- 1Animal Physiological Ecology, Institute for Evolution and Ecology, University of Tübingen, Tübingen, Germany
- 2Environmental Analytical Chemistry, University of Tübingen, Tübingen, Germany
- 3Steinbeis Transfer Center for Ecotoxicology and Ecophysiology, Rottenburg, Germany
Pharmaceuticals can enter surface waters via sewage treatment plants. In the environment, the substances and their transformation products, formed by the degradation of the parent compounds, can affect aquatic wildlife, including freshwater invertebrates. However, research on pharmaceutical-induced effects in wild freshwater organisms other than fish is still scarce. In our study, we investigated the impact of the highly consumed antidiabetic drug metformin and its main transformation product, guanylurea, on the health of a freshwater gastropod—the big ramshorn snail (Planorbarius corneus) by analysing its biochemical and cellular stress responses and apical parameters. The snails were exposed to different concentrations of the drug (0, 0.01, 0.1, 1, and 10 mg/L) and its transformation product (0, 0.1, 10, and 100 mg/L). The examined parameters were mortality, weight, tissue integrity of the hepatopancreas, and the levels of stress proteins and lipid peroxides. Mortality and the levels of stress proteins and lipid peroxides were not influenced by the two substances. In response to the highest concentrations of both chemicals, the weight of the snails was slightly but not significantly reduced. The histopathological investigation of the hepatopancreas revealed a significant effect of guanylurea at a concentration of 100 mg/L with an increased number of symptoms of cellular responses in the tissue (e.g., dilated lumen, disturbed compartmentation of the digestive cells, nucleus deformation, hyperplasia, and hypertrophy of crypt cells). For the parent compound, a similar trend was also observed for the highest concentration. Overall, the observed effects did not occur at environmentally relevant concentrations, but at concentrations which were 10,000 times higher than these. Thus, the results did not give rise to a major concern that metformin and guanylurea could pose a risk to the big ramshorn snail in the environment.
Introduction
The antidiabetic drug metformin, which is used to treat type 2 diabetes, is one of most prescribed pharmaceuticals worldwide (Gong et al., 2012; Scheurer et al., 2012). Its pharmaceutical effect is related to a reduction in blood glucose level due to a reduction in gluconeogenesis and glycogenolysis, and an increase in glucose uptake into cells (Hundal et al., 1992; Wiernsperger and Bailey, 1999; Natali and Ferrannini, 2006; An and He, 2016). The hepatic metabolism of metformin is negligible (Scheen, 1996) and ~52% of the drug is excreted in the urine after 48 h in humans (Pentikäinen et al., 1979). Consequently, it can enter sewage treatment plants at concentrations of 101–129 μg/L (Scheurer et al., 2009). During wastewater treatment, ~90% of the drug is removed from the treated sewage and microbially transformed into guanylurea (Scheurer et al., 2012; Oosterhuis et al., 2013; Tisler and Zwiener, 2018, 2019). In surface waters, Scheurer et al. (2012) measured metformin and its transformation product at concentrations of up to 3 μg/L and up to 28 μg/L, respectively. Several studies have addressed possible metformin-induced effects in fish (Niemuth and Klaper, 2015, 2018; Niemuth et al., 2015; Crago et al., 2016; Jacob et al., 2018; Ussery, 2018), however, to the best of our knowledge, only a single study has reported the negative effects of guanylurea on fish (Ussery, 2018). Furthermore, data on the effects of metformin and its transformation product on invertebrates are scarce. In two studies, the acute toxicity of metformin and guanylurea was determined by means of the acute Daphnia magna immobilisation test, resulting in EC50 (effective concentration)-values of 64 mg/L for metformin (Cleuvers, 2003) and of 40 mg/L for guanylurea (Markiewicz et al., 2017) after 48 h. Caldwell et al. (2019) showed that the geometric average of the no observed effect concentrations (NOEC) of five OECD 211 chronic reproduction tests with Daphnia magna was 45.2 mg/L for metformin. In a chronic reproduction study with Ceriodaphnia dubia according to the ISO 30665, a NOEC of 8 mg/L was determined for guanylurea (Caldwell et al., 2019). García-García et al. (2017) showed that the population increase of two rotifers was affected by metformin at a concentration of 25 μg/L, however the effects of guanylurea have not been investigated. To the best of our knowledge, no study so far has focussed on the effects of metformin and guanylurea on freshwater gastropods. We chose the big ramshorn snail (Planorbarius corneus) as a representative gastropod since it is widespread and has a relevant function in freshwater ecosystems serving as prey for fish and birds (Costil and Bailey, 1998). Thus, the aim of our study was to investigate whether the two substances can influence the health of the big ramshorn snail (Planorbarius corneus) by: (1) investigating mortality and body weight, (2) analysing stress proteins in the hepatopancreas and the lipid peroxide level in the foot, and (3) examining the histopathological effects in the hepatopancreas.
The levels of heat shock proteins with an approximate weight of 70 kDa were measured in the hepatopancreas of the test organisms. These proteins belong to the Hsp70 stress protein family and are highly conserved across a wide range of taxa (Margulis et al., 1989). They serve to fold unfolded proteins and to re-fold partially damaged proteins. Analysis of the level of stress proteins is a general, unspecific marker for all kinds of proteotoxic stressors (Köhler and Triebskorn, 2004). Piro et al. (2012) showed that expression of Hsp70 in the pancreas of rats exposed to high free fatty acids levels can be reduced by metformin. Thus, we wanted to investigate whether metformin might exert similar effects on the Hsp70 level in an aquatic non-target organism. To our knowledge, no data is available on possible influences of guanylurea on stress proteins.
To verify oxidative damage, the lipid peroxide level was analysed in the foot of the snails, which is an organ in direct contact with the test medium containing metformin or guanylurea. Lipid peroxides result from reaction of reactive oxygen species (ROS) with polyunsaturated lipids (Monserrat et al., 2003). These ROS are produced by a variety of biochemical processes, such as aerobic respiration or the metabolism of xenobiotics (Betteridge, 2000; Valavanidis et al., 2006). Whenever the balance between the generation of ROS and the anti-oxidative defence system is disturbed, oxidative stress arises (Betteridge, 2000). Under oxidative stress conditions, lipid peroxides might lead to a loss of essential cellular functions (Hermes-Lima et al., 1995). For example, the membrane fluidity of mitochondria can be altered (Chen and Yu, 1994) resulting in an impaired function of the respiration chain and cellular energetics (Bindoli, 1988). Metformin is known to reduce oxidative stress and to attenuate lipid peroxidation in vitro in human leukocytes and in vivo in rodents and humans (Tessier et al., 1999; Anurag and Anuradha, 2002; Bonnefont-Rousselot et al., 2003; Martin-Montalvo et al., 2013). It is not known, whether metformin exerts similar effects in non-target organisms such as the big ramshorn snail. Furthermore, there is no information about the effects of guanylurea on oxidative stress.
The hepatopancreas (syn. midgut gland, digestive gland) is the main metabolic organ in molluscs. Its functions include the digestion and absorption of nutrients, as well as the detoxification and immobilisation of xenobiotics (Dallinger and Wieser, 1984; Roszer, 2014). Several studies have shown that chemicals and other stressors can affect the hepatopancreas of snails both structurally and biochemically (Klobučar et al., 1997; Otludil et al., 2004; Zaldibar et al., 2007; Dittbrenner et al., 2008; Osterauer et al., 2010), thus, histopathological investigations are a suitable tool to evaluate the health status of stress-exposed gastropods.
Overall, we assessed the effects of metformin and guanylurea in the big ramshorn snail (Planorbarius corneus), focusing on biochemical and histological analyses as well as apical parameters.
Materials and Methods
Test Organism
The big ramshorn snail (Planorbarius corneus) is a freshwater pulmonate that belongs to the Planorbidae family. This hermaphroditic snail lives in stagnant or slowly moving waters in Europe (Glöer, 2002). The individuals used for the present study were the progeny of snails obtained from the pet shop Kölle Zoo (Stuttgart, Germany) and the shipping company Bachflohkrebse.de (Stuttgart, Germany). The big ramshorn snail is a good model organism since it is easy to handle in the laboratory and has a rather short generation time (Costil and Daguzan, 1995). Moreover, it is an important representative of non-target freshwater gastropods and is sensitive to pollutants (Otludil et al., 2004).
Test Substances
Metformin hydrochloride was purchased from Sigma-Aldrich (CAS number: 1115-70-4; Batch number: BCBP0558V; purity 99.9%; St. Louis, MO, USA). It was readily soluble in water with a water solubility of 16.56 g/L (TOCRIS, 2017). The concentrations of metformin investigated in the present study refer to the metformin free base (C4H11N5) without the anion.
Guanylurea sulphate was purchased from Tokyo Chemical Industry (CAS number: 591-01-5; Lot: WIA7F and AKJLG; purity 98%; Tokyo, Japan). With a solubility in water of 50 g/L (ChemIDplus1), the substance was readily soluble in water. The concentrations of guanylurea applied in the present study were based on the pure ingredient (C2H6N4O) without the anion.
Exposure Experiments
The snails were exposed to different nominal water concentrations of metformin (0, 0.01, 0.1, 1, 10 mg/L) and guanylurea (0, 0.1, 10, 100 mg/L) at 11°C in a climate chamber with three replicates per concentration. This temperature was chosen to simulate spring conditions. The experiment with metformin lasted 35 d (11.11.2016–16.12.2016), the test with guanylurea 21 d (02.06.2017–23.06.2017). The exposure took place in a semi-static system in glass aquaria containing 10 L medium and seven snails each. Once a week, 50% of the medium was replaced with freshly prepared medium using aerated, filtered tap water (iron filter, active charcoal filter, particle filter). The snails were fed every second day with commercial algae tablets (NovoPleco, JBL, Neuhofen, Germany). Excess food or faeces were removed during the water exchange process. The illumination conditions were set to a 10 h light/14 h dark-cycle to account for the mean day length under spring conditions. Water temperature, oxygen content, conductivity, and pH were measured at the beginning and end of the experiments. At the end of the experiments, the snails were weighed and their shells were removed. For 15 snails per treatment (five individuals per replicate aquarium), the anterior part of the hepatopancreas was chemically fixed with glutardialdehyde for histological examinations, and the posterior part was frozen in liquid nitrogen for stress protein analyses. Moreover, from every dissected individual, samples of the foot were frozen in liquid nitrogen for lipid peroxide analysis.
Chemical Analyses
For the quantification of metformin and guanylurea in the respective aquaria, water samples were taken at the beginning and end of the experiments as well as before and after a water exchange at days 7 and 21 for the experiment with metformin, and at days 6 and 12 for the experiment with guanylurea (see Supplement Paragraph 1, Tables S4, S5). The samples were stored at−20°C until processing. The determination of the actual water concentrations was performed by liquid chromatography coupled with mass spectrometry (LC-MS) using a 1,260 Infinity high pressure liquid chromatography (HPLC) system (Agilent Technologies, Waldbronn, Germany) with a triple quadrupole mass spectrometer (QqQ-MS 6490, Agilent Technologies, Santa Clara, CA, USA). For the separation, a Phenomenex LUNA 5 u HILIC 200 A column (150 × 3 mm; 5 μm particle size) with a flow rate of 0.5 mL/min at 40°C was used. A gradient elution was performed with eluent A (aqueous buffer with 15 mM ammonium formate and 0.1% formic acid) and eluent B (acetonitrile with 0.1% formic acid) (all chemicals purchased from Fisher Scientific, Schwerte, Germany). For 0–4 min, 95% of eluent B was used, decreased to 50% within 4 min and held for 6 min. After switching back to the starting conditions, post time was 8 min.
Samples (composed of 90% acetonitrile and 10% H2O due to dilution) were kept in the autosampler at 10°C and the injection volume was 10 μL. A calibration was typically performed between 0.1 and 10 μg/L in 90% acetonitrile and 10% H2O with metformin-D6 as an internal standard. The concentration of metformin-D6 was 1 μg/L in the diluted samples and calibration standards. The ionisation of substances was performed in the positive ionisation mode. Further details of the operating parameters of the electrospray ionisation source and the Agilent Jet Stream technology are provided in the (Supplement Paragraph 1, Table S1). The acquired data were processed using the software Mass Hunter (Agilent Technologies). For quantification and confirmation, mass transitions were monitored for each analyte in the dynamic multiple reaction monitoring mode. Details are given in the (Supplement Paragraph 1, Table S2). The limit of quantification (LoQ) was 1 ng/L for metformin and 10 ng/L for guanylurea. Intra- and inter-day variations in the analytical method are given in the (Supplement Paragraph 1, Table S3).
Stress Protein Analyses
To quantify Hsp70 in the hepatopancreas of the snails, samples were homogenised with a mixture of 98% extraction buffer and 2% protease inhibitor (3 mL mixture/g sample) according to the protocol described by Dieterich et al. (2015). The total protein content was determined according to Bradford (1976). The proteins were separated according to their weight using sodium dodecyl sulphate polyacrylamide gel electrophoresis (SDS-PAGE). A standardised amount of 40 μg total protein was analysed per sample. After semi-dry blotting onto a nitrocellulose membrane, a primary antibody (monoclonal α-Hsp70 IgG; Dianova, Hamburg, Germany) was used to specifically detect all isoforms of the Hsp70 protein family, followed by the binding of a secondary antibody (peroxidase-coupled α-IgG; Jackson Immunoresearch, West Grove, PA, USA) to the primary antibody. Membranes were stained with 4-chloro-1-naphthol, scanned, and the optical volume (band area × average grey scale value) of the samples was quantified using the image processing program ImageJ in relation to an internal standard (brown trout whole body homogenate).
Lipid Peroxide Analyses
The level of lipid peroxides in the foot of the snails was determined using the FOX (ferrous oxidation xylenol orange)-assay according to a modified version of the protocol from Hermes-Lima et al. (1995) and Monserrat et al. (2003). Foot samples were diluted 1:4 in HPLC-grade methanol, homogenised, and centrifuged at 4°C at 14,000 rpm for 5 min. In each well, 50 μL each of FeSO4, H2SO4, and xylenol orange, 25 μL sample supernatant, and 25 μL bi-distilled water were added resulting in a total volume of 200 μL. Each sample was tested in triplicate and also as sample blank without FeSO4 to account for endogenous ferrous in the foot sample. Subsequently, the samples were incubated for 75 min (for the metformin experiment) and for 65 min (for the guanylurea experiment) at room temperature. Then, the absorbance was measured at 570 nm using an automated microplate reader (Bio-Tek Instruments; Winooski, VT, USA) and 1 μL of 1 mM cumene hydroperoxide (CHP) solution was added per well. After 30 min of incubation at room temperature, the absorbance was measured again and cumene hydroperoxide equivalents (CHPequiv.) were calculated according to the following equation:
where volumeCHP = 1 μL; total volume = 200 μL; sample volume = 25 μL; and dilution factor = 4.
Histopathological Investigations
The samples were fixed in 2% glutardialdehyde (Merck KGaA, Darmstadt, Germany) diluted with a cacodylate-sodium buffer (0.01 M, pH 7.4; AppliChem GmbH, Darmstadt, Germany) for at least 2 weeks. After washing with the buffer, the samples were dehydrated with a graded ethanol series and decalcified with a 1:2 mixture of formic acid and 70% ethanol. Then, the samples were infiltrated with paraffin wax (Parablast, Leica, Wetzlar, Germany) in a tissue processor (TP 1020, Leica). Subsequently, the samples were cut into 3 μm slices with a microtome (SM 2000 R, Leica) and stained with haematoxylin-eosin in an automatic slide stainer (Varistain 24-4, Thermo Scientific, Germany; protocol in the Supplement Paragraph 2). The investigation of the hepatopancreas was conducted using a light microscope (Axioskop 2, Zeiss, Oberkochen, Germany) in three steps: first, the status of the tissue was qualitatively described, second, the samples were classified in a semi-quantitative manner into one of five categories (1: control, 2: slight reaction, 3: medium reaction, 4: strong reaction, 5: destruction) according to the classification scheme of Osterauer et al. (2010). In the third step, the samples were categorised again in a blinded and randomised manner to avoid observer bias in the final evaluation. Two people each performed the histological examination for one experiment and both analyses were verified by the first author.
Statistical Analysis
Statistical analyses were performed using JMP 13 from SAS (Cary, NC, USA). Upon necessity to achieve normal distribution and homoscedasticity, data were transformed with square root (body weight data of metformin) or natural log (body weight data of guanylurea) functions. Mortality data were analysed using a COX-regression. For weight, stress protein and lipid peroxide analyses, a nested ANOVA using the replicate as a nesting factor, followed by a Dunnett's post-hoc test was performed. For the histopathological investigation, a likelihood-ratio-χ2-test was used to detect significant differences between the exposure groups. The statistical tests used and the corresponding p-values are provided in the “Results” section. Further information (degrees of freedom, number of individuals investigated, χ2- and F-values) is provided in the (Supplement Paragraph 3). The α-level was set to 0.05; in the case of multiple comparisons, the α-level was adjusted with a sequential Bonferroni correction. A statistical comparison of the results of the experiment with metformin with those of the experiment with guanylurea was not possible since the experiments were not conducted at the same time and the exposure time was different. Consequently, we only compared the data from the two experiments in a qualitative way.
Credibility of Data
Details about the fulfilment of the criteria for reporting and evaluating ecotoxicity data (CRED) according to Moermond et al. (2016) are given in the (Supplement Paragraph 4).
Results
Water Quality Parameters
Temperature, oxygen concentration, pH, and conductivity were measured at the beginning and end of the experiments (metformin: temperature = 10.30 ± 0.13°C; oxygen concentration = 10.03. ± 0.27 mg/L.; pH = 8.16 ± 0.09; conductivity = 460.73 ± 6.60 μS/cm; guanylurea: temperature = 10.55 ± 0.23°C; oxygen concentration = 10.58 ± 0.17 mg/L; pH = 8.32 ± 0.04; conductivity = 499.42 ± 26.93 μS/cm). Further details regarding these measurements are given in the (Supplement Paragraph 5).
Chemical Analysis
The measured water concentrations in the test aquaria were in good accordance with the nominal water concentrations of metformin and guanylurea (Tables 1, 2). In the experiment with guanylurea, a slight contamination in the control medium was detected, however, the contamination was a factor of 100 less than the lowest guanylurea concentration, therefore, we assumed the guanylurea test concentrations to be high enough to tolerate the traces of guanylurea in the control.
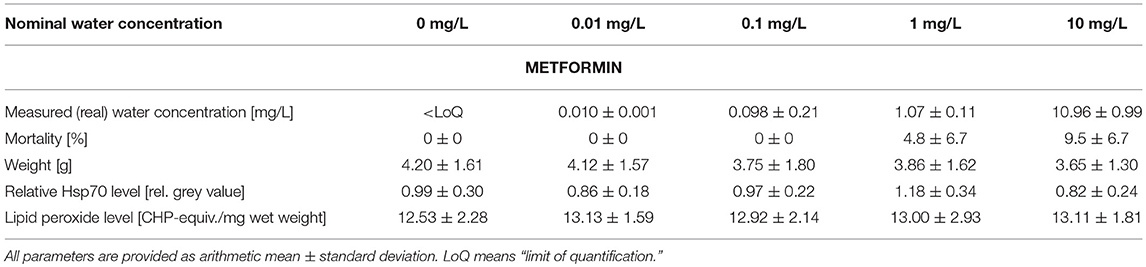
Table 1. Summary of measured metformin water concentrations as well as data on mortality, weight, stress proteins, and lipid peroxides measured in big ramshorn snails exposed to metformin.
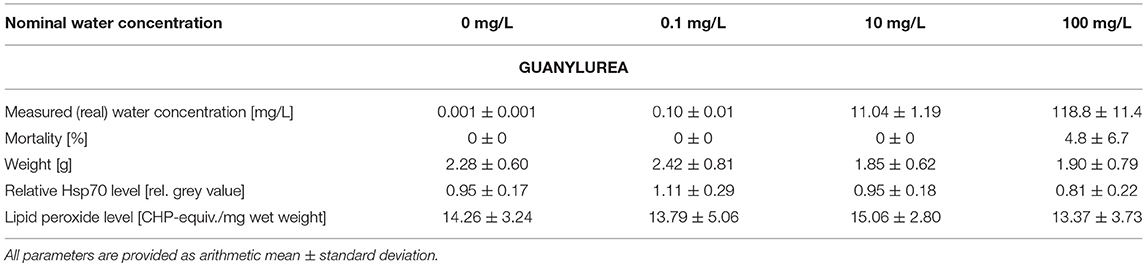
Table 2. Summary of measured guanylurea water concentrations as well as data on mortality, weight, stress proteins, and lipid peroxides measured in big ramshorn snails exposed to guanylurea.
Mortality and Body Weight
The mortality varied between 0.0 and 9.5% in all treatments and was insignificantly increased by metformin or guanylurea (COX-regression: metformin: p = 1; guanylurea: p = 1) (Tables 1, 2). There was a trend of reduced body weight in the exposure groups with 0.1–10 mg/L metformin and 10 and 100 mg/L guanylurea. However, the body weight of the snails did not differ significantly among the exposure groups in both experiments (nested ANOVA: metformin: p = 0.7719; guanylurea: p = 0.0172; with Dunnett's test: all p > 0.05) (Tables 1, 2).
Biochemical Markers
The Hsp70 level was slightly enhanced in the exposure group with 0.1 mg/L guanylurea and 1 mg/L metformin compared to the respective controls (nested ANOVA: metformin: p = 0.0050 with Dunnett's test: (0 and 1 mg/L): p = 0.0075; guanylurea: p = 0.0091; with Dunnett's test: all p > 0.05) (Tables 1, 2). The lipid peroxide level analysis did not reveal any differences among the groups exposed to metformin or guanylurea (nested ANOVA: metformin: p = 0.1264; guanylurea: p = 0.6889) (Tables 1, 2).
Histopathology
The hepatopancreas of gastropods consists of numerous tubules surrounded by connective and muscle tissue and haemolymph spaces. Each tubule consists of three main cell types: (a) digestive cells with a columnar shape and vacuoles that increase in size towards the cell base (Figure 1A), (b) crypt cells (syn. basophilic or calcium cells) with a conic shape and a big, centrally located nucleus (Figure 1A), and (c) excretory cells with large vacuoles containing cellular debris prior to excretion (Triebskorn, 1989). Possible pathological alterations include: (1) dilation of the tubule's lumen, (2) the protrusion of the apices, irregular compartmentation, and an increase in the number and size of the vacuoles in the digestive cells, and (3) alterations and deformations of the nuclei, hyperplasia, and hypertrophy, as well as increase in vacuoles' size and number in the cytoplasm of the crypt cells (Osterauer et al., 2010).
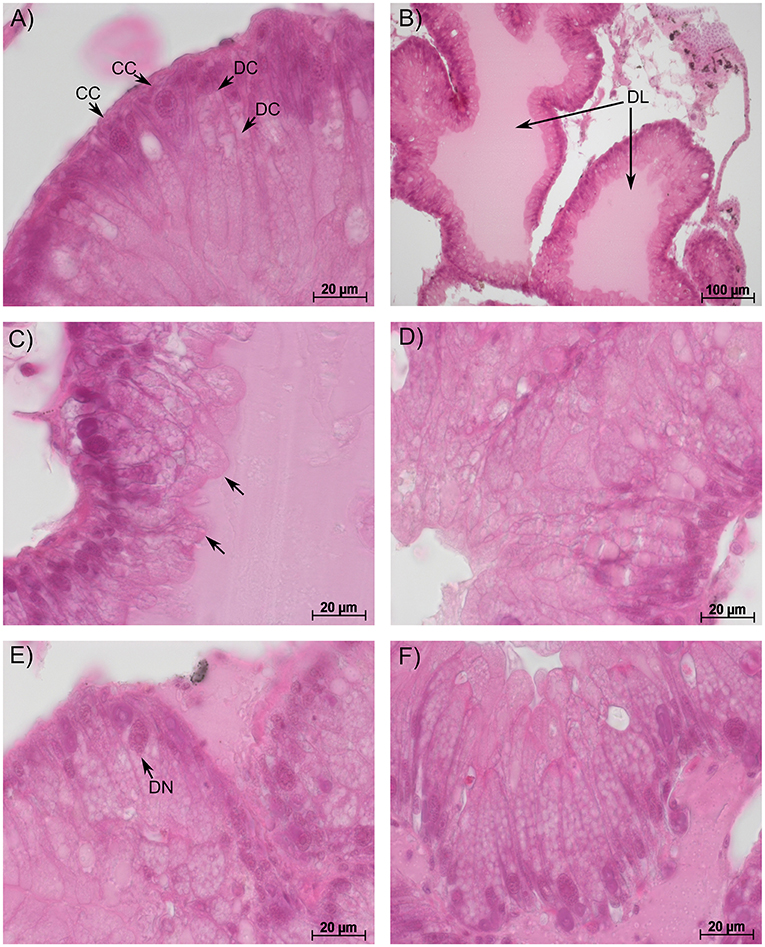
Figure 1. Representative sections of the hepatopancreas in big ramshorn snails. (A): control snails showed conically shaped crypt cells [CC] with a large nucleus and column-shaped digestive cells [DC] with vacuoles increasing in size from the apex to the base; (B): snails exposed to 100 mg/L guanylurea showed a dilated lumen [DL] of the tubules; (C): in snails exposed to 10 mg/L metformin, the apex of the digestive cells was irregularly shaped and protruded into the lumen [arrow]; Snails exposed to 100 mg/L guanylurea displayed (D) irregularly shaped digestive cells with an affected cell compartmentation, (E) deformation of crypt cell nuclei [DN] and (F) a severe vacuolisation of the hypertrophic crypt cells. In this exposure group almost all crypt cells were hypertrophic and increased in number. All sections were stained with haematoxylin-eosin.
In the semi-quantitative assessment, the samples were solely classified into categories 1, 2, or 3. Destruction of the tissue and, thus, a classification into categories 4 and 5, did not occur. The hepatopancreas of snails exposed to metformin did not significantly differ to that of controls (Likelihood-ratio-χ2-test: p = 0.5159). However, in metformin-exposed individuals, tissues were more often classified into category 3 (reactions), particularly for the exposure group with the highest metformin concentration (Figure 2). In these animals, reactions such as dilation of the lumen, vacuolisation in crypt cells, and protrusion of the apex in digestive cells (Figure 1C) were observed with a tendentially higher frequency than in controls.
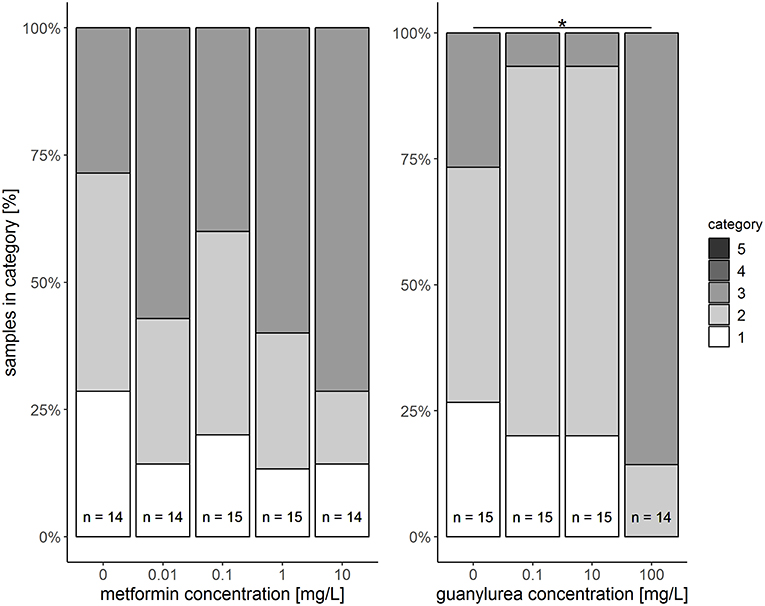
Figure 2. Semi-quantitative assessment of histopathological symptoms in the hepatopancreas of big ramshorn snails exposed to metformin and guanylurea. Samples were classified in category 1 to 5. Categories 4 and 5 were not allocated to any of the samples. The number n of examined individuals is given at the bases of the bars. The asterisk indicates a significant difference compared to the respective control at the α = 0.05 level.
The hepatopancreas of snails exposed to the highest guanylurea concentration (100 mg/L) significantly differed from that of the controls (Likelihood-ratio-χ2-test: p < 0.0001; pairwise comparison: (0 mg/L and 100 mg/L): p = 0.0018) (Figure 2). The lumen of the tubules was enlarged (Figure 1B), compartmentation in the digestive cells was disturbed (Figure 1D), nuclei were deformed (Figure 1E), and hyperplasia, hypertrophia, and vacuolisation of crypt cells became evident (Figure 1F). No such effects were observed in snails exposed to 0.1 and 10 mg/L guanylurea.
Discussion
The survival of the snails was not affected by the antidiabetic drug or its transformation product. A trend to a reduction in the weight of the snails exposed to the medium and high concentrations of each substance was observed, however, there was a high variability in weight within the groups, which may have contributed to these differences lacking statistical significance. Moreover, a decreased food uptake was observed during the experiments for the above mentioned exposure groups. Unfortunately, this was not quantifiable. Nevertheless, the observed trend to weight reduction is in accordance with other studies with metformin-exposed fish (Niemuth and Klaper, 2015; Jacob et al., 2018; Ussery, 2018) and with the application of metformin as weight loss drug in humans (Seifarth et al., 2013; Malin and Kashyap, 2014). Upon administration of metformin in humans, appetite is downregulated by attenuating the activity of the adenosine monophosphate-activated kinase, which is a main sensor for cellular energy homeostasis (Malin and Kashyap, 2014; Jeon, 2016). It is not known how guanylurea could have influenced food uptake in the snails.
The analyses of the stress proteins revealed a significantly enhanced Hsp70 level in the group treated with 1 mg/L metformin, and a tendentially increased value for the exposure group with 0.1 mg/L guanylurea. This might be ascribed to the higher variability in these two exposure groups. However, an increased induction of Hsp70 caused by the tested substances is unlikely since the histopathological examinations do not show any reactions in these exposure groups (see below). Furthermore, a decrease in the Hsp70 base level caused by metformin as shown by Piro et al. (2012) was not observed. We assume, therefore, that metformin could not exert its stress protein lowering effect in the big ramshorn snail, since the Hsp70 level was not elevated prior to metformin treatment, as in the study by Piro et al. (2012) which used a Hsp70 overexpression model. For guanylurea, a comparison of our results with the literature is not possible, since other studies dealing with the effects of guanylurea on stress proteins are—to our knowledge—lacking.
An effect of metformin or its transformation product on the lipid peroxide level in the foot of Planorbarius corneus could not be found. Since the oxidative stress level is enhanced in type 2 diabetic patients (Ceriello et al., 1997), metformin has been shown to act as an antioxidant and to reduce oxidative stress and lipid peroxidation in these patients (Tessier et al., 1999) and high fructose-fed rats with insulin resistance (Anurag and Anuradha, 2002). Thus, an explanation for the absence of a lipid peroxide level-reducing effect of metformin in Planorbarius corneus could be that the oxidative stress level in the snails was not enhanced before metformin treatment. However, it is also possible that the activity of antioxidant enzymes, such as glutathione peroxidase, superoxide dismutase, or catalase, was enhanced by metformin when an external oxidative stress inducer was present, as it has been shown for all three enzymes in human macrophages (Bułdak et al., 2014) and for catalase in mouse livers (Dai et al., 2014), prior to the effects of the drug on lipid peroxidation. To prove that metformin leads to an elevated activity of these three antioxidant enzymes in Planorbarius corneus, further measurements would be necessary. However, this was not possible in the present study due to the restrictions of tissue availability for the different analyses. Furthermore, data in the literature regarding guanylurea and its effect on lipid peroxides is lacking.
The histopathological examination revealed significantly stronger reactions in snails exposed to 100 mg/L guanylurea than the controls. For metformin, a similar trend was found, particularly for the highest concentration. The observed reaction pattern was characterised by a dilation of the tubule lumen and a disturbed compartmentation of the digestive cells. These reactions have also been observed in other studies with snails exposed to copper, lithium (Sawasdee et al., 2011), or platinum (Osterauer et al., 2010). Furthermore, protrusions of the apices of the digestive cells into the lumen occurred. This symptom has also been reported in a study of Dittbrenner et al. (2008) on snails exposed to heat stress. The observed hyperplasia of the crypt cells has been described as a very common response to all types of stressors (Cajaraville et al., 1990; Garmendia et al., 2011). Possible explanations for the increase in cell number and the vacuolisation of the crypt cells could be an enhanced demand of enzymes to support affected digestive cells in their function (Cajaraville et al., 1990) or increasing detoxification processes (Zaldibar et al., 2007). Another finding was the deformation of cell nuclei, which has also been observed in other studies on the hepatopancreas of snails during exposure to molluscicides (Triebskorn, 1989; Hamed et al., 2007) or to the cyanobacterium Microcystis aeruginosa (Zhu et al., 2011). Generally, the histopathological findings in our study indicate that metformin and guanylurea lead to unspecific pathological symptoms in the hepatopancreas of the exposed snails.
However, it is important to emphasise that all of our observed histological reactions only occurred at concentrations which are 10,000 times higher than those in the aquatic environment. In addition, even at these high concentrations, only moderate reactions, if any, and never destruction of the tissue became evident. From this point of view, metformin and guanylurea seem not to pose a risk to aquatic gastropods (represented by the big ramshorn snail in this study). However, due to the lack of studies with other invertebrates we cannot exclude the possibility that metformin and its transformation product can affect other species that were not tested. Recent studies by Niemuth et al. (2015), Niemuth and Klaper (2018, 2015), and Crago et al. (2016) showed that metformin can affect the hormonal system of the fathead minnow at environmentally relevant concentrations. This result is in line with metformin acting on the hormonal system in humans, which explains its application as a drug for the treatment of polycystic ovary syndrome (Lord et al., 2003). Since in our study endocrine effects were not investigated, we cannot comment on any potential effects of metformin or its transformation product on the hormonal system in the big ramshorn snail. Thus, further studies are necessary that focus on the possible hormonal-like effects of metformin and guanylurea in snails.
Finally, it is important to stress that there is a general dearth of studies dealing with ecotoxicological effects of guanylurea in aquatic organisms. Only two such studies on invertebrates exist to date. To assess the risk of this transformation product on a more solid basis, further investigations on the ecotoxicological effects of guanylurea in invertebrates are thus highly needed.
Conclusion
Our study showed that metformin and guanylurea did not produce lethal effects in exposed Planorbarius corneus. Furthermore, the biochemical stress markers (Hsp70 and lipid peroxide level) were not influenced by the antidiabetic drug or its transformation product. The histopathological investigation revealed an increase in unspecific reactions in the hepatopancreas of the snails, but only at concentrations 10,000 times higher than the concentrations measured in the environment. In addition, the weight of the snails tended to be reduced, but also not at environmentally relevant concentrations.
Generally, studies focussing on the effects of pharmaceuticals and their transformation products in gastropods and other invertebrates are scarce, and metformin and its transformation product guanylurea do not constitute an exception. Thus, a final risk assessment of the investigated substances for freshwater invertebrates is not yet possible.
Author Contributions
ST analysed the water samples during the experiment. CZ supervised the chemical analyses of the water samples. SJ performed the exposure experiment, conducted the lipid peroxide analysis, was involved in the stress proteins analysis, examination of the hepatopancreas tissue, and the corresponding statistical analyses. RT supervised the histological examinations and designed the study. H-RK supervised the biochemical analyses. All authors critically revised and approved the final manuscript.
Funding
The study was funded by the Wassernetzwerk Baden-Württemberg framework of the Ministry for Science, Research and Arts of Baden-Württemberg (Grant No. 33-5733-25-11t32/2).
Conflict of Interest Statement
The authors declare that the research was conducted in the absence of any commercial or financial relationships that could be construed as a potential conflict of interest.
Acknowledgments
This study is part of the project Effect-Net (Effect Network in Water Research) funded within the Wassernetzwerk Baden-Württemberg framework by the Ministry for Science, Research and Arts of Baden-Württemberg. Particular thanks go to Thomas Braunbeck for the coordination of this project. We acknowledge support by Deutsche Forschungsgemeinschaft and the Open Access Publishing Fund of the University of Tübingen. We are grateful to Melanie Biecker, Joanna Probst, and Jochen Schwantzer for the histological examination and the analysis of stress proteins which were conducted in the context of their bachelor and master theses. Furthermore, we thank Andreas Dieterich, Helene Eckstein, Stefanie Krais, Katharina Peschke, Carla Lorenz, Hannah Schmieg, Simon Schwarz, Sabrina Wilhelm, and Michael Ziegler for technical support and assistance in the lab.
Supplementary Material
The Supplementary Material for this article can be found online at: https://www.frontiersin.org/articles/10.3389/fenvs.2019.00045/full#supplementary-material
Footnotes
1. ^ChemIDplus. TOXNET Toxicology Data Network [Online]. Available online at: https://chem.nlm.nih.gov/chemidplus/name/guanylurea (accessed August 16, 2018).
References
An, H., and He, L. (2016). Current understanding of metformin effect on the control of hyperglycemia in diabetes. J. Endocrinol. 228, R97–R106. doi: 10.1530/JOE-15-0447
Anurag, P., and Anuradha, C. (2002). Metformin improves lipid metabolism and attenuates lipid peroxidation in high fructose-fed rats. Diabetes Obes. Metab. 4, 36–42. doi: 10.1046/j.1463-1326.2002.00178.x
Betteridge, D. J. (2000). What is oxidative stress? Metabolism 49, 3–8. doi: 10.1016/s0026-0495(00)80077-3
Bindoli, A. (1988). Lipid peroxidation in mitochondria. Free Radic. Biol. Med. 5, 247–261. doi: 10.1016/0891-5849(88)90018-4
Bonnefont-Rousselot, D., Raji, B., Walrand, S., Gardes-Albert, M., Jore, D., Legrand, A., et al. (2003). An intracellular modulation of free radical production could contribute to the beneficial effects of metformin towards oxidative stress. Metabolism 52, 586–589. doi: 10.1053/meta.2003.50093
Bradford, M. M. (1976). A rapid and sensitive method for the quantitation of microgram quantities of protein utilizing the principle of protein-dye binding. Anal. Biochem. 72, 248–254. doi: 10.1016/0003-2697(76)90527-3
Bułdak, Ł., Łabuzek, K., Bułdak, R. J., Kozłowski, M., Machnik, G., Liber, S., et al. (2014). Metformin affects macrophages' phenotype and improves the activity of glutathione peroxidase, superoxide dismutase, catalase and decreases malondialdehyde concentration in a partially AMPK-independent manner in LPS-stimulated human monocytes/macrophages. Pharmacol. Rep. 66, 418–429. doi: 10.1016/j.pharep.2013.11.008
Cajaraville, M. P., Diez, G., Marigómez, J., and Angulo, E. (1990). Responses of basophilic cells of the digestive gland of mussels to petroleum hydrocarbon exposure. Dis. Aquat. Org. 9, 221–228. doi: 10.3354/dao009221
Caldwell, D. J., D'Aco, V., Davidson, T., Kappler, K., Murray-Smith, R. J., Owen, S. F., et al. (2019). Environmental risk assessment of metformin and its transformation product guanylurea: II. Occurrence in surface waters of Europe and the United States and derivation of predicted no-effect concentrations. Chemosphere 216, 855–865. doi: 10.1016/j.chemosphere.2018.10.038
Ceriello, A., Bortolotti, N., Falleti, E., Taboga, C., Tonutti, L., Crescentini, A., et al. (1997). Total radical-trapping antioxidant parameter in NIDDM patients. Diabetes Care 20, 194–197. doi: 10.2337/diacare.20.2.194
Chen, J. J., and Yu, B. P. (1994). Alterations in mitochondrial membrane fluidity by lipid peroxidation products. Free Radical Biol. Med. 17, 411–418. doi: 10.1016/0891-5849(94)90167-8
Cleuvers, M. (2003). Aquatic ecotoxicity of pharmaceuticals including the assessment of combination effects. Toxicol. Lett. 142, 185–194. doi: 10.1016/S0378-4274(03)00068-7
Costil, K., and Bailey, S. (1998). Influence of water temperature on the activity of Planorbarius corneus (L.) (Pulmonata, Planorbidae). Malacologia 39, 141–150.
Costil, K., and Daguzan, J. (1995). Comparative life-cycle and growth of 2 freshwater gastropod species, Planorbarius corneus (L.) and Planorbis planorbis (L.) Malacologia 37, 53–68.
Crago, J., Bui, C., Grewal, S., and Schlenk, D. (2016). Age-dependent effects in fathead minnows from the anti-diabetic drug metformin. Gen. Comp. Endocrinol. 232, 185–190. doi: 10.1016/j.ygcen.2015.12.030
Dai, J., Liu, M., Ai, Q., Lin, L., Wu, K., Deng, X., et al. (2014). Involvement of catalase in the protective benefits of metformin in mice with oxidative liver injury. Chem. Biol. Interact. 216, 34–42. doi: 10.1016/j.cbi.2014.03.013
Dallinger, R., and Wieser, W. (1984). Patterns of accumulation, distribution and liberation of Zn, Cu, Cd and Pb in different organs of the land snail Helix pomatia L. Comp. Biochem. Physiol. Part C Comp. Pharmacol. 79, 117–124. doi: 10.1016/0742-8413(84)90173-7
Dieterich, A., Troschinski, S., Schwarz, S., Di Lellis, M. A., Henneberg, A., Fischbach, U., et al. (2015). Hsp70 and lipid peroxide levels following heat stress in Xeropicta derbentina (Krynicki 1836) (Gastropoda, Pulmonata) with regard to different colour morphs. Cell Stress Chaperones 20, 159–168. doi: 10.1007/s12192-014-0534-3
Dittbrenner, N., Lazzara, R., Köhler, H.-R., Mazzia, C., Capowiez, Y., and Triebskorn, R. (2008). Heat tolerance in Mediterranean land snails: histopathology after exposure to different temperature regimes. J. Molluscan Stud. 75, 9–18. doi: 10.1093/mollus/eyn033
García-García, G., Reyes-Carrillo, G., Sarma, S., and Nandini, S. (2017). Population level responses of rotifers (Brachionus calyciflorus and Plationus patulus) to the anti-diabetic drug, metformin. J. Environ. Biol. 38, 1213–1219. doi: 10.22438/jeb/38/6(SI)/06
Garmendia, L., Soto, M., Vicario, U., Kim, Y., Cajaraville, M. P., and Marigómez, I. (2011). Application of a battery of biomarkers in mussel digestive gland to assess long-term effects of the Prestige oil spill in Galicia and Bay of Biscay: tissue-level biomarkers and histopathology. J. Environ. Monit. 13, 915–932. doi: 10.1039/c0em00410c
Glöer, P. (2002). Die Süßwassergastropoden Nord-und Mitteleuropas–Bestimmungsschlüssel, Lebensweise, Verbreitung. Die Tierwelt Deutschlands, 73. Teil. Hackenheim: ConchBooks.
Gong, L., Goswami, S., Giacomini, K. M., Altman, R. B., and Klein, T. E. (2012). Metformin pathways: pharmacokinetics and pharmacodynamics. Pharmacogenet. Genomics 22, 820–7. doi: 10.1097/FPC.0b013e3283559b22
Hamed, S. S., Abdelmeguied, N. E., Essawy, A. E., Radwan, M. A., and Hegazy, A. E. (2007). Histological and ultrastructural changes induced by two carbamate molluscicides on the digestive gland of Eobania vermiculata. J. Biol. Sci. 7, 1017–1037. doi: 10.3923/jbs.2007.1017.1037
Hermes-Lima, M., Willmore, W. G., and Storey, K. B. (1995). Quantification of lipid peroxidation in tissue extracts based on Fe (III) xylenol orange complex formation. Free Radical Biol. Med. 19, 271–280. doi: 10.1016/0891-5849(95)00020-X
Hundal, H., Ramlal, T., Reyes, R., Leiter, L., and Klip, A. (1992). Cellular mechanism of metformin action involves glucose transporter translocation from an intracellular pool to the plasma membrane in L6 muscle cells. Endocrinology 131, 1165–1173. doi: 10.1210/endo.131.3.1505458
Jacob, S., Dötsch, A., Knoll, S., Köhler, H.-R., Rogall, E., Stoll, D., et al. (2018). Does the antidiabetic drug metformin affect embryo development and the health of brown trout (Salmo trutta f. fario)? Environ. Sci. Eur. 30:48. doi: 10.1186/s12302-018-0179-4
Jeon, S.-M. (2016). Regulation and function of AMPK in physiology and diseases. Exp. Mol. Med. 48:e245. doi: 10.1038/emm.2016.81
Klobučar, G., Lajtner, J., and Erben, R. (1997). Lipid peroxidation and histopathological changes in the digestive gland of a freshwater snail Planorbarius corneus L. (Gastropoda, Pulmonata) exposed to chronic and sub-chronic concentrations of PCP. Bull. Environ. Contam. Toxicol. 58, 128–134. doi: 10.1007/s001289900310
Köhler, H. R., and Triebskorn, R. (2004). Stress im Boden: früherkennung ökotoxikologischer Effekte durch Biomarker. Biol. Unserer Zeit 34, 240–248. doi: 10.1002/biuz.200410256
Lord, J. M., Flight, I. H., and Norman, R. J. (2003). Metformin in polycystic ovary syndrome: systematic review and meta-analysis. BMJ 327:7951. doi: 10.1136/bmj.327.7421.951
Malin, S. K., and Kashyap, S. R. (2014). Effects of metformin on weight loss: potential mechanisms. Curr. Opin. Endocrinol. Diabetes 21, 323–329. doi: 10.1097/MED.0000000000000095
Margulis, B., Antropova, O., and Kharazova, A. (1989). 70 kDa heat shock proteins from mollusc and human cells have common structural and functional domains. Comp. Biochem. Physiol. Part B Comp. Biochem. 94, 621–623. doi: 10.1016/0305-0491(89)90138-7
Markiewicz, M., Jungnickel, C., Stolte, S., Białk-Bielinska, A., Kumirska, J., and Mrozik, W. (2017). Ultimate biodegradability and ecotoxicity of orally administered antidiabetic drugs. J. Hazard. Mater. 333, 154–161. doi: 10.1016/j.jhazmat.2017.03.030
Martin-Montalvo, A., Mercken, E. M., Mitchell, S. J., Palacios, H. H., Mote, P. L., Scheibye-Knudsen, M., et al. (2013). Metformin improves healthspan and lifespan in mice. Nat. Commun. 4:2192. doi: 10.1038/ncomms3192
Moermond, C. T., Kase, R., Korkaric, M., and Ågerstrand, M. (2016). CRED: Criteria for reporting and evaluating ecotoxicity data. Environ. Toxicol. Chem. 35, 1297–1309. doi: 10.1002/etc.3259
Monserrat, J. M., Geracitano, L., Pinho, G. L. L., Vinagre, T., Faleiros, M., Alciati, J. C., et al. (2003). Determination of lipid peroxides in invertebrates tissues using the Fe (III) xylenol orange complex formation. Arch. Environ. Contam. Toxicol. 45, 177–183. doi: 10.1007/s00244-003-0073-x
Natali, A., and Ferrannini, E. (2006). Effects of metformin and thiazolidinediones on suppression of hepatic glucose production and stimulation of glucose uptake in type 2 diabetes: a systematic review. Diabetologia 49, 434–441. doi: 10.1007/s00125-006-0141-7
Niemuth, N. J., Jordan, R., Crago, J., Blanksma, C., Johnson, R., and Klaper, R. D. (2015). Metformin exposure at environmentally relevant concentrations causes potential endocrine disruption in adult male fish. Environ. Toxicol. Chem. 34, 291–296. doi: 10.1002/etc.2793
Niemuth, N. J., and Klaper, R. D. (2015). Emerging wastewater contaminant metformin causes intersex and reduced fecundity in fish. Chemosphere 135, 38–45. doi: 10.1016/j.chemosphere.2015.03.060
Niemuth, N. J., and Klaper, R. D. (2018). Low-dose metformin exposure causes changes in expression of endocrine disruption-associated genes. Aquat. Toxicol. 195, 33–40. doi: 10.1016/j.aquatox.2017.12.003
Oosterhuis, M., Sacher, F., and ter Laak, T. L. (2013). Prediction of concentration levels of metformin and other high consumption pharmaceuticals in wastewater and regional surface water based on sales data. Sci. Total Environ. 442, 380–388. doi: 10.1016/j.scitotenv.2012.10.046
Osterauer, R., Köhler, H.-R., and Triebskorn, R. (2010). Histopathological alterations and induction of hsp70 in ramshorn snail (Marisa cornuarietis) and zebrafish (Danio rerio) embryos after exposure to PtCl2. Aquat. Toxicol. 99, 100–107. doi: 10.1016/j.aquatox.2010.04.001
Otludil, B., Cengiz, E. I., Yildirim, M. Z., Ünver, Ö., and Ünlü, E. (2004). The effects of endosulfan on the great ramshorn snail Planorbarius corneus (Gastropoda, Pulmonata): a histopathological study. Chemosphere 56, 707–716. doi: 10.1016/j.chemosphere.2004.04.027
Pentikäinen, P., Neuvonen, P., and Penttilä, A. (1979). Pharmacokinetics of metformin after intravenous and oral administration to man. Eur. J. Clin. Pharmacol. 16, 195–202. doi: 10.1007/BF00562061
Piro, S., Rabuazzo, A., Renis, M., and Purrello, F. (2012). Effects of metformin on oxidative stress, adenine nucleotides balance, and glucose-induced insulin release impaired by chronic free fatty acids exposure in rat pancreatic islets. J. Endocrinol. Invest. 35, 504–510. doi: 10.3275/7866
Roszer, T. (2014). The invertebrate midintestinal gland (“hepatopancreas”) is an evolutionary forerunner in the integration of immunity and metabolism. Cell Tissue Res. 358, 685–695. doi: 10.1007/s00441-014-1985-7
Sawasdee, B., Köhler, H.-R., and Triebskorn, R. (2011). Histopathological effects of copper and lithium in the ramshorn snail, Marisa cornuarietis (Gastropoda, Prosobranchia). Chemosphere 85, 1033–1039. doi: 10.1016/j.chemosphere.2011.07.030
Scheen, A. J. (1996). Clinical pharmacokinetics of metformin. Clin. Pharmacokinet. 30, 359–371. doi: 10.2165/00003088-199630050-00003
Scheurer, M., Michel, A., Brauch, H.-J., Ruck, W., and Sacher, F. (2012). Occurrence and fate of the antidiabetic drug metformin and its metabolite guanylurea in the environment and during drinking water treatment. Water Res. 46, 4790–4802. doi: 10.1016/j.watres.2012.06.019
Scheurer, M., Sacher, F., and Brauch, H.-J. (2009). Occurrence of the antidiabetic drug metformin in sewage and surface waters in Germany. J. Environ. Monit. 11, 1608–1613. doi: 10.1039/b909311g
Seifarth, C., Schehler, B., and Schneider, H. (2013). Effectiveness of metformin on weight loss in non-diabetic individuals with obesity. Exp. Clin. Endocrinol. 121, 27–31. doi: 10.1055/s-0032-1327734
Tessier, D., Maheux, P., Khalil, A., and Fülöp, T. (1999). Effects of gliclazide versus metformin on the clinical profile and lipid peroxidation markers in type 2 diabetes. Metabolism 48, 897–903. doi: 10.1016/S0026-0495(99)90226-3
Tisler, S., and Zwiener, C. (2018). Formation and occurrence of transformation products of metformin in wastewater and surface water. Sci. Total Environ. 628, 1121–1129. doi: 10.1016/j.scitotenv.2018.02.105
Tisler, S., and Zwiener, C. (2019). Aerobic and anaerobic formation and biodegradation of guanyl urea and other transformation products of metformin. Water Res. 149, 130–135. doi: 10.1016/j.watres.2018.11.001
TOCRIS (2017). Safety Data Sheet of Metformin Hydrochloride [Online]. Available online at: https://documents.tocris.com/pdfs/tocris_msds/2864_sds.pdf?1542620235 (Accessed January 09, 2019).
Triebskorn, R. (1989). Ultrastructural changes in the digestive tract of Deroceras reticulatum (Müller) induced by a carbamate molluscicides and by metaldehyde. Malacologia 31, 141–156.
Ussery, E. (2018). The Ecotoxicological Effects of Metformin and Its Metabolite, Guanylurea, on Japanese Medaka (Oryzias latipes). [dissertation]. [Ontario]: University of Ontario.
Valavanidis, A., Vlahogianni, T., Dassenakis, M., and Scoullos, M. (2006). Molecular biomarkers of oxidative stress in aquatic organisms in relation to toxic environmental pollutants. Ecotoxicol. Environ. Saf. 64, 178–189. doi: 10.1016/j.ecoenv.2005.03.013
Wiernsperger, N. F., and Bailey, C. J. (1999). The antihyperglycaemic effect of metformin. Drugs 58, 31–39. doi: 10.2165/00003495-199958001-00009
Zaldibar, B., Cancio, I., and Marigomez, I. (2007). Reversible alterations in epithelial cell turnover in digestive gland of winkles (Littorina littorea) exposed to cadmium and their implications for biomarker measurements. Aquat. Toxicol. 81, 183–196. doi: 10.1016/j.aquatox.2006.12.007
Keywords: gastropod, pharmaceutical, transformation product, histology, stress proteins, lipid peroxides
Citation: Jacob S, Köhler H-R, Tisler S, Zwiener C and Triebskorn R (2019) Impact of the Antidiabetic Drug Metformin and Its Transformation Product Guanylurea on the Health of the Big Ramshorn Snail (Planorbarius corneus). Front. Environ. Sci. 7:45. doi: 10.3389/fenvs.2019.00045
Received: 14 January 2019; Accepted: 20 March 2019;
Published: 09 April 2019.
Edited by:
Aziz Ullah, Kohat University of Science and Technology, PakistanReviewed by:
Joana Luísa Pereira, University of Aveiro, PortugalYehia ElTemsah, Cairo University, Egypt
Copyright © 2019 Jacob, Köhler, Tisler, Zwiener and Triebskorn. This is an open-access article distributed under the terms of the Creative Commons Attribution License (CC BY). The use, distribution or reproduction in other forums is permitted, provided the original author(s) and the copyright owner(s) are credited and that the original publication in this journal is cited, in accordance with accepted academic practice. No use, distribution or reproduction is permitted which does not comply with these terms.
*Correspondence: Stefanie Jacob, c3RlZmFuaWUuamFjb2JAdW5pLXR1ZWJpbmdlbi5kZQ==