- 1LETA—Laboratory of Applied Ethology—Department of Zootechny and Rural Development, Federal University of Santa Catarina, Florianopolis, Brazil
- 2Graduate Program on Agroecosystems, Federal University of Santa Catarina, Florianópolis, Brazil
A significant share of Greenhouse Gases (GHG) produced from agriculture comes from cattle farming. The reduction in GHG emissions from ruminants fed with grains has led some researchers to recommend such a diet as a means of mitigating emissions in the sector. A more accurate balance of emissions, however, must include the carbon (C) stocked by feed crops. Within the grain production system, no-tillage (NT) cultivation systems have a greater capacity to increase and store soil organic carbon (SOC). Within grazing management systems, the rotation used in Voisin's Rational Grazing (VRG) allows the accumulation of SOC through root growth. The objective of this study was to assess the C stock of pasture under VRG and compare soil C stock between VRG pasture and fields under no-tillage management, in two seasons over a period of 1 year. The study included five dairy farms in Santa Catarina State, Brazil. In each property, we collected soil to quantify SOC from VRG pasture and NT fields, in summer and winter. In the pasture, to determine the total stock, we also collected samples from the aerial parts of plants and the roots. Further, we estimated how efficient would be producing milk from those pastures or from those crops. The VRG pasture showed a greater capacity to stock C in the soil than the no-tillage fields (VRG = 115.0 Mg C ha−1; NT = 92.5 Mg C ha−1; p < 0.00009), with the greatest difference at a depth of 0–10 cm (VRG = 41 Mg C ha−1; NT = 32 Mg C ha−1; p < 0.00008). In VRG, 95% of C was in the soil, 1% in the aerial part of plants, and 4% in the roots. On pasture was produced 0.15 kg of milk.kg−1 of C stored, and on NT system 0.13 kg of milk.kg−1 of C stored. In this study, we conclude that independent of season, the soil in well managed pastures had a greater stock of C, produced more milk and produced more milk.kg−1 of stored C than fields under NT management. Therefore, when comparing GHG emissions of ruminants with different diets, we must also quantify the SOC resulting from distinct feed production systems.
Introduction
The amount of CO2 equivalents (CO2-e) in the atmosphere, had a great increase in the last 150 years, but specially from 1960 to date (Meinshausen et al., 2017). The increase in CO2 and other Greenhouse Gas (GHG) has been considered the main cause of global warming. Livestock farming has been considered responsible for 14.5% of GHG emissions produced by humans, of which cattle farming for beef and dairy would represent 41 and 21% of emissions produced by the sector, respectively, either from pasture-based or confinement systems (Gerber et al., 2013). Another study shows that from 2006 to 2015, human activities produced 10.3 GtCyr−1 (or 37.7 GtCO−2yr1; Le Quéré et al., 2016), but only half of this was added to the atmosphere, highlighting the regulatory capacity of biosphere C reserves (Stockmann et al., 2013), such as pasture (Soussana et al., 2007).
The sequestration of C by pasture plays an important role in partially mitigating the GHG emitted through ruminant production systems (Soussana et al., 2010; de Oliveira Silva et al., 2016). Carbon sequestration occurs principally as a result of the fixation of C via photosynthesis, which removes CO2 from the atmosphere, and stores it as soil organic carbon (SOC). SOC represents 58% of the soil organic matter (SOM), which is a mixture of partially decomposed plant, animal, and microbial material (Stockmann et al., 2013). In pasture, more than 90% of the C stock is stored in the soil (Reeder and Schuman, 2002). However, it takes much longer to build a C stock than to lose it (Soussana et al., 2010; Smith, 2014), which underscores the importance of conserving this ecosystem service to help keep CO2-e out of the atmosphere.
The improvement of pasture in tropical soils provides conditions for C sequestration (Conant et al., 2001) and its subsequent storage. Voisin's Rational Grazing (VRG) is an agroecological pasture management system that brings together practices that increase and conserve the SOM through increased biocenosis (Machado, 2010). In VRG, the area, divided into plots, is occupied based on the growth dynamics of the forage and the needs of the animals. The occupation of paddocks must be short enough so that the animals do not consume the plant regrowth before the necessary recovery time, while the recovery time must be long enough to enable plants to accumulate reserves in the roots before the next occupation (Voisin, 1961). VRG management in Brazil is based on four laws (Voisin, 1961) and some technical recommendations (Machado, 2010), such as planting trees, diversifying species in all paddocks, no tilling of the soil, no use of agrochemicals, and avoiding the use of chemical fertilizers. This system has been widely used in the South of Brazil. In Santa Catarina, more than 600 projects have been implemented, mainly by small dairy farmers (Farley et al., 2012). However, at our knowledge there are no studies in the international literature on C stock in these systems in Brazil.
Despite this interesting scenario in the South of Brazil, great attention has been given to the role of enteric emissions from ruminants in global warming, especially since FAO published two worldwide influencers reports (Steinfeld et al., 2006; Gerber et al., 2013). Farm animals, mainly ruminants, are reported to be one of the main contributors of anthropogenic GHG. The message of those reports has not only damaged the reputation of animal production but in special the grassland based production systems in Latin America. This has triggered some reaction, and other research have challenged the mentioned reports (Glatzle et al., 2014; de Oliveira Silva et al., 2016). The global warming reports and other papers have stressed how the use of grain in the feed of ruminants can reduce enteric emission of GHG (Peters et al., 2010; Doreau et al., 2011). This well-known phenomena (Hungate, 1966) has been considered as an alternative ignoring the unique ability of these animals to convert cellulose into milk and meat (Flysjö et al., 2012), and the distinct capacity that pasture and grain crop systems have in stocking SOC.
Soil has the largest terrestrial C stock and soil C sequestration has a tremendous potential to mitigate GHG emission (Lal, 2004). Grassland soils may stock a large portion of C, and pasture management impact directly on C storage of its soils (Conant et al., 2003; Chen et al., 2015). Likewise, a number of practices are recommended for crops management in order to increase C soil storage, as for example no tillage and crop rotation (Lal, 2004).
Stocking C while producing food in agriculture is a relevant task to production systems on mitigating climate change. As more C is stocked per unit area, and more food is produced per C stocked, the better the balance is. In this work we compare the C stocks of two different systems of feeding ruminants: grain base from the most widely used C conservationist grain production system—no-tillage (NT) and pasture based from VRG, an intensive pasture management known to incorporate many C conservationist practices. So, since SOC is a manageable C sink (Stockmann et al., 2013), and its storage in soil depends on the management system used, our objective in this study was to characterize the C stock in VRG pasture and compare the C stock in the soil of the VRG system with the C stock in the soil from NT fields, in two different seasons over a period of 1 year.
Materials and Methods
Study Area
The study was conducted in five properties, each considered as a block, and chosen as follows. From a frame of 104 properties/farmers in the region practicing VRG's grazing system and cropping with no tillage system, 12 properties were randomly chosen. Then the following criteria were applied to these twelve: the land use/management of Rational Grazing and no-till was consolidated with 5 years or more of the same practices (main criterion), and with the same previous use of the soil (tillage crop); same micro region (minimum differences of landscapes and soil); same climate; similar ethnic background. From the 12, five farms accomplished with these criteria and were studied.
All farms included in the study were located in Western Santa Catarina State, Brazil, with similar edaphoclimatic characteristics. The climate is classified as humid subtropical (Cfa in Köppen classification) with annual rainfall between 1,900 and 2,200 mm, and mean air temperature of 18–20°C (Alvares et al., 2013). The farmers used similar agricultural techniques. Therefore, we assume that soil management was the main factor affecting SOC. The coordinates of the five properties are: 26°56′40.39″S and 53°36′9.57″W; 26°16′53.49″S and 53°37′16.90″W; 26°48′2.64″S and 53°20′37.71″W; 26°30′23.33″S and 53°32′6.23″W; 26°23′8.88″S and 53°28′31.81″W.
The land-use and soil characteristics for each farm are shown in Tables 1, 2, respectively. The VRG pasture were perennial and species diverse in all farms, with one to five legumes (Trifolium repens, Trifolium pratense, Medicago sativa, and Lotus corniculatus during the winter, and Arachis pintoi and Desmodium spp., consistent throughout the cycle), along with five to nine grasses (Avena sativa, Lolium multiflorum, Pennisetum purpureum, Axonopus catharinensis, Hemartria altissima, Cynodon nlemfluensis, Cynodon spp. (Tifton), Sorghum sudanense, Brachiaria plantaginea, Digitaria decumbens, Axonopus compressus, and Pennisetum clandestinum). During the summer, the pasture was dominated by C4 grasses, and in the winter by C3 species. The NT field from all farms had corn monoculture in summer, and a mixture of oat-ryegrass in the winter. This pasture mixture in NT fields was always used in winter for direct grazing in rotation for 3 months.
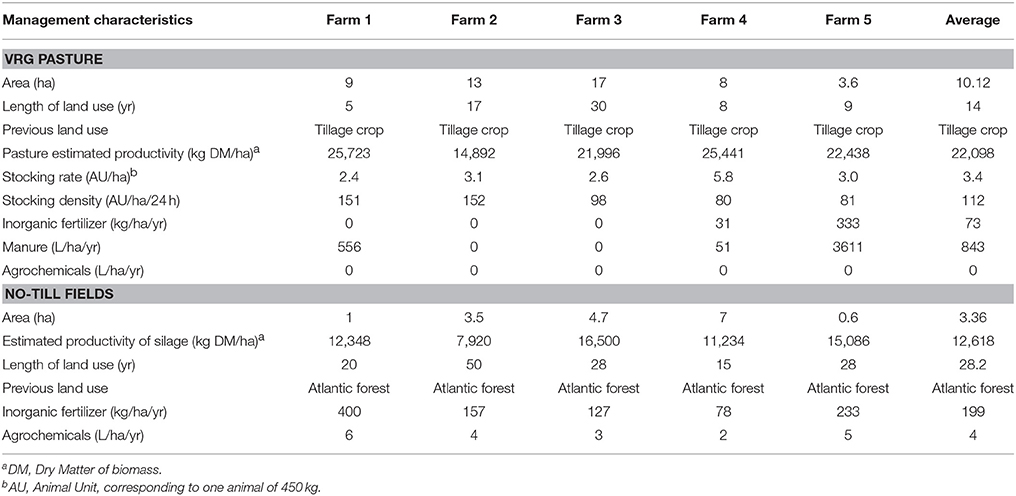
Table 1. Land-use characteristics on each of the five properties studied for carbon stock in Voisin's Rational Grazing (VRG) and No-tillage crop system (NT).
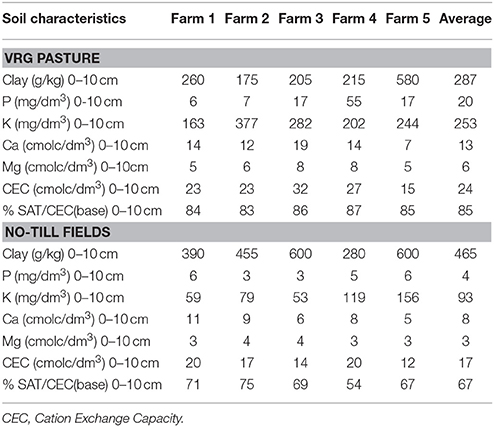
Table 2. Soil characteristics on each of the five properties studied for carbon stock in 2014 for Voisin's Rational Grazing (VRG) and No-tillage crop system (NT).
Sampling of above and Belowground Biomass of Pasture Plants
Sample collection of soil and plant biomass (pasture only) was undertaken in February (summer) and July (winter) in 2014. In each property, at the time of the first visit, out of 60 paddocks three paddocks reaching the optimal recovery period of pasture (Machado Filho, 2011) were chosen and considered as subplots. From these, we sampled the above and belowground biomass of pasture plants, always when the optimal recovery period was reached, according to the following procedure: a transect was randomly established in each paddock and a 0.25 m2 square was placed randomly five times along the transect (Gardner, 1986), creating five subsamples per paddock. The aerial part of the plants was completely removed. The 15 subsamples from the paddocks were amalgamated into one sample per property per season, which were then weighed and dried at 65°C until reaching a constant weight.
The samples of roots were collected from three soil layers (0–10, 10–20, and 20–40 cm) using a steel auger. The roots were separated from the soil with the use of three sieves of 1.4 mm, 1 mm, and 500 μm mesh sizes as recommended by Böhm (1979) and Bolinder et al. (2002). The subsamples were amalgamated into a single sample per depth per property. The roots were dried at 65°C until reaching a constant weight. After drying, the aerial plant samples and roots were weighed to determine the dry material (DM) and ground (<2 mm) for analysis of total organic C using the adapted (Walkley and Black, 1934; Tedesco et al., 1995) wet combustion method.
Soils Samples from Pasture and Fields
Soil samples were collected to compare the SOC between the VRG pasture and the NT fields. From the pasture, five subsamples (0–10, 10–20, 20–40 cm) were collected, as described above, in the same three paddocks as the biomass, totalizing 15 subsamples. The subsamples were combined to provide a single sample for each soil depth per property, per season. We dried the soil samples at 40°C until reaching a constant weight. The samples were then ground (<2 mm) for SOC analysis using the adapted (Walkley and Black, 1934; Tedesco et al., 1995) wet combustion method.
The NT fields averaged 3.4 ha (0.6–7 ha). At each sampling event, the sampler walked in zigzag throughout the field. The zigzag path was divided in 15 randomly assigned collection points, at which a 0.25 m2 square was thrown back. The soil sample was then collected at each of the points where the square fell on the floor at a depth of 0–10, 10–20, and 20–40 cm. The 15 subsamples were then mixed to make one sample per farm NT field. This procedure was performed in each season.
Three undisturbed samples were collected with the use of a volumetric steel ring (78 cm3) to determine the bulk density of the soil for each type of land-use and each evaluated depth. The bulk density and the C stock at fixed depths was calculated following Ellert et al. (2006). The total C stock of the soil and roots was the sum of the values obtained for each sample depth (0–10, 10–20, and 20–40 cm; Assad et al., 2013).
Data Analysis
The sum of the stocks obtained from the aboveground biomass, and each belowground biomass and soil layer, provided the total C stock of the pasture. Land-use was compared only through the C stock in the soil. Statistical analyses were made using R (R Core Team, 2013). In our observational study, a mixed effect linear model was fitted using lme4 package of R (Bates et al., 2015) to perform a linear mixed effects analysis of the relationship between land use and C stock in soil. P-values were estimated through Type II Wald Chi-squared test. Land use and season were included as fixed effects. Farm was included as random effect to reflect the residual variation in soil C between each of these units. The land use plots were nested into each farm to reflect the residual variation between collection points that were nested within each farm. Goodness of fit of the model (homoscedasticity, normality distribution of residuals, linearity of residuals vs. fitted, and normality of random effects) was checked through standard plot analysis.
Results
Carbon Stock in the Soil of VRG Pasture and NT Fields
Season and land use system were not inter-dependent on each other [ = 2.3, p = 0.1]. Regarding land use, when comparing the whole layer (0–40 cm), the C stock was higher in the soil of the VRG pasture (average = 115.0 Mg C ha−1) than in the NT fields [average = 92.5 Mg C ha−1; = 15.3, p < 0.00009; Figure 1]. In VRG, SOC stock was 22.5 Mg C ha−1 ± 6 (SE) higher than NT fields, on average (Table 3). When analyzing soil by layers, C stock was also higher on VRG's soil at the deepest [20–40 cm; = 6.7, p = 0.009] and the shallowest layer [0–10 cm; = 15.5, p < 0.00008], but not on the layer 10–20 cm [ = 2.3, p = 0.12]. Among seasons, SOC did not differ on the 0–40 cm depth [ = 0.6, p = 0.4; Table 4]. However, season showed effect on C stock on the range of 10–20 cm [ = 11.3, p < 0.0007]. The soil density in the pasture (1.3 g cm−3) was similar to the fields [1.29 g cm−3; = 0.34, p = 0.6].
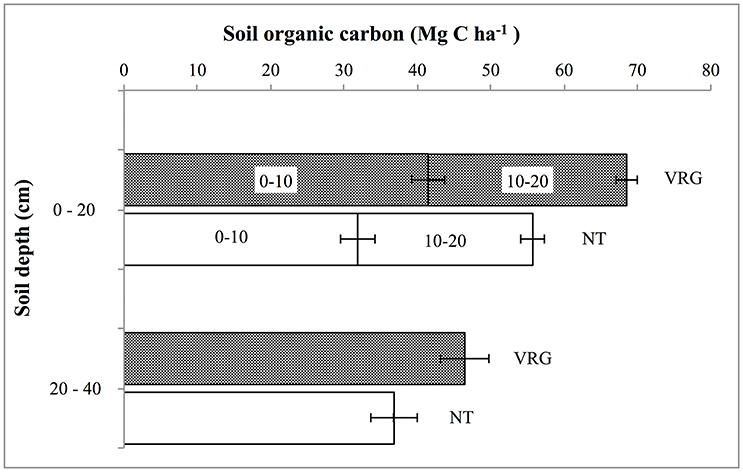
Figure 1. Average ± SE of the distribution of organic carbon in the soil by depth for Voisin's Rational Grazing (VRG) pasture management and for fields with No-tillage crop system (NT) in Western Santa Catarina State, Brazil (n = 5).
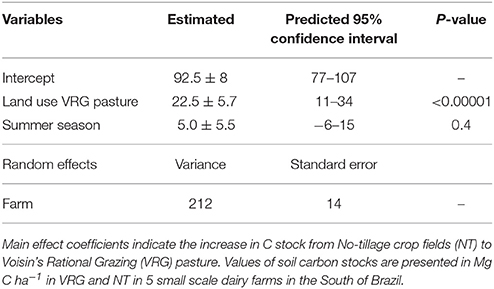
Table 3. List of investigated parameters with coefficients, predicted 95% confidence intervals, standard errors, and p-values from the statistical models described in the paper.
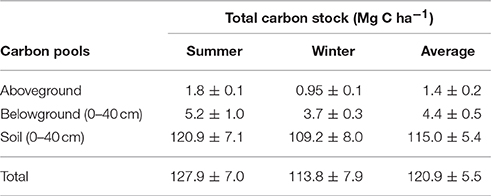
Table 4. Average ± SE of the carbon stock (Mg ha−1) in pasture under Voisin's Rational Grazing for carbon pools and the total carbon stock in summer and winter (n = 5).
Carbon Stock in the VRG Pasture
Total Carbon Stock in the VRG Pasture
The total C stock in the VRG pasture is presented in Table 4, along with the results for each C pool: aboveground biomass, belowground biomass, and SOC. We found no differences between seasons for the total stock in the pasture [ = 1.89, p = 0.2]. Among pools, 95% of the total stock was in SOM, 4% in belowground biomass, and 1% from the aboveground biomass of plants. The aerial section showed greater C storage in summer [ = 63.4, p < 0.00001], while the amount in the roots [ = 2.5615, p = 0.1] and in the soil did not show differences between seasons [ = 0.7849, p = 0.4].
Above and Belowground Biomass
As expected, the average biomass production of the aerial part of plants per cut was greater [ = 48.2, p < 0.000001] in summer (4,455 kg DM ha−1) than winter (2,396 kg DM ha−1). The level of C in the aerial section was on average 40%, with no differences between seasons (p = 0.3). Average root biomass to a depth of 40 cm was 13,375 kg DM ha−1, with no statistical difference (p = 0.2) between summer and winter on belowground biomass. However, there was difference among layers. The surface layer showed 76% of the roots (10,123 kg DM ha−1), greater (p < 0.001) than the 10–20 cm layer (13%; 1,707 kg DM ha−1), and 20–40 cm layer (11%; 1,545 kg DM ha−1). The average concentration of C was 31% and did not differ between samples (p = 0.9). We found a positive correlation between SOC and roots' DM (r = 0.67; p < 0.0001). The data on above and below ground biomass represent the average collected from the three plots from each property (n = 5), with one sampling conducted in summer and the other in winter.
Discussion
This is the first study to compare the C stock in VRG pasture with soils from fields under NT. Both systems were adopted at least 5 years ago (Table 1) in actual productive (not experimental) properties, and therefore represent consolidated management systems. We can see that the stock of SOC in the VRG pastures were ~25% greater in the 0–40 cm layer than that found for the fields with NT.
The difference in C stock between the two systems can be related to some practices adopted in VRG management that increases C in the soil, as observed in previous studies, including: the diversity of species (Steinbeiss et al., 2008), the deposition of cow manure on the pasture (Matsuura et al., 2014), high stocking rates (Dubeux et al., 2006a), herd effect (Savory and Butterfield, 1999), length of recovery period of plants (Abberton et al., 2010), and the use of perennial species (Bell et al., 2012). The richness of species in VRG pastures varied according to property, but it was never a monoculture. In diversified pastures, there can be 2.7 times more biomass above and below ground than a monoculture (Tilman et al., 2001) and a deeper root distribution due to the plasticity of root biomass allocation in diverse communities (Mueller et al., 2013), factors which contribute to a positive relationship between species richness and SOC. Furthermore, the interaction between legumes and grasses represents an important mechanism that combines the stabilization of C in the soil with the recycling of nutrients for the plants (Redin et al., 2014).
Although the VRG showed better results for C stock, the fields under NT are known to provide less oxidative environments for SOC than traditional tilled systems (Souza et al., 2014). This might be a reason why we could not detect the influence of land use on the second layer. The layer from 10 to 20 cm showed difference between seasons. In both land uses, the SOC stock in this layer decreased from summer to winter (VRG pasture: 30 Mg C.ha−1 in summer and 23 Mg C.ha−1 in winter; NT fields: 25 Mg C.ha−1 in summer and 22 Mg C.ha−1 in winter). We suggest this might occur due to higher temperatures in summer, raising microbial processes and plant growth that gradually consume C stocks into winter. Differences in the surface layer might be offset due the higher C return of aboveground biomass, since both systems had predominantly C4 species in summer. Interestingly, despite a direct relationship between belowground biomass production and SOC, there was no significant difference between seasons for root biomass, but there was for aboveground biomass production. Besides, C return from aboveground biomass may take a while from winter to summer to be incorporated from the surface to the second layer, and doing so, showing higher C stock on the summer.
The NT system involves a higher use of inorganic fertilizers than in VRG pasture which can increase the production of biomass (Cheng-Fang et al., 2012) and contribute to an increase in C stock in the system. On the other hand, in VRG, the presence of manure, along with the lack of agrochemicals which can inhibit the micro- and macro-organisms in the soil and the manure (Hussain et al., 2009; Yasmin and D'Souza, 2010), suggest that the flow of SOM is more dynamic in VRG than NT systems, which receive an annual application of herbicides and insecticides.
The belowground biomass found in the VRG system is nine times greater than that found for corn (Redin, 2014), the crop cultivated in the fields during the summer on the studied properties, and four times greater than the biomass of oat plus ryegrass roots from Redin (2014), the crop cultivated in the winter. According to Rasse et al. (2005), the rhizodeposition of C is 2.4 times that derived from the aerial part of plants, and thus makes up the greatest share of SOC (Toma et al., 2013). Nevertheless, in the VRG pasture, the belowground biomass was four times greater than the aboveground biomass.
The largest pool in VRG system is in the soil. That underscores the importance of never revolving it to a means of preserving the large stocks of C in the soil. The differences in C stock between seasons in the aerial section were due to predominance of C4 species in summer and C3 species in winter, respectively. As with the soil, the C derived from the roots has a high level of physical stability because it is incorporated directly into the soil (Soussana et al., 2010). We observed a strong correlation between soil C and root biomass, which emphasizes again the relationship between these factors.
However, the C in the aerial section in VRG can play an important role through more rapid C return cycles than C in the roots (Soussana et al., 2010). Bovine waste can return between 2.1 and 3.3 Mg C ha−1 per year of the aerial part of plants, an estimate based on the percentages provided by Soussana et al. (2010), the average production of the aerial section estimated for the year, the level of C, and the 8 grazing episodes by cows per year, as occurred on the studied properties.
As such, the presence of animals can be one explanation for the differences found between the pasture and the field. The animals are removed from pasture only during the milking period, approximately 3 h a day. The average stocking rate (3.4 AU/ha) and the mean instant stocking density (112 AU/ha/day; Table 1) suggest not only a high rate of return of residues from animal waste, but also frequent fertilization of the pasture associated with a long period of recovery, increasing the productivity of the pasture and favoring root growth.
In fact, Dubeux et al. (2006b) observed a greater return and quality of residue at higher stocking rates, as well as greater C sequestration (Conant et al., 2001; Dubeux et al., 2006a), particularly in rotational systems. In VRG, the division of pasture plays an important role in guaranteeing a period of recovery from herbivory for the plants, preventing overgrazing and the consequent degradation of C stock (Tanentzap and Coomes, 2012). Furthermore, Tilman (1998) observed that the addition of manure enabled a return to original levels of soil fertility, measured as organic C and total N, in 40 years, despite the greater proportion and diversity of recalcitrant compounds in the manure. A return to original levels would take 200 years through natural succession, while a reduced tillage system would recuperate only 20% of the original fertility in 150 years (Tilman, 1998). This demonstrates that conservationist practices are important to elevate SOC, while accumulation practices have the greatest potential to increase SOC.
Greater C stock in pasture soil than crop soil is usually challenged by the fact that one hectare of crop would produce more food than one hectare of pasture (Garnett et al., 2017). However, this statement depend very much on pasture management. Pasture management can significantly affect C stock in soil and biomass production, and therefore animal production. As more efficient the management is, applying correct stocking rate and allowing regrowth, higher C levels are stocked (Chen et al., 2015). Intensive rotational grazing, as VRG is (also called MIG—Management Intensive Grazing), produces more and stocks more C than extensively managed pastures (Conant et al., 2003).
Using data from our field study, we estimated how efficient would be producing milk from those pastures or from those crops. Once it is well established that emission intensity is higher for grazing (2.9 kg CO2 e.kg−1 product) than mixed grain and forage (2.6 kg CO2 e.kg−1 product) based milk production (Gerber et al., 2013), we estimated what would be the production of milk per hectare from VRG or from NT. Further, we calculated the quantity of milk produced per C stored. As more C is stocked per unit area, and more food is produced per C stocked, the better the balance.
The average cow for the five farms studied had around 450 kg, was a crossbred Holstein and Jersey, producing around 15 kg.day−1 of milk. This is in line with the typical cow of the region found in VRG dairy systems (Kuhnen et al., 2015; Balcão et al., 2017). From NRC Dairy Cattle (2001) requirements and feed tables, the potential for milk production in 1 ha of NT corn plus ryegrass vs. 1 ha of VRG pasture was calculated. All detailed calculations are in Supplementary Material. One hectare of pasture in VRG farms could produce a total of 17,085 kg of milk/ha/yr if all forage consumed was destined to lactating cows. Yet one hectare of corn silage intercropping with ryegrass can produce 12,240 kg of milk/ha/yr, if all feed consumed was destined to lactating cows. As C stock in VRG soils was 115.0 Mg C ha−1 and in NT fields 92.5 Mg C ha−1, we may estimate that on pasture, it is produced 0.15 kg of milk.kg−1 of C stored, and on NT corn silage plus ryegrass it is produced 0.13 kg of milk.kg−1 of C stored. In this study, VRG managed pastures stored more C, produced more milk and produced more milk per kg of stored C than corn silage plus ryegrass from NT fields.
Pastures under VRG management and fields under NT can be important C reserves and their conservation should be a priority in agricultural policies, as the loss of SOC occurs much more easily and rapidly than its storage. Food production systems stock different quantities of organic C in the soil, and these differences must be considered when comparing GHG emissions by ruminants with different diets.
The increase in C sequestration through intensive pasture management with deep roots and without deforestation can offset GHG emissions from ruminant production (de Oliveira Silva et al., 2016). This indicates that the high productivity of pasture produced using VRG may be a tool to intensify livestock farming and reduce its GHG emissions, as indicated by the accumulation of SOC. As such, the recuperation of degraded pasture is an important opportunity in achieving Brazil's C emission mitigation plan (de Oliveira Silva et al., 2016).
Conclusion
In this study, soils from VRG pastures stocked more C than soils from fields managed with a direct planting system in both seasons of the year. The frequent fertilization of the pasture due to a high return of waste residues, related to the high cattle stocking density, and the perennial and diverse pasture roots might be influential factors in the accumulation of C.
The different SOC storage capacities between the pasture system and grain crop production, accompanied by the fact that one hectare of pasture on VRG produces more milk than one hectare of corn plus ryegrass, must be taken into consideration in studies on climate change that compare different diets for ruminants.
Author Contributions
HS conceived, designed, and conducted the field research for her master's degree, performed the sample and data analyses, literature research, drafted the manuscript, had the final responsibility for the editing and the submission. LM was supervisor of HS, conceived and designed the field research, performed part of the statistical analyses, contributed to writing, critically reviewed the manuscript and its final edition. DB organized and performed the field research, the laboratory analyses and the literature research.
Funding
This study was funded by CNPq (National Council on Scientific and Technological Development), through the Inter-institutional Network of the Agroecological Dairy Productive Chain, Project n° 562908/2010-2, Edital 22/2010. We acknowledge CAPES for the scholarship provided to HS, and CNPq for the scholarship for LM.
Conflict of Interest Statement
The authors declare that the research was conducted in the absence of any commercial or financial relationships that could be construed as a potential conflict of interest.
Acknowledgments
We would like to thank Rodolfo Assis for the help with the soil analyses, José Bran and Ivone de Bem with the statistical analyses, Arcangelo Loss with the field research, Eve Nimmo with the translation, and Denis Angers with the first steps, as well as the staff of the Núcleo de Pastoreio Racional Voisin and the Soil Laboratory at the Federal University of Santa Catarina. We thank especially the farmers, for their patience and collaboration during the experiment.
Supplementary Material
The Supplementary Material for this article can be found online at: https://www.frontiersin.org/articles/10.3389/fenvs.2017.00087/full#supplementary-material
References
Abberton, M., Conant, R., and Batello, C. (2010). Grassland Carbon Sequestration : Management, Policy and Economics. Rome.
Alvares, C. A., Stape, J. L., Sentelhas, P. C., De Moraes Gonçalves, J. L., and Sparovek, G. (2013). Köppen's climate classification map for Brazil. Meteorol. Zeitschrift 22, 711–728. doi: 10.1127/0941-2948/2013/0507
Assad, E. D., Pinto, H. S., Martins, S. C., Groppo, J. D., Salgado, P. R., Evangelista, B., et al. (2013). Changes in soil carbon stocks in Brazil due to land use: paired site comparisons and a regional pasture soil survey. Biogeosciences 10, 6141–6160. doi: 10.5194/bg-10-6141-2013
Balcão, L. F., Longo, C., Costa, J. H. C., Uller-Gómez, C., Machado Filho, L. C. P., and Hötzel, M. J. (2017). Characterisation of smallholding dairy farms in southern Brazil. Anim. Prod. Sci. 57, 735–745. doi: 10.1071/AN15133
Bates, D., Maechler, M., Bolker, B., and Walker, S. (2015). Fitting linear mixed-effects models using lme4. J. Stat. Softw. 67, 1–48. doi: 10.18637/jss.v067.i01
Bell, L. W., Sparling, B., Tenuta, M., and Entz, M. H. (2012). Soil profile carbon and nutrient stocks under long-term conventional and organic crop and alfalfa-crop rotations and re-established grassland. Agric. Ecosyst. Environ. 158, 156–163. doi: 10.1016/j.agee.2012.06.006
Böhm, W. (1979). Methods of Studying Root Systems, 1st Edn. Göttingen: Springer-Verlag; Berlin Heidelberg.
Bolinder, M. A., Angers, D. A., Bélanger, G., Michaud, R., and Laverdière, M. R. (2002). Root biomass and shoot to root ratios of perennial forage crops in eastern Canada. Can. J. Plant Sci. 82, 731–737. doi: 10.4141/P01-139
Chen, W., Huang, D., Liu, N., Zhang, Y., Badgery, W. B., Wang, X., et al. (2015). Improved grazing management may increase soil carbon sequestration in temperate steppe. Sci. Rep. 5, 1–13. doi: 10.1038/srep10892
Cheng-Fang, L., Dan-Na, Z., Zhi-Kui, K., Zhi-Sheng, Z., Jin-Ping, W., Ming-Li, C., et al. (2012). Effects of tillage and nitrogen fertilizers on CH4 and CO2 emissions and soil organic carbon in paddy fields of central China. PLoS ONE 7:e34642. doi: 10.1371/journal.pone.0034642
Conant, R. T., Paustian, K., and Elliott, E. T. (2001). Grassland management and conversion into grassland: effects on soil carbon. Ecol. Appl. 11, 343–355. doi: 10.1890/1051-0761(2001)011[0343:GMACIG]2.0.CO;2
Conant, R. T., Six, J., and Paustian, K. (2003). Land use effects on soil carbon fractions in the southeastern United States. I. Management-intensive versus extensive grazing. Biol. Fertil. Soils 38, 386–392. doi: 10.1007/s00374-003-0652-z
de Oliveira Silva, R., Barioni, L. G., Hall, J. A. J., Folegatti Matsuura, M., Zanett Albertini, T., Fernandes, F. A., et al. (2016). Increasing beef production could lower greenhouse gas emissions in Brazil if decoupled from deforestation. Nat. Clim. Chang. 6, 493–497. doi: 10.1038/nclimate2916
Doreau, M., van der Werf, H. M. G., Micol, D., Dubroeucq, H., Agabriel, J., Rochette, Y., et al. (2011). Enteric methane production and greenhouse gases balance of diets differing in concentrate in the fattening phase of a beef production system. J. Anim. Sci. 89, 2518–2528. doi: 10.2527/jas.2010-3140
Dubeux, J. C. B. Jr., Sollenberger, L. E., Comerford, N. B., Scholberg, J. M., Ruggieri, A. C., Vendramini, J. M. B., et al. (2006a). Management intensity affects density fractions of soil organic matter from grazed bahiagrass swards. Soil Biol. Biochem. 38, 2705–2711. doi: 10.1016/j.soilbio.2006.04.021
Dubeux, J. C. B. Jr., Sollenberger, L. E., Vendramini, J. M. B., Stewart, R. L., and Interrante, S. M. (2006b). Litter mass, deposition rate, and chemical composition in bahiagrass pastures managed at different intensities. Crop Sci. 46, 1299. doi: 10.2135/cropsci2005.08-0262
Ellert, B. H., Janzen, H. H., and Bremer, E. (2006). “Measuring change in soil organic carbon storage,” in Soil Sampling and Methods of Analysis, eds M. Carter and E. Gregorich (Boca Raton, FL: CRC Press), 25–38.
Farley, J., Schmitt, F. A., Alvez, J., and De Freitas, N. R. Jr. (2012). How valuing nature can transform agriculture. Solut. Sustain. Desirable Futur. 2, 64–73.
Flysjö, A., Cederberg, C., Henriksson, M., and Ledgard, S. (2012). The interaction between milk and beef production and emissions from land use change – critical considerations in life cycle assessment and carbon footprint studies of milk. J. Clean. Prod. 28, 134–142. doi: 10.1016/j.jclepro.2011.11.046
Gardner, A. L. (1986). Técnicas de Pesquisa em Pastagem e Aplicabilidade de Resultados em Sistema de Produção. Braslia: IICA/EMBRAPA CNPGL.
Garnett, T., Godde, C., Muller, A., Röös, E., Smith, P., de Boer, I. J. M., et al. (2017). Grazed and Confused? Ruminating on Cattle, Grazing Systems, Methane, Nitrous Oxide, the Soil Carbon Sequestration Question – and What It All Means for Greenhouse Gas Emissions. FCRN, University of Oxford.
Gerber, P. J., Steinfeld, H., Henderson, B., Mottet, A., Opio, C., Dijkman, J., et al. (2013). Tackling Climate Change through Livestock – A Global Assessment of Emissions and Mitigation Opportunities. Rome: Food and Agriculture Organization of the United Nations (FAO).
Glatzle, A., Alley, R., Aydin, M., Verhulst, K., Saltzman, E., Battle, M., et al. (2014). Questioning key conclusions of FAO publications ‘Livestock’s Long Shadow' (2006) appearing again in ‘Tackling Climate Change Through Livestock’ (2013). Pastor. Res. Policy Pract. 4:1. doi: 10.1186/2041-7136-4-1
Hussain, S., Siddique, T., Saleem, M., Arshad, M., and Khalid, A. (2009). “Impact of pesticides on soil microbial diversity, enzymes, and biochemical reactions,” in Advances in Agronomy, ed D. L. Sparks (Hardbound: Elsevier Inc.), 159–200.
Kuhnen, S., Stibuski, R. B., Honorato, L. A., and Machado Filho, L. C. P. (2015). Farm management in organic and conventional dairy production systems based on pasture in Southern Brazil and its consequences on production and milk quality. Animals 5, 479–494. doi: 10.3390/ani5030367
Lal, R. (2004). Soil carbon sequestration to mitigate climate change. Geoderma 123, 1–22. doi: 10.1016/j.geoderma.2004.01.032
Le Quéré, C., Andrew, R. M., Canadell, J. G., Sitch, S., Ivar Korsbakken, J., Peters, G. P., et al. (2016). Global carbon budget 2016. Earth Syst. Sci. Data 8, 605–649. doi: 10.5194/essd-8-605-2016
Machado, L. C. P. (2010). Pastoreio Racional Voisin: Tecnologia Agroecológica Para o Terceiro Milênio, 2nd Edn. São Paulo: Expressão Popular.
Machado Filho, L. C. P. (2011). Conceituando o “tempo ótimo de repouso” em Pastoreio Racional Voisin. Cad. Agroecol. 6, 1–2.
Matsuura, S., Miyata, A., Mano, M., Hojito, M., Mori, A., Kano, S., et al. (2014). Seasonal carbon dynamics and the effects of manure application on carbon budget of a managed grassland in a temperate, humid region in Japan. Jpn. Soc. Grassl. Sci. 60, 76–91. doi: 10.1111/grs.12042
Meinshausen, M., Vogel, E., Nauels, A., Lorbacher, K., Meinshausen, N., Etheridge, D. M., et al. (2017). Historical greenhouse gas concentrations for climate modelling (CMIP6), Geosci. Model Dev. 10, 2057–2116. doi: 10.5194/gmd-10-2057-2017
Mueller, K. E., Tilman, D., Fornara, D. A., and Hobbie, S. E. (2013). Root depth distribution and the diversity—productivity relationship in a long-term grassland experiment. Ecology 94, 787–793. doi: 10.1890/12-1399.1
National Research Council (NRC). (2001). Nutrient Requirements of Dairy Cattle. 7th Rev. Ed. Washington, DC: National Academy of Sciences.
Peters, G. M., Rowley, H. V., Wiedemann, S., Tucker, R., Short, M. D., and Schulz, M. (2010). Red meat production in Australia: life cycle assessment and comparison with overseas studies. Environ. Sci. Technol. 44, 1327–1332. doi: 10.1021/es901131e
Rasse, D. P., Rumpel, C., and Dignac, M.-F. (2005). Is soil carbon mostly root carbon? Mechanisms for a specific stabilisation. Plant Soil 269, 341–356. doi: 10.1007/s11104-004-0907-y
R Core Team (2013). R: A Language and Environment for Statistical Computing. Vienna: R Foundation for Statistical Computing. Available online at: http://www.R-project.org/
Redin, M. (2014). Biomass Production, Chemical Composition and Decomposition of Crop Residues from Shoot and Roots in Soil. Ph.D. Thesis, Federal University of Santa Maria, Santa Maria.
Redin, M., Guénon, R., Recous, S., Schmatz, R., de Freitas, L. L., Aita, C., et al. (2014). Carbon mineralization in soil of roots from twenty crop species, as affected by their chemical composition and botanical family. Plant Soil 378, 205–214. doi: 10.1007/s11104-013-2021-5
Reeder, J. D., and Schuman, G. E. (2002). Influence of livestock grazing on C sequestration in semi-arid mixed-grass and short-grass rangelands. Environ. Pollut. 116, 457–463. doi: 10.1016/S0269-7491(01)00223-8
Savory, A., and Butterfield, J. (1999). Holistic Management: A New Framework for Decision Making. Washington, DC: Island Press.
Smith, P. (2014). Do grasslands act as a perpetual sink for carbon? Glob. Chang. Biol. 20, 2708–2711. doi: 10.1111/gcb.12561
Soussana, J. F., Allard, V., Pilegaard, K., Ambus, P., Amman, C., Campbell, C., et al. (2007). Full accounting of the greenhouse gas (CO2, N2O, CH4) budget of nine European grassland sites. Agric. Ecosyst. Environ. 121, 121–134. doi: 10.1016/j.agee.2006.12.022
Soussana, J. F., Tallec, T., and Blanfort, V. (2010). Mitigating the greenhouse gas balance of ruminant production systems through carbon sequestration in grasslands. Animal 4, 334–350. doi: 10.1017/S1751731109990784
Souza, E. D., Costa, S. E. V. G. A., Anghinoni, I., Carneiro, M. A. C., Martins, A. P., and Bayer, C. (2014). Soil quality indicators in a Rhodic Paleudult under long term tillage systems. Soil Tillage Res. 139, 28–36. doi: 10.1016/j.still.2014.02.001
Steinbeiss, S., Beßler, H., Engels, C., Temperton, V. M., Buchmann, N., Roscher, C., et al. (2008). Plant diversity positively affects short-term soil carbon storage in experimental grasslands. Glob. Chang. Biol. 14, 2937–2949. doi: 10.1111/j.1365-2486.2008.01697.x
Steinfeld, H., Gerber, P., Wassenaar, T., Castel, V., Rosales, M., and Haan, C., de. (2006). Livestock's Long Shadow. Environmental Issues and Options. Rome: Food and Agriculture Organization of the United Nations (FAO).
Stockmann, U., Adams, M. A., Crawford, J. W., Field, D. J., Henakaarchchi, N., Jenkins, M., et al. (2013). The knowns, known unknowns and unknowns of sequestration of soil organic carbon. Agric. Ecosyst. Environ. 164, 80–99. doi: 10.1016/j.agee.2012.10.001
Tanentzap, A. J., and Coomes, D. A. (2012). Carbon storage in terrestrial ecosystems: do browsing and grazing herbivores matter? Biol. Rev. Camb. Philos. Soc. 87, 72–94. doi: 10.1111/j.1469-185X.2011.00185.x
Tedesco, M. J., Gianello, C., Bissani, C. A., Bohnen, H., and Volkweiss, S. J. (1995). Análises De Solo, Plantas e Outros Materiais, 2nd Edn. Boletim técnico, 5. Porto Alegre: Federal University of Rio Grande do Sul.
Tilman, D., Reich, P. B., Knops, J., Wedin, D., Mielke, T., and Lehman, C. (2001). Diversity and productivity in a long-term grassland experiment. Science 294, 843–845. doi: 10.1126/science.1060391
Toma, Y., Clifton-Brown, J., Sugiyama, S., Nakaboh, M., Hatano, R., Fernández, F. G., et al. (2013). Soil carbon stocks and carbon sequestration rates in seminatural grassland in Aso region, Kumamoto, Southern Japan. Glob. Chang. Biol. 19, 1676–1687. doi: 10.1111/gcb.12189
Walkley, A., and Black, I. A. (1934). An examination of the degtyareff method for determining soil organic matter and a proposed modification of the cromic acid titration method. Soil Sci. 37, 29–38. doi: 10.1097/00010694-193401000-00003
Keywords: soil organic carbon, feed production system, pasture, roots, no-till, Voisin's grazing management
Citation: Seó HLS, Machado Filho LCP and Brugnara D (2017) Rationally Managed Pastures Stock More Carbon than No-Tillage Fields. Front. Environ. Sci. 5:87. doi: 10.3389/fenvs.2017.00087
Received: 27 June 2017; Accepted: 27 November 2017;
Published: 21 December 2017.
Edited by:
Peter Thorburn, Commonwealth Scientific and Industrial Research Organisation (CSIRO), AustraliaReviewed by:
Yong Liu, Hunan Plant Protection Institute, ChinaA. M. Keith, Centre for Ecology & Hydrology, United Kingdom
Inacio de Barros, Brazilian Agricultural Research Corporation, Brazil
Copyright © 2017 Seó, Machado Filho and Brugnara. This is an open-access article distributed under the terms of the Creative Commons Attribution License (CC BY). The use, distribution or reproduction in other forums is permitted, provided the original author(s) or licensor are credited and that the original publication in this journal is cited, in accordance with accepted academic practice. No use, distribution or reproduction is permitted which does not comply with these terms.
*Correspondence: Hizumi L. S. Seó, hizumi@hotmail.com
Luiz C. P. Machado Filho, pinheiro.machado@ufsc.br