- 1Department of Freshwater and Marine Ecology, Institute for Biodiversity and Ecosystem Dynamics, University of Amsterdam, Amsterdam, Netherlands
- 2Department of Freshwater Ecology, Wageningen Environmental Research, Wageningen, Netherlands
Bioturbation activity of sediment-dwelling organisms promotes the release of contaminants across the benthic-pelagic ecosystem boundary, thereby affecting the exposure to and uptake of sediment associated contaminants at the sediment-water interface by themselves and the entire community around them. This way, bioturbation activity may contribute to species specific sensitivities to sediment associated compounds. Therefore we assessed, based on literature data, if invertebrate bioturbation activity determines species specific sensitivities to sediment contamination. For two metals, Ni and Cu, sufficient data were available to construct Species Sensitivity Distributions (SSD). The position of the species in the SSDs could indeed be linked to their bioturbation rate: the most active bioturbators being the most sensitive benthic invertebrates. Active bioturbators thus enhance their exposure and therewith their sensitivity to sediment associated toxicants. Moreover, active bioturbators can hence promote the release of sediment-associated contaminants across the benthic-pelagic ecosystem boundary, thereby stimulating delivery of contaminants from what is often the most polluted environmental compartment in freshwater ecosystems. It is concluded that trait based ecotoxicology offers a possibly potent tool for predicting sensitivity of benthic invertebrates and the benthic community to sediment-associated contaminants.
Introduction
The largest group of animals making up the species rich benthic communities of freshwater ecosystems are invertebrates, consisting of many different taxa, like chironomids, amphipods, tubificids, and bivalves, that all perform different ecological roles (Covich et al., 1999, 2004; Hillebrand and Matthiessen, 2009). Accordingly, benthic invertebrates exhibit different ways of locomotion and different ways in which they process and rework sediments, due to differences in feeding mechanism and food acquisition (Mermillod-Blondin et al., 2002; Jonsson and Malmqvist, 2003; Nogaro et al., 2009). These ecological functional traits in turn influence the environmental conditions and sediment properties adjacent to the organisms (François et al., 1997; Gérino et al., 2003; Nogaro et al., 2009). In this way, benthic invertebrates alter and influence their own environment, as well as that of the entire community around them (Covich et al., 1999). Consequently, benthic invertebrates may also affect the exposure to and uptake of sediment associated contaminants by the whole benthic invertebrate community.
Sediment contamination in freshwater ecosystems is a major environmental issue in industrialized countries. Over the past decades, contaminants like metals, pesticides, PAHs and pharmaceuticals ended up in water bodies. A considerable part of such contaminants accumulates in sediments, which act as a sink for hydrophobic compounds (Eggleton and Thomas, 2004). While the contaminant concentrations in the water column have decreased in many instances due to improved pollution- and runoff management, the often persistent sediment-associated contaminants remain (Dsa et al., 2008; De Deckere et al., 2011). Hence, sediments nowadays play an important role in contaminant transfer and water quality, systematically causing partitioning of compounds back into the water column (Bilotta and Brazier, 2008). Thus, the sediment now acts as a contaminant source, affecting the benthic as well as the pelagic community (Taylor and Owens, 2009).
It has been shown that sediment-dwelling organisms promote the release of contaminants across the benthic-pelagic ecosystem boundary, thereby affecting the exposure to and uptake of sediment associated contaminants at the sediment-water interface by themselves and other organisms (Pang et al., 2012). This way, bioturbation activity may subsequently contribute to species specific sensitivities to sediment associated compounds (Milani et al., 2003; De Lange et al., 2005; Wang, 2013). It is these frequently observed species specific responses in sediment toxicity tests that indeed raise the question what actually determines the observed sensitivity of benthic organisms in terms of ECx values. Here we hypothesize that ecological functional traits, especially bioturbation activity, contribute to species specific sensitivities to sediment contamination. Clear indications for the importance of sediment reworking on sediment toxicity were already reported by Chandler et al. (2014), who showed that contrasting sediment reworking intensity of two infaunal benthic invertebrates, equally sensitive in water only tests, caused a significantly different sensitivity in sediment tests through increased nickel mobilization to the pore water. Based on these results, the aim of the present desk study was to assess if benthic invertebrate bioturbation activity determines species specific sensitivities to sediment contamination. To this purpose we screened available literature for sediment toxicity data, attempting to obtain sufficient data to construct Species Sensitivity Distributions (SSD). Next, it was evaluated if the position of the species in the SSD could be linked to their ecological functional traits.
Materials and Methods
We screened the available literature for freshwater sediment toxicity data. Since this study focused on sediment associated toxicants and bioturbation, only sediment toxicity data for organisms at the sediment-water interface were selected, excluding species which mainly occur in submerged vegetation. To assess the relationship between bioturbation and species-specific sensitivity, we aimed to construct a SSD for common sediment associated contaminants and benthic invertebrates with different levels of bioturbation activity. A SSD for benthic invertebrates was already constructed for nickel by Vangheluwe et al. (2013), however this study did not relate the position of species in the distribution to their bioturbation traits. The present study selected research reporting on toxicity of other sediment associated compounds to benthic invertebrates. The only substance with enough data points to construct a robust SSD was copper, with four studies reporting on the effect of sediment associated copper on the survival of eight benthic invertebrate species (Table 1 and references therein). The Cu LC50 values from these papers were combined to construct a SSD using a SSD generator (US EPA, 2016). In case a species LC50 was reported in multiple studies, the LC50 value with the longest exposure duration was used. If two articles had the same exposure period, an average of the two reported LC50 values was taken. Next it was evaluated if the position of the species in the SSD could be linked to their ecological functional traits. To this purpose the invertebrates were divided into three groups based on their species-specific bioturbation activity: high, intermediate and low. To do so, literature relevant to the bioturbation activity of the species was consulted and summarized to attribute a bioturbation activity classification (Table 1).
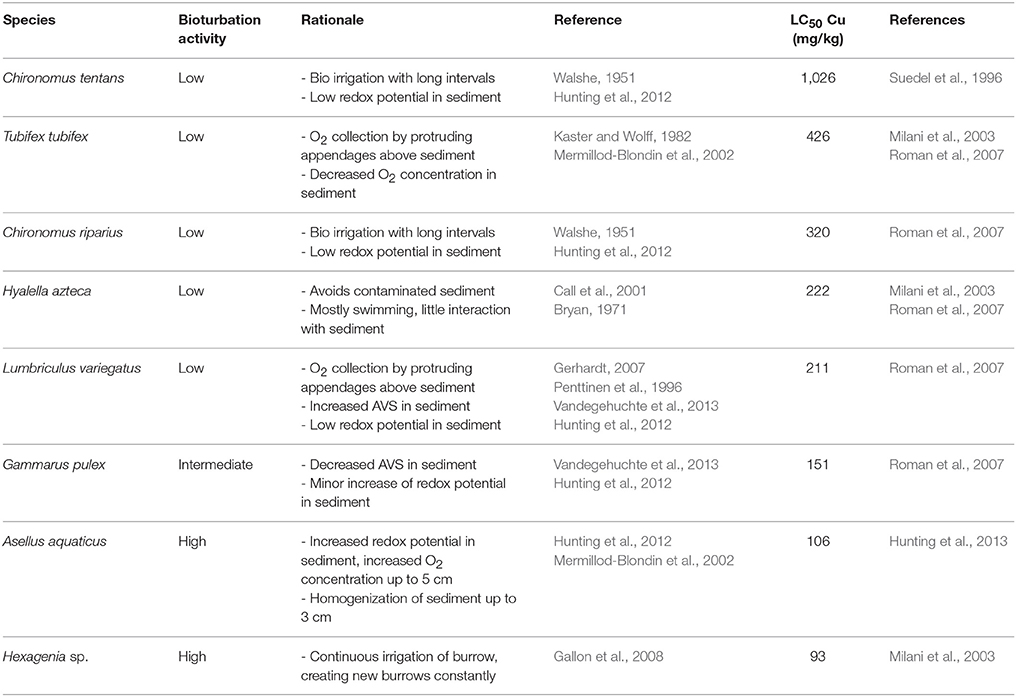
Table 1. Benthic invertebrate bioturbation activity and sediment associated Cu LC50 values derived from literature.
Results and Discussion
Kwok et al. (2014), reporting on a workshop held in 2011, stated that the paucity of sediment toxicity data posed the largest obstacle to improving current, and deriving new sediment quality guidelines (SQGs). Now, in 2017, the situation has scarcely improved. Only for two metals, Ni and Cu, sufficient sediment toxicity data were available to construct a SSD. The Ni SSD was previously reported by Vangheluwe et al. (2013), however, the link between the reported SSD and the bioturbation rate of the examined species was not described. Figure 1 shows the presently constructed SSD for Cu, based on the literature derived experimental data presented in Table 1. The position of the species in the present SSD could indeed be linked to their bioturbation rate. The most active bioturbators, Hexagenia sp., and A. aquaticus, appeared to be most sensitive to sediment associated copper, followed by G. pulex, exhibiting intermediate bioturbation activity. The species least sensitive to sediment associated copper, C. tentans, C. riparius, T. tubifex, H. azteca, and L. variegatus, were categorized as the least active bioturbators.
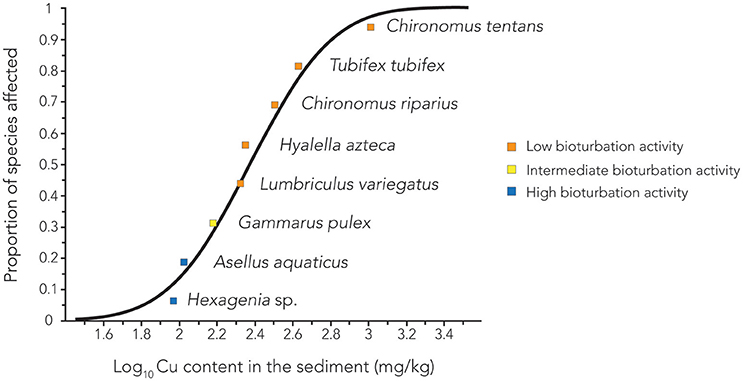
Figure 1. Species sensitivity distribution for sediment associated copper: Benthic invertebrates are categorized according to their bioturbation activity.
In agreement with the present study, Vangheluwe et al. (2013) reported that G. pseudolimneus and Hexagenia sp. were relatively sensitive to sediment bound Ni, with EC10 values all below 236 mg/kg. L. variegatus, C. riparius, C. dilitus, L. siliquoidea, and T. tubifex were less sensitive: the EC10 for L. variegatus was 554 mg/kg, while for the other species the NOEC was at least 762 mg/kg (Vangheluwe et al., 2013). Thus, the sensitive species were active bioturbating epifaunal biodiffusors, whereas the less sensitive species were infaunal conveyor belt transporters or gallery diffusors. L. siliquoidea is a sedentary bivalve and therefore not an active bioturbator. Only for H. azteca this agreement was not observed, with the amphipod exhibiting a relatively high Cu LC50 value and being classified as having a low bioturbation rate in the present study, while being relatively sensitive to sediment associated Ni in the study by Vangheluwe et al. (2013). This might be explained by the ability of H. azteca to avoid contaminated sediment by their swimming capabilities (Table 1). Hence, H. azteca is a facultative bioturbator, and in a test setup where it is able to avoid exposure by obtaining its food away from the sediment, as was the case in the Ni test (Besser et al., 2011), low bioturbation and thus low exposure to sediment associated compounds may occur. Contrastingly, in a test setup where H. azteca is compelled to obtain its food from within the sediment, active bioturbation and thus high exposure may ensue. This can in turn result in differences in observed sensitivity, caused by differential bioturbation activity within the same species, causing a shift in the position of that species in a SSD.
Besser et al. (2013) argued that the relatively high sensitivity of G. pseudolimneus and Hexagenia sp. was due to their specific sediment mixing and/or bioirrigation rates. These high rates of bioturbation and bioirrigation lead to an increase in oxygen content of the sediment and therewith to a reduction in acid volatile sulfides (AVS) concentration. This reduction in AVS concentration in turn increases the bioavailability of metals in the sediment, and promotes release of contaminants from the sediment to the pore water and the pelagic zone (De Jonge et al., 2012; Simpson et al., 2012). Although other infaunal invertebrates, like chironomids and oligochaetes, also bioirrigate their burrows, their bioirrigation rate is lower than that of the mayfly larvae. While mayfly larvae need to pump oxygen into their burrows almost continuously (Gallon et al., 2008), oligochaetes collect their oxygen by protruding their appendages above the sediment, and chironomids irrigate their burrows with long intervals, as they contain hemoglobin that allows them to withstand low oxygen concentrations (Walshe, 1951). This results in low oxygen concentrations in the sediment around oligochaetes and chironomids relative to mayfly larvae. Chironomids can, nevertheless, increase oxygen penetration depth or sediment oxygen consumption (De Haas et al., 2005), but the redox values in the sediment remain generally reducing. Hunting et al. (2012) also observed low oxygen concentrations and reducing conditions around chironomids and oligochaetes, thus potentially increasing AVS concentration. In contrast, the epifaunal biodiffusor A. aquaticus increased the sediment oxygen concentration by its bioturbation activities (Hunting et al., 2012), which may lead to a decrease in AVS concentration. In agreement, Vandegehuchte et al. (2013) demonstrated that the presence of G. pulex decreased the AVS concentration in the sediment, while the mayfly E. virgo had little effect on the AVS concentration and the presence of the oligochaete L. variegatus resulted in an increase in AVS concentration in the sediment.
Conclusion
The studies cited above all support the here presented cascade of high bioturbation activity leading to oxygenation of the sediment and therewith to low AVS concentration, in turn causing a higher metal bioavailability and leading to higher exposure and thus higher sensitivity of the test species. It is therefore concluded that active bioturbators enhance their own exposure to toxicants, therewith increasing the observed sensitivity in terms of ECx. Moreover, active bioturbators can hence promote release of sediment-associated contaminants across the benthic-pelagic ecosystem boundary, thereby stimulating delivery of contaminants from what is often the most polluted environmental compartment in freshwater ecosystems (Burton, 2013; Roig et al., 2015). Although research linking traits to toxicant sensitivity is still relatively rare, trait based ecotoxicology offers a possibly potent tool for predicting sensitivity of benthic invertebrates and the benthic community to sediment-associated contaminants (Baird et al., 2008; Archaimbault et al., 2010). It is alarming though, that the paucity of sediment toxicity data still poses the largest obstacle to deriving reliable SQGs. We therefore stress that future reliable sediment toxicity data derivation should incorporate trait based ecotoxicological assessment.
Author Contributions
Conception or design of the work: TvdM and MK. Data collection: TvdM. Data analysis and interpretation: TvdM, MdB, PV and MK. Drafting the article: TvdM, MdB, PV and MK. Critical revision of the article: MdB, PV and MK. Final approval of the version to be published: TvdM, MdB, PV and MK.
Conflict of Interest Statement
The authors declare that the research was conducted in the absence of any commercial or financial relationships that could be construed as a potential conflict of interest.
References
Archaimbault, V., Usseglio-Polatera, P., Garric, J., Wasson, J., and Babut, M. (2010). Assessing pollution of toxic sediment in streams using bio-ecological traits of benthic macroinvertebrates. Freshw. Biol. 55, 1430–1446. doi: 10.1111/j.1365-2427.2009.02281.x
Baird, D. J., Rubach, M. N., and Van den Brink, P. J. (2008). Trait-based ecological risk assessment (TERA): the new frontier? Integr. Environ. Assess. Manage. 4, 2–3. doi: 10.1897/IEAM_2007-063.1
Besser, J. M., Brumbaugh, W. G., Ingersoll, C. G., Ivey, C. D., Kunz, J. L., Kemble, N. E., et al. (2013). Chronic toxicity of nickel-spiked freshwater sediments: variation in toxicity among eight invertebrate taxa and eight sediments. Environ. Toxicol. Chem. 32, 2495–2506. doi: 10.1002/etc.2271
Besser, J. M., Brumbaugh, W. G., Kemble, N. E., Ivey, C. D., Kunz, J. L., Ingersoll, C. G., et al. (2011). Toxicity of Nickel-Spiked Freshwater Sediments to Benthic Invertebrates: Spiking Methodology, Species Sensitivity and Nickel Bioavailability. Scientific Investigations Report 2011-5225. US Geological Survey, Reston, VA.
Bilotta, G. S., and Brazier, R. E. (2008). Understanding the influence of suspended solids on water quality and aquatic biota. Water Res. 42, 2849–2861. doi: 10.1016/j.watres.2008.03.018
Bryan, A. D. (1971). Some Aspects of the Behavioural Ecology of Two Amphipod Species in Marion Lake, British Columbia. Doctoral dissertation, University of British Columbia, Vancouver, BC.
Burton, G. A. (2013). Assessing sediment toxicity: past, present and future. Environ. Toxicol. Chem. 32, 1438–1440. doi: 10.1002/etc.2250
Call, D. J., Cox, D. A., Geiger, D. L., Genisot, K. I., Markee, T. P., Brooke, L. T., et al. (2001). An assessment of the toxicity of phthalate esters to freshwater benthos. 2. Sediment exposures. Environ. Toxicol. Chem. 20, 1805–1815. doi: 10.1002/etc.5620200826
Chandler, G. T., Schlekat, C. E., Garman, E. R., He, L., Washburn, K. M., Stewart, et al. (2014). Sediment nickel bioavailability and toxicity to estuarine crustaceans of contrasting bioturbative behaviors – An evaluation of the SEM- AVS paradigm. Environ. Sci. Technol. 48, 12893–12901. doi: 10.1021/es5025977
Covich, A. P., Austen, M. C., Bärlocher, F., Chauvet, E., Cardinale, B. J., Biles, C. L., et al. (2004). The role of biodiversity in the functioning of freshwater and marine benthic systems. Bioscience 54, 767–775. doi: 10.1641/0006-3568(2004)054[0767:TROBIT]2.0.CO;2
Covich, A. P., Palmer, M. A., and Crowl, T. A. (1999). The role of benthic invertebrate species in freshwater ecosystems - Zoobenthic species influence energy flows and nutrient cycling. Bioscience 49, 119–127. doi: 10.2307/1313537
De Deckere, E., De Cooman, W., Leloup, V., Meire, P., Schmitt, C., and Von der Ohe, P. C. (2011). Development of sediment quality guidelines for freshwater ecosystems. J. Soils Sed. 11, 504–517. doi: 10.1007/s11368-010-0328-x
De Haas, E. M., Kraak, M. H. S., Koelmans, A. A., and Admiraal, W. (2005). The impact of sediment reworking by opportunistic chironomids on specialised mayflies. Freshw. Biol. 50, 770–780. doi: 10.1111/j.1365-2427.2005.01356.x
De Jonge, M., Teuchies, J., Meire, P., Blust, R., and Bervoets, L. (2012). The impact of increased oxygen conditions on metal-contaminated sediments part I: effects on redox status, sediment geochemistry and metal bioavailability. Water Res. 46, 2205–2214. doi: 10.1016/j.watres.2012.01.052
De Lange, H. J., De Haas, E. M., Maas, H., and Peeters, E. T. H. M. (2005). Contaminated sediments and bioassay responses of three macroinvertebrates, the midge larva Chironomus riparius, the water louse Asellus aquaticus and the mayfly nymph Ephoron virgo. Chemosphere 61, 1700–1709. doi: 10.1016/j.chemosphere.2005.03.083
Dsa, J. V., Johnson, K. S., Lopez, D., Kanuckel, C., and Tumlinson, J. (2008). Residual toxicity of acid mine drainage-contaminated sediment to stream macroinvertebrates: relative contribution of acidity vs. metals. Water Air Soil Pollut. 194, 185–197. doi: 10.1007/s11270-008-9707-y
Eggleton, J., and Thomas, K. V. (2004). A review of factors affecting the release and bioavailability of contaminants during sediment disturbance events. Environ. Int. 30, 973–980. doi: 10.1016/j.envint.2004.03.001
François, F., Poggiale, J. C., Durbec, J. P., and Stora, G. (1997). A new approach for the modelling of sediment reworking induced by a macrobenthic community. Acta Biotheor. 45, 295–319. doi: 10.1023/A:1000636109604
Gallon, C., Hare, L., and Tessier, A. (2008). Surviving in anoxic surroundings: how burrowing aquatic insects create an oxic microhabitat. J. North Am. Benthol. Soc. 27, 570–580. doi: 10.1899/07-132.1
Gerhardt, A. (2007). Importance of exposure route for behavioural responses in Lumbriculus variegatus Müller (Oligochaeta: Lumbriculida) in short-term exposures to Pb. Environ. Sci. Pollut. Res. Int. 14, 430–434. doi: 10.1065/espr2006.12.371
Gérino, M., Stora, G., François-Carcaillet, F., Gilbert, F., Poggiale, J. C., Mermillod-Blondin, et al. (2003). Macro-invertebrate functional groups in freshwater and marine sediments: a common mechanistic classification. Vie et Milieu 53, 221–232.
Hillebrand, H., and Matthiessen, B. (2009). Biodiversity in a complex world: consolidation and progress in functional biodiversity research. Ecol. Lett. 12, 1405–1419. doi: 10.1111/j.1461-0248.2009.01388.x
Hunting, E. R., Mulder, C., Kraak, M. H. S., Breure, A. M., and Admiraal, W. (2013). Effects of copper on invertebrate-sediment interactions. Environ. Pollut. 180, 131–135. doi: 10.1016/j.envpol.2013.05.027
Hunting, E. R., Whatley, M. H., van der Geest, H. G., Mulder, C., Kraak, M. H. S., Breure, A. M., et al. (2012). Invertebrate footprints on detritus processing, bacterial community structure, and spatiotemporal redox profiles. Freshw. Sci. 31, 724–732. doi: 10.1899/11-134.1
Jonsson, M. B., and Malmqvist, B. (2003). Importance of species identity and number for process rates within different stream invertebrate functional feeding groups. J. Anim. Ecol. 72, 453–459. doi: 10.1046/j.1365-2656.2003.00714.x
Kaster, J. L., and Wolff, R. J. (1982). A convoluted respiratory exchange surface in Tubibifex tubifex (Tubificidae). Trans. Am. Microsc. Soc. 101, 91–95. doi: 10.2307/3225574
Kwok, K. W. H., Batley, G. E., Wenning, R. J., Zhu, L., Vangheluwe, M., and Lee, S. (2014). Sediment quality guidelines: challenges and opportunities for improving sediment management. Environ. Sci. Pollut. Res. 21, 17–27. doi: 10.1007/s11356-013-1778-7
Mermillod-Blondin, F., Gerino, M., Des Chatelliers, M. C., and Degrange, V. (2002). Functional diversity among 3 detritivorous hyporheic invertebrates: an experimental study in microcosms. J. North Am. Benthol. Soc. 21, 132–149. doi: 10.2307/1468305
Milani, D., Reynoldson, T. B., Borgmann, U., and Kolasa, J. (2003). The relative sensitivity of four benthic invertebrates to metals in spiked-sediment exposures and application to contaminated field sediment. Environ. Toxicol. Chem. 22, 845–854. doi: 10.1002/etc.5620220424
Nogaro, G., Mermillod-Blondin, F., Valett, H. M., François-Carcaillet, F., Gaudet, J. P., Lafont, M., et al. (2009). Ecosystem engineers at the sediment-water interface: bioturbation and consumer-substrate interaction. Oecologia 161, 125–138. doi: 10.1007/s00442-009-1365-2
Pang, J., Sun, B., Li, H., Mehler, W. T., and You, J. (2012). Influence of bioturbation on bioavailability and toxicity of PAHs in sediment from an electronic waste recycling site in South China. Ecotoxicol. Environ. Saf. 84, 227–223. doi: 10.1016/j.ecoenv.2012.07.007
Penttinen, O.-P., Kukkonen, J., and Pellinen, J. (1996). Preliminary study to compare body residues and sublethal energetic responses in benthic invertebrates exposed to sediment-bound 2,4,5-trichlorophenol. Environ. Toxicol. Chem. 15, 160–166. doi: 10.1002/etc.5620150214
Roig, N., Sierra, J., Nadal, M., Moreno-Garrido, I., Nieto, E., Hampel, et al. (2015). Assessment of sediment ecotoxicological status as a complementary tool for the evaluation of surface water quality: the Ebro River Basin case study. Sci. Tot. Environ. 503–504, 269–278. doi: 10.1016/j.scitotenv.2014.06.125
Roman, Y. E., De Schamphelaere, K. A. C., Nguyen, L. T. H., and Janssen, C. R. (2007). Chronic toxicity of copper to five benthic invertebrates in laboratory-formulated sediment: sensitivity comparison and preliminary risk assessment. Sci. Tot. Environ. 387, 128–140. doi: 10.1016/j.scitotenv.2007.06.023
Simpson, S. L., Ward, D., Strom, D., and Jolley, D. F. (2012). Oxidation of acid-volatile sulfide in surface sediments increases the release and toxicity of copper to the benthic amphipod Melita plumulosa. Chemosphere 88, 953–961. doi: 10.1016/j.chemosphere.2012.03.026
Suedel, B. C., Deaver, E., and Rodgers, J. H. (1996). Experimental factors that may affect toxicity of aqueous and sediment-bound copper to freshwater organisms. Arch. Environ. Contamin. Toxicol. 30, 40–46. doi: 10.1007/BF00211327
Taylor, K. G., and Owens, P. N. (2009). Sediments in urban river basins: a review of sediment–contaminant dynamics in an environmental system conditioned by human activities. J. Soils Sediments 9, 281–303. doi: 10.1007/s11368-009-0103-z
US EPA (2016). Species Sensitivity Distribution Generator. Internet: Available online at: https://www.epa.gov/caddis-vol4/caddis-volume-4-data-analysis-download-software
Vandegehuchte, M. B., Nguyen, L. T. H., De Laender, F., Muyssen, B. T. A., and Janssen, C. R. (2013). Whole sediment toxicity tests for metal risk assessments: on the importance of equilibration and test design to increase ecological relevance. Environ. Toxicol. Chem. 32, 1048–1059. doi: 10.1002/etc.2156
Vangheluwe, M. L. U., Verdonck, F. A. M., Besser, J. M., Brumbaugh, W. G., Ingersoll, C. G., Schlekat, C. E., et al. (2013). Improving sediment-quality guidelines for nickel: development and application of predictive bioavailability models to assess chronic toxicity of nickel in freshwater sediments. Environ. Toxicol. Chem. 32, 2507–2519. doi: 10.1002/etc.2373
Walshe, B. M. (1951). The function of haemoglobin in relation to filter feeding in leaf-mining chironomid larvae. J. Exp. Biol. 28, 57–61.
Keywords: benthic invertebrates, bioturbation, species specific sensitivity, sediment contamination, SSD
Citation: van der Meer TV, de Baat ML, Verdonschot PFM and Kraak MHS (2017) Benthic Invertebrate Bioturbation Activity Determines Species Specific Sensitivity to Sediment Contamination. Front. Environ. Sci. 5:83. doi: 10.3389/fenvs.2017.00083
Received: 18 July 2017; Accepted: 21 November 2017;
Published: 06 December 2017.
Edited by:
Aziz Ullah, Kohat University of Science and Technology, PakistanReviewed by:
Mazhar Iqbal Zafar, Quaid-i-Azam University, PakistanNafsika Papageorgiou, Hellenic Centre for Marine Research, Greece
Copyright © 2017 van der Meer, de Baat, Verdonschot and Kraak. This is an open-access article distributed under the terms of the Creative Commons Attribution License (CC BY). The use, distribution or reproduction in other forums is permitted, provided the original author(s) or licensor are credited and that the original publication in this journal is cited, in accordance with accepted academic practice. No use, distribution or reproduction is permitted which does not comply with these terms.
*Correspondence: Milo L. de Baat, bS5sLmRlYmFhdEB1dmEubmw=