi: 10.1006/jcat.2001.3167
Reed, C., Lee, Y.-K., and Oyama, S. T. (2006). Structure and oxidation state of silica-supported manganese oxide catalysts and reactivity for acetone oxidation with ozone. J. Phys. Chem. B 110, 4207–4216. doi: 10.1021/jp054288w
Rene, E. R., Murthy, D. V. S., and Swaminathan, T. (2009). Treatment of benzene vapors from contaminated air stream in a laboratory-scale compost biofilter. Macedonian J. Chem. Chem. Eng. 28, 119–123.
Rezaeia, E., Soltan, J., and Chen, N. (2013). Catalytic oxidation of toluene by ozone over alumina supported manganese oxides: effect of catalyst loading. Appl. Catal. B 136–137, 239–247. doi: 10.1016/j.apcatb.2013.01.061
Schwitzgebel, J., Ekerdt, J. G., Gerischer, H., and Heller, A. (1995). Role of the oxygen molecule and of the photogenerated electron in TiO2-photocatalyzed air oxidation reactions. J. Phys. Chem. 99, 5633–5638. doi: 10.1021/j100015a055
Shayan, K., and Vahedpour, M. (2013). Computational study on the reaction mechanism of atmospheric oxidation of ethanol with ozone. Struct. Chem. 24, 611–621. doi: 10.1007/s11224-012-0111-2
Spigno, G., Pagella, C., Fumi, M. D., Molteni, R., and De Faveri, D. M. (2003). VOCs removal from waste gases:gas-phase bioreactor for the abatement of hexane by Aspergillus niger. Chem. Eng. Sci. 58, 739–746. doi: 10.1016/S0009-2509(02)00603-6
Standeker, S., Novak, Z., and Knez, Z. (2009). Removal of BTEX vapours from waste gas streams using silica aerogels of different hydrophobicity. J. Hazard. Mater. 165, 1114–1118. doi: 10.1016/j.jhazmat.2008.10.123
Thiruvenkatachari, R., Vigneswaran, S., and Moon, I. S. (2008). A review on UV/TiO2 photocatalytic oxidation process (Journal Review). Korean J. Chem. Eng. 25, 64–72. doi: 10.1007/s11814-008-0011-8
Tompkins, D. T., Lawnicki, B. J., Zeltner, W. A., and Anderson, M. A. (2005). Evaluation of photocatalysis for gas-phase air cleaning–Part 1: Process, technical and sizing considerations. ASHRAE Trans. 111, 60–84.
Wang, C.-H. (2004). Al2O3-supported transition-metal oxide catalysts for catalytic incineration of toluene. Chemosphere 55, 11–17. doi: 10.1016/j.chemosphere.2003.10.036
Wang, C.-H., Lin, S.-S., Chen, C.-L., and Weng, H.-S. (2006). Performance of the supported copper oxide catalysts for the catalytic incineration of aromatic hydrocarbons. Chemosphere 64, 503–509. doi: 10.1016/j.chemosphere.2005.11.023
Wang, D., Oppenländer, T., El-Din, M. G., and Bolton, J. R. (2010). Comparison of the disinfection effects of Vacuum-UV (VUV) and UV light on Bacillus subtilis spores in aqueous suspensions at 172, 222 and 254 nm. Photochem. Photobiol. 86, 176–181. doi: 10.1111/j.1751-1097.2009.00640.x
Wang, S., Ma, J., Liu, B., Jiang, Y., and Zhang, H. (2008). Degradation characteristics of secondary effluent of domestic wastewater by combined process of ozonation and biofiltration. J. Hazard. Mater. 150, 109–114. doi: 10.1016/j.jhazmat.2007.04.092
Wang, Y., Ge, C., Zhan, L., Li, C., Qiao, W., and Ling, L. (2012). MnOx–CeO2/activated carbon honeycomb catalyst for selective catalytic reduction of NO with NH3at low temperatures. Ind. Eng. Chem. Res. 51, 11667–11673. doi: 10.1021/ie300555f
Wu, X. M., Fan, Z. T., Zhu, X., Jung, K. H., Ohman-Strickland, P., Weisel, C. P., et al. (2012). Exposures to volatile organic compounds (VOCs) and associated health risks of socio-economically disadvantaged population in a “hot spot” in Camden, New Jersey. Atmos. Environ. 57, 72–79. doi: 10.1016/j.atmosenv.2012.04.029
Xing, D., Cao, Y., Li, H., and Liu, F. (2000). Applications of membrane separation systems for VOC recovery. Membr. Sci. Technol. 4:011.
Yang, L., Liu, Z., Shi, J., Zhang, Y., Hu, H., and Shangguan, W. (2007). Degradation of indoor gaseous formaldehyde by hybrid VUV and TiO2/UV processes. Sep. Purif. Technol. 54, 204–211. doi: 10.1016/j.seppur.2006.09.003
Yang, Z., Yang, Y., Zhu, X., Chen, G., and Zhang, W. (2014). An outward coating route to CuO/MnO2Nanorod array films and their efficient catalytic oxidation of acid fuchsin dye. Ind. Eng. Chem. Res. 53, 9608–9615. doi: 10.1021/ie500358p
Ye, J., Shang, J., Li, Q., Xu, W., Liu, J., Feng, X., et al. (2014). The use of vacuum ultraviolet irradiation to oxidize SO(2) and NOx for simultaneous desulfurization and denitrification. J. Hazard. Mater. 271, 89–97. doi: 10.1016/j.jhazmat.2014.02.011
Ye, J., Shang, J., Song, H., Li, Q., and Zhu, T. (2013). Generation of reactive oxygen species in simulated flue gas under vacuum ultraviolet radiation. Chem. Eng. J. 232, 26–33. doi: 10.1016/j.cej.2013.07.056
Yu, B. F., Hu, Z. B., Liu, M., Yang, H. L., Kong, Q. X., and Liu, Y. H. (2009). Review of research on air-conditioning systems and indoor air quality control for human health. Int. J. Refrig. 32, 3–20. doi: 10.1016/j.ijrefrig.2008.05.004
Yu, K. P., and Lee, G. W. M. (2007). Decomposition of gas-phase toluene by the combination of ozone and photocatalytic oxidation process (TiO2/UV, TiO2/UV/O3, and UV/O3). Appl. Catal. B 75, 29–38. doi: 10.1016/j.apcatb.2007.03.006
Yuan, J., Huang, X., Chen, M., Shi, J., and Shangguan, W. (2013). Ozone-assisted photocatalytic degradation of gaseous acetaldehyde on TiO2/M-ZSM-5 (M=Zn, Cu, Mn). Catal. Today 201, 182–188. doi: 10.1016/j.cattod.2012.06.003
Zhang, P. Y., and Liu, J. (2004). Photocatalytic degradation of trace hexane in the gas phase with and without ozone addition: kinetic study. J. Photochem. Photobiol. A 167, 87–94. doi: 10.1016/j.jphotochem.2004.05.015
Zhao, J., and Yang, X. (2003). Photocatalytic oxidation for indoor air purification: a literature review. Build. Environ. 38, 645–654. doi: 10.1016/S0360-1323(02)00212-3
Zhao, X. S., and Lu, G. Q. (1998). Modification of MCM-41 by Surface Silylation with Trimethylchlorosilane and Adsorption Study. J. Phys. Chem. 102, 1556–1561. doi: 10.1021/jp972788m
Zhu, W., Xiao, S., Zhang, D., Liu, P., Zhou, H., Dai, W., et al. (2015). Highly efficient and stable Au/CeO2-TiO2 photocatalyst for nitric oxide abatement: potential application in flue gas treatment. Langmuir 31, 10822–10830. doi: 10.1021/acs.langmuir.5b02232
Zoschke, K., Bornick, H., and Worch, E. (2014). Vacuum-UV radiation at 185 nm in water treatment–a review. Water Res. 52, 131–145. doi: 10.1016/j.watres.2013.12.034
Keywords: VUV-PCO, photolysis, ozone-assisted oxidation, photocatalysis, air pollutants
Citation: Huang H, Lu H, Huang H, Wang L, Zhang J and Leung DYC (2016) Recent Development of VUV-Based Processes for Air Pollutant Degradation. Front. Environ. Sci. 4:17. doi: 10.3389/fenvs.2016.00017
Received: 09 January 2016; Accepted: 29 February 2016;
Published: 15 March 2016.
Edited by:
Rajasekhar Balasubramanian, National University of Singapore, SingaporeReviewed by:
Eduardo Landulfo, Instituto de Pesquisas Energéticas e Nucleares, BrazilCláudia Gomes Silva, Associate Laboratory LSRE-LCM, Portugal
Copyright © 2016 Huang, Lu, Huang, Wang, Zhang and Leung. This is an open-access article distributed under the terms of the Creative Commons Attribution License (CC BY). The use, distribution or reproduction in other forums is permitted, provided the original author(s) or licensor are credited and that the original publication in this journal is cited, in accordance with accepted academic practice. No use, distribution or reproduction is permitted which does not comply with these terms.
*Correspondence: Haibao Huang, c2VhYmFvOEBnbWFpbC5jb20=;
Dennis Y. C. Leung, eWNsZXVuZ0Boa3UuaGs=