- 1Department of Agroecology, Aarhus University, Tjele, Denmark
- 2Aarhus University Centre for Circular Bioeconomy, Aarhus University, Tjele, Denmark
- 3Department of Animal Science, Aarhus University, Tjele, Denmark
Paludiculture, and in particular the cultivation of perennial grasses as biomass feedstock for green biorefineries, may be an economic and environmentally sustainable option for agricultural peatlands in temperate regions. However, the optimal biomass quality for protein extraction from flood-tolerant grasses is largely unknown. The aim of this study was to define the combined effect of harvest and fertilization frequency, with one to five annual cuts, on protein yield and extractability for the grasses tall fescue (TF) and reed canary grass (RCG), cultivated on an agricultural fen peatland in Denmark.The content of protein fractions was determined according to the Cornell Net Carbohydrate and Protein System (CNCPS). We assessed protein extractability by lab-scale biorefinery techniques using a screw-press followed by acid precipitation of true protein. The two methods were compared to correlate potential extractable protein yields with actual biorefinery outputs. We found the highest annual biomass and crude protein (CP) yields in the two cut treatments, with 13.4 and 15.6 t dry matter (DM) ha−1 year−1, containing 2.9–3.4 t CP ha−1 year−1 for TF and RCG, respectively. The highest neutral-extractable (fractions B1 and B2) true protein yields of 1.1 and 1.5 t ha−1 year−1 were found in the two cut treatments, representing 39% (TF) - 45% (RCG) of total CP. Using biorefining techniques, we were able to precipitate up to 2.2 t DM ha−1 year−1 of protein concentrate, containing up to 39% CP. Significant correlations between methods were found, with a distinct relationship between CNCPS fractions B1 + B2 and CP yield of the protein concentrate, indicating the suitability of the CNCPS as an indicator for extractable protein yields. Biomass and CP yields were not significantly improved beyond two annual cuts. However, timing and harvest frequencies significantly affected plant maturity and consequently extractable CP contents and protein concentrate yields. We conclude that TF and RCG are promising feedstocks for green biorefineries due to high biomass, extractable CP, and protein concentrate yields, and highlight the potential of flood-tolerant grasses, cultivated on wet agricultural peatlands, for an enhanced valorisation beyond the common utilisation for bioenergy.
Introduction
With an increasing world population, being expected to reach 9.7 billion in 2050 (United Nations, 2019), and the associated escalating demand for food and clean water (Ibarrola-Rivas et al., 2017) the competition for productive land areas is growing (Joosten et al., 2016). At the same time, there is an environmental crisis, with never seen records of ocean temperature and sea level rise, as well as atmospheric greenhouse gas (GHG) concentrations (Kappelle, 2020). Peatlands, being the dominating wetland type globally (Yu et al., 2010) and covering about 3% of the continental surface (Joosten and Clarke, 2002) contribute significantly to GHG emissions. Anthropogenic degradation of peatlands by drainage for agriculture led not only to a substantial loss of global peatlands and the associated ecosystem functions (Wichmann, 2017), but also to emission hotspots releasing 6% of the total anthropogenic emissions in carbon dioxide equivalents (CO2eq) (Joosten, 2012), contributing up to 37% of the total emissions from the European agricultural sector (Geurts, et al., 2019).
A transition towards more sustainable cultivation practices on peatlands after rewetting may accelerate the extensification of currently drained peatlands. Paludiculture, defined as agriculture on wet or rewetted peatlands, has been proposed as a suitable mitigation strategy (Günther et al., 2014) in order to reverse traditional farming with unsustainable environmental concerns such as land subsidence, nutrient leaching and GHG emissions to the atmosphere (Tanneberger and Wichtmann, 2011; Giannini et al., 2017). In particular, the production of biomass from flood-tolerant perennial grasses for the provision of e.g., fodder and raw materials (Wichtmann and Wichmann, 2011a) or for the purpose of renewable energy (Kandel et al., 2013b) is a well-known option for an economic biomass utilisation. Nevertheless the revenues of the peatland biomass for these purposes have so far been too low to provide a competitive sustainable business plan.
Previous research has shown promising results on the use of perennial grasses as feedstock for protein extraction (Kromus et al., 2006) with subsequent application as a high-quality substitute for soy. Current estimates indicated that up to 47% of the crude protein (CP) fraction present in the biomass can be extracted in a protein concentrate that may substitute the currently used soybean meal in diets for monogastric animals (Santamaría-Fernández et al., 2017; Stødkilde et al., 2018; la Cour et al., 2019). Furthermore, by-products resulting from the biorefining process, such as the fibre fraction and the brown juice, may additionally be used directly as valuable forage for ruminants (Damborg et al., 2018) or can be further refined into value-added products (Ambye-Jensen et al., 2014).
The flood-tolerant perennial grasses reed canary grass (Phalaris arundinacea) (RCG) and tall fescue (Festuca arundinacea) (TF) can produce high biomass yields on poorly drained peatsoils (Kandel et al., 2013a; Kandel et al., 2016). While there has been extensive research concluding their suitability as efficient energy crops (Kandel et al., 2013b; Tilvikiene et al., 2016), a further assessment of their applicability as biomass feedstock for value-added products from biorefineries has been lacking so far. Assessments of CP contents in RCG and TF cultivated on mineral soils showed yields of up to 3.2 t CP ha−1 year−1 and 3.1 t CP ha−1 year−1, respectively, of which about 40% were potentially extractable (Solati et al., 2018b). However, there is a desideratum concerning the CP yield and extractability of these grass species cultivated on wet agricultural peatlands. This also applies to the evaluation of biomass quality for protein extraction as a response to plant maturity and nitrogen (N) uptake, and hence the effect of agricultural management regarding annual harvest and fertilization frequencies.
Until now, there has been research on the content of potential extractable protein fractions in grasses and legumes (e.g., Elizalde et al., 1999; Solati et al., 2017), mainly in order to determine forage quality, following the CNCPS. Further, efforts regarding protein extraction optimisation are at the heart of green biorefinery strategies (Santamaria-Fernandez et al., 2019; Pihlajaniemi et al., 2020) and increasing attention has been paid on the protein extractability and quality using the rather simple method of mechanical screw-press extraction followed by chemical protein precipitation (Stødkilde et al., 2018; Damborg et al., 2020). However, to the authors knowledge, no correlation between the theoretical extractable protein yields as indicated by means of the soluble CNCPS fractions B1 + B2, respectively acid extractable fractions B1 + B2 + B3, and the actual protein yields by precipitation in biorefineries, has yet been conducted. The protein content and allocation in grasses depends on a variety of factors, including environmental conditions, stress factors (e.g., drought; Roy-Macauley et al., 1992) and N availability. Generally, grasses are known to positively respond to N input by fertilization, with reported linear increases of 50–90 g CP kg−1 DM per 100 kg N ha−1 applied (Peyraud and Astigarraga, 1998) as well as a significant decrease of CP content with N limitation (Dindová et al., 2019). However, the process of lignification in grasses was also found to be positively associated with N fertilisation (Coblentz et al., 2017).
Nevertheless, grass maturity and harvesting time are known to be important factors for biomass quality (Butkutė et al., 2013; Solati et al., 2017). Plant nitrogen is mainly stored as protein in chloroplast (Peoples and Dalling, 1988), and hence located in the form of true proteins within leaves. It has further been shown, that leaf-protein contents decrease with plant maturity as a reaction to senescence and subsequent lignification (Sanderson and Wedin, 1989), contributing to a substantial increase in total plant lignin to about 30% (Butkutė et al., 2013). These constant alterations with increasing plant development facilitate bounding of proteins to cell walls, and hence increases the content of insoluble protein fractions B3 and C according to the CNCPS method, while the soluble protein fractions B1 and B2 decrease.
We hypothesized that high protein yields and extractability can be obtained in flood-tolerant perennial grasses by manipulating the harvest frequency during the growth season under provision of adequate nutrient availability. The aims of this study were to, 1) determine the combined effect of harvest and fertilization frequency on biomass and protein yields of the perennial grasses RCG and TF grown on a wet fen peatland, 2) quantify theoretically and potentially extractable protein fractions by the CNCPS method, and 3) assess the extractability of soluble plant protein fractions using a screw-press as the initial biorefining separation step followed by protein precipitation.
Materials and Methods
Site Description
The field experiment was conducted in 2019 on a riparian fen in Vejrumbro, located in Central Jutland, Denmark (56°26′15.3″N, 9°32′44.1″E; Figure 1). Environmental variables were taken from the meteorological station at Foulumgård, Aarhus University, located 6.5 km from the field site. During the study period from May until October 2019, ranged the temperature between 8.7 and 16.8°C, with August as the warmest month. Monthly precipitation ranged between 57 and 122 mm, with May as the driest and October as the wettest month. The site has been shallow drained in the first half of the 20th century and has primarily been used as pasture until the establishment of the present experiment in 2018. Average peat depth at the site is 2 m, overlying a layer of 8 m gyttja. The upper soil layer (0– 20 cm) contains 35.8% total carbon (TC), 2.5% total nitrogen (TN), 0.2% phosphorus (P), 0.2% potassium (K), with a bulk density (BD) of 0.2 g cm−3, and an average pH of 5.1. The lower soil layer (20–100 cm) contains 45.6% TC, 2.6% TN, with 0.1 g cm−3 BD, and pH 5.8. The site had in 2019 a mean annual water table depth (WTD) of −0.13 cm, with on average −0.22 cm during the study period.
Experimental Design and Agricultural Management
The effects of species and combined harvest and fertilizer management were tested within a complete split-plot design, with four block replicates, species as main plot and management as sub-plot. The five management sub-plots (3 m × 16 m) were assigned randomly on the established main plot. A total of eight plots (15 m × 16 m) were established in June 2018, with four plots each cultivated with either RCG (Phalaris arundinacea, cultivar: Lipaula) or TF (Festuca arundinacea, cultivar: Swaj). Soil cultivation and sowing of the seeds occurred in one mechanical process following previous spraying of original vegetation using Round Up (Bayer AG, Leverkusen, Germany). The seeding rates were 25 kg ha−1 for both species and all subplots in each plot were fertilized with 40 kg N ha−1, 9 kg P ha−1, and 43 kg K ha−1, using 286 kg ha−1 of combined NPK fertilizer with a grade of 14-3-15, after emergence of the seeds. Treatments with TF experienced partial invasion by velvet grass (Holcus lanatus). In 2019, subplots were fertilized and harvested according to the treatment plan (Figure 2). The one cut treatment was fertilized with 100 kg N ha−1 year−1 and 100 kg K ha−1 year−1. Split-fertilizer applications with a total of 200 kg N ha−1 year−1 and 200 kg K ha−1 year−1 were applied to two cut, three cut, four cut, and five cut strategies in equal doses for each harvest within each strategy. First fertilization prior to growth onset was performed on April 16, 2019. The remaining split-fertilizer applications were applied the day following the last harvest day for each occurrence. No fertiliser was applied following the last cut. The grass species were harvested up to five times, depending on treatment, in the growth period of May 12 to October 16, 2019, between 15.00 and 18.00 CET. Grass samples were harvested in stripes of between 1.5 and 3.0 m length and 1.18 m width at stubble height of approximately 7 cm within the middle of the sub-plots, using a sickle bar mower (Grillo G107, Grillo SpA, Cesena, Italy). The grass was manually collected and immediately brought to the laboratory for determination of fresh weight and subsequently dry-matter. The dried plant material was milled (Retsch ZM 200, Retsch GmbH, Haan, Germany) and stored for further analysis.
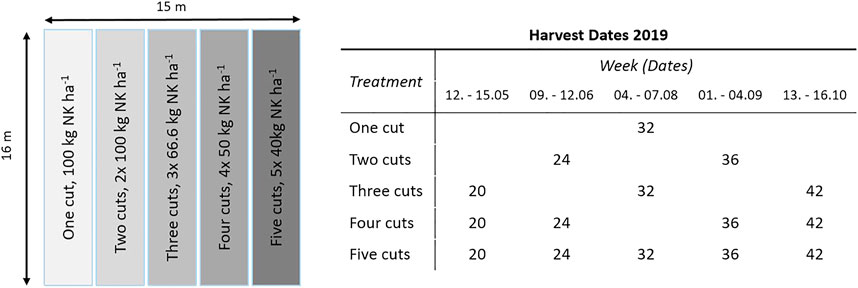
FIGURE 2. Exemplary split-plot distribution in one main plot, indicating number of annual cuts and corresponding fertilization rates. The distribution of subplots has been randomized on each main plot. Harvest weeks and dates are given for the corresponding treatments and harvest occurrences.
Screw-Pressing and Protein Extraction
A portion of the harvested grass was stored overnight at 2°C and processed the following morning at room temperature in a lab-scale twin-screw-press (Angelia 8500S Angel slow-juicer, Angel Co. Ltd., Busan, Korea) without prior chopping or discarding of wilted plant fragments or weeds. The resulting pulp and a sample fraction of the juice were frozen at −18°C. The grass protein was isolated from the remaining juice fraction using a two-step procedure as described by Stødkilde et al. (2019). Proteins were precipitated by acidifying the juice to pH 4, using 14.8 mol L−1 phosphoric acid. Following overnight-storage at 4°C, the sample was centrifuged for 12 min at 2000×g at 4°C for final separation of the acid-precipitated protein from the juice, leading to a protein concentrate and the residual brown juice fraction. All samples were stored frozen at −18°C until drying at 60°C for further analysis. Biomass samples from the one cut treatment could not be processed by the screw-press due to advanced lignification. Total nitrogen (TN) of the screw-pressed fractions were analysed using a Vario MAX CN (Elementar Analysesysteme GmbH, Hanau, Germany), with following conversion to crude protein (CP) by using the conversion factor 6.25 (Eq. 1). CP within screw-pressed fractions is referred to as CP content within this text.
Protein Fractionation Following Cornell Net Carbohydrate and Protein System
Fractionation of proteins within the dried whole grass sample was performed following the Cornell Net Carbohydrate and Protein System (CNCPS) as described by Licitra et al. (1996) and as modified by Solati et al. (2018a). Total nitrogen in the plant material was determined using the Kjeldahl method (Kjeltec 8400, FOSS A/S, Hillerød, Denmark) with subsequent conversion to CP by using the conversion factor 6.25 (Eq. 1) as described by AOAC (1990), and within the text further referred to as CP content. The fractions A, B1 and B2 are soluble in neutral detergent solution, whereas B3 is soluble in acid detergent solution. Fraction A was determined by subtracting trichloroacetic acid (TCA)-precipitated proteins from TN (Eq. 2). Fraction B1 was determined by subtracting the borate-phosphate buffer insoluble N from the TCA insoluble N (Eq. 3). Fraction B2 was estimated by subtracting the neutral-detergent insoluble N (NDIN) from the borate-phosphate buffer insoluble N (Eq. 4). Determination of NDIN was based on a neutral detergent fibre (NDF) assay where 50 μl of heat stable amylase (No. A3306, Sigma-Aldrich, Denmark) was added to the sample, following the procedure described by Van Soest et al. (1991). Both NDF and acid-detergent fibre (ADF) included the residual ash. The insoluble protein fraction B3 was determined by subtracting the acid-detergent insoluble N (ADIN) from NDIN (Eq. 5). Fraction C was equal to ADIN (Eq. 6). ADF and NDF analyses were performed on a FOSS Fibertech 2010 (FOSS A/S, Hillerød, Denmark).
Statistical Analysis
Mean yields per cut were summed up to annual yields for treatments with more than one harvest event. Standard deviations are reported to present the distribution of data. Analysis was performed using a linear mixed model with the function lmer of the package lme4 (Bates et al., Version 1.1-23, 2015) in the statistical software R [R Core Team (2020) Version 4.0.2 – “Taking Off Again”], in which the following model was used:
Where
Results
Biomass Yield
Annual average biomass yield across treatments was on average 34% higher in reed canary grass (RCG) than in tall fescue (TF) treatments (Table 1). In particular, RCG four and five cut treatments yielded, respectively, 50 and 54% more than TF treatments. The overall highest annual DM yields for both species were found in the two cut treatments, with 15.6 (±7.7) t DM ha−1 year−1 for RCG and 13.4 (±4.8) t DM ha−1 year−1 for TF. However, for RCG, there was no difference between the two, four (15.4 (±7.0) t DM ha−1 year−1) and five cut (14.9 (±5.1) t DM ha−1 year−1) treatments. In treatments receiving 200 kg NK ha−1 year−1 of fertilization (two, to five cut), harvest frequencies did not significantly (p > 0.05) affect the annual biomass yield. The one cut treatment yielded for both species least biomass and was not significantly different to the three cut treatment (p > 0.05).
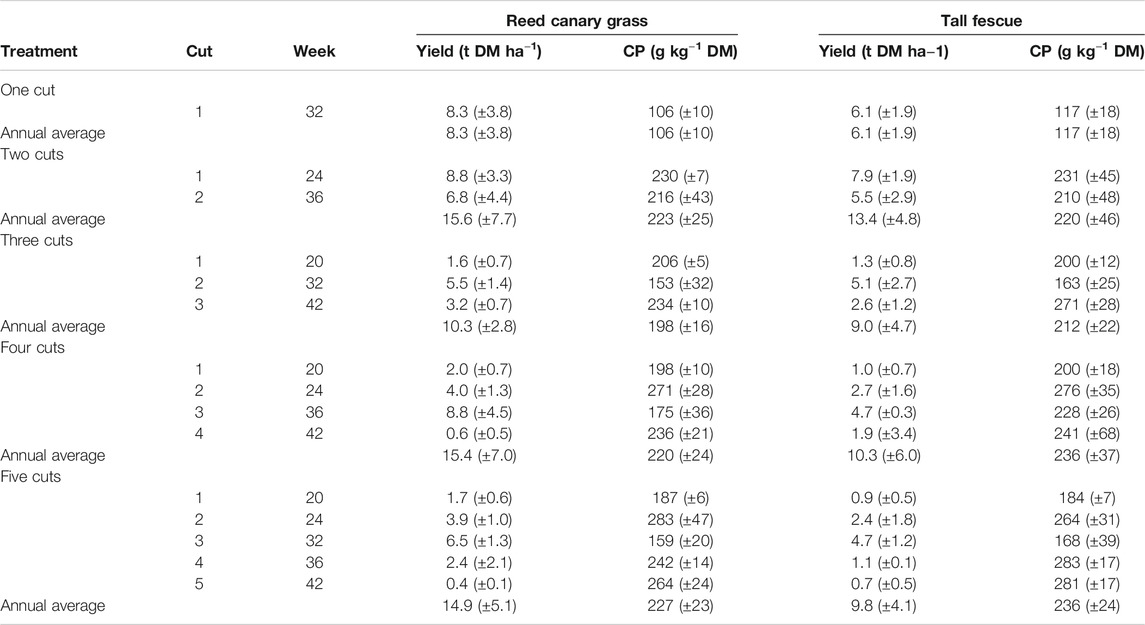
TABLE 1. Total annual average biomass yields in dry matter (t DM ha−1) and averaged crude protein (CP) contents (g kg−1 DM) per harvest and treatment for reed canary grass and tall fescue. Standard deviation is giving in brackets.
Crude Protein in Biomass
The CP content (g kg−1 DM) of the two grass species was similar, with TF having significantly higher contents as compared to RCG only in weeks 36 (four-, and five cuts) and 42 (three cuts). Further, the content as well as the annual average crude protein yield (in t ha−1 year−1) were significantly affected by the number of annual cuts [χ2 (4) = 29.9, p < 0.001] as well as harvest date [χ2 (4) = 77.9, p < 0.001]. The mean annual CP content of RCG and TF increased from 106 (±10) g kg−1 DM and 117 (±18) g kg−1 DM for one cut treatments to 227 (±23) g kg−1 DM and 236 (±24) g kg−1 DM for five cut treatments, respectively (Table 1). However, there was no difference between averaged annual CP content for the two to five cut treatments. Further, a general significant decline in CP content was observed in all treatments during summer growth. This applied for stands harvested in late July and August. The highest annual CP yields in biomass for both species were found in the two cut treatments, with 3.4 (±0.9) t ha−1 year−1 for RCG and 2.9 (±0.4) t ha−1 year−1 for TF. However, for RCG there was no difference in CP yields for the two, four [3.0 (±0.1) t ha−1 year−1], and five cut [3.1 (±0.0) t ha−1 year−1] treatments. The lowest yield was, with 0.7–0.9 t ha−1 year−1, found in the one cut treatment for TF and RCG, respectively.
CNCPS Fractions in Biomass
Fraction A
The content of fraction A ranged between 28 g kg−1 DM (RCG, one cut) and 63 g kg−1 DM (TF, two cuts) on an annual average per treatment, and was more affected by harvest date [χ2 (4) = 36.3, p < 0.001] than number of annual cuts [χ2 (4) = 18.4, p < 0.01]. However, species did not affect fraction A content. Initial increases in the content of fraction A were observed during spring growth in the period between May and June for the four and five cut treatments of both species (Figure 3B). However, this was followed by a decline over summer months. A subsequent significant change (p < 0.001) was observed for RCG (three cuts), with a relative increase from 23% (week 20) to 50% (week 42) based on total CP. On an annual average basis, has fraction A only been slightly decreasing with increasing number of harvests (Supplementary Tables S1, S2).
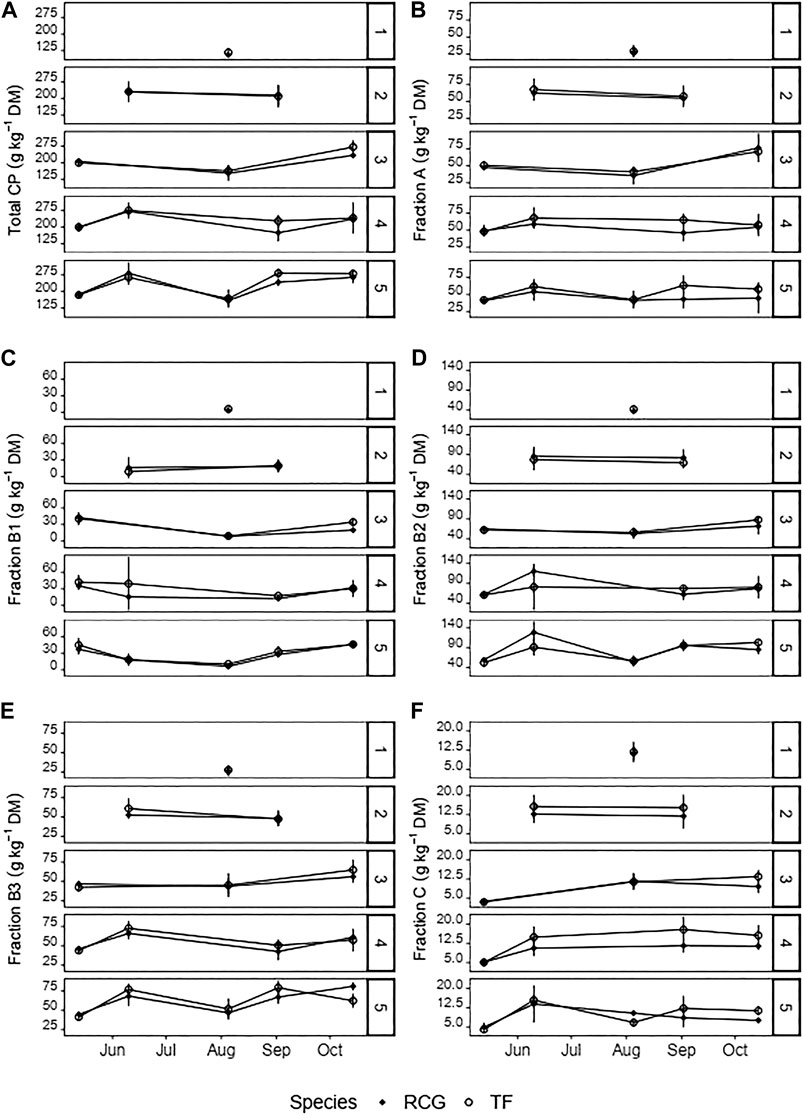
FIGURE 3. Contents of (A) crude protein (CP), and (B–F) protein fractions according to the Cornell Net Carbohydrate and Protein System (CNCPS) in the biomass (g kg−1 DM) for the various treatments and species on the different harvest dates. Attention should be paid to the different magnitudes of the y-axes. Error-bars show standard deviations.
Fraction B1
The biomass content of the neutral buffer soluble protein fraction B1 ranged between 3 g kg−1 DM (RCG, one cut) and 32 g kg−1 DM (TF, four cuts) on an annual average per treatment. Based on total CP, this equaled to between 3 and 14% for RCG (one cut) and TF (four cuts), respectively. The three to five cut treatments for both species showed the highest average annual contents with 24–27 g kg−1 DM (RCG) and 28–31 g kg−1 DM (TF). Harvest week [χ2 (4) = 100.5, p < 0.001] and cut frequency [χ2 (4) = 22.2, p < 0.001] affected the contents of fraction B1. Fraction B1 declined in all treatments in dependency of plant maturity, with the lowest average values in week 32 (Figure 3C).
Fraction B2
The neutral detergent soluble protein, fraction B2, was the largest fraction in relation to CP and was affected by the number of annual cuts [χ2 (4) = 22.2, p < 0.001] In harvests during the spring growth period, RCG had a higher content of fraction B2 than TF, with a significant difference for the four and five cut treatments. However, spring growth led to a significant increase of fraction B2 for the four and five cut treatments of both, RCG and TF, showing in week 24 contents of 120 g kg−1 DM and 129 g kg−1 DM (RCG), and 81 g kg−1 DM and 91 g kg−1 DM (TF), respectively (Figure 3D). Relative to total CP, remained average annual contents of fraction B2 constant over all treatments within a range of 32–38% for RCG, and 32–36% for TF.
Fraction B3
The annual average content of the hemicellulose-bound protein fraction B3 increased with increasing number of annual cuts from 26 g kg−1 DM to 61 g kg−1 DM (RCG) and 28 g kg−1 DM to 62 g kg−1 DM (TF). However, the share of B3 relative to total annual CP remained constant with 23% (RCG, two cuts)–27% (RCG, five cuts) throughout all treatments and species. In line with contents of other fractions, a decline of B3 during summer cuts was observed (Figure 3E). Fraction B3 content was significantly affected by number of annual cuts [χ2 (4) = 67.1, p < 0.001] and harvest week [χ2 (4) = 78.2, p < 0.001]. There was no effect of species on B3 content (p > 0.05).
Fraction C
The cellulose- and lignin-bound protein fraction C was more affected by harvest date [χ2 (4) = 128.9, p < 0.001] than number of annual cuts (p > 0.01) or species (p > 0.05). The one cut treatment of both species had the highest share with 11% (11 g kg−1 DM) in RCG and 10% (12 g kg−1 DM) in TF of total CP. However, a decline in the annual average content of fraction C relative to total CP was observed with increasing number of annual cuts, with 4% each, or 9 g kg−1 DM (RCG) and 10 g kg−1 DM (TF), for the five cut treatments. Fraction C was lowest in early spring cuts (week 20) with contents between 2% or 3 g kg−1 DM (TF, three cuts), to 3% or 5 g kg−1 DM, (RCG and TF, four cuts) relative to total biomass CP (Figure 3F).
Cumulative Neutral Extractable and Acid Extractable Protein Fractions
The groups of combined neutral extractable fractions B1 and B2 (soluble proteins) as well as the acid extractable fractions B1, B2 and B3 (potentially extractable protein) both followed the patterns of biomass CP yield in t DM ha−1 (Figures 4A–C), as well as annual average CP in t ha−1 year−1 from biomass harvest, across all treatments. The share of the cumulative fractions B1 and B2 ranged from 38% (RCG, one cut) to 49% (RCG, five cuts; and TF, three cuts) relative to total CP (Table 2), equivalent to 41 g kg−1 DM (RCG, one cut) and 112 g kg−1 DM (RCG, five cuts). The lowest neutral extractable protein contents in biomass (61 g kg−1 DM, respectively 67 g kg−1 DM for RCG and TF, five cuts) was found in summer cuts (week 32). Based on annual average yields of biomass in t DM ha−1 year−1, RCG yielded in all treatments more of the cumulative fractions B1 and B2 than TF. The two-cut management for both species yielded with 1.5 t DM ha−1 year−1 (RCG) and 1.1 t DM ha−1 year−1 (TF) most. There were no significant differences between yields of two, four, and five cut treatments of RCG, as well as two and five cuts of TF (Figure 5). The share of neutral extractable protein fractions to total CP yield increased from 39% (one cut) to 47% (five cuts) for RCG, whereas this increase in share was not observed for the TF species.
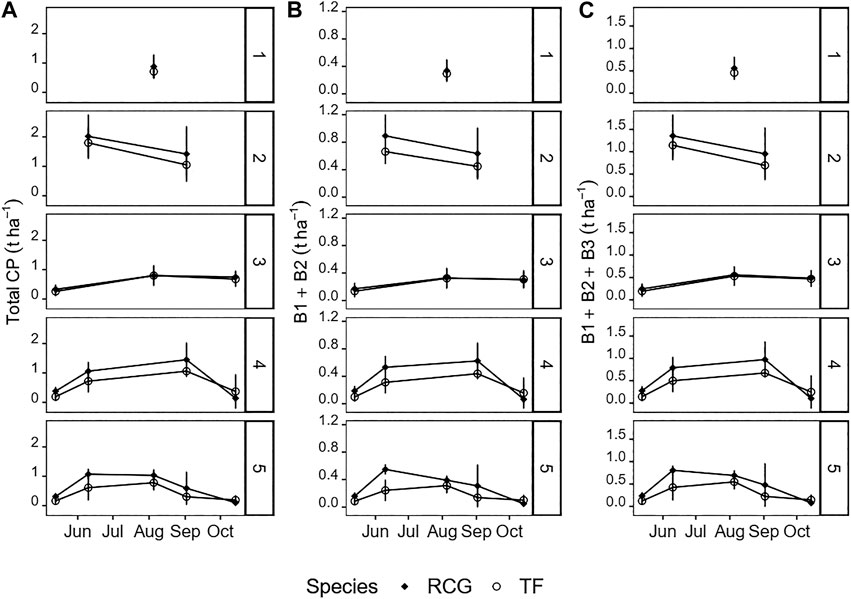
FIGURE 4. Yields (t DM ha−1) of (A) total crude protein (CP), (B) protein fractions B1 + B2, and (C) protein fractions B1 + B2 + B3 for the various treatments and species on the different harvest dates. Attention should be paid to the different magnitudes of the y-axes. Error-bars show standard deviations.
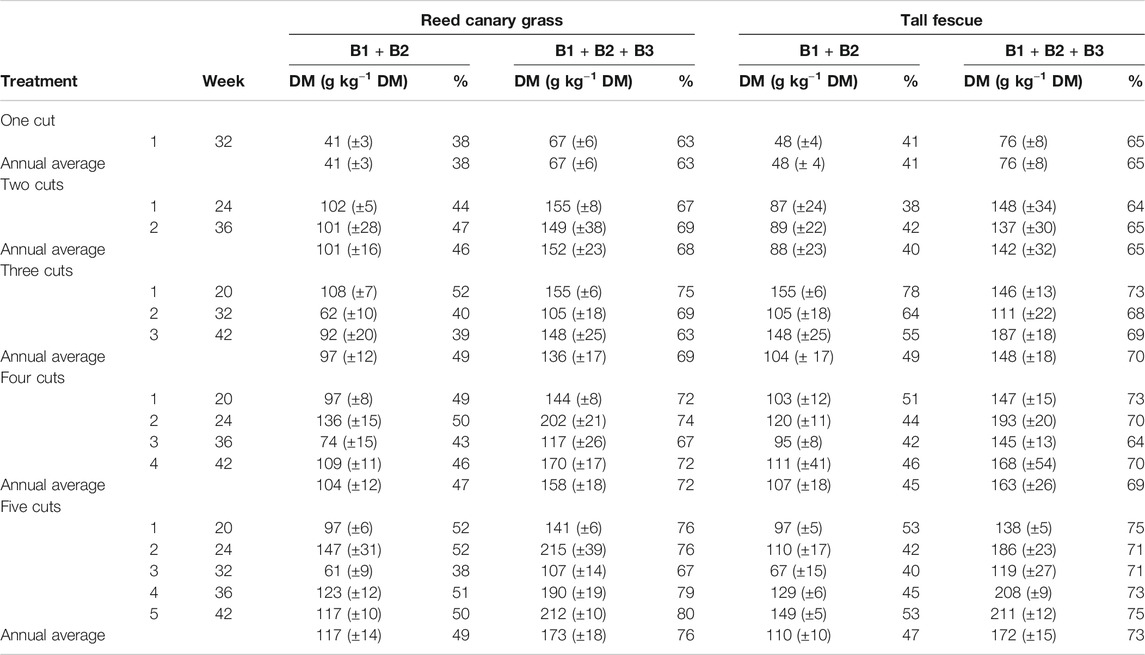
TABLE 2. Share of neutral extractable protein fractions B1 + B2, and potentially extractable fractions B1 + B2 + B3 based on total biomass crude protein (CP) for reed canary grass and tall fescue in dry matter (DM).
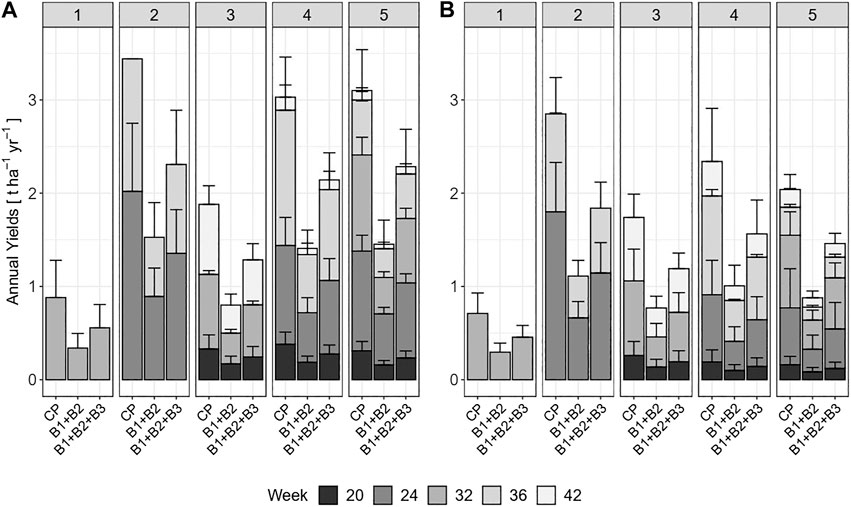
FIGURE 5. Cumulative annual yields (t ha−1 year−1) and yields per cut of crude protein (CP), protein fractions B1 + B2, and B1 + B2 + B3 for the various treatments in (A) reed canary grass, and (B) tall fescue. Error-bars show standard deviations.
However, including the potentially acid extractable protein fraction B3 raised the theoretical extractability to between 63% (RCG, one cut) as well as 76% (RCG, five cuts), equivalent to 67 g kg−1 DM and 173 g kg−1 DM, respectively. RCG had higher contents of both combined B1 and B2, as well as B1 + B2 + B3, during spring growth as compared to TF (Figure 4). Cumulative fractions of B1 + B2 + B3 were only marginally higher in the two cut treatments (2.3 t DM ha−1 year−1 for RCG, 1.8 t DM ha−1 year−1 for TF) than in four and five cut treatments (Figure 5). On an annual yield basis, the potentially extractable fraction B3 increased the theoretical protein yield by on average 25% as compared to B1 + B2 based on total CP (g kg−1 DM) for both species across all treatments, with the two and five cut treatments experiencing the highest increase with 27% for RCG and 28% for TF.
Screw-Pressed Fractions
Of the fractions produced during biorefining of biomass, the pulp fraction had the lowest CP content, which was highest in TF treatments with 119 g kg−1 DM (two cut)–164 g kg−1 DM (five cuts). CP in the residual brown juice ranged between 138 g kg−1 DM (RCG, five cuts) and 206 g kg−1 DM (TF, four cuts). The precipitated protein fraction had the highest CP content in the five cut treatment for TF with 393 g kg−1 DM, and for RCG in the four cut treatment with 383 g kg−1 DM. Lowest contents in precipitated protein fractions were observed in three cut treatments for both species (Tables 3, 4). Common for all fractions, had summer cuts the lowest CP contents, whereas late-spring and late-autumn cuts showed the highest values.
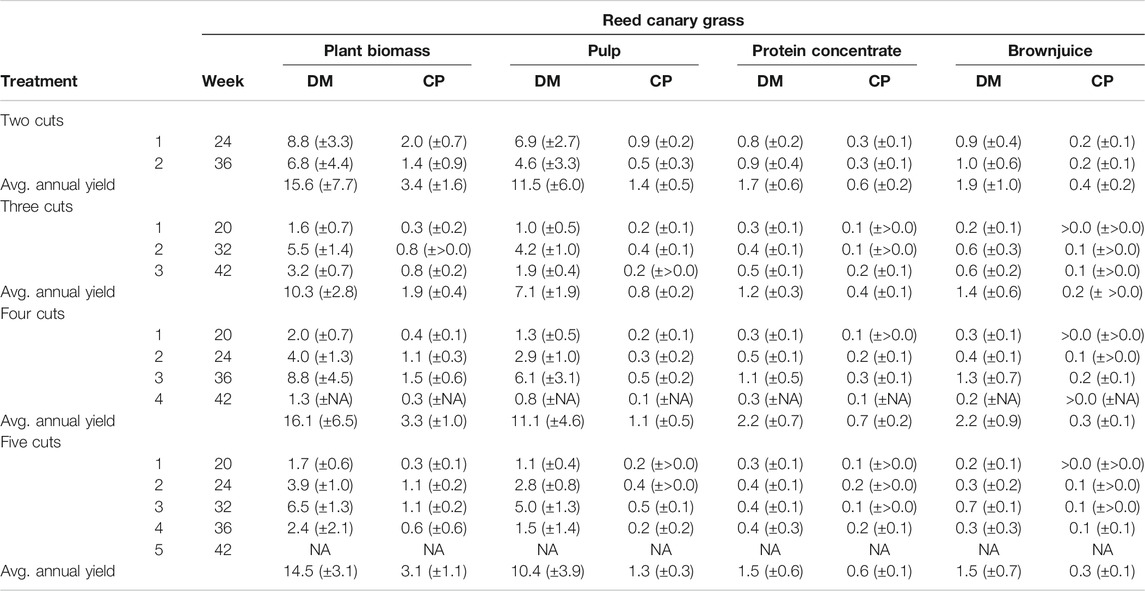
TABLE 3. Dry matter (DM) and crude protein (CP) yields in plant biomass and screw-pressed fractions for reed canary grass. All yields in t ha−1 year−1. Standard deviation (SD) is giving in brackets. Missing SD values due to only one replicate as a consequence of insufficient biomass for processing. NA’s due to a lack of sufficient biomass for processing with the screw-press.
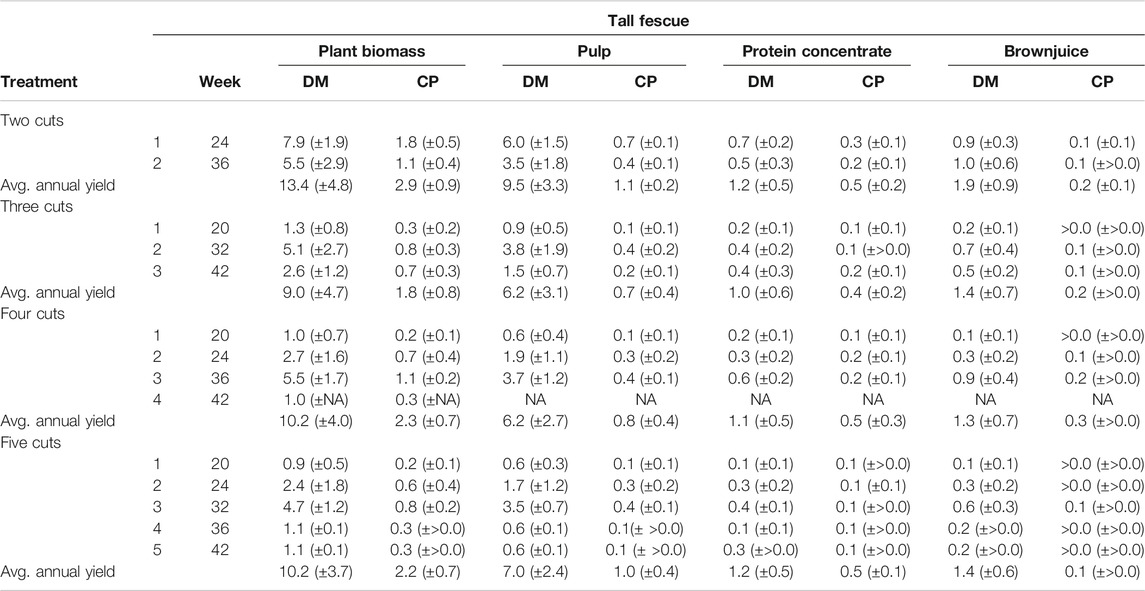
TABLE 4. Dry matter (DM) and crude protein (CP) yields in plant biomass and screw-pressed fractions for tall fescue. All yields in t ha−1 year−1. Standard deviation (SD) is giving in brackets. Missing SD values due to only one replicate as a consequence of insufficient biomass for processing with the screw-press.
Cumulative annual CP yields of precipitated protein concentrate were significantly affected by harvest date [χ2 (4) = 42.8, p < 0.001], indicating plant maturity, as also the number of annual cuts [χ2 (3) = 25.8, p < 0.001]. Between 1.0 t DM ha−1 year−1 (TF, three cuts) and 2.2 t DM ha−1 year−1 (RCG, four cuts) of the protein concentrate could be precipitated, resulting in protein concentrate CP yields of 0.4–0.7 t DM ha−1 year−1, respectively (Tables 3, 4). Pulp fraction yield (t DM ha−1 year−1) was highest in the two cut treatments, representing 72% (TF) and 73% (RCG) of the total annual biomass input. The CP yield in pulp ranged between 0.7 t DM ha−1 year−1 (TF, three cuts) and 1.4 t DM ha−1 year−1 (RCG, two cuts), representing 29% (RCG, five cuts) and 45% (TF, five cuts) on a mass percentage basis. The brown juice fraction yielded least CP in t DM ha−1 year−1 as well as on a percentage basis. The lowest CP yields in all fractions were observed in early-spring cuts as well as late-autumn cuts in frequent-harvest treatments following an initial increase in yieldable CP during late-summer (Figures 6A–D). Losses within the CP balance originate from foaming and related overflow of juice during screw-pressing as well as fibre residues left in the screw-press (Figure 7). Composition of DM (g kg−1 FW) and CP contents (g kg−1 DM) for the different fractions and treatments are provided in the Supplementary Material.
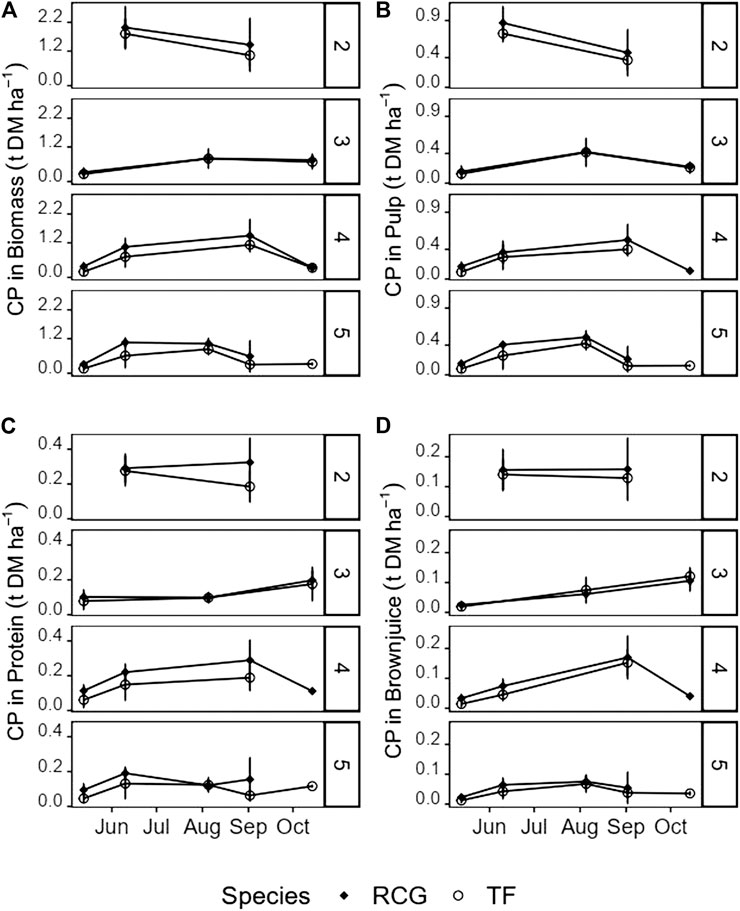
FIGURE 6. Yields of crude protein (t ha−1) in (A) unseparated biomass, and the screw-pressed fractions (B) pulp, (C) protein precipitate, and (D) brownjuice for the various treatments and species on the different harvest dates. Attention should be paid to the different magnitudes of the y-axes. Missing values for late cuts of the four, and five cut treatments due to insufficient biomass for juicing in the screw-press. Error-bars show standard deviations.
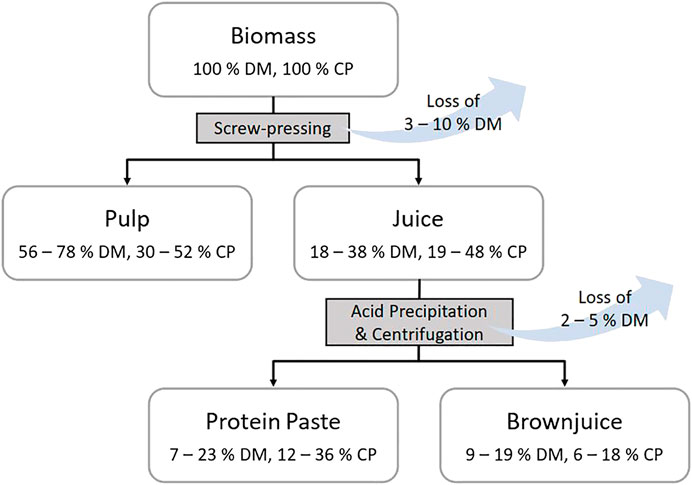
FIGURE 7. Process flowchart of the biorefinery screw-press technique, indicating dry matter (DM) and crude protein (CP) contents relative to the biomass input. Magnitudes of dry matter losses during processing are given at the two stages. Contents of dry matter (g kg−1 fresh weight) and crude protein (g kg−1 dry matter) for the various fractions and treatments on different harvest dates are provided in the supplementary material for reed canary grass (Supplementary Table S3) and tall fescue (Supplementary Table S4).
Correlation of Crude Protein Yields in Biomass and Precipitated Protein
Results of the Pearson correlation showed a significant positive association between annual cumulative CP yield in the precipitated protein concentrate and the corresponding CP yields in biomass, CNCPS fractions B1 + B2, as well as CNCPS fractions B1 + B2 + B3, across all treatments but depending on species. The highest correlation [r(98) = 0.9, p < 0.001] was observed between CP yield in the protein concentrate and CNCPS fractions B1 + B2 for TF. The correlation decreased with increasing CP yield in the corresponding counterpart (B1 + B2 > B1 + B2 + B3 > Biomass), with TF in all scenarios having a higher correlation coefficient compared to RCG (Figure 8).
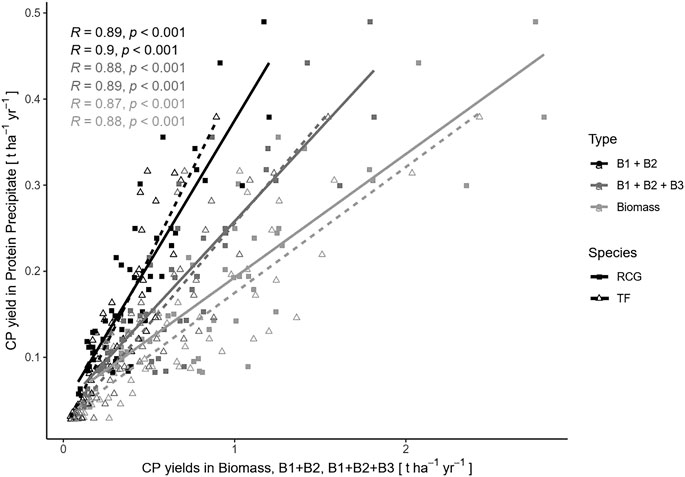
FIGURE 8. Pearson correlations between yields of crude protein (CP) in precipitated protein and crude protein in biomass (light-grey lines), neutral soluble Cornell Net Carbohydrate and Protein System (CNCPS) fractions B1 + B2 (black lines), and the acid soluble CNCPS fractions B1 + B2 + B3 (grey lines) for the species reed canary grass (RCG, solid lines) and tall fescue (TF, dashed lines), across all treatments and harvest dates.
However, we found distinct differences in correlations for the miscellaneous treatments on a product basis for CP in precipitated protein as compared to CP yields in biomass, and for CNCPS fractions B1 and B2, as well as CNCPS fractions B1 + B2 + B3. For reed canary grass (Figures 9A,C,E), the largest positive association to other CP yields was found for the treatment with four cuts per year [r(98) = 0.98, p < 0.001], whereas the highest correlation for tall fescue (Figures 9B,D,F) was found in the two cut treatment [r(98) = 0.97, p < 0.001]. Generally, tall fescue treatments showed higher positive associations in all correlation tests.
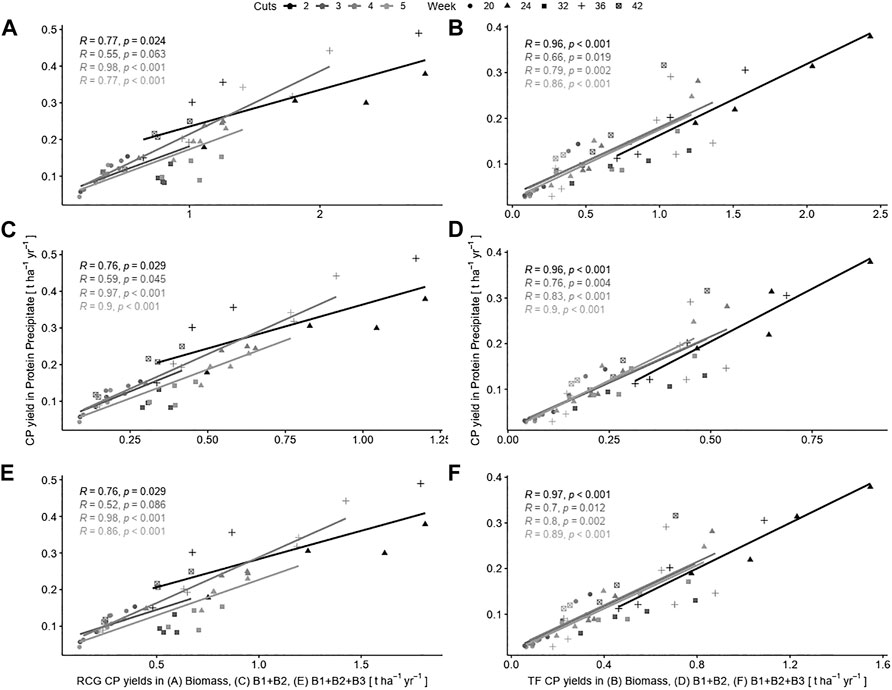
FIGURE 9. Pearson correlations between crude protein (CP) yields in precipitated protein and crude protein in biomass (A, B), neutral soluble Cornell Net Carbohydrate and Protein System (CNCPS) fractions B1 + B2 (C, D), and acid soluble CNCPS fractions B1 + B2 + B3 (E, F) for the species reed canary grass (RCG; A, C, D) and tall fescue (TF; B, D, F). Treatments are indexed by differently shaded correlation lines, and harvest dates by symbols.
Discussion
Biomass Yield and Crude Protein Content
Prevailing environmental variables and variations in nutrient availability for different sites and years seem to be the driving factors for inconsistencies of biomass yields and CP contents as a response to cut frequency and hence plant maturity (Guretzky et al., 2017; Vitra et al., 2019), making sound comparisons between different study results a vague venture. This is in particular true for yields from paludiculture crops, where we expected to see differences as compared to perennial grass cultivation on mineral soils, due to lower prevailing soil temperatures and requirements for mineral fertilizer application. However, the average annual yield for the two cut treatment of 15.6 t DM ha−1 year−1 (RCG) was within the range of previously reported yields from the same area and soil type (Kandel et al., 2013b; Karki et al., 2019). For other soil types and management intensities, we found that RCG yields were in the upper range of reported yields (7–13 t DM ha−1 year−1) for Europe and North America (Lewandowski et al., 2003; Tahir et al., 2011; Butkute et al., 2014; Tilvikiene et al., 2016). Similar observations were made regarding the yields for TF, where our results meet annual average yields of 8–14 t DM ha−1 year−1 reported in other studies (Lewandowski et al., 2003; Kandel et al., 2016; Tilvikiene et al., 2016; Manevski et al., 2017). No effect of annual harvest frequency on biomass yield was observed for more than two annual cuts. A sole effect of fertilization on DM yields of RCG as well as TF could not be assessed due to the nested nature of the nitrogen application as a covariate to the number of annual cuts. It was unexpected that intense harvesting frequency on peatsoils provided no additive annual yields, which is in line with the results from Marten and Hovin (1980), but contrary to Guretzky et al. (2017), reporting about 30% more yield when harvesting three times as compared to twice annually.
Our study showed a significant increase by 44% (193–277 g kg−1 DM) for RCG and 39% (195–270 g kg−1 DM) for TF in biomass CP content in the second as compared to the first cut, for treatments with frequent annual cuts. This is contrary to the results from Buxton and Marten (1989), reporting a decrease of CP in the same magnitude from 282 to 172 g kg−1 DM (RCG) and 220 to 131 g kg−1 DM (TF). Overall, the results indicated that increased harvest frequencies did not significantly improve average annual CP yields beyond two cuts annually, but that CP contents, and hence grass quality, for the various cuts remained high throughout the year with increased number of cuts. Perotti et al. (2021) found that biomass yields and N contents are higher under lower temperatures, maintaining sufficient soil moisture as an important factor for N cycling and biomass development (Meissner et al., 2019). Further, a decline of CP content with plant maturity (Myhr et al., 1978; Buxton and Marten, 1989; Guretzky et al., 2017; Solati et al., 2018a) caused by lignification and higher stem to leaf ratios are also positively correlated with increased temperatures. The observed increase of CP content in our study was hence potentially favored by adequate soil fertility and moisture (Supplementary Material) on the fen peatland, but also by a delayed onset of plant growth due to generally lower soil temperatures in wet peatlands. As highlighted by Huang et al. (2012), root temperature sensitivity is more susceptible to minor increases in soil temperature, affecting not only plant growth but also metabolic processes such as the distribution of N among photosynthetic proteins and thus CP content and allocation (Yin et al., 2018). Wet agricultural peatlands in northern Europe might hence be favorable locations for the cultivation of flood-tolerant perennial grasses for the purpose of green protein biorefinery.
Generally, our results highlight the importance for timing of harvest to match optimal biomass development stages for maximizing biomass and CP yields. This was indicated by differences in annual average biomass and CP yields for the one to three cut treatments of both species. While the one cut treatment was subject to a too late harvest under the experimental climatic conditions relative to the plants developmental stage, resulting in increased plant maturity and lignification, the three cut treatment was subject to a too early first cut, preventing optimal plant growth and resulting in unimproved annual yields. Contrary to these observations, the interplay between timing of harvest and climatic conditions seem to have been more favorable for the two, four, and five cut treatments. Our results highlight the need to further elucidate site-specific and climatic drivers for differences in biomass and CP yields.
Cornell Net Carbohydrate and Protein System
As aforementioned and according to Buxton and Marten (1989), environmental factors such as temperature and solar radiation are crucial factors for plant protein allocation within tissue. Considering the enhanced performance of RCG on soils with a water table depth of - 30 cm (Ustak et al., 2019), as in our study, and in years with high rain fall, one should be aware of the theoretical complexity when comparing protein fractions within plants of, though, the same species but in significantly different ecological settings.
In our study, fraction B1, the smallest of the extractable crude protein partitions, showed to account for up to 20% of total CP in early spring cuts. The ascertained decline of fraction B1 content during summer months coincided with the observations made by Solati et al. (2018a), likely caused by plant maturity and the associated increase of cell wall and lignin-bound protein fraction C (Solati et al., 2017). The increase of fraction B1 in our study with increasing number of cuts, and hence prolonged plant adolescence, supports this hypothesis.
Fraction B2 was the largest CP fraction in perennial grasses, as also stated by other studies (e.g., Elizalde et al., 1999; da Silva et al., 2013; Solati et al., 2017). While our results showed increasing B2 contents during spring, followed by a minor decline during summer months, Solati et al. (2017) stated a decline of B2 throughout the growing season. This dependency of fraction B2 in response to plant maturity and lignification, driven by an interplay of environmental factors and plant developmental stage, is also evident by the constant high contents in the two cut treatments. With averaged annually 84 g kg−1 DM for RCG and 73 g kg−1 DM for TF, timing of harvest was likely to be optimal for the avoidance of a plant maturity and temperature related decline in fraction B2. However, B2 fractions reported in our study were lower than results from other studies (Elizalde et al., 1999; Solati et al., 2018a) which are likely due to plant phenological behavior in relation to soil type and wetness.
The cellulose bound and only theoretically extractable protein fraction B3 had an average share of 25% on total biomass CP across treatments and species, which was higher than in other studies (e.g., Elizalde et al., 1999; Jardstedt et al., 2017). Generally, the pattern of B3 development throughout the year in response to harvest frequencies and dates resembled protein fraction B2 and was caused by an overall increase in total CP. A similar observation was highlighted by Loaiza et al. (2019), stating that management regarding harvest and fertilization affects the content of total biomass CP rather than the relative distribution of protein fractions. On a share basis, fraction B3 increased with enhanced plant maturity, in particular during summer months, highlighting its characteristic cell-wall bonds.
However, there is a research gap regarding type of plant protein fractions following the CNCPS for perennial grasses grown on wet agricultural peatlands. Not only differences in soil physics and biogeochemical properties, but also the plants response to wetter circumstances should be considered in discussions of results from different ecological settings as well as in future research.
Screw-Pressed Biomass Fractions
The extraction of plant biomass CP by refining techniques for the purpose of potential fodder applications is not a new notion (e.g., Davys and Pirie, 1963), though it only recently gained attention as a tangible future for new pathways in agriculture (Corona et al., 2018). Our results demonstrate that 1.2–2.2 t DM ha−1 year−1 (RCG) and 1.0–1.2 t DM ha−1 year−1 (TF) of precipitated protein concentrate were extractable using simple lab-scale biorefining techniques. Average annual CP yields within the protein precipitate ranged between 0.4–0.7 t DM ha−1 year−1 for RCG, and 0.4–0.5 t DM ha−1 year−1 for TF. This is similar to the 0.7 t ha−1, reported by Jørgensen et al. (2020) for green triticale, an annual cereal, grown on productive arable land, and to perennial ryegrass, with a reported CP content of 245 g CP kg−1 DM (Damborg et al., 2020). Considering that the flood-tolerant perennial grasses in this study were cultivated on a riparian fen peatland, a soil-type being widely considered as an unproductive marginal land area (Wichtmann and Wichmann, 2011b), our results indicated a high efficiency from an ecosystem-perspective.
For the five cut treatments, we were able to extract a protein concentrate with a content of up to between 37% CP (RCG), and 39% (TF), which is lower than the values of up to 40–47% reported for red clover, clover grass blends, alfalfa and oilseed radish concentrates (Santamaría-Fernández et al., 2017; la Cour et al., 2019). However, additional to differences in plant species and families, it should be noticed that, contrary to our study, the fresh biomass input of the reviewed studies was frozen prior to processing, additionally explaining differences in CP content. Freeze-thawing of biomass has the potential to positively contribute to the protein extractability due to tissue denaturation (la Cour et al., 2019), therewith increasing efficiencies. Nonetheless, the difference of only 10% recovered CP in the protein concentrate indicates the potential for perennial grasses grown on peatlands to compete with the more efficient legume species grown on mineral soils. This is also made evident on a product yield basis by a comparison with values published by Santamaría-Fernández et al. (2017), who yielded 6–13 kg of dried protein product per ton of fresh biomass input, having a total CP content of to 46%. In our study, RCG across all treatments yielded between 22–32 kg of dried green protein concentrate per ton of fresh weight input. Though having, with up to 39%, a lower CP content within the green protein product, high protein concentrate yields indicate the potential to offset reduced protein recovery rates as compared to the results from Santamaría-Fernández et al. (2017). However, the quality of the proteins still needs to be defined by an assessment of amino acid composition and digestibility in order to evaluate the appropriateness of paludicrop proteins for animal fodder. The majority of CP within biomass remained in the fibrous pulp fraction (Figures 10A,B), with average annual yields of up to 1.4 t DM ha−1 year−1 (RCG, two cuts) and 1.1 t DM ha−1 year−1 (TF, two cuts). On a yield basis, this represents approximately 38% of the total biomass CP all treatments. However, the increased valorisation of the fibrous pulp as ruminant forage was highlighted by Damborg et al. (2018).
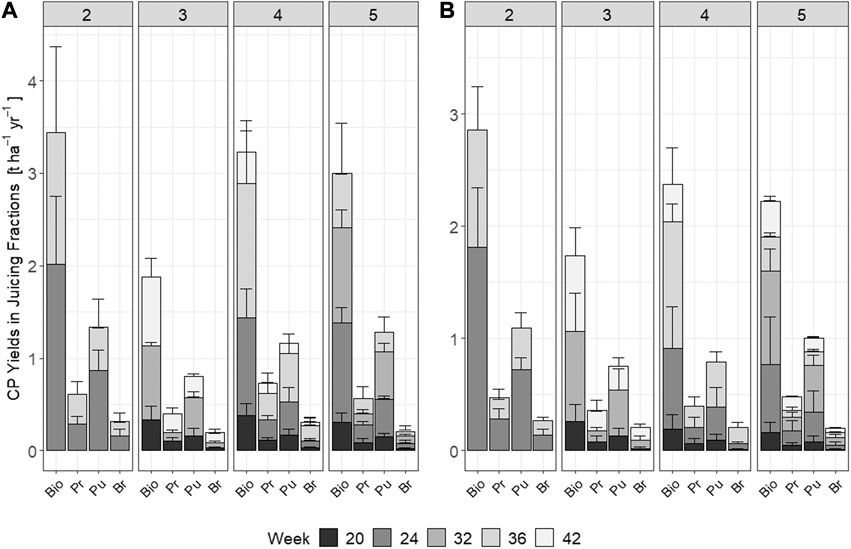
FIGURE 10. Total annual yields (t ha−1 year−1) and yields (t ha−1) for individual harvest weeks of crude protein (CP) in the biomass and in the screw-pressed fractions for the various treatments and species (A) reed canary grass (left-hand panel), and (B) tall fescue (right-hand panel). Abbreviations: Bio = Biomass, Pr = Protein Paste, Pu = Pulp, Br = Brownjuice. Error-bars show standard deviations.
Future research should hence not only put emphasis on more screw-press fractionation trials regarding flood-tolerant perennial grasses, but in particular of those grown on wet agricultural peatlands in order to further determine their potential for the generation of value-added protein products resulting from biorefining. Further, differences in precipitation efficiencies should be evaluated, as also claimed by Jørgensen et al. (2020). For instance have lactic-acid fermentation (Santamaría-Fernández et al., 2017), microfiltration (Santamaría-Fernández et al., 2020) and lignosulfonates (la Cour et al., 2019), shown to be promising improvements for protein recovery as compared to precipitation by acid precipitation or heat treatment.
Comparison of extractable and potentially extractable proteins indicated by the CNCPS vs. protein precipitated following screw-pressing
Maximising product yield has been highlighted as the most critical optimisation parameter for biorefineries from an enviro-economic perspective (Corona et al., 2018; Santamaría-Fernández et al., 2020). Our results showed maximum yields for fractions B1 and B2 of 1.5 t DM ha−1 year−1 (RCG, two cuts) and 1.1 t DM ha−1 year−1 (TF, two cuts), representing 45%, respectively 39% of the total biomass CP. However, for these treatments, only 18 and 16% of biomass CP, respectively 40 and 41% of the summed fractions B1 and B2, were extracted during the biorefinery process. When also accounting for the potentially extractable protein fraction B3, the potential for biomass CP recovery by biorefinery increases to 2.3 t DM ha−1 year−1 and 1.8 t DM ha−1 year−1, representing 67 and 64% of total biomass CP. These values for extractable and potentially extractable protein fractions are similar and only marginally higher compared to those reported by Solati et al. (2018b), though cultivated on different soil types and under different environmental conditions.
The strong correlation of methods showed, that using CNCPS fractions B1 and B2 as an indicator provides a promising opportunity for prediction of protein extractability, and hence obtainable yields, with current biorefinery techniques. However, there are obstacles regarding the improvement of precipitation efficiencies that need to be overcome in order to make the green biorefinery concept a not only sustainable, but also economically viable enterprise. Adding fraction B3 to the extraction potential, increasing the potential protein yield by on average 24%, might be a hindering for the near future, when even the easily-soluble protein fractions can not entirely be extracted. However, this should be interpreted as an incentive for more prompt improvements. Additional improvements for the protein precipitation potential, and hence increased protein yields, might be found in cutting of the biomass prior to screw-pressing or multiple pressing of the protein-rich pulp fraction, as indicated by the low DM content of the latter.
Conclusion
Paludiculture is a promising option for promoting rewetting of drained agricultural peatlands to mitigate adverse environmental impacts by the provision of critical ecosystem services, while at the same time providing an economic output. However, new opportunities for the generation of value-added products derived from paludicrops have yet to be defined. We found the highest annual biomass and CP yields in the two cut treatments, with 13.4 and 15.6 t DM ha−1 year−1, yielding 2.9 and 3.4 t CP ha−1 year−1 for TF and RCG, respectively. Overall, the results indicated that increased harvest frequencies did not significantly improve average annual biomass and CP yields beyond two cuts annually, but that CP contents, and hence grass quality, for the various cuts remained high throughout the year. Our results showed maximum neutral-extractable (fractions B1 and B2) true protein yields of 1.1 and 1.5 t ha−1 year−1 in the two cut treatments, representing 39% (TF) and 45% (RCG) of total CP. When also accounting for the acid extractable protein fraction B3, the potential for biomass CP recovery by biorefinery increased to 1.8 t (TF) and 2.3 t (RCG) ha−1 year−1. The allocation of plant protein between CNCPS fractions was significantly affected by harvest date, underlining the importance of plant maturity, and frequency of annual cuts. This also applied for cumulative annual CP yields of precipitated protein concentrate, with a content of up to 39% CP, where 1.0 and 2.2 t DM ha−1 year−1 were precipitated for TF and RCG, respectively. We found a significant positive correlation between CP yield in the protein concentrate and CNCPS fractions B1 + B2, indicating that the CNCPS analysis is suitable for the prediction of protein extractability with current biorefinery techniques. Harvest dates and frequencies should be adjusted to match optimal site-specific climatic conditions and biomass development stages for protein extraction. In conclusion, the results from this study indicated not only high biomass and CP yields, as well as reasonable protein concentrate outputs from biorefining, but also the suitability of paludiculture crops, such as reed canary grass and tall fescue, as promising feedstock candidates for value-added product chains.
Data Availability Statement
The raw data supporting the conclusions of this article will be made available by the authors, without undue reservation.
Author Contributions
CN developed and performed the study design and experimental work, the analysis of the data, and the writing of the manuscript. All authors contributed to the study design, the writing and reading of the manuscript, and approved the final manuscript.
Funding
This study was financially supported by the PEATWISE project (https://www.eragas.eu/en/eragas/Research-projects/PEATWISE.htm) in the frame of the ERA-NET FACCE ERA-GAS. FACCE ERA-GAS received funding from the European Union’s Horizon 2020 research and innovation programme under the grant agreement No. 696356. It further was financially supported by the GrassBot2 project, receiving funding from the European Regional Development Fund (ERDF) and Region Midtjylland, Danmark. In addition, the study was partly supported by the Aarhus University Center for Circular Bioeconomy (CBIO, https://cbio.au.dk/en/).
Conflict of Interest
The authors declare that the research was conducted in the absence of any commercial or financial relationships that could be construed as a potential conflict of interest.
Acknowledgments
The authors would like to thank technical and laboratory staff at the Departments of Agroecology and Animal Science, and in particular, the agricultural technologists at Foulumgård, Aarhus University, for excellent guidance as well as assistance with crop management and analyses.
Supplementary Material
The Supplementary Material for this article can be found online at: https://www.frontiersin.org/articles/10.3389/fenvs.2021.619258/full#supplementary-material.
References
Ambye-Jensen, M., Johansen, K. S., Didion, T., Kádár, Z., and Meyer, A. S. (2014). Ensiling and hydrothermal pretreatment of grass: consequences for enzymatic biomass conversion and total monosaccharide yields. Biotechnol. Biofuels 7 (1), 95. doi:10.1186/1754-6834-7-95
AOAC (Editor) (1990). Official methods of analysis. 15th Edn. Arlington, VA: Association of Official Analytical Chemists).
Bates, D., Mächler, M., Bolker, B., and Walker, S. (2015). Fitting linear mixed-effects models using lme4. J. Stat. Softw. 67 (1), 1–48. doi:10.18637/jss.v067.i01
Butkute, B., Lemežiene, N., Kanapeckas, J., Navickas, K., Dabkevičius, Z., and Venslauskas, K. (2014). Cocksfoot, tall fescue and reed canary grass: dry matter yield, chemical composition and biomass convertibility to methane. Biomass Bioenergy 66, 1–11. doi:10.1016/j.biombioe.2014.03.014
Butkutė, B., Lemežienė, N., Cesevičienė, J., Liatukas, Ž., and Dabkevičienė, G. (2013). Carbohydrate and lignin partitioning in switchgrass biomass Panicum virgatum L. as a bioenergy feedstock. Zemdirbyste-Agriculture 100 (3), 251–260. doi:10.13080/z-a.2013.100.032
Buxton, D. R., and Marten, G. C. (1989). Crop quality and utilization: forage quality of plant parts of perennial grasses and relationship to phenology. Crop Sci. 29 (2), 429–435. doi:10.2135/cropsci1989.0011183X0029000200039x
Coblentz, W. K., Akins, M. S., Cavadini, J. S., and Jokela, W. E. (2017). Net effects of nitrogen fertilization on the nutritive value and digestibility of oat forages. J. Dairy Sci. 100 (3), 1739–1750. doi:10.3168/jds.2016-12027
Corona, A., Ambye-Jensen, M., Vega, G. C., Hauschild, M. Z., and Birkved, M. (2018). Techno-environmental assessment of the green biorefinery concept: combining process simulation and life cycle assessment at an early design stage. Sci. Total Environ. 635, 100–111. doi:10.1016/j.scitotenv.2018.03.357
da Silva, M. S., Tremblay, G. F., Bélanger, G., Lajeunesse, J., Papadopoulos, Y. A., Fillmore, S. A. E., et al. (2013). Energy to protein ratio of grass-legume binary mixtures under frequent clipping. Agron. J. 105 (2), 482–492. doi:10.2134/agronj2012.0281
Damborg, V. K., Jensen, S. K., Weisbjerg, M. R., Adamsen, A. P., and Stødkilde, L. (2020). Screw-pressed fractions from green forages as animal feed: chemical composition and mass balances. Anim. Feed Sci. Technol. 261, 114401. doi:10.1016/j.anifeedsci.2020.114401
Damborg, V. K., Stødkilde, L., Jensen, S. K., and Weisbjerg, M. R. (2018). Protein value and degradation characteristics of pulp fibre fractions from screw pressed grass, clover, and lucerne. Anim. Feed Sci. Technol. 244, 93–103. doi:10.1016/j.anifeedsci.2018.08.004
Davys, M., and Pirie, N. (1963). Batch production of protein from leaves. J. Agric. Eng. Res. 8 (1), 70–73.
Dindová, A., Hakl, J., Hrevušová, Z., and Nerušil, P. (2019). Relationships between long-term fertilization management and forage nutritive value in grasslands. Agric. Ecosyst. Environ. 279, 139–148. doi:10.1016/j.agee.2019.01.011
Elizalde, J. C., Merchen, N. R., and Faulkner, D. B. (1999). Fractionation of fiber and crude protein in fresh forages during the spring growth. J. Anim. Sci. 77 (2), 476–484. doi:10.2527/1999.772476x
Geurts, J. J., van Duinen, G.-J. A., van Belle, J., Wichmann, S., Wichtmann, W., and Fritz, C. (2019). Recognize the high potential of paludiculture on rewetted peat soils to mitigate climate change. J. Sustain. Org. Agric. Syst. 69 (1), 5–8. doi:10.3220/LBF1576769203000
Giannini, V., Silvestri, N., Dragoni, F., Pistocchi, C., Sabbatini, T., and Bonari, E. (2017). Growth and nutrient uptake of perennial crops in a paludicultural approach in a drained Mediterranean peatland. Eco. Eng. 103, 478–487.
Günther, A. B., Huth, V., Jurasinski, G., and Glatzel, S. (2014). Scale-dependent temporal variation in determining the methane balance of a temperate fen. Greenh. Gas Meas. Manag. 4 (1), 41–48. doi:10.1080/20430779.2013.850395
Guretzky, J. A., Dunn, C. D., and Bishop, A. (2017). Plant community structure and forage nutritive value of reed canarygrass-invaded wetlands. Agron. J. 110 (1), 200–209. doi:10.2134/agronj2017.05.0277
Huang, B., Rachmilevitch, S., and Xu, J. (2012). Root carbon and protein metabolism associated with heat tolerance. J. Exp. Bot. 63 (9), 3455–3465. doi:10.1093/jxb/ers003
Ibarrola-Rivas, M. J., Granados-Ramírez, R., and Nonhebel, S. (2017). Is the available cropland and water enough for food demand? a global perspective of the land-water-food nexus. Adv. Water Resour. 110, 476–483. doi:10.1016/j.advwatres.2017.09.018
Jardstedt, M., Hessle, A., Nørgaard, P., Richardt, W., and Nadeau, E. (2017). Feed intake and urinary excretion of nitrogen and purine derivatives in pregnant suckler cows fed alternative roughage-based diets. Livest. Sci. 202, 82–88. doi:10.1016/j.livsci.2017.05.026
Joosten, H., and Clarke, D. (2002). Wise use of mires and peatlands – background and Principles, including a Framework for Decision‐making. Jyväskylä, Finland: International Mire Conservation Group and International Peat Society.
Joosten, H., Gaudig, G., Tanneberger, F., Wichmann, S., and Wichtmann, W. (2016). “Paludiculture: sustainable productive use of wet and rewetted peatlands,” in Peatland restoration and ecosystem services: science, policy and practice. Editor A. Bonn, T. Allott, M. Evans, H. Joosten, and R. Stoneman (Bangkok, Thailand: C. U. Press), 339–357.
Joosten, H. (2012). Status and prospects of global peatlands. Nat. Landsch. 87 (2), 50–55. doi:10.17433/2.2012.50153141.50-55
Jørgensen, H., Thomsen, S. T., and Schjoerring, J. K. (2020). The potential for biorefining of triticale to protein and sugar depends on nitrogen supply and harvest time. Ind. Crops Prod. 149, 112333. doi:10.1016/j.indcrop.2020.112333
Kandel, T. P., Elsgaard, L., Andersen, M. N., and Lærke, P. E. (2016). Influence of harvest time and frequency on light interception and biomass yield of festulolium and tall fescue cultivated on a peatland. Eur. J. Agron. 81, 150–160. doi:10.1016/j.eja.2016.09.010
Kandel, T. P., Elsgaard, L., Karki, S., and Lærke, P. E. (2013a). Biomass yield and greenhouse gas emissions from a drained fen peatland cultivated with reed canary grass under different harvest and fertilizer regimes. BioEnergy Res. 6 (3), 883–895. doi:10.1007/s12155-013-9316-5
Kandel, T. P., Sutaryo, S., Møller, H. B., Jørgensen, U., and Lærke, P. E. (2013b). Chemical composition and methane yield of reed canary grass as influenced by harvesting time and harvest frequency. Bioresour. Technol. 130, 659–666. doi:10.1016/j.biortech.2012.11.138
Kappelle, M. (2020). WMO statement on the state of the global climate in 2019. Geneva, Switzerland: World Meteorological Association. doi:10.13140/RG.2.2.13705.19046
Karki, S., Kandel, T. P., Elsgaard, L., Labouriau, R., and Lærke, P. E. (2019). Annual CO2 fluxes from a cultivated fen with perennial grasses during two initial years of rewetting. Mires Peat 25 (01), 01–22. doi:10.19189/MaP.2017.DW.322
Kromus, S., Kamm, B., Kamm, M., Fowler, P., and Narodoslawsky, M. (2006). The green biorefinery concept – fundamentals and potential. Green Biorefineries 1, 253–294. doi:10.1002/9783527619849.ch12
la Cour, R., Schjoerring, J. K., and Jørgensen, H. (2019). Enhancing protein recovery in green biorefineries by lignosulfonate-assisted precipitation. Front. Sustain. Food Syst. 3, 1–9. doi:10.3389/fsufs.2019.00112
Lewandowski, I., Scurlock, J. M. O., Lindvall, E., and Christou, M. (2003). The development and current status of perennial rhizomatous grasses as energy crops in the US and Europe. Biomass Bioenergy 25 (4), 335–361. doi:10.1016/s0961-9534(03)00030-8
Licitra, G., Hernandez, T. M., and Van Soest, P. J. (1996). Standardization of procedures for nitrogen fractionation of ruminant feeds. Anim. Feed Sci. Technol. 57 (4), 347–358. doi:10.1016/0377-8401(95)00837-3
Loaiza, P., Balocchi, O., de la Barra, C., and López, I. F. (2019). Perennial ryegrass productivity and nutritive quality as affected by frequency of nitrogen fertilizer addition. Grassland Sci. 65 (2), 86–92. doi:10.1111/grs.12227
Manevski, K., Lærke, P. E., Jiao, X., Santhome, S., and Jørgensen, U. (2017). Biomass productivity and radiation utilisation of innovative cropping systems for biorefinery. Agric. For. Meteorol. 233, 250–264. doi:10.1016/j.agrformet.2016.11.245
Marten, G. C., and Hovin, A. W. (1980). Harvest schedule, persistence, yield, and quality interactions among four perennial grasses1. Agron. J. 72 (2), 378. doi:10.2134/agronj1980.00021962007200020030x
Meisser, M., Vitra, A., Deléglise, C., Dubois, S., Probo, M., Mosimann, E., et al. (2019). Nutrient limitations induced by drought affect forage N and P differently in two permanent grasslands. Agric. Ecosyst. Environ. 280, 85–94. doi:10.1016/j.agee.2019.04.027
Myhr, K., Solderg, Y., and Selmer-Olsen, A. R. (1978). The content of minerals, fibre, protein and amino acids in reed canary grass, timothy and meadow fescue. Acta Agric. Scand. 28 (3), 269–278. doi:10.1080/00015127809435179
Peoples, M. B., and Dalling, M. J. (1988). “The interplay between proteolysis and amino acid metabolism during senescence and nitrogen reallocation,” in Senescence and Aging in Plants. Editors L. D. Nooden, and A. C. Leopold. San Diego, United States: Academic Press Inc.
Perotti, E., Huguenin-Elie, O., Meisser, M., Dubois, S., Probo, M., and Mariotte, P. (2021). Climatic, soil, and vegetation drivers of forage yield and quality differ across the first three growth cycles of intensively managed permanent grasslands. Eur. J. Agron. 122, 126194. doi:10.1016/j.eja.2020.126194
Peyraud, J. L., and Astigarraga, L. (1998). Review of the effect of nitrogen fertilization on the chemical composition, intake, digestion and nutritive value of fresh herbage: consequences on animal nutrition and N balance. Anim. Feed Sci. Technol. 72 (3), 235–259. doi:10.1016/S0377-8401(97)00191-0
Pihlajaniemi, V., Ellilä, S., Poikkimäki, S., Nappa, M., Rinne, M., Lantto, R., et al. (2020). Comparison of pretreatments and cost-optimization of enzymatic hydrolysis for production of single cell protein from grass silage fibre. Bioresour. Technol. Rep. 9, 100357. doi:10.1016/j.biteb.2019.10035
R Core Team (2020). R: a language and environment for statistical computing. Vienna, Austria: R Foundation for Statistical ComputingAvailable at: https://www.R-project.org/.
Roy-Macauley, H., Zuily-Fodil, Y., Kidric, M., Thi, A. T. P., and Silva, J. V. (1992). Effect of drought stress on proteolytic activities in Phaseolus and Vigna leaves from sensitive and resistant plants. Physiol. Plant. 85 (1), 90–96. doi:10.1111/j.1399-3054.1992.tb05268.x
Sanderson, M. A., and Wedin, W. F. (1989). Nitrogen in the detergent fibre fractions of temperate legumes and grasses. Grass Forage Sci. 44 (2), 159–168. doi:10.1111/j.1365-2494.1989.tb01923.x
Santamaria-Fernandez, M., Ambye-Jensen, M., Damborg, V. K., and Lübeck, M. (2019). Demonstration-scale protein recovery by lactic acid fermentation from grass clover – a single case of the production of protein concentrate and press cake silage for animal feeding trials. Biofuels Bioprod. Biorefin. 13 (3), 502–513. doi:10.1002/bbb.1957
Santamaría-Fernández, M., Molinuevo-Salces, B., Kiel, P., Steenfeldt, S., Uellendahl, H., and Lübeck, M. (2017). Lactic acid fermentation for refining proteins from green crops and obtaining a high quality feed product for monogastric animals. J. Clean. Prod. 162, 875–881. doi:10.1016/j.jclepro.2017.06.115
Santamaría-Fernández, M., Schneider, R., Lübeck, M., and Venus, J. (2020). Combining the production of L-lactic acid with the production of feed protein concentrates from alfalfa. J. Biotechnol. 323, 180–188. doi:10.1016/j.jbiotec.2020.08.010
Solati, Z., Jørgensen, U., Eriksen, J., and Søegaard, K. (2017). Dry matter yield, chemical composition and estimated extractable protein of legume and grass species during the spring growth. J. Sci. Food Agric. 97, 3958–3966. doi:10.1002/jsfa.8258
Solati, Z., Jørgensen, U., Eriksen, J., and Søegaard, K. (2018a). Estimation of extractable protein in botanical fractions of legume and grass species. Grass Forage Sci. 73 (2), 572–581. doi:10.1111/gfs.12325
Solati, Z., Manevski, K., Jørgensen, U., Labouriau, R., Shahbazi, S., and Lærke, P. E. (2018b). Crude protein yield and theoretical extractable true protein of potential biorefinery feedstocks. Ind. Crops Prod. 115, 214–226. doi:10.1016/j.indcrop.2018.02.010
Stødkilde, L., Damborg, V. K., Jørgensen, H., Laerke, H. N., and Jensen, S. K. (2018). White clover fractions as protein source for monogastrics: dry matter digestibility and protein digestibility-corrected amino acid scores. J. Sci. Food Agric. 98 (7), 2557–2563. doi:10.1002/jsfa.8744
Stødkilde, L., Damborg, V. K., Jørgensen, H., Lærke, H. N., and Jensen, S. K. (2019). Digestibility of fractionated green biomass as protein source for monogastric animals. Animal 13 (9), 1817–1825. doi:10.1017/s1751731119000156
Tahir, M. H. N., Casler, M. D., Moore, K. J., and Brummer, E. C. (2011). Biomass yield and quality of reed canarygrass under five harvest management systems for bioenergy production. BioEnergy Res. 4 (2), 111–119. doi:10.1007/s12155-010-9105-3
Tanneberger, F., and Wichtmann, W. (2011). Carbon credits from peatland rewetting: climate – biodiversity – land use. Stuttgart, Germany: Schweizerbart’sche Verlagsbuchhandlung.
Tilvikiene, V., Kadziuliene, Z., Dabkevicius, Z., Venslauskas, K., and Navickas, K. (2016). Feasibility of tall fescue, cocksfoot and reed canary grass for anaerobic digestion: analysis of productivity and energy potential. Ind. Crops Prod. 84, 87–96. doi:10.1016/j.indcrop.2016.01.033
United Nations, Department of Economic and Social Affairs, Population Division (2019). World Population Prospects 2019: Highlights (ST/ESA/SER.A/423).
Ustak, S., Šinko, J., and Muňoz, J. (2019). Reed canary grass (Phalaris arundinacea L.) as a promising energy crop. J. Cent. Eur. Agric., 20 (4), 1143–1168. doi:10.5513/JCEA01/20.4.2267
Van Soest, P. J., Robertson, J. B., and Lewis, B. A. (1991). Methods for dietary fiber, neutral detergent fiber, and nonstarch polysaccharides in relation to animal nutrition. J. Dairy Sci. 74 (10), 3583–3597. doi:10.3168/jds.s0022-0302(91)78551-2
Vitra, A., Deléglise, C., Meisser, M., Risch, A. C., Signarbieux, C., Lamacque, L., et al. (2019). Responses of plant leaf economic and hydraulic traits mediate the effects of early- and late-season drought on grassland productivity. AoB Plants 11 (3), plz023. doi:10.1093/aobpla/plz023
Wichmann, S. (2017). Commercial viability of paludiculture: a comparison of harvesting reeds for biogas production, direct combustion, and thatching. Ecol. Eng. 103, 497–505. doi:10.1016/j.ecoleng.2016.03.018
Wichtmann, W., and Wichmann, S. (2011a). Paludikultur: standortgerechte bewirtschaftung wiedervernässter moore. TELMA - Berichte der Deutschen Gesellschaft für Moor- und Torfkunde, Beiheft 4, 215–234. doi:10.23689/fidgeo-2979
Wichtmann, W., and Wichmann, S. (2011b). Environmental, social and economic aspects of a sustainable biomass production. J. Sustain. Energy Environ. Spec. Issue, 77–81.
Yin, X., Schapendonk, A. H. C. M., and Struik, P. C. (2018). Exploring the optimum nitrogen partitioning to predict the acclimation of C3 leaf photosynthesis to varying growth conditions. J. Exp. Bot. 70 (9), 2435–2447. doi:10.1093/jxb/ery277
Keywords: Biorefinery, crude protein, paludiculture, plant fractionation, reed canary grass, tall fescue
Citation: Nielsen CK, Stødkilde L, Jørgensen U and Lærke PE (2021) Effects of Harvest and Fertilization Frequency on Protein Yield and Extractability From Flood-Tolerant Perennial Grasses Cultivated on a fen Peatland. Front. Environ. Sci. 9:619258. doi: 10.3389/fenvs.2021.619258
Received: 21 October 2020; Accepted: 04 February 2021;
Published: 21 April 2021.
Edited by:
Line Rochefort, Laval University, CanadaReviewed by:
Mengyang You, Northeast Institute of Geography and Agroecology (CAS), ChinaYansheng Li, Chinese Academy of Science, China
Copyright © 2021 Nielsen, Stødkilde, Jørgensen and Lærke. This is an open-access article distributed under the terms of the Creative Commons Attribution License (CC BY). The use, distribution or reproduction in other forums is permitted, provided the original author(s) and the copyright owner(s) are credited and that the original publication in this journal is cited, in accordance with accepted academic practice. No use, distribution or reproduction is permitted which does not comply with these terms.
*Correspondence: Claudia Kalla Nielsen, Y2xhdWRpYUBhZ3JvLmF1LmRr