- 1College of Resources and Environment, University of Chinese Academy of Sciences, Beijing, China
- 2Quebec Research and Development Centre, Agriculture and Agri-Food Canada, Quebec, QC, Canada
- 3Department of Soil Science, King Saud University, Riyadh, Saudi Arabia
Industrial by-products such as paper mill biosolids (PBs) have been used as fertilizers and amendments in agriculture for many decades. However, their content of plant essential nutrients including phosphorus (P) varies largely with sources, and availability of these nutrients once applied to soil still remains unknown. Five PBs differing in their C/N ratio (12–47) were used in an incubation study to assess the P forms and availability from both PBs and PB-amended soils. Biosolids were added to three contrasting soils varying in texture and pH in 500-ml jars at the rate of 50 Mg wet ha–1, supplying between 45 and 112 kg total P ha–1. An unfertilized control and a mineral NP treatment (30 kg P ha–1) were also included. Biosolids and soil P fractions were determined by a modified Hedley fractionation method following 2 and 16 weeks of incubation. Phosphorus forms in PBs varied across type of materials with labile P ranging from 20 to 72% and organic P (Po) ranging from 28 to 66% of total P. Soil incubated with PB showed changes in all P fractions due to amendment, soil type, and duration of incubation. Resin-P and labile P, the most available forms, were significantly higher with addition of high PB–P content. Soil labile P represented up to 50% of total P added by PB. Overall, most inorganic P (Pi) fractions and labile P further increased with incubation duration. Close relationships were established between resin-P and labile P content in PB and the increases of those fractions in incubated soils, notably after 2 weeks. The P recovery as labile P represented on average 34% of total P added or 68% in terms of mineral P. We concluded that PB could be used as an efficient source of P for fertilizing crops.
Introduction
The non-renewable status of extractable phosphorus (P) on Earth (Cordell et al., 2009) has resulted in an increasing interest of alternative sources such as the use of biosolids in agriculture (Shu et al., 2016). Biosolids are by-products of industrial or urban wastewater treatment which are commonly used as fertilizer and soil amendment to improve crop productivity (Su et al., 2007). Biosolids application can positively enhance crop dry matter production and yield through their N availability (Cogger et al., 2006; Al-Dhumri et al., 2013). Henry et al. (1994) confirmed the potential of biosolids to increase the productivity of many forests.
Some concerns about biosolids application, such as potential environment contamination, make its management a very important part when used in agriculture soils. Biosolids are often applied based on crop N requirements, which may lead to soil P accumulation and increase the risk of P loss to surface or groundwater (Kelling et al., 1977; Shober and Sims, 2003; Pierzynski and Gehl, 2005). The N/P ratios for nutrient crop uptake in field crops varied from 4.5 to 8.7 (Sadras, 2006), which are considerably higher than those found in municipal biosolids (2.7) but much closer to PB (5.7) (Miller et al., 2000; Charbonneau et al., 2001; Maguire et al., 2001). Moreover, biosolids properties and their treatment plant process may have a significant impact on their fertilizer value, which can affect soil P availability (Frossard et al., 1996; Maguire et al., 2001; Penn and Sims, 2002; Torri et al., 2017).
Many studies documented soil P status once biosolids are applied. Maguire et al. (2001) recommended testing biosolids for P availability rather than total P, since it was a more appropriate indicator for predicting extractable P from biosolids-amended soils. Conversely, biosolids with similar total P may vary widely in the amount of P measured in various extracts (O’Connor et al., 2004). Different methods, including water-extractable P, Olsen P, or Mehlich-3 P (Wang et al., 2004; Su et al., 2007; Shu et al., 2016), can be used to investigate the effect of biosolids on soil P availability. Ippolito et al. (2007) reported that characterizing fractions into labile and non-labile pools could be a typical method to predict P availability in biosolids-amended soils. To address this, soil P fractionation can serve to predict the availability of P in soil as well as the likelihood of its transport (Sui et al., 1999).
The pulp and paper industry constitutes a major component of the Canadian economy, and their activities generate huge amounts of organic residues from the treatment of derived wastewater. Most recent statistics indicate that 1.5 million dry Mg paper mill biosolids (PBs) are produced annually (Rashid et al., 2006), but a low percentage (<25%) is applied on cropland whereas the rest of the residues are burnt or landfilled (MDDELCC, 2016). Large volumes of PBs are also available in the United States (5.5 million dry Mg year–1; Thacker, 2007) and Europe (11 million dry Mg year–1 including rejects and ash; Monte et al., 2009). Land application of PB as municipal biosolids can benefit crop growth by supplying N and P while improving soil physical properties (Cabral et al., 1998; Camberato et al., 2006). Better knowledge of nutrient contribution from PB including P might help promote their appropriate use in the field.
Studies conducted to characterize municipal biosolids indicated that labile P fraction composes a small proportion of total P but this does depend on sludge type (Frossard et al., 1996; Sui et al., 1999; Ajiboye et al., 2004; Shober et al., 2006). For example, addition of lime or metal salts during the wastewater treatment process to stabilize biosolids can reduce solubility of P (Frossard et al., 1996; Pierzynski and Gehl, 2005; Su et al., 2007; Torri et al., 2017). With PB, the treatment of effluent generally consists of primary clarification to remove suspended solids followed by an activated secondary process in which microorganisms are used to reduce organic pollutants and biological oxygen demand (Nurmesniemi et al., 2007; Monte et al., 2009). To our knowledge, little is known about P forms in this particular material. When applied to soil, municipal biosolids were often reported to cause a net increase of relatively available Pi forms (H2O, NaHCO3, and NaOH) at the expense of more stable or recalcitrant forms (NaOH-Po, HCl-P, and residual P) (Sui et al., 1999; Kashem et al., 2004; Su et al., 2007; Alleoni et al., 2012). This transformation of NaOH-Po and residual P to more labile forms was also observed with PB after 6 years of application (Fan et al., 2010). All these studies, however, did not assess the P response of PB under different soil types and their possible interactions.
The objectives of this study were to (1) characterize the P forms in several PBs used in Canada and (2) determine the P forms after PB addition to soils with contrasting chemical properties after 2 (short-term) and 16 (typical duration of growing season) weeks under controlled conditions.
Materials and Methods
Soils and Biosolids Characterization
The soils used for the incubation study were collected from the upper layer (0–15 cm) of three fields. Two soils came from near Quebec City, QC, Canada (47°N, 71°W): a Kamouraska silty clay (KAM; umbric Gleysol; FAO soil classification) from a cornfield and a St-Antoine sandy loam (StA; haplic Podzol) from a field under alfalfa/timothy pasture. The other soil came from near Lethbridge, AB, Canada (50°N, 113°W): a Chin fine sandy loam (CHIN; haplic Kastanozems) from a potato field. The soils were air-dried and sieved to 2 mm before incubation. Each soil was characterized in triplicate for particle size, pH, organic C, Mehlich-3 extractable elements, and P fractions (Table 1). The KAM and StA soils were selected to evaluate the response of PB in acidic soils, where P fixation occurs predominantly with Fe and Al oxides/hydroxides. The alkaline soil, CHIN, was selected to account for P fixation by Ca. According to critical P/Al or P/(Al + Fe) values, the KAM and StA soils had a greater capacity to retain P against losses into the environment (Pellerin et al., 2006; Wang et al., 2015).
Five different PBs were tested in this study: (1) mixed PB (primary and secondary treated sludge) from thermomechanical pulp (PB1); (2) mixed PB from bleached Kraft pulp with some municipal effluent (PB2); (3 and 4) mixed PB from bleached acid-treated Kraft pulp issued from different production times (PB3a and PB3b); and (5) de-inking sludge from recycled papers (PB4). These PBs have been previously used in field experiments (Gagnon et al., 2010, 2012b; Gagnon and Ziadi, 2012) and respected limits for trace metal contaminants (Camberato et al., 2006). Selected PBs differed largely in their properties, notably pH, total N and P, and C/N ratio (Table 2). PB2 and PB3b showed the highest concentrations in total N and P and the lowest C/N ratio.
Incubation Study
Biosolids were mixed with 100 g of air-dried soil at a rate equivalent to 50 Mg wet ha–1 (4.17 g fresh material per jar considering a depth incorporation of 10 cm and a soil bulk density of 1.2 g cm–3) and incubated in 500-ml MasonTM glass jars. The PB supplied between 45 and 112 kg total P ha–1 (Table 2). Unamended controls containing soils only were prepared in a similar manner in order to estimate the contribution of soil-derived P. In addition, a mineral NP treatment [(30 kg KH2PO4-P ha–1 and 100 kg N ha–1 as NH4NO3 (35% N)] was added to evaluate the relative P contribution of each biosolids.
The experiment was laid out as a factorial of seven treatments (five PBs, NP, and the control) and three soils completely randomized in three replications. Distilled water was added to the soil–PB mixtures to adjust water-filled pore space to 60%. The jars were closed and incubated in the dark in a controlled environment chamber at 25°C for 16 weeks. Water loss was monitored twice a week and corrected if needed. At the same time, the soils were well aerated and carefully remixed before reclosing to maintain constant aerobic and mineralization conditions.
Soil Phosphorus Fractionation
Subsamples (5 g) of the soil–PB mixtures were collected after 2 and 16 weeks of incubation and analyzed for P fractions. The two sampling periods were chosen to simulate short-term (early root growth) and typical duration for a growing season in Canada. Soil samples were air-dried, sieved to pass a 2-mm screen, and then ground to 0.2 mm.
The Hedley sequential extraction procedure was performed as described by Tiessen and Moir (2008) with modifications for soil digestion as proposed by Zheng et al. (2001) and used in the Fan et al. (2010) study. Thus, a 0.5-g subsample of ground soil was weighed into 50- ml centrifuge tubes and sequentially extracted according to the following scheme:
(1) Resin-P (Pi): 25 ml of water and two resin strips, shake for 16 h at 25°C, remove the strips, centrifuge, decant, and discard supernatant. P was recovered from strips in 25 ml of 0.5 M HCl.
(2) NaHCO3 (Pi and Po): 25 ml of 0.5 M NaHCO3 at pH 8.5, shake for 16 h at 25°C, centrifuge, and collect supernatant.
(3) NaOH (Pi and Po): 25 ml of 0.1 M NaOH, shake for 16 h at 25°C, centrifuge, and collect supernatant.
(4) HCl (Pi): 25 ml of 1.0 M HCl, shake for 16 h at 25°C, centrifuge, and collect supernatant.
(5) Residual P: 10 ml of 0.9 M H2SO4 and 0.5 g of K2S2O8, digestion at 121°C in an autoclave for 90 min.
The concentration of Pi in extracts and digests was determined by the ammonium molybdate–ascorbic acid method (Murphy and Riley, 1962). The concentrations of Po in each of these two extractions (NaHCO3 and NaOH) were calculated as the difference between total P determined after H2SO4–K2S2O8 digestion and Pi. Total P in soils was also determined the same way as for residual P with 0.1 g of ground soil. This allowed evaluation of the P recovery from fractionation which varied from 83 to 103% (93 ± 5%). Additionally, the soil pH (1:2 soil:water) was determined on the air-dried 2-mm samples at the end of incubation.
Total Pi was the sum of resin-P, NaHCO3-Pi, NaOH-Pi, and HCl-Pi, while total Po was the sum of NaHCO3-Po, NaOH-Po, and residual P. Usually, but not exclusively, resin-P is considered as freely available Pi; NaHCO3-P is assigned to Pi sorbed on crystalline Al and Fe oxides and to easily mineralized Po; NaOH-P is assigned to Pi sorbed to amorphous Al and Fe oxides and to stable Po; and HCl-P is considered to be Ca–P compounds (Negassa and Leinweber, 2009). According to the criteria of Bowman and Cole (1978) and Tiessen et al. (1984), the sum of resin-P and NaHCO3-P was considered as labile P, NaOH-P as moderately labile P, and HCl-P and residual P as stable P.
Biosolids Phosphorus Fractionation
Paper mill biosolids P was also fractionated before application using the same method as described for soil. Since the method was developed for mineral soils, some modifications were made considering the organic nature of the material. Thus, fresh PB (0.5 g wet basis for P fractionation and 0.1 g wet basis for total P) was used for the extraction, as suggested by Ajiboye et al. (2004). The supernatant of the resin step was kept to determine both Pi and Po (after digestion) in the water extract. No Pi was found in the water extract. Organic P in 1.0 M HCl was also determined as recommended by He et al. (2010) to provide a more accurate P characterization of the materials. Usually, this fraction is recovered in the residual P pool and has been simply assumed to be negligible. Finally, extracts of residual P and total P were digested twice to completely release Pi from materials.
Data Analysis
All data for soil incubation were checked for normality with Shapiro–Wilk. Only NaOH-Pi was transformed based on the Box–Cox model to improve the normality of distribution. Treatment effects were evaluated as a factorial of 3 × 7 replicated three times using the MIXED procedure (SAS Institute Inc., 2004) with replicates and replicates × treatments as random effects, soil types and treatments as fixed effects, and incubation time as repeated effect. Main treatment effects and their interactions were tested using differences of least squares means. The NP treatment in the CHIN soil was omitted due to the unexpected negative total Pi recovery (−10%) at the end of incubation as compared with KAM (86%) and StA (91%) soils. As a consequence, this treatment was excluded from the statistical analysis. Statistical significance was defined as P < 0.05.
Results and Discussion
Biosolids Phosphorus Fractions
Total P content in PB ranged from 1,535 to 6,904 mg kg–1, with the PB3b having the highest concentration and the PB4 showing the lowest (Table 3). The P recovery from fractionation was high, varying between 93% and 101%, in agreement with other studies performed on organic materials (79–113%; Ajiboye et al., 2004; Turner and Leytem, 2004; Gagnon et al., 2012a).
Total Po, with the exception of PB1, accounted for a large part of total P (46–66%, Table 3). This contrasts with municipal biosolids in which Pi was dominant (>71%; Sui et al., 1999; Ajiboye et al., 2004; O’Connor et al., 2004; Su et al., 2007). In this study, PBs have been mostly subjected to a secondary biological treatment for further purification and stabilization before discharge to the water bodies. This treatment increases the microbial activity and could explain the high Po proportion in such materials, a tendency also observed when sewage sludge was treated by the activated process (Frossard et al., 1996).
The relative contribution of P in each fraction varied with the material (Table 3). Resin-P (Pi + H2O-Po), the most readily available form, ranged from 2% in PB4 to 40% and 59% in PB3a and PB3b, respectively. Moreover, a significant part (around 40%) of Po was found in resin + H2O and NaHCO3 extracts for PB3a and PB3b. These fractions are considered mobile in the soil and biologically available (Turner and Leytem, 2004). Either PB3a and PB3b was treated with phosphoric acid to stimulate P degradation by microorganisms and reduce odor (Gagnon and Ziadi, 2012). Both materials came from the same source and were subjected to the same treatment process. Despite differences in total P, they were more related to each other than to other materials.
The labile P (resin + NaHCO3), which is considered plant available, was the highest with PB2, PB3a, and PB3b (44–72%). It constituted, however, only 20–26% of total P in the other two materials. In counterpart, PB1 and PB4 contained 36–40% of their P in the stable (HCl + residual) fractions. This could be attributed to their high pH, which may contribute to the formation of recalcitrant Ca phosphate minerals (Torri et al., 2017) and to the high total Al and Fe contents (Table 2), which were found to result in materials having a low-availability P concentration (Krogstad et al., 2005; Torri et al., 2017). Khiari et al. (2020) reported that biosolids with a (Fe + Al)total >1,100 mmol kg–1 were classified as medium to low for their P bioavailability and were similar to a slow-release source of P for crops. Overall, the relative distribution of P forms in PBs indicated that P in this material could constitute a good source to crops, sometimes more efficient than many municipal biosolids and composts (Sharpley and Moyer, 2000; Ajiboye et al., 2004; Xu et al., 2012). To this end, Ebeling et al. (2016) found that municipal biosolids with a biological P removal process had consistently the highest soil test P values in opposition to biosolids treated with alum or FeCl2.
Effect of Incubation on Soil pH
Variations in soil pH during incubation induced by mineralization of PB may have a large impact on the distribution and form of soil P fractions. In acidic soils, Fe and Al phosphates are more dominant, whereas in alkaline soils, Ca phosphate dominates. In this study, addition of PB4 due to its high Ca content (Table 2) induced a large increase in soil pH, 0.1 unit in the alkaline CHIN but 1.6–1.8 in the acidic KAM and StA (Table 4). On the opposite, PB3a and NP decreased the soil pH by an average of 0.2–0.3 units, while PB3b caused the largest drop in pH with 0.6 units. PB3b was the most acidifying material of all those tested (Table 2).
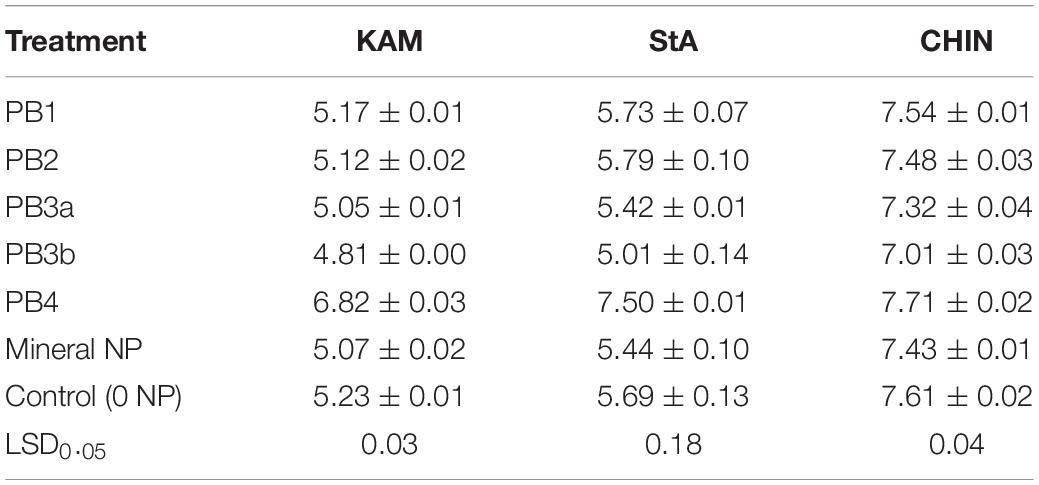
Table 4. Effect of paper mill biosolids (PBs) and mineral P fertilizer on the soil pH at the end of the 16-week incubation.
Effect of Incubation Time, Soil Type, and Biosolids on Soil Phosphorus Fractions
There were significant changes in the different P fractions with time during the incubation (Supplementary Table S1). From the beginning to week 16, there was a general increase in all Pi fractions, notably the labile forms (resin-P and NaHCO3-Pi; Supplementary Table S2). This was made at the expense of Po and residual P fractions for StA and CHIN and NaHCO3-Po and residual P for KAM. Kashem et al. (2004) investigated the forms and distribution of P after 1, 4, and 16 weeks of incubation in a silty clay loam soil and reported that the amendment with municipal biosolids caused a net conversion of Po and residual forms toward H2O-P, NaOH-Pi, and HCl-P fractions. Sui et al. (1999) and Su et al. (2007) also found a decrease in the most recalcitrant forms (HCl-P, NaOH-Po, and residual P) and a concomitant increase in labile forms (H2O-P and NaHCO3-Pi) after repeated applications of municipal biosolids. Fan et al. (2010) reported a large decrease in NaOH-Po and residual P after 6 years of continuous PB application, which was attributed to the mobility and/or mineralization of NaOH-Po and the transformation of recalcitrant P to more labile forms with time.
Soil type had a very significant effect (P < 0.001) on every soil P fraction (Supplementary Table S1). This was expected due to the significant variability in P distribution with the three soils selected in this study (Table 1). Globally, the greater P sorption capacity of KAM and StA soils made resin-P to be lower in these soils and NaOH-P associated with Al and Fe oxides to be higher as compared with CHIN (Supplementary Table S2). However, there was a soil × treatment interaction for resin-P and labile P, meaning that these P fractions behave differently depending on which soil the different PBs have been incorporated in.
Application of PB was particularly important for soil resin-P, a freely available fraction, and labile P (Supplementary Table S1). The increases reached 26 to 40 mg kg–1 in resin-P and 39 to 51 mg kg–1 in labile P (Figures 1A,B). The strongest differences were noted for resin-P in CHIN soil. Such wider range has been already observed in a preceding study evaluating different composts (Gagnon et al., 2012a) and was attributed to the relatively low potential of this soil to fix added P. The Fe and Al oxides are the most important phosphate adsorbents in soil (Parfitt, 1979; Borggaard et al., 1990), and phosphate sorption increases with decreasing pH (Lopez-Hernandez and Burnham, 1974), which can mask the differences between PBs. Conversely, application of P in soils of temperate climate was responsible for the largest variations over the years in labile P (Negassa and Leinweber, 2009). To this end, Fan et al. (2010) obtained a linear increase in resin-P and a tendency (P = 0.07) for increase in NaHCO3-Pi after 3 years of continuous application of 90 Mg wet PB ha–1 to a loamy soil.
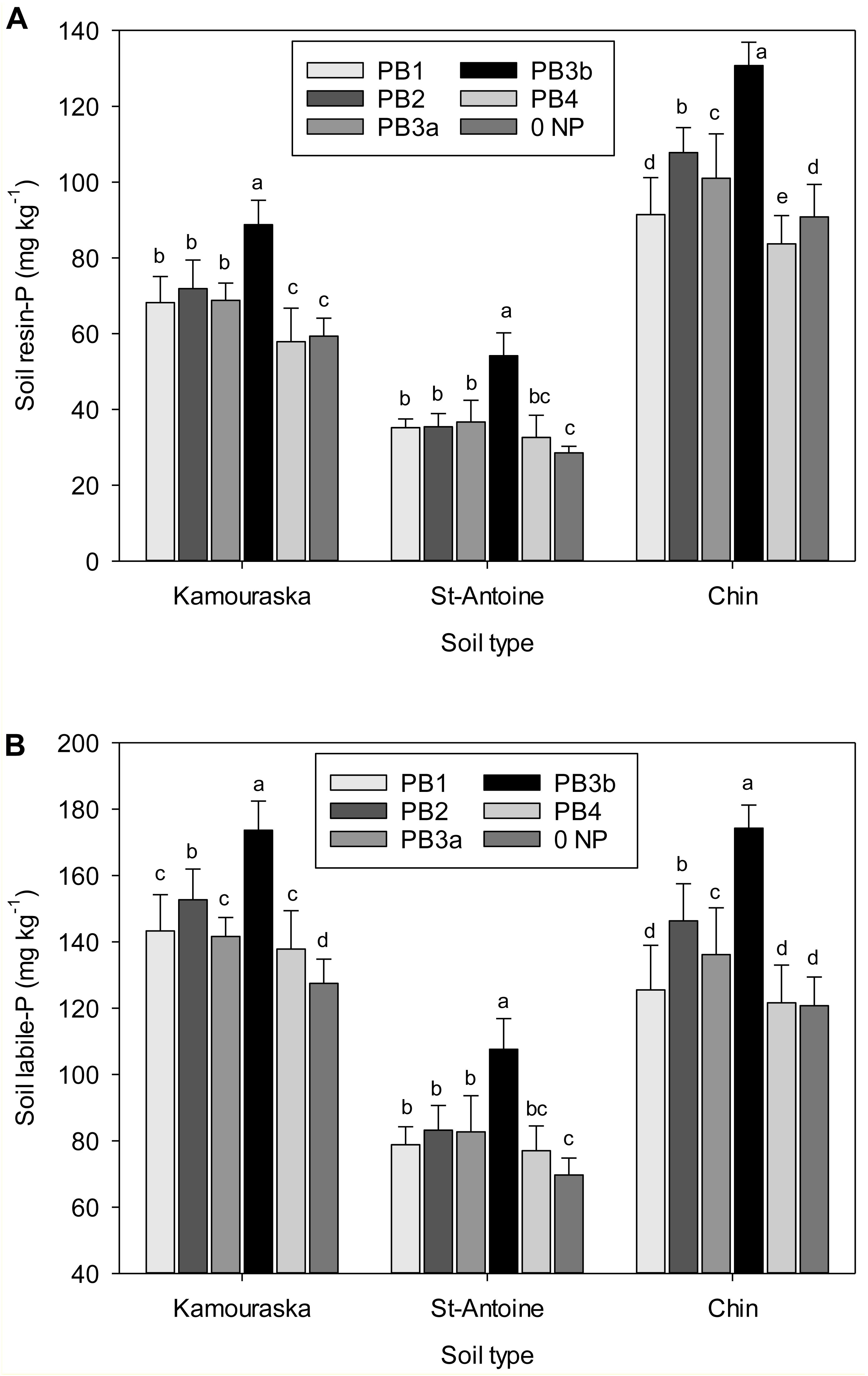
Figure 1. Effects of paper mill biosolids (PBs) addition in function of soil type on the soil (A) resin-P and (B) labile P fractions. Values (mean ± standard deviation) are averaged over time.
In this study, PB3b caused the largest increase in P concentrations in all soils (Table 5 and Figure 1A). This is expected with the supply of a high total P amount by this material (112 kg P ha–1; Table 2). In terms of total P added, PB3b (42–55%) followed by PB2 and PB3a (26–49%) was an interesting source to increase soil labile P. Unfortunately, PB1 and PB4 were relatively inefficient at releasing P in the alkaline CHIN soil (Figure 1B). Moreover, PB4 induced a shift from NaOH-Po to NaHCO3-Pi (Table 5). Accordingly, it appears that its very high Ca content (Table 2) and good liming potential (40% CaCO3 equivalent; Gagnon and Ziadi, unpublished data) promoted soil pH increase (Table 4), which may have reduced the mobility of Al (Casséus et al., 2011).
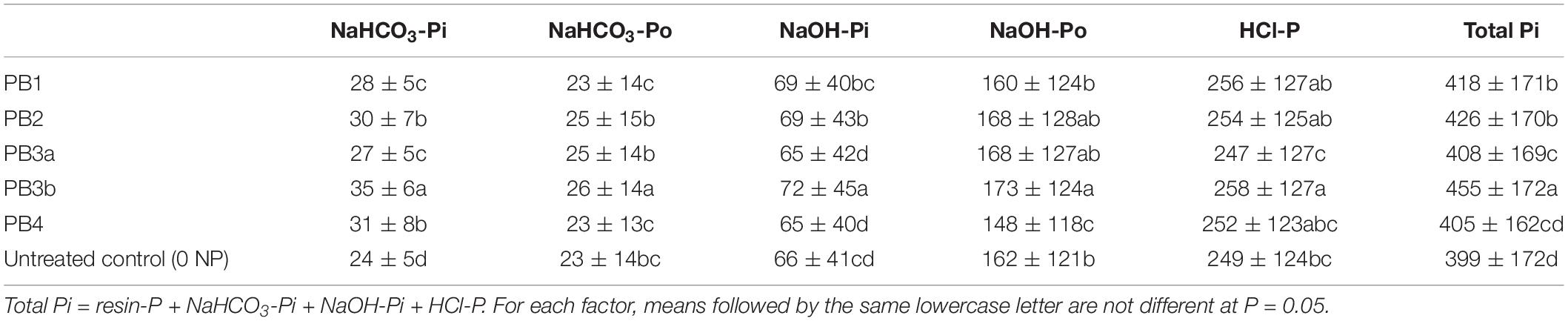
Table 5. Effects of paper mill biosolids addition on selected soil P fractions averaged over soils and time (mg kg–1 dry basis).
Despite Po forming a large proportion of total P in some of these materials (Table 3), significant effects of PB were mostly detected on Pi fractions (Supplementary Table S1). This indicated that PB had a good potential for P mineralization in the studied soils. Increases in labile Pi were also observed with municipal biosolids even if these materials comprised Pi in less soluble forms (Sui et al., 1999). The net increase in labile P (soil labile P + PB minus soil labile P in 0 NP minus labile P applied with PB) was mainly negative, particularly for PB3a and PB3b (−11 and −21%, respectively). Negative values mean that PBs served more as a direct source of P fertilizer than soil P conditioner because they did not enhance the release of P from the soil.
Considering the application rate and the forms and distribution of P in the material, the PB in this study (excluding PB4 considered more as a soil conditioner) supplied 17 (6–38) kg resin-P ha–1 and 25 (9–55) kg labile P ha–1 after the early stage of application, based on the 2-week incubation data, and a little bit more for the entire incubation (18 and 28 kg P ha–1, respectively). This covers a large part of the P requirements for corn and small cereals (26–28 kg P ha–1; CRAAQ, 2010) produced in eastern Canada in the low-P KAM and StA soils. The P availability at week 2 is important for early plant growth, particularly in temperate areas where the soil is usually cool at springtime (Grant et al., 2001). Even if this study did not include a crop test, the accompanying NP treatment can be used to assess the potential direct P contribution of PB sources by assuming that labile P measured in the NP treatment is considered to represent plant available P (Tiessen and Moir, 2008). In terms of mineral P, the P recovery as labile P represented on average 68%, with the lowest recovery with PB1 (48%) and the highest with PB3b (87%). Hence, PB could constitute an excellent source of P, more than the conventional treated sewage sludge in which application to the field indicated a much lower P availability than the commercial P fertilizer based on soil NaHCO3-P extraction and corn plant P concentration (Tian et al., 2016).
Relationships Between Phosphorus Fractions in Biosolids and Soils
Soil resin-P and labile P increases over the control were closely related to total P added at both week 2 (R2 = 0.79–0.83) and week 16 (R2 = 0.76–0.79) of the incubation (Figure 2A). This indicates a high potential for PB to be a valuable source of P for crops. Su et al. (2007) also reported an increase in these fractions with municipal biosolids addition to a sandy forest soil. Soil labile P increases were also related to the amount of PO4-P added (Figure 2B). Total P concentration and water-extractable P have been reported as the best indicators for predicting P release from composts and manures (Sharpley and Moyer, 2000; Zvomuya et al., 2006). In this study, a relationship could be established between PO4-P concentration and pH of materials, all determined by water extraction (R2 = 0.53). This indicates that total P and pH might be selected as controlling variables to estimate P contribution from PB.
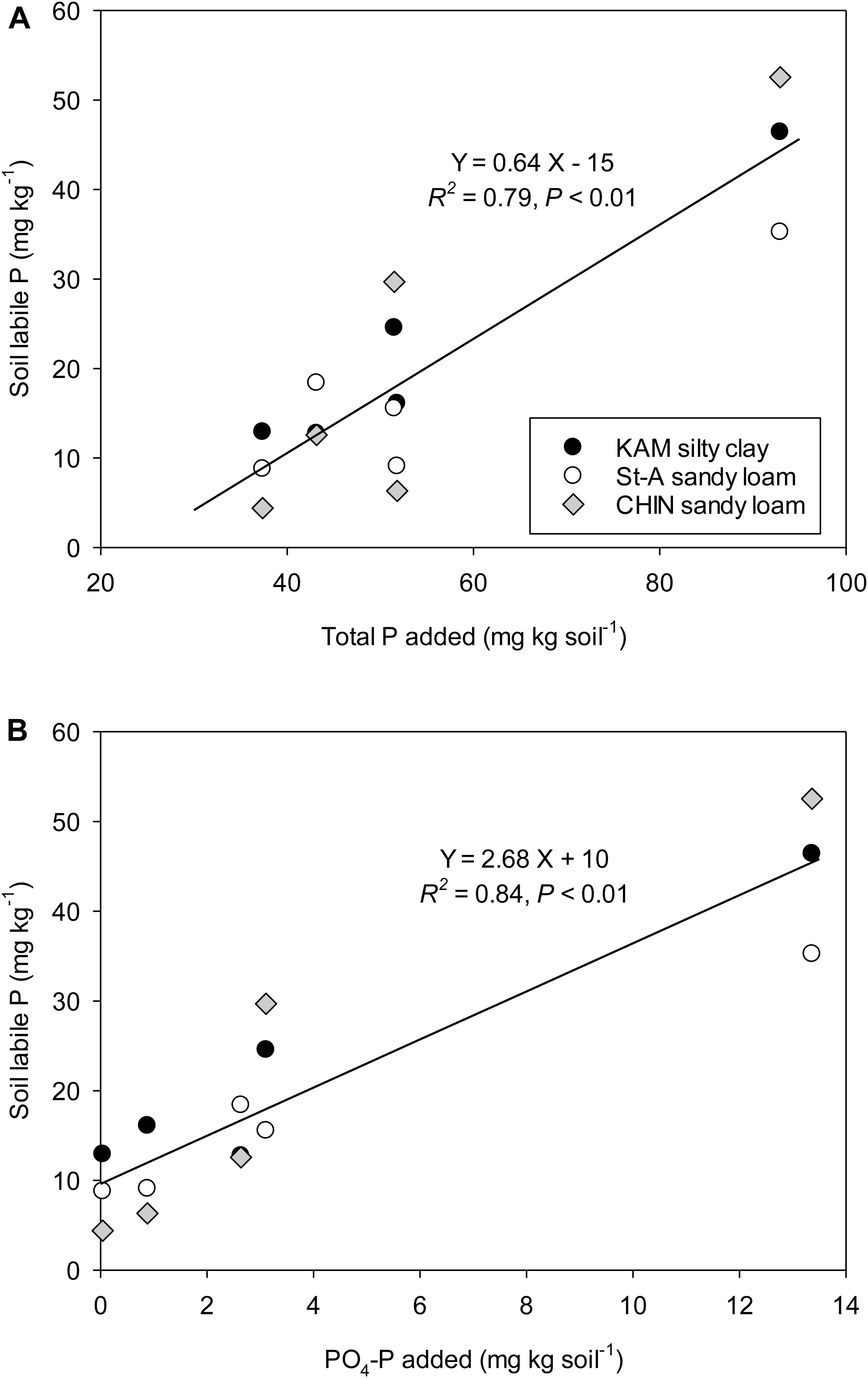
Figure 2. Relationship between total P (A) and PO4-P (B) added by the paper mill biosolids and the net increase (treated soil minus control) in soil labile P after 16 weeks of incubation.
When expressed on a total P basis, close relationships were obtained between net increases in soil resin-P and labile P and a proportion of these fractions in biosolids at both 2 and 16 weeks (Supplementary Table S3). There was also a negative correlation between soil resin-P and labile P and materials with the most recalcitrant (NaOH-P, HCl-P, and residual) P fractions. Besides the magnitude of labile P, which can be used for evaluating potential environmental impact (Sharpley and Moyer, 2000; Ajiboye et al., 2004) and plant P bioavailability (Zvomuya et al., 2006), the fate of P of the organic amendments once applied to soil has always been hard to predict. Despite several attempts, the biogeochemistry of the soil plays a role in the fixation of phosphate ions by Al and Fe oxides in acidic soil and by Ca ions in alkaline soil and causes a redistribution of the different P forms with time.
Conclusion
This study revealed that PB could potentially be an efficient P source for fertilizing crops based on our soil P test. These materials generally contained a high percentage of freely available resin-P and labile P and low Al and Fe contents, which enhanced the P availability in soil. The increases in soil labile P, which is considered plant available, may represent up to 50% of total P added and can even reach amounts obtained with the mineral P fertilizer. All soils can benefit from application of PB, at least in the short term for the development of young plants. However, the total P content and P forms varied largely, even within the same production plant. These wide variations could therefore translate into differences in PB response when applied in field and various beneficial P effects on crops.
Data Availability Statement
All datasets generated for this study are included in the article/Supplementary Material.
Author Contributions
XZ, a Ph.D. student under the supervision of NZ and ZH, did all the lab work, performed all calculations and statistical analyses, and wrote a first version of this manuscript. BG conducted the incubation study, validated statistical analyses, and contributed to the results and discussion. NZ initiated the study, supervised the Ph.D. student, and contributed to the whole manuscript. AC discussed and validated the statistical analyses and revised and commented on the manuscript. KA and ZH revised and commented on the manuscript.
Funding
This study was supported by an Agriculture and Agri-Food Canada (AAFC) A base program.
Conflict of Interest
The authors declare that the research was conducted in the absence of any commercial or financial relationships that could be construed as a potential conflict of interest.
Acknowledgments
The authors would like to thank Haixiao Li and Guoqi Wen for their comments on a first version of the manuscript and Sylvie Côté and Claude Lévesque for their technical assistance. The authors also extend their appreciation to the International Scientific Partnership Program (ISPP) at King Saud University for supporting KA’s collaboration with AAFC through ISPP-128. XZ is the recipient of a scholarship from the China Scholarship Council.
Supplementary Material
The Supplementary Material for this article can be found online at: https://www.frontiersin.org/articles/10.3389/fenvs.2020.00038/full#supplementary-material
References
Ajiboye, B., Akinremi, O. O., and Racz, G. J. (2004). Laboratory characterization of phosphorus in fresh and oven-dried organic amendments. J. Environ. Qual. 33, 1062–1069. doi: 10.2134/jeq2004.1062
Al-Dhumri, S., Beshah, F. H., Porter, N. A., Meehan, B., and Wrigley, R. (2013). An assessment of the guidelines in Victoria, Australia, for land application of biosolids based on plant-available nitrogen. Soil. Res. 51, 529–538. doi: 10.1071/SR13191
Alleoni, L. R. F., Fernandes, A. R., and Correia, B. L. (2012). Sequential extraction of phosphorus in an Oxisol amended with biosolids in a long-term field experiment in Brazil. Agric. Ecosyst. Environ. 161, 145–151. doi: 10.1016/j.agee.2012.07.029
Borggaard, O. K., Jørgensen, S. S., Møberg, J. P., and Raben-Lange, B. (1990). Influence of organic matter on phosphate adsorption by aluminium and iron oxides in sandy soils. J. Soil Sci. 41, 443–449. doi: 10.1111/j.1365-2389.1990.tb00078.x
Bowman, R. A., and Cole, C. V. (1978). Transformations of organic phosphorus substrates in soils as evaluated by NaHCO3 extraction. Soil Sci. 125, 49–54. doi: 10.1097/00010694-197801000-00008
Cabral, F., Vasconcelos, E., Goss, M. J., and Cordovil, C. M. S. (1998). The value, use, and environmental impacts of pulp-mill sludge additions to forest and agricultural lands in Europe. Environ. Rev. 6, 55–64. doi: 10.1139/a98-004
Camberato, J. J., Gagnon, B., Angers, D. A., Chantigny, M. H., and Pan, W. L. (2006). Pulp and paper mill by-products as soil amendments and plant nutrient sources. Can. J. Soil Sci. 86, 641–653. doi: 10.4141/S05-120
Casséus, L. M. C., Karam, A., and Parent, L.-E. (2011). Effect of liming on the distribution of aluminum and aluminum uptake by maize grown in a coarse textured soil. Paper Presented at the 11th International Multidisciplinary Scientific Geoconference SGEM 2011, Bulgaria.
Charbonneau, H., Hébert, M., and Jaouich, A. (2001). Portrait de la valorisation agricole des matières résiduelles fertilisantes au Québec – partie 2: contenu en éléments fertilisants et qualité environnementale. Vecteur Environ. 34, 56–60.
Cogger, C. G., Forge, T. A., and Neilsen, G. H. (2006). Biosolids recycling: nitrogen management and soil ecology. Can. J. Soil Sci. 86, 613–620. doi: 10.4141/S05-117
Cordell, D., Drangert, J. O., and White, S. (2009). The story of phosphorus: global food security and food for thought. Glob. Environ. Change 19, 292–305. doi: 10.1016/j.gloenvcha.2008.10.009
CRAAQ (2010). Guide de Référence en Fertilisation, 2nd Edn, Québec, QC: Centre de Référence en Agriculture et Agroalimentaire du Québec.
Ebeling, A. M., Spaciel, S., McLain, H., Klug, A., and Sheahan, C. (2016). Different biosolids, different phosphorus availabilities. Paper Presentation at the 2016 ASA, CSSA and SSSA International Annual Meeting, Phoenix, AZ.
Fan, J. L., Ziadi, N., Gagnon, B., and Hu, Z. (2010). Soil phosphorus fractions following annual paper mill biosolids and liming materials application. Can. J. Soil Sci. 90, 467–479. doi: 10.4141/CJSS09037
Frossard, E., Sinaj, S., and Dufour, P. (1996). Phosphorus in urban sewage sludges as assessed by isotopic exchange. Soil Sci. Soc. Am. J. 60, 179–182. doi: 10.2136/sssaj1996.03615995006000010029x
Gagnon, B., Demers, I., Ziadi, N., Chantigny, M. H., Parent, L.-E., Forge, T. A., et al. (2012a). Forms of phosphorus in composts and in compost-amended soils following incubation. Can. J. Soil Sci. 92, 711–721. doi: 10.4141/cjss2012-032
Gagnon, B., Ziadi, N., Chantigny, M. H., Bélanger, G., and Massé, D. I. (2012b). Biosolids from treated swine manure and papermill residues affect corn fertilizer value. Agron. J. 104, 483–492. doi: 10.2134/agronj2011.0327
Gagnon, B., and Ziadi, N. (2012). Papermill biosolids and alkaline residuals affect crop yield and soil properties over nine years of continuous application. Can. J. Soil Sci. 92, 917–930. doi: 10.4141/cjss2012-026
Gagnon, B., Ziadi, N., Côté, C., and Foisy, M. (2010). Environmental impact of repeated applications of combined paper mill biosolids in silage corn production. Can. J. Soil Sci. 90, 215–227. doi: 10.4141/CJSS09055
Grant, C. A., Flaten, D. N., Tomasiewicz, D. J., and Sheppard, S. C. (2001). The importance of early season phosphorus nutrition. Can. J. Plant Sci. 81, 211–224. doi: 10.4141/P00-093
He, Z., Zhang, H., Toor, G. S., Dou, Z., Honeycutt, C. W., Haggard, B. E., et al. (2010). Phosphorus distribution in sequentially extracted fractions of biosolids, poultry litter, and granulated products. Soil Sci. 175, 154–161. doi: 10.1097/SS.0b013e3181dae29e
Henry, C. L., Cole, D. W., Hinckley, T. M., and Harrison, R. B. (1994). The use of municipal and pulp and paper sludges to increase production in forestry. J. Sustainable For. 1, 41–55. doi: 10.1300/J091v01n03_04
Ippolito, J. A., Barbarick, K. A., and Norvell, K. L. (2007). Biosolids impact soil phosphorus accountability, fractionation, and potential environmental risk. J. Environ. Qual. 36, 764–772. doi: 10.2134/jeq2006.0308
Isaac, R. A., and Johnson, W. C. (1976). Determination of total nitrogen of plant tissue, using a block digestor. J. Assoc. Off. Anal. Chem. 59, 98–100.
Kashem, M. A., Akinremi, O. O., and Racz, G. J. (2004). Phosphorus fractions in soil amended with organic and inorganic phosphorus sources. Can. J. Soil Sci. 84, 83–90. doi: 10.4141/S03-018
Kelling, K. A., Walsh, L. M., Keeney, D. R., Ryan, J. A., and Peterson, A. E. (1977). A field study of the agricultural use of sewage sludge: II. Effect on soil N and P. J. Environ. Qual. 6, 345–352. doi: 10.2134/jeq1977.00472425000600040003x
Khiari, L., Joseph, C.-A., Gallichand, J., Beecher, N., and Bouslama, S. (2020). Classification and assessment models of first year biosolids phosphorus bioavailability. Waste Biomass Valor 11, 1443–1452. doi: 10.1007/s12649-018-0490-8
Krogstad, T., Sogn, T. A., Asdal, A., and Saebø, A. (2005). Influence of chemically and biologically stabilized sewage sludge on plant-available phosphorous in soil. Ecol. Eng. 25, 51–60. doi: 10.1016/j.ecoleng.2005.02.009
Lopez-Hernandez, D., and Burnham, C. P. (1974). The effect of pH on phosphate adsorption in soils. J. Soil Sci. 25, 207–216. doi: 10.1111/j.1365-2389.1974.tb01117.x
Maguire, R. O., Sims, J. T., Dentel, S. K., Coale, F. J., and Mah, J. T. (2001). Relationships between biosolids treatment process and soil phosphorus availability. J. Environ. Qual. 30, 1023–1033. doi: 10.2134/jeq2001.3031023x
MDDELCC (2016). Bilan Annuel de Conformité Environnementale 2013: Secteur des Pâtes et Papiers. Québec, QC: Ministère du Développement durable.
Miller, D. M., Miller, W. P., Dudka, S., and Sumner, M. E. (2000). “Characterization of industrial by-products,” in Land Application of Agricultural, Industrial, and Municipal By-products, ed. W. A. Dick (Madison, WI: Soil Science Society of America), 107–126.
Monte, M. C., Fuente, E., Blanco, A., and Negro, C. (2009). Waste management from pulp and paper production in the European Union. Waste Manage. 29, 293–308. doi: 10.1016/j.wasman.2008.02.002
Murphy, J., and Riley, J. P. (1962). A modified single solution method for the determination of phosphates in natural waters. Anal. Chem. Acta 27, 31–36. doi: 10.1016/S0003-2670(00)88444-5
Negassa, W., and Leinweber, P. (2009). How does the Hedley sequential phosphorus fractionation reflect impacts of land use and management on soil phosphorus: a review. J. Plant Nutr. Soil Sci. 172, 305–325. doi: 10.1002/jpln.200800223
Nurmesniemi, H., Pöykiö, R., and Keiski, R. L. (2007). A case study of waste management at the Northern Finnish pulp and paper mill complex of Stora Enso Veitsiluoto Mills. Waste Manage. 27, 1939–1948. doi: 10.1016/j.wasman.2006.07.017
O’Connor, G. A., Sarkar, D., Brinton, S. R., Elliott, H. A., and Martin, F. G. (2004). Phytoavailability of biosolids phosphorus. J. Environ. Qual. 33, 703–712. doi: 10.2134/jeq2004.7030
Parfitt, R. L. (1979). Anion adsorption by soils and soil materials. Adv. Agron. 30, 1–50. doi: 10.1016/S0065-2113(08)60702-6
Pellerin, A., Parent, L. E., Fortin, J., Tremblay, C., Khiari, L., and Giroux, M. (2006). Environmental Mehlich-III soil phosphorus saturation indices for Quebec acid to near neutral mineral soils varying in texture and genesis. Can. J. Soil Sci. 86, 711–723. doi: 10.4141/S05-070
Penn, C. J., and Sims, J. T. (2002). Phosphorus forms in biosolids-amended soils and losses in runoff: effects of wasterwater treatment processes. J. Environ. Qual. 31, 1349–1361. doi: 10.2134/jeq2002.1349
Pierzynski, G. M., and Gehl, K. A. (2005). Plant nutrient issues for sustainable land application. J. Environ. Qual. 34, 18–28. doi: 10.2134/jeq2005.0018
Rashid, M. T., Barry, D., and Goss, M. (2006). Paper mill biosolids application to agricultural lands: benefits and environmental concerns with special reference to situation in Canada. Soil Environ. 25, 85–98.
Sadras, V. O. (2006). The N:P stoichiometry of cereal, grain legume and oilseed crops. Field Crops Res. 95, 13–29. doi: 10.1016/j.fcr.2005.01.020
Sharpley, A., and Moyer, B. (2000). Phosphorus forms in manure and compost and their release during simulated rainfall. J. Environ. Qual. 29, 1462–1469. doi: 10.2134/jeq2000.00472425002900050012x
Shober, A. L., Hesterberg, D. L., Sims, J. T., and Gardner, S. (2006). Characterization of phosphorus species in biosolids and manures using XANES spectroscopy. J. Environ. Qual. 35, 1983–1993. doi: 10.2134/jeq2006.0100
Shober, A. L., and Sims, J. T. (2003). Phosphorus restrictions for land application of biosolids: current status and future trends. J. Environ. Qual. 32, 1955–1964. doi: 10.2134/jeq2003.1955
Shu, W., Price, G. W., Sharifi, M., and Cade-Menun, B. J. (2016). Impact of annual and single application of alkaline treated biosolids on soil extractable phosphorus and total phosphorus. Agric. Ecosyst. Environ. 219, 111–118. doi: 10.1016/j.agee.2015.12.009
Su, J., Wang, H., Kimberley, M. O., Beecroft, K., Magesan, G. N., and Hu, C. (2007). Fractionation and mobility of phosphorus in a sandy forest soil amended with biosolids. Environ. Sci. Pollut. Res. 14, 529–535. doi: 10.1065/espr2007.08.443
Sui, Y., Thompson, M. L., and Shang, C. (1999). Fractionation of phosphorus in a Mollisol amended with biosolids. Soil Sci. Soc. Am. J. 63, 1174–1180. doi: 10.2136/sssaj1999.6351174x
Thacker, B. (2007). Management of Byproduct Solids Generated in the Pulp and Paper Industry. Washington, DC: EPA.
Tian, G., Cox, A. E., Kumar, K., Granato, T. C., O’Connor, G. A., and Elliott, H. A. (2016). Assessment of plant availability and environmental risk of biosolids-phosphorus in a U.S. Midwest corn-belt soil. J. Environ. Manage. 172, 171–176. doi: 10.1016/j.jenvman.2016.02.031
Tiessen, H., and Moir, J. O. (2008). “Characterization of available P by sequential extraction,” in Soil Sampling and Methods of Analysis, 2nd Edn, eds M. R. Carter and E. G. Gregorich (Boca Raton, FL: Lewis Publishers), 293–306.
Tiessen, H., Stewart, J. W. B., and Cole, C. V. (1984). Pathways of phosphorus transformations in soils of differing pedogenesis. Soil Sci. Soc. Am. J. 48, 853–858. doi: 10.2136/sssaj1984.03615995004800040031x
Torri, S. I., Corrêa, R. S., and Renella, G. (2017). Biosolid application to agricultural land – a contribution to global phosphorus recycle: a review. Pedosphere 27, 1–16. doi: 10.1016/S1002-0160(15)60106-0
Turner, B. L., and Leytem, A. B. (2004). Phosphorus compounds in sequential extracts of animal manures: chemical speciation and a novel fractionation procedure. Environ. Sci. Technol. 38, 6101–6108. doi: 10.1021/es0493042
Wang, H., Magesan, G. N., Kimberley, M. O., Payn, T. W., Wilks, P. J., and Fisher, C. R. (2004). Environmental and nutritional responses of a Pinus radiata plantation to biosolids application. Plant Soil 267, 255–262. doi: 10.1007/s11104-005-0107-4
Wang, Y. T., Zhang, T. Q., O’Halloran, I. P., Hu, Q. C., Tan, C. S., Speranzini, D., et al. (2015). Agronomic and environmental soil phosphorus tests for predicting potential phosphorus loss from Ontario soils. Geoderma 241–242, 51–58. doi: 10.1016/j.geoderma.2014.11.001
Xu, H., Zhang, H., Shao, L., and He, P. (2012). Fraction distributions of phosphorus in sewage sludge and sludge ash. Waste Biomass Valor. 3, 355–361. doi: 10.1007/s12649-011-9103-5
Zheng, Z., Simard, R. R., Lafond, J., and Parent, L. E. (2001). Changes in phosphorus fractions of a humic gleysol as influenced by cropping systems and nutrient sources. Can. J. Soil Sci. 81, 175–183. doi: 10.4141/S00-666
Keywords: phosphorus, paper mill biosolids, soil incubation, phosphorus fractions, phosphorus availability
Citation: Zhang X, Gagnon B, Ziadi N, Cambouris AN, Alotaibi KD and Hu Z (2020) Soil Phosphorus Fractionation as Affected by Paper Mill Biosolids Applied to Soils of Contrasting Properties. Front. Environ. Sci. 8:38. doi: 10.3389/fenvs.2020.00038
Received: 21 December 2019; Accepted: 17 March 2020;
Published: 23 April 2020.
Edited by:
Paulo Sergio Pavinato, University of São Paulo, BrazilReviewed by:
Leônidas Carrijo Azevedo Melo, Universidade Federal de Lavras, BrazilRoland Bol, Helmholtz Association of German Research Centres (HZ), Germany
Copyright © 2020 Zhang, Gagnon, Ziadi, Cambouris, Alotaibi and Hu. This is an open-access article distributed under the terms of the Creative Commons Attribution License (CC BY). The use, distribution or reproduction in other forums is permitted, provided the original author(s) and the copyright owner(s) are credited and that the original publication in this journal is cited, in accordance with accepted academic practice. No use, distribution or reproduction is permitted which does not comply with these terms.
*Correspondence: Noura Ziadi, bm91cmEuemlhZGlAY2FuYWRhLmNh