- 1Department of Estuarine and Delta Studies, NIOZ Royal Netherlands Institute for Sea Research, Yerseke, Netherlands
- 2Department of Physical Geography, Utrecht University, Utrecht, Netherlands
- 3Wageningen Marine Research, Wageningen University and Research, Den Helder, Netherlands
- 4Marine and Animal Ecology Group, Wageningen University and Research, Wageningen, Netherlands
- 5Witteveen + Bos, Park View Plaza, Jakarta Selatan, Indonesia
- 6Faculty of Fisheries & Marine Sciences, Diponegoro University, Semarang, Indonesia
- 7Wetlands International, Wageningen, Netherlands
- 8Unit for Marine and Coastal Systems, Deltares, Delft, Netherlands
- 9Department of Hydraulic Engineering, Delft University of Technology, Delft, Netherlands
Global change processes such as sea level rise and the increasing frequency of severe storms threaten many coastlines around the world and trigger the need for interventions to make these often densely-populated areas safer. Mangroves could be implemented in Nature-Based Flood Defense, provided that we know how to conserve and restore these ecosystems at those locations where they are most needed. In this study, we investigate how best to restore mangroves along an aquaculture coast that is subject to land-subsidence, comparing two common mangrove restoration methods: 1) mangrove restoration by planting and 2) Ecological Mangrove Restoration (EMR); the assistance of natural mangrove regeneration through mangrove habitat restoration. Satellite data revealed that historically, landward mangrove expansion into the active pond zone has mainly occurred through mangrove planting on pond bunds. However, there is potential to create greenbelts along waterways by means of EMR measures, as propagule trap data from the field revealed that propagules of pioneer species were up to 21 times more abundant in creeks of the pond zone than near their source in the coastal zone. This was especially true during the prevailing onshore winds of the wet-season, suggesting that smart seasonal sluice gate management could help to efficiently trap seeds in target ponds. In the coastal zone, field experiments showed that permeable brushwood dams, aimed at expanding mangrove habitat, could not sufficiently overcome subsidence rates to increase natural mangrove expansion in the seaward direction, but did significantly increase the survival of already established (planted) seedlings compared to more wave-exposed sites. The survival and growth rate of EMR-supported plantings greatly varied between species. Out of the four planted species, Rhizophora mucronata had the highest survival (67%) but the lowest growth rate. Whereas the pioneer species Avicennia alba and Avicennia marina had lower survival rates (resp. 35 and 21%), but significantly higher growth rates, even resulting in fruiting young trees within a 16-month timeframe. Overall, we conclude that 1) EMR has potential in the pond zone, given that propagules were observed to reach well into the backwaters; and 2) that mangrove recovery in the coastal zone may be facilitated even at very challenging coastal sites by combining EMR with the planting of pioneer species.
1 Introduction
Climate change effects such as sea level rise and an increased frequency and severity of storms threaten many coastlines around the world (IPCC, 2019). The impact of global climate change is often magnified by regional problems such as land subsidence (Hallegatte et al., 2013; Nicholls et al., 2021), and land use change (Zhang et al., 2021), leading to coastal encroachment. Hence, there is a pressing need for intervention strategies with which to adapt to climate change and reduce flood risks along populated coastlines. Coastal wetlands such as mangroves and salt marshes have gained interest as important ecosystems to reduce vulnerability of coastal communities (e.g. Temmerman et al., 2013; Zhu et al., 2020; Zhang et al., 2021). Coastal wetlands are able to attenuate waves even during storms (Möller et al., 2014; Willemsen et al., 2020), dampen storm surges (Stark et al., 2015; Montgomery et al., 2019), trap sediment and grow with rising sea level (Kirwan et al., 2016). This makes conservation and restoration of such wetlands interesting for coastal protection, and consequently economically interesting. Not only in areas where construction of conventional coastal defense structures, such as sea walls and levees, is not feasible (Winterwerp et al., 2013), but also to reduce costs of such conventional structures at locations where they are feasible (Sutton-Grier et al., 2015; Narayan et al., 2016; van Wesenbeeck et al., 2016; Schoonees et al., 2019).
In tropical regions, mangrove restoration is regarded as a widely applicable strategy to enhance coastal safety (e.g. Alongi, 2008; McIvor et al., 2012). In this, planting has long been a favored approach (Ellison, 2000), especially in the lower intertidal zone where land rights are often not an issue (Erftemeijer and Lewis, 1999). However, this type of planting projects have often given low success rates due to a combination of inappropriate species selection (non-pioneer species) (Ellison, 2000; Primavera and Esteban, 2008) and/or unfortunate site selection (mudflats below mean sea level where mangroves would not naturally occur). When planting is reported to be successful in terms of survival, there is the risk of hampered natural succession, as seedlings are often planted at such high density that the resulting stand leaves little sunlight for potential natural recruitment (Barnuevo et al., 2017; Pranchai et al., 2018; Proisy et al., 2018).
To overcome plantation failure, interests have been shifting increasingly towards restoration of mangrove habitat to promote natural recruitment, rather than using active planting of mangrove propagules or seedlings (Balke and Friess, 2016; Lewis, 2005; Winterwerp et al., 2020, 2013). The focus of mangrove habitat restoration (i.e. EMR), lies in mitigating the establishment thresholds that limit natural propagule settlement and survival (Lewis and Brown, 2014). Lewis (2005) demonstrated the importance of addressing the first threshold, (i.e. limited propagule availability) (Figure 1, panel 0), by digging a creek, thus reestablishing aquatic connectivity at a propagule-deprived location and thereby initiating mangrove regeneration over the course of 6.5 years. At more exposed sites, propagules need to overcome additional thresholds to establish successfully for which they need to surpass three size-dependent windows of opportunity: 1) a flooding-free phase, 2) followed by a wave-free period and 3) an erosion-free period (Figure 1, panels 1–3) (Balke et al., 2015, 2013, 2011). Van Cuong et al. (2015) demonstrated that these windows of opportunity can be extended along eroding shores by placing permeable fences parallel to the coastline. These fences both increased the bed level through sediment trapping (i.e. creating first window) and mitigated the wave stress (i.e. creating second window), allowing natural seedling recruitment to increase from zero to 24,000 seedlings ha−1 over the course of 3 years. The examples provided by Lewis and Van Cuong et al. clearly show that informed mitigation of stressors restricting natural mangrove recruitment can be sufficient for successful restoration. It is noted that this approach requires proper understanding of the natural system, as the optimal method may differ depending on the specific abiotic and biotic constraints at the selected sites.
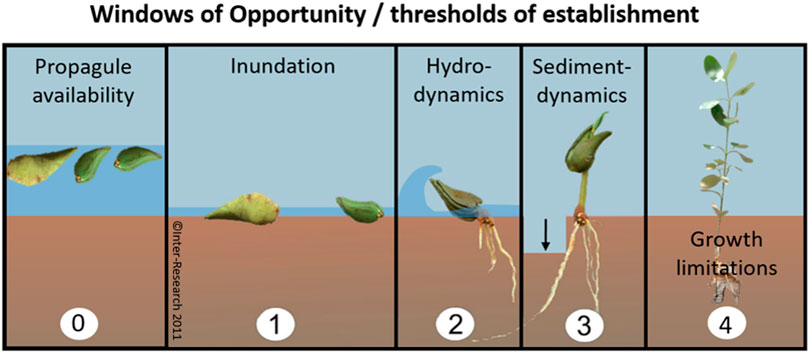
FIGURE 1. Windows of opportunity that propagules should encounter (or threshold of establishment that propagules should overcome) before they can colonize a site. EMR makes use of measures that extend the window of opportunity for natural establishment (0–3). Mangrove planting is either aimed at overcoming propagule limitation (0) or at skipping the thresholds of propagule establishment (1–3) by using larger seedlings or saplings that would, to a certain extent, be able to withstand conditions that are too harsh for smaller seedlings. Finally, saplings need to overcome limitations of growth (4) such as predation, disease or other stressors to grow into reproductive young trees (panels 0 and 4 are additions to Balke et al. (2011)’s Windows of Opportunity figure).
Despite the shift in interest towards EMR instead of mangrove planting, the latter remains a popular practice. From a scientific perspective, planting of larger seedlings may be expected to be effective, as it can either overcome propagule limitations (Figure 1, panel 0) or skip many of the size-dependent establishment thresholds that propagules need to surpass (Figure 1, panel 4). After all, if propagules have established and grown taller, they can persist at locations where newly established seedlings would be uprooted (Balke et al., 2015, 2013, 2011). In that sense, there may be merit to mangrove planting when the purpose is to accelerate mangrove recovery by skipping the most sensitive propagule life-stages at sites where EMR is not entirely sufficient to improve all conditions required for natural colonization. In other words, could mangrove planting help to accelerate the recovery process when it is combined with EMR, especially at challenging locations?
The purpose of this study was to advance current insights into the best mangrove restoration practices, using a 20 km coastline stretch of Demak district, Java, Indonesia as example of a challenging restoration site. The area has a history of aquaculture and is marked by the associated impaired hydrological connectivity. The ongoing subsidence and subsequent erosion of the shoreline further complicate mangrove restoration. Various restoration projects, initiated by the government, NGO’s and local communities, have however attempted to expand the district’s existing mangroves into a greenbelt using either planting or EMR measures. In seaward direction, restoration has mainly been attempted through planting of Rhizophora species. Although more recently, sediment-trapping brushwood dams have also been implemented as an EMR measure to facilitate natural mangrove expansion (Tonneijck et al., 2015). In landward direction, mangrove restoration has mainly focused on compensating the loss of terrestrial vegetation, brought on by salinization after the conversion of rice paddies to aquaculture in the past. Local communities and NGO’s therefore planted Rhizophora spp. in the pond zone, both to re-enforce the pond bunds and to create a local source of firewood. More recently, EMR in the active pond zone has also been initiated in the form of the creation of mangrove habitat in active ponds lining the waterway. To this end, pond owners have partitioned their pond, and sacrificed the parts lining the creek for mangrove rehabilitation, motivated by higher yields from mangrove-associated aquaculture (Bosma et al., 2020). The observed natural expansion in the coastal zone, the various attempts to plant mangroves, and the EMR measures in both the coastal zone and aquaculture pond zone have all had their positive effect on the mangrove cover in Demak in the last decade.
We here evaluated both the effectiveness of the already installed EMR-measures and mangrove plantings, and the effectiveness of combined EMR-measures and plantings in an experimental setting. The aim was to investigate how mangrove regeneration can best be achieved in landward and seaward direction from the subsiding coastline. We explored this question with the following sub-questions: 1) how has mangrove expansion occurred in seaward and landward direction in the past, mainly through planting or mainly through natural expansion? 2) Can hydrological EMR measures (e.g. sluice gate management) induce natural mangrove recovery in the pond zone (i.e. would enough propagules be available at landward sites if hydrological connectivity to target ponds was increased)? 3) Can wave-reducing and sediment-trapping measures (EMR-dams) induce natural mangrove recovery at challenging sites in the coastal zone by increasing the chances of a) new seedling establishment or b) survival of established seedlings? and; 4) Can mangrove planting in combination with EMR-dams accelerate mangrove recovery at challenging coastal sites?
2 Methods
2.1 Site Description
The study site is located along the coast of Demak district at 6.53°S, 110.30°E on Java, Indonesia. The shore is characterized by a mixed, mainly diurnal tide, with a tidal range of 1.1 m (MMAF, 2012). The region has an average annual rainfall of 2,200 mm (Suryadi et al., 2018), with a dry season dominated by an offshore wind (SE) from June to August, and a wet season with onshore wind (NW) from December to February (MMAF, 2012). During the wet season, the maximum significant wave height 4 km offshore is reported to be 1.5 m, with a period of 5.5 s (Tonneijck et al., 2015). The onshore waves during this season leave the coastline of Demak prone to erosion and flooding, which are further exacerbated by land subsidence. These processes have led to two major erosion events of Demak’s shoreline: One between 2002 and 2003 causing the coastline directly east of Semarang city to retreat with 3 km, and one between 2007 and 2009 affecting our study area, 5 km east of Semarang (Figure 2). This erosion event removed the majority of the aquaculture ponds 1 km seaward of the coastal road, making the coastal road an unintended coastal defense structure.
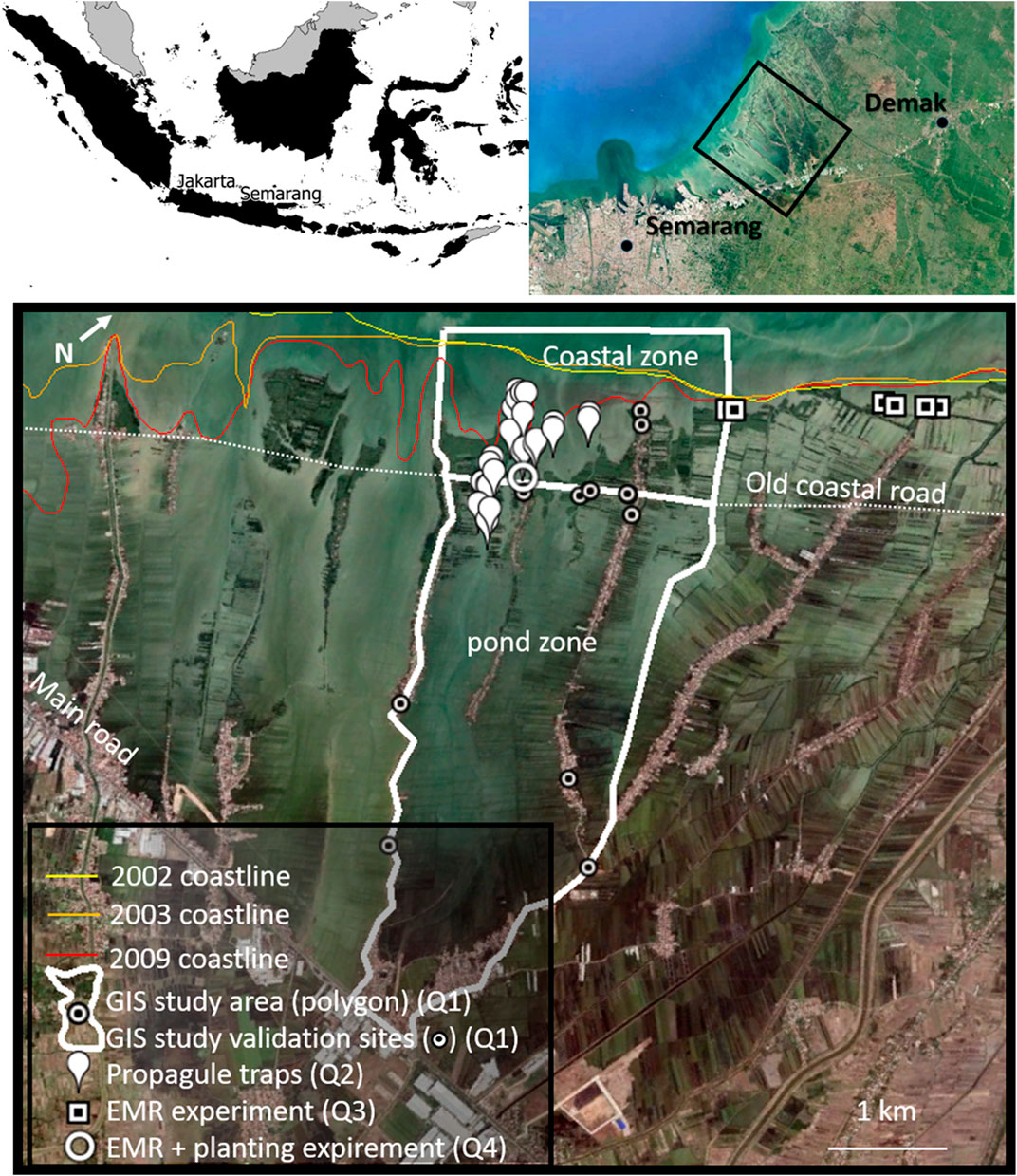
FIGURE 2. The focus area of this paper, the coastline of Sayung subdistrict (black rectangle) in Demak, Indonesia. Historic coastlines are indicated (before, in between and after two major erosion events), as well as the old coastal road which became the last standing structure resisting coastal erosion, dividing the active aquaculture zone from the dynamic coastal zone. The locations of the four experiments linked to the four research questions are indicated with the marks displayed in the legend. Detailed location descriptions and overviews per experiment are shown in the method section.
2.2 Rationale
We studied the natural and assisted processes of mangrove expansion in landward direction (i.e. the pond zone) and seaward direction (i.e. the coastal zone) from the current coastline in the project area (the old coastal road). The pond zone in the study area is characterized by active traditional aquaculture ponds, and drowning abandoned aquaculture ponds towards the coast. The coastal zone in the study is defined as the area ranging from MHW to MLW (the maximum depth at which the EMR-dams are placed), which ranges roughly 0–600 m from the shoreline (Tonneijck et al., 2015). In order to shed light on best practices for mangrove recovery, we addressed research questions one to four in the introduction using the following correspondingly numbered methods. 1) GIS monitoring of natural and planted mangrove vegetation in the coastal zone and pond zone using time series of satellite images (GIS study in Figure 2). 2) A year-round propagule monitoring campaign seawards and landwards of the old coastal road (propagule traps in Figure 2) to assess the potential for natural mangrove colonization in the active pond zone if maximal EMR-hydrology would be applied. 3) A field experiment at multiple coastal sites with different wave exposure conditions to understand the effect of EMR-dams on mangrove recruitment and seedling survival (EMR-experiment in Figure 2). Finally, 4) a field experiment to study the effect of mangrove planting in combination with EMR-dams on seedling growth and survival (EMR + planting in Figure 2). These four experiments are further elaborated on below.
2.3 Q1. GIS Study: Which Mangrove Recovery Process is Dominant in Each Zone?
To quantify the importance of the two contrasting processes of mangrove recovery in Demak, we quantified changes in vegetation types (i.e. natural mangroves, planted mangroves and terrestrial vegetation) in the coastal zone and the pond zone of the study area (Figure 2) over multiple years. We analysed high resolution (<1 m2) satellite images in Google Earth for vegetation cover, starting in 2005 (i.e. before the 2007 erosion event), in 2010, and then on a yearly basis from 2013 until 2018 (Supplementary Table S1). The timeline tool of Google Earth displays mosaics of multiple high-resolution satellite images (i.e. acquired at different dates or from different sources) for the area of interest. We therefore attempted to select the most complete image (no mosaic) for each year of interest (Supplementary Table S1). Due to limited coverage, we were obligated to use a mosaic of two different years for the baseline year 2005, where the majority of the image was comprised of a 22-4-2005 image, and a small corner of the area of interest was dated 31-5-2003. Vegetation cover in both 2003 and 2005 was very low however, so the mosaic was deemed appropriate to use as a pre-erosion baseline.
Images from each year were exported from Google Earth as kml files and imported to ArcGIS with the “kml to layer tool”. Vegetation was manually digitized per image in the two areas of interest, the coastal zone seawards of the old coastal road, and the pond zone landwards of that road (e.g. Supplementary Figure S1). The sparse and fragmented mangroves in the pond zone, interspaced with muddy and algae rich aquaculture ponds, made semi-automated recognition of vegetation cover challenging for our study area. Especially since the imagery was limited to wavelengths in the visible range of the light spectrum (Red, Green and Blue). However, the high-resolution imagery did allow for precise “manual” photointerpretation. A method recognized as reliable for both coastal object identification (Chinnasamy and Parikh, 2020) and fine-scale forest-cover mapping in general (Castilla et al., 2008). In our study area, we identified and categorized vegetation “objects” based on location and pattern. For instance, vegetation along the southern main road (Figure 2) or near active rice paddies (i.e. fresh water available) was classified as “terrestrial”, whereas vegetation lining the coast or aquaculture ponds was classified as “mangroves”. Planted mangroves were distinguished from natural stands based on their darker green color and structured pattern of evenly spaced straight lines, as opposed to the grey-green color and more diffuse pattern displayed by natural mangrove stands. Ultimately, we classified all vegetation into one of three categories: natural mangrove cover (predominantly Avicennia species), planted mangrove cover (predominantly Rhizophora mucronata), or terrestrial tree cover (e.g. garden plants, fruit trees).
Validation of these three vegetation types was conducted during a field-truthing visit by the corresponding author in November 2018 in which the vegetation in randomly selected plots of differing sizes (40–2,400 m2) was identified to species level (Supplementary Figure S1). Within each plot, we also collected forest structure data, both by counting the number of individuals per species and by recording each tree’s diameter at breast height (DBH). From these parameters, we calculated forest parameters such as tree density (n ha−1) and basal area (m2 ha−1). In addition to validation plots inside the study area, 13 additional validation plots were selected in the pond area 10 km to the northeast, which was still unaffected by erosion and salinization. These served as a reference for terrestrial vegetation diversity in inside the area back in 2005, before the onset of coastal erosion. Field validation of the 2018 GIS vegetation categories showed that the visual characterization of vegetation from the satellite images was accurate, with an overall accuracy of 89% and a kappa-coefficient of 0.81.
Trends in vegetation cover changes over time for planted and natural mangroves were investigated per zone and species with a generalized regression model, using the surface area of the vegetation category of interest as a response variable, assuming a Gaussian distribution for the two mangrove types (planted vs. natural). A logarithmic regression model was used to analyze the decline in terrestrial vegetation. Data from the field campaign in terms of DBH, basal area and stem density were compared between planted and natural mangrove stands with Kruskal-Wallis tests, and the tree density differences between species and stand types was tested with a two-way ANOVA after log-transformation of tree density.
2.4 Q2. Propagule Traps: Can EMR-Hydrology Support Natural Mangrove Recovery in the Pond Zone?
To investigate if natural mangrove recovery in the pond zone could be supported through EMR-hydrology, we studied landwards propagule dispersal throughout the main creek of the pond zone and compared that to propagule abundance in the coastal zone (the propagule source). We did this by trapping floating propagules in modified crab-traps, to compare weekly propagule abundance in both zones. The propagule traps (Supplementary Figure S2A) were designed as netted bamboo cylinders 120 cm long, with a circular 55 cm diameter opening at one end. During deployment, 50% of this opening was always above the water surface, as the traps were maintained horizontally in the water using floats. The floating traps were loosely anchored to an anchoring pole in such a way that the trap could rotate freely around the pole, so that the circular opening was always facing the current.
The traps were placed across the creek and throughout the coastal bay. In total, 40 traps were placed throughout the basin, nine traps in the creek of the pond zone and 31 traps in the coastal zone (Figure 2, close-up in Supplementary Figure S2B) and they were emptied weekly throughout the year in 2017. Data from the wet season are partially missing as the coastal traps were continuously destroyed by waves, despite repeated efforts to replace them. In addition, the rough seas made it hard to reach and empty the remaining traps regularly.
The variability of propagule abundance over time and in space was compared between the pond zone and coastal zone (source zone) using a generalized linear regression model, assuming a negative binomial distribution (package glmmTMB in RStudio version 1.0.143). Propagule counts of the three most abundant species observed in the traps (Avicennia marina, Avicennia alba and Rhizophora mucronata) were used as response variables, using week number and zone of interest (near the source or in the pond area) as explanatory variables. Two other species were sporadically found in the traps, but their numbers were too low for meaningful comparison between the two zones. The effect of week number on the residuals of the model was furthermore tested by fitting a smoothing term using a general additive model (GAM) from R package mgcv to investigate the effects and significance of seasonality (ie. the non-linear effect of “week number”) on propagule counts.
2.5 Q3. EMR-Dams: Can EMR-Dams Induce Natural Mangrove Recovery at Coastal Sites?
We quantified the effect of EMR-dams (i.e. sediment trapping brushwood dams) on natural seedling establishment and survival of established seedlings. To do so, we set up a seedling monitoring study using 2 × 2 m plots at coastal sites above mean sea level (MSL) (Figure 2). To evaluate the wave sheltering effect of the dams, we set up seven monitoring blocks: three blocks that were artificially sheltered by permeable dams constructed the previous season (EMR-dam sites) (Supplementary Figure S3C), three blocks at a naturally sheltered site behind a vegetated chenier (i.e. elevated sand bank with vegetation on top; positive controls) (Supplementary Figure S3B), and one block at an exposed site above MSL (negative control) (Supplementary Figure S3C). We were only able to include one block at an exposed site, due to the limited availability of wave-exposed sites above MSL. Each block consisted of three 2 × 2 m plots which were used to monitor natural mangrove recruitment in terms of number of seedlings per species, seedling survival and new seedling recruitment over 1 year in 2017 (Supplementary Figure S3D).
To investigate the survivability of established mangrove seedlings at these locations, we designed a small-scale planting experiment in which seedlings of two mangrove species were planted in two of the three 2 × 2 m plots per block in the following year (i.e. 2018; Supplementary Figure S3E). One of the 2 × 2 m plots per block was used to plant R. mucronata seedlings (the species most commonly used for mangrove planting in this area) and the other was used to plant A. alba seedlings (the most abundant, naturally expanding mangrove species in the area). The seedlings were planted in a matrix of 5 × 5 individuals per plot, resulting in a planted seedling density of 6.5 m−2. Seedling height and viability was recorded at (t0), and again after half a year and a full year. Differences in survival between the species and between the shelter-types was tested with a Kaplan-Meijer survival test, and differences in average growth rate between species were tested with a t-test.
2.6 Q4. EMR-Dams + Planting: Can Mangrove Planting Combined with EMR-Dams Accelerate Recovery?
To further investigate if and how mangrove planting could accelerate mangrove recovery at sites with EMR-dams, we designed a larger mangrove planting experiment with which we closely monitored the survival and growth of the multiple mangrove species planted at the oldest EMR site in the area (constructed and maintained since 2013, open circle in Figure 2). The planting experiment was set up in 20 plots of 2 × 2 m in which four mangrove species were randomly planted in a matrix of 5 × 4 individuals (Supplementary Figure S4). The four species that were planted all occurred naturally in the vicinity: A. alba, A. marina, R. mucronata and Rhizophora apiculata. Prior to planting, the seedlings were raised in a nursery. A. marina and A. alba were collected as propagules from the water, and R. mucronata and R. apiculata were collected as young wildlings from underneath a variety of mature trees in the region to ensure some genetic heterogeneity. Propagules and wildlings were then raised in a nursery for 2 months, of which 6 weeks in the shade and 2 weeks in full sunlight to acclimatize the seedlings to life in the field.
3 Results
3.1 Q1. GIS Study: Which Mangrove Recovery Process is Dominant per Zone?
3.1.1. Satellite Data
The GIS time series of different types of vegetation cover showed a decline of terrestrial vegetation and an expansion of mangrove vegetation throughout both the coastal and pond zone from 2005 until 2018 (Figure 3). The 64.9 ha of terrestrial vegetation in the pond zone in 2005 decreased logarithmically with e−0.24 ha.y−1 (R2 = 0.9, p < 0.001) between 2005 and 2018, leaving only 2.8 ha of terrestrial vegetation in 2018. Meanwhile, natural mangrove recovery was especially dominant in the coastal zone and showed an average increase of 3.9 ha per year (R2 = 0.85, p < 0.001), increasing from 6.3 ha in 2005 to 46.8 ha in 2018. Mangrove expansion through planting was significantly less rapid in the coastal zone (p < 0.01), with an average expansion rate of 1.3 ha per year (R2 = 0.56, p = 0.03).
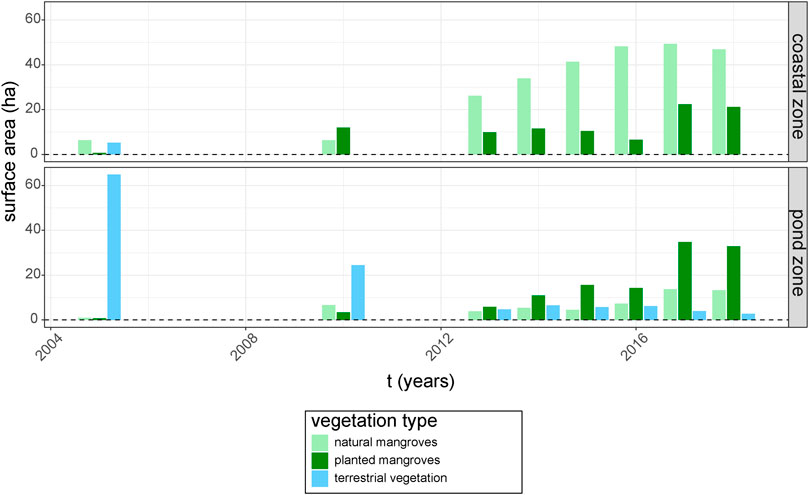
FIGURE 3. Trends in total surface area (ha), digitized from high resolution images available in Google Earth, of the three vegetation types [terrestrial forestation, planted mangroves (R. mucronata) and naturally-recovered mangroves (Avicennia spp.)] in the coastal zone and the pond zone from 2005 until 2018.
In contrast, mangrove expansion in the pond zone could be mostly attributed to planting efforts, with an average increase of 2.5 ha per year (R2 = 0.62, p = 0.01) of planted mangroves as opposed to an average expansion rate of 0.8 ha per year in natural mangrove stands (R2 = 0.53, p = 0.02). This resulted in 32.9 ha of planted mangroves in the pond zone in 2018 (almost exclusively on pond bunds), while natural mangrove cover (inside abandoned ponds) amounted to only 13.3 ha. The relative success of planting in the pond zone can most likely be attributed to the much slower natural mangrove expansion in this area (p < 0.01), as the absolute expansion rates through planting were not significantly faster in the pond zone compared to the coastal zone (p = 0.17).
3.1.2 Field Data
In general, the composition and structure of the mangrove stands classified as “planted” in the GIS study were significantly different from the stands classified as “natural”. Planted mangrove stands showed a significantly different species composition than natural mangrove stands (F = 19.6, df = 2, p < 0.001), with 95% of the planted stands consisting of R. mucronata. In contrast, natural mangrove stands consisted of 92% A. marina, and only 4% R. mucronata (Figure 4). There were no significant differences found between planted stands and natural stands in terms of basal area (m2 ha−1) and tree density (n ha−1) (Supplementary Table S2), although the trees in the natural stands had a significantly larger DBH than the trees in the planted stands (X2 = 10.5, df = 1, p < 0.01). Most trees in the planted stands had a DBH of 3–7 cm, whereas the trees in the natural stands were much more diverse in terms of stem diameter, with many young trees, and progressively fewer older and thicker trees (Figure 4).
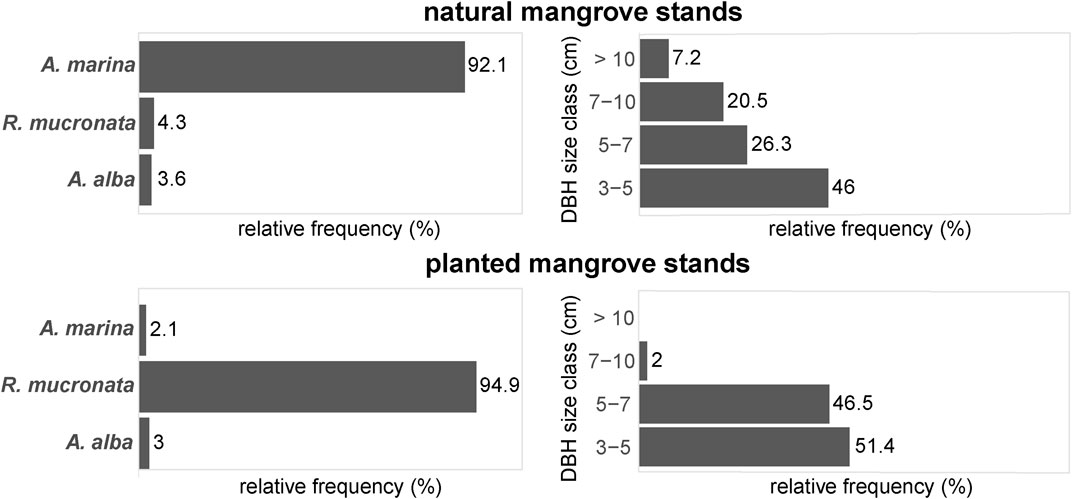
FIGURE 4. Forest structure of natural and planted mangrove stands in field validation plots of the GIS study in terms of relative tree density per species (left) and the relative distribution of trees per DBH size class (right).
The field observations further revealed that the class of declining terrestrial vegetation was composed of fresh to brackish-water village “forest gardens”, used by the villagers for basic fruit and materials provision. Based on a tally of 3.5 thousand trees in remaining forest garden fragments (also including the nearby reference area which was not severely affected by increased salinization), these forests were found to be composed of more than 50 different species of trees, almost all of which had an important utilitarian role in terms of food, forage or materials provision. More details on the composition and uses of these forest gardens as well as their degradation and loss caused by coastal erosion and salinization will be presented elsewhere.
3.2 Q2. Propagule Traps: Can EMR-Hydrology Induce Natural Mangrove Recovery in the Pond Zone?
The propagule traps that were deployed throughout the area proved effective in trapping propagules of multiple mangrove species. Propagules of A. marina, A. alba and R. mucronata were the most abundant (Figure 5), although a few propagules of Rhizophora stylosa and Avicennia officinalis were sporadically found as well between week 32 and 35 (not visible in Figure 5). The relative abundance of propagules in the coastal zone versus the pond zone depended significantly on the time of year (p = 0.01), with propagule counts in the pre-wet season (week 39–42) being 2 to 21 times higher in the pond zone than in the coastal zone (Figure 5). While in the mid-dry season (week 28–31), propagule counts in the pond zone were lower than in the coastal zone. This temporal variation in propagule abundance was caused by the timing of the propagule rains of the two most abundant pioneer species in the area: A. alba and A. marina.
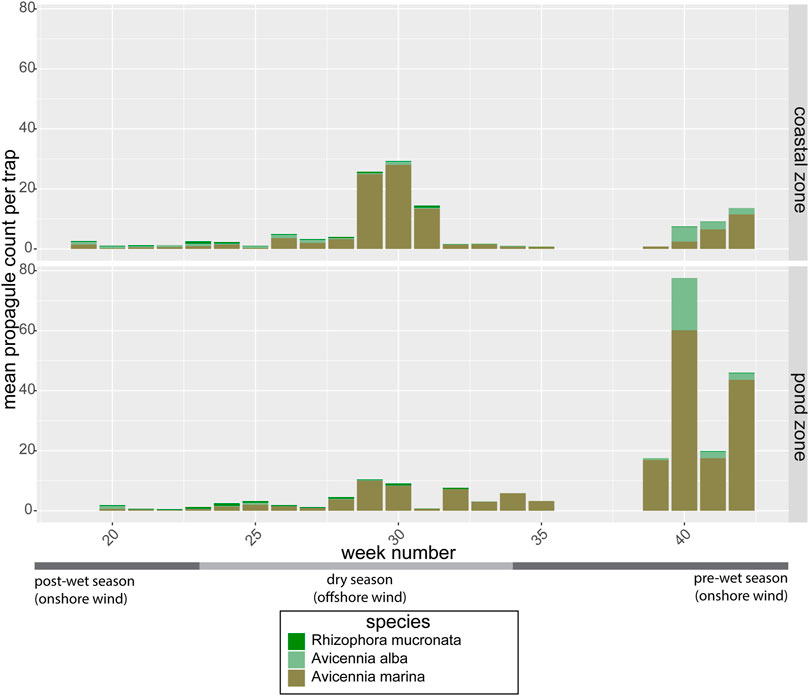
FIGURE 5. Average weekly propagule counts of the three most abundant mangrove species per trap in the coastal zone (propagule source) and in the pond zone. Data from the mid-wet season are missing due to storm damage to the traps.
Both Avicennia species showed a clear temporal signal that explained 9.6 and 3% of the variance in seed abundance of, respectively, A. marina (gam: F = 5.6, edf = 8.2, p < 0.0001) and A. alba (gam: F = 3.9, edf = 2.7, p = 0.007). A. marina showed a clear peak around week 30 (in the middle of the dry season) and an increase in propagule counts from week 40 onward at the start of the wet season. The pre-wet season peak was visible in both the coastal zone and the pond zone, although more pronounced in the pond zone (Figure 5). The mid-dry season peak was only observed in the coastal zone. A. alba only showed a peak before the wet season around week 40 and at the end of the wet season around week 20. This could be caused by one big propagule rain over the whole wet season, but due to missing data over the wet season we cannot be sure of this. R. mucronata did not show a significant seasonal pattern in propagule availability, with propagules present year round, though in low abundance.
3.3 Q3. EMR-Dams: Can EMR-Dams Induce Natural Mangrove Recovery at Coastal Sites?
Monitoring of plots at coastal sites revealed that most sites behind EMR-dams and the exposed sites could not (yet) support natural mangrove recruitment in 2017. Only the naturally sheltered site harbored an abundance of natural seedlings at the start of the experiment, and showed significant new recruitment of natural seedlings over the course of the monitoring campaign (Figure 6A). One of the EMR-sites did have a few seedlings of A. marina that survived over the subsequent wet season (Figure 6A), and it showed the establishment of one new A. marina seedling, suggesting that this particular EMR site had favourable establishment conditions.
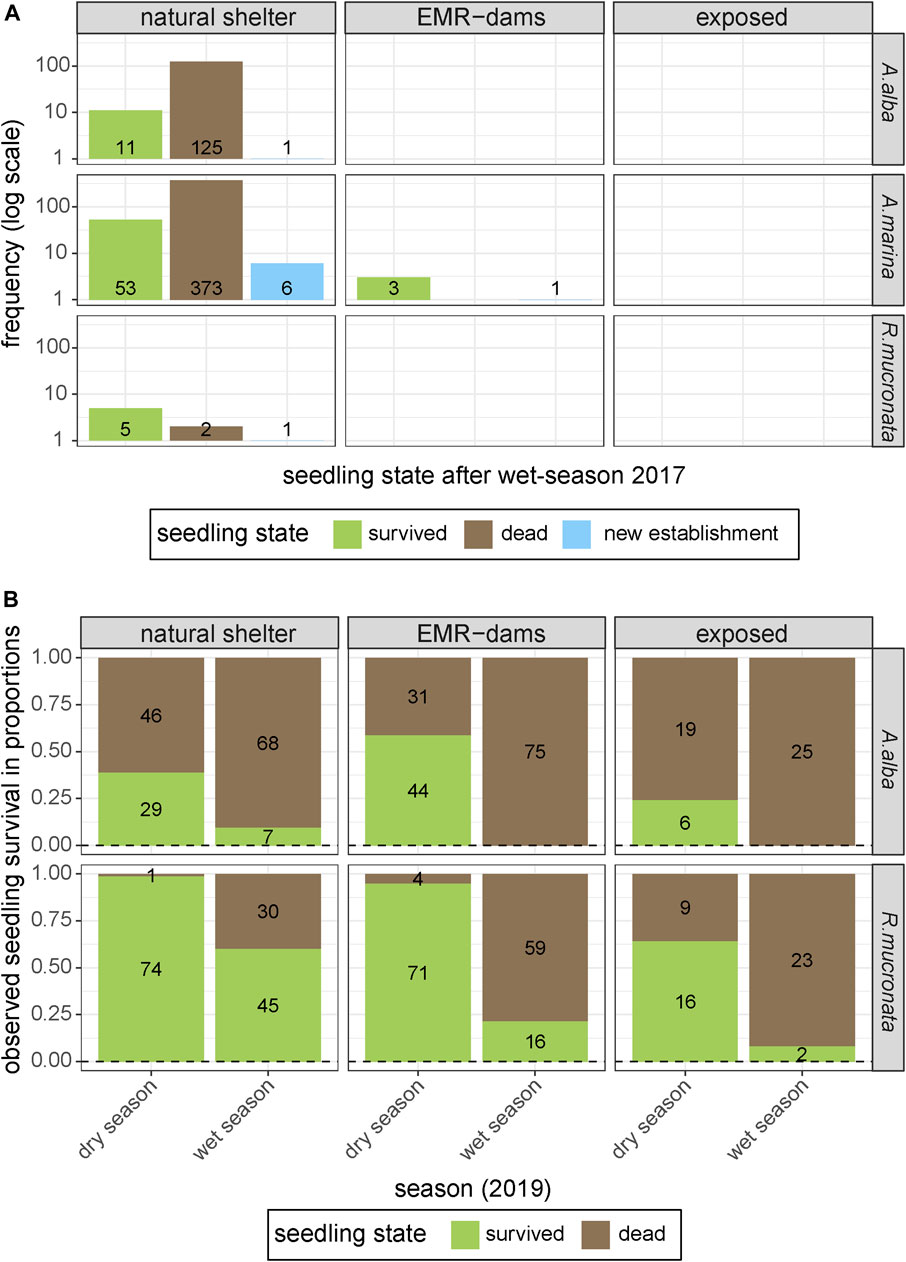
FIGURE 6. (A) Existing and new natural establishment of mangrove seedlings at the three differently sheltered coastal sites above MSL [natural shelter, artificial shelter (EMR), and wave-exposed], and survival of existing natural recruits over the course of the experiment. (B) Survival of A. alba and R. mucronata seedlings that were planted at naturally sheltered sites, at habitat restoration sites behind an EMR-dam, and at an exposed site.
The small-scale experimental planting of R. mucronata and A. alba seedlings in the following year, to quantify the effect of EMR-dams on seedling survival and growth of established seedlings, showed that survival of both species decreased over time (Figure 6B). This is an expected natural process in such a dynamic environment, but there were some clear differences between species. Overall, the relatively large R. mucronata seedlings showed significantly higher survival rates than the smaller A. alba seedlings (Kaplan-Meier, Χ2 = 109, p < 0.0001). However, A. alba grew 61 mm.month−1 faster on average than R. mucronata (p < 0.001). Survival rates at the exposed sites and EMR-dam sites were significantly lower than at the naturally-sheltered sites (Kaplan-Meier, Χ2 = 27.8, p < 0.0001). However, the EMR-dams did increase the survival of R. mucronata compared to the wave-exposed site (binom.test, p < 0.001). Ultimately, the best surviving seedlings were those at the naturally-sheltered site behind a vegetated chenier (Kaplan-Meier, Χ2 = 148, p < 0.0001).
3.4 Q4. EMR-Dams + Planting: Can Mangrove Planting Combined with EMR-Dams Accelerate Recovery?
The four species that were planted above mean sea level at an EMR-dam site, but just below the threshold of natural mangrove recruitment, showed clear differences in survival and growth (Figure 7A). Over the course of 16 months, the survival rate of the R. mucronata seedlings was significantly higher than of the A. alba, A. marina and R. apiculata seedlings (Kaplan Meier, Χ2 = 131, p < 0.0001). At the end of the experiment, only 6% of R. apiculata seedlings had survived, as opposed to 21 and 35% of the A. marina and A. alba seedlings, and 67% of the R. mucronata seedlings (6% > 21% > 35% > 67%, p < 0.001). However, a comparison of the growth rates of the surviving seedlings (Figure 7B) showed that A. alba and A. marina had significantly higher growth rates than R. mucronata and R. apiculata survivors (GLM, p < 0.001), which even resulted in two fruiting A. marina individuals by the end of the experiment.
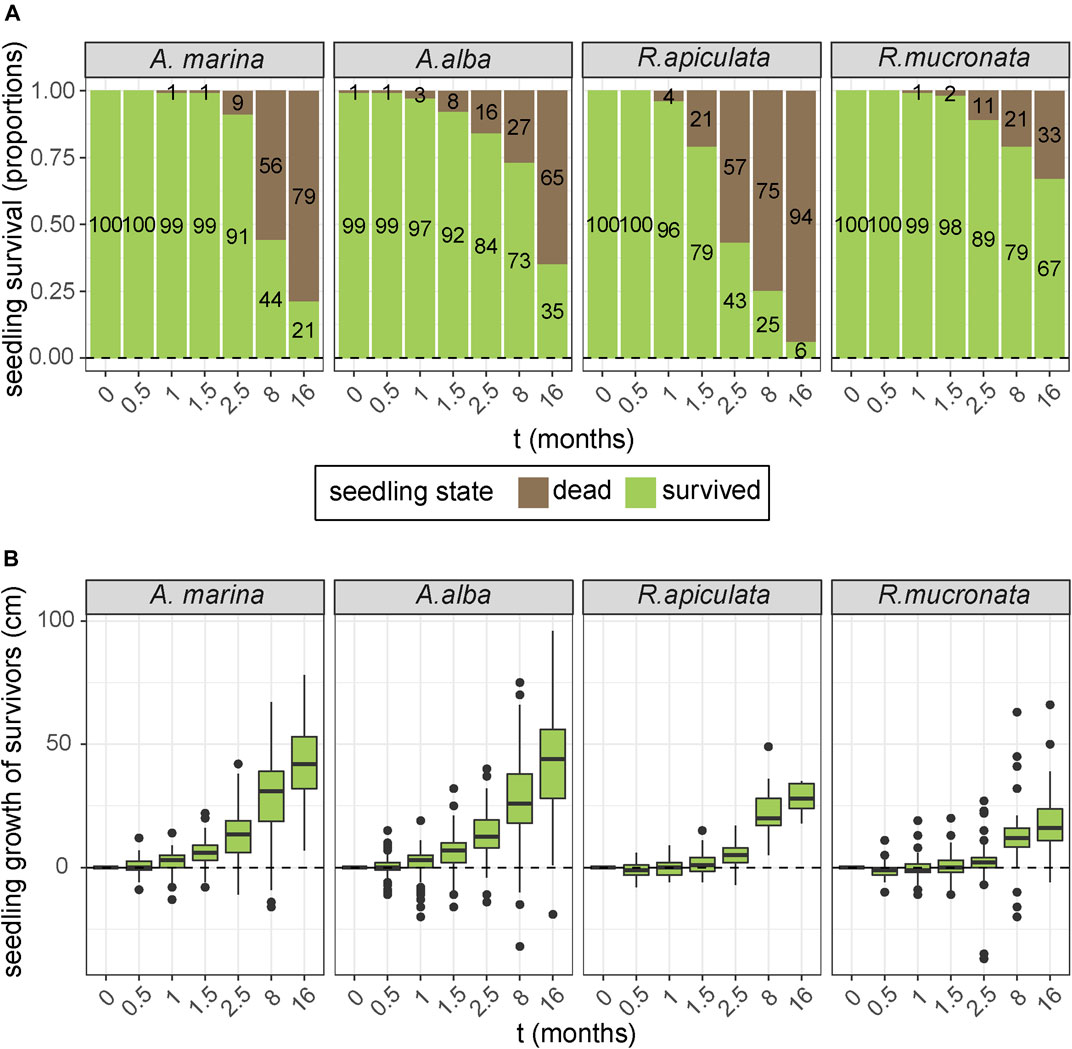
FIGURE 7. (A) Observed survival over time of four different mangrove species that were planted at a sheltered site behind an EMR-dam around mean sea level. (B) Average seedling growth of surviving seedlings from the four planted mangrove species.
4 Discussion
In this study, we aimed to evaluate the potential for- and the effectiveness of EMR and mangrove planting in the pond zone and coastal zone of a site in Northern Java. Our aim was to understand under what conditions planting, EMR or a combination of both can best be applied.
4.1 Mangrove Restoration in the Pond Zone
4.1.1 Q1.a Which Mangrove Recovery Process is Dominant in the Pond Zone?
In the pond zone, GIS information showed that disappearing terrestrial trees were partly substituted by salt tolerant mangroves over time, mostly as a result of planting on pond bunds. The spatial continuity of these planted stands was therefore fragmented, with a very limited patch size (max 5 m wide cross-shore and 200 m long long-shore) of only a single species. Small forest patches are known to be vulnerable to disturbance and likely have little value in terms of coastal protection (Koch et al., 2009) or biodiversity (Hanski, 2015). However, when viewed as fragments to the larger forest, these smaller patches may provide a propagule source for further forest expansion, although natural expansion of Rhizophora species was neither observed on satellite images nor in the field, suggesting that there was little suitable natural habitat for this particular species. Nevertheless, the planted stands may account for some ecological connectivity and structural heterogeneity of the larger system (Fahrig, 2017). In addition, the planted patches might still be useful for shade, timber harvest (Van Oudenhoven et al., 2015), mitigation of some of the CO2 release from the pond bunds (Sidik and Lovelock, 2013), and some limited fish pond water-quality regulation (Rönnbäck and Primavera, 2000). However, decomposing leaves may also worsen the water quality (Bosma et al., 2020). Finally, planted mangrove stands are valued by the local communities for their aesthetic value in a lowland previously devoid of tree-like vegetation (pers. comm. Local communities). While mangrove planting on banks of active ponds may have some advantages, planting mangroves inside disused ponds without EMR measures is reportedly a poor practice, either resulting in slow growth (Proisy et al., 2018) or mortality of planted seedlings (Brown et al., 2014). Also, in the case that natural colonizers can reach the site, additional planting often hampers the growth and succession of natural colonizers (Matsui et al., 2010; Proisy et al., 2018). Therefore, we conclude that planting may be a valuable measure in the pond zone but only when applied on pond bunds when aquaculture ponds are still active, bearing in mind that this will not result in a fully functional mangrove forest (Figure 8).
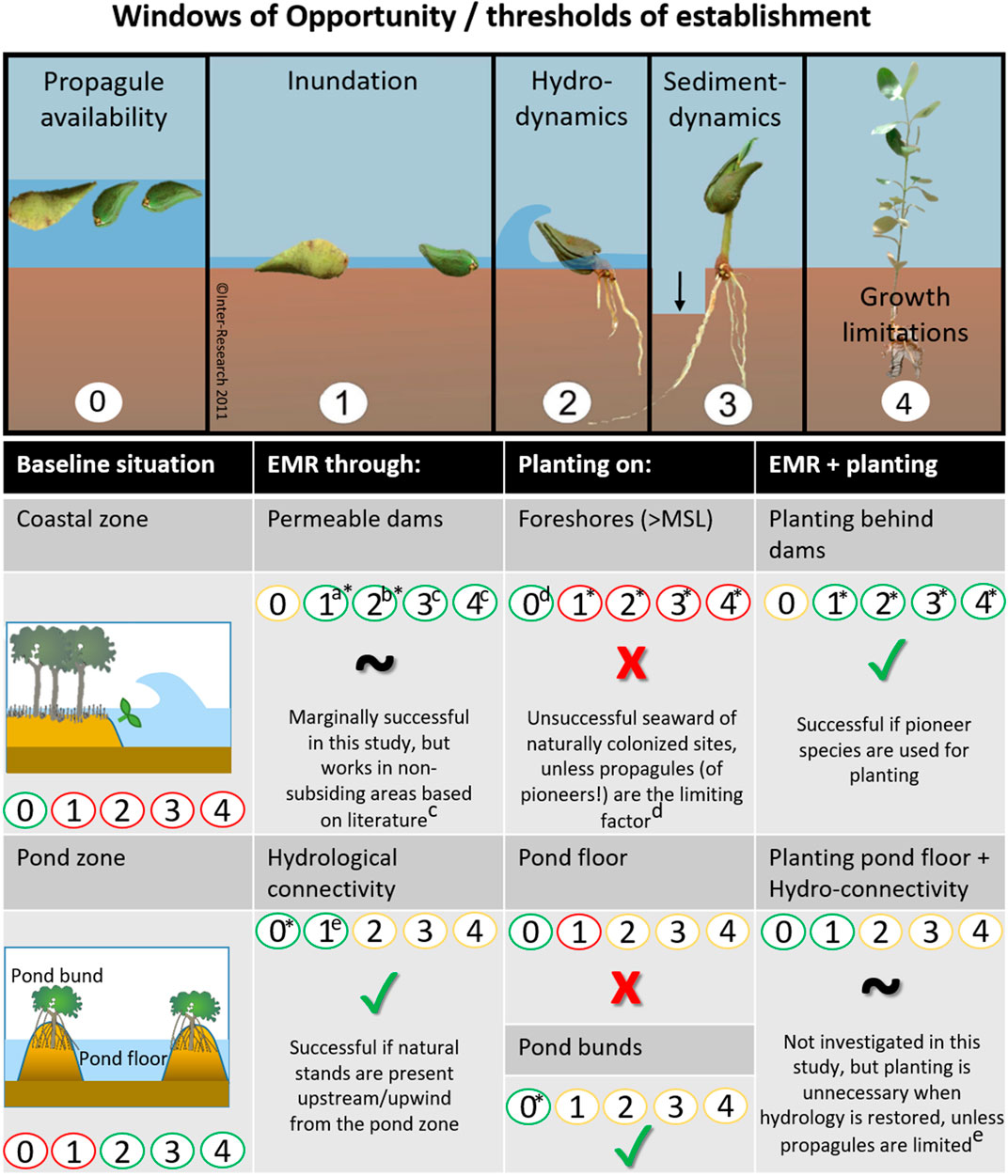
FIGURE 8. Summary of the effectiveness of different restoration methods (EMR, planting, and a combination of both) to overcome thresholds of establishment in the coastal zone and the pond zone (red: ineffective, green: effective, yellow: condition already met in baseline situation), based on the findings of this study (*) and literature [a: (Cado et al., 2021), b: (Winterwerp et al., 2020), c: (Van Cuong et al., 2015), d: (Saenger and Siddiqi, 1993), e: e.g. (Lewis, 2005; Matsui et al., 2010; Proisy et al., 2018; van Bijsterveldt et al., 2020)]. The top panel of this figure was expanded from Balke et al. (2011)’s original Windows of Opportunity figure (panel 1-3).
4.1.2 Q2. Can EMR-Hydrology Support Natural Mangrove Recovery in the Pond Zone?
Besides planting on pond bunds, there is also potential for natural mangrove recovery inside aquaculture ponds. That hydrological EMR measures can be effective in ponds has been demonstrated in several studies on the effect of strategic pond breaching in disused or degraded ponds (e.g. Brown et al., 2014; Matsui et al., 2010). In such areas, natural recruitment often fails, either because propagules do not reach the targeted aquaculture pond (Di Nitto et al., 2013), or because the sediment condition of the pond floor is not yet favorable for seedling survival. This was for instance the case in degraded ponds in North Sulawesi, where six planting attempts over the course of 9 years all resulted in total seedling mortality. It was not until man-made drainage channels were filled-in and pond bunds were breached in strategic directions that the sediment condition improved (Brown et al., 2014). These measures subsequently resulted in the recruitment of 32 mangrove species, with overall seedling densities up to 20.000 ha−1. In Demak, disused aquaculture ponds are generally abandoned because pond bunds can no longer be maintained under the rising water levels. Along the coast, these ponds typically fill up with sediment quickly and mangrove recruitment follows not long after (van Bijsterveldt et al., 2020). Recruitment in abandoned ponds therefore does not seem to be an issue in this area. However, our findings show that propagules can travel further inland than where the current natural recruitment occurs based on the satellite data. In addition, despite the lack of mid-wet season data, it is clear that the major propagule release peak of the two pioneer species (i.e. A. marina and A. alba) co-occurs (at least partly) with the NW monsoon. Wind is known to be an important factor in propagule transport (Di Nitto et al., 2013; Van der Stocken et al., 2015, 2013). Therefore, the onshore winds during the wet season most likely propelled large quantities of propagules further inland, explaining the relative larger abundance of propagules in the pond zone compared to the coastal zone during that season. These observations suggest that there is additional unutilized potential for EMR measures in the active aquaculture zone (Figure 8). For instance, season optimized sluice gate management could ensure that sufficient propagules enter target sites in the pond zone, if seasonality of propagule release and wind direction are taken into account. Additional measures, such as pond partitioning and localized pond-floor raising (Bosma et al., 2020), would of course be necessary to create habitat for the propagules to land, and eventually realize mixed-mangrove aquaculture or greenbelt-rimmed ponds along rivers and creeks.
4.2 Mangrove Restoration in the Coastal Zone
4.2.1 Q1.b Which Mangrove Recovery Process is Dominant in the Coastal Zone?
Our GIS study revealed that mangrove expansion in the coastal zone could be attributed mostly to dispersion of pioneer species, and only for a small extent to planting efforts (Figure 3). We know from frequent field visits that planting efforts in the coastal zone of Demak have been initiated by various organizations on a yearly or two-yearly basis. In most cases, this involved large-scale planting on exposed, partly low-lying coastal mudflats (e.g. Figure 9, white polygon), or at sites that were already colonized by pioneers (e.g. Figure 9 2013, 2015, behind breakwater). The use of natural recruits as an indicator for a sites’ suitability for mangrove planting in the coastal zone is a common practice in community-based restoration efforts (Wodehouse and Rayment, 2019), but has also been reported to be unnecessary 70% of the time, as mangroves are already colonizing those sites naturally (Wodehouse and Rayment, 2019). In the case of Demak’s breakwater, the practice has led to a mangrove stand of extremely dense and stunted R. mucronata trees, with a few surviving A. alba trees that rise head and shoulders above the rest (Figure 9, top, 2017 and 2018). This illustrates that planting (especially of non-pioneer species) at already newly-colonized sites, is a poor practice.
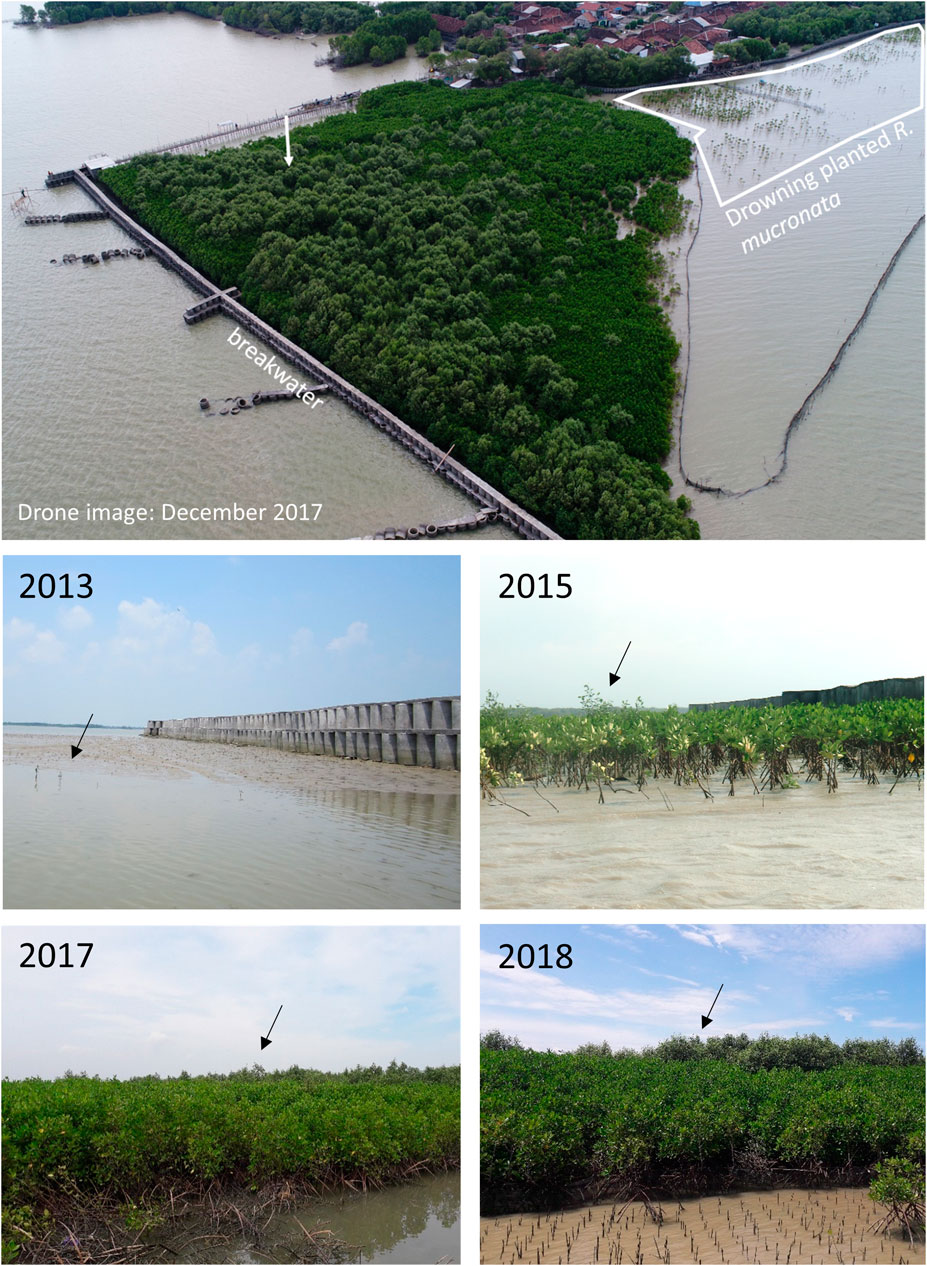
FIGURE 9. Top: Bird’s-eye view of the breakwater seaward of Wonerejo, Demak in 2017 (drone image courtesy of CoREM-UNDIP). The white arrow indicates the direction of the picture time series. The time series show the development of a mudflat after construction of the breakwater in 2012, followed by natural colonization in 2013 and subsequent planting of R. mucronata in 2014. Note the relative height difference in all pictures of the naturally colonized A. alba trees (black arrows) versus the surrounding planted R. mucronata trees (2015–2018).
Most of the large-scale plantations that were done at tidal levels below the threshold of natural recruitment in Demak disappeared before our field visit in a following year. In the literature, there have been examples of successful planting on unvegetated foreshores in the coastal zone (Saenger and Siddiqi, 1993; Uddin and Hossain, 2013). For example, a series of large-scale afforestation projects on newly accreted foreshores in Bangladesh successfully planted an area of 120,000 ha with several mangrove species in the 1980s, including pioneer species such as Sonneratia apetela and Avicennia officinalis (Saenger and Siddiqi, 1993). The elevated foreshores on which the mangroves were planted were subject to complete desiccation during dry seasons and would normally have been colonized by salt tolerant grasses. One reason for the reported high survival rates of 52% might therefore be that the supply of propagules from pioneer species was the limiting factor for natural mangrove expansion in these projects. These examples suggest that planting, as the sole restoration method, in the coastal zone is only useful if it is done to reintroduce species that are moreover suitable to colonize the site (Figure 8). In Demak, natural mangrove cover showed a linear increase on the satellite images, indicating that propagule limitation of pioneer species was not an issue. However, the cover of natural mangroves seemed to stabilize in recent years (Figure 3), suggesting that most of the suitable mangrove habitat has now been occupied.
4.2.2 Q3. EMR-Dams: Can EMR-Dams Induce Natural Mangrove Recovery at Coastal Sites?
The aimed-for effect of the EMR-dams in our study area was to expand the existing mangrove habitat by means of wave attenuation, so that the resulting sediment deposition would raise the bed level above the threshold for natural colonization. This method has been effective along several eroding mangrove mud-coasts around the globe (Winterwerp et al., 2020; Figure 8). However, along the subsiding coastline of Demak, EMR with the use of permeable structures did not result in large-scale mangrove establishment over the years that the plots were monitored, even though a few seedlings were able to colonize the EMR-sites. It is possible that seedling establishment did occur at a larger scale at the EMR sites, but that the seedlings had disappeared by the time we assessed the plots. This would be in accordance with the findings of Cado et al. (2021), who found that there was abundant seedling establishment behind the dams, but that these seedlings did not persist longer than a few months and did not grow into mature mangroves. This rapid mortality of seedlings could reportedly be explained by a sequence of two processes: 1) the permeable dams do elevate the bed level of the targeted coastal sites, especially during the wet season (Winterwerp et al., 2020), but 2) the whole area is subsequently subject to rapid land subsidence (Kuehn et al., 2009). Hence, the freshly accumulated sediment might initially surpass the elevation threshold for seedling establishment during the wet season, but later sink below the threshold of survival, thus killing the young mangrove seedlings. EMR-dams as a restoration method alone therefore appear not to be enough to support mangrove recovery in this severely subsiding coastal area (Figure 8). For mangrove recovery to be successful in Demak, subsidence urgently needs to be addressed, especially by halting ground water extraction (Nicholls et al., 2021).
4.2.3 Q4. EMR-Dams + Planting: Can Mangrove Planting Combined with EMR-Dams Accelerate Recovery?
Despite the low success in natural recruitment at the EMR sites, EMR did increase the survival of established (planted) seedlings (Figure 6B), even at sites where natural recruitment behind the dams was not yet taking place (Figure 7A). This suggests that a combination of planting and EMR might be useful to overcome (temporary) thresholds of establishment and accelerate revegetation of challenging sites in the coastal zone. However, species choice appears an important factor in the development of the revegetated site after initial recruitment. R. mucronata, the species that is most often used in large-scale planting (Wodehouse and Rayment, 2019), proved to have the highest initial survival rate (Figure 7A), which might explain the popularity of these species in many voluntary planting projects (Primavera and Esteban, 2008; Wodehouse and Rayment, 2019). However, the slow growth rate of R. mucronata, such as observed at this muddy coast (Figure 7B), might lead to a mangrove stand that is, more stunted and/or less regenerative than when a pioneer species appropriate to this site would have been used. This outcome has indeed been observed in various studies (e.g. Barnuevo et al., 2017; Fickert, 2020; Proisy et al., 2018), as well as behind the breakwater in Demak (Figure 9). It should be noted that in carbonate systems, Rhizophora species have been observed as pioneers of the coastal zone [e.g. (McKee, 1993; Piou et al., 2006; Prabakaran et al., 2021)]. This illustrates that it is important to look at natural example sites with a similar biophysical typology as the restoration site [e.g. minerogenic versus organogenic soil (Worthington et al., 2020)], when selecting an appropriate pioneer species. The presence of a few fruiting pioneers can reportedly revegetate a site more efficiently than a planting effort with better surviving, but slower growing species (Fickert, 2020). Although we were not able to include formerly native pioneers such as Sonneratia alba and Aegialitis annulata (Balun, 2011; Ilman et al., 2016), our Q4 experiment indeed demonstrated that planting with pioneers can quickly lead to the presence of a few reproductive young trees. This illustrates that the trade-off between a species’ survival and growth rate should be taken into consideration when choosing species to plant in combination with EMR-measures, preferably using pioneer species at newly accreted sites (Figure 8).
5 Conclusions and Management Implications
Although the coastline of Demak is a rather extreme case of erosion and land-subsidence, this study does give some useful insights regarding when and how planting and EMR are likely (un)successful. Through a combination of GIS, propagule monitoring, and planting experiments, our results show that:
1) Even in areas with mainly active aquaculture, there is potential for ecological mangrove restoration in ponds lining creeks and rivers, as long as there is a propagule source upstream/upwind. EMR measures in active ponds along rivers could include sluice gate management during the most optimal season (in terms of wind and propagule availability), in combination with partitioning of the pond by adding an inner dike to the river-side of the pond and allowing that part to fill up with sediment so that pioneers can colonize (i. e. greenbelt ponds). Planting may be done on pond bunds or at locations where propagule supply of the target species is limited.
2) Seaward mangrove expansion through planting without additional EMR measures to restore mangrove habitat is likely to be unsuccessful (at low-lying sites) or unnecessary (at proper elevations with existing seedlings), unless species need to be (re)introduced. EMR measures to restore mangrove habitat through sediment trapping along eroding mud-coasts could include placement of permeable (brushwood) dams. Along subsiding coasts, these dams may however not be enough to maintain the bed level above the threshold for mangrove establishment. However, mangrove planting with fast growing pioneer species in combination with sediment trapping by EMR-dams could accelerate mangrove recovery at such sites.
Data Availability Statement
Data in support of this article are available at: https://doi.org/10.4121/17927198.
Author Contributions
CB: writing-original draft, experimental design and execution, data analysis, student supervision. AD: experimental design and execution, funding acquisition, field data collection, review, conceptualization, supervision. TB: experimental design, funding acquisition, review, supervision. MM: Resources, Investigation (field data collection), writing-review. RP: Resources, Investigation (field data collection), writing-review. JS: Investigation (GIS data collection), Data Curation, writing-review. FT: Resources, Validation, conceptualization, writing-review. BW: Conceptualization, experimental design, Writing-review, Supervision.
Funding
This work is part of the BioManCo project with project number 14753, which is (partly) financed by NWO Domain Applied and Engineering Sciences, and Engineering Sciences, and co-financed by Boskalis Dredging and Marine experts, Van Oord Dredging and Marine Contractors bv, Deltares, Witteveen + Bos and Wetlands International. The Netherlands Sustainable Water Fund provided partial funding for this work through project number FDW14R14. Co-funding to cover the participation by WMR staff was provided by the Netherlands Ministry of Agriculture, Nature and Food Quality through grant KB 36 #4318300114 and through WMR project # 4311500013.
Conflict of Interest
The authors declare that the research was conducted in the absence of any commercial or financial relationships that could be construed as a potential conflict of interest.
Publisher’s Note
All claims expressed in this article are solely those of the authors and do not necessarily represent those of their affiliated organizations, or those of the publisher, the editors and the reviewers. Any product that may be evaluated in this article, or claim that may be made by its manufacturer, is not guaranteed or endorsed by the publisher.
Acknowledgments
This work is part of the BioManCo project with project number 14753, which is (partly) financed by NWO Domain Applied and Engineering Sciences, and Engineering Sciences, and co-financed by Boskalis Dredging and Marine experts, Van Oord Dredging and Marine Contractors bv, Deltares, Witteveen + Bos and Wetlands International. The BioManCO project is a collaboration between TU Delft, NIOZ and UNDIP and makes use of the framework set up by Building with Nature Indonesia, a program by Ecoshape, Wetlands International, the Indonesian Ministry of Marine Affairs and Fisheries (MMAF), the Indonesian Ministry of Public Works and Housing (PU) and other partners. Co-funding to cover the participation by WMR staff was provided by the Netherlands Ministry of Agriculture, Nature and Food Quality through grant KB 36 #4318300114 and through WMR project # 4311500013. We thank the WMR internship students Ben ten Oever, Inge Kok, Olav Dobber, Jan Burger, Calvin Damen and Joop Bonnet as well as the UNDIP students Hatta Adi Failusuf, Vita Fitriana Mayasari, Wiwid Andriyani Lestariningsih, Marlia Ulfa and Eldita Amalia for contributing to propagule monitoring and the various planting experiments. WMR further generously provided internship and lodging support for these students. Pak Slamet and Ibu Paini are thanked for providing a place to stay and for their all-around hospitality during the fieldwork periods and Pak Muis’ skills as a translator are greatly appreciated. Pak Yus Rusila Noor of Wetlands International, Bogor, is thanked for providing many pieces of missing local information. The local Wetlands field team, led by Pak Eko Has is thanked for logistical support and advice, and Apri Susanto Astra, the Wetlands Coastal Safety Manager is thanked for setting up initial contacts and arrangements for all this work. Jenny Cremer, Jan-Tjalling van der Wal of WMR contributed importantly to the GIS mangrove mapping work.
Supplementary Material
The Supplementary Material for this article can be found online at: https://www.frontiersin.org/articles/10.3389/fenvs.2021.690011/full#supplementary-material
References
Alongi, D. M. (2008). Mangrove Forests: Resilience, protection from Tsunamis, and Responses to Global Climate Change. Estuarine, Coastal Shelf Sci. 76, 1–13. doi:10.1016/j.ecss.2007.08.024
Balke, T., Bouma, T., Horstman, E., Webb, E., Erftemeijer, P., and Herman, P. (2011). Windows of Opportunity: Thresholds to Mangrove Seedling Establishment on Tidal Flats. Mar. Ecol. Prog. Ser. 440, 1–9. doi:10.3354/meps09364
Balke, T., Bouma, T. J., Herman, P. M. J., Horstman, E. M., Sudtongkong, C., and Webb, E. L. (2013). Cross-shore Gradients of Physical Disturbance in Mangroves: Implications for Seedling Establishment. Biogeosciences 10, 5411–5419. doi:10.5194/bg-10-5411-2013
Balke, T., and Friess, D. A. (2016). Geomorphic Knowledge for Mangrove Restoration: A Pan-Tropical Categorization. Earth Surf. Process. Landforms 41, 231–239. doi:10.1002/esp.3841
Balke, T., Swales, A., Lovelock, C. E., Herman, P. M. J., and Bouma, T. J. (2015). Limits to Seaward Expansion of Mangroves: Translating Physical Disturbance Mechanisms into Seedling Survival Gradients. J. Exp. Mar. Biol. Ecol. 467, 16–25. doi:10.1016/j.jembe.2015.02.015
Balun, L. (2011). Functional Diversity in the Hyper-Diverse Mangrove Communities in Papua New Guinea. [Doctoral Diss. Knoxville: Univ. Tennessee.
Barnuevo, A., Asaeda, T., Sanjaya, K., Kanesaka, Y., and Fortes, M. (2017). Drawbacks of Mangrove Rehabilitation Schemes: Lessons Learned from the Large-Scale Mangrove Plantations. Estuarine, Coastal Shelf Sci. 198, 432–437. doi:10.1016/j.ecss.2017.02.015
Bosma, R. H., Debrot, A. O., Rejeki, S., Tonneijck, F., Yuniati, A. W., and Sihombing, W. (2020). Technical Guidelines #4: Associated Mangrove Aquaculture Farms; Building with Nature to Restore Eroding Tropical Muddy Coasts. Ecoshape, Dordrecht, Netherlands.
Brown, B., Fadillah, R., Nurdin, Y., Soulsby, I., and Ahmad, R. (2014). Community Based Ecological Mangrove Rehabilitation (CBEMR) in Indonesia. S.a.p.i.en.s 7, 1–13.
Cado, A., van Rees, F., de Lucas Pardo, M., and van Wesenbeeck, B. (2021). Ecological Mangrove Restoration with Permeable Structures: Monitoring Report. Delft: Deltares.
Castilla, G., Hay, G. G., and Ruiz-Gallardo, J. R. (2008). Size-constrained Region Merging (SCRM). Photogramm. Eng. Remote Sensing 74, 409–419. doi:10.14358/PERS.74.4.409
Chinnasamy, P., and Parikh, A. (2020). Remote Sensing-Based Assessment of Coastal Regulation Zones in India: a Case Study of Mumbai, India. Environ. Dev. Sustain. 23, 7931–7950. doi:10.1007/S10668-020-00955-Z
Di Nitto, D., Erftemeijer, P. L. A., Van Beek, J. K. L., Dahdouh-Guebas, F., Higazi, L., Quisthoudt, K., et al. (2013). Modelling Drivers of Mangrove Propagule Dispersal and Restoration of Abandoned Shrimp Farms. Biogeosciences 10, 5095–5113. doi:10.5194/bg-10-5095-2013
Ellison, A. M. (2000). Mangrove Restoration: Do We Know Enough. Restoration Ecol. 8, 219–229. doi:10.1046/j.1526-100x.2000.80033.x
Erftemeijer, P. L. A., and Lewis, R. R. (1999). “Planting Mangroves on Intertidal Mudflats-Habitat Restoration or Habitat Conversion,” in Ecotone, VIIIth Seminar, Enhancing Coastal Ecosystem Restoration for the 21st Century (Ranong and Phuket, FAO, Rome), 1–13.
Fahrig, L. (2017). Ecological responses to habitat fragmentation per se. Ann. Rev. Ecol. Evolut. Syst. 48, 1–23. doi:10.1146/annurev-ecolsys-110316-022612
Fickert, T. (2020). To Plant or Not to Plant, that Is the Question: Reforestation vs. Natural Regeneration of hurricane-disturbed Mangrove Forests in Guanaja (Honduras). Forests 11, 1068–1117. doi:10.3390/f11101068
Hallegatte, S., Green, C., Nicholls, R. J., and Corfee-Morlot, J. (2013). Future Flood Losses in Major Coastal Cities. Nat. Clim Change 3, 802–806. doi:10.1038/nclimate1979
Hanski, I. (2015). Habitat Fragmentation and Species Richness. J. Biogeogr. 42, 989–993. doi:10.1111/jbi.12478
Ilman, M., Dargusch, P., Dart, P., and Onrizal, (2016). A Historical Analysis of the Drivers of Loss and Degradation of Indonesia's Mangroves. Land use policy 54, 448–459. doi:10.1016/j.landusepol.2016.03.010
IPCC (2019). IPCC Special Report on the Ocean and Cryosphere in a Changing Climate, United Nations, New York.
Kirwan, M. L., Temmerman, S., Skeehan, E. E., Guntenspergen, G. R., and Fagherazzi, S. (2016). Overestimation of Marsh Vulnerability to Sea Level Rise. Nat. Clim Change 6, 253–260. doi:10.1038/nclimate2909
Koch, E. W., Barbier, E. B., Silliman, B. R., Reed, D. J., Perillo, G. M., Hacker, S. D., et al. (2009). Non-linearity in Ecosystem Services: Temporal and Spatial Variability in Coastal protection. Front. Ecol. Environ. 7, 29–37. doi:10.1890/080126
Kuehn, F., Hoffmann-rothe, A., Albiol, D., Cooksley, G., Duro, J., Granda, J., et al. (2009). “Detection of Land Subsidence in Semarang/Indonesia Using Persistent Scatterer Interferometry,” in Asian Association of Remote Sensing Proceedings, 6.
Lewis, R. R., and Brown, B. (2014). Ecological Mangrove Rehabilitation-A Field Manual for Practitioners. This manual is available at: www.mangroverestoration.com.www.rcl.or.id.
Lewis, R. R. (2005). Ecological Engineering for Successful Management and Restoration of Mangrove Forests. Ecol. Eng. 24, 403–418. doi:10.1016/j.ecoleng.2004.10.003
Matsui, N., Suekuni, J., Nogami, M., Havanond, S., and Salikul, P. (2010). Mangrove Rehabilitation Dynamics and Soil Organic Carbon Changes as a Result of Full Hydraulic Restoration and Re-grading of a Previously Intensively Managed Shrimp Pond. Wetlands Ecol. Manage. 18, 233–242. doi:10.1007/s11273-009-9162-6
McIvor, A. L., Spencer, T., Möller, I., and Spalding, M. (2012). Storm Surge Reduction by Mangroves. Natural Coastal Protection Series: Report 2. Cambridge Coastal Research Unit Working Paper 41, Cambridge, UK.
McKee, K. L. (1993). Soil Physicochemical Patterns and Mangrove Species Distribution--Reciprocal Effects. J. Ecol. 81, 477–487. doi:10.2307/2261526
Mmaf, (2012). Oceanography Condition in Coastal of Sayung Sub-district, District of Demak. Province of Central Java.
Möller, I., Kudella, M., Rupprecht, F., Spencer, T., Paul, M., Van Wesenbeeck, B. K., et al. (2014). Wave Attenuation over Coastal Salt Marshes under Storm Surge Conditions. Nat. Geosci 7, 727–731. doi:10.1038/NGEO2251
Montgomery, J. M., Bryan, K. R., Mullarney, J. C., and Horstman, E. M. (2019). Attenuation of Storm Surges by Coastal Mangroves. Geophys. Res. Lett. 46, 2680–2689. doi:10.1029/2018GL081636
Narayan, S., Beck, M. W., Reguero, B. G., Losada, I. J., Van Wesenbeeck, B., Pontee, N., et al. (2016). The Effectiveness, Costs and Coastal protection Benefits of Natural and Nature-Based Defences. PLoS One 11, e0154735–17. doi:10.1371/journal.pone.0154735
Nicholls, R. J., Lincke, D., Hinkel, J., Brown, S., Vafeidis, A. T., Meyssignac, B., et al. (2021). A Global Analysis of Subsidence, Relative Sea-Level Change and Coastal Flood Exposure. Nat. Clim. Chang. 11, 338–342. doi:10.1038/s41558-021-00993-z
Othman, M. A. (1994). Value of Mangroves in Coastal protection. Hydrobiologia 285, 277–282. doi:10.1007/BF00005674
Piou, C., Feller, I. C., Berger, U., and Chi, F. (2006). Zonation Patterns of Belizean Offshore Mangrove Forests 41 Years after a Catastrophic Hurricane1. Biotropica 38, 365–374. doi:10.1111/j.1744-7429.2006.00156.x
Prabakaran, N., Bayyana, S., Vetter, K., and Reuter, H. (2021). Mangrove Recovery in the Nicobar Archipelago after the 2004 Tsunami and Coastal Subsidence. Reg. Environ. Change 21. doi:10.1007/s10113-021-01811-0
Pranchai, A., Jenke, M., Vogt, J., Grueters, U., Yue, L., Mehlig, U., et al. (2018). Density-dependent Shift from Facilitation to Competition in a dwarf Avicennia Germinans forest. Wetlands Ecol. Manage. 26, 139–150. doi:10.1007/s11273-017-9561-z
Primavera, J. H., and Esteban, J. M. A. (2008). A Review of Mangrove Rehabilitation in the Philippines: Successes, Failures and Future Prospects. Wetlands Ecol. Manage. 16, 345–358. doi:10.1007/s11273-008-9101-y
Proisy, C., Viennois, G., Sidik, F., Andayani, A., Enright, J. A., Guitet, S., et al. (2018). Monitoring Mangrove Forests after Aquaculture Abandonment Using Time Series of Very High Spatial Resolution Satellite Images: A Case Study from the Perancak Estuary, Bali, Indonesia. Mar. Pollut. Bull. 131, 61–71. doi:10.1016/j.marpolbul.2017.05.056
Rönnbäck, P., and Primavera, J. H. (2000). Illuminating the Need for Ecological Knowledge in Economic Valuation of Mangroves under Different Management Regimes - A Critique. Ecol. Econ. 35, 135–141. doi:10.1016/S0921-8009(00)00208-1
Saenger, P., and Siddiqi, N. A. (1993). Land from the Sea: The Mangrove Afforestation Program of Bangladesh. Ocean Coastal Manag. 20, 23–39. doi:10.1016/0964-5691(93)90011-M
Schoonees, T., Gijón Mancheño, A., Scheres, B., Bouma, T. J., Silva, R., Schlurmann, T., et al. (2019). Hard Structures for Coastal Protection, towards Greener Designs. Estuaries and Coasts 42, 1709–1729. doi:10.1007/s12237-019-00551-z
Sidik, F., and Lovelock, C. E. (2013). CO2 Efflux from Shrimp Ponds in Indonesia. PLoS One 8, e66329. doi:10.1371/journal.pone.0066329
Stark, J., Van Oyen, T., Meire, P., and Temmerman, S. (2015). Observations of Tidal and Storm Surge Attenuation in a Large Tidal Marsh. Limnol. Oceanogr. 60, 1371–1381. doi:10.1002/lno.10104
Suryadi, Y., Nugroho Sugianto, D., and Hadiyanto, H. (2018). Climate Change in Indonesia (Case Study : Medan, Palembang, Semarang). E3s Web Conf. 31, 09017–09018. doi:10.1051/e3sconf/20183109017
Sutton-Grier, A. E., Wowk, K., and Bamford, H. (2015). Future of Our Coasts: The Potential for Natural and Hybrid Infrastructure to Enhance the Resilience of Our Coastal Communities, Economies and Ecosystems. Environ. Sci. Pol. 51, 137–148. doi:10.1016/j.envsci.2015.04.006
Temmerman, S., Meire, P., Bouma, T. J., Herman, P. M. J., Ysebaert, T., and De Vriend, H. J. (2013). Ecosystem-based Coastal Defence in the Face of Global Change. Nature 504, 79–83. doi:10.1038/nature12859
Tonneijck, F., Winterwerp, H., Weesenbeeck, B. van., Bosma, R., Debrot, D., Noor, Y. R., et al. (2015). Building with Nature Indonesia Securing Eroding Delta Coastlines: Design and Engineering Plan. Ecoshape, Dordrecht, Netherlands.
Uddin, M. M., and Hossain, M. K. (2013). Status and Protective Role of Mangrove Plantations : a Case Study of Mirsharai Coastal Forest , Bangladesh. Int. J. Agric. Sci. Bioresour. Eng. Res. 2, 47–59.
van Bijsterveldt, C. E. J., van Wesenbeeck, B. K., van der Wal, D., Afiati, N., Pribadi, R., Brown, B., et al. (2020). How to Restore Mangroves for greenbelt Creation along Eroding Coasts with Abandoned Aquaculture Ponds. Estuarine, Coastal Shelf Sci. 235, 106576. doi:10.1016/j.ecss.2019.106576
Van Cuong, C., Brown, S., To, H. H., and Hockings, M. (2015). Using Melaleuca Fences as Soft Coastal Engineering for Mangrove Restoration in Kien Giang, Vietnam. Ecol. Eng. 81, 256–265. doi:10.1016/j.ecoleng.2015.04.031
Van der Stocken, T., De Ryck, D. J. R., Balke, T., Bouma, T. J., Dahdouh-Guebas, F., and Koedam, N. (2013). The Role of Wind in Hydrochorous Mangrove Propagule Dispersal. Biogeosciences 10, 3635–3647. doi:10.5194/bg-10-3635-2013
Van der Stocken, T., Vanschoenwinkel, B., De Ryck, D. J. R., Bouma, T. J., Dahdouh-Guebas, F., and Koedam, N. (2015). Interaction between Water and Wind as a Driver of Passive Dispersal in Mangroves. PLoS One 10, e0121593–17. doi:10.1371/journal.pone.0121593
Van Oudenhoven, A. P. E., Siahainenia, A. J., Sualia, I., Tonneijck, F. H., Van der Ploeg, S., De Groot, R. S., et al. (2015). Effects of Different Management Regimes on Mangrove Ecosystem Services in Java, Indonesia. Ocean Coastal Manag. 116, 353–367. doi:10.1016/j.ocecoaman.2015.08.003
van Wesenbeeck, B. K., de Boer, W., Narayan, S., van der Star, W. R. L., and de Vries, M. B. (2016). Coastal and Riverine Ecosystems as Adaptive Flood Defenses under a Changing Climate. Mitig Adapt Strateg. Glob. Change 22, 1087–1094. doi:10.1007/s11027-016-9714-z
Willemsen, P. W. J. M., Borsje, B. W., Vuik, V., Bouma, T. J., and Hulscher, S. J. M. H. (2020). Field-based Decadal Wave Attenuating Capacity of Combined Tidal Flats and Salt Marshes. Coastal Eng. 156, 103628. doi:10.1016/j.coastaleng.2019.103628
Winterwerp, J. C., Albers, T., Anthony, E. J., Friess, D. A., Mancheño, A. G., Moseley, K., et al. (2020). Managing Erosion of Mangrove-Mud Coasts with Permeable Dams - Lessons Learned. Ecol. Eng. 158, 106078. doi:10.1016/j.ecoleng.2020.106078
Winterwerp, J. C., Erftemeijer, P. L. A., Suryadiputra, N., Van Eijk, P., and Zhang, L. (2013). Defining Eco-Morphodynamic Requirements for Rehabilitating Eroding Mangrove-Mud Coasts. Wetlands 33, 515–526. doi:10.1007/s13157-013-0409-x
Wodehouse, D. C. J., and Rayment, M. B. (2019). Mangrove Area and Propagule Number Planting Targets Produce Sub-optimal Rehabilitation and Afforestation Outcomes. Estuarine, Coastal Shelf Sci. 222, 91–102. doi:10.1016/j.ecss.2019.04.003
Worthington, T. A., zu Ermgassen, P. S. E., Friess, D. A., Krauss, K. W., Lovelock, C. E., Thorley, J., et al. (2020). A Global Biophysical Typology of Mangroves and its Relevance for Ecosystem Structure and Deforestation. Sci. Rep. 10. doi:10.1038/s41598-020-71194-5
Zhang, M., Dai, Z., Bouma, T. J., Bricker, J., Townend, I., Wen, J., et al. (2021). Tidal-flat Reclamation Aggravates Potential Risk from Storm Impacts. Coastal Eng. 166, 103868. doi:10.1016/j.coastaleng.2021.103868
Keywords: mangrove restoration, EMR, planting, aquaculture, land subsidence, building with nature
Citation: van Bijsterveldt CEJ, Debrot AO, Bouma TJ, Maulana MB, Pribadi R, Schop J, Tonneijck FH and van Wesenbeeck BK (2022) To Plant or Not to Plant: When can Planting Facilitate Mangrove Restoration?. Front. Environ. Sci. 9:690011. doi: 10.3389/fenvs.2021.690011
Received: 01 April 2021; Accepted: 30 December 2021;
Published: 07 February 2022.
Edited by:
Boris van Zanten, World Bank Group, United StatesReviewed by:
Pennan Chinnasamy, Indian Institute of Technology Bombay, IndiaNehru Prabakaran, Wildlife Institute of India, India
Pelayo Menendez Fernandez, University of California, Santa Cruz, United States
Copyright © 2022 van Bijsterveldt, Debrot, Bouma, Maulana, Pribadi, Schop, Tonneijck and van Wesenbeeck. This is an open-access article distributed under the terms of the Creative Commons Attribution License (CC BY). The use, distribution or reproduction in other forums is permitted, provided the original author(s) and the copyright owner(s) are credited and that the original publication in this journal is cited, in accordance with accepted academic practice. No use, distribution or reproduction is permitted which does not comply with these terms.
*Correspondence: Adolphe O. Debrot, dolfi.debrot@wur.nl