- 1Natural Capital Singapore, Singapore ETH Centre, ETH Zürich, Zürich, Singapore
- 2Campus for Research Excellence and Technological Enterprise, Singapore, Singapore
- 3School of Design and Environment, National University of Singapore, Singapore, Singapore
Rapid urbanization in many parts of the world has increasingly put the environment under pressure, with natural landscapes cleared to make way for built infrastructure. Urban ecosystems, and the services that they provide, can offer nature-based solutions to the challenges of urbanization. There is increasing interest in better incorporating ecosystems into urban planning and design in order to deliver greater provision of ecosystem services and enhance urban liveability. However, there are few examples of built or proposed urban developments that have been designed specifically with ecosystem services in mind–partly because there are few modeling tools available to support urban planners and designers by informing their design workflows. Through using Singapore’s latest nature-centric town as a case study, this article assesses the impacts of nature-based solutions in urban design on ecosystem services performance, through a spatially explicit modeling approach. The proposed future scenario for the nature-centric town was projected to result in substantial declines in the provision of all ecosystem services, as a result of the removal of large areas of natural vegetation cover. However, the future scenario compared favourably against three older towns that have been constructed in Singapore, showing the best performance for four out of six ecosystem services. This simulation exercise indicates that designing towns with ecosystem services in mind, and incorporating nature-based solutions into urban design, can help to achieve enhanced performance in providing ecosystem services. The models developed for this study have been made publicly available for use in other tropical cities.
Introduction
The world is urbanizing at an unprecedented rate, with the United Nations projecting that 68% of the world’s population will be living in urban areas by 2050 (United Nations, 2018). While urbanization has brought about significant benefits for humankind, it has also brought detrimental impacts to the environment and the ecosystem services provided (Seto et al., 2011). Urban areas face a range of pressing environmental and societal problems, such as the urban heat island (Arnfield, 2003; Richards and Edwards, 2017), poor air quality (Shi et al., 2020), elevated flood risk (Chadwick et al., 2006), and a lack of public access to nature (Cox et al., 2018). There is an increasing awareness that humans need to make better use of ecosystem services in order to regulate and sustain a healthy planet (Zhang et al., 2019), particularly in urban areas (Bolund and Hunhammar, 1999; Gómez-Baggethun and Barton, 2013; Elmqvist et al., 2015; Richards and Thompson, 2019). Through designing greener urban areas with contrasting types of green spaces, it may be possible to provide nature-based solutions to some of the challenges of urbanization (Depietri and McPhearson, 2017; Babí Almenar et al., 2021).
Despite the significant impact that urban designs have on the ecosystem services provided by a landscape, efforts to design urban areas with ecosystem services performance in mind have often been inadequate or overlooked (Heyman et al., 2019). Even in cases where urban designs have attempted to improve ecosystem services, a lack of simulation tools has prevented quantitative feedback between ecologists and architects, to determine whether such designs are likely to perform as intended (Andreu and Oreszczyn, 2004). There is thus a need for iterative feedback loops to be instituted in order to ensure that urban designs can meet local and global environmental sustainability needs (Richards et al., 2019). In addition, there is a need to evaluate the effectiveness of new, “nature-based” urban design scenarios in contributing to ecosystem services performance (Richards et al., 2019).
Singapore is a world leader in urban greening (Construction Climate Challenge, 2019), and over the past few years has increasingly formulated urban designs with the intention of increasing urban ecosystem services. Urban designers are now adopting “nature-based solutions,” defined as interventions that use nature to provide solutions to climate mitigation and adaptation challenges (Nesshöver et al., 2017). In particular, nature-based solutions that have been implemented in Singapore include the implementation of green and blue infrastructures, which refer to “natural, semi-natural and man-made green and blue features” (Nesshöver et al., 2017), and ecological engineering techniques, which design systems that integrate the human environment into its natural environment (Nesshöver et al., 2017). Such nature-based solutions have been adopted into planning frameworks under several initiatives, for example the Green Towns Program initiated by the Housing and Development Board (HDB). The HDB is Singapore’s major residential developer, providing housing for around 80% of the population (Ng, 2017). The Green Towns Program is guided by a Biophilic Town Framework that aims to increase the interconnectedness between developments and nature, and sets targets for the quantity and quality of urban greenery that should be incorporated into developments (HDB, 2018). Another example of an initiative that is aimed at encouraging the uptake of nature based solutions is the “Active, Beautiful, Clean” Waters (ABC Waters) Program that is implemented by Singapore’s Public Utilities Board (PUB). This program aims to create aesthetic and functional blue spaces in neighbourhoods, that regulate water supply and flow, and allow residents to benefit from the cultural ecosystem services provided by waterbodies (Lim and Lu, 2016; Public Utilities Board, 2016).
While Singapore is a pioneer in urban greening, the incorporation of nature into built environments has also been gaining interest worldwide. At the global policy level, the Sustainable Development Goals refer to enhancing the vegetation of cities to enable sustainable and just future growth (United Nations Economic and Social Council, 2016). Specific examples of projects underway currently include the Hafner-Ring in Konstanz, Germany, which aims to embrace the existing conditions of the natural landscape and utilizes natural green and blue features in an iconic “Green Ribbon” to enhance social cohesion and ecological resilience and functioning of the area (Rambol Studio Dreiseitl, 2019). Similarly, the Jinga Eco-community is another urban planning and design proposal that uses nature-based solutions in response to increasing urbanization in Abuja, the capital city of Nigeria (Skidmore and Merrill, 2021b). The proposal aims to safeguard and enhance the existing ecosystems in the site by proposing a development that is lush green and connects the Usuma reservoir and an existing city. Despite the increasing implementation of nature-based solutions in urban design, little is known about the effectiveness of such designs in comparison to pre-development natural ecosystems or older design paradigms. The reason is twofold: 1) there are still relatively few large-scale nature-centric urban design projects, and many of the existing development projects have not been built yet, and 2) there is a lack of analytical simulation tools to simulate the ecosystem service performance of such projects.
The aim of this study is to investigate the potential ecosystem service impacts of an urban design that is intended to enhance the provision of ecosystem services. We use the case study example of Singapore’s forthcoming Tengah town, which is the latest town to be designed under HDB’s Green Town Program. The Tengah development has been marketed as “Tengah, the Forest Town,” highlighting its ecological design vision (HDB, 2016). The specific objectives of this study are to (1) compare the modeled ecosystem service provision of the proposed Tengah development against the current pre-development ecosystem services of this area, and (2) compare the ecosystem service provision of the proposed development with three older towns in Singapore that were not designed with a specific focus on urban greening or ecosystem services. We analyzed indicators of six ecosystem services using a spatially explicit modeling approach, and have released the six ecosystem service models as a publicly available software package for the R programming language (Richards et al., 2021).
Methods
Tengah Town Project Description
Located in the western region of Singapore and spanning 700 ha in size, Tengah town will be the next town to be developed in Singapore (Heng, 2016). The region is currently dominated by secondary rainforest, which has been abandoned for several decades (Gaw et al., 2019; Yee et al., 2015). The design concept for Tengah town was largely influenced by its history as a former Kampung1 with abundant plantations and lush vegetation, thereby inspiring town planners to place greenery as central to Tengah’s development (Heng, 2017). The development has also been guided by HDB’s Biophilic Town Framework, which aims to heighten the interconnectedness of development and nature, and increase the benefits that landscapes and residents can receive from natural ecosystems (HDB, 2018; Wong, 2018). Following three years of planning, HDB formally announced the official masterplan for Tengah in 2016, dubbing Tengah as Singapore’s first ‘Forest Town’ that will house around 42,000 new homes (HDB, 2016; Yeo, 2016).
The new Tengah town features the adoption of several nature-based solutions in its proposed development. Ecological principles are featured in the design of the Tengah Nature Way, a 5 km long and 100 m wide forest corridor that is aimed at increasing ecological connectivity between the neighboring biodiversity hotspots of the Western Water Catchment Area, Bukit Timah Nature Reserve and the Central Catchment Nature Reserve (Chan, 2013; HDB, 2016). This corridor will be landscaped using tropical rainforest trees to create a rich forest habitat (HDB, 2016). Hiking trails have also been integrated to bring the community closer to nature (HDB, 2016). A forest fringe is seen to run along the perimeter of Tengah, enveloping Tengah within a forest habitat (Heng, 2016). Running through the center of Tengah, green and blue infrastructures can also be found–notably, a 20 ha large “Central Park” that will provide residents with a place for recreation, as well as feature ponds and canals to regulate stormwater flow and grant residents easy access to blue spaces (HDB, 2016).
The Tengah town will be segmented into a business district called the Jurong Innovation District (JID), a Market Place District that features Tengah’s town center, as well as five unique residential districts, each possessing a specific theme surrounding the concepts of community and nature, while still aligning closely with the overarching theme of a “Forest Town” (Choo, 2018; Figure 1). Plantation District, for instance, adopts the theme of “Community Farming” and will feature Community Farmways, which are plots of land that are made available for community farming (Choo, 2018; HDB, 2020a). Facilities to allow for “farm-to-table” dining and farmers’ markets for residents to sell their own home-grown produce are also part of HDB’s plans in developing the Plantation District (HDB, 2020a). The other four residential districts include the Garden District, Brickland District, Forest Hill District and Park District (Figure 1).
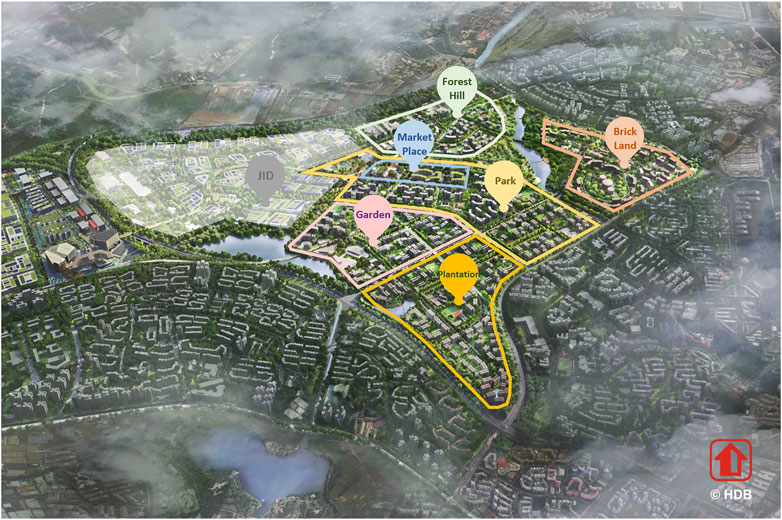
FIGURE 1. Artist’s impression of the proposed Tengah Town, showing separate districts (HDB, 2020a).
Current Urban Characteristics of Tengah and Comparison Towns
To run spatially explicit urban ecosystem service models requires a number of data layers that describe key ecological, physical, and social characteristics of the landscape (Schröter et al., 2014; Baró et al., 2016). Singapore has a wealth of such datasets for the current situation at a national scale, which were used to run the baseline ecosystem services assessment for Tengah, and the comparison assessments for older towns. Existing datasets for Singapore include maps of ecosystem cover (Gaw et al., 2019), topography (Dissegna et al., 2019), vegetation height (Dissegna et al., 2019), leaf area index (LAI) (Richards and Wang, 2020), building locations and heights (Dissegna et al., 2019), the locations of publicly accessible nature spaces (Richards et al., 2020a), and the road network (OpenStreetMap, 2020).
In addition to these data layers, we collated new data to fulfill other key parameters. The normalized-difference vegetation index (NDVI) was mapped over the study area using satellite images from the Sentinel 2 satellite (Richards and Wang, 2020). To remove artifacts due to cloud cover and temporal variation in NDVI, the median NDVI from all cloud-free Sentinel 2 images taken between the 1st of January 2016 and 30th of March 2018 was composited in Google Earth Engine (Gorelick et al., 2017; Richards and Wang, 2020).
Population data for Singapore are available at the level of planning region sub-zones, which are equivalent to large neighbourhoods (Tan and Samsudin, 2017). To estimate the population at a finer scale of resolution, we combined the sub-zone population dataset with a dataset of building locations and volume. These two layers were combined using an approach similar to dasymetric mapping, which is a commonly used technique for increasing the resolution of population and other datasets (Mennis, 2003; Holt et al., 2004). Population data of Singapore residents (Citizens and Permanent Residents) from June 2016 were downloaded from the data. gov.sg archive (Singstat, 2016). Building data were taken from a dataset originally sourced from OpenStreetMap (OpenStreetMap, 2020), but with additional digitization to include all buildings in Singapore in approximately 2017 (Dissegna et al., 2019). This dataset also included the height of all buildings, by cross-referencing with a high resolution digital surface model (Dissegna et al., 2019). To identify the likely residential buildings in Singapore, we first cross-referenced the buildings dataset to include only those buildings that were located within “residential,” “residential with commercial at first storey,” and “residential/institution” land use classes as defined on the 2008 Singapore Master Plan. Despite the older date of this dataset, it is expected that most regions have not changed in residential land use. Within the buildings located in residential regions, we excluded very large buildings as these are unlikely to be residential. Very large buildings were defined as those with a building volume (area × height) greater than the 99th percentile (124910.1 m2). The population within each subzone was shared among all residential buildings falling within the subzone, in proportion to the volume of each building. We therefore assume that within each residential neighborhood, each resident occupies an equal living volume.
Projected Urban Impacts of Future Development at Tengah
At the time of the study, the exact plans for buildings and infrastructure within the new Tengah town were yet to be finalized. We thus developed a systematic method to collate the available information regarding this proposed development, and integrate it into a format comparable to our mapping of the current situation outlined in Current Urban Characteristics of Tengah and Comparison Towns. Hence, for the purposes of this study, placement of physical structures and landscape elements within the town was estimated with reference to limited available information, such as artists’ renderings, and our interpretation of this available information. The results of this study therefore do not constitute an expectation of the final ecosystem service study provided in the Tengah Forest Town, but an estimate based on a hypothetical scenario created from our current understanding of likely future development at the site. The following sections provide a brief overview of how the ecosystem coverage and landscape parameters of Tengah town were estimated and simulated. For a more comprehensive description of this process, the detailed methods can be found in Section 1.1 and 1.2 of the Supplementary Material. An overview of the workflow used to generate this potential scenario is given in Figure 2.
Projected Ecosystem Coverage for Proposed Tengah Development
The outline design of the main roads, parcels, and land uses was digitized from the Singapore Masterplan 2019, which outlines the expected development proposed over the next five years (URA, 2020a). Land use categories found within the new Tengah town, according to the updated 2019 Masterplan, can be found in Figure 3.
To simulate how Tengah might appear after development, elements such as buildings, roads and vegetation were digitized into the land use categories to create a simulated town plan. These elements were digitized with close reference to a range of artist impressions and site plans for each land use type to achieve the most accurate representation of Tengah town. The final digitized town map created can be found in Figure 4.
The simulated Tengah town plans were reclassified to match the ecosystem land cover categories specified in Singapore’s national ecosystem cover map (Gaw et al., 2019), to facilitate comparable ecosystem service modeling. The various ecosystem land cover types found in each land use type can be found in Table 1.
With reference to Table 1, several assumptions were made during the categorization process. The first assumption was that all reserve sites would be classified as having only ‘Managed Shrub/Turf’ present, with little to no tree cover. This is in accordance with what has been observed in other reserve sites in HDB New Towns. The second assumption is that the Tengah Nature Way would be classified entirely as “Unmanaged Trees,” despite Tengah Nature Way being classified as a “Park” in the 2019 URA Masterplan. The rationale for this is attributed to HDB’s intention to transform the Tengah Nature Way into a rich forest habitat, planting it with rainforest tree species and designed with the intention of “replicating the natural structure of forests as much as possible” (HDB, 2016; National Parks Board, 2020a, para. 2). Tengah Nature Way would therefore, feature forest-like vegetation instead of managed trees that are more commonly found in parks. The third assumption involves the Tengah Forest Fringe, which runs along the perimeters of the entire Tengah town. Although little information has been released on what vegetation type the Forest Fringe would comprise of, its name alludes to the presence of forest-like vegetation. Hence, it would similarly be categorized under the “Unmanaged Trees” category rather than “Managed Trees”. The fourth and last assumption is that the land cover categories of “Utility,” “Place of Worship,” “Civic and Community Institutions” and “Mass Rapid Transit” would have little vegetation found within their premises. Therefore, these land cover types were assigned an ecosystem category of “Artificial Impervious Surface” only. A comparison of Tengah’s pre-development land cover and simulated proposed development can be found in Figure 5.
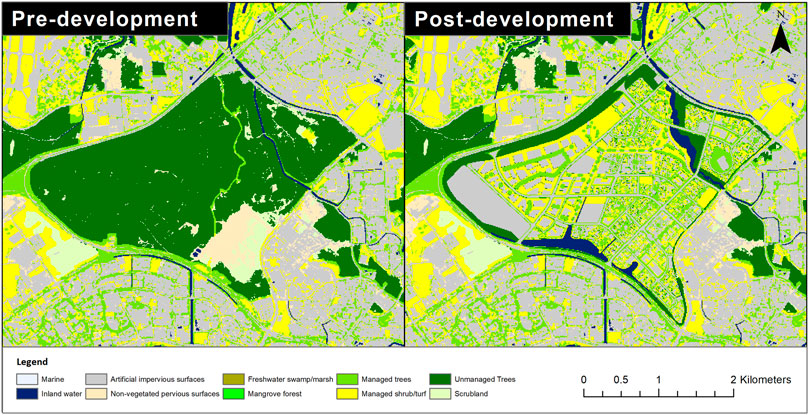
FIGURE 5. Comparison of the Tengah Forest Town and surrounding regions in the pre-development and simulated proposed post-development situation.
Projection of Other Landscape Parameters for Proposed Tengah Development
The supplementary layers required to run ecosystem service models were first updated to include the proposed land cover changes at Tengah. Updated layers were created to simulate NDVI, LAI, topology, maximum vegetation height, accessibility of an area to the public, population, buildings and road network (see Current Urban Characteristics of Tengah and Comparison Towns above). The following paragraphs briefly describe how each layer was updated, and a more detailed description of his process can be found in the Supplementary Material.
For NDVI, LAI and vegetation height, the median values of these parameters were calculated for each ecosystem cover type currently present in Singapore. These median values were then assigned to their respective ecosystem category type represented in the proposed post-development Tengah land cover map. New roads that were digitized in Tengah were merged with the original road layer to reflect the updated road networks. The accessibility layer was also updated to amend the accessibility of the entire Tengah region from ‘inaccessible’ to “accessible” to the public.
The population and building data layers, which contain information on the location of residential buildings and all buildings respectively (represented as point features), together with building height and the population residing in these buildings, were also updated to reflect the proposed construction of buildings and incoming population. The building heights of all residential buildings within Tengah were assigned a uniform number based on calculating the average number of storeys residential blocks would have. These calculations were performed separately for each residential plot type (Residential and Residential/Commercial). Building heights for other building types were estimated from SLA’s 2014 elevation data of Punggol town, another town within Singapore that is in close proximity to an aerodrome, similar to Tengah.
The number of people living in each residential block was assigned a uniform population number based on a set of calculations that estimates the number of people living in each residential block. Similar to calculations performed for estimating building height, these calculations were also performed separately for each residential plot type. An assumption of the approximate number of people living in each flat type, (i.e. 2-room Flexi, 3-room etc.) was made and multiplied with the total number of each flat type within each residential development. Table 2 presents these assumptions and calculations. All buildings found in other land use parcels were assigned a population of zero. A summary of the building heights and population residing in each building type can be found in Table 3.
Comparison of Ecosystem Services Provided Between Tengah Town and Three Other Older Towns
In addition to assessing the ecosystem service provision at Tengah under pre-development and proposed development scenarios, we compared ecosystem service provision against three other towns, namely Sengkang, Pasir Ris and Bishan. These three towns were selected based on their maturity, with Sengkang being the newest estate, which completed its first phase of development in 1997 (Tee, 2000). Pasir Ris and Bishan were completed in 1988 and 1985 respectively (Choo, 2018; Lee, 2015). Supplementary Figure S1 shows where these towns are located in Singapore and Table 4 briefly compares distinctions between these towns in relation to structural information such as land area (Data.gov.sg, 2021), population (Department of Statistics Singapore, 2019), population density, and land use makeup. All information provided is based on data acquired from 2019.
Being developed earlier, the urban designs for these three towns were conceived with a different focus, compared to the more nature-centric and environmentally sustainable designs used in the development of Tengah town. In the 1980s and 1990s, which coincided with Singapore’s early years independence and development, urban greening was functional and not designed with a specific focus on ecosystem services (Neo, 2020). Urban designs during this period were pragmatic and people-centric, with a focus on strengthening town identities and communities (Neo, 2020). The identity of Pasir Ris town was deeply anchored in its history as a recreational town, where early settlers had set up resorts and holiday bungalows, as well as its location along the eastern coast of Singapore (Choo, 2018). This led to planners centering Pasir Ris’s urban design around the theme of “a town by the sea” and its identity as a resort-like recreational town, which explains the sight of “seashell motifs” and “portholes” in many urban designs throughout the estate (Choo, 2018; Lim, 2019). Similar to Pasir Ris, Sengkang town was built around a theme that was inspired by its history as well–in particular, its history as a fishing village and port (Chua, 2017). This led to planners designing Sengkang around the theme of a “Town for the Seafarer” (Cornelius-Takahama, 2016). In the case of Bishan town, its design was inspired by its former history as a burial ground for the Chinese community, where pavilions were commonly found throughout (Lee, 2015). These iconic pavilions were the inspiration behind the distinct pitched roofs on public housing buildings that Singaporeans closely associate Bishan with (Lee, 2015). Parks and open spaces were present throughout these urban designs for recreation and inter-mingling (Neo, 2020), however, extensive urban greening in buildings and other infrastructures were not prominent features. This explains the observations in Pasir Ris and Bishan town, for example, where both towns feature flagship parks such as the Pasir Ris Town Park and Bishan-Ang Mo Kio Park respectively, but have an absence of rooftop gardens and other urban greening features that are prominently found in Tengah’s proposed development.
By selecting these three more mature towns for comparison, a comparison of results between these towns can provide an assessment of how effective nature-based solutions—that underpin the “Forest Town” principles—allow for greener and more sustainable towns, while also affirming if these solutions have any impact on improving urban ecosystem services. Data on the landscape structure of the three comparison towns were extracted from the national-level datasets outlined in Current Urban Characteristics of Tengah and Comparison Towns.
Ecosystem Service Modeling
A series of ecosystem services models were run for each scenario and case study to assess the impact on ecosystem service provision. These models evaluated the different developments for their impacts on the following aspects: local air temperature, noise attenuation, removal of PM10 particulate matter, air pollution, runoff retention, access to green and blue spaces for recreation and, soil erosion. These indicators were chosen to cover a range of regulating, provisioning, and cultural services, (Elmqvist et al., 2015), and correspond to services which have been recognized as important by the general public of Singapore (Drillet et al., 2020). With the exception of the public access to outdoor spaces model, the ecosystem service models focused on evaluating the supply of services to people, rather than incorporating aspects of demand. This constraint to supply side modeling constrains the relevance of the indicators here, because demand for ecosystem services is also required in order for the benefits to be realized (Nedkov and Burkhard, 2012; Wolff et al., 2015). However, it is complex to develop models for ecosystem service demand, particularly when projecting future scenarios, as human preferences and opinions can change unpredictably over time (Richards et al., 2020b). In the context of urban planning, it may be sufficient for quantitative models to provide information on ecosystem service supply for most services, as the magnitude and spatial variation in demand can be assessed qualitatively by designers and planners. The urban ecosystem service models were implemented in the R programming language (R Core Team, 2020), and have been made publicly available as an R package; the NCS2020 package (Richards et al., 2021). The following sections explain the methods behind the creation of each ecosystem service model, with more details provided in the Supplementary Material and R package documentation (Richards et al., 2021).
Runoff Retention
For the purpose of estimating the proportion of rainfall runoff that is prevented by the presence of the ecosystem, we modeled runoff under extreme precipitation conditions. In Singapore, “large storm” events are defined as > 70 mm h−1 falling within an hour (Chow et al., 2016). The frequency of such events, and their magnitude, has increased over time, with the modeled annual maximum hourly rainfall in 2010 around 110 mm h−1 (Chow et al., 2016). We therefore used 110 mm h−1 as the input rainfall for this model, to simulate an extreme but plausible rainfall event. It was assumed that this rainfall fell uniformly over the study region.
The runoff per pixel from a 110 mm storm event was estimated using the curve number table provided in USDA. (1986), which assigns curve numbers to all ecosystem types defined by USDA. The curve numbers used for different vegetation cover types are given in Supplementary Table S1. It was assumed that all urban area soils were of low quality (Grade D), and all forest soils were of high quality (Grade A). A more detailed explanation of the runoff retention model can be found in Section 2.4 of the Supplementary Material document attached to this paper, or the R package documentation (Richards et al., 2021).
Access to Nature Spaces
The accessibility that people have to nature spaces was modeled by cross-referencing population data at the building level against the spatial extent of three types of nature spaces; (1) patches of green space larger than 1 ha in size, (2) high-quality natural ecosystems, and (3) blue spaces. We defined nature spaces that were within 300 m of the buildings housing each respondent as “accessible,” based on a typical short walking distance for an urban resident. This measure of accessibility thus characterizes neighborhood accessibility in the home surroundings of each person (Annerstedt Van Den Bosch et al., 2016; WHO, 2016; Huang et al., 2017).
Patches of green space larger than 1 ha have previously been proposed as an indicator of green spaces of recreational importance, and particularly relevant for positive health outcomes (Annerstedt Van Den Bosch et al., 2016; WHO, 2016; Huang et al., 2017). We considered all types of green spaces as eligible for inclusion in this parameter, including all types of managed and unmanaged vegetation, and wetlands (Gaw et al., 2019). Accessibility and exposure to high-quality natural ecosystems may be important in improving people’s exposure to biodiversity and their feelings of connectedness to nature (Shanahan et al., 2017; Richards et al., 2020a). We defined “high-quality natural” ecosystems as those terrestrial, coastal, and freshwater that are generally less heavily managed by humans, including unmanaged vegetation with and without tree canopy cover, freshwater marshes and swamps, and mangroves (Gaw et al., 2019). Similar to the recreational green spaces, a minimum patch size of 1 ha was applied to define high-quality natural ecosystems. We defined blue spaces as those that had open water; including freshwater reservoirs, canals, and rivers, and the sea (Gaw et al., 2019). We did not set a minimum patch size for the blue space indicator, due to the lower coverage of this ecosystem type across Singapore. For each scenario or town, we quantified the proportion of the total town population living with access to each outdoor space type as an indicator of overall access in the town. For mapping spatial variation in access to outdoor spaces within each scenario or town, we quantified the proportion of the town population that had access to each pixel of outdoor space. Higher values thus indicate outdoor spaces which are accessible to more people. A more detailed explanation of the accessibility model can be found in the R package documentation (Richards et al., 2021).
Road Traffic Noise Attenuation
We modeled the potential of vegetation to attenuate road traffic noise by generating hypothetical noise sources as 30,000 points located randomly along the road network within each study region. We assumed that the source noise level at each point was 70 dB—an intermediate-high value for traffic noise (Ma and Yano, 2004; Chin et al., 2019). Noise was modeled only at 1000 Hz, as this approximates the dominant frequency of traffic noise (Can et al., 2010).
Noise attenuation was modeled using a simple 2-dimensional mechanistic model, following the methodology of the System for the Prediction of Acoustic Detectability (SPreAD) model, which is freely available as a package for ArcGIS (Reed et al., 2012). The general approach and equations used to model the component forms of noise loss are similar to the International Organization for Standardization guideline on attenuation of sound during propagation outdoors (ISO 9613-2, 1996). Our model evaluates four types of noise attenuation over space, including attenuation due to spherical spreading loss, atmospheric absorption, topographic and barrier effects, and vegetation cover loss. The ecosystem service is quantified as the noise attenuated by vegetation, after accounting for the other types of noise attenuation. A more detailed explanation of how each type of noise attenuation was modeled can be found in Section 2.2 of the Supplementary Material document attached to this paper, or the R package documentation (Richards et al., 2021).
Ambient Air Temperature Reduction
The impact of urban ecosystems on the ambient air temperature was modeled using a land cover regression approach. Data on air temperature were collated from two existing weather station networks between the 1st of October 2015 and the 2nd October 2018, covering 45 weather stations in different parts of Singapore (Supplementary Figure S2). Data were aggregated into hourly means for each weather station by taking the mean of all measurements within the hour, and modeled as a function of vegetation cover explanatory variables at a range of distances from the weather station locations. The resulting model coefficients were used to estimate the average ambient temperature reduction due to the presence of surrounding vegetation at 3pm across the study regions. A more detailed description of the methods taken to model ambient air temperature reduction can be found in Section 2.1 of the Supplementary Material document attached to this paper, or the R package documentation (Richards et al., 2021).
Removal of PM10 Particulate Matter Air Pollution
The removal of PM10 particulate matter from the air by vegetation over the course of 24 h was modeled under no precipitation and relatively high ambient PM10 conditions, using a model commonly used in urban ecosystem service modeling studies (Zinke, 1967; Nowak et al., 1998; Escobedo and Nowak, 2009; Manes et al., 2014; Bottalico et al., 2016). This model requires data on spatial variation in the leaf area index, as well as information on the background PM10 concentration, deposition flux of PM10, and resuspension rate. Deposition flux and resuspension rates were parameterized using commonly used global values (Lovett, 1994; Nowak et al., 1998; Escobedo and Nowak, 2009; Manes et al., 2014; Bottalico et al., 2016). Particulate matter concentration data are collected and reported by the National Environment Agency (NEA) of Singapore on an hourly basis. We took the median of the annually reported 99th percentile daily (24 h) mean values reported online between (NEA, 2020). This gave a value of 84 μg per m3 (Supplementary Figure S3), a relatively high exposure to PM10 in the Singapore context.
Leaf area index was parameterized in tree-covered areas using a national map of leaf area index developed using remote sensing (Richards and Wang, 2020). We assigned all areas of unmanaged or managed vegetation without a tree canopy to have a minimum leaf area index of 2 following the URA LUSH program guidelines (URA, 2014). Further details on the modeling of the PM10 removal ecosystem service can be found in Section 2.3 of the Supplementary Material document attached to this paper, or the R package documentation (Richards et al., 2021).
Soil Erosion
The ecosystem service of soil erosion was modeled using a variation of the Universal Soil Loss Equation (Wischmeier and Smith, 1978), proposed by Guerra et al. (2014). This ecosystem service is defined as the difference between the total actual soil loss and the potential soil loss estimated assuming that no vegetation was present. The results are presented as the proportion of the possible erosion that was prevented by the presence of vegetation.
The Universal Soil Loss Equation requires data on the rainfall runoff factor, topographic factor, soil erodibility factor, and vegetation cover factor. The rainfall runoff factor was taken from a global mapping of erosivity (Panagos et al., 2017). The topographic factor represents the propensity of the landform to soil erosion, and was calculated from the national digital elevation model using an established equation (Moore and Burch, 1986). The soil erodibility factor was modeled based on field data for soil particle size distribution and clay content, following a method previously proposed by the Universal Soil Loss Equation (Renard et al., 1997). Field samples were collected from 24 locations for each vegetation cover type, with samples taken from the top 20 cm of soil. The median values for the five vegetation types were taken and applied to the corresponding vegetation cover categories (Supplementary Table S2). The vegetation cover factor was derived using satellite imagery, using a conversion from the normalized difference vegetation index (Van der Knijff et al., 2000). A more detailed explanation of the runoff retention model can be found in Section 2.5 of the Supplementary Material document attached to this paper, or the R package documentation (Richards et al., 2021).
Results
Comparison of Modeled Ecosystem Service Provision in Tengah, Before and After Proposed Development
The proposed Tengah redevelopment was modeled to result in reduced ecosystem service provision in relation to retaining runoff, reducing air temperatures, absorbing PM10 particulate matter, reducing road traffic noise, and protecting soil from potential soil erosion (Figure 6). The magnitude of losses in ecosystem service provision was estimated to be substantial; ranging from 35% in the case of rainfall retention to 62% for noise attenuation (Figure 6). It was not possible to compare the accessibility of nature spaces to the public quantitatively using the models, because the region of Tengah is currently inaccessible to the public and has been for several decades. Hence, while the accessibility model would not provide any valuable information for our analysis, it is true that the opening up of the Tengah region to the public will significantly increase people’s accessibility and exposure to nature in the area.
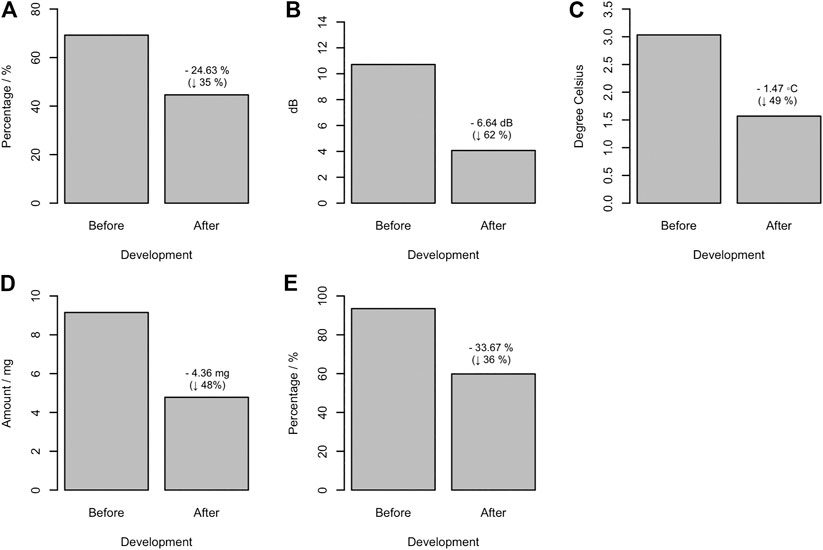
FIGURE 6. Comparison of ecosystem service performance between modeled results for Tengah before and after the proposed development (A) Average percentage of rainfall retention by vegetation (B) Average noise reduction contributed by vegetation (C) Average reduction in air temperature contributed by vegetation (D) Average reduction in PM10 particulate matter by vegetation (E) Average proportion of soil protected from erosion.
The average percentage of rainfall retained in Tengah before development was modeled to amount to an average of 69.24% for a 110 mm rainfall event. Conversely, for the same rainfall event, the average percentage of rainfall retained in Tengah after development was recorded at 44.61 %—a 24.63% decrease from Tengah’s retention ecosystem service prior to development (Figure 6A). There was considerable spatial variation in modeled changes in rainfall retention capacity within Tengah post-development (Figure 7A). A large proportion of the eastern half of Tengah recorded either a slight decrease or increase in rainfall retention capacity. These areas belong to mainly “Residential” and “Residential/Commercial” plots, as well as areas categorized as “Unmanaged Trees” and “Parks.” Conversely, areas recording a more significant decrease in rainfall retention capacity were found to be congregated toward the western half of Tengah, belonging mainly to the “Business 2” and “Transport Facilities” land use cover sites.
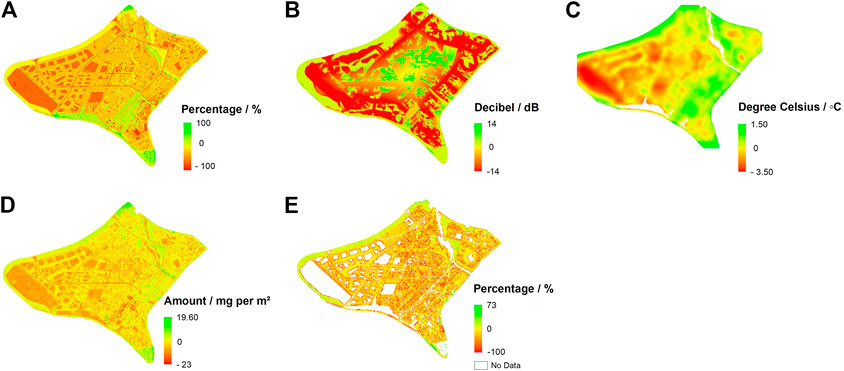
FIGURE 7. Maps depicting the change in ecosystem service provision for each Ecosystem Service Model, derived from performing a raster subtraction of Tengah after development from Tengah before development (A) Average percentage of rainfall retention by vegetation (B) Average noise reduction contributed by vegetation (C) Average reduction in air temperature contributed by vegetation (D) Average reduction in PM10 particulate matter by vegetation (E) Average proportion of soil protected from erosion.
Results from running the noise model also show expected declines in road traffic noise attenuation ecosystem service provision in the post-development scenario. In particular, areas surrounding the edge of the proposed development region showed greater declines in the provision of this ecosystem services, most likely due to the removal of vegetation for urban development (Figure 7B). Conversely, some areas toward the center of the site show projected increases in the provision of the road traffic noise attenuation service. This is due to the closer proximity of roads to the center of the site under the proposed redevelopment. Under the current scenario, the center of Tengah is too distant from any roads to experience the benefits of road traffic noise reductions.
Vegetation in Tengah before development was modeled to reduce air temperatures by an average of 3.03°C, while vegetation in Tengah after development was modeled to reduce air temperature by an average of 1.57°C. The temperature reduction ecosystem service provided by vegetation in Tengah after development is thus evaluated to have decreased by 49%, compared to the temperature reduction ecosystem service provided by vegetation in Tengah before development (Figure 6C). A significant contributor to the loss in temperature reduction ecosystem services provision can be traced mainly to the “Business 2” and “Transport Facilities” land use regions that are situated in the western half of Tengah, the loss of temperature reduction services was greater (Figure 7C). These areas record a maximum loss of −3.43°C in temperature reduction.
The amount of PM10 particular matter removed per m2 in post-development Tengah was modeled to be approximately half of that of the pre-development scenario (Figure 6D). The spatial variation in the changes to the amount of PM10 particulate matter removed post-development appears relatively homogeneous, with most developments within Tengah recording a decrease in PM10 particulate removal ecosystem service (Figure 7D). A significant decrease in PM10 particulate removal ecosystem service can be observed in the “Business 2” and “Transport Facilities” site in the west, due to the lower vegetation cover in these areas. The Tengah Forest Fringe region, as well as the Tengah Nature Way are the only few areas within Tengah to contribute to a net increase in PM10 particulate removal ecosystem service, due to the high-quality unmanaged vegetation that was simulated to grow in these areas in the future.
A general net loss in the soil erosion prevention ecosystem service provision was modeled in Tengah after development, with an average of 33.67 mg of soil no longer protected from potential erosion (Figure 6E). The spatial variation of changes in soil protection appears rather homogeneous, with a large proportion of land area within Tengah recording approximately −50 mg in soil erosion ecosystem service loss (Figure 7E). Areas that feature no net change in soil erosion ecosystem service provision are found in areas that are categorized under the “Unmanaged Trees” ecosystem category. Considerable areas of the Tengah region could not be modeled for soil erosion loss under the post-development scenario, because they were simulated to be covered in impervious surfaces that would not experience surface soil loss. These regions are mapped as “No Data” (Figure 7E).
Comparison of Proposed Tengah Forest Town Development Against Other Towns
A comparison of eight indicators derived from six ecosystem service models reveal that the proposed Tengah development would provide higher provision of five indicators when compared to the three older towns (Figure 8; Supplementary Table S3). Overall, the proposed Tengah scenario leads in four out of six ecosystem services; runoff retention, access to nature, air temperature reductions, and PM10 removal (Figure 8).
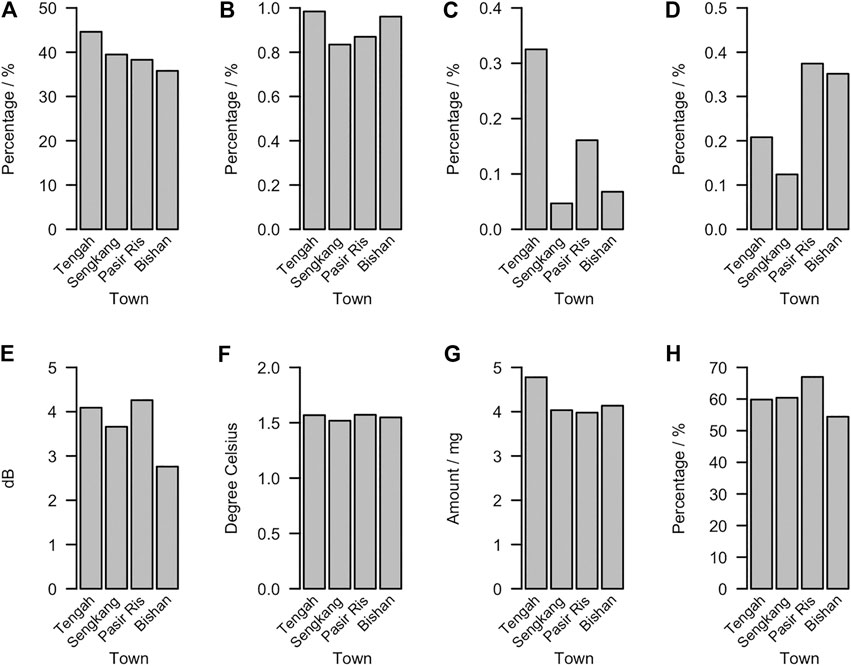
FIGURE 8. Comparison of ecosystem services performance for each town; Tengah Town, Sengkang Town, Pasir Ris Town and Bishan Town. Ecosystem services include (A) average percentage of rainfall retention by vegetation (B) percentage of population with access to green spaces of at least 1 ha (C) percentage of population with access to high-quality natural ecosystems of least 1 ha (D) percentage of population with access to blue spaces (E) average noise reduction contributed by vegetation (F) average reduction in air temperature contributed by vegetation (G) average reduction in PM10 particulate matter by vegetation (H) average proportion of soil protected from erosion.
Vegetation was modeled to retain 44.61% of rainfall under the simulated extreme event in Tengah, while Sengkang, Pasir Ris and Bishan recorded rainfall retention values of 39.49%, 38.29%, and 35.79% respectively (Supplementary Table S3). This places Tengah at the forefront of providing the rainfall retention ecosystem service, followed by the towns of Bishan, Pasir Ris, and Sengkang.
The results of the accessibility to nature spaces model show the strong performance of the proposed Tengah scenario in providing this ecosystem service to residents. In particular, there was a marked increase in the proportion of residents who are within 300 m to both natural green spaces and green spaces of at least 1 ha in Tengah, with Tengah recording a percentage of 32.51 and 98.41% for each category respectively (Supplementary Table S3). This is in contrast to the other towns, which in comparison to Tengah, record lower percentages in both categories. Bishan is evaluated to have 6.78% and 96.08% of its population within 300 m of natural green spaces and green spaces respectively (Supplementary Table S3). This is lower than the proportion of residents with access to green and natural green spaces in Tengah. Sengkang is found to record percentages of 4.68% and 83.45% respectively (Supplementary Table S3), the lowest proportion of residents with access to natural green spaces and green spaces among the four towns. Pasir Ris, on the other hand, records percentages of 16.10% and 86.96% respectively (Supplementary Table S3). Evaluating residents’ access to blue spaces, we find that Pasir Ris has the highest proportion of residents who have access to blue spaces compared to the four other towns, with 37.42% of Pasir Ris’s residents living within 300 m from a waterbody (Supplementary Table S3). Bishan comes in second, with 35.12% of its residents living within 300 m of a waterbody (Supplementary Table S3). Fewer residents in Tengah and Sengkang, on the other hand, are found to live more than 300 m away from blue spaces and therefore, recording 20.80% and 12.40% of its residents with access to blue spaces respectively.
Results obtained from running the noise attenuation model reveals that the new Tengah town does not provide the best noise abatement ecosystem service. Out of the four towns, Tengah is placed second, with the vegetation noise attenuation effect averaged at 4.09 dB (Supplementary Table S3). Pasir Ris is found to have provided the best noise abatement ecosystem service, with vegetation reducing noise levels by an average of 4.26 dB within the neighborhood (Supplementary Table S3)–approximately 4% greater than that of Tengah. Sengkang is found to come in third, with vegetation reducing noise by an average of 3.66 dB. Conversely, Bishan was found to have provided the weakest noise abatement ecosystem service (Supplementary Table S3). Overall, however, the noise abatement ecosystem service provided by vegetation in all four towns are low; typically lower than 10 dB. While the decibel scale is nonlinear, making it complex to assess the magnitude of effect for human well-being, a reduction of 10 dB from a 70 dB maximum may not have a substantial impact on improving human comfort given the magnitude of the background noise (Centers for Disease and Control Prevention, 2019). This is particularly likely to be the case when considering that other forms of noise attenuation–such as attenuation over distance, and the effect of buildings in blocking sound waves, act to reduce noise levels in the urban environment. Attenuation from these non-vegetation mechanisms was modeled first in our approach.
Results from the air temperature reduction modeling reveal that Tengah provides among the highest levels of this ecosystem service. Alongside Pasir Ris, vegetation within these two towns provide the best cooling effect among the other towns, reducing air temperature by an average of 1.57°C (Supplementary Table S3). Bishan comes behind Tengah and Pasir Ris in its temperature reduction ecosystem service performance, modeling a temperature decrease of 1.55°C (Supplementary Table S3). This is followed by Sengkang, which models a temperature decrease of 1.52°C (Supplementary Table S3).
The mean amount of PM10 particulate matter removed by vegetation in Tengah was modeled to amount to 4.78 mg per m2, the highest value recorded among the four towns (Supplementary Table S3). Bishan came in the second highest, modeling a removal of 4.14 mg per m2 (Supplementary Table S3). This is followed by Sengkang and finally, Pasir Ris, modeling a removal of 4.01 and 3.98 mg per m2 respectively (Supplementary Table S3). Among the four towns, Tengah was the only town that modeled a removal of close to 5.00 mg of PM10 particulate matter m2 (Supplementary Table S3), thereby indicating a substantial improvement in PM10 particulate matter removal ecosystem service compared to other towns.
Among the four towns, results attained from running the soil erosion prevention model reveal that Pasir Ris provides the best soil protection ecosystem service, protecting 66.99% of soil from potential soil erosion (Supplementary Table S3). Tengah town comes in as the third best town, protecting 59.83% of soil from soil erosion, 0.57% behind Sengkang (Supplementary Table S3). Bishan comes in the last in soil protection ecosystem service provision, protecting 54.42% of soil from potential soil erosion (Supplementary Table S3).
Discussion
Ecosystem Service Impacts of Developing the Tengah Town
In general, pre-development Tengah showed higher simulated ecosystem service values than the proposed Forest Town development. This is largely a result of expected removal of the well-developed secondary forest vegetation that currently covers much of the site (Gaw et al., 2019), and its proposed replacement with urban development. Natural and semi-natural ecosystems, such as secondary forests, typically provide higher levels of many ecosystem services, such as air temperature reduction and protection from soil erosion (McDonald et al., 2002; Richards et al., 2020b). Despite the anticipated declines in many ecosystem services at Tengah, the opening-up of the region to the public will increase the recreational opportunities provided. This is important in an urban area, as access to green spaces for recreation is important in improving the quality of life and health outcomes (James et al., 2015), and prioritized strongly by the public in Singapore (Drillet et al., 2020).
The modeling exercise also revealed priority areas where design changes could help to enhance the provision of ecosystem services. For example, the western region, where the future industrial region will be located, is projected to experience the greatest ecosystem services provision losses in Tengah. This is observed in majority of the models run for the before and after development analysis in Tengah. On the contrary, the eastern region, where most residential sites are located, appear to contribute the least to losses in ecosystem services provision. In fact, in some models, the eastern region appears to have maintained and even improved some ecosystem services provision. A possible explanation for this maintenance and improvement can be attributed to the greenery integrated into residential buildings, which is part of HDB’s Biophilic Town Framework. In Plantation Village, for example, ground-level landscaping and park spaces are planned throughout the development (HDB, 2020b), contributing to a collective increase in vegetation found throughout the town. A comparison of ecosystem services maps (Supplementary Figures S5–9) also reveal that Tengah’s residential areas are projected to provide greater ecosystem service values than older sites in the comparison towns, which do not feature as much vegetation.
To further improve ecosystem service provision in the new Tengah town, we may focus on the “Business 2” sites, which are currently not planned in detail. Current reports have cited plans to set aside 40% of spaces for green coverage within the “Business 2” district (Jurong Town Corporation, 2020). To complement this strategy, “Business 2” sites may consider adopting incentives under URA’s Landscaping for Urban Spaces and High-rizes (LUSH) Program, a program which incentivizes developers to integrate more greenery into urban spaces (URA, 2017); for example, by implementing green roofs and vertical and horizontal green surfaces (URA, 2017). Furthermore, the ecosystem service response is sensitive to the type of vegetation that is planted in new developments (Richards et al., 2020b). Where possible, multi-layered canopy planting, or less heavily managed vegetation, may be beneficial (McDonald et al., 2002; Richards et al., 2020b). These strategies may contribute to enhancing Tengah’s ecosystem service provision, while also enabling developers to enjoy exemptions from Gross Floor Area restrictions originally imposed by URA (URA, 2020c).
Ecosystem Service Performance of Nature-based Urban Design in Comparison With Older Paradigms
Overall, the proposed development at Tengah shows significant promise in providing ecosystem services, compared to older towns in Singapore. These modeling results provide evidence that the “Forest Town” concept can to some degree help balance the trade-off between development and ecosystem services. It also demonstrates how ecosystem services provision can be enhanced, even in a built environment. A summation of rankings indicate that the currently proposed Tengah development will provide ecosystem services more effectively than the comparison towns, particularly Bishan, which is the oldest town studied (Supplementary Table S4). This suggests that there have been significant advancements in planning and design that have better incorporated ecosystem services within new developments. This improvement has come alongside efforts from planning agencies, notably the HDB and URA, to position environmental sustinability as central to development (Wong, 2018; URA, 2020d).
Our findings have implications for urban design in other cities around the world. First, the modeling results have affirmed the effectiveness of incorporating nature-based solutions into new developments to enhance urban ecosystem services. Our analysis also reveals key insights into how to achieve better balance between development and ecosystem services. The greater ecosystem service performance of Tengah compared to Pasir Ris and Bishan highlights the value added by integrating green spaces throughout the development. Both Pasir Ris and Bishan feature large flagship parks that are known to provide substantial ecosystem service benefits to residents (Hwang et al., 2015). This suggests that while large park areas are important in providing ecosystem services, it is also important to consistently integrate green infrastructure throughout urban elements within an estate. Such findings align closely with research carried out by Hostetler et al. (2011), which highlighted that urban developments and green infrastructures should not exist as two separate entities, mainly because the functionality of green infrastructures and conservation of urban biodiversity are contingent on how well surrounding urban infrastructures are designed and managed. Consequently, urban developments should be built to complement and minimally impact surrounding green infrastructures to ensure their functionality and contribution to ecosystem services (Hostetler et al., 2011). Therefore, to achieve better performance in urban ecosystem services, consistent integration of green infrastructure throughout estate elements should be a mainstay for future development plans.
When designing nature-based towns, it is important to consider the scale and context in which these solutions and infrastructures are implemented in, as well as the impact such solutions would have for the particular town or city in the future. Given Tengah town’s small size of 7 km2, maintenance of green infrastructures such as green roofs and vertical and horizontal green surfaces within the town would be relatively manageable to carry out. Maintenance of such green infrastructures is critical to avoid uncontrolled growth of plant species and drainage failure, which can result in corrosion and damage of building walls (Chew et al., 2018). To reduce maintenance costs over large developments, unmanaged vegetation may be incorporated as an alternative to manicured parks or gardens. The less heavily managed rainforest features at Tengah were projected to contribute significantly to ecosystem services provision (namely the Tengah Nature Way and the Tengah Forest Fringe).
Supporting Ecosystem Service Modeling for Singapore and Other Tropical Cities
The ecosystem service models used in this study have been made freely available for download and re-use, as part of the NCS2020 software package for the R statistical computing language (Richards et al., 2021). The availability of these models may encourage use by researchers, planners, and designers in Singapore, thus leading to increased interaction and feedback between ecosystem service science and the process of designing and planning cities (Andreu and Oreszczyn, 2004; Richards et al., 2019). Furthermore, many of the models developed here will be applicable to other cities—either as currently written—or with some reparamaterization (Richards et al., 2021). The current parameters may be directly applicable to other cities in Southeast Asia that have similar climates, vegetation types, and socio-economic contexts. As such, this software package aims to support the application of ecosystem services principles in tropical cities, which have been under-represented in previous research and development (Song et al., 2017; Song et al., 2018). Of the six tools presented in this study, only the air temperature reduction model is highly specific to Singapore and should be interpreted cautiously if applied to other cities (Richards et al., 2021). The other five models are general in principle, and could be applied to any city once parameterized for the local context (Richards et al., 2021).
Conclusion
Using Singapore’s newest nature-centric Tengah town as a case study, this study assessed the effectiveness of using nature-based solutions as a means to improve urban ecosystem services within new developments. Through a spatially explicit modeling approach, six ecosystem service models were run on the new Tengah town to assess its performance. A comparison of Tengah’s ecosystem service performance results was then compared to its performance prior to development, as well as to three other more matured towns in Singapore that were designed with a different design paradigm. We observe that a consistent application of nature-based solutions throughout all elements within a development can significantly contribute to an enhancement in urban ecosystem services. With continued rapid urbanization, a paradigm shift in urban design toward a nature-based approach could help to not only to enhance urban ecosystem services within our built environments, but also inculcate in developers and architects the critical role that urban designs have in contributing to an environmentally sustainable future for the world. A greater synergy between ecologists and architects in developing urban designs may help us to achieve more liveable, sustainable and resilient towns and cities.
Data Availability Statement
The raw data supporting the conclusion of this article will be made available by the authors, without undue reservation.
Author Contributions
Conceptualization, BT, LG, and DR; methodology, BT, LG, MM, and DR; software, BT, LG, and DR; formal analysis, BT; investigation, BT, LG, MM, and DR; resources, DR; writing—original draft preparation, BT, MM; writing—review and editing, BT, LG, and DR; visualization, BT; supervision, DR; project administration, LG and DR; funding acquisition, DR.
Funding
This research is supported by the National Research Foundation, Prime Minister’s Office, Singapore under its Campus for Research Excellence and Technological Enterprise (CREATE) Program (NRF2016-ITC001-013).
Conflict of Interest
The authors declare that the research was conducted in the absence of any commercial or financial relationships that could be construed as a potential conflict of interest.
Acknowledgments
We would like to thank Mr Ng Zhen Hao from the Survey and Geomatics Division of the Singapore Land Authority (SLA). The Digital Terrain Model and the Digital Surface Model were produced from the aerial mapping exercise by SLA in 2014.
Supplementary Material
The Supplementary Material for this article can be found online at: https://www.frontiersin.org/articles/10.3389/fenvs.2021.610155/full#supplementary-material
Footnotes
1The term “Kampung” is a Malay and Indonesian term for “village” and refers to urban informal settlements that were very commonly found in Singapore for early settlers (Ministry of National Development, 2019; Akbar and Edelenbos, 2020).
References
Akba, P. N. G., and Edelenbos, J. (2020). Social Impacts of Place-Making in Urban Informal Settlements: A Case Study of Indonesian Kampungs. Soc. Sci. 9 (6), 104. doi:10.3390/socsci9060104
Andreu, I. C., and Oreszczyn, T. (2004). Architects Need Environmental Feedback. Building Res. Inf. 32 (4), 313–328. doi:10.1080/09613210410001679857
Annerstedt van den Bosch, M., Mudu, P., Uscila, V., Barrdahl, M., Kulinkina, A., Staatsen, B., et al. (2016). Development of an Urban Green Space Indicator and the Public Health Rationale. Scand. J. Public Health. 44 (2), 159–167. doi:10.1177/1403494815615444
Arnfield, A. J. (2003). Two Decades of Urban Climate Research: A Review of Turbulence, Exchanges of Energy and Water, and the Urban Heat Island. Int. J. Climatol. 23 (1), 1–26. doi:10.1002/joc.859
Babí Almenar, J., Elliot, T., Rugani, B., Philippe, B., Navarrete Gutierrez, T., Sonnemann, G., et al. (2021). Nexus between Nature-Based Solutions, Ecosystem Services and Urban Challenges. Land Use Policy. 100, 104898. doi:10.1016/j.landusepol.2020.104898
Baró, F., Palomo, I., Zulian, G., Vizcaino, P., Haase, D., and Gómez-Baggethun, E. (2016). Mapping Ecosystem Service Capacity, Flow and Demand for Landscape and Urban Planning: A Case Study in the Barcelona Metropolitan Region. Land Use Policy. 57, 405–417. doi:10.1016/j.landusepol.2016.06.006
Bolund, P., and Hunhammar, S. (1999). Ecosystem Services in Urban Areas. Ecol. Econ. 29 (2), 293–301. doi:10.1016/s0921-8009(99)00013-0
Bottalico, F., Chirici, G., Giannetti, F., De Marco, A., Nocentini, S., Paoletti, E., et al. (2016). Air Pollution Removal by Green Infrastructures and Urban Forests in the City of Florence. Agric. Agric. Sci. Proced. 8, 243–251. doi:10.1016/j.aaspro.2016.02.099
Can, A., Leclercq, L., Lelong, J., and Botteldooren, D. (2010). Traffic Noise Spectrum Analysis: Dynamic Modeling vs. Experimental Observations. Appl. Acoust. 71 (8), 764–770. doi:10.1016/j.apacoust.2010.04.002
Centers for Disease Control and Prevention. (2019). Loud Noise Can Cause Hearing Loss. Retrieved fromhttps://www.cdc.gov/nceh/hearing_loss/what_noises_cause_hearing_loss.html
Chadwick, M. A., Dobberfuhl, D. R., Benke, A. C., Huryn, A. D., Suberkropp, K., and Thiele, J. E. (2006). Urbanization Affects Stream Ecosystem Function by Altering Hydrology, Chemistry, and Biotic Richness. Ecol. Appl. 16 (5), 1796–1807. doi:10.1890/1051-0761(2006)016[1796:uasefb]2.0.co;2
Chan, R. (2013). Tengah Nature Way to be Launched Next Year: PM Lee. The Straits Times. Retrieved from https://www.straitstimes.com/singapore/tengah-nature-way-to-be-launched-next-year-pm-lee. doi:10.23943/princeton/9780691158617.001.0001
Chew, M. Y. L., Conejos, S., and Azril, F. H. B. (2018). Design for Maintainability of High-Rise Vertical Green Facades. Building Res. Inf. 47 (4), 453–467. doi:10.1080/09613218.2018.1440716
Chin, C., Thang, Z. Y., and Saju, S. (2019). Study on Impact of Noise Annoyance from Highway Traffic in Singapore City. 178th Meet. Acoust. Soc. America 39, 015001. doi:10.1121/2.0001116
Choo, C. (2018). Tengah HDB Town to Offer Spaces for Community Gardening and Farming in Plantation District. Today Online. Retrieved from https://www.todayonline.com/singapore/tengah-hdb-town-offer-spaces-community-gardening-and-farming-plantation-district. doi:10.1201/9781315274829
Choo, M. K. (2018). Pasir Ris: Written in the Sand. MUSE SG. Retrieved from:https://www.roots.sg/learn/stories/pasir-ris-written-in-the-sand/story.accessed June 22, 2020. doi:10.1201/9781315274829
Chow, W. T. L., Cheong, B. D., and Ho, B. H. (2016). A Multimethod Approach towards Assessing Urban Flood Patterns and its Associated Vulnerabilities in Singapore. Adv. Meteorology., 7159132
Chua, V. (2017). Navigating the Vales of Sengkang. MyNiceHome. Retrieved fromhttps://www.mynicehome.gov.sg/article/navigating-the-vales-of-sengkang. doi:10.1142/10478
Construction Climate Challenge (2019). Singapore is Leading the Way in Green Urban infrastructure. Retrieved from https://constructionclimatechallenge.com/2019/02/20/singapore-is-leading-the-way-in-green-urban-infrastructure/
Cornelius-Takahama, V. (2016). Sengkang. Singapore Infopedia. Retrieved fromhttps://eresources.nlb.gov.sg/infopedia/articles/SIP_248_2005-01-20.html. doi:10.5194/amt-2016-2-ac3
Cox, D. T. C., Shanahan, D. F., Hudson, H. L., Fuller, R. A., and Gaston, K. J. (2018). The Impact of Urbanisation on Nature Dose and the Implications for Human Health. Landscape Urban Plann. 179, 72–80. doi:10.1016/j.landurbplan.2018.07.013
Data.gov.sg. (2021). Land Areas and Dwelling Units by Town. Retrieved fromhttps://data.gov.sg/dataset/land-area-and-dwelling-units-by-town?view_id=ef3d1ad0-61fe-413d-a165-92b9670fc00f&resource_id=898d985a-0996-4efd-b2c2-7d9fab4138e9.
Department of Statistics Singapore (2019). Population Trends 2019. Retrieved fromhttps://www.singstat.gov.sg/-/media/files/publications/population/population2019.pdf.
Depietri, Y., and McPhearson, T. (2017). “Integrating the Grey, Green, and Blue in Cities: Nature-Based Solutions for Climate Change Adaptation and Risk Reduction,” in Nature-Based Solutions to Climate Change Adaptation in Urban Areas: Linkages between Science, Policy and Practice. Editors N. Kabisch, H. Korn, J. Stadler, and A. Bonn (Springer International Publishing), 91–109. doi:10.1007/978-3-319-56091-5_6
Dissegna, M. A., Yin, T., Wei, S., Richards, D., and Grêt-Regamey, A. (2019). 3-D Reconstruction of an Urban Landscape to Assess the Influence of Vegetation in the Radiative Budget. Forests 10 (8), 700. doi:10.3390/f10080700
Drillet, Z., Fung, T. K., Leong, R. A. T., Sachidhanandam, U., Edwards, P., and Richards, D. (2020). Urban Vegetation Types Are Not Perceived Equally in Providing Ecosystem Services and Disservices. Sustainability 12 (5), 2076. doi:10.3390/su12052076
Elmqvist, T., Setälä, H., Handel, S., van der Ploeg, S., Aronson, J., Blignaut, J., et al. (2015). Benefits of Restoring Ecosystem Services in Urban Areas. Curr. Opin. Environ. Sustainability. 14, 101–108. doi:10.1016/j.cosust.2015.05.001
Escobedo, F. J., and Nowak, D. J. (2009). Spatial Heterogeneity and Air Pollution Removal by an Urban Forest. Landscape Urban Plann. 90 (3–4), 102–110. doi:10.1016/j.landurbplan.2008.10.021
Gaw, L. Y. F., Yee, A. T. K., and Richards, D. R. (2019). A High-Resolution Map of Singapore’s Terrestrial Ecosystems. Data 4 (3), 1–10. doi:10.3390/data4030116
Gómez-Baggethun, E., and Barton, D. N. (2013). Classifying and Valuing Ecosystem Services for Urban Planning. Ecol. Econ. 86, 235–245. doi:10.1016/j.ecolecon.2012.08.019
Gorelick, N., Hancher, M., Dixon, M., Ilyushchenko, S., Thau, D., and Moore, R. (2017). Google Earth Engine: Planetary-Scale Geospatial Analysis for Everyone. Remote Sensing Environ. doi:10.1016/j.rse.2017.06.031
Guerra, C. A., Pinto-Correia, T., and Metzger, M. J. (2014). Mapping Soil Erosion Prevention Using an Ecosystem Service Modeling Framework for Integrated Land Management and Policy. Ecosystems 17 (5), 878–889. doi:10.1007/s10021-014-9766-4
HDB (2020b). Aerial View of 3D Model of Plantation Village Retrieved fromhttps://esales.hdb.gov.sg/bp25/launch/19nov/bto/19NOVBTOTG_images_7523/02-3.jpg.
HDB (2018). Homes at One with Nature. Retrieved from https://www.hdb.gov.sg/cs/infoweb/about-us/news-and-publications/press-releases/homes-at-one-with-nature1
HDB (2020a). Tengah. Retrieved fromhttps://www.hdb.gov.sg/cs/infoweb/about-us/history/hdb-towns-your-home/tengah#
HDB (2016). Unveiling the Masterplan for Tengah: At Home with Nature. Retrieved from https://www.hdb.gov.sg/cs/infoweb/about-us/news-and-publications/press-releases/corporate-pr-unveiling-the-masterplan-for-tengah-08092016
Heng, J. (2017). Tengah's Kampung History inspires Farmway Concept. The Straits Times. Retrieved from https://www.straitstimes.com/singapore/housing/tengahs-kampung-history-inspires-farmway-concept. doi:10.31899/rh7.1025
Heng, J. (2016). Tengah, the First HDB Town since Punggol, to Have Forest Fringe, Car-free Town Centre. The Straits Times. Retrieved from https://www.straitstimes.com/singapore/housing/tengah-the-first-hdb-town-since-punggol-to-have-forest-fringe-car-free-town-centre
Heymans, A., Breadsell, J., Morrison, G., Byrne, J., and Eon, C. (2019). Ecological Urban Planning and Design: A Systematic Literature Review. Sustainability 11 (13), 3723. doi:10.3390/su11133723
Holt, J. B., Lo, C. P., and Hodler, T. W. (2004). Dasymetric Estimation of Population Density and Areal Interpolation of Census Data. Cartography Geogr. Inf. Sci. 31 (2), 103–121. doi:10.1559/1523040041649407
Hostetler, M., Allen, W., and Meurk, C. (2011). Conserving Urban Biodiversity? Creating Green Infrastructure Is Only the First Step. Landscape Urban Plann. 100 (4), 369–371. doi:10.1016/j.landurbplan.2011.01.011
Huang, C., Yang, J., Lu, H., Huang, H., and Yu, L. (2017). Green Spaces as an Indicator of Urban Health: Evaluating its Changes in 28 Mega-Cities. Remote Sensing. 9 (12), 1266. doi:10.3390/rs9121266
Hwang, Y. H., Lum, Q. J. G., and Chan, Y. K. D. (2015). Micro-scale Thermal Performance of Tropical Urban Parks in Singapore. Building Environ. 94, 467–476. doi:10.1016/j.buildenv.2015.10.003
ISO 9613-2 (1996). Acoustics Attenuation of Sound during Propagation Outdoors. Part 2: General Method of Calculation. ISO/DIS Standard No. 9613-2. Available online:https://www.iso.org/standard/20649.html (accessed February 18, 2020)
James, P., Banay, R. F., Hart, J. E., and Laden, F. (2015). A Review of the Health Benefits of Greenness. Curr. Epidemiol. Rep. 2 (2), 131–142. doi:10.1007/s40471-015-0043-7
Jurong Town Corporation (2020). Jurong Innovation District. Retrieved from https://www.jtc.gov.sg/industrial-land-and-space/Pages/jurong-innovation-district.aspx.
Lee, G. (2015). Bishan. Singapore Infopedia. Retrieved fromhttps://eresources.nlb.gov.sg/infopedia/articles/SIP_731_2005-01-20.html?s=bishan
Lim, H. S., and Lu, X. X. (2016). Sustainable Urban Stormwater Management in the Tropics: An Evaluation of Singapore's ABC Waters Program. J. Hydrol. 538, 842–862. doi:10.1016/j.jhydrol.2016.04.063
Lim, K. (2019). Pasir Ris: My Town and Nature Getaway by the Sea. The Straits Times. Retrieved fromhttps://www.straitstimes.com/singapore/my-town-and-nature-getaway-by-the-sea. doi:10.1142/11375
Lovett, G. M. (1994). Atmospheric Deposition of Nutrients and Pollutants in North America: An Ecological Perspective. Ecol. Appl. 4 (4), 629–650. doi:10.2307/1941997
Ma, H., and Yano, T. (2004). An Experiment on Auditory and Non-auditory Disturbances Caused by Railway and Road Traffic Noises in Outdoor Conditions. J. Sound Vibration. 277 (3), 501–509. doi:10.1016/j.jsv.2004.03.011
Manes, F., Silli, V., Salvatori, E., Incerti, G., Galante, G., Fusaro, L., et al. (2014). Urban Ecosystem Services: Tree Diversity and Stability of PM10 Removal in the Metropolitan Area of Rome. Annali Di Botanica. 4, 19–26. doi:10.4462/annbotrm-11746
McDonald, M. A., Healey, J. R., and Stevens, P. A. (2002). The Effects of Secondary Forest Clearance and Subsequent Land-Use on Erosion Losses and Soil Properties in the Blue Mountains of Jamaica. Agric. Ecosyst. Environ. 92 (1), 1–19. doi:10.1016/s0167-8809(01)00286-9
Mennis, J. (2003). Generating Surface Models of Population Using Dasymetric Mapping. The Prof. Geographer. 55 (1), 31–42. doi:10.1111/0033-0124.10042
Ministry of National Development (2019). Our Early Struggles. Our City, Our Home. Retrieved fromhttps://www.mnd.gov.sg/our-city-our-home/our-early-struggles
Moore, I. D., and Burch, G. J. (1986). Physical Basis of the Length-Slope Factor in the Universal Soil Loss Equation. Soil Sci. Soc. America J. 50 (5), 1294–1298. doi:10.2136/sssaj1986.03615995005000050042x
National Parks Board (2020a). Nature Ways. Gardens, Parks & Nature. Retrieved from https://www.nparks.gov.sg/gardens-parks-and-nature/nature-ways.
National Parks Board (2020b). Pasir Ris Park. Retrieved from https://www.nparks.gov.sg/gardens-parks-and-nature/parks-and-nature-reserves/pasir-ris-park.
National Parks Board (2020c). Pasir Ris Town Park. Retrieved fromhttps://www.nparks.gov.sg/gardens-parks-and-nature/parks-and-nature-reserves/pasir-ris-town-park.
NEAData.gov.sg (2020). Air Pollutant - Particulate Matter PM10 (Hr Mean -99th Percentile). Retrieved on from https://data.gov.sg/dataset/air-pollutant-particulate-matter-pm10.(accessed on June 1, 2020)
Nedkov, S., and Burkhard, B. (2012). Flood Regulating Ecosystem Services-Mapping Supply and Demand, in the Etropole Municipality, Bulgaria. Ecol. Indicators. 21, 67–79. doi:10.1016/j.ecolind.2011.06.022
Neo, X. (2020). Evolution of HDB Designs. The Straits Times. Retrieved from https://www.straitstimes.com/singapore/housing/evolution-of-hdb-designs.
Nesshöver, C., Assmuth, T., Irvine, K. N., Rusch, G. M., Waylen, K. A., et al. (2017). The Science, Policy and Practice of Nature-Based Solutions: An Interdisciplinary Perspective. Sci. Total Environ. 579, 1215–1227. doi:10.1016/j.scitotenv.2016.11.106
Ng, J. S. (2017). Parliament: HDB Flats Made up 73% of Singapore's Total Housing Stock in 2016. The Straits Times. Retrieved from https://www.straitstimes.com/politics/parliament- hdb-flats-made-up-73-of-singapores-total-housing-stock-in-2016.
Nowak, D. J., McHale, P. J., Ibarra, M., Crane, D., Stevens, J. C., and Luley, C. J. (1998). Modelling the Effects of Urban Vegetation on Air Pollution. in Air Pollution Modelling and its Application XII. Editors S. Grynin, and N. Chaumerliac (Plenum Press), 399–407. doi:10.1007/978-1-4757-9128-0_41
OpenStreetMap (2020). Openstreet Map of Singapore. Available online: https://www.openstreetmap.org/search?query=singapore#map=12/1.2905/103.8520 (accessed on December 1, 2018).
Panagos, P., Borrelli, P., Meusburger, K., Yu, B., Klik, A., Lim, K. J., et al. (2017). Global Rainfall Erosivity Assessment Based on High-Temporal Resolution Rainfall Records. Scientific Rep. 7 (1), 1–12. doi:10.1038/s41598-017-04282-8
Public Utilities Board (2016). Active, Beautiful, Clean Waters Programme. About ABC Waters. Retrieved from https://www.pub.gov.sg/abcwaters/about.
R Core Team, R. (2020). A Language and Environment for Statistical Computing. Vienna, Austria: R Core Team.
Reed, S. E., Boggs, J. L., and Mann, J. P. (2012). A GIS Tool for Modeling Anthropogenic Noise Propagation in Natural Ecosystems. Environ. Model. Softw. 37, 1–5. doi:10.1016/j.envsoft.2012.04.012
Renard, K., Foster, G., Weesies, G., McCool, D., and Yoder, D. (1997). Predicting Soil Erosion by Water: A Guide to Conservation Planning with the Revised Universal Soil Loss Equation (RUSLE). In Agriculture Handbook Number 703.
Richards, D., Fung, T. K., Meili, N., Song, X. P., Dissegna, A., Drillet, Z., et al. (2019). “An Ecosystem Service Design Loop for Using Vegetation to Mitigate the Urban Heat Island Effect,” in Future Cities Lab Indicia 2. Editors S. Cairns, and D. Tunas (Zurich, ZRH: Lars Müller Publishers)
Richards, D. R., and Edwards, P. J. (2017). Quantifying Street Tree Regulating Ecosystem Services Using Google Street View. Ecol. Indicators. 77, 31–40. doi:10.1016/j.ecolind.2017.01.028
Richards, D. R., Fung, T. K., Leong, R. A. T., Sachidhanandam, U., Drillet, Z., and Edwards, P. J. (2020a). Demographic Biases in Engagement with Nature in a Tropical Asian City. PLoS One. 15 (4), e0231576. doi:10.1371/journal.pone.0231576
Richards, D. R., Law, A., Tan, C. S. Y., Shaikh, S. F. E. A., Carrasco, L. R., Jaung, W., et al. (2020b). Rapid Urbanisation in Singapore Causes a Shift from Local Provisioning and Regulating to Cultural Ecosystem Services Use. Ecosystem Serv. 46, 101193. doi:10.1016/j.ecoser.2020.101193
Richards, D. R., and Thompson, B. S. (2019). Urban Ecosystems: A New Frontier for Payments for Ecosystem Services. People Nat. 1 (2), 249–261. doi:10.1002/pan3.20
Richards, D., Tan, B. A., Gaw, L. Y-F., and Masoudi, M. (2021). NCS2020: An R Package for Modelling Ecosystem Service Provision in Singapore. Available on Github:https://github.com/drexrichards/ncs2020 (Accessed March 13th, 2021). doi:10.5194/essd-2020-387-rc3
Richards, D., and Wang, J. W. (2020). Fusing Street Level Photographs and Satellite Remote Sensing to Map Leaf Area Index. Ecol. Indicators. 115, 106342. doi:10.1016/j.ecolind.2020.106342
Schröter, M., Barton, D. N., Remme, R. P., and Hein, L. (2014). Accounting for Capacity and Flow of Ecosystem Services: A Conceptual Model and a Case Study for Telemark, Norway. Ecol. Indicators. 36, 539–551. doi:10.1016/j.ecolind.2013.09.018
Seto, K. C., Fragkias, M., Güneralp, B., and Reilly, M. K. (2011). A Meta-Analysis of Global Urban Land Expansion. PLoS One. 6 (8), e23777. doi:10.1371/journal.pone.0023777
Shanahan, D. F., Cox, D. T. C., Fuller, R. A., Hancock, S., Lin, B. B., Anderson, K., et al. (2017). Variation in Experiences of Nature across Gradients of Tree Cover in Compact and Sprawling Cities. Landscape Urban Plann. 157, 231–238. doi:10.1016/j.landurbplan.2016.07.004
Shi, Y., Bilal, M., Ho, H. C., and Omar, A. (2020). Urbanization and Regional Air Pollution across South Asian Developing Countries – A Nationwide Land Use Regression for Ambient PM2.5 Assessment in Pakistan. Environ. Pollut. 266. doi:10.1016/j.envpol.2020.115145
Singstat, Data.gov.sg (2016). Singapore Residents by Subzone and Type of Dwelling. (Accessed June 15, 2020) Retrieved fromhttps://data.gov.sg/dataset/singapore-residents-by-subzone-and-type-of-dwelling-june-2016.
Skidmore, Owings., and Merrill, (2021b). Jigna Eco-Community. Accessed March 26, 2021, Retrieved fromhttps://www.som.com/projects/jigna_eco-community.
Song, X. P., Tan, P. Y., Edwards, P., and Richards, D. (2017). The economic benefits and costs of trees in urban forest stewardship: A systematic review. Urban For. Urban Gree. 29, 162–170. doi:10.1016/j.ufug.2017.11.017
Song, X. P., Richards, D., Edwards, P., and Tan, P. Y. (2018). Benefits of trees in tropical cities. Science 356, 1241. doi:10.1126/science.aan6642
Studio Dreiseitl, Rambo. (2019). Competition Win for Hafner-Ring in Constance. Germany: Press Release. Retrieved fromhttp://www.dreiseitl.com/data/NEWS/2019/Hafner_Constance_release.pdf. doi:10.46354/i3m.2019.emss.046
Tan, P. Y., and Samsudin, R. (2017). Effects of Spatial Scale on Assessment of Spatial Equity of Urban Park Provision. Landscape Urban Plann. 158, 139–154. doi:10.1016/j.landurbplan.2016.11.001
United Nations (2018). 68% of the World Population Projected to Live in Urban Areas by 2050, Says UN. News. Retrieved fromhttps://www.un.org/development/desa/en/news/population/2018-revision-of-world-urbanization-prospects.html.
United Nations Economic and Social Council (2016). Report of the Inter-Agency and Expert Group on Sustainable Development Goal Indicators. http://ggim.un.org/knowledgebase/Attachment1333.aspx?AttachmentType=1.
URA (2017). Enhanced LUSH to Take Urban Greenery to New Heights. Retrieved from https://www.ura.gov.sg/Corporate/Media-Room/Media-Releases/pr17-77.
URA (2014). Landscaping for Urban Spaces and High- Rises (LUSH) 2.0 Programme: Landscape Replacement Policy for Strategic Areas.
URA (2020b). Master Plan 2019 Land Use Layer. Data.gov.sg. Retrieved from https://data.gov.sg/dataset/master-plan-2019-land-use-layer.
URA (2020d). More Greenery & Play Spaces. Liveable and Inclusive Communities. Retrieved from https://www.ura.gov.sg/Corporate/Planning/Master-Plan/Themes/Liveable-and-Inclusive-Communities/More-Greenery-Play-Spaces.
URA (2020c). Sports And Recreation Greenery. Non- Residential Handbook. Retrieved from https://www.ura.gov.sg/Corporate/Guidelines/Development-Control/Non-Residential/SR/Greenery.
USDA (1986). Urban Hydrology for Small Watersheds. United States Department of Agriculture.(Issue Technical Release 55 (TR-55))
Van der Knijff, J. M., Jones, R. J. A., and Montanarella, L. (2000). Soil Erosion Risk Assessment in Europe. EUR 19044 EN. Luxembourg. Office for Official Publications of the European Communities. Available at:https://doi.org/citeulike-article-id:13481385.
WHO (2016). Urban Green Spaces and Health. Copenhagen: WHO Regional Office for Europe. Available at:https://www.euro.who.int/__data/assets/pdf_file/0005/321971/Urban-green-spaces-and-health-review-evidence.pdf.
Wischmeier, W. H., and Smith, D. D. (1978). Predicting Rainfall Erosion Losses—A Guide to Conservation planning.U.S. Department of Agriculture, Agriculture Handbook No. 537. Available online:https://naldc.nal.usda.gov/download/CAT79706928/PDF.
Wolff, S., Schulp, C. J. E., and Verburg, P. H. (2015). Mapping Ecosystem Services Demand: A Review of Current Research and Future Perspectives. Ecol. Indicators. 55, 159–171. doi:10.1016/j.ecolind.2015.03.016
Wong, D. (2018). Nature to Play a big Part in Future HDB Estates. The Straits Times. Retrieved from https://www.straitstimes.com/singapore/housing/nature-to-play-big-part-in-future-hdb-estates.
Yee, A. T. K., Chong, K. Y., Neo, L., and Tan, H. T. W. (2015). Updating the Classification System for the Secondary Forests of Singapore. RAFFLES BULLETIN ZOOLOGY. 11–21.
Yeo, S. J. (2016). Tengah to be Developed into a ‘Forest Town’. The Straits Times. Retrieved fromhttps://www.straitstimes.com/singapore/housing/tengah-to-be-developed-into-a-forest-town.
Zhang, W., Dulloo, E., Kennedy, G., Bailey, A., Sandhu, H., and Nkonya, E. (2019). “Biodiversity and Ecosystem Services,” in Sustainable Food and Agriculture. Editors C. Campanhola, and S. Pandey (Academic Press), 137–152. doi:10.1016/b978-0-12-812134-4.00008-x
Keywords: natural capital, land cover, environmental modeling, urban planning, scenarios
Citation: Tan BA, Gaw LY-F, Masoudi M and Richards DR (2021) Nature-Based Solutions for Urban Sustainability: An Ecosystem Services Assessment of Plans for Singapore’s First “Forest Town”. Front. Environ. Sci. 9:610155. doi: 10.3389/fenvs.2021.610155
Received: 25 September 2020; Accepted: 15 April 2021;
Published: 30 April 2021.
Edited by:
Teodoro Semeraro, University of Salento, ItalyReviewed by:
Weiqi Zhou, Research Center for Eco-environmental Sciences (CAS), ChinaChiara Cortinovis, Humboldt University of Berlin, Germany
Copyright © 2021 Tan, Gaw, Masoudi and Richards. This is an open-access article distributed under the terms of the Creative Commons Attribution License (CC BY). The use, distribution or reproduction in other forums is permitted, provided the original author(s) and the copyright owner(s) are credited and that the original publication in this journal is cited, in accordance with accepted academic practice. No use, distribution or reproduction is permitted which does not comply with these terms.
*Correspondence: Beverley Anne Tan, beverley.annetherese@gmail.com; Leon Yan-Feng Gaw, gaw@arch.ethz.ch