- School of Natural Resources and The Environment, University of Arizona, Tucson, AZ, United States
Rock pools are important desert ecosystems that provide rare sources of surface water in arid regions. Hydroperiod is one of the primary limiting factors on aquatic macroinvertebrates living in rock pools. Resident macroinvertebrates must complete their life cycles before drying, and may employ active or passive dispersal strategies to survive drying. Quantifying dispersal and functional feeding traits across rock pool macroinvertebrate communities in multiple regions could provide insight into how rock pool ecosystems will respond to shorter hydroperiods predicted by climate change models. Here, we analyze taxonomic data (26 species lists) obtained from 24 published studies of rock pools to assess the dispersal and feeding strategies of macroinvertebrates, and how ecosystem functions may change with shorter hydroperiods. On average across all datasets, taxa were equally comprised of active dispersers and passive dispersers. Most active disperser taxa were predators (60%) and gatherers (33%). In contrast, passive disperser taxa were generally filterers (39%), gatherers (29%), and scrapers (21%). Climate change scenarios may result in shorter rock pool hydroperiods in many regions, which could reduce habitat availability for passive dispersers with weak overland dispersal abilities. If passive disperser populations decrease, their associated ecosystem functions, such as fine organic matter processing, could be disrupted. These results provide a foundation for future work investigating changes in rock pool ecosystem function due to altered hydroperiods.
Introduction
Rock pools are small (<1 hectare area), often temporary, bodies of water located in depressions on rock outcrops. Commonly found in arid regions, these pools fill with rain and slowly evaporate over time, making them important habitat “islands” for aquatic macroinvertebrates in a harsh, dry landscape (Jocqué et al., 2010b). Rock pools are often regarded as hotspots of biodiversity and endemism due to their unique biotas (Bogan et al., 2014; McBurnie et al., 2015; Porembski et al., 2016). Macroinvertebrate communities within rock pools are tightly controlled by hydroperiod (Table 1), the duration of inundation from filling to drying. Hydroperiod itself is a function of pool size and geometry, precipitation, runoff, climate variability, and evaporation rates (Hulsmans et al., 2008; Vanschoenwinkel et al., 2009b).
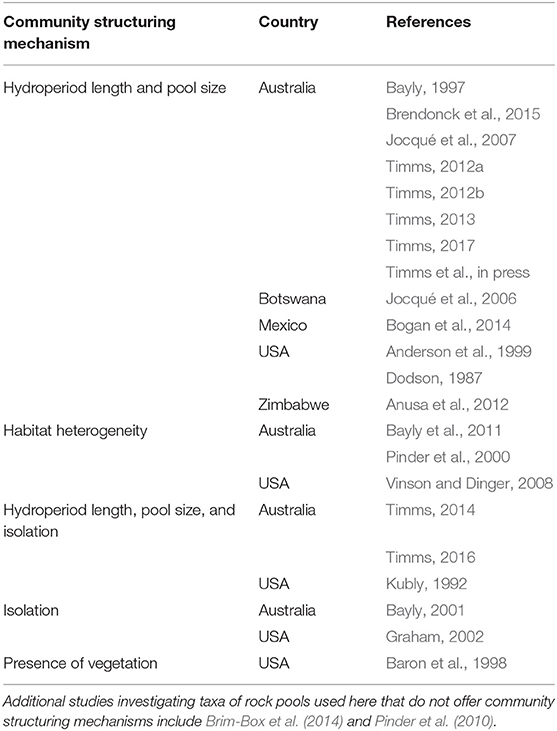
Table 1. Rock pool studies investigating controls on taxonomic composition, sorted by the primary community structuring mechanisms identified by the authors.
To survive in temporary water bodies, resident species have to either persist through drying events or disperse away from drying habitats. Jocqué et al. (2010b) categorized rock pool taxa into two major mobility groups: active dispersers and passive dispersers. Active dispersers are less restricted by hydroperiod because they fly or crawl overland from pool to pool to escape drying (Jocqué et al., 2010b). These macroinvertebrates can colonize rock pools almost immediately upon inundation. Depending on expected pool permanence, which is partly cued by habitat size, active dispersers lay eggs in newly-filled pools to start the next generation, which must fully develop before the pool dries (Jocqué et al., 2010b). When they reach their adult stage, these macroinvertebrates fly to more permanent water sources to wait until rock pools fill again. Active dispersers are commonly insect taxa, such as hemipterans, coleopterans, and dipterans, and are likely habitat generalists due to their ability to travel to other water bodies (Jocqué et al., 2010b). Many of these same insect taxa are also predators (e.g., hemipterans) that need to consume high-protein prey to reach adulthood and become aerial dispersers before pools dry (Jocqué et al., 2010b; O'Neill and Thorp, 2014; Brendonck et al., 2016; Lund et al., 2016).
Conversely, passive dispersers employ strategies that allow them to survive drying in situ. These organisms are largely branchiopod crustaceans, such as anostracans, notostracans, and cladocerans, and other crustaceans such as ostracods (Jocqué et al., 2010b). When rock pools begin to dry, these crustaceans lay eggs that remain viable in dry sediment for years. When pools refill, conductivity, and temperature cue the eggs to break dormancy, and crustaceans continue their life cycles (Calabrese et al., 2016). Occasionally, wind disperses dormant eggs to other rock depressions, inadvertently maintaining metacommunities (Vanschoenwinkel et al., 2009a). Given these life history traits, rock pool crustaceans are considered specialists for temporary habitats, and are rarely found in permanent systems (Jocqué et al., 2007). These same crustaceans frequently exhibit herbivorous functional feeding traits (Jocqué et al., 2010a,b; Brendonck et al., 2016). These feeding traits allow passive dispersers to take advantage of newly deposited organic matter and spikes in microbial production when pools refill (Jocqué et al., 2010a).
Climate change is expected to alter aquatic communities in rock pools via its effect on hydroperiod. Climate change models for many arid regions predict fewer rainy days, decreased mountain snowpack, longer dry seasons, and increased temperatures (Tadross et al., 2005; Abatzoglou and Kolden, 2011; Hughes and Steffen, 2013; Jaeger et al., 2014; Meixner et al., 2016). These climatic changes are also expected to reduce hydrologic connectivity and groundwater recharge (Jaeger et al., 2014; Meixner et al., 2016). Longer dry seasons will likely result in shorter hydroperiods for rock pools through a combination of reduced filling and faster drying. In fact, a hydrologic model predicted shorter hydroperiods for 85% of rock pools in Botswana, as well as decreased hydroperiod predictability (Hulsmans et al., 2008). Models also suggest shorter hydroperiods may impede the reproduction and survival of passively-dispersing crustaceans in smaller, shallower pools (Hulsmans et al., 2008). Specifically, reduced hydroperiods in small pools may alter water chemistry dynamics and eliminate the temperature or conductivity cues that trigger dormant eggs to hatch (Spencer and Blaustein, 2001; Jocqué et al., 2010b). Furthermore, hydroperiods may become too short for passive dispersers to complete their life cycles before drying (Hulsmans et al., 2008; Timms et al., in press).
The objectives of this review were to (i) assess the macroinvertebrate dispersal and functional feeding traits of rock pools across arid regions globally and (ii) to explore how macroinvertebrate communities and the ecosystem functions they provide could shift under climate change. We hypothesized that rock pool taxa are dominated by active dispersers, because aerial dispersal allows individuals to colonize new habitat and escape drying habitat. We also hypothesized that overall functional feeding group composition would be dominated by predators (given their previously documented association with active dispersal traits), but that herbivorous traits would dominate within the passive dispersers group. If the latter hypothesis is supported, then the shorter hydroperiods predicted under climate change could negatively impact ecosystem processes in rock pools by eliminating populations of herbivorous passive dispersers.
Analyses
Taxa lists provided in studies of rock pool communities were reviewed to understand the distribution of taxa, and their dispersal and functional feeding traits, across and within arid regions. Data were collected through Google Scholar and Web of Science searches for the terms “rock pool,” “pool,” “tinaja,” “gnamma,” “macroinvertebrate,” “community,” “arid,” “desert,” “freshwater,” “ephemeral,” and “temporary.” Studies containing macroinvertebrate community data for freshwater, non-riverine rock pools were selected, and taxa lists were analyzed. In cases where taxa lists were not provided, the authors were contacted to request taxa lists. Our analyses include 26 taxa lists from 24 studies worldwide (see Table 1 for citations).
Taxa were assigned into passive and active disperser groups based on their dispersal abilities. Passive dispersers included Crustacea, Turbellaria, Rotifera, Tardigrada, Nematoda, Oligochaeta, Mollusca, and Orobatidae, while active dispersers included most aquatic insects and non-Orobatid mites (Williams, 1998; Jocqué et al., 2010b; Brendonck et al., 2016). However, insect taxa were classified as passive if they have dormant egg or larval stages that can survive drying (e.g., Dasyhelea, Diptera: Ceratopogonidae). For example, genera of non-biting midge (Diptera: Chironomidae) were classified as passive if they were included in a published list of drought-resistant taxa (Cañedo-Argüelles et al., 2016) or if they were known to be endemic to rock pools (Pinder et al., 2000).
Taxa were further assigned to one of the following functional feeding groups: predators/parasites (hereafter predators), gatherers, shredders, scrapers, filterers (Kubly, 1992; Ode, 2003; Merritt et al., 2008; DraŽina et al., 2014; Ramírez and Gutiérrez-Fonseca, 2014; Centre for Freshwater Ecosystems, 2019). This metric is coarse for two reasons. First, some studies only identified taxa to family, so we used coarser family-level feeding group assignments. Second, most studies did not provide the life stage or the proportion of larvae to adults for Coleoptera, which may change functional feeding groups. In these cases, we assigned coleopterans to adult-stage feeding groups unless the presence of larvae was noted. If larvae and adults were distinguished, then larvae were assigned to different functional feeding groups when appropriate (e.g., Hydrophilidae: Berosus larvae- predators, adults- gatherers).
For each dataset, the percentages of taxa classified as passive dispersers, active dispersers, predators, and herbivore functional feeding groups (scraper, shredder, gatherer, and filterer) were calculated. The number of taxa in each group was divided by the total number of taxa in the list to calculate the percentages. Percentages of each group were then compared to identify patterns in trait dominance. The comparisons calculated were: (i) average percent active and passive dispersers for the taxa in each study, (ii) percent of each functional feeding group overall, (iii) percent of each functional feeding group represented within actively dispersing taxa, and (iv) percent of each functional feeding group represented within passively dispersing taxa. Differences between percentages of active and passive disperser taxa were tested through analysis of variance (ANOVAs), and differences in percentages of each functional feeding group were tested through Kruskal-Wallis tests and post-hoc paired Wilcoxon tests. Test types were based on the distribution of data, which could not always able to be normalized. All statistics were run in the R computing program (R Core Team, 2017). To explore continental differences, each test was also run for the subsets of data from North American and Australian studies. There were not enough studies from Africa to run separate statistical analyses.
Taxa lists were utilized in this study because they were readily available in published journal articles or in the Supplementary Materials of articles. However, these lists lack abundance measurements. By not accounting for the abundances of each taxon, we are likely overestimating the contributions of rare species and underrepresenting those of dominant species. Abundance data were unavailable because full datasets were not accessible, and authors were reluctant to share raw data. Despite these shortcomings, taxa lists provide a coarse examination of the entire community and allow the development of testable hypotheses for future studies.
Taxonomic Structure
We gathered macroinvertebrate community data from 24 rock pool studies in arid regions on three continents. On average, there was no difference between percentages of active and passive disperser taxa in rock pools (ANOVA (n = 26): F1,50 = 0.60, p = 0.444). Active dispersers composed an average of 52% of listed taxa for rock pools, while passive dispersers contributed 48% (Figure 1i). Not all studies included microfauna, such as cladocerans, copepods and rotifers, but those that did reported numerous taxa, which may account for the similarity in percentages between active and passive taxa. This similarity may also result from lack of separation between pools of different sizes during analyses, as small and large pools have been shown to support distinct macroinvertebrate taxa (Anderson et al., 1999; Anusa et al., 2012; Timms, 2013, 2016). Refining the resolution of data by pool type and by abundance measurements would aid in refining analyses of dispersal trait structure.
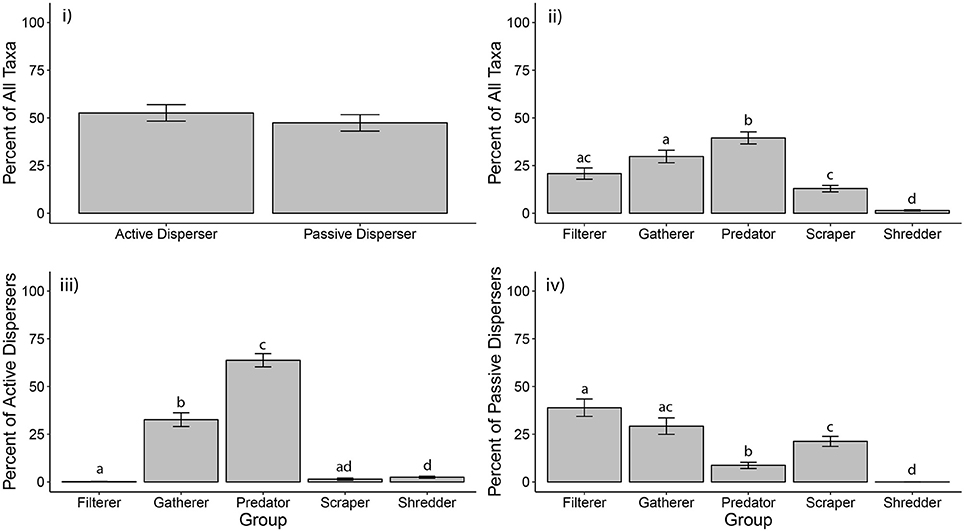
Figure 1. Composition of macroinvertebrate dispersal and functional feeding traits in rock pools. Globally, the percentages of taxa classified as active vs. passive disperser were equal (i), but more taxa were classified as predators and gatherers than any other functional feeding group (ii). Within active dispersing taxa, most taxa were predators and gatherers (iii), but the majority of passive dispersing were filterers, gatherers, and scrapers (iv). Letters above bars indicate groups by significance, such that two bars with the same letter are not statistically different. Letters can only be compared within a graph, not across graphs.
In contrast to these equivocal global patterns, studies from Australian rock pools had significantly more passive than active dispersers [p ANOVA (n = 15): F1,28 = 7.85, p = 0.009], while studies from North America had significantly more active than passive dispersing taxa [ANOVA (n = 9): F1,16 = 37.87, p <0.001; Figure S1]. These opposing patterns are likely due to varying levels of taxonomic resolution between continents. Australian studies usually identified microfauna to species, whereas North American studies generally identified to order. Thus, the number of passive dispersing microfauna reported from Australian studies was usually higher than in North American studies. This finding highlights the need for taxonomic consistency across studies to make robust regional comparisons.
Globally, the proportions of taxa belonging to various functional feeding groups were not equal [K-W (n = 26): χ2 = 77.49, df = 4, p <0.001]. On average, the highest percentage of taxa were predators, followed by gatherers, filterers, scrapers, and shredders (Figure 1ii). Functional feeding group composition within continents was similar to the global pattern (Figure S2). Within herbivorous functional feeding groups, a majority of taxa fed on fine particulate organic matter (FPOM). FPOM is washed in during rain events (Crawford, 1981) and also is produced autochthonously upon the death of algae (Kubly, 1990). Coarse particulate organic matter (CPOM), and the macroinvertebrate shredders that feed on it, may be rare in rock pools because organic matter is flushed away during heavy rain events (Kubly, 1990; Griffis-Kyle et al., 2014).
Similar to overall patterns, functional feeding groups within active disperser taxa also differed in the proportion of taxa they contained (K-W (n = 26): χ2 = 101.91, df = 4, p <0.001). Predators and gatherers dominated, with filterers, scrapers, and shredders being relatively uncommon among active disperser taxa (Figure 1iii). In fact, on average, over 60% of active dispersers were predators, while <10% of passive dispersers were predators. Analyses within Australia and North America largely mirrored global patterns (Figure S3). Examples of active disperser predators included hemipterans, such as notonectids and corixids, as well as coleopterans (e.g., Dytiscidae) and odonates (e.g., Aeshnidae). Gatherers within the active disperser taxa were mainly dipterans, such as culicids and chironomids, but also included adult hydrophilids and an occasional ephemeropteran (e.g., Baetidae).
Functional feeding group percentages within passive dispersers were also unequal [K-W (n = 26): χ2 = 70.26, df = 4, p <0.001]. Filterers, gatherers, and scrapers dominated, while predators were rare, and not statistically different from shredders, which were completely absent (Figure 1iv). Regional patterns were similar to global patterns (Figure S4). Filter-feeding passive dispersers included ostracods, copepods, and various crustaceans, while gatherers were mainly Rotifera and desiccation-resistant chironomids (Diptera).
Community Dynamics and Ecosystem Function in Rock Pools
Although little research has been done to quantify ecosystem functions within rock pools, analyzing rock pool taxa by functional feeding groups can lend insight to the ecosystem functions these taxa provide (Wallace and Webster, 1996). Predators were the dominant functional feeding group overall, and were especially dominant within active disperser taxa. Macroinvertebrate predators are known to structure local communities through prey depletion (Wallace and Webster, 1996; Downing, 2005). In rock pools, the influence of predators on prey assemblages may be heightened because prey emigration is limited (i.e., leaving the pool may lead to desiccation or terrestrial predation). By reducing prey abundance, predators influence the functions that prey perform, such as grazing and organic matter processing. Cascading impacts of predators on prey can affect ecosystem functions, such as primary productivity and carbon cycling (Downing and Leibold, 2002; Atwood et al., 2013, 2014a). However, different predators, or different combinations of predators, do not affect prey and ecosystem function uniformly (Atwood et al., 2014b). Overall, functional outcomes resulting from increased predator abundances in rock pools are difficult to predict given the high abundances and diversity of predators, suggesting the need for manipulative experimental studies to disentangle this complexity.
Gatherers and filterers were the most dominant herbivore groups in rock pools, and these functional feeding traits were strongly associated with passively dispersing taxa. Collector-gatherers are known for their roles in detritus processing and nitrogen cycling, and when they are consumed by aquatic or terrestrial predators, they link basal and upper levels in food webs (Grimm, 1988). As gatherers seek food, they disturb sediments and particulate matter (bioturbation), resulting in re-suspension and movement of food and nutrients throughout the pool (Wallace and Webster, 1996; Brendonck et al., 2016). This process is important to filter-feeding taxa, which consume FPOM from the water column. Filtering can change nutrient cycling through removal of organic matter from suspension and the transformation of nutrients through filter-feeder excretion, which in turn affects primary production (Wallace and Webster, 1996; Cardinale and Palmer, 2002). Because passive dispersers are frequently gatherers and filterers, future climate regimes and hydroperiods that are unfavorable to passive dispersers could greatly reduce the ecosystem functions they provide.
Broader Implications
Hydroperiod and predation likely will interact to assemble novel communities under climate change. With decreasing hydroperiods in shallow rock pools, inundation time may be so short that passive dispersers lack time for reproduction (Hulsmans et al., 2008; Timms et al., in press). Moreover, crustaceans cannot actively seek longer-lasting pools in which to lay their eggs (Jocqué et al., 2010b). If smaller pools dry quickly, FPOM processing and nutrient cycling could be disrupted through the loss of specialist crustaceans. In addition to hydroperiod disrupting passive disperser life cycles, increased prevalence of active dispersing predators may further modify local communities. In fact, predation may be nearly as strong of a community filter as hydroperiod (Jocqué et al., 2010b), because predators have the capacity to shape the primary consumer communities through prey selection (Dodson, 1987; Kubly, 1992; Blaustein et al., 1995; Anderson et al., 1999; Jocqué et al., 2007; Waterkeyn et al., 2016).
Although smaller rock pools will almost certainly face reduced hydroperiods under climate change, the presence of larger rock pools, which are generally slower to dry, may help to reduce local extinction rates of rock pool taxa. Larger rock pools have a wider variety of microhabitats and relatively stable physiochemical conditions, and thus tend to support a higher diversity of macroinvertebrates (Bayly, 1997; Vanschoenwinkel et al., 2009b; Anusa et al., 2012; Bogan et al., 2014). However, the hydroperiods of larger rock pools can be difficult to model due to high variability in the extent of inundation resulting from varying levels of rainfall (Vanschoenwinkel et al., 2009b; Cross et al., 2015). Thus, it is unclear whether these habitats will serve as long-term refugia under a drier or warmer climate. Furthermore, taxa associated with smaller rock pools may not be able to move to larger rock pools as climate changes, or even coexist with the taxa that usually inhabit larger rock pools. More permanent (often larger) pools tend to be dominated by active dispersers (Dodson, 1987; Anderson et al., 1999; Timms, 2013, 2014, 2016, 2017), the majority of which are predators (Figure 1). Many passive dispersers have evolved to live in shallow pools to escape predation (Jocqué et al., 2007), and these taxa may be unable to coexist with predators in deeper pools. Moreover, passive dispersers rely on seasonal or hydroperiod-dependent water chemistry cues to time their life cycles (Spencer and Blaustein, 2001; Jocqué et al., 2010b). In larger and more physiochemically-stable pools, passively dispersing taxa may not receive appropriate cues to trigger development.
As Vanschoenwinkel et al. (2009b) found, small rock pools are important—they may contain specialized taxa, and they may function as travel refuges between larger pools. Increasing regional habitat homogeneity, such as that resulting from altered hydroperiods and subsequent loss of smaller pools, may result in both reduced regional biodiversity (Kassen, 2002) and functional homogenization of remaining communities (Gámez-Virués et al., 2015). Thus, as climate change alters drying regimes, the likely outcome is reduced diversity within rock pool metacommunities and decreased ecosystem functionality in terms of organic matter processing and nutrient cycling. Because rock pools are small enough to experimentally modify, future studies should directly test these hypothesized outcomes by manipulating hydroperiod and the abundances of different taxa, and measuring the resulting impacts on ecosystem function.
Author Contributions
SW conceptualized paper, compiled and reviewed data, wrote manuscript, created figure and table. MB assisted in conceptualization, manuscript writing, table creation, and contributed conceptual knowledge to the paper.
Conflict of Interest Statement
The authors declare that the research was conducted in the absence of any commercial or financial relationships that could be construed as a potential conflict of interest.
Supplementary Material
The Supplementary Material for this article can be found online at: https://www.frontiersin.org/articles/10.3389/fenvs.2019.00106/full#supplementary-material
References
Abatzoglou, J. T., and Kolden, C. A. (2011). Climate change in Western US deserts: potential for increased wildfire and invasive annual grasses. Rangel. Ecol. Manag. 64, 471–478. doi: 10.2111/REM-D-09-00151.1
Anderson, C. R., Peckarsky, B. L., and Wissinger, S. A. (1999). “Tinajas of southeastern Utah: invertebrate reproductive strategies and the habitat templet,” in Invertebrates in Freshwater Wetlands of North America: Ecology and Management, eds D. P. Batzer, R. B. Rader, and S. A. Wissinger (New York, NY: Wiley, 791–810.
Anusa, A., Ndagurwa, H. G. T., and Magadza, C. H. D. (2012). The influence of pool size on species diversity and water chemistry in temporary rock pools on Domboshawa Mountain, northern Zimbabwe. African J. Aquat. Sci. 37, 89–99. doi: 10.2989/16085914.2012.666378
Atwood, T. B., Hammill, E., Greig, H. S., Kratina, P., Shurin, J. B., Srivastava, D. S., et al. (2013). Predator-induced reduction of freshwater carbon dioxide emissions. Nat. Geosci. 6, 191–194. doi: 10.1038/ngeo1734
Atwood, T. B., Hammill, E., and Richardson, J. S. (2014a). Trophic-level dependent effects on CO2 emissions from experimental stream ecosystems. Glob. Chang. Biol. 20, 3386–3396. doi: 10.1111/gcb.12516
Atwood, T. B., Hammill, E., Srivastava, D. S., and Richardson, J. S. (2014b). Competitive displacement alters top-down effects on carbon dioxide concentrations in a freshwater ecosystem. Oecologia 175, 353–361. doi: 10.1007/s00442-013-2877-3
Baron, J. S., Lafrancois, T., and Kondratieff, B. C. (1998). Chemical and biological characteristics of desert rock pools in intermittent streams of Capitol Reef National Park, Utah. Gt. Basin Nat. 58, 250–264.
Bayly, I. A. E. (1997). Invertebrates of temporary waters in gnammas on granite outcrops in Western Australia. J. R. Soc. West. Aust. 80, 167–172.
Bayly, I. A. E. (2001). Invertebrate occurrence and succession after episodic flooding of a Central Australian rock-hole. J. R. Soc. West. Aust. 84, 29–32.
Bayly, I. A. E., Halse, S. A., and Timms, B. V. (2011). Aquatic invertebrates of rockholes in the south-east of Western Australia. J. R. Soc. West. Aust. 94, 549–555.
Blaustein, L., Kotler, B. P., and Ward, D. (1995). Direct and indirect effects of a predatory backswimmer (Notonecta maculata) on community structure of desert temporary pools. Ecol. Entomol. 20, 311–318. doi: 10.1111/j.1365-2311.1995.tb00462.x
Bogan, M. T., Noriega-Felix, N., Vidal-Aguilar, S. L., Findley, L. T., Lytle, D. A., Gutiérrez-Ruacho, O. G., et al. (2014). Biogeography and conservation of aquatic fauna in spring-fed tropical canyons of the southern Sonoran Desert, Mexico. Biodivers. Conserv. 23, 2705–2748. doi: 10.1007/s10531-014-0745-z
Brendonck, L., Jocqué, M., Tuytens, K., Timms, B. V., and Vanschoenwinkel, B. (2015). Hydrological stability drives both local and regional diversity patterns in rock pool metacommunities. Oikos 124, 741–749. doi: 10.1111/oik.01710
Brendonck, L., Lanfranco, S., Timms, B. V., and Vanschoenwinkel, B. (2016). “Invertebrates in Rock Pools,” in Invertebrates in Freshwater Wetlands, eds D. Batzer and D. Boix (Cham: Springer International Publishing), 1–642.
Brim-Box, J., Davis, J., Strehlow, K., McBurnie, G., Duguid, A., Brock, C., et al. (2014). Persistence of central Australian aquatic invertebrate communities. Mar. Freshw. Res. 65, 562–572. doi: 10.1071/MF13131
Calabrese, A., McCullough, C., Knott, B., and Weeks, S. C. (2016). Environmental characteristics of ephemeral rock pools explain local abundances of the clam shrimp. J. R. Soc. Victoria 99, 9–15.
Cañedo-Argüelles, M., Bogan, M. T., Lytle, D. A., and Prat, N. (2016). Are Chironomidae (Diptera) good indicators of water scarcity? Dryland streams as a case study. Ecol. Indic. 71, 155–162. doi: 10.1016/j.ecolind.2016.07.002
Cardinale, B. J., and Palmer, M. A. (2002). Disturbance moderates biodiversity-ecosystem function relationships: experimental evidence from caddisflies in stream mesocosms. Ecology 83, 1915–1927. doi: 10.1890/0012-9658(2002)083[1915:DMBEFR]2.0.CO;2
Centre for Freshwater Ecosystems (2019). Identification and Ecology of Australian Freshwater Invertebrates. Available online at: https://www.mdfrc.org.au/bugguide/index.htm (accessed June 5, 2019).
Crawford, C. S. (1981). “The invertebrate community of ephemeral waters,” in Biology of Desert Invertebrates, ed C. S. Crawford (Berlin: Springer-Verlag, 234–247.
Cross, A. T., Turner, S. R., Merritt, D. J., Van Niekerk, A., Renton, M., Dixon, K. W., et al. (2015). Vegetation patterns and hydro-geological drivers of freshwater rock pool communities in the monsoon-tropical Kimberley region, Western Australia. J. Veg. Sci. 26, 1184–1197. doi: 10.1111/jvs.12318
Dodson, S. I. (1987). Animal assemblages in temporary desert rock pools: Aspects of the ecology of Dasyhelea sublettei (Diptera: Ceratopogonidae). J. North Am. Benthol. Soc. 6, 65–71. doi: 10.2307/1467525
Downing, A. L. (2005). Relative effects of species composition and richness on ecosystem properties in ponds. Ecology 86, 701–715. doi: 10.1890/03-0239
Downing, A. L., and Leibold, M. A. (2002). Ecosystem consequences of species richness and composition in pond food webs. Nature 416, 837–841. doi: 10.1038/416837a
DraŽina, T., Špoljar, M., Primc, B., and Habdija, I. (2014). Nematode feeding types in a tufa-depositing environment (Plitvice Lakes, Croatia). Nat. Croat. 23, 89–99.
Gámez-Virués, S., Perović, D. J., Gossner, M. M., Börschig, C., Blüthgen, N., De Jong, H., et al. (2015). Landscape simplification filters species traits and drives biotic homogenization. Nat. Commun. 6:8568. doi: 10.1038/ncomms9568
Graham, T. B. (2002). Survey of aquatic macroinvertebrates and amphibians at Wupatki National Monument, Arizona, USA: an evaluation of selected factors affecting species richness in ephemeral pools. Hydrobiologia 486, 215–224. doi: 10.1023/A:1021311120799
Griffis-Kyle, K. L., Kovatch, J. J., and Bradatan, C. (2014). Water quality: a hidden danger in anthropogenic desert catchments. Wildl. Soc. Bull. 38, 148–151. doi: 10.1002/wsb.358
Grimm, N. B. (1988). Role of macroinvertebrates in nitrogen dynamics of a desert stream. Ecology 69, 1884–1893. doi: 10.2307/1941165
Hughes, L., and Steffen, W. (2013). Climate change in Victoria: trends, predictions and impacts. Proc. R. Soc. Victoria 125, 5–13. doi: 10.1071/RS13003
Hulsmans, A., Vanschoenwinkel, B., Pyke, C., Riddoch, B. J., and Brendonck, L. (2008). Quantifying the hydroregime of a temporary pool habitat: a modelling approach for ephemeral rock pools in SE Botswana. Ecosystems 11, 89–100. doi: 10.1007/s10021-007-9110-3
Jaeger, K. L., Olden, J. D., and Pelland, N. A. (2014). Climate change poised to threaten hydrologic connectivity and endemic fishes in dryland streams. Proc. Natl. Acad. Sci. U.S.A. 111, 13894–13899. doi: 10.1073/pnas.1320890111
Jocqué, M., Martens, K., Riddoch, B. J., and Brendonck, L. (2006). Faunistics of ephemeral rock pools in southeastern Botswana. Arch. für Hydrobiol. 165, 415–431. doi: 10.1127/0003-9136/2006/0165-0415
Jocqué, M., Timms, B. V., and Brendonck, L. (2007). A contribution on the biodiversity and conservation of the freshwater fauna of rocky outcrops in the central Wheatbelt of Western Australia. J. R. Soc. West. Aust. 90, 137–142.
Jocqué, M., Vanschoenwinkel, B., and Brendonck, L. (2010a). Anostracan monopolisation of early successional phases in temporary waters? Fundam. Appl. Limnol. 176, 127–132. doi: 10.1127/1863-9135/2010/0176-0127
Jocqué, M., Vanschoenwinkel, B., and Brendonck, L. (2010b). Freshwater rock pools: a review of habitat characteristics, faunal diversity and conservation value. Freshw. Biol. 55, 1587–1602. doi: 10.1111/j.1365-2427.2010.02402.x
Kassen, R. (2002). The experimental evolution of spetialists, generalist, and the maintenance of diversity. J. Evol. Biol. 15, 173–190. doi: 10.1046/j.1420-9101.2002.00377.x
Kubly, D. M. (1990). “Limnological features of desert mountain rock pools,” in Wildlife Water Development: A proceedings of the Wildlife Water Development Symposium, eds G. K. Tsukamoto and S. J. Stiver (Las Vegas, NV: U.S. Bureau of Land Management, The Wildlife Society Nevada Chapter, and the Nevada Department of Wildlife, 103–120.
Kubly, D. M. (1992). Aquatic invertebrates in desert mountain rock pools: the White Tank Mountains, Maricopa County, Arizona. J. Arizona-Nevada Acad. Sci. 26, 55–69.
Lund, J. O., Wissinger, S. A., and Peckarsky, B. L. (2016). Caddisfly behavioral responses to drying cues in temporary ponds: implications for effects of climate change. Freshw. Sci. 35, 619–630. doi: 10.1086/685583
McBurnie, G., Davis, J., Thompson, R. M., Nano, C., and Brim-Box, J. (2015). The impacts of an invasive herbivore (Camelus dromedaries) on arid zone freshwater pools: an experimental investigation of the effects of dung on macroinvertebrate colonisation. J. Arid Environ. 113, 69–76. doi: 10.1016/j.jaridenv.2014.09.011
Meixner, T., Manning, A. H., Stonestrom, D. A., Allen, D. M., Ajami, H., Blasch, K. W., et al. (2016). Implications of projected climate change for groundwater recharge in the western United States. J. Hydrol. 534, 124–138. doi: 10.1016/j.jhydrol.2015.12.027
Merritt, R. W., Cummins, K. W., and Berg, M. B. (2008). An Introduction to the Aquatic Insects of North America, 4th Edn. Dubuque, IA: Kendall Hunt Publishing.
Ode, P. (2003). List of Californian Macroinvertebrate Taxa and Standard Taxonomic Effort. Rancho Cordova, CA: California Department of Fish and Game.
O'Neill, B. J., and Thorp, J. H. (2014). Untangling food-web structure in an ephemeral ecosystem. Freshw. Biol. 59, 1462–1473. doi: 10.1111/fwb.12358
Pinder, A. M., Halse, S. A., Shiel, R. J., and McRae, J. M. (2000). Granite outcrop pools in south-western Australia: Foci of diversification and refugia for aquatic invertebrates. J. R. Soc. West. Aust. 83, 149–161.
Pinder, A. M., Halse, S. A., Shiel, R. J., and Mcrae, J. M. (2010). An arid zone awash with diversity: patterns in the distribution of aquatic invertebrates in the Pilbara region of Western Australia. Rec. West. Aust. Museum 78, 205–246. doi: 10.18195/issn.0313-122x.78(1).2010.205-246
Porembski, S., Silveira, F. A. O., Fiedler, P. L., Watve, A., Rabarimanarivo, M., Kouame, F., et al. (2016). Worldwide destruction of inselbergs and related rock outcrops threatens a unique ecosystem. Biodivers. Conserv. 25, 2827–2830. doi: 10.1007/s10531-016-1171-1
R Core Team (2017). R: A Language and Environment for Statistical Computing. Available online at: https://www.r-project.org/ (accessed June 5, 2019).
Ramírez, A., and Gutiérrez-Fonseca, P. E. (2014). Functional feeding groups of aquatic insect families in Latin America: a critical analysis and review of existing literature. Rev. Biol. Trop. 62, 155–167. doi: 10.15517/rbt.v62i0.15785
Spencer, M., and Blaustein, L. (2001). Hatching responses of temporary pool invertebrates to signals of environmental quality. Isr. J. Zool. 47, 397–417. doi: 10.1560/23F2-2XBW-252B-DU5C
Tadross, M., Jack, C., and Hewitson, B. (2005). On RCM-based projections of change in southern African summer climate. Geophys. Res. Lett. 32, 1–4. doi: 10.1029/2005GL024460
Timms, B. V. (2012a). Influence of climatic gradients on metacommunities of aquatic invertebrates on granite outcrops in southern Western Australia. J. R. Soc. West. Aust. 95, 125–135.
Timms, B. V. (2012b). Seasonal study of aquatic invertebrates in five sets of latitudinally separated gnammas in southern Western Australia. J. R. Soc. West. Aust. 95, 13–28.
Timms, B. V. (2013). Aquatic invertebrates of pit gnammas in southwest Australia. J. R. Soc. West. Aust. 96, 55–67.
Timms, B. V. (2014). Community ecology of aquatic invertebrates in gnammas (rock-holes) of north-western Eyre Peninsula, South Australia. Trans. R. Soc. South Aust. 138, 147–160. doi: 10.1080/03721426.2014.11649006
Timms, B. V. (2016). A study on the pools of a granitic mountain top at Moonbi, New South Wales. Proc. Linn. Soc. New South Wales 138, 61–68.
Timms, B. V. (2017). A study of the gnammas (rock pools) in some granitic outcrops in central Victoria, with a comparison of their invertebrate communities across southern Australia. J. R. Soc. Victoria 129, 21–36. doi: 10.1071/RS17002
Timms, B. V., Booth, C., Newman, M., and McCann, J. (in press). The ecology of gnammas (weathering pits) on the Stanthorpe Plateau, Northern New England Tablelands, with special reference to the clam shrimp Paralimnadia urukhai (Webb Bell) (Crustacea: Branchiopoda: Spinicaudata). Proc. R. Soc. Queensl.
Vanschoenwinkel, B., Gielen, S., Seaman, M., and Brendonck, L. (2009a). Wind mediated dispersal of freshwater invertebrates in a rock pool metacommunity: differences in dispersal capacities and modes. Hydrobiologia 635, 363–372. doi: 10.1007/s10750-009-9929-z
Vanschoenwinkel, B., Hulsmans, A., De Roeck, E., De Vries, C., Seaman, M., and Brendonck, L. (2009b). Community structure in temporary freshwater pools: Disentangling the effects of habitat size and hydroregime. Freshw. Biol. 54, 1487–1500. doi: 10.1111/j.1365-2427.2009.02198.x
Vinson, M. R., and Dinger, E. C. (2008). Aquatic invertebrates of the Grand Staircase-Escalante National Monument, Utah. Southwest. Nat. 53, 374–384. doi: 10.1894/JS-20.1
Wallace, J. B., and Webster, J. R. (1996). The role of macroinvertebrates in stream ecosystem function. Annu. Rev. Entomol. 41, 115–139. doi: 10.1146/annurev.en.41.010196.000555
Waterkeyn, A., Grillas, P., and Brendonck, L. (2016). Experimental test of the ecosystem impacts of the keystone predator Triops cancriformis (Branchiopoda: Notostraca) in temporary ponds. Freshw. Biol. 61, 1392–1404. doi: 10.1111/fwb.12779
Keywords: rock pool, functional feeding group, macroinvertebrates, aridlands, dispersal
Citation: Washko S and Bogan MT (2019) Global Patterns of Aquatic Macroinvertebrate Dispersal and Functional Feeding Traits in Aridland Rock Pools. Front. Environ. Sci. 7:106. doi: 10.3389/fenvs.2019.00106
Received: 14 March 2019; Accepted: 20 June 2019;
Published: 05 July 2019.
Edited by:
Rebecca Elizabeth Tharme, Riverfutures Ltd., United KingdomReviewed by:
Luiz Ubiratan Hepp, Universidade Regional Integrada do Alto Uruguai e das Missões, BrazilRenato Tavares Martins, Universidade Federal de Goiás, Brazil
Copyright © 2019 Washko and Bogan. This is an open-access article distributed under the terms of the Creative Commons Attribution License (CC BY). The use, distribution or reproduction in other forums is permitted, provided the original author(s) and the copyright owner(s) are credited and that the original publication in this journal is cited, in accordance with accepted academic practice. No use, distribution or reproduction is permitted which does not comply with these terms.
*Correspondence: Susan Washko, c3dhc2hrb0BlbWFpbC5hcml6b25hLmVkdQ==