- 1Institute of Epidemiology, Helmholtz Zentrum München—German Research Center for Environmental Health (GmbH), Neuherberg, Germany
- 2IBE-Chair of Epidemiology, Ludwig-Maximilians-Universität München, Munich, Germany
- 3Helmholtz Centre for Environmental Research–UFZ, Leipzig, Germany
- 4Department of Experimental Aerosol and Cloud Microphysics, Leibniz Institute for Tropospheric Research, Leipzig, Germany
- 5School of Public Health, Peking University Health Science Center, Beijing, China
To improve ambient air quality during the 2008 Summer Olympic and Paralympic Games, the Chinese Government and Beijing’s municipal government implemented comprehensive emission control policies in Beijing and its neighboring regions before and during this period. The goal of this study was to investigate the association between particulate air pollution and cause-specific respiratory mortality before, during and after the period of the Olympic Games. Further, we wanted to assess whether changes in pollutant concentrations were linked to changes in respiratory mortality. We obtained daily data on mortality due to respiratory diseases (coded as J00-J99 according to the International Classification of Diseases and Related Health Problems 10th revision [ICD10]) and pneumonia (ICD10: J12–18), meteorology, particulate matter less than 10 µm or 2.5 μm in diameter (PM10, PM2.5) and particle number size distribution from official monitoring networks and sites located on the Peking University campus between May 20 and December 1, 2008. We assessed the effects of particulate air pollution on daily respiratory mortality using confounder-adjusted Quasi-Poisson regression models. Furthermore, we estimated air pollution effects for three periods—before (May 20 to July 20, 2008), during (August 1 to September 20, 2008) and after (October 1 to December 1, 2008)—by including interaction terms in the models. We found associations between different particle metrics and respiratory and pneumonia mortality, with more pronounced effects in smaller particle size ranges. For example, an interquartile range increase of 7,958 particles/cm3 in ultrafine particles (particles <100 nm in diameter) led to a 16.3% (95% confidence interval 4.3%; 26.5%) increase in respiratory mortality with a delay of seven days. When investigating the sub-periods, results indicate that a reduction in air pollution during the Olympics resulted in reduced (cause-specific) respiratory mortality. This reduction was especially pronounced for pneumonia mortality. The findings suggest that even a short-term reduction in pollution concentrations may lead to health benefits and that smaller particles in the ultrafine size range may be particularly important for respiratory health.
Introduction
Numerous epidemiological studies have shown a link between exposure to elevated mass concentrations of particulate matter (PM) and increased cardiorespiratory morbidity and mortality (Thurston et al., 2017). Findings have been the most robust for fine particles (PM2.5) (Frampton and Rich, 2016). Besides PM, particle number concentrations (PNC) in different size fractions may provide a more suitable exposure metric regarding the toxicity of PM exposure (Hennig et al., 2018; Chen et al., 2020). Particularly, ultrafine particles (UFP, particles <100 nm in diameter) may contribute to the observed health effects due to the small size, high total particle number concentration, large active surface area, and a high pulmonary deposition efficiency (HEI, 2013; Ohlwein et al., 2019; Schraufnagel, 2020). However, the epidemiological evidence for health effects of UFP is limited and inconsistent (Stafoggia et al., 2017; Ohlwein et al., 2019). Especially in China, the availability of appropriate exposure data is limited despite high particle concentrations.
To significantly improve the air quality during the 2008 Summer Olympic and Paralympic Games, the Chinese Government and Beijing’s municipal government implemented comprehensive pollution control measures in Beijing and the neighboring regions before and during the Olympics. Main measures aimed at controlling vehicular emissions; for example, stringent vehicle emission standards were established, and high-emitting vehicles were banned from entering the city. Further measures included the shutdown of manufactories with high air pollutant emissions during the Olympics period or the relocation of polluting industries. Also, more than 16,000 small coal-fired boilers were converted to cleaner natural gas (Fang et al., 2009; Schleicher et al., 2012; Su et al., 2015). These regulations resulted in significant decreases in levels of PM and several gaseous co-pollutants in Beijing during August and September 2008 (Wang et al., 2009; Sun et al., 2011; Schleicher et al., 2012).
These pollution control efforts provided a unique opportunity to evaluate whether a short-run improvement in air quality would also result in acute changes in human health outcomes (Peel et al., 2010; Su et al., 2015). Using this quasi-experimental design, a number of studies have been conducted that explored the association between air pollution and health in Beijing (Wu et al., 2010; Lin et al., 2011; Huang et al., 2012; Rich et al., 2012; Zhang et al., 2013; Gong et al., 2014; Mu et al., 2014; Su et al., 2015). For example, Rich et al. (2012) reported an association between the change in air quality during the Beijing Olympics and short-term changes in inflammatory and thrombotic biomarkers in healthy young participants. Biomarkers of pulmonary and systemic inflammation showed similar results (Lin et al., 2011; Huang et al., 2012; Zhang et al., 2013; Gong et al., 2014). However, these studies were mostly limited to PM2.5 and cardiorespiratory biomarkers; none of them was related to respiratory mortality.
We aimed at investigating the association between particulate air pollution and cause-specific respiratory mortality in Beijing before, during, and after the period of the Olympic Games from May 20, to December 1, 2008. Further, we wanted to assess whether changes in pollutant concentrations were linked to changes in respiratory mortality.
Methods
We conducted this study in the urban area of Beijing, China. In 2008, this area had a land area of about 1,368 km2, consisting of eight districts with approximately 12.3 million registered inhabitants (http://www.bjstats.gov.cn/tjnj/2009-tjnj/).
In our main analysis, we investigated the data in three specific periods: pre-Olympic period (May 20, to July 20–62 days), during-Olympic period (August 1, to September 20–51 days), and post-Olympic period (October 1, to December 1–62 days) (Su et al., 2015). We further specified a 10-days transition interval between each sub-period. Also, we examined data for the period May 20, to December 1, 2008 (“whole period”).
Data Collection
Mortality Data
We obtained mortality data from the Beijing Center for Disease Control (CDC). Regarding death outcomes, we particularly investigated mortality data of respiratory diseases (International Classification of Diseases and Related Health Problems 10th revision [ICD10]: J00-J99) and pneumonia (ICD10: J12–18). For overall respiratory mortality, we also investigated different sex (male vs. female) and age groups (age<75 years vs. age≥75 years).
Air Pollution and Meteorological Data
Hourly PM2.5 concentrations were obtained at an urban background monitoring site located in the Haidian district, about 5 km north from Peking University (PKU) (Supplementary Figure S1). Monitoring was done using a TEOM(R) RP1400A air sampler (Su et al., 2015). We calculated daily averages of PM2.5 only if at least 75% of the hourly data was available. There were no missing values for the daily PM2.5 concentrations.
Particle size distribution data was measured on top of a 6-?oor building on the PKU campus, also located in the Haidian District (Supplementary Figure S1). The campus is located in a primarily residential and commercial area with no industrial or agricultural sources. Main local emission sources within a radius of 1000 m included road traffic emissions, fuel combustion for domestic cooking and heating, and construction (Wehner et al., 2008). An earlier examination on the spatial variability showed similar average particle number size distributions during summer at the PKU site and another regional monitoring site, located around 50 km to the south (Yue et al., 2009). Therefore, we consider the PKU site to be an urban background station (Breitner et al., 2011). The setup of the measurement devices has been described in detail elsewhere (Wehner et al., 2004; Wehner et al., 2008). Briefly, a Twin Differential Mobility Particle Sizer was used to sample the size range from 3 – 800 nm (mobility diameter). Data were corrected for losses due to diffusion and sedimentation within the inlet line, as described by Wehner et al. (2004). The instruments were calibrated regularly, and the data were quality checked by the Leibniz Institute for Tropospheric Research.
We calculated 24 h average particle number concentrations (PNC) for the following size fractions (nm): 10–30, 30–50, 50–100, 100–300, 300–1,000, and also for UFP (10–100 nm). We selected these size ranges based on particle behavior, origin, and deposition in the respiratory tract (Gu et al., 2012; Su et al., 2015). About 4% of the data were missing.
We obtained data on PM with a diameter of less than 10 μm (PM10) and the gaseous air pollutant nitrogen dioxide (NO2) from the Beijing Municipal Environmental Monitoring Center’s network. This network included eight measurement sites spread over the urban area of Beijing (Supplementary Figure S1). Sites are “mandated not to be in the direct vicinity of traffic or of industrial sources and not to be influenced by local pollution sources, thus also avoiding buildings or housing and large emitters such as coal-, waste-, or oil-burning boilers; furnaces; and incinerators” (Chen et al., 2012). We obtained daily average concentrations from the eight monitoring locations. If there were missing air pollutant concentrations from one station on a given day, we used data of the remaining sites to calculate the average concentration (Su et al., 2015).
Data on 24 h mean air temperature and relative humidity were available from a station of the China Meteorological Data Sharing Service, located in the southeastern part of Beijing within the Daxing District (Su et al., 2015).
We further obtained data on air mass history by use of the Hybrid Single-Particle Lagrangian Integrated Trajectory model (version 4.8, http://ready.arl.noaa.gov/HYSPLIT.php). We generated 72 h back-trajectories to determine the origin of air masses (Breitner et al., 2011; Su et al., 2015). We classified the back-trajectories by cluster analysis resulting in two clusters: the first cluster consisted of air masses arriving from the neighboring (mainly southern) regions of Beijing with low wind speed to stagnant conditions, whereas the second cluster represented air masses mainly coming from north-western directions with average to high wind speed.
Statistical Analysis
We calculated descriptive statistics for each particle metric (size-segregated PNC, PM2.5, PM10) and NO2, air temperature, relative humidity, and the different mortality outcomes for the whole reporting period, and for the three sub-periods. We also used Poisson regression models to estimate the between-period changes in mortality (Zhang et al., 2013; Su et al., 2015).
In the next step, we evaluated the relationship between air pollution and daily (cause-specific) respiratory death counts using confounder-adjusted Quasi-Poisson regression models. As potential confounders, we included same-day air temperature (penalized spline with four degrees of freedom (df)), a three-day average of relative humidity (spline with three df), and dummy variables for the three sub-periods, weekday, public holidays, and the heating period (heating period: October 20-December 1; non-heating period: May 20-October 19). Similarly to Su et al. (2015), the df of the weather parameters were selected according to the Generalized Cross-Validation (GCV) criterion. For the “whole period” analysis (May 20 to December 1, 2008), we investigated associations between respiratory mortality and air pollutants with different lags, from lag 0 (exposure on the event day) to lag 10 (exposure eight days before the event), according to previous publications (Stafoggia et al., 2017). Moreover, air pollution effects were estimated for the three sub-periods by the use of two dummy variables and corresponding interaction terms in the models.
We also investigated the effects of different particle metrics on respiratory mortality in sex and age groups. We further assessed the modification of particle effects by air mass origin using an interaction term to estimate the particle effects of the corresponding cluster. Two-pollutant models were used to examine the independent effects of PM, UFP, and NO2.
We conducted several sensitivity analyses on the confounder model, for example, varying the df for meteorological variables to test the robustness of the exposure-response function.
Results
Descriptive Statistics
Table 1 and Table 2 summarize the descriptive statistics of the different particle metrics, NO2, meteorology, and (cause-specific) respiratory mortality for the whole study period and the three sub-periods. Air pollutants showed lowest concentrations during the Olympics period. Pollutant levels decreased when comparing the pre-to during-Olympics period, and increased again after the Olympic and Paralympic Games. The number of death counts due to respiratory diseases and pneumonia were similar or slightly lower in the pre-Olympics period, while mortality was higher afterward in comparison with the period during the Games (Supplementary Figure S2).
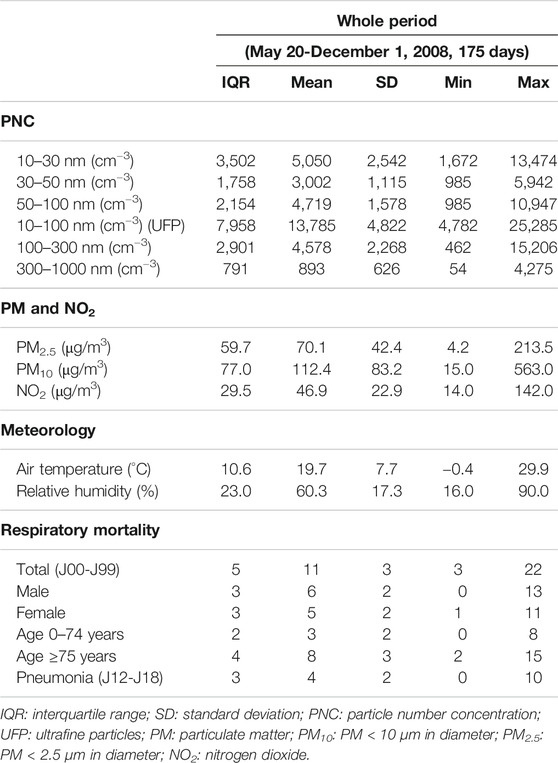
TABLE 1. Descriptive statistics of particle metrics, meteorology and respiratory mortality for the period May 20 to December 1, 2008.
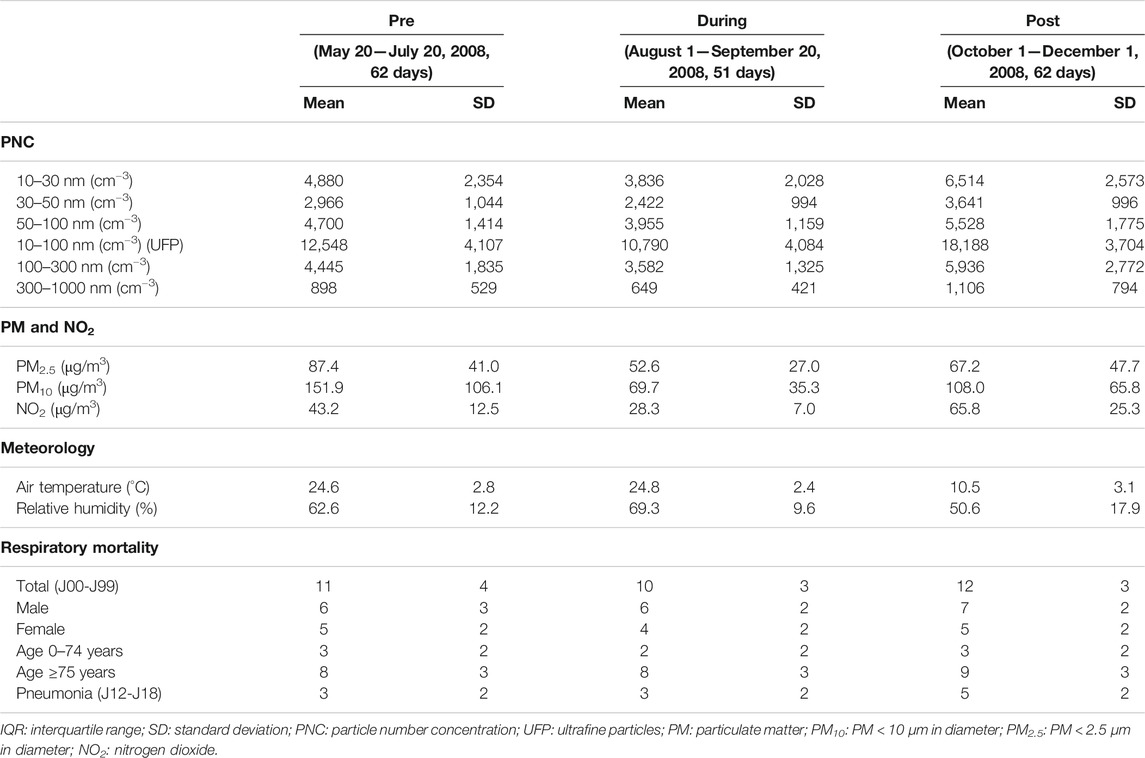
TABLE 2. Description of particle metrics, meteorological variables and respiratory mortality pre, during, and post the Olympic Games 2008 in Beijing.
PM mass was moderately to highly correlated with the PNC size fractions 100–300 nm and 300–1000 nm and NO2, but not correlated with PNC size fractions below 100 nm (Table 3). PNC in the smallest size fraction 10–30 nm as well as PNC in the size class 30–50 nm were highly correlated with each other, as were PNC in the size fractions 30–50 nm and 50–100 nm. PNC in the smaller size fractions showed high correlations with UFP, negative correlations with meteorological variables, and no correlations with PNC size fractions above 300 nm, PM mass, or NO2.
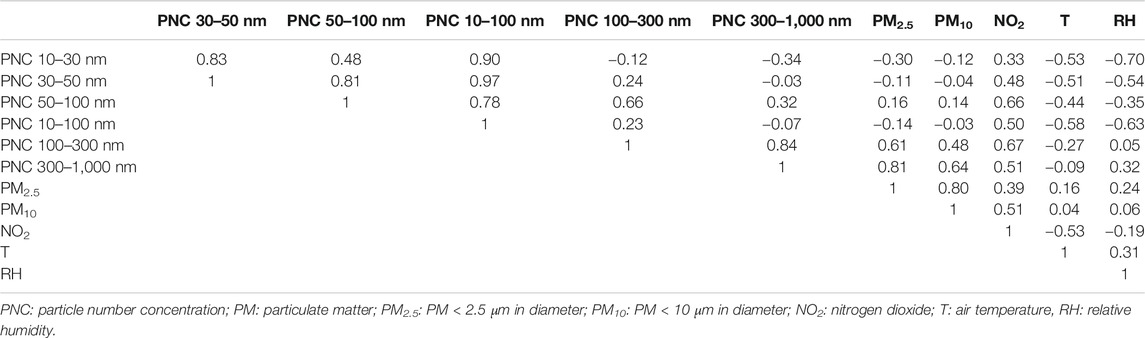
TABLE 3. Spearman rank correlation coefficients between daily mean values of air pollutants and meteorological variables for the period May 20 to December 1, 2008.
Effects of Particulate Air Pollution on Respiratory Mortality for the Whole Study Period
Associations between respiratory mortality and air pollution were investigated for exposure lags of 0–10 days. As the effects declined after a lag of seven days, we only present selected lags reflecting immediate (lag1) and delayed associations (lag4 and lag7). Figure 1 shows the associations between air pollutants and overall respiratory and pneumonia mortality for the whole study period, respectively. The effects are given as percent changes in mortality per an interquartile range (IQR) increase in the respective pollutant. We observed strongest effects of PM mass and NO2 on respiratory mortality and pneumonia mortality at a lag of four days. For the different PNC size fractions, the strongest effects were observed with a delay of seven days. Effects on respiratory mortality were more pronounced for the size ranges 30–50 nm, and 50–100 nm as well as 10–100 nm (UFP); for pneumonia mortality, the effects were stronger in size ranges 10–30 nm, 30–50 nm, and 10–100 nm (UFP). In our age- and gender-specific analyses, we did not find a consistent pattern (Supplementary Figure S3).
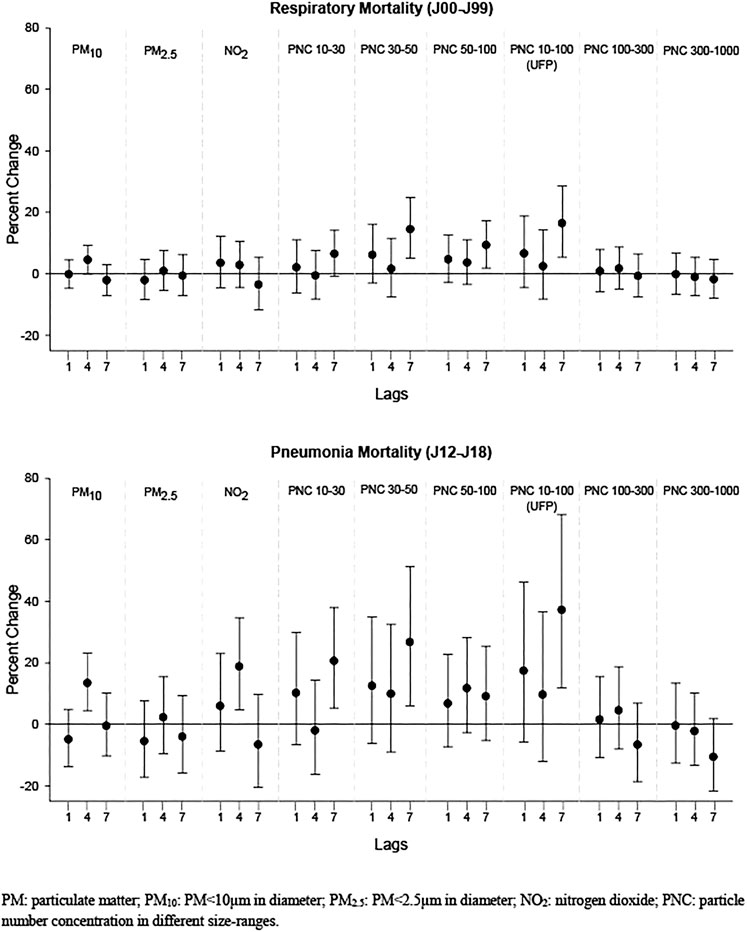
FIGURE 1. Percent change (95%-confidence interval) in respiratory mortality (top) as well as pneumonia mortality (bottom) per an interquartile range increase in air pollutants at lags 1, 4, and 7 in the urban area of Beijing for the period May 20 to December 1, 2008.
Our two-pollutant models showed that the effects of UFP stayed relatively stable when adjusting for PM10, PM2.5, and NO2 (Figure 2). However, PM10, PM2.5, and NO2 effects all slightly increased when additionally considering another pollutant in the models.
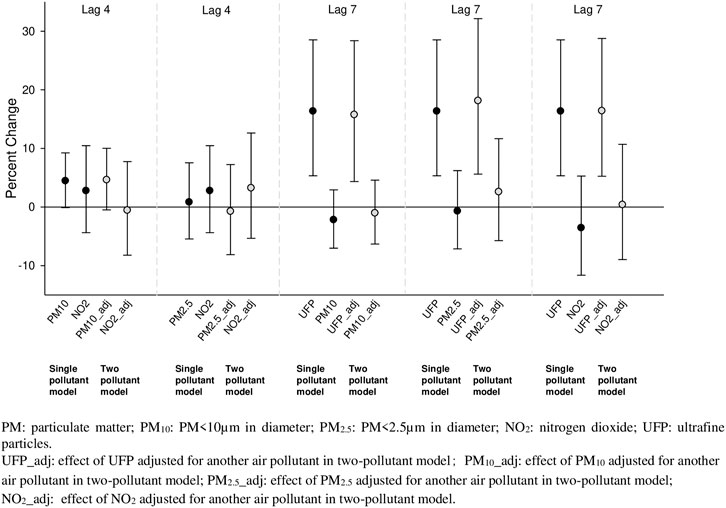
FIGURE 2. Percent change (95%-confidence interval) in respiratory mortality per an interquartile range increase in PM mass, NO2 and UFP for the period May 20 to December 1, 2008. Single-pollutant and two-pollutant models.
Further, we assessed the potential modification of particle effects by air mass origin; this approach indirectly considers possible variation within the chemical composition of PM. Results showed that associations between respiratory mortality and air pollutants were (slightly) stronger for air masses from areas south of or around Beijing (cluster 1) compared with the air masses arriving from north-western directions with average to high wind speed (cluster 2) (Supplementary Figure S4); however, the differences between the two clusters were not statistically significant.
Effects of Particulate Air Pollution on Mortality in the Sub-periods
We assessed potential effect modifications by period (pre vs. during vs. post Olympics and Paralympics) only for selected lags—for PM mass and NO2 with a lag of four days and the different PNC fractions at lag7. We overall found decreases in the effects of particulate air pollution over the sub-periods (from the pre-to post-Olympic period), except for the larger PNC size ranges (Figure 3). There, associations were lowest during the Olympic Games, and increased again afterward. Regarding pneumonia mortality, we observed that the risks were lowest during the Olympic period when strictest control measures have been applied. These differences were especially pronounced for PM2.5 and the PNC size fractions 100–300 nm and 300–1000 nm; for the latter, effect modifications reached significance.
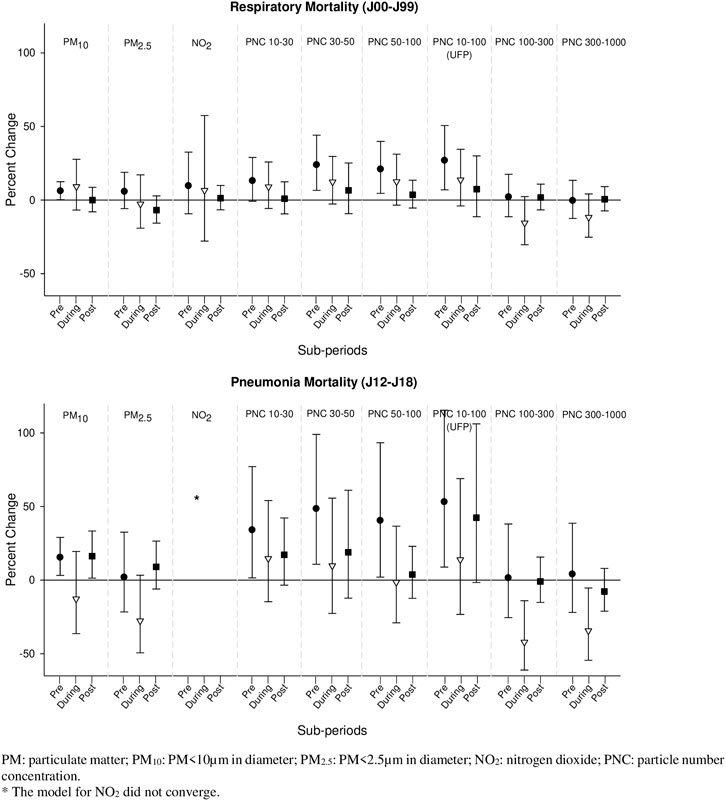
FIGURE 3. Percent change (95%-confidence interval) in respiratory mortality per an interquartile range increase in PM mass (lag4), NO2 (lag4), and PNC (lag7). Effect modification by period (pre-Olympic period (May 20–July 20), during-Olympic period (August 1-September 20), and post-Olympic period (October 1–December 1)).
Sensitivity Analyses
Applying an alternative definition of the sub-periods (Zhang et al., 2013) or using different df for air temperature and relative humidity did not result in notable changes in the air pollution effects. We also did not observe deviations from linearity of the exposure-response relationships (data not shown).
Discussion
Air quality improved during the 2008 Olympic and Paralympic Games in Beijing–we observed large concentration decreases in all of the examined air pollutants. However, after the relaxation of the emission control measures in October 2008, air pollution concentrations increased again; some air pollutants even reached or exceeded the levels observed before the Olympic and Paralympic Games. For the whole study period (May 20 to December 1, 2008), we observed an increase in respiratory and pneumonia events in association with PM mass and different PNC size ranges—effects were more pronounced in smaller particle size ranges (10–30 nm, 30–50 nm and 50–100 nm). When assessing effect modification by sub-periods, findings indicate that improved air quality during the Olympic and Paralympic Games resulted in decreased respiratory and pneumonia mortality. Respiratory mortality continued to be decreased even in the post-Olympics period.
Decreases in air pollution concentrations during the 2008 Olympic and Paralympic Games have already been reported (Wang et al., 2009; Schleicher et al., 2012; Schleicher et al., 2011; Sun et al., 2011). For example, a study applying a mobile laboratory to measure PM, black carbon, and gaseous pollutants along Beijing’s Fourth Ring Road on selected days before, during, and after the Olympics found large reductions in pollutants’ concentrations during the Games period (Wang et al., 2009). This study also observed concentration increases after the relaxation of the emission control measures (after September 20), which is in agreement with our results. Further, Schleicher et al. (2011) and Schleicher et al. (2012) examined size-segregated mass concentrations of particles before, during, and after the period of the Olympic Summer Games and also found considerably reduced air pollution levels.
In this study, a 7,958 particles/cm3 in UFP led to a 16.3% increase (95% confidence interval [CI]: 4.3%; 26.5%) in respiratory mortality with a delay of seven days. UFP effects were also seen on pneumonia mortality. Epidemiologic evidence on short-term association between UFP and respiratory mortality is still limited mostly due to the limited availability of appropriate measurement data (Ohlwein et al., 2019). In one of the very first studies conducted in Erfurt, Germany, researchers found an increase of 15.5% (95% CI: 5.5%; 26.4%) in respiratory mortality per 12,690 particles/cm3 increase in UFP one day after exposure (Wichmann et al., 2000), a result later confirmed in the same city (Stölzelet al., 2007). Moreover, Atkinson and colleagues found excess risks for respiratory mortality one day after PNC exposure in London, with an estimate of 2.3% (95% CI: 0.1%; 4.8%) per 10,166 particles/cm3 increase in PNC (Atkinson et al., 2010). A study conducted in the Ruhr Area, Germany, between March 2009 and December 2014, found a 4.51% (95% CI: 0.37%; 8.81%) increase in respiratory mortality with a delay of six days in association with 4,900.2 particles/cm3. Further, in a study conducted in five Central-Eastern European cities, results suggested a delayed association between UFP and respiratory mortality (9.9% [95% CI: −6.3%; 28.8%] increase in association with an increase of 2,750 particles/cm3 in the 6-days average of UFP) (Lanzinger et al., 2016). However, studies conducted in Helsinki, Finland (Halonen et al., 2009), Prague, Czech Republic (Branis et al., 2010), and a multi-city study in eight European urban areas (Stafoggia et al., 2017) failed to show associations between PNC and respiratory mortality.
Whereas earlier studies on UFP/PNC and respiratory mortality pointed to more immediate effects with UFP/PNC (Wichmann et al., 2000; Atkinson et al., 2010), more recent studies—including this study–pointed to stronger effects with a longer delay up to seven days (Lanzinger et al., 2016; Stafoggia et al., 2017; Hennig et al., 2018). Possible explanations for the change in the lag pattern might be changes in the particle sources, physical or chemical properties.
Furthermore, we also observed effects of PM10 at a lag four days, but no associations between PM2.5 and respiratory or pneumonia mortality. This finding is somehow contradictory to previous studies; for example, a recent global multi-city study reported that PM2.5 accounted for a larger proportion of the effects of PM10 and PM2.5 combined (Liu et al., 2019). However, our findings are in line with a very recent systematic review and meta-analysis (Orellano et al., 2020).
In our study, particles in the size range of 30–50 nm might have a strong role. It has been shown that traffic emissions strongly influence Aitken mode particles (defined as particles with a diameter between 30 and 100 nm) such as soot, which is also the case in Beijing (Breitner et al., 2011). These soot particles may have adverse effects on human health as they may contain a high amount of polycyclic aromatic hydrocarbons (PAH) and toxic metals (Branis et al., 2010; Breitner et al., 2011). However, Leitte et al. (2012) investigated the association between PNC and respiratory mortality in Beijing from March 2004 until August 2005 and only observed increases in respiratory mortality for PNC in the size range of 300–1000 nm. Possible explanations for the inconsistent results might be changes in the particle sources. Further, Halonen et al. (2009) also found stronger associations between accumulation mode particles (AMP, 0.1–0.29 μm) and mortality due to respiratory causes and pneumonia. The authors hypothesized that their finding of a stronger association between AMP and respiratory mortality may be related to a better exposure assessment of these particles compared to particles in other size fractions (including UFP) (Halonen et al., 2009).
The biological mechanisms proposed in the literature by which particulate air pollution–including UFP—could cause, exacerbate, or accelerate the progression of respiratory diseases are mainly related to altered immune system response, oxidant-mediated cellular damage (including the production of reactive oxygen species (ROS) and oxidative stress) and exaggerated local and systemic inflammatory response (Leikauf et al., 2020; Pompilio and Di Bonaventura, 2020). Thereby, particles may induce an inflammatory response in the lung and modify immune response to subsequent stimuli, such as infectious pathogens. Once an infection occurs, increased pulmonary and systemic inflammation may also contribute to, for example, greater pneumonia (Pirozzi et al., 2017; Croft et al., 2019).
Accountability studies are defined as “a subset of epidemiologic studies that attempt to exploit policy-related, planned, or controlled interventions that result in changes (usually reductions) in exposure and/or exposure variability,” according to a publication by the Health Effects Institute (HEI) (Pope 2009). Examples are studies on the ban of the sale of coal in Dublin, Ireland (Dockery et al., 2013), or traffic restrictions during the 1996 Summer Olympic Games in Atlanta, Georgia, United States (Peel et al., 2010) or the 2002 summer Asian games in Busan, Korea (Lee et al., 2007). The current study fits this description: The Chinese government and Beijing’s municipal government launched strict emission control policies before and during the Olympic period which resulted in a substantial reduction of levels of air pollutants. In the analysis of the modification effects by periods (pre-during-post Olympic Games), we observed improved air quality during the Olympic and Paralympic Games which resulted in a reduction in respiratory mortality. Respiratory mortality continued to be decreased even in the post-Olympics period, although levels of air pollution concentrations were increasing again. One possible explanation for this finding might be a delayed benefit from air pollution reductions in addition to a prompt reduction in risk during the Olympics. Regarding pneumonia mortality, we observed that the risks were lowest during the period of the Olympic Games where strongest control measures have been applied. These differences were especially pronounced for PM2.5 and the PNC size fractions 100–300 nm and 300–1000 nm.
Strengths and Limitations
Accountability studies are considered to be the “closest epidemiologic equivalent to controlled experimental studies,” since “there is more exogeneity with regard to the changes in air pollution exposures and, thus, less opportunity for confounding” (Pope 2009). In this study, we defined three sub-periods according to when the emission control measures came into force. We also specified a transition interval of ten days between each of the sub-periods to allow enough time for a change in the air pollution levels after the measures came into force (Su et al., 2015). Moreover, we adjusted for air temperature, relative humidity–both known risk factors of mortality—and day of the week as well as public holidays as potential confounders (Welty and Zeger, 2005). We also considered the heating season during which increasing air pollution concentrations were observed. Our final models therefore can be considered to be conservative and robust, as indicated by additional sensitivity analyses.
There were also concerns about a change in the population during the Olympics period, which could lead to changes in the rates of respiratory mortality. Although millions of visitors from across the globe visited Beijing because of the Olympics, we used mortality data that based on records for registered permanent residence in Beijing; therefore, we can assume a probably stable population.
In this study, particle number size distribution data was measured at one fixed monitoring site; therefore, exposure misclassification might be possible due to greater spatial variability, especially for UFP with higher spatial variability compared to fine PM mass concentrations. However, our monitoring site could be classified as an urban background station (Breitner et al., 2011; Leitte et al., 2012); moreover, mass concentrations of fine PM were shown to be evenly distributed over the urban area of Beijing (Cheng et al., 2007; Wang et al., 2010). Hence, it can be assumed that our monitoring site collected the average exposure of the study area (Su et al., 2015). Furthermore, concurrent measurements of UFP at different sites within one city/urban area have shown good correlations regarding day-to-day variations despite differing magnitudes in space (Cyrys et al., 2008; Meier et al., 2015). Therefore, if the background site is carefully chosen, it might well represent UFP exposures of the average population for short-term studies (Cyrys et al., 2008; Meier et al., 2015). Unfortunately, we did not have particle number size distribution data for a longer post-Olympic period to see if the particle effects on respiratory mortality increased again later on. Moreover, we were not able to investigate the effects of particulate air pollution on cause-specific respiratory mortality, as we did not have enough power for other cause-specific respiratory mortality than pneumonia. We also cannot rule out that inherent immunity might have influenced the results of this study. However, studies have suggested that inhalation of particulate air pollution may also modulate the innate immune system and affect respiratory innate immune responses (Bauer et al., 2012; Mishra et al., 2020). Further, we do not have information on previous illnesses of the deceased persons.
Conclusion
In summary, we observed large concentration decreases in all measured air pollutants during the 2008 Summer Olympic Games and Paralympics in Beijing. Particulate air pollution showed associations with respiratory mortality, with more pronounced effects in the smaller particle size ranges (10–30 nm, 30–50 nm, and UFP). Results from the specific sub-periods analysis indicated a reduction in respiratory mortality due to a reduction of the air pollution concentrations during the Olympics. The findings further suggest that ultrafine particles may be particularly adverse for human health, and the effect is largely independent of other airborne pollutants. At present, administrative air quality measurements of national networks, not only in China, measure only particle mass concentrations such as PM10 or PM2.5. In the future, regular air pollution observations should be extended by measurements of particle number size distributions.
Data Availability Statement
The datasets presented in this article are not readily available because data cannot be made available due to data security reasons. Requests to access the datasets should be directed to c3VzYW5uZS5icmVpdG5lckBoZWxtaG9sdHotbXVlbmNoZW4uZGU=.
Author Contributions
SB contributed to data collection, data management, data analysis, and drafted the manuscript; CS contributed to data collection and data management, analyzed the data, and drafted the manuscript; UF was lead partner of the project and contributed to data management and manuscript editing; AW contributed to data collection, data management, and manuscript editing; JC contributed to data collection, data management, and manuscript editing; XP. was lead partner of the project and contributed to data collection and manuscript editing; H-EW coordinated the project, contributed to data analysis and manuscript editing; AS contributed to data management, data analysis, and manuscript editing; AP contributed to data collection and manuscript editing.
Funding
This work was supported by the German Research Foundation (DFG) (WI 621/16-1, FR 1417/4-1, HE 2805/3-1; WI 1449/18-1). Parts of this work were funded by a scholarship being awarded to Chang Su (File no.2011601062) under the State Scholarship Fund by the China Scholarship Council (CSC).
Conflict of Interest
The authors declare that the research was conducted in the absence of any commercial or financial relationships that could be construed as a potential conflict of interest.
Publisher’s Note
All claims expressed in this article are solely those of the authors and do not necessarily represent those of their affiliated organizations, or those of the publisher, the editors and the reviewers. Any product that may be evaluated in this article, or claim that may be made by its manufacturer, is not guaranteed or endorsed by the publisher.
Supplementary Material
The Supplementary Material for this article can be found online at: https://www.frontiersin.org/articles/10.3389/fenvs.2021.624180/full#supplementary-material.
References
Atkinson, R. W., Fuller, G. W., Anderson, H. R., Harrison, R. M., and Armstrong, B. (2010). Urban Ambient Particle Metrics and Health: a Time-Series Analysis. Epidemiology 21 (4), 501–511. doi:10.1097/EDE.0b013e3181debc88
Bauer, R. N., Diaz-Sanchez, D., and Jaspers, I. (2012). Effects of Air Pollutants on Innate Immunity: the Role of Toll-like Receptors and Nucleotide-Binding Oligomerization Domain-like Receptors. J. Allergy Clin. Immunol. 129 (1), 14–24. doi:10.1016/j.jaci.2011.11.004
Branis, M., Vyskovska, J., Maly, M., and Hovorka, J. (2010). Association of Size-Resolved Number Concentrations of Particulate Matter with Cardiovascular and Respiratory Hospital Admissions and Mortality in Prague, Czech Republic. Inhal Toxicol. 22 (Suppl. 2), 21–28. doi:10.3109/08958378.2010.504758
Breitner, S., Liu, L., Cyrys, J., Brüske, I., Franck, U., Schlink, U., et al. (2011). Sub-micrometer Particulate Air Pollution and Cardiovascular Mortality in Beijing, China. Sci. Total Environ. 409 (24), 5196–5204. doi:10.1016/j.scitotenv.2011.08.023
Chen, K., Schneider, A., Cyrys, J., Wolf, K., Meisinger, C., Heier, M., et al. (2020). Hourly Exposure to Ultrafine Particle Metrics and the Onset of Myocardial Infarction in Augsburg, Germany. Environ. Health Perspect. 128 (1), 017003. doi:10.1289/EHP5478
Chen, R., Kan, H., Chen, B., Huang, W., Bai, Z., Song, G., et al. (2012). Association of Particulate Air Pollution with Daily Mortality: the China Air Pollution and Health Effects Study. Am. J. Epidemiol. 175 (11), 1173–1181. doi:10.1093/aje/kwr425
Cheng, S., Chen, D., Li, J., Wang, H., and Guo, X. (2007). The Assessment of Emission-Source Contributions to Air Quality by Using a Coupled MM5-ARPS-CMAQ Modeling System: A Case Study in the Beijing Metropolitan Region, China. Environ. Model. Softw. 22 (11), 1601–1616. doi:10.1016/j.envsoft.2006.11.003
Croft, D. P., Zhang, W., Lin, S., Thurston, S. W., Hopke, P. K., Masiol, M., et al. (2019). The Association between Respiratory Infection and Air Pollution in the Setting of Air Quality Policy and Economic Change. Ann. ATS 16 (3), 321–330. doi:10.1513/AnnalsATS.201810-691OC
Cyrys, J., Pitz, M., Heinrich, J., Wichmann, H.-E., and Peters, A. (2008). Spatial and Temporal Variation of Particle Number Concentration in Augsburg, Germany. Sci. Total Environ. 401 (1-3), 168–175. doi:10.1016/j.scitotenv.2008.03.043
Dockery, D. W., Rich, D. Q., Goodman, P. G., Clancy, L., Ohman-Strickland, P., George, P., et al. (2013). Effect of Air Pollution Control on Mortality and Hospital Admissions in Ireland. Res. Rep. Health Eff. Inst. 176, 3–109.
Fang, M., Chan, C. K., and Yao, X. (2009). Managing Air Quality in a Rapidly Developing Nation: China. Atmos. Environ. 43 (1), 79–86. doi:10.1016/j.atmosenv.2008.09.064
Frampton, M. W., and Rich, D. Q. (2016). Does Particle Size Matter? Ultrafine Particles and Hospital Visits in Eastern Europe. Am. J. Respir. Crit. Care Med. 194 (10), 1180–1182. doi:10.1164/rccm.201606-1164ED
Gong, J., Zhu, T., Kipen, H., Wang, G., Hu, M., Guo, Q., et al. (2014). Comparisons of Ultrafine and Fine Particles in Their Associations with Biomarkers Reflecting Physiological Pathways. Environ. Sci. Technol. 48 (9), 5264–5273. doi:10.1021/es5006016
Gu, J., Pitz, M., Breitner, S., Birmili, W., von Klot, S., Schneider, A., et al. (2012). Selection of Key Ambient Particulate Variables for Epidemiological Studies—Applying Cluster and Heatmap Analyses as Tools for Data Reduction. Sci. Total Environ. 435-436, 541–550. doi:10.1016/j.scitotenv.2012.07.040
Halonen, J. I., Lanki, T., Yli-Tuomi, T., Tiittanen, P., Kulmala, M., and Pekkanen, J. (2009). Particulate Air Pollution and Acute Cardiorespiratory Hospital Admissions and Mortality Among the Elderly. Epidemiology 20 (1), 143–153. doi:10.1097/EDE.0b013e31818c7237
Hei, (2013). “Understanding the Health Effects of Ambient Ultrafine Particles,” in HEI Perspectives. Editor H. R. P. o. U. Particles (Boston, MA: Health Effects Institute).
Hennig, F., Quass, U., Hellack, B., Küpper, M., Kuhlbusch, T. A. J., Stafoggia, M., et al. (2018). Ultrafine and Fine Particle Number and Surface Area Concentrations and Daily Cause-specific Mortality in the Ruhr Area, Germany, 2009-2014. Environ. Health Perspect. 126 (2), 027008. doi:10.1289/EHP2054
Huang, W., Wang, G., Lu, S.-E., Kipen, H., Wang, Y., Hu, M., et al. (2012). Inflammatory and Oxidative Stress Responses of Healthy Young Adults to Changes in Air Quality during the Beijing Olympics. Am. J. Respir. Crit. Care Med. 186 (11), 1150–1159. doi:10.1164/rccm.201205-0850OC
Lanzinger, S., Schneider, A., Breitner, S., Stafoggia, M., Erzen, I., Dostal, M., et al. (2016). Associations between Ultrafine and Fine Particles and Mortality in Five Central European Cities—Results from the UFIREG Study. Environ. Int. 88, 44–52. doi:10.1016/j.envint.2015.12.006
Lee, J.-T., Son, J.-Y., and Cho, Y.-S. (2007). Benefits of Mitigated Ambient Air Quality Due to Transportation Control on Childhood Asthma Hospitalization during the 2002 Summer Asian Games in Busan, Korea. J. Air Waste Manag. Assoc. 57 (8), 968–973. doi:10.3155/1047-3289.57.8.968
Leikauf, G. D., Kim, S.-H., and Jang, A.-S. (2020). Mechanisms of Ultrafine Particle-Induced Respiratory Health Effects. Exp. Mol. Med. 52 (3), 329–337. doi:10.1038/s12276-020-0394-0
Leitte, A. M., Schlink, U., Herbarth, O., Wiedensohler, A., Pan, X.-C., Hu, M., et al. (2012). Associations between Size-Segregated Particle Number Concentrations and Respiratory Mortality in Beijing, China. Int. J. Environ. Health Res. 22 (2), 119–133. doi:10.1080/09603123.2011.605878
Lin, W., Huang, W., Zhu, T., Hu, M., Brunekreef, B., Zhang, Y., et al. (2011). Acute Respiratory Inflammation in Children and Black Carbon in Ambient Air before and during the 2008 Beijing Olympics. Environ. Health Perspect. 119 (10), 1507–1512. doi:10.1289/ehp.1103461
Liu, C., Chen, R., Sera, F., Vicedo-Cabrera, A. M., Guo, Y., Tong, S., et al. (2019). Ambient Particulate Air Pollution and Daily Mortality in 652 Cities. N. Engl. J. Med. 381 (8), 705–715. doi:10.1056/NEJMoa1817364
Meier, R., Eeftens, M., Aguilera, I., Phuleria, H. C., Ineichen, A., Davey, M., et al. (2015). Ambient Ultrafine Particle Levels at Residential and Reference Sites in Urban and Rural Switzerland. Environ. Sci. Technol. 49 (5), 2709–2715. doi:10.1021/es505246m
Mishra, R., Krishnamoorthy, P., Gangamma, S., Raut, A. A., and Kumar, H. (2020). Particulate Matter (PM10) Enhances RNA Virus Infection through Modulation of Innate Immune Responses. Environ. Pollut. 266, 115148. doi:10.1016/j.envpol.2020.115148
Mu, L., Deng, F., Tian, L., Li, Y., Swanson, M., Ying, J., et al. (2014). Peak Expiratory Flow, Breath Rate and Blood Pressure in Adults with Changes in Particulate Matter Air Pollution during the Beijing Olympics: a Panel Study. Environ. Res. 133, 4–11. doi:10.1016/j.envres.2014.05.006
Ohlwein, S., Kappeler, R., Kutlar Joss, M., Künzli, N., and Hoffmann, B. (2019). Health Effects of Ultrafine Particles: a Systematic Literature Review Update of Epidemiological Evidence. Int. J. Public Health 64 (4), 547–559. doi:10.1007/s00038-019-01202-7
Orellano, P., Reynoso, J., Quaranta, N., Bardach, A., and Ciapponi, A. (2020). Short-term Exposure to Particulate Matter (PM10 and PM2.5), Nitrogen Dioxide (NO2), and Ozone (O3) and All-Cause and Cause-specific Mortality: Systematic Review and Meta-Analysis. Environ. Int. 142, 105876. doi:10.1016/j.envint.2020.105876
Peel, J. L., Klein, M., Flanders, W. D., Mulholland, J. A., Tolbert, P. E., and Committee, H. E. I. H. R. (2010). Impact of Improved Air Quality during the 1996 Summer Olympic Games in Atlanta on Multiple Cardiovascular and Respiratory Outcomes. Res. Rep. Health Eff. Inst. 148, 3–33. discussion 25-33.
Pirozzi, C. S., Jones, B. E., VanDerslice, J. A., Zhang, Y., Paine, R., and Dean, N. C. (2018). Short-Term Air Pollution and Incident Pneumonia. A Case-Crossover Study. Ann. ATS 15 (4), 449–459. doi:10.1513/AnnalsATS.201706-495OC
Pompilio, A., and Di Bonaventura, G. (2020). Ambient Air Pollution and Respiratory Bacterial Infections, a Troubling Association: Epidemiology, Underlying Mechanisms, and Future Challenges. Crit. Rev. Microbiol. 46 (5), 600–630. doi:10.1080/1040841X.2020.1816894
Pope, C. A. I. (2009). “Accountability Studies of Air Pollution and Human Health: where Are We Now and where Does the Research Go Next?,”. in: HEI Workshop on Further Research to Assess the Health Impacts of Actions Taken to Improve Air Quality. Boston, MA: Health Effects Institute. doi:10.1093/obo/9780199756797-0122
Rich, D. Q., Kipen, H. M., Huang, W., Wang, G., Wang, Y., Zhu, P., et al. (2012). Association between Changes in Air Pollution Levels during the Beijing Olympics and Biomarkers of Inflammation and Thrombosis in Healthy Young Adults. Jama 307 (19), 2068–2078. doi:10.1001/jama.2012.3488
Schleicher, N., Norra, S., Chen, Y., Chai, F., and Wang, S. (2012). Efficiency of Mitigation Measures to Reduce Particulate Air Pollution-A Case Study during the Olympic Summer Games 2008 in Beijing, China. Sci. Total Environ. 427-428, 146–158. doi:10.1016/j.scitotenv.2012.04.004
Schleicher, N., Norra, S., Dietze, V., Yu, Y., Fricker, M., Kaminski, U., et al. (2011). The Effect of Mitigation Measures on Size Distributed Mass Concentrations of Atmospheric Particles and Black Carbon Concentrations during the Olympic Summer Games 2008 in Beijing. Sci. Total Environ. 412-413, 185–193. doi:10.1016/j.scitotenv.2011.09.084
Schraufnagel, D. E. (2020). The Health Effects of Ultrafine Particles. Exp. Mol. Med. 52 (3), 311–317. doi:10.1038/s12276-020-0403-3
Stafoggia, M., Schneider, A., Cyrys, J., Samoli, E., Andersen, Z. J., Bedada, G. B., et al. (2017). Association between Short-Term Exposure to Ultrafine Particles and Mortality in Eight European Urban Areas. Epidemiology 28 (2), 172–180. doi:10.1097/EDE.0000000000000599
Stölzel, M., Breitner, S., Cyrys, J., Pitz, M., Wölke, G., Kreyling, W., et al. (2007). Daily Mortality and Particulate Matter in Different Size Classes in Erfurt, Germany. J. Expo. Sci. Environ. Epidemiol. 17 (5), 458–467. doi:10.1038/sj.jes.7500538
Su, C., Hampel, R., Franck, U., Wiedensohler, A., Cyrys, J., Pan, X., et al. (2015). Assessing Responses of Cardiovascular Mortality to Particulate Matter Air Pollution for Pre-, during- and Post-2008 Olympics Periods. Environ. Res. 142, 112–122. doi:10.1016/j.envres.2015.06.025
Sun, Y., Wang, L., Wang, Y., Quan, L., and Zirui, L. (2011). In situ measurements of SO2, NOx, NOy, and O3 in Beijing, China during August 2008. Sci. Total Environ. 409 (5), 933–940. doi:10.1016/j.scitotenv.2010.11.007
Thurston, G. D., Kipen, H., Annesi-Maesano, I., Balmes, J., Brook, R. D., Cromar, K., et al. (2017). A Joint ERS/ATS Policy Statement: what Constitutes an Adverse Health Effect of Air Pollution? an Analytical Framework. Eur. Respir. J. 49 (1), 1600419. doi:10.1183/13993003.00419-2016
Wang, M., Zhu, T., Zheng, J., Zhang, R. Y., Zhang, S. Q., Xie, X. X., et al. (2009). Use of a Mobile Laboratory to Evaluate Changes in On-Road Air Pollutants during the Beijing 2008 Summer Olympics. Atmos. Chem. Phys. 9 (21), 8247–8263. doi:10.5194/acp-9-8247-2009
Wang, Z., Chen, L., Tao, J., Zhang, Y., and Su, L. (2010). Satellite-based Estimation of Regional Particulate Matter (PM) in Beijing Using Vertical-And-RH Correcting Method. Remote Sensing Environ. 114 (1), 50–63. doi:10.1016/j.rse.2009.08.009
Wehner, B., Birmili, W., Ditas, F., Wu, Z., Hu, M., Liu, X., et al. (2008). Relationships between Submicrometer Particulate Air Pollution and Air Mass History in Beijing, China, 2004-2006. Atmos. Chem. Phys. 8 (20), 6155–6168. doi:10.5194/acp-8-6155-2008
Wehner, B., Wiedensohler, A., Tuch, T. M., Wu, Z. J., Hu, M., Slanina, J., et al. (2004). Variability of the Aerosol Number Size Distribution in Beijing, China: New Particle Formation, Dust Storms, and High Continental Background. Geophys. Res. Lett. 31 (22), L22108. doi:10.1029/2004gl021596
Welty, L. J., and Zeger, S. L. (2005). Are the Acute Effects of Particulate Matter on Mortality in the National Morbidity, Mortality, and Air Pollution Study the Result of Inadequate Control for Weather and Season? A Sensitivity Analysis Using Flexible Distributed Lag Models. Am. J. Epidemiol. 162 (1), 80–88. doi:10.1093/aje/kwi157
Wichmann, H. E., Spix, C., Tuch, T., Wolke, G., Peters, A., Heinrich, J., et al. (2000). Daily Mortality and Fine and Ultrafine Particles in Erfurt, Germany Part I: Role of Particle Number and Particle Mass. Res. Rep. Health Eff. Inst. 98, 5–94. discussion 87-94.
Wu, S., Deng, F., Niu, J., Huang, Q., Liu, Y., and Guo, X. (2010). Association of Heart Rate Variability in Taxi Drivers with Marked Changes in Particulate Air Pollution in Beijing in 2008. Environ. Health Perspect. 118 (1), 87–91. doi:10.1289/ehp.0900818
Yue, D., Hu, M., Wu, Z., Wang, Z., Guo, S., Wehner, B., et al. (2009). Characteristics of Aerosol Size Distributions and New Particle Formation in the Summer in Beijing. J. Geophys. Res. 114 (D2). doi:10.1029/2008jd010894
Keywords: particulate air pollution, ultrafine particles, respiratory mortality, accountability study, Beijing 2008 Olympic and Paralympic games
Citation: Breitner S, Su C, Franck U, Wiedensohler A, Cyrys J, Pan X, Wichmann H-E, Schneider A and Peters A (2021) The Association Between Particulate Air Pollution and Respiratory Mortality in Beijing Before, During, and After the 2008 Olympic and Paralympic Games. Front. Environ. Sci. 9:624180. doi: 10.3389/fenvs.2021.624180
Received: 02 November 2020; Accepted: 12 April 2021;
Published: 23 July 2021.
Edited by:
Nina Jasmin Schleicher, Imperial College London, United KingdomReviewed by:
Michael Edward Deary, Northumbria University, United KingdomKirpa Ram, Banaras Hindu University, India
Copyright © 2021 Breitner, Su, Franck, Wiedensohler, Cyrys, Pan, Wichmann, Schneider and Peters. This is an open-access article distributed under the terms of the Creative Commons Attribution License (CC BY). The use, distribution or reproduction in other forums is permitted, provided the original author(s) and the copyright owner(s) are credited and that the original publication in this journal is cited, in accordance with accepted academic practice. No use, distribution or reproduction is permitted which does not comply with these terms.
*Correspondence: Susanne Breitner, c3VzYW5uZS5icmVpdG5lckBoZWxtaG9sdHotbXVlbmNoZW4uZGU=
†These authors share first authorship