- 1Departamento de Patologia, Faculdade de Medicina, Universidade de São Paulo, São Paulo, Brazil
- 2Departamento de Estatistica, Instituto de Matemática e Estatística, Universidade de São Paulo, São Paulo, Brazil
- 3Departamento de Geografia, Faculdade de Filosofia, Letras e Ciências Humanas, Universidade de São Paulo, São Paulo, Brazil
- 4Instituto de Estudos Avançados, Universidade de São Paulo, São Paulo, Brazil
- 5Instituto de Pesquisas Energéticas e Nucleares, Comissão Nacional de Energia Nuclear, São Paulo, Brazil
- 6Instituto de Biociências, Universidade de São Paulo, São Paulo, Brazil
The analysis of chemical elements in the barks of trees is an alternative procedure to access spatial heterogeneity of traffic related air pollution. However, the role of tree species in the characterization of the variability of airborne pollution is poorly known. We present an observational study conducted in São Paulo, Brazil, based on the analysis of 498 trees from three common species: Tipuana tipu, Poincianella pluviosa, and Ligustrum sp. We considered ANCOVA models to compare the concentrations of Al, Fe, Zn, Cu, Mn, Ba, and S in the bark (periderm) of trees located close to streets with different levels of traffic intensity controlling for the extension of nearby green areas. The expected trend of increasing elemental concentration in the bark of trees located near streets with greater traffic intensity or close to smaller green areas was only fully evidenced by T. tipu. For instance, the concentrations of Zn, Fe, Al, and Ba increase by 200, 350, 230, and 280% respectively, for trees of this species located near arterial streets when compared to those observed near local streets. On the other hand, the concentrations of Zn, Fe, Al, and Ba are reduced by 41, 45, 50, and 30%, respectively, for trees located near green areas. For P. pluviosa, the capacity to suggest an association between the tree bark concentration of chemical elements with increasing levels of air pollution and presence of green areas was only fully observed for Zn and Cu. For Ligustrum sp., weaker and sometimes non-expected associations between bark concentrations of the chemical elements and either street classification or green area extension were observed. Our results indicate that the choice of species is a key element in the use of tree barks as a biomonitoring tool in urban landscapes. Species like T. tipu, with rough and highly porous bark, are the most appropriate for such purpose.
Introduction
Airborne pollution is a current problem in highly populated megacities such as São Paulo, Brazil, one of the largest in the world (World Urbanization Prospects, 2017). It has about 12 million inhabitants (IBGE, 2017) and a fleet of 8 million vehicles (DETRAN-State Department of Traffic of São Paulo, 2017) corresponding to almost 1 car for each 1.5 inhabitants. Currently, its citizens are exposed to an average annual concentration of 21.7 μg/m3 of PM2.5 (Cheng et al., 2016), mostly generated by vehicular traffic (Andrade et al., 2012). The characterization of spatial variability of airborne pollutant concentrations and source apportionment is a topic of major importance for studies designed to quantify their adverse effects on human health (Pope et al., 2002; Mauad et al., 2008; Pereira Filho et al., 2008; Baccarelli, 2009; Zanobetti et al., 2009; de Brito et al., 2010; Santos et al., 2016). The environmental heterogeneity observed within cities, including non-uniform distribution of traffic intensity and extension of green areas (Janhäll, 2015), demands high-resolution measurements of airborne pollution. Accessing the variability of air pollution in such complex urban environments requires a dense network of measuring devices, which are usually scarce in developing countries. In addition, many of the available monitoring sites only provide measurements of particulate matter concentration with no indication of the associated chemical composition.
As an alternative, biomonitoring methods are already used in many countries (Falla et al., 2000) to address variability of air pollution in large geographical areas (e.g., Kuik and Wolterbeek, 1994; Böhm et al., 1998). Tree barks have been recently used to evaluate a fine spatial distribution of chemical elements concentration (Guéguen et al., 2012; Ejidike and Onianwa, 2015), which increases with the levels of traffic intensity (Moreira et al., 2016). Such biomonitoring results may have an accuracy of 100 m (Carneiro et al., 2011). However, the complexity of air pollution in cities depends not only on its sources, but also on the filtering properties of green areas. Although generally neglected in biomonitoring studies, the presence and the size of green spaces are also important to understand and adequately characterize the dispersion and the concentration of air pollution. Several studies suggest that local air pollution is reduced by the presence of trees (Vailshery et al., 2013; Nowak et al., 2014; Janhäll, 2015; Jeanjean et al., 2016). Several factors are involved in the filtering and dispersion of air pollution by the presence of trees (Selmi et al., 2016). The main interaction is the dry deposition which is the mechanism by which vegetation removes air pollutants from the troposphere in non-precipitation periods. It depends on the combination of pollutants (e.g., gaseous/particles), surfaces (e.g., size; roughness; chemical nature), and microclimate (e.g., wind speed and direction; temperature; solar radiation; air turbulence; Selmi et al., 2016).
Despite the recent advances stemming from tree bark studies (Catinon et al., 2009; Perelman et al., 2010; Guéguen et al., 2012; Moreira et al., 2016), identification of tree species with better capacity to record the fine spatial variability of air pollution is still poorly known and constitutes an important research topic. Bark morphology is subject to a high degree of interspecific variability (Yunus et al., 1990; Junikka, 1994) with potential to influence the species ability to record airborne element concentrations (Szczepaniak and Biziuk, 2003). This issue is of special concern because some species might better suit the biomonitoring role than others.
The objective of this observational study is to compare different trees species via the concentrations of chemical elements in their barks with respect to their potential to discriminate traffic generated air pollution, controlling for the presence of green areas. In particular, we focus our attention on Al, Fe, and Cu, that are related to re-suspension of road dust, on Ba, Cu, and Zn, that are related to brake and tire wear and on Mn and S, that are related to fossil fuel emissions as indicated in Liu et al. (2007), de Almeida Albuquerque et al. (2012), and Hetem and Andrade (2016). These specific emission sources were also identified in São Paulo in the study conducted by Moreira et al. (2016). We considered three tree species, Poincianella pluviosa (DC.) L.P. Queiroz (former Caesalpinia pluviosa), Tipuana tipu (Benth.) Kuntze, and Ligustrum sp. L., to quantify their abilities to record the fine spatial variability of air pollution. These species were chosen because they are widely distributed in São Paulo and have different external bark morphologies.
Methods
Study Site and Species
Samples of tree barks of the three selected species were collected in a large region of São Paulo (Figure 1), which includes areas under the influence of no green spaces to large urban parks. São Paulo has a tropical to subtropical climate with a well-defined dry season from June to August. Mean temperature is 20.7°C, and annual precipitation is 1,545 mm. Overall, the main wind direction is southeast with an average speed of 2.6 m/s (refer to the Supplementary Material for a climate diagram and wind rose, Figure S1). This heterogeneous region includes residential and commercial areas, but no industrial activities. The automotive fleet in São Paulo is composed by cars (78.9%), motorcycles (16.3%), trucks (1.6%) and buses (2.7%) (Companhia de Engenharia de Tráfego, 2017a).
The distribution of the sampled trees is a consequence of the distinct density and heterogeneity of their location in each district of São Paulo; this explains the low number of trees selected in the eastern district. Both street trees and trees growing in parks were sampled in the vicinity of streets with different traffic intensities classified according to the city traffic authority (Companhia de Engenharia de Tráfego, 2017b). This classification is based on street characteristics and on the importance of the traffic flow and consists of the following four categories in decreasing order of traffic intensity: Express, Arterial, Collector or Local. Given the existence of few trees located in the vicinity of Express streets, we combined the Express and Arterial categories. We also reclassified the trees originally placed near local streets into two groups: Local I, if their distances to streets with higher traffic intensity were < 100 m and Local II, otherwise. We also classified the sampled trees according to the spatial proportion of green areas as: Inexistent/Very small (0–20% of green area cover), Small (20–40%), Medium (40–60%), and Dominant/Large (>60%) (Tarifa and Azevedo, 2001).
The sampled trees belong to the three most common tree species in São Paulo Tipuana tipu is an exotic species from Bolivia that was extensively planted in the middle of the twentieth century in São Paulo. It is also present in other Brazilian cities, as well as in other countries from South America (Breuste, 2013; Mazza et al., 2013; Teixeira, 2014), North America (Legumes, 2017), Africa (Kuruneri-Chitepo and Shackleton, 2011), Europe (Sashua-Bar et al., 2010; Soares et al., 2011), and Oceania (check the Supplementary Material for the map of species distribution based on herbaria records, GBIF, 2018, Figures S2–S4). These trees are deciduous and they reach large sizes in São Paulo, with diameter at breast height (DBH) close to one meter and height of 20 m. Ligustrum sp. is also an exotic tree species from Asia, that is largely available in cities from different continents. These trees are usually smaller, with DBH up to 50 cm and height up to 15 m in São Paulo. Ligustrun sp. trees are evergreen in São Paulo. The only native species is Poincinella pluviosa, reaching DBH values up to 60 cm and height of up to 20 m.
Morphological Characterization of the Species Bark
In order to support the discussion of the role of tree species in the results of biomonitoring studies using tree barks, we qualitatively characterized the external morphology of the studied species. Since the external bark morphology is a rather conservative characteristic (Junikka, 1994), we only evaluated major differences in bark macroscopic rugosity and microscopic cellular characteristics, not accounting for the effects of bark plasticity on the results. For this purpose, we selected similarly sized individuals of each species, with diameter at breast height (DBH) of about 30 cm, from which we collected 5 × 5 cm samples of the bark. These samples were analyzed by two different methods to qualitatively describe their macroscopic and microscopic bark characteristics. Rugosity was evaluated as the variation in surface depth. We used a stereomicroscope (Leica M205 FA) to create optical slices of the bark external surface and compute their depth profile (LAS software V 4.8) in three transects. These profiles represent the amplitude of variability of the surface depth. Then, we obtained a 5 × 5 mm sample to analyse the cellular characteristics of the bark surface using a scanning electron microscope (SEM, Zeiss Sigma VP). Before running the analysis, all samples were coated with gold to enhance contrast in the SEM images.
Bark Sampling and Preparation
The sample consisted of 498 trees (including 158 from Moreira et al., 2016) of which 193 trees were of the species T. tipu (38.9%), 228 trees were of the species P. pluviosa (45.6%) and 77 trees were of the species Ligustrum sp. (15.5%). Sampling occurred in the years of 2012, 2013, and 2014. The spatial distribution of the sampled trees according to species as well as to the different São Paulo districts is displayed in Figure 2. Sampling was conducted in the dry seasons (from June to August, when monthly precipitation is lower than 50 mm) in order to avoid rain washing and sample degradation by fungi. All tree barks were obtained with titanium tools to avoid contamination. From each selected tree, a portion of 8 × 8 cm of the periderm was collected from each quadrant of the trunk according to the cardinal points at a height of 1.5–2.0 m from the topsoil and these portions were pooled for analysis. The harvesting of bark in four sectors is a conservative approach to have a representative sample independently of the possible existence of a predominant wind direction or of other external factors that may create variations of environmental exposure along the trunk surface. Samples were placed in paper bags and stored in a cabinet under low humidity conditions until the analysis could be conducted. Information regarding tree geographical coordinates was also recorded.
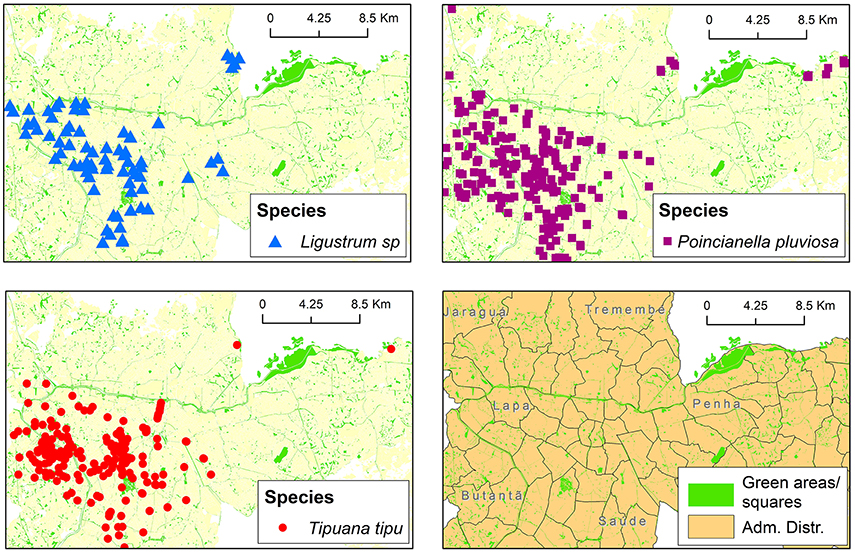
Figure 2. Location of the sampled trees by species in São Paulo and the presence of green areas in the study site.
The collected tree barks were first cleaned using a soft nylon dental brush to eliminate external materials such as dead insects, dust and lichens. The samples were obtained by grating the outermost 3 mm of the bark with a titanium tool (Wolterbeek and Bode, 1995). Each sample was then ground to powder using a vibratory micro-mill with agate mortar (Fritsch Pulverisette 0, Fritsch GmbH, Idar-Oberstein, DE). The powder was compressed by applying a pressure of 3.9 ton/cm2 to make a double layer pellet composed of 0.5 g of tree bark powder and 1 g of boric acid (H3BO3, p.a. ACS reagent).
Elemental Analysis
The bark samples were submitted to an energy dispersive X-ray fluorescence spectrometer-EDXRF (EDX 700-HS, Shimadzu Corp., Kyoto, Japan). The pellets were irradiated with X-rays (source). The emitted fluorescent X-rays (secondary) have a unique wavelength and energy that characterizes each element contained in the sample. As the fluorescent X-ray intensity is a function of the concentration, quantitative analysis is performed by measuring the amount of X-rays of each element (Van Grieken and Markowicz, 2002). This technique was chosen due to its multi-element capability, sensitivity, easy sample preparation without sample digestion, accuracy of the results and analysis cost. The concentrations of Al, Fe, Zn, Cu, Mn, Ba, and S were recorded for having well-described sources in São Paulo (Moreira et al., 2016). These elements may be considered as proxies of the complex mixture generated by vehicular emissions. Although other elements were also measured via the EDXRF, their values were close to the limits of detection. The measurement parameters were: time (240 s), target (Rh, 5–50 kV _ 1–1,000 A) and Si (Li) detector in a vacuum atmosphere. The calibration curves were fitted via linear regression methods using specific parameters of the equipment to correct the matrix effects. A certified reference material [Peach Leaves #1547, National Institute of Standards and Technology (NIST), Gaithersburg, MD, USA] was also analyzed using the same procedure for quality control purposes (Table S1).
Statistical Analysis
The distribution of elemental concentration was described via boxplots constructed by species, street classification and green area extension, as well as via tables with descriptive statistics. The association between element concentration in the tree barks and air pollution as measured via street classification, controlling for species, green area extension, diameter at breast height and sampling campaign was assessed by analysis of covariance (ANCOVA) models (Neter et al., 2005). Therefore, differences in the size of the trees and sampling campaign are accounted for with this model. An interaction term between species and street classification was included in the model to verify if the three species record different patterns of air pollution in their barks. Because residual analyses suggested coarse deviations from the usual Gaussian model assumptions, the data were reanalysed considering the logarithm of element concentration as response.
Non-significant (for α = 0.05) variables and interactions between variables were dropped from the initial model and predicted concentrations of the chemical elements for different street classifications by species and extension of green areas were computed via the final model equation. Bootstrap estimators of prediction standard errors (Efron, 1982) were considered. The model predicted values were used to compare the mean concentrations of the elements across streets with different traffic intensities for each species, controlling by the other factors. Detailed results are given for Zn in the main text because of space limitations, but all other results are presented in the Supplementary Material (Tables S3.1–S3.7).
Results
The three studied species have different external bark morphologies (Figure 3). Our qualitative analysis shows that Tipuana tipu has a relatively deeply furrowed bark consisting of a loose tissue where cells have their lumen exposed to the atmosphere. Not only the outermost layer of the cork has its lumen open to the outside environment, but it seems that this is also true for the lumen of few inner layers of cork cells. Neither P. pluviosa nor Ligustrum sp. have a striped bark like the former species, but they have different porosity characteristics. The analyzed portion of the bark of P. pluviosa is slightly more compact when compared to that of T. tipu. The cork cells of P. pluviosa are stacked and only the lumen of the outermost cells is exposed to the atmosphere. Cells of the bark of Ligustrum sp. are even more compactly arranged and their lumen is rarely exposed to the environment.
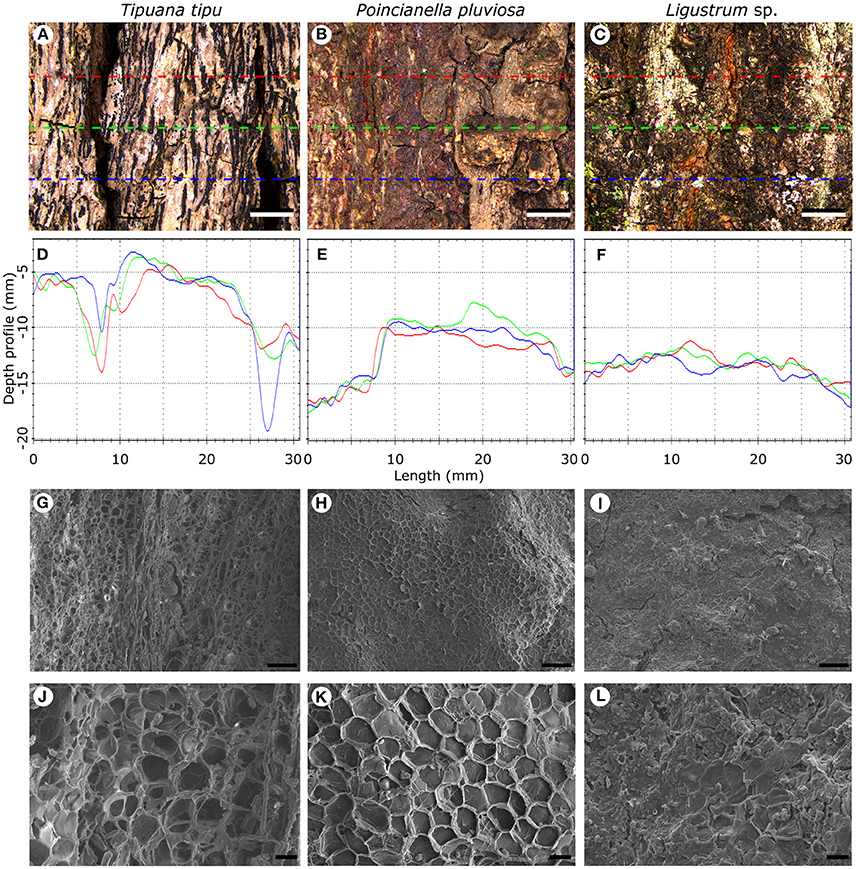
Figure 3. External bark morphology of Tipuana tipu, Poincianella pluviosa and Ligustrum sp. including a macroscopic view of the bark surface (A–C, 5 mm) and the respective depth profiles of surface height variability (D–F; line colors represent the transects highlighted as dashed lines in the macroscopic images); a microscopic view of the surface porosity in lower (G–I, 100 μm) and higher magnification (J–L, 20 μm).
In general, the observed median concentration of Al, Fe, Zn, Cu, Mn, and Ba in the barks of T. tipu increased with their proximity to streets with higher traffic intensity, from Local II to Arterial (Figure 4). Only S did not show such a trend. In the barks of P. pluviosa, a similar pattern was observed for the median concentrations of Zn, Cu, and Ba. Ligustrum sp. was the only species for which the trends in the median tree bark concentrations were not as consistent as the trends observed for the other two species. For T. tipu, the median elemental concentrations of all elements but S tend to increase as the extension of nearby green areas extensions decrease from Dominant/Large to Very Small/Inexistent (Figure 5). For P. pluviosa the same pattern was observed for the median concentrations of all elements but S, Mn, and Ba. Such a trend was not detected for any element in the barks of Ligustrum sp.
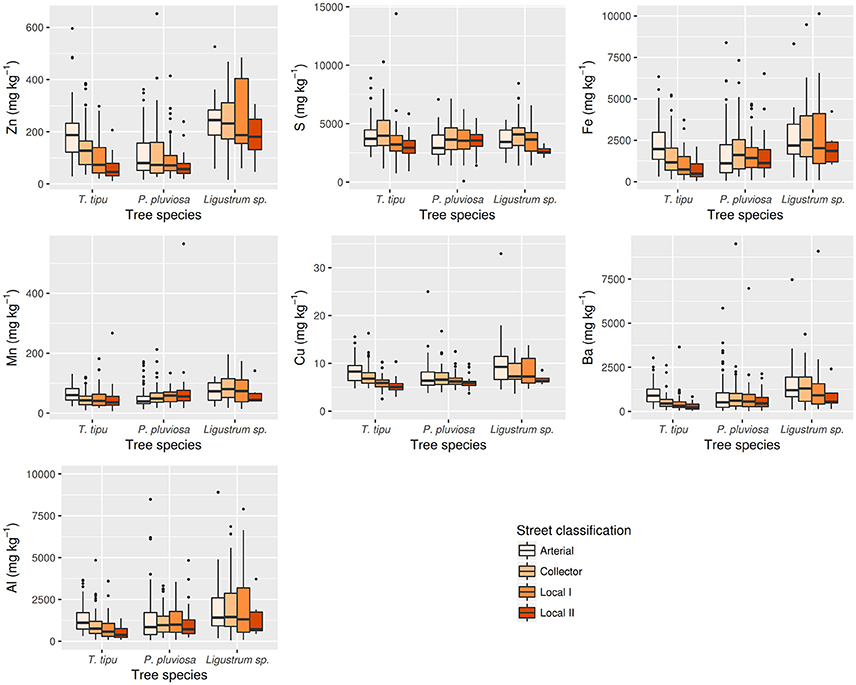
Figure 4. Boxplots of elemental concentration on tree barks of Tipuana tipu, Poincianella pluviosa and Ligustrum sp. by different street categories: Arterial, Collector, Local I, and Local II.
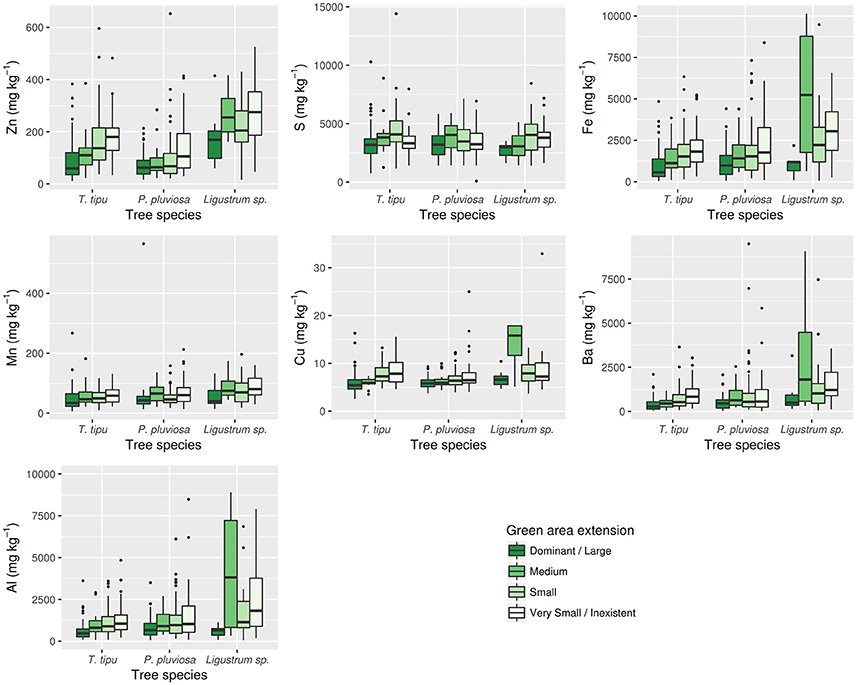
Figure 5. Boxplots of elemental concentration on tree barks of Tipuana tipu, Poincianella pluviosa, and Ligustrum sp. by different green areas extension: Dominant/Large, Medium, Small, Very Small/Inexistent.
Details on the distribution of the observed Zn concentration in T. tipu tree barks taking into account both street classification and the extension of green areas are shown in Table 1. Overall, the average and median values of Zn concentration decrease for trees located near Arterial to Local II categories, within each green area extension category. The highest values of the concentration of Zn are found in trees located near Arterial or Collector streets with Small to Inexistent green areas. On the other hand, the smallest values are found in the bark of trees located near Local II streets with Large to Dominant green areas. But these results do not take into account the possible role of other factors like tree size, differences of sampling campaigns, and driving conditions including speed and intensity of breaks use. These factors, however, were taken into account in the ANCOVA model to precisely define the variation of the elemental concentration attributed to traffic intensity and green area extension. The P-values corresponding to significance tests for the main effects and interaction terms of the ANCOVA models are displayed in Table 2. The interaction between species and street classification is significant for all elements. On the other hand, the effect of the sampling campaigns is significant for all elements but Zn, and DBH is only associated with the concentration of Cu, Mn and Ba. This result, along with an analysis of the boxplots, suggest different patterns of association between the element concentration and traffic intensity for the three species. In particular, T. tipu stands out as the species with the highest sensibility to changes in traffic intensity, followed by P. pluviosa. Ligustrum sp. is less sensible to changes in traffic intensity.
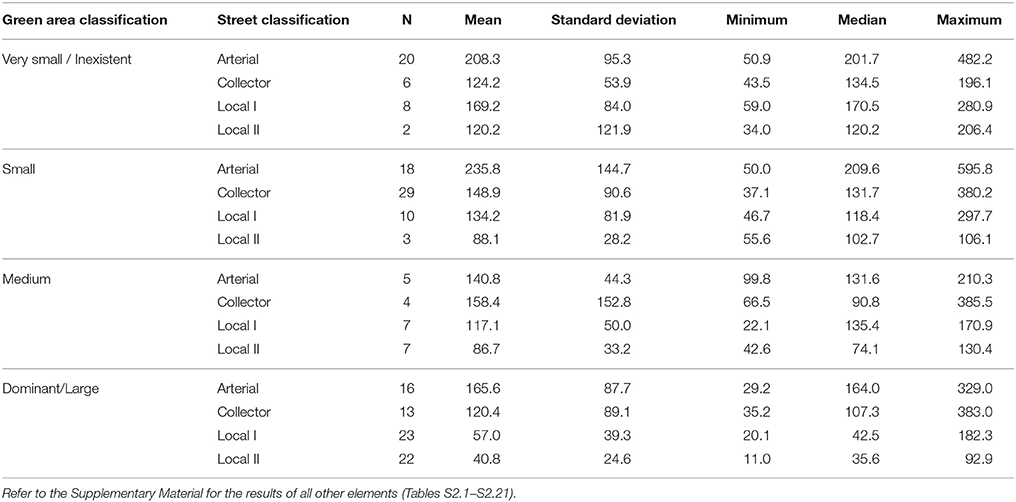
Table 1. Descriptive statistics of Zn concentrations (mg kg−1) in the barks of Tipuana tipu according to green areas size and street classification.
The predicted mean concentrations of Zn from the ANCOVA models are shown in Table 3 (the predicted mean concentrations of Fe, Cu, S, Al, Mn, and Ba are available in the Tables S3.1–S3.6). These predicted means (adjusted for the mean DBH) were computed from the final model containing only significant effects. The results presented in Table 3 clearly show that the expected trend of decreasing mean element concentrations from streets with heavy traffic to local streets is more evident for T. tipu, corroborating the previous conclusions. In the bark of this species, the concentration of Zn increased by 200% from Local II to Arterial streets. On the other hand, it decreased by 41% from inexistent to large green areas. This trend is still present for P. pluviosa, in which the concentrations of Zn increased by 37% from Local II to Arterial streets, and decreased by 41% from inexistent to large green areas. Although the concentrations of Zn increased by 40% from Local II to Arterial streets, and decreases by 41% from inexistent to large green areas for Ligustrum sp., the prediction error is higher for this species.
The concentration of Fe is also strongly dependent on traffic intensity and green area extension as evidenced by T. tipu. In the bark of this species, the concentration of Fe increased by 350% from Local II to Arterial streets. On the other hand, it decreased by 45% from inexistent to large green areas. Aluminum is another element that showed relevant differences evidenced by the bark of T. tipu. The concentration of Al increased by 230% from Local II to Arterial streets, while it decreased by 50% from inexistent to large green areas. Such differences were also found for Ba, that increases by 280% from Local II to Arterial streets, while it decreases by 38% from inexistent to large green areas. Differences for Cu, Mn, and S are less evident, especially when analyzed in the barks of P. pluviosa and Ligustrum sp.
Discussion
Most urban agglomerations with more than 10 million inhabitants are currently found in developing countries (United Nations, 2012). These regions have high levels of urban air pollution (Baklanov et al., 2016) concentrating more than 80% of related deaths (Baklanov et al., 2016; WHO, 2017). In general, their urban landscapes are highly complex and the evaluation of the effect of air pollution on health outcomes must consider their inherent heterogeneity. Biomonitoring methods based on tree barks have been considered as an alternative and/or as a complement to field measurements (Falla et al., 2000) and to satellite image data (Van Donkelaar et al., 2016), which are highly cost restrictive specially for developing countries (Molina et al., 2004). We evaluate the role of tree species in low cost passive biomonitoring techniques designed to provide a higher spatial resolution of exposure to chemical elements composition required by environmental epidemiology (Pope et al., 2009; Bravo et al., 2015).
In this context, a higher element concentration is expected in trees located close to streets with high traffic intensity. This is especially true in São Paulo where most of the air pollution is generated by traffic (Moreira et al., 2016). Indeed, this is exactly the trend observed in the elemental concentration in the tree barks of this study. This trend is more evident for trees of the species T. tipu, as well as for few elements measured in P. pluviosa. Ligustrum sp., on the other hand, stands out as a species that is not reliable for biomonitoring studies based on tree barks. Element concentration in the barks of trees of this species does not discriminate between streets with different traffic intensities. These expected trends, which are usually higher in the barks of trees located in the vicinity of Arterial streets, were not observed for S in any species. Sulfur captured by tree barks in this study is mainly particulate and has a stronger interaction with tree surface (Yli-Pelkonen et al., 2017). The gaseous phase (SO2) is relatively negligible in São Paulo (Miranda et al., 2012). This odd trend is probably a result of the higher dispersion capability of S which is subject to long-range transportation (Masri et al., 2015).
In addition to traffic intensity, the presence of different extensions of green areas is also associated with the concentration of elements in tree barks. Indeed, air pollution is considerably lower in areas under the influence of vegetation, not only because of the filtering properties of leaves (Bolund and Hunhammar, 1999), but also because of the passive deposition on the surface of the plant body, aerodynamic dispersion properties of tree structure (Janhäll, 2015; Jeanjean et al., 2016) and higher wind speed and lower temperature (Kassomenos et al., 2014) in open green areas (Oliveira et al., 2011). Based on these properties, a decreasing element concentration in the tree barks along a gradient of increasing influence of green areas is expected. Once more, this is the trend observed with the barks of T. tipu for all studied elements but Mn and S. A similar result was found in the barks of P. pluviosa but not in the barks of Ligustrum sp. In fact, this species showed a rather unexpected result, with a higher concentration of elements in trees under the influence of medium sized green areas.
Our results point toward the importance of the choice of the appropriate species for biomonitoring studies based on tree barks. The biodiversity available in an urban environment like the one in São Paulo adds a complication to the problem of choosing the most appropriate species for this type of study (Falla et al., 2000). This choice must be based on the combination of spatial coverage and ability to distinguish between different levels of local air pollution. Geographic distribution is probably the main reason used for choosing a species (Böhm et al., 1998; Pacheco et al., 2002; Minganti et al., 2015). However, the effect of the interspecific morphology of barks on the results, although important (Sawidis et al., 2011), is generally poorly considered (e.g., Oliva and Mingorance, 2006) especially in studies whose goal is a fine characterization of urban air pollution.
Some species may not show differences in element concentrations where they actually exist or, even worse, may generate misleading results. The different abilities to detect spatial variability of air pollution seems to depend on the bark external morphology. In our study, the species specific bark morphologies ranged from a highly porous and rough bark on T. tipu to a rather smooth bark with low porosity on Ligustrum sp., while P. pluviosa shows intermediate bark characteristics. This gradient of qualitative features seems to be in accordance with the results of the ANCOVA model. However, a quantitative evaluation of the association between bark morphology and the ability to absorb the chemical elements under investigation was not the objective of the present study, and it is an interesting topic for further research. The lack of statistical significance of the results for Ligustrum sp. could be attributed to the smaller sample size, but there is no evidence that the results are biased by it. Providentially, T. tipu is an abundant tree species not only in cities of Brazil, but also in other cities including those in a few developing countries (Sashua-Bar et al., 2010; Kuruneri-Chitepo and Shackleton, 2011; Soares et al., 2011; Breuste, 2013; Mazza et al., 2013; Teixeira, 2014; Legumes, 2017). If local conditions do not influence the favorable characteristics of the external bark of this species, it may be used in biomonitoring studies in such localities. Similarly, to T. tipu, some Pinus spp. are usually regarded as good biomonitoring species because of their favorable bark characteristics (Oliva and Mingorance, 2006; Sawidis et al., 2011). These interspecific differences are clearly relevant when studying finer gradients of air pollution (Ejidike and Onianwa, 2015) like in our study.
Large-scale estimation or reconstruction of air pollution usually relies on remote sensing or land-use regression techniques. In both cases, the use of local data obtained from automatic stations and/or passive monitoring is required to validate and improve the simulations (Van Donkelaar et al., 2016). Air pollution data obtained from the analysis of element concentration in the bark of trees can be used as additional observational data in this context (Wolterbeek, 2002), either with a complementary role or even replacing traditional methods. The use of tree barks for biomonitoring purposes has few reported limitations. Despite the role of tree species, tree barks do not provide the temporal resolution required for acute exposure risk (Marć et al., 2015). Nonetheless, tree barks may be more suitable for studying long-term exposures (Catinon et al., 2009) usually related to chronic diseases. The specification of standard procedures (Marć et al., 2015) to account for the many sources of data noise, in addition to the choice of species, should be object of future research.
Conclusions
Tree barks are reliable biomonitors and this is the first study showing that they can be used to distinguish both traffic generated pollution and the filtering properties of green areas. Our results suggest that biomonitoring studies based on tree barks are strongly dependent on species. The choice of species with a rough and highly porous bark favors the discrimination of different levels of air pollution accounting for the complex landscape found within cities. Out of the studied species, T. tipu stands out as a reliable one for such studies. Fortunately, this species occurs in different cities around the world where they can be used in biomonitoring programs. The use of tree barks may complement or, whenever needed, replace local measurements used in studies based on satellite images or land-use regression.
Author Contributions
TM, LA-L, GdS, CS, PdA, LB, JMS, PS, MS, and GL conceived the study. TM and LA-L sampled the trees and analyzed the chemical composition of the barks. GL analyzed the external bark morphology; GdS, CS, PdA, LB, and JMS analyzed the data. TM, LA-L, GdS, CS, PdA, LB, JMS, PS, MS, and GL contributed to the interpretation of the results. TM, LA-L, GdS, CS, PdA, LB, JMS, PS, MS, and GL wrote the manuscript.
Funding
Authors thank the São Paulo Research Foundation (FAPESP 13/21728-2) and Coordination for the Improvement of Higher Education Personnel (CAPES), from Brazil for financial support. The author TM is grateful for a fellowship from the Coordination for the Improvement of Higher Education Personnel (CAPES-1078178). LA-L is grateful for a fellowship from the São Paulo Research Foundation (FAPESP 2014/19201-9). GL is grateful for a fellowship from the São Paulo Research Foundation (FAPESP 2015/25511-3). JMS had partial financial support from Conselho Nacional de Desenvolvimento Científico e Tecnológico (CNPq-304126/2015). Authors also thank the valuable contribution of the reviewers.
Conflict of Interest Statement
The authors declare that the research was conducted in the absence of any commercial or financial relationships that could be construed as a potential conflict of interest.
Acknowledgments
The authors thank SVMA-SP for providing the permissions for sampling trees bark in the city.
Supplementary Material
The Supplementary Material for this article can be found online at: https://www.frontiersin.org/articles/10.3389/fenvs.2018.00072/full#supplementary-material
References
Andrade, M. F., Miranda, R. M., Fornaro, A., Kerr, A., Oyama, B., Andrade, P. A., et al. (2012). Vehicle emission and PM2.5 mass concentration in six Brazilian cities. air quality Atmos. Hlth. 5, 79–88. doi: 10.1007/s11869-010-0104-5
Baccarelli, A. (2009). Breathe deeply into your genes genetic variants and air pollution effects. Am. J. Resp. Crit. Care 1796, 431–432. doi: 10.1164/rccm.200812-1808ED
Baklanov, A., Molina, L. T., and Gauss, M. (2016). Megacities, air quality and climate. Atmos. Environ. 126, 235–249. doi: 10.1016/j.atmosenv.2015.11.059
Böhm, P., Wolterbeek, H., Verburg, T., and Musilek, L. (1998). The use of tree bark for environmental pollution monitoring in the Czech Republic. Environ. Pollut. 1022, 243–250. doi: 10.1016/S0269-7491(98)00082-7
Bolund, P., and Hunhammar, S. (1999). Ecosystem services in urban areas. Ecol. Econ. 292, 293–301. doi: 10.1016/S0921-8009(99)00013-0
Bravo, M. A., Son, J., de Freitas, C. U., Gouveia, N., and Bell, M. L. (2015). Air pollution and mortality in São Paulo, Brazil: effects of multiple pollutants and analysis of susceptible populations. J. Expo. Sci. Env. Epid. 262, 150–161. doi: 10.1038/jes.2014.90
Breuste, J. H. (2013) Investigations of the urban street tree forest of Mendonza, Argentina. Urban Ecosyst. 16, 801–818. doi: 10.1007/s11252-012-0255-2.
Carneiro, M. F. H., Ribeiro, F. Q., Fernandes-Filho, F. N., Lobo, D. J. A., Barbosa, F., Rhoden, C. R., et al. (2011). Pollen abortion rates, nitrogen dioxide by passive diffusive tubes and bioaccumulation in tree barks are effective in the characterization of air pollution. Environ. Exp. Bot. 722, 272–277. doi: 10.1016/j.envexpbot.2011.04.001
Catinon, M., Ayrault, S., Clocchiatti, R., Boudouma, O., Asta, J., Tissut, M., et al. (2009). The anthropogenic atmospheric elements fraction: a new interpretation of elemental deposits on tree barks. Atmos. Environ. 435, 1124–1130. doi: 10.1016/j.atmosenv.2008.11.004
Cheng, Z., Luo, L., Wang, S., Wang, Y., Sharma, S., Shimadera, H., et al. (2016). Status and characteristics of ambient PM 2.5 pollution in global megacities. Environ. Int. 89, 212–221. doi: 10.1016/j.envint.2016.02.003
Companhia de Engenharia de Tráfego (2017a). Pesquisa de Monitoração Damobilidade. Mobilidade no Sistema Viário Principal. São Paulo. Available online at: http://www.cetsp.com.br/media/499255/2015.pdf (Accessed November and December, 2017).
Companhia de Engenharia de Tráfego (2017b). Companhia de Engenharia de Tráfego. Available online at: http://www.cetsp.com.br/sobre-a-cet/relatorios-corporativos.aspx (Accessed December 13, 2017).
de Almeida Albuquerque, T. T., de Fátima Andrade, M., and Ynoue, R. Y. (2012). Characterization of atmospheric aerosols in the city of São Paulo, Brazil: comparisons between polluted and unpolluted periods. Environ. Monit. Assess. 184, 969. doi: 10.1007/s10661-011-2013-y
de Brito, J. M., Belotti, L., Toledo, A. C., Antonangelo, L., Silva, F. S., Alvim, D. S., et al. (2010). Acute cardiovascular and inflammatory toxicity induced by inhalation of diesel and biodiesel exhaust particles. Toxicol. Sci. 1161, 67–78. doi: 10.1093/toxsci/kfq107
DETRAN-State Department of Traffic of São Paulo (2017). Frota De Veículos Em SP-Por Tipo De Veículo. Available online at: https://www.detran.sp.gov.br/wps/wcm/connect/portaldetran/detran/detran/estatisticastransito/sa-frotaveiculos/d28760f7-8f21-429f-b039-0547c8c46ed1 (Accessed January 13, 2017).
Efron, B. (1982). The Jackknife, the Bootstrap and Other Resampling, 38th Edn. Philadelphia, PA: Society For Industrial And Applied Mathematics.
Ejidike, I. P., and Onianwa, P. C. (2015). Assessment of trace metals concentration in tree barks as indicator of atmospheric pollution within Ibadan City, South-West, Nigeria. J. Anal. Methods Chem. 2015:243601. doi: 10.1155/2015/243601
Falla, J., Laval-Gilly, P., Henryon, M., Morlot, D., and Ferard, J. F. (2000). Biological air quality monitoring: a review. Environ. Monit. Assess. 643, 627–644. doi: 10.1023/A:1006385924945
GBIF (2018). GBIF.org. GBIF Occurrence Download. Available online at: https://doi.org/10.15468/dl.7hk9m6
Guéguen, F., Stille, P., Geagea, M. L., and Boutin, R. (2012). Atmospheric pollution in an urban environment by tree bark biomonitoring–(Pt) I: trace element analysis. Chemosphere 8610, 1013–1019. doi: 10.1016/j.chemosphere.2011.11.040
Hetem, I. G., and Andrade, M. F. (2016). Characterization of fine particulate matter emitted from the resuspension of road and pavement dust in the metropolitan area of São Paulo, Brazil. Atmos. Basel. 7:31. doi: 10.3390/atmos7030031
IBGE (2017). IBGE Cidades São Paulo, São Paulo. Available online at: https://cidades.ibge.gov.br/brasil/sp/sao-paulo/panorama (Accessed March 4, 2017).
Janhäll, S. (2015). Review on urban vegetation and particle air pollution–deposition and dispersion. Atmos. Environ. 105, 130–137. doi: 10.1016/j.atmosenv.2015.01.052
Jeanjean, A. P. R., Monks, P. S., and Leigh, R. J. (2016). Modelling the effectiveness of urban trees and grass on PM2.5 reduction via dispersion and deposition at a city scale. Atmos. Environ. 147, 1–10. doi: 10.1016/j.atmosenv.2016.09.033
Junikka, L. (1994). Survey of English macroscopic bark terminology. IAWA J., 151, 3–45. doi: 10.1163/22941932-90001338
Kassomenos, P. A., Vardoulakis, S., Chaloulakou, A., Paschalidou, A. K., Grivas, G., Borge, R., et al. (2014). Study of PM 10 and PM 2.5 levels in three European cities: analysis of intra and inter urban variations. Atmos. Environ. 87, 153–163. doi: 10.1016/j.atmosenv.2014.01.004
Kuik, P., and Wolterbeek, H. T. H. (1994). Factor analysis of trace-element data from tree-bark samples in the Netherlands. Environ. Monit. Assess. 32, 207–226. doi: 10.1007/BF00546277
Kuruneri-Chitepo, C., and Shackleton, C. M. (2011) The distribution, abundance composition of street trees in selected towns of the Eastern Cape, South Africa. Urban For. Urban Green. 10, 247–254. doi: 10.1016/j.ufug.2011.06.001
Legumes (2017). Version 10.38 20 July 2010-Scientific Names: Tipuana tipu (Benth.) Kuntze. Available online at: http://www.legumes-online.net/ildis/aweb/td006/td_01183.htm (Accessed December 13, 2017).
Liu, Y., Franklin, M., Kahn, R., and Koutrakis, P. (2007). Using aerosol optical thickness to predict ground-level PM2.5 concentrations in the St. Louis area: a comparison between MISR and MODIS. REMOTE Sens Environ. 107, 33–44. doi: 10.1016/j.rse.2006.05.022
Marć, M., Tobiszewski, M., Zabiegała, B., de la Guardia, M., and Namieśnik, J. (2015). Current air quality analytics and monitoring: a review. Anal. Chim. Acta 853, 116–126. doi: 10.1016/j.aca.2014.10.018
Masri, S., Kang, C. M., and Koutrakis, P. (2015). Composition and sources of fine and coarse particles collected during 2002–2010 in Boston, MA. J. Air Waste Mgt. 653, 287–297. doi: 10.1080/10962247.2014.982307
Mauad, T., Rivero, D. H. R. F., de Oliveira, R. C., de Faria Coimbra Lichtenfels, A. J., Guimaraes, E. T., de Andre, P. A., et al. (2008). Chronic exposure to ambient levels of urban particles affects mouse lung development. Am. J. Resp. Crit. Care 1787, 721–728. doi: 10.1164/rccm.200803-436OC
Mazza, M., Refojo, N., Bosco-Borgeat, M. E., Taverna, C. G., Trovero, A. C., Rog,é, A., et al. (2013). Cryptococcus gattii in urban trees from cities in North-eastern Argentina. Mycoses 56, 645–650. doi: 10.1111/myc.12084
Minganti, V., Drava, G., De Pellegrini, R., Anselmo, M., Modenesi, P., Malaspina, P., et al. (2015). The bark of holm oak Quercus ilex, L. for airborne Cr VI monitoring. Chemosphere 119, 1361–1364. doi: 10.1016/j.chemosphere.2014.02.026
Miranda, R. M., Andrade, M. F., Fornaro, A., Astolfo, R., Andrade, P. A., and Saldiva, P. (2012). Urban air pollution: a representation survey of PM2.5 mass concentrations in six Brazilian cities. Air Qual. Atmos. Health 5, 63–77. doi: 10.1007/s11869-010-0124-1
Molina, L. T., Molina, M. J., Slott, R. S., Kolb, C. E., Gbor, P. K., Meng, F., et al. (2004). Air quality in selected megacities. J. Air Waste Manag. Assoc. 54, 1–73. doi: 10.1080/10473289.2004.10471015
Moreira, T. C. L., de Oliveira, R. C., Amato, L. F. L., Kang, C. M., Saldiva, P. H. N., and Saiki, M. (2016). Intra-urban biomonitoring: source apportionment using tree barks to identify air pollution sources. Environ. Int. 91, 271–275. doi: 10.1016/j.envint.2016.03.005
Neter, J., Kutner, M. H., Nachtsheim, C. J., and Li, W. (2005). Applied Linear Statistical Models. 5th Edn. Chicago, IL: Irwin.
Nowak, D. J., Hirabayashi, S., Bodine, A., and Greenfield, E. (2014). Tree and forest effects on air quality and human health in the United States. Environ. Pollut. 193, 119–129. doi: 10.1016/j.envpol.2014.05.028
Oliva, S. R., and Mingorance, M. D. (2006). Assessment of airborne heavy metal pollution by aboveground plant parts. Chemosphere 652, 177–182. doi: 10.1016/j.chemosphere.2006.03.003
Oliveira, S., Andrade, H., and Vaz, T. (2011). The cooling effect of green spaces as a contribution to the mitigation of urban heat: a case study in Lisbon. Build Environ. 46, 2186–2194. doi: 10.1016/j.buildenv.2011.04.034
Pacheco, A. M. G., Barros, L. I. C., Freitas, M. C., Reis, M. A., Hipólito, C., and Oliveira, O. R. (2002). An evaluation of olive-tree bark for the biological monitoring of airborne trace-elements at ground level. Environ. Pollut. 1201, 79–86. doi: 10.1016/S0269-7491(02)00130-6
Pereira Filho, M. A., Pereira, L. A. A., Arbex, F. F., Arbex, M., Conceição, G. M., Santos, U. P., et al. (2008). Effect of air pollution on diabetes and cardiovascular diseases in São Paulo, Brazil. Braz. J. Med. Biol. Res. 416, 526–532. doi: 10.1590/S0100-879X2008005000020
Perelman, P., Faggi, A., Castro, M., and Carretero, E. M. (2010). Pollution trends using bark of Morus alba in the cities of Buenos Aires and Mendoza Argentina. Rev. Arvore 343, 505–511. doi: 10.1590/S0100-67622010000300014
Pope, C. A. III., Burnett, R. T., Thun, M. J., Calle, E. E., Krewski, D., Ito, K., et al. (2002). Lung cancer, cardiopulmonary mortality, and long-term exposure to fine particulate air pollution. Jama, 2879, 1132–1141. doi: 10.1001/jama.287.9.1132
Pope, C. A. III., Ezzati, M., and Dockery, D. W. (2009). Fine-particulate air pollution and life expectancy in the United States. New Engl J. Med. 3604, 376–386. doi: 10.1056/NEJMsa0805646
Santos, U. P., Garcia, M. L. S. B., Braga, A. L. F., Pereira, L. A. A., Lin, C. A., de André, P. A., et al. (2016). Association between traffic air pollution and reduced forced vital capacity: a study using personal monitors for outdoor workers. PLoS ONE 1110:e0163225. doi: 10.1371/journal.pone.0163225
Sashua-Bar, L., Potchter, O., Bitan, A., Boltansky, D., and Yaakov, Y. (2010). Microclimate modelling of street tree species effects within the varied urban morphology in the Mediterranean city of Tal Avivi, Israel. Int. J. Climatol. 30, 44–47. doi: 10.1002/joc.1869
Sawidis, T., Breuste, J., Mitrovic, M., Pavlovic, P., and Tsigaridas, K. (2011). Trees as bioindicator of heavy metal pollution in three European cities. Environ. Pollut. 15912, 3560–3570. doi: 10.1016/j.envpol.2011.08.008
Selmi, W., Weber, C., Rivière, E., Blond, N., Mehdi, L., and Nowak, D. (2016). Air pollution removal by trees in public green spaces in Strasbourg city, France. Urban For. Urban Green. 17, 192–201. doi: 10.1016/j.ufug.2016.04.010
Soares, A. L., Rego, F. C., McPherson, E. G., Simpson, J. R., Peper, P. J., and Xiao, Q. (2011). Benefits and costs of street trees in Lisbon, Portugal Urban For. Urban Green. 10, 69–70. doi: 10.1016/j.ufug.2010.12.001
Szczepaniak, K., and Biziuk, M. (2003). Aspects of the biomonitoring studies using mosses and lichens as indicators of metal pollution. Environ. Res. 93, 221–230. doi: 10.1016/S0013-9351(03)00141-5
Tarifa, J., and Azevedo, T. (2001). Os Climas na Cidade de São Paulo.: Universidade de São Paulo, Pró-Reitoria de Cultura e Extensão Universitária (São Paulo).
Teixeira, E. (2014). Evaluación de la estructura y comportamiento del arbolado urbano en Motevideo, Ph.D Thesis, Universidade de la República, Facultad de Agronomia, Montivideo, Uruguay.
United Nations (2012). Department of Economic and Social Affairs. Population Division. World Urbanization Prospects: The 2011 Revision. United Nations.
Vailshery, L. S., Jaganmohan, M., and Nagendra, H. (2013). Effect of street trees on microclimate and air pollution in a tropical city. Urban For. Urban Green. 123, 408–415. doi: 10.1016/j.ufug.2013.03.002
Van Donkelaar, A., Martin, R. V., Brauer, M., Hsu, N. C., Kahn, R. A., Levy, R. C., et al. (2016). Global estimates of fine particulate matter using a combined geophysical-statistical method with information from satellites, models, and monitors. Environ. Sci. Technol. 507, 3762–3772. doi: 10.1021/acs.est.5b05833
Van Grieken, R. E., and Markowicz, A. A. (2002). Handbook of X-ray Spectrometry, 2nd Edn. Revised And Expanded. (New York, NY; Basel: Marcel Dekker).
WHO (2017). 7 Million Premature Deaths Annually Linked to Air Pollution. Available online at: http://www.who.int/mediacentre/news/releases/2014/air-pollution/en/ (Accessed January 4, 2017).
Wolterbeek, B. (2002). Biomonitoring of trace element air pollution: principles, possibilities and perspectives. Environ. Pollut. 1201, 11–21. doi: 10.1016/S0269-7491(02)00124-0
Wolterbeek, H. T., and Bode, P. (1995). Strategies in sampling and sample handling in the context of large-scale plat biomonitoring surveys of trace element air pollution. Sci. Total Environ. 176, 33-43. doi: 10.1016/0048-9697(95)04828-6
World Urbanization Prospects (2017). World Urbanization Prospects-Population Division-United Nations. Available online at: http://esa.un.org/unpd/wup/Documentation/final-report.htm (Accessed June 13, 2017).
Yli-Pelkonen, V., Setälä, H., and Viippola, V. (2017). Urban forests near roads do not reduce gaseous air pollutant concentrations but have an impact on particles levels. Landscape and Urban Plan. 158, 39–47. doi: 10.1016/j.landurbplan.2016.09.014
Yunus, M., Yunus, D., and Iqbal, M. (1990). Systematic bark morphology of some tropical trees. Bot. J. Linn. Soc. 1034, 367–377. doi: 10.1111/j.1095-8339.1990.tb00196.x
Keywords: bark morphology, biomonitoring, EDXRF, green areas, tree species
Citation: Moreira TCL, Amato-Lourenço LF, da Silva GT, Saldiva de André CD, de André PA, Barrozo LV, Singer JM, Saldiva PHN, Saiki M and Locosselli GM (2018) The Use of Tree Barks to Monitor Traffic Related Air Pollution: A Case Study in São Paulo–Brazil. Front. Environ. Sci. 6:72. doi: 10.3389/fenvs.2018.00072
Received: 13 February 2018; Accepted: 15 June 2018;
Published: 13 July 2018.
Edited by:
Maurice Millet, Université de Strasbourg, FranceReviewed by:
Laurent Y. Alleman, IMT Lille Douai, FranceEleni Drakaki, National Technical University of Athens, Greece
Copyright © 2018 Moreira, Amato-Lourenço, da Silva, Saldiva de André, de André, Barrozo, Singer, Saldiva, Saiki and Locosselli. This is an open-access article distributed under the terms of the Creative Commons Attribution License (CC BY). The use, distribution or reproduction in other forums is permitted, provided the original author(s) and the copyright owner(s) are credited and that the original publication in this journal is cited, in accordance with accepted academic practice. No use, distribution or reproduction is permitted which does not comply with these terms.
*Correspondence: Tiana C. L. Moreira, dGlhbmFjYXJsYUB1c3AuYnI=
† These authors have contributed equally to this work.