- 1Laboratorio de Restauración Ecológica, Instituto de Biología, Universidad Nacional Autónoma de México, Mexico City, Mexico
- 2Department of Ecology, Evolution and Natural Resources, Rutgers, The State University of New Jersey, New Brunswick, NJ, United States
Fragmentation of natural landscapes during urbanization processes has been well-linked to biodiversity loss and changes in ecological and ecosystem function. Fragmentation can also have profound effects on provision of ecosystem services and on human social dynamics and well-being within cities. The ways in which ecological, ecosystem service, and social factors and the interactions among these are affected by fragmentation is not well-studied. Understanding these relationships is particularly important in cities where high densities of human population create intense and dynamic interactions within green spaces. Using the rapidly developing campus of the National Autonomous University of Mexico (UNAM) in Mexico City as a case study, we examine the effects of natural area fragmentation over time on ecosystem services, human well-being, and biodiversity. We compare fragmentation of the campus natural areas between 1954 and 2015 to non-native plant species distribution maps, models of water infiltration, and mobility and citizen-science data. We found that the loss and fragmentation of the campus's natural areas has had varied effects on ecosystem services, and negative effects on human well-being and biodiversity. The relationships between fragmentation and ecosystem services depends on the ecosystem service being measured; water infiltration was negatively impacted by fragmentation but engagement with biodiversity was greatest in areas with a mix of natural and urban land covers. Our human well-being variable, mobility (the average travel time within the campus of walkers, bicyclists, and cars), was negatively affected by fragmentation. Walkers and cyclists spent significantly more time traveling across campus in 2015 compared to 1954. Finally, the most fragmented areas of the campus's natural areas support large populations of invasive exotic species, indicating negative effects of fragmentation on biodiversity. Although campus urban development is necessary for supporting the academic community at this growing university, we suggest a renewed focus on sustainable campus development in which land sparing in emphasized; connecting instructional, research, administrative, and other campus facilities within urban clusters and providing sustainable transport systems. Thus, eliminating further loss and fragmentation of the natural areas on campus, supporting both human well-being and the unique biodiversity of the region.
Introduction
Urban landscapes are rapidly expanding around the world and one major consequence of the urbanization process is the loss and fragmentation of natural areas. Fragmentation, from large continuous natural areas of relatively undisturbed vegetation to small, increasingly isolated patches of natural land cover, has significant negative effects on ecology, ecosystem function, and human well-being (Savard et al., 2000). The ecological effects of fragmentation are primarily negative on all taxa and have been well-documented, ranging from habitat loss, reduction in species richness of plants and animals (Collinge, 1996; Haddad et al., 2015), alterations to life-history dynamics, dispersal, social systems, metapopulation dynamics, and species interactions to population decline and regional extirpation (Fischer and Lindenmayer, 2007). Ecosystem processes such as nutrient cycling and productivity are also reduced compared to connected landscapes (Haddad et al., 2015), often resulting in diminished ecosystem services provision (Mitchell et al., 2013). The consequences of habitat fragmentation on human well-being are less well-studied, and relatively unknown in urban areas.
It is well-established that human health and well-being are positively influenced by nature, and specifically, biodiversity, in urban areas (Sandifer et al., 2015). Urban development processes, particularly landscape fragmentation, threaten human well-being by reducing the amount of green space in cities as well as the provision of ecosystem services. The importance of urban greenspaces for human physical and mental health has been well-established (Di Giulio et al., 2009) and city planners are utilizing these human health connections to justify increases in parks and other green spaces in cities (Ayala-Azcárraga et al., 2019). However, human well-being may also be threatened by fragmentation in ways unrelated to nature access and ecosystem services. Most work in this realm has examined the effects of roads and traffic on humans, finding that roads and traffic decreases pedestrian movement and divide human communities, limiting interaction and social cohesion (Di Giulio et al., 2009). Additionally, planning focused on car travel often results in sedentary lifestyles, increasing rates of depression, obesity, and heart disease (Sandifer et al., 2015). No studies, to our knowledge, have specifically linked habitat fragmentation to human well-being in urban landscapes.
In cities, the ecosystem services framework has been used to determine the value particular urban ecosystems or habitats offer people and in some cases, has provided an important foundation for decision-making and urban planning (Elmqvist et al., 2015; Nilon et al., 2017; Saarikoski et al., 2018). However, we do not well-understand the links between fragmentation and ecosystem services (Mitchell et al., 2013, 2015). Recently, Mitchell et al. (2015) proposed a conceptual model to explain the probable effects of fragmentation on ecosystem service flow and provision. They propose that relationships among ecosystem services and fragmentation are influenced by the spatial configuration of the landscape (interspersion, isolation, patch size, edge, etc.), the particular ecosystem service in question, and the mechanisms by which that ecosystem service is provided (organism mobility, energy, matter flow, etc.). Thus, the relationship between fragmentation and ecosystem service provision can be negative or positive, linear or non-linear. For example, in a modeling scenario, Chaplin-Kramer et al. (2015) found that the effect of fragmentation of forest landscapes on two ecosystem services differed depending on the type of ecosystem service and the type of fragmentation. Carbon sequestration and biodiversity differed in their responses to agricultural expansion into edge or core forest habitats (Chaplin-Kramer et al., 2015), but both ultimately had a negative relationship with fragmentation. Changes in ecosystem services due to fragmentation can be more intense in urban regions, due to the limited green spaces remaining and greater densities of people relying on these services (Elmqvist et al., 2015). Additionally, the speed and number of people interacting with urban green spaces increase the importance of understanding the effect fragmentation has on ecosystem services provided in cities.
In order to plan and design cities as resilient social-ecological systems, we need to understand the influence of fragmentation processes on not only biodiversity but also on the provision of ecosystem services and on human well-being in cities. While conceptual frameworks have been presented that hypothesize the possible relationships between fragmentation and biodiversity and ecosystem services (Mitchell et al., 2015), very few studies have actually used empirical data to examine these relationships and none, that we know of, have presented the relationships between fragmentation and human well-being not related directly to ecosystem services. Here, we present a case-study examining how urban planning generates relationships among landscape fragmentation, biodiversity, ecosystem services, and human well-being at the main campus of the National Autonomous University of Mexico (UNAM), in Mexico City. Understanding the effects of fragmentation will help to generate knowledge on how ecosystem services and human well-being in urban green spaces are modified during the urbanization process, leading to better management and planning policies in cities.
Methods
The National Autonomous University of Mexico (UNAM) is the oldest university in America, established in 1551, when the Universidad Real y Pontificia was founded in Mexico City (Coll-Hurtado and Aclántara, 2011). Most of its campus was scattered around the city center in antique colonial buildings, until 1954, when the university moved to its current campus south in which those days was the edge of the city, after two decades of searching for an area large enough to establish the University (Morales Schechinger and García Jimenez, 2008). The area for the establishment became a challenge for development because it was on a lava stone ecosystem—Pedregal—generated from the Xitle volcano eruptions 1,600 years ago (Carrillo-Trubea, 1995). These eruptions covered close to 80,000 ha of lava in what is now the southern part of Mexico City (Delgado et al., 1998). After the volcano, a new ecosystem developed along the different type of rocks and cracks. This ecosystem is relatively young and consequently there is very little soil formation (Estañol-Tecuatl and Cano-Santana, 2017), which has generated a highly diverse xerophytic shrubland community (see Annex 1; de Rzedowski and Rzedowski, 2001; Cano-Santana et al., 2008). This fractured rock landscape is important for ground-water recharge; almost all precipitation infiltrates to a shallow aquifer encompassing the southern part of the city (Canteiro et al., 2019).
The total area given to the UNAM was 723 ha, but at the beginning the campus was established in <100 ha. Plans in the first decades after 1954 were to use the rest of the campus area to lend buildings to the government (Morales Schechinger and García Jimenez, 2008), but an academic/student protest helped to generate a reserve within the University (García Barrios, 2014) and the Ecological Reserve of San Angel Stone System (REPSA, as it stands in Spanish), was established in 1983, consisting of 124 ha, and is currently 237 ha. However, the existing reserve is highly fragmented by streets, buildings, and other facilities that fragments the once continuous lava stone shrubland ecosystem. The reserve has three core areas divided by wide and highly used roads and highways, and 17 isolated patches that are smaller and more isolated than the core areas. The surrounding parts of Mexico City have also undergone rapid development. Consequently, the REPSA is the last remnant of the Pedregal ecosystem in the city.
Building locations from 1954 to 2010 were digitized based on Coll-Hurtado and Aclántara (2011). Data representing 2015 was digitized from an orthophoto obtained from the Geography Institute at National University Autonomous of Mexico. We categorized different types of urban land use: (1) buildings (any type of construction that holds facilities); (2) roads; (3) parking lots; and (4) sports facilities (any court or fields utilized for various sporting activities including: gymnasium, basketball, racquetball, baseball, or football). The rest were considered green spaces, which included gardens and preserved areas. We also gathered data from General Direction of Planning UNAM (Dirección General de Planeación), to evaluate the population growth (student, academics, and workers) of the campus since it was established in 1954.
The fragmentation analysis was generated using “Landscape Fragmentation” (Center for Land Use Education and Research, University of Connecticut), installed in Spatial Analyst ArcGis 9.2. This extension is based on analyses in which fragmentation patterns in the landscape are based on the Morphological Image Processing of a binary image classified as: Forest—No forest (in this case Vegetation—No vegetation), maps algebra and analysis window of 8 × 8 pixels (Vogt et al., 2007). As a result, we categorized the landuse/landcover into five categories, urban land use (as described above) and four fragmentation natural land cover categories: (1) core, which are preserved areas that were either larger than 100 ha (large core shrubland) or smaller than 100 ha (small core shrubland); (2) perforations, perforated shrubland/urban areas within core areas that represent a urban-developed hole in the continuous vegetation generating edge effects, including paths within core areas; (3) edges, which were defined as the border between core areas and other categories, size in this category depends upon the resolution of the analysis, in this case, 10 m width; and (4) patches, which are small areas, smaller than any core areas, that are isolated from any other natural area, and are subjected to large edge effects. We divided the campus area into 1-ha hexagon units, in which the proportion urban land cover and each of the four fragmentation categories were classified. To test if these land use/land cover categories changed in area over time (1954–2015), we used a repeated measures ANOVA in R.
We evaluated the relationship between fragmentation of the campus natural areas and ecosystem services, human well-being, and biodiversity. The ecosystem services (ESS) we examined were water infiltration (provisioning ESS; TEEB, 2011) and biodiversity engagement (cultural ESS; TEEB, 2011). The biodiversity variables we examined were exotic species invasions, and the human well-being variable we examined was mobility (or movement across campus). Since these ES have different responses and a diverse availability of historical data, each had a particular temporal and/or spatial analysis.
In order to predict the effect of landscape fragmentation on water infiltration, we examined the water infiltration capacity of the campus landscape using three scenarios. Water filtration is reduced with increasing urban land cover (Zambrano et al., 2017). Therefore, we first modeled the effect of land use change on water infiltration capacity. Land use change was measured as the hectares that was converted from natural area to urban land use and natural area to anthropogenic green space (grass playing fields and managed gardens) between 1954 and each time point thereafter. In the land use change scenario, we assumed 0% infiltration in urban land uses, 50% in anthropogenic green spaces, and 100% in natural areas. Infiltration capacities were based on land use obtained from Zambrano et al. (2018). The assumption of the null infiltration in urban areas is based on the impermeable soil that was used as fill during construction in order to level the area for infrastructure (buildings, sewage, roads, sidewalks). This assumption was also made because it is common to have floods in lowlands of the campus and adjacent areas during storm events (Zambrano et al., 2018), because of saturation of the sewage system, proving the very low infiltration capacity of this category.
There are variables related to infiltration other than precipitation and urban land use, such as temperature, vegetation coverage, and soil (Mohammad and Adam, 2010; Green et al., 2011; Schosinsky and Losilla, 2011; Neris et al., 2012; Wang et al., 2013), but their relative importance to infiltration is not well-known and controversial (Green et al., 2011). However, there is much evidence that these variables change across fragmentation categories (Saunders et al., 1991). Therefore, we modeled the infiltration capacity of the campus using two scenarios by modifying the infiltration capacity of each fragmentation category with assumptions based on the literature (Table 1). The first scenario assumes that the rainwater in the “urban” category flows directly to the sewage system (0% infiltration); half of the water in the “edge” category infiltrates (50% and the other half goes to the sewage system; and the rest of the fragmentation categories have 100% infiltration. We assumed that the soil of the other categories have 100% infiltration because the soil of the preserved areas is based on lava stone ecosystem, which has a hydraulic conductivity between 10−2 and 10−7 m/s (Freeze and Cherry, 1979). This is higher than any soil in the area (Walker, 1993). Because the vegetation communities in the preserved areas is xerophytic, the vegetation cover is low; therefore, with low rates of evapotranspiration. Soil characteristics in perforations are similar to urban category, because they experience compacted soils that make them impermeable. Because perforations are urban land use in the middle of natural areas, the rainwater that falls in perforations results in runoff to the lava soil of the natural areas.
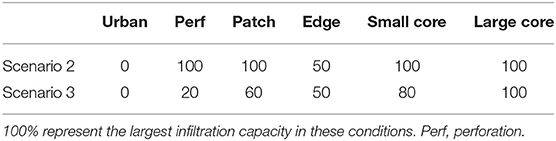
Table 1. Scenarios with different percentages of water infiltration based on capabilities of each fragmentation category to infiltrate rainwater to the aquifer.
The second scenario modifies those categories that were considered 100% infiltration, assuming that temperature plays an important role in the infiltration and will vary within each fragmentation category. Water that falls in the perforation category, for example, may have higher evaporation rates, because it spends a longer time on surfaces. Therefore, we considered that 80% of the water is lost in this category based on evaporation rates. Patches are surrounded by urban areas with lower vegetation coverage, leading to higher temperatures and higher evaporation rates. The same principle applies for smaller core areas, but with lower temperatures and consequently a higher rate of infiltration. These scenarios are hypothetical and based on the uncertainty of the importance of the size and other effects on infiltration capacities of green spaces. We then plotted the infiltration capacity of the campus in 1954, 1970, 1980, 1990, 2000, and 2015 based on the area of the campus within each land use and fragmentation category.
To examine biodiversity engagement on the UNAM campus, we used iNaturalist (https://www.inaturalist.org/) to evaluate the number of observations recorded in each 1-ha unit. Observations included records of any species of plants (mostly angiosperms, as flowers are an attractive attribute) and animals (mostly insects, birds, and mammals). We then plotted the number of observations in response to percent urban land cover and each of the four fragmentation categories for 2015 only to examine how biodiversity engagement differs across land use/land cover. Most of the 1-ha units in which the campus was divided had <5 observations, therefore we focused our analysis on 1-ha units with more than 20 observations.
For mobility within the campus, we consider that urban planning is highly related to mobility needs. Therefore, moving a school modifies the fragmentation of the area and the mobility of many students and staff faculty around the campus. We used an accessibility model based on friction surfaces, type of transport infrastructure, and a target location, based on Farrow and Nelson (2001). Target locations were seven schools that have been moved around the campus since it was established: Schools of Philosophy, Science, Political Sciences, Veterinary, Engineering, Administration, and Postgraduate facilities. We measured the time spent traveling from their schools to the main building of the campus, using cars, bicycles, or by walking. We used R version 3.53 for a paired t-test to evaluate differences in traveling time.
To examine the relationship between fragmentation and biodiversity, we used shapefiles of the distribution of two exotic plants within the campus based on Lot et al. (2012): trees in the genus Eucalyptus (particularly E. globulus) and the grass Pennisetum clandestinum. We then compared the distribution of these exotics to the fragmentation of 2015 only. We used the same 1-ha units to examine the correlation between percentages of exotic cover and percent urban land cover and each of the four fragmentation categories.
Results
The campus has undergone a large transformation since its establishment in 1954. New building construction has been dispersed across the entire campus area and not concentrated (Figure 1). Concurrently, population growth within the University has increased (Figure 2A). All of the different urban land use types have increased in area since 1954, but the area of buildings in particular surpassed parking lots, streets, and sports, indicating a densification of buildings (Figure 2B).
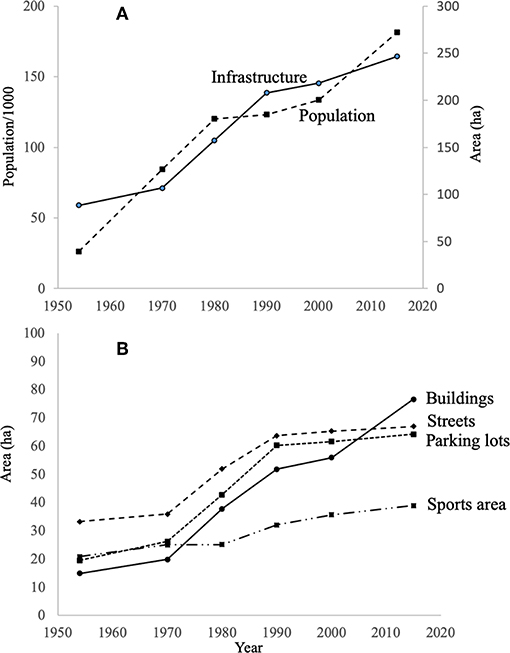
Figure 2. Infrastructure and population of the UNAM campus (A) and different types of urban infrastructure growth (B) from 1954 to 2015. Population numbers are in thousands and refers to the people (academics, students, and workers).
In the first three decades (1954–1983), many schools and facilities moved from the central campus to other regions in the campus, resulting in a landscape of scattered buildings and parking lots. This type of urban development resulted in fragmentation of the natural areas on campus (Figure 3). Statistically, all categories in the fragmentation classification changed with p-values below 0.001 among the years and most of these categories showed the important change between 1970 and 1990 (for the statistical analysis, please refer to the table in Supplementary Material). Core areas were reduced, large core areas completely disappeared between 1980 and 1990, as urban areas, small patches, and edges have significantly increased. Patches and perforations show a different pattern, as they are highly dynamic in this type of development, they have slowly increased, and for three decades they have been stable. Furthermore, the dispersion of categories such as urban, perforations, and patches in the campus suggest that fragmentation should generate large modification at different levels of the ecosystem dynamics, and in consequence in the ecosystem services.
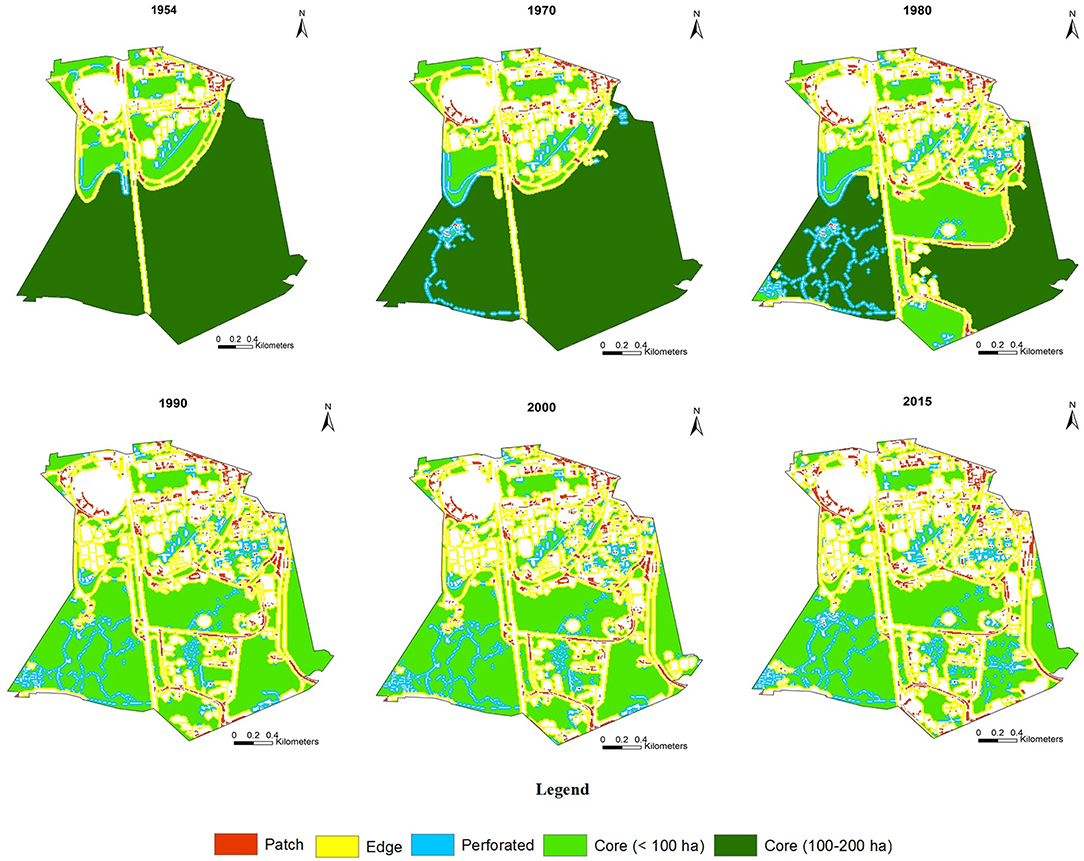
Figure 3. Fragmentation maps of the UNAM campus between 1954 to 2015. For information about the analyses used to generate each category see methods.
Ecosystem Services
Water Infiltration
In the first scenario, considering only land use change, there was a clear reduction in water infiltration capacity across time (Figure 4); this reduction corresponds with the urban growth patterns illustrated in Figure 2. In all scenarios, the highest infiltration reduction (40%) was during the same time as the most rapid urbanization of the campus. In scenarios 1 and 2, both considering that fragmentation patterns, not just land use change, would affect water infiltration, we found a further reduction in water infiltration capacity (Figure 4). All scenarios show a non-linear reduction of infiltration across time. Scenario 2 demonstrated the greatest reduction, where infiltration capacity was at most 60%, due to increases in evaporation compared to scenario 1, in which only urban and edge categories reduced infiltration and the rest of the categories, although fragmented, are capable of infiltrating the total amount of water received.
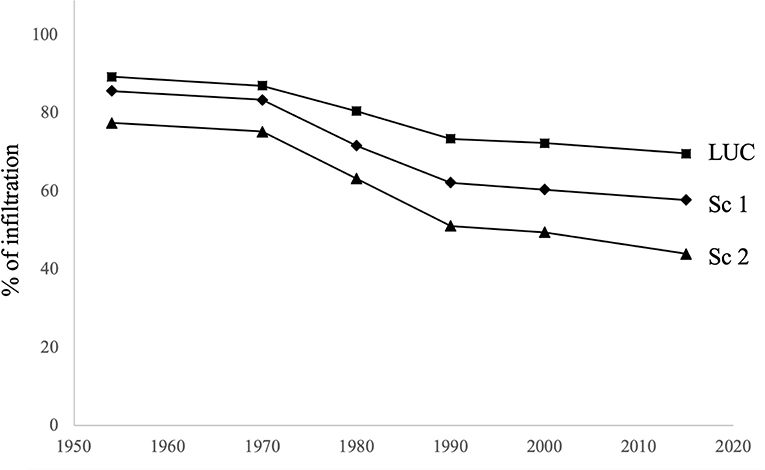
Figure 4. Rainwater infiltration capacities reduction based on LUC across years and hypothetical scenarios, considering fragmentation. Squares represents infiltration changes of infiltration based on land use change (LUC). Rhomboid show first scenario based on fragmentation data (Sc 1) in which urban category reduces its infiltration capacity with edge effect, and the rest of the categories have the highest infiltration rate. Triangles show the second fragmentation scenario (Sc 2) in which edges and perforations also have a reduction in the infiltration rate. See text for specific considerations of each scenario.
Biodiversity Engagement
The greatest number of species observations occurred when the urban proportion of any given 1-ha unit was lower than 40%, and the proportion of core habitat between 30 and 50% (Figure 5). Additionally, areas with >40% perforations had large numbers of observations. Although, there was also a peak in observations in 1-ha units of ~100% core habitat and 0% perforations. Therefore, 1-ha units with a mixed proportion of land use/land cover categories had the largest number of observations.
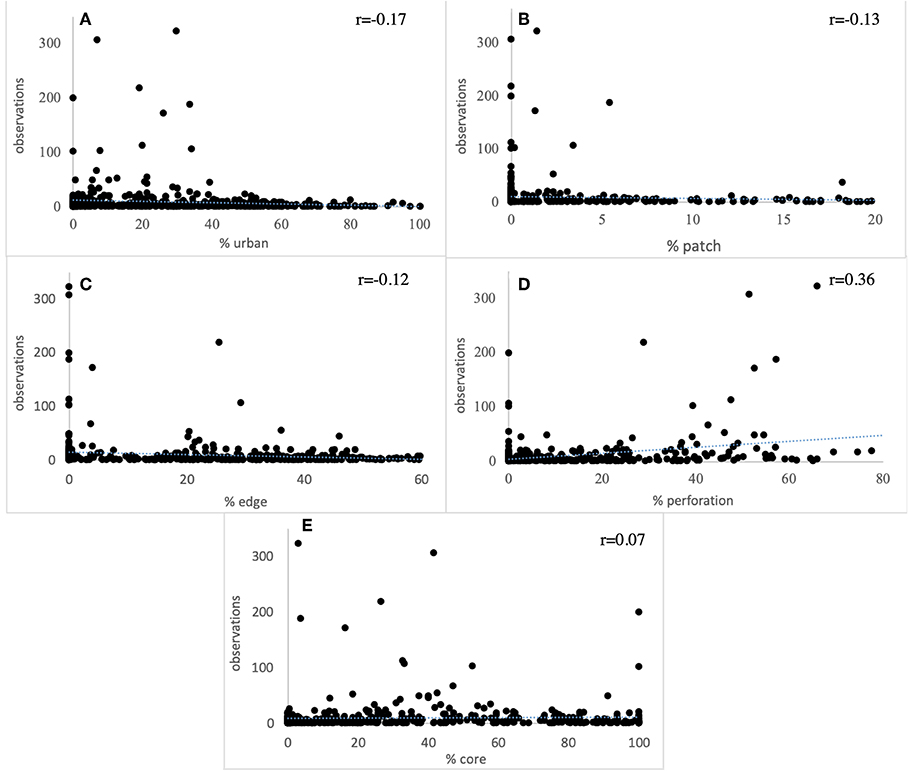
Figure 5. Number of iNaturalist observations compared to (A) % urban land cover, (B) % patch land cover, (C) % edge habitat, (D) %perforations, and (E) % core habitat in each 1-ha unit.
Human Well-Being
There is a clear change in time spent traveling within the campus along years based on the urban planning that generated fragmentation of the campus (Figure 6). Traveling by car reduced mobility time (t = 3.20; p = 0.018), while cycling (t = 4.02; p = 0.007) and pedestrians (t = 3.38; p = 0.014) increased almost twice the time of traveling from a school to the main building in the University.
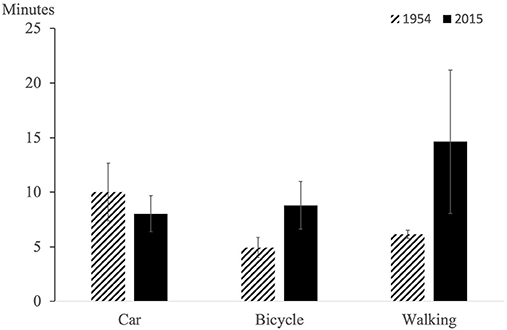
Figure 6. Time spent traveling from schools and other facilities to the main university building in 1954 and 2015. Error bars refer to standard deviation.
Biodiversity
While urban cover did not appear to be related to exotic plant abundance, other landscape fragmentation categories were closely related to the abundance and distribution of these species (Figures 7A–J). The exotic grass, Pennisetum clandestinum, abundance was most closely related to cover of perforations (Figure 7G); as the proportion of perforation cover increased, so did the cover of P. clandestinum. Pennisetum clandestinum was negatively related to urban, patch, and edge cover. Eucalyptus abundance increased with increasing edge cover (Figure 7F). Additionally, Eucalyptus cover peaked in areas with between 20 and 50% urban cover (Figure 7J).
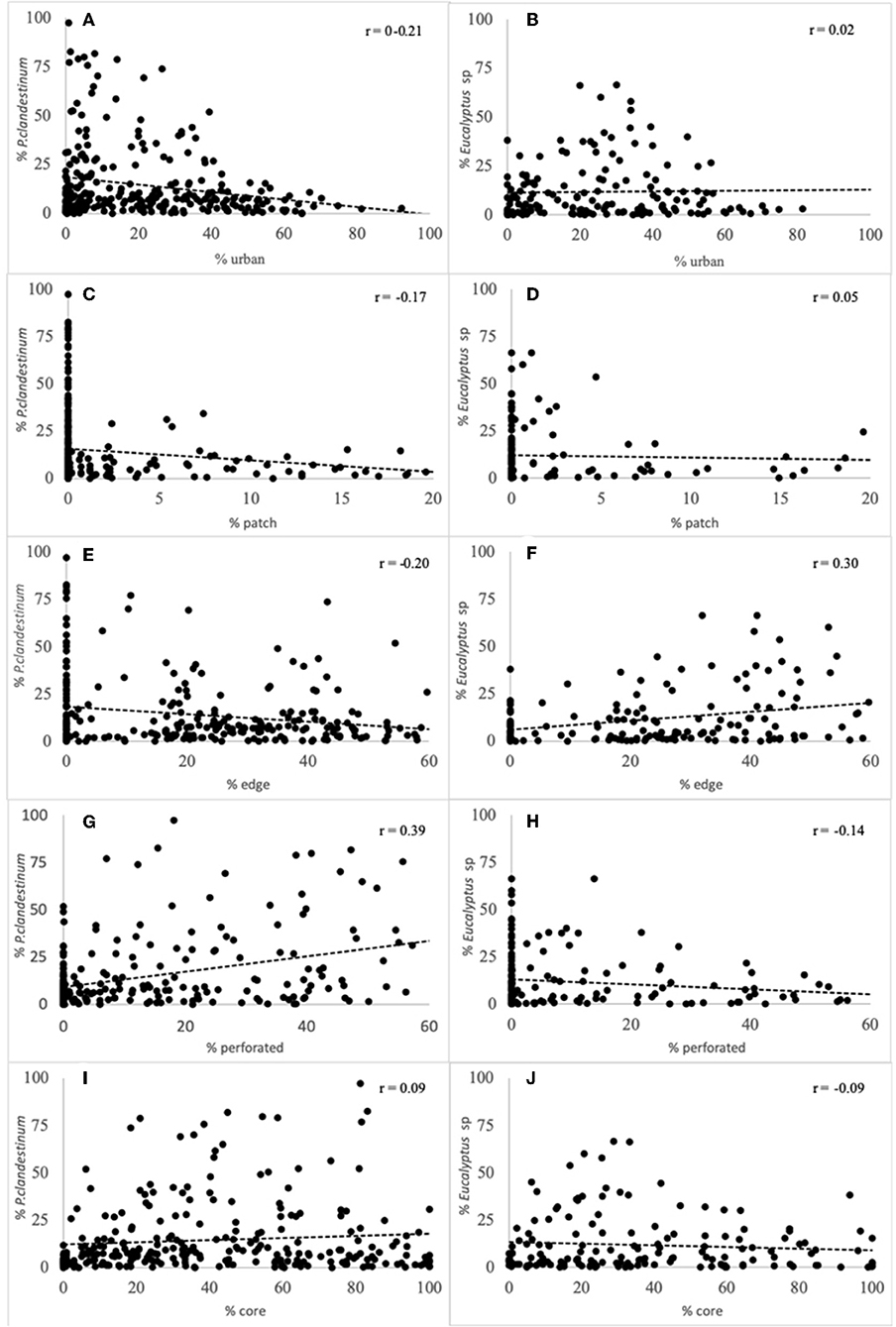
Figure 7. Percent cover of Pennisetum clandestinum (A,C,E,G,I) and Eucalyptus spp. (B,D,F,H,J) compared to % urban land cover, and % patch, edge, perforated, and core fragmentation categories in each 1-ha unit.
Discussion
Urban development policies have had direct influences on the fragmentation process since the campus was established in 1954. Some of these policies are related to historical and political trends that the university has been involved. The first urban design suggested a campus planned to avoid any internal movement with cars, considering that most of the students and workers arrived with public transportation. Also, this urban design focused on facilitating the interaction among students and faculty to increase multidisciplinary collaboration and was the answer to separated schools originally scattered across the city center from its foundation (Morales Schechinger and García Jimenez, 2008). However, in the subsequent years, the urban development in which the campus expanded, using the amount of undeveloped area it still had, was the product of three main factors. First, the type of expansion of Mexico City following the international urban development trends of the creation of suburbs and the increment of the use of cars for mobility (Jacobs, 1961). The campus aligned to that type of development, pushing schools and research facilities away from the central areas. Second, those schools that were pushed away were often the ones with larger numbers of student-activists (i.e., political science and sciences students), that were involved in the social movement in 1968 that ended in violence just before the Olympic games. The new distribution philosophy was to reduce interaction among students that could generate further social movements (García Barrios, 2014). Finally, the creation of REPSA along different areas of the campus, leaving scattered areas for development, generated random decision making for placing new buildings (Morales Schechinger and García Jimenez, 2008) and the need of roads and parking lots for each one, to supply the necessities of the gentrified academy able to own a car.
These development processes increased the fragmentation of the large natural areas on campus, which subsequently affected the ecosystem services provided to the campus and surrounding city. The loss of water infiltration, for example, appeared to be highly related not only to the increase of urban land cover over time (Zambrano et al., 2017), but also to fragmentation patterns. Land use change based on urban development is the strongest driver of water infiltration loss. However, our results show that fragmentation, and the size, location, and type of greenspaces, exacerbates the effect of land use change on infiltration, causing a further reduction. Future planning scenarios should consider the ramifications of this loss, preserving large natural areas in order to maintain water infiltration and reduce lowland flooding events. However, future research is needed to consider potential effects of fragmentation on temperature related to local infiltration to confirm or reject these scenarios.
Here we utilized iNaturalist, one of the largest interactive online platforms for recording, sharing, and identifying plants and animals, to measure the UNAM community's engagement with nature on the campus. We found that experience with nature was highest in areas of the campus that have a mix of land cover, indicating that nature engagement may not always be negatively affected by fragmentation. Biodiversity engagement on the UNAM campus was greatest in areas with <40% urban land cover, between 20 and 50% core forest cover, and >40% perforation cover. These are regions of the campus that are on the edges of the core natural areas and that have paths into the natural areas. In fact, the largest number of iNaturalist observations come from the Botanical Garden, which acts as a gateway to trails that traverse the core forest areas on the western side of the campus. University and public community members use these trails and the less remote portions of the natural areas when engaging with nature. Online platforms such as iNaturalist and others (e.g., eBird), have been primarily used for citizen science, such as determining distributions of particular species or groups of species (Sullivan et al., 2014). They have not, to our knowledge, been critically used to measure engagement and the ways in which people engage across different land uses. Data such as these can help us manage and plan natural areas in cities by understanding where urbanites are most likely to engage with nature in the city and where to focus programming to increase education and stewardship.
Urban planning leading to fragmentation effects on mobility is a phenomenon that must be reviewed from the historical perspective mentioned above. As the interactions among students from different schools were to be avoided (García Barrios, 2014), fragmentation has been produced by infrastructure mainly for cars. Routes for cycling and pedestrian were not considered, and only recently a policy for better cycling routes, but not for pedestrians, has been implemented (many streets do not even have a sidewalk for pedestrians). Roads without sidewalks or cycling infrastructure were built fragmenting all the green spaces. Public transport is also deficient and therefore only cars prevail using this infrastructure. This has produced a large modification of social interactions within the campus (Sheller and John, 2000), making a campus more car dependent with negative social consequences (Kenworthy and Laube, 1999). One of the consequences of increased cars on campus has been traffic jams and scarcity of parking lots, both within the campus. The time spent looking for parking or in traffic is not included in our study of mobility. Therefore, time spent may well have increased for cars over time, in addition to walking and bicycling.
More than 75% of the population (mostly students and workers) within the university move by walking, public transportation, or cycling. If they have to move among buildings at different regions of the campus, they could spend more than 70 min in this activity. Moreover, in the last 5 years, fences have been built in some pedestrian paths as a response of insecurity of some areas of the campus. These fences, surround many areas that could be crossed directly previously, have increased traveling times for pedestrians (pers obs). Therefore, fragmentation based on car mobility influence influenced human well-being in this particular case as happens in cities with fast urban expansion (Travisi et al., 2010; Zhao, 2010; De Vos and Witlox, 2013).
Prevalence and abundance of invasive exotic species are often used as an indicator of biodiversity loss (Butchart et al., 2010). Two metrics of fragmentation were most closely related to exotic species invasions: perforations and edges. Abundance of P. clandestinum increased with increasing perforations in the landscape. Pennisetum clandestinum is an invasive C4 grass, native to Africa, that is highly drought tolerant (Williams and Baruch, 2000). This species is also shade intolerant (Funk and McDaniel, 2010) and most often found at forest edges, where it spreads rapidly into newly cleared areas via vegetative spread (Wilen and Holt, 1996; Williams and Baruch, 2000; Steffen et al., 2011). At UNAM, P. clandestinum is often found along the paths and cleared areas in RESPA. Over time, perforations have increased (Figure 3), facilitating the spread of this invasive grass. Eucalyptus, on the other hand, was associated with edge habitat on the campus and does not as readily invade the core habitats. Other studies have found that Eucalyptus is primarily restricted to edges (Calviño-Cancela and van Etten, 2018), indicating that this species may be less likely to invade the core habitats of RESPA. However, where Eucalyptus has invaded, it likely has altered hydrology and nutrient cycling (Dukes and Mooney, 2004), as well as reduced native species establishment (Zhang and Fu, 2009). These data suggest that we can expect more invasions as the campus natural areas continue to be fragmented, and edge effects increase due to both increases in edges and perforations.
One of the most important consequences of the development process at the UNAM was the fragmentation of core natural habitat. In only a decade, between 1980 and 1990, the largest core natural areas of over 100 ha in size were lost. Although we do not have historic biodiversity data, this loss of these largest core habitats, followed by further loss of additional core habitat <100 ha, has important consequences for regional biodiversity. As Mexico City has undergone rapid urbanization, REPSA is one of the only remaining lava stone ecosystems in the region, making it an essential refuge for the plants and animals indigenous to this system (Ramirez-Cruz et al., 2019). The reserve continues to be threatened by urban development, human use, edge effects, and invasive species. Managing this landscape to reduce these impacts is imperative to preserving the unique natural history of the region.
For the last 30 years, the rapid campus development has led to conflicts between ensuring conservation of the natural areas and infrastructure needs of the University, leading to discussions among parties whenever new urban development is proposed (Zambrano and Cano-Santana, 2016), as has happened in the rest of the city (Calderón-Contreras and Quiroz-Rosas, 2017). These process, and other factors described above, have led to haphazard development of the campus and construction of campus buildings and other facilities that do not follow the existing master plans. Our results here support the idea that fragmentation promotes different responses in ecosystem services. For this reason, the importance of developing, following, and adapting a comprehensive master plan for a sustainable and resilient campus. Ideally, one in which land sparing is emphasized, as well as connecting instructional, research, administrative, and other campus facilities in dense urban clusters and sustainable transport planning, focusing on walking, bicycling, and public transport (Balsas, 2003). These factors have been shown to lead to better retention and graduation rates, because of positive student experiences (Hajrasouliha, 2017). Additionally, when land sparing is emphasized, biodiversity and ecosystem services, such as water infiltration, are enhanced (Soga et al., 2014; Stott et al., 2015) leading to more sustainable and resilient urban areas.
Conclusions
Although our study represents urban development processes in a relatively small area in a large mega-city, we show important implications of fragmentation for ecosystem services, human well-being, and biodiversity. These relationships are moderated by natural history, human activities, culture, and socio-political events. We show here that the effects of fragmentation may not always be negative but depends these complex factors. Importantly, we show that the outcome of fragmentation on ecosystem services provision is dependent not only on the characteristics of the landscape, but also the ecosystem service in question and the historical and social characteristics of urbanization. While it is clear that fragmentation has important negative consequences for biodiversity, biodiversity engagement is enhanced with some level of fragmentation. Future research should focus on evaluating general patterns of the relationship between ecosystem services and human well-being under complex system focuses. For the UNAM campus, and cities as a whole, understanding the influences of fragmentation processes on ecosystem services, human well-being and biodiversity can aid in integrating the multiple, sometimes conflicting, needs of people and biodiversity in cities.
Data Availability Statement
The datasets generated for this study are available on request to the corresponding author.
Author Contributions
LZ and MA participated on the design of the project, generated the hypothesis, and wrote the manuscript. TF gathered data, performed the spatial analysis, and discussed results.
Funding
This work was financially supported by the Dirección General de Asuntos del Personal Académico - Universidad Nacional Autónoma de México through the project PAPIIT IV200117 Eco-social analysis of an urban reserve for sustainability on the main campus of the National Autonomous University of Mexico and the US National Science Foundation (NSF RCN: DEB 1354676/1355151). CONACYT and UNAM helped with the sabbatical award for LZ.
Conflict of Interest
The authors declare that the research was conducted in the absence of any commercial or financial relationships that could be construed as a potential conflict of interest.
The handling editor declared a shared affiliation, though no other collaboration, with two of the authors, LZ and TF.
Acknowledgments
We thank the SEREPSA staff for information provided, particularly to Merly Yaridi Fábila. This project was financed by PAPIIT-UNAM IV200117. Scholarships from DGAPA and Consejo Nacional de Ciencia y Tecnología CONACYT were provided for the collaboration among researchers.
Supplementary Material
The Supplementary Material for this article can be found online at: https://www.frontiersin.org/articles/10.3389/fenvs.2019.00152/full#supplementary-material
Supplementary Table 1. Repeated measures analysis of each category along years.
Annex 1. Pictures of the described ecosystem in dry (1) and wet season (2).
References
Ayala-Azcárraga, C., Diaz, D., and Zambrano, L. (2019). Characteristics of urban parks and their relation to user well-being. Landsc. Urban Plan. 189, 27–35. doi: 10.1016/j.landurbplan.2019.04.005
Balsas, C. J. L. (2003). Sustainable transportation planning on college campuses. Transp. Policy 10, 35–49. doi: 10.1016/S0967-070X(02)00028-8
Butchart, S. H. M., Walpole, M., Collen, B., van Strien, A., Scharlemann, J. P. W., Almond, R. E. A., et al. (2010). Global biodiversity: indicators of recent declines. Science. 328, 1164–1168. doi: 10.1126/science.1187512
Calderón-Contreras, R., and Quiroz-Rosas, L. E. (2017). Analysing scale, quality and diversity of green infrastructure and the provision of urban ecosystem services: a case from Mexico City. Ecosyst. Serv. 23, 127–137. doi: 10.1016/j.ecoser.2016.12.004
Calviño-Cancela, M., and van Etten, E. J. B. (2018). Invasive potential of Eucalyptus globulus and Pinus radiata into native eucalypt forests in Western Australia. For. Ecol. Manage. 424, 246–258. doi: 10.1016/j.foreco.2018.05.001
Cano-Santana, Z., Castillo-Argüero, S., Martínez-Orea, Y., and Juárez Orozco, S. (2008). Análisis de la riqueza vegetal y el valor de conservación de tres áreas incorporadas a la Reserva Ecológica del Pedregal de San Ángel, D.F. (México). Bol. Soc. Bot. Méx. 82, 1–14. doi: 10.17129/botsci.1777
Canteiro, M., Olea, S., Escolero, O., and Zambrano, L. (2019). Relationships between urban aquifers and preserved areas south of Mexico City. Groundw. Sustain. Dev. 8, 373–380. doi: 10.1016/j.gsd.2018.12.007
Chaplin-Kramer, R., Sharp, R. P., Mandle, L., Sim, S., Johnson, J., Butnar, I., et al. (2015). Spatial patterns of agricultural expansion determine impacts on biodiversity and carbon storage. Proc. Natl. Acad. Sci. U.S.A. 112, 7402–7407. doi: 10.1073/pnas.1406485112
Coll-Hurtado, A., and Aclántara, I. (2011). Un siglo de la Universidad Nacional de México, 1910-2010. Sus huellas en el espacio a través del tiempo. Mexico: Instituto de Geografía Mexico: Universidad Nacional Autónoma de México.
Collinge, S. K. (1996). Ecological consequences of habitat fragmentation: Implications for landscape architecture and planning. Landsc. Urban Plan. 36, 59–77. doi: 10.1016/S0169-2046(96)00341-6
de Rzedowski, G. C., and Rzedowski, J. (2001). Flora fanerogámica del Valle de México. Xalapa: Instituto de Ecologica, A.C.
De Vos, J., and Witlox, F. (2013). Transportation policy as spatial planning tool; reducing urban sprawl by increasing travel costs and clustering infrastructure and public transportation. J. Transp. Geogr. 33, 117–125. doi: 10.1016/j.jtrangeo.2013.09.014
Delgado, H., Molinero, R., Cervantes, P., Nieto-Obregón, J., Cruz, R. L. S., Madas-González, H. L., et al. (1998). Geology of Xitle volcano in southern Mexico City - A 2000-year-old monogenetic volcano in an urban area. Rev. Mex. Ciencias Geol. 15, 115–131.
Di Giulio, M., Holderegger, R., and Tobias, S. (2009). Effects of habitat and landscape fragmentation on humans and biodiversity in densely populated landscapes. J. Environ. Manage. 90, 2959–2968. doi: 10.1016/j.jenvman.2009.05.002
Dukes, J. S., and Mooney, H. A. (2004). Disruption of ecosystem processes in western North America by invasive species. Rev. Chil. Hist. Nat. 77, 411–437. doi: 10.4067/S0716-078X2004000300003
Elmqvist, T., Setälä, H., Handel, S. N., van der Ploeg, S., Aronson, J., Blignaut, J. N., et al. (2015). Benefits of restoring ecosystem services in urban areas. Curr. Opin. Environ. Sustain. 14, 101–108. doi: 10.1016/j.cosust.2015.05.001
Estañol-Tecuatl, F., and Cano-Santana, Z. (2017). Recovery of basalt substrate for xeric scrub restoration in a lava field in Mexico City. Ecol. Restor. 35, 41–51. doi: 10.3368/er.35.1.41
Farrow, A., and Nelson, A. (2001). Modelación de la Accesibilidad en ArcView 3: Una extensión para calcular el tiempo de viaje y obtener información sobre captación de mercados. Cali: Centro Internacional de Agricultura Tropical (CIAT), 22.
Fischer, J., and Lindenmayer, D. B. (2007). Landscape modification and habitat fragmentation: A synthesis. Glob. Ecol. Biogeogr. 16, 265–280. doi: 10.1111/j.1466-8238.2007.00287.x
Freeze, R. A., and Cherry, J. (1979). Groundwater: Englewood Cliffs. Prentince. Englewood Cliffs. NJ: Prentince Hall.
Funk, J. L., and McDaniel, S. (2010). Altering light availability to restore invaded forest: the predictive role of plant traits. Restor. Ecol. 18, 865–872. doi: 10.1111/j.1526-100X.2008.00515.x
García Barrios, R. (2014). El origen de la reserva ecológica de la UNAM en CU: historia de un conflicto patrimonial y ambiental. Cult. Represent. Soc. 9, 177–226.
Green, T. R., Taniguchi, M., Kooi, H., Gurdak, J. J., Allen, D. M., Hiscock, K. M., et al. (2011). Beneath the surface of global change: Impacts of climate change on groundwater. J. Hydrol. 405, 532–560. doi: 10.1016/j.jhydrol.2011.05.002
Haddad, N. M., Brudvig, L. A., Clobert, J., Davies, K. F., Gonzalez, A., Holt, R. D., et al. (2015). Habitat fragmentation and its lasting impact on Earth's ecosystems. Sci. Adv. 1:e1500052. doi: 10.1126/sciadv.1500052
Hajrasouliha, A. (2017). Campus score: measuring university campus qualities. Landsc. Urban Plan. 158, 166–176. doi: 10.1016/j.landurbplan.2016.10.007
Jacobs, J. (1961). The Death and Life of Great American Cities. The Failure of Town Planning. New York, NY: Random House.
Kenworthy, J. R., and Laube, F. B. (1999). Patterns of automobile dependence in cities: An international overview of key physical and economic dimensions with some implications for urban policy. Transp. Res. Part A Policy Pract. 33, 691–723. doi: 10.1016/S0965-8564(99)00006-3
Lot, A., Escovedo, M. P., Alarcon, G. G., Palacios, S. R., and Camarena, P. (2012). La reserva ecológica del pedregal de san Ángel: atlas de riesgos., ed. Universidad Nacional Autónoma de México Mexico.
Mitchell, M. G. E., Bennett, E. M., and Gonzalez, A. (2013). linking landscape connectivity and ecosystem service provision: current knowledge and research gaps. Ecosystems. 16, 894–908. doi: 10.1007/s10021-013-9647-2
Mitchell, M. G. E., Suarez-Castro, A. F., Martinez-Harms, M., Maron, M., McAlpine, C., Gaston, K. J., et al. (2015). Reframing landscape fragmentation's effects on ecosystem services. Trends Ecol. Evol. 30, 190–198. doi: 10.1016/j.tree.2015.01.011
Mohammad, A. G., and Adam, M. A. (2010). The impact of vegetative cover type on runoff and soil erosion under different land uses. Catena. 81, 97–103. doi: 10.1016/j.catena.2010.01.008
Morales Schechinger, C., and García Jimenez, S. (2008). “Mexico City and Univeristy City: a story of struggle for autonomy though land,” in Globla Universities and Urban Development Case Studies and Analysis, eds W. Wiewel, and D. Perry (London: Routledge, 119–154.
Neris, J., Jiménez, C., Fuentes, J., Morillas, G., and Tejedor, M. (2012). Vegetation and land-use effects on soil properties and water infiltration of Andisols in Tenerife (Canary Islands, Spain). Catena. 98, 55–62. doi: 10.1016/j.catena.2012.06.006
Nilon, C. H., Aronson, M. F. J., Cilliers, S. S., Dobbs, C., Frazee, L. J., Goddard, M. A., et al. (2017). Planning for the future of urban biodiversity: a global review of city-scale initiatives. Bioscience. 67, 332–342. doi: 10.1093/biosci/bix012
Ramirez-Cruz, G., Solano-Zavaleta, I., Mendoza-Hernández, P. E., Méndez-Janovitz, M., Suarez-Rodriguez, M., and Zúñiga-Vega, J. (2019). This town ain't big enough for the both of us — or is it? Spatial co-ocurrence between exotic and native species in an urban reserve. PLoS ONE, 14:e0211050. doi: 10.1371/journal.pone.0211050
Saarikoski, H., Primmer, E., Saarela, S. R., Antunes, P., Aszalós, R., Baró, F., et al. (2018). Institutional challenges in putting ecosystem service knowledge in practice. Ecosyst. Serv. 29, 579–598. doi: 10.1016/j.ecoser.2017.07.019
Sandifer, P. A., Sutton-Grier, A. E., and Ward, B. P. (2015). Exploring connections among nature, biodiversity, ecosystem services, and human health and well-being: opportunities to enhance health and biodiversity conservation. Ecosyst. Serv. 12, 1–15. doi: 10.1016/j.ecoser.2014.12.007
Saunders, D. A., Hobbs, R. J., and Margules, C. R. (1991). Biological consequences of ecosystem fragmentation: a review. Conserv. Biol. 5, 18–32. doi: 10.1111/j.1523-1739.1991.tb00384.x
Savard, J. P. L., Clergeau, P., and Mennechez, G. (2000). Biodiversity concepts and urban ecosystems. Landsc. Urban Plan. 48, 131–142. doi: 10.1016/S0169-2046(00)00037-2
Schosinsky, G., and Losilla, M. (2011). Modelo analítico para determinar la infiltración con base en la lluvia mensual. Rev. Geológica América Cent. 23, 43–55. doi: 10.15517/rgac.v0i23.8579
Sheller, M., and John, U. (2000). The city and the car. Int. J. Urban Reg. Res. 24, 737–757. doi: 10.1111/1468-2427.00276
Soga, M., Yamaura, Y., Koike, S., and Gaston, K. J. (2014). Land sharing vs. land sparing: does the compact city reconcile urban development and biodiversity conservation? J. Appl. Ecol. 51, 1378–1386. doi: 10.1111/1365-2664.12280
Steffen, W., Persson, Å., Deutsch, L., Zalasiewicz, J., Williams, M., Richardson, K., et al. (2011). The anthropocene: from global change to planetary stewardship. Ambio 40, 739–761. doi: 10.1007/s13280-011-0185-x
Stott, I., Soga, M., Inger, R., and Gaston, K. J. (2015). Land sparing is crucial for urban ecosystem services. Front. Ecol. Environ. 13, 387–393. doi: 10.1890/140286
Sullivan, B. L., Aycrigg, J. L., Barry, J. H., Bonney, R. E., Bruns, N., Cooper, C. B., et al. (2014). The eBird enterprise: an integrated approach to development and application of citizen science. Biol. Conserv. 169, 31–40. doi: 10.1016/j.biocon.2013.11.003
TEEB (2011). The Economics of Ecosystems and Biodiversity in National and International Policy Making, ed P. ten Brink. London; Washington: Earthscan.
Travisi, C. M., Camagni, R., and Nijkamp, P. (2010). Impacts of urban sprawl and commuting: a modelling study for Italy. J. Transp. Geogr. 18, 382–392. doi: 10.1016/j.jtrangeo.2009.08.008
Vogt, P., Riitters, K. H., Estreguil, C., Kozak, J., Wade, T. G., and Wickham, J. D. (2007). Mapping spatial patterns with morphological image processing. Landsc. Ecol. 22, 171–177. doi: 10.1007/s10980-006-9013-2
Walker, G. P. L. (1993). “Basaltic-volcano systems,” in MagmaticProcesses and PlateTectonics, eds H. M. Richard, T. Alabaster, N. B. W. Harris, and C. R. Neary (London, UK: Geological Society Publications), 3–38.
Wang, S., Fu, B., Gao, G., Liu, Y., and Zhou, J. (2013). Responses of soil moisture in different land cover types to rainfall events in a re-vegetation catchment area of the Loess Plateau, China. Catena. 101, 122–128. doi: 10.1016/j.catena.2012.10.006
Wilen, C., and Holt, J. (1996). Spatial growth of Kikyograss (Pennisetum clandestinum). Weed Sci. Soc. Am. Stable 444, 323–330. doi: 10.1017/S0043174500093954
Williams, D. G., and Baruch, Z. (2000). African grass invasion in the Americas: Ecosystem consequences and the role of ecophysiology. Biol. Invasions 2, 123–140. doi: 10.1023/A:1010040524588
Zambrano, L., and Cano-Santana, Z. (2016). Historias que brotan de las rocas - Experiencias sobre el Pedregal de San Ángel y su reserva ecológica. Mexico: UNAM.
Zambrano, L., Pacheco-Muñoz, R., and Fernández, T. (2017). A spatial model for evaluating the vulnerability of water management in Mexico City, São Paulo and Buenos Aires considering climate change. Anthropocene 17, 1–12. doi: 10.1016/j.ancene.2016.12.001
Zambrano, L., Pacheco-Muñoz, R., and Fernández, T. (2018). Influence of solid waste and topography on urban floods: the case of Mexico City. Ambio. 47, 771–780. doi: 10.1007/s13280-018-1023-1
Zhang, C., and Fu, S. (2009). Allelopathic effects of eucalyptus and the establishment of mixed stands of eucalyptus and native species. For. Ecol. Manage. 258, 1391–1396. doi: 10.1016/j.foreco.2009.06.045
Keywords: biodiversity, ecosystem services, human well-being, iNaturalist, landscape fragmentation, university campus planning, urban development processes
Citation: Zambrano L, Aronson MFJ and Fernandez T (2019) The Consequences of Landscape Fragmentation on Socio-Ecological Patterns in a Rapidly Developing Urban Area: A Case Study of the National Autonomous University of Mexico. Front. Environ. Sci. 7:152. doi: 10.3389/fenvs.2019.00152
Received: 08 May 2019; Accepted: 20 September 2019;
Published: 18 October 2019.
Edited by:
Constantino Macías Garcia, National Autonomous University of Mexico, MexicoReviewed by:
Elzbieta Antczak, University of Łódz, PolandPerrine Hamel, Stanford University, United States
Copyright © 2019 Zambrano, Aronson and Fernandez. This is an open-access article distributed under the terms of the Creative Commons Attribution License (CC BY). The use, distribution or reproduction in other forums is permitted, provided the original author(s) and the copyright owner(s) are credited and that the original publication in this journal is cited, in accordance with accepted academic practice. No use, distribution or reproduction is permitted which does not comply with these terms.
*Correspondence: Luis Zambrano, emFtYnJhbm9AaWIudW5hbS5teA==