- 1Key Laboratory of Grassland Resources of the Ministry of Education, Key Laboratory of Forage Cultivation, Processing and High Efficient Utilization of the Ministry of Agriculture and Rural Affairs, Inner Mongolia Key Laboratory of Grassland Management and Utilization, College of Grassland, Resources and Environment, Inner Mongolia Agricultural University, Hohhot, China
- 2State Key Laboratory of Tibetan Plateau Earth System Science, Institute of Tibetan Plateau Research, Chinese Academy of Sciences, Beijing, China
- 3Key Laboratory of Ecosystem Network Observation and Modeling, Lhasa Plateau Ecosystem Research Station, Institute of Geographic Sciences and Natural Resources Research, Chinese Academy of Sciences, Beijing, China
Although dissolved organic nitrogen (DON) plays an important role in the dynamic processes of nitrogen mineralization, fixation, and leaching, the ecological driving forces of DON across the Tibetan Plateau remain largely unknown. Here, we measured climate, soil, plant indicators, and DON concentration on the Tibetan Plateau, and used “change-point” analysis to determine DON patterns. Then correlation analysis was applied to analyze the relationship between DON and each index. Finally, the structural equation modelling (SEM) is used to explain the overall effect between DON and environmental factors. Our results showed that two DON patterns were presented across the Tibetan Plateau, that is, low-DON (5.43 g/mg) and high-DON (16.36 g/mg) patterns. In the low-DON pattern, biologic factors such as microbial carbon, microbial nitrogen, and productivity were the main influencing factors of DON concentration. In the high-DON pattern, DON was strongly governed by hydrothermal synchronization and superior soil environment. This study can help us to have a more comprehensive understanding of the response mechanism of soil soluble nitrogen pool in alpine ecosystems under climate change.
Introduction
Soil dissolved organic nitrogen (DON) is an important component of soil nitrogen (N) pool and plays a crucial role in regulating N cycling and plant productivity in terrestrial ecosystems (Chapin et al., 1993; Raab et al., 1996; Jiang et al., 2020). For example, DON in the form of low molecular amino acids can be utilized directly by plant roots and some mycorrhiza, which increase the cycling of N by reducing the reliance of plants on soil microorganisms turning soil organic matter into inorganic nitrogen (Streeter et al., 2015). Moreover, DON also represents the net balance between inputs (plant and microbial turnover and root exudation) and outputs (N mineralization, plant uptake, and leaching) of N (Jones et al., 2004). The concentration of DON is highly sensitive to abiotic and biotic forces (Jiang et al., 2020). In terrestrial ecosystems, plants and microorganisms are competing in utilizing N (e.g., small molecule dissolve nitrogen) (Dunn et al., 2006). The competition between plants and microorganisms for N is a key ecological process that affects soil DON concentration (Jones et al., 2004). DON has been divided into two distinct pools, that is., low molecular weight (LMW) and high molecular weight (HMW), by their molecular weight (Yu et al., 2002). Previous studies also demonstrated that the rate of ammonification and nitrification in soil can be regulated directly by the LMW-DON pool (Jahnel et al., 1994) while indirectly by HMW-DON through nonspecific inhibition of enzymes like proteases (Stepanauskas et al., 1999).
DON is not only influenced by biological processes but also regulated by climatic variables and hydrological conditions (Zhang et al., 2015). It has been estimated that the global annual mean temperature will increase by 2°C at the end of this century, which will be accompanied with changes in the global precipitation pattern (Tollefson, 2018). The change of hydrothermal synchronization has important influences on the N cycle in terrestrial ecosystems (Chapin et al., 2002). For example, water availability may increase the DON concentration by enhancing ecosystem the productivity (Jiang et al., 2020). In addition, the turnover of DON in the forest ecosystem can be positively (Kalbitz et al., 2010) or negatively (Ueda et al., 2013) correlated with the soil temperature. Notably, the belt transect survey on the Tibetan Plateau elucidated that the concentration of DON increased linearly with the increase of the annual mean temperature (Luo et al., 2010; Wang et al., 2012). Generally, these studies showed that the influences of biotic and abiotic factors on DON are inconsistent among ecosystems (Aitkenhead-Peterson, 2003; Vanderbilt et al., 2003; Sebestyen et al., 2008; Casson et al., 2012; Zhang et al., 2015). Soil DON can be generated through a variety of pathways, but the primary drivers of DON production are still unclear (Kalbitz et al., 2000; Mcdowell, 2003). In particular, few studies have examined how hydrothermal conditions affect DON concentrations at the regional scale across Tibetan Plateau (Jiang et al., 2020).
The Tibetan Plateau, known as the “Third Pole,” is the highest plateau on Earth (Sun et al., 2020). The fragile and sensitive climate and unique biological conditions on the Tibetan Plateau make it an ideal region for exploring the distribution pattern of DON (Zhou et al., 2020b). At present, some of the studies have shown that DON is affected by both biologic and abiotic factors in alpine ecosystems (Warren, 2009; Vestgarden et al., 2010; Warren, 2013). For instance, previous studies reported that DON is mainly regulated by precipitation rather than temperature because it is not sensitive to temperature at the regional scale in the Tibetan Plateau (Jiang et al., 2020). In addition, recent evidence found that plant biomass is a key influencing factor of DON concentration and turnover in alpine grassland (Jiang et al., 2020). Meanwhile, the lower temperature of the alpine ecosystem also effects the DON by limiting the rate of mineralization (Jaeger et al., 1999). Although the above studies have analyzed the relationship between biotic and abiotic factors with DON, little attention has been shown on their internal mechanism. Here, we conducted a belt transect survey in alpine grasslands along water and heat gradients across the Tibetan Plateau. We aimed to answer the following questions: 1) the distribution pattern of DON along environment factors in Tibetan Plateau and 2) the mechanism of response between DON and biotic and abiotic factors in different patterns.
Methods
Study Area
Located to the west of China, Tibetan Plateau (80°−105°E, 27°−37°N) has an alpine climate, with an average altitude of more than 4,000 m. The annual mean precipitation (AMP) of the Tibetan Plateau is 400 mm and decreased from the southeast (c. 1,000 mm) to the northwest (c. 100 mm). The annual mean temperature (AMT) decreases from 20°C in the south of Tibet to −5°C in the northwest. The alpine grassland and alpine meadow are the most widely distributed ecosystem types in the Plateau. The main soil types include felt soil, cold calcareous soil, and brown calcareous soil.
From July to August 2015, plant and soil samples were collected in 115 sample sites along a 5,000-km belt across the Tibetan Plateau. The sampling sites covered three typical grassland types, that is, alpine meadow, alpine grassland, and alpine desert grassland. The rainfall gradient of the sampling sites showed an increasing trend from the northwest to the southeast, with an annual mean precipitation range of 83–898 mm (Supplementary Figure S1).
Soil Plant and Microbial Properties
At each site, three random sampling surveys (50 cm × 50 cm) were carried out in each plot (10 m × 10 m). Meanwhile, the plant community characteristics (species height, number, and coverage) were investigated. The aboveground parts of plants in the sampling square were harvested and calculated as aboveground biomass (AGB). Three soil samples (0–30 cm soil layer) were collected from each small quadrate using drills with a diameter of 5 cm. The roots in soil were removed by rinsing in water and then oven-dried (65°C) for 48 h, and weighted to represent belowground biomass (BGB).
The community diversity index of Shannon–Wiener was calculated by Eq. 1 as follows:
where Pi is the proportion of the total number of individuals of this species in the community.
The plant diversity index of Gleason was calculated by Eq. 2
where A is the area of plots and S is the total number of species in the community.
The climate index of aridity index (AI) was calculated by Eq. 3.
where AMP and AMT are the annual mean precipitation and annual mean temperature, respectively.
Correspondingly, aboveground and belowground plant was stored in paper bags, oven-dried at 65°C, and weighed to calculate AGB and BGB. Soil total dissolved N (TDN) concentrations were measured on a TOC/TN analyzer (Shimadzu 5,000, Japan). Soil NH4+-N (NH4+-N) and soil NO3−-N (NO3−-N) were determined by an Elementar TOC analyzer (Liqui TOCC II, Germany). Dissolved organic nitrogen (DON) was calculated by Eq. 4 (Jiang et al., 2016).
Soil water content (SWC) was measured by drying the plant for 48 h at 105°C (Lu et al., 2015). The potassium dichromate oxidation method was applied to determine soil organic carbon and multiplied by a universal conversion factor of 1.724 to determine soil organic matter (SOM) (Chen et al., 2018). Soil bulk density (SBD) and soil clay (CLAY) were measured by the knife change method and a laser particle size analyzer (A22 Nano Tec, Germany), respectively. And the chloroform fumigation method with a universal conversion factor of 0.45 was used to obtain soil microbe biomass carbon (MBC) and soil microbe biomass nitrogen (MBN) (Vance et al., 1987; Joergensen, 1996).
Climate Database
Climate data in 2015 were collected and collated from the Meteorology Information Center of the Chinese National Bureau of Meteorology (http://data.cma.cn/). Meanwhile, AMP and AMT were spatially interpolated by Anusplin 4.2 (Centre for Resource and Environmental Studies, Australian National University, Canberra, Australia) (Sun et al., 2018), and were used to extract the climatic data of our experiment sites.
Data Analysis
We used the gradient regression analysis of “gbm” package in R software (R Development Core Team, 2015) to determine the relative contribution rate of environmental factors to DON, in which Friedman’s H-statistic was calculated to evaluate the relative strength of the interaction effect in the nonlinear model (Sun et al., 2019). Next, the distribution pattern of DON is divided according to the environmental factors with the highest relative importance. This step is implemented by the package “changepoint” in R software. The different object patterns can be easily detected with the “AMOC” method in the “changepoint” analysis (Killick and Eckley, 2015). Then, the significant environmental factors screened out in the previous step were categorized (soil, plant, climate, and microbe), and the “FactoMineR,” “Factoextra,” and “Corrplot” packages of R software were used for the principal component analysis (Zhou et al., 2020a). With the package “vegan” in R, the variation partitioning analysis was employed to quantify the individual contribution of each group (the first component) on DON (Supplementary Figure S2). Finally, to reveal the control mechanisms of environment factors on DON, structural equation modeling (SEM) was generated by Amos software (17.0.2, Amos Development Corporation, Crawfordville, FL, United States) and evaluated the direct and indirect effect factors on DON.
Results
DON Patterns Across Tibetan Plateau
The DON concentrations of our studied sites are normally distributed between 0.08 and 39.50 mg/kg (Figure 1A). The “BRT” analysis showed that SOM was the most critical factor in affecting DON, with the relative importance of 35.82% (Figure 1B). Therefore, we divided DON into two patterns according to SOM (Figure 1C), namely, low DON (LDON) and high DON (HDON). The mean value of DON concentration in LDON and HDON was 5.43 and 16.36 mg/kg, respectively (Figure 1D).
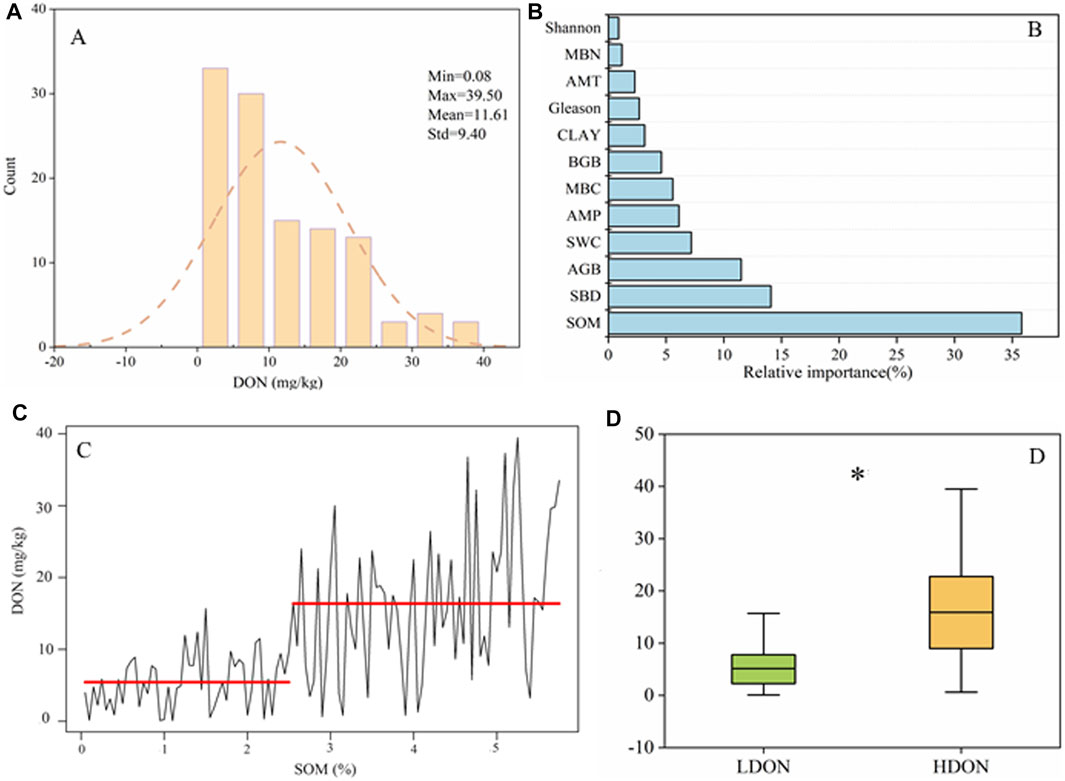
FIGURE 1. Frequency distributions of the dissolved organic nitrogen (DON): (A) relative importance of environmental and biological factors explaining DON, (B) the change points of DON along soil organic matter (SOM), (C) change in dissolve organic nitrogen in different patterns, and (D) “*” indicates significant differences between different patterns.
Characteristics of Climatic, Soil, Plant, and Microbal Factors in Different DON Patterns
The SWC, SBD, MBC, MBN, AMP, SOM, BGB, AGB, Shannon–Winer index, and Gleason index were significantly larger in the HDON pattern than in the LDON pattern (Figures 2A,C–F, and H–L, p < 0.05). There was no significant difference in AMT and CLAY between the two patterns (Figures 2B,G, p < 0.05).
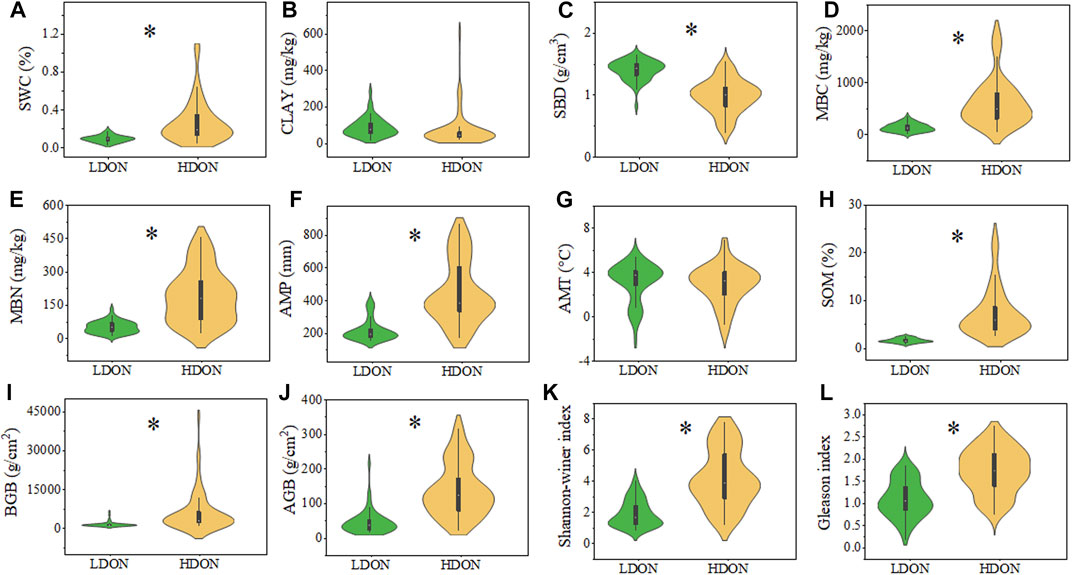
FIGURE 2. Violin plots showing changes in the (A) soil water content (SWC), (B) soil clay (CLAY), (C) soil bulk density (SBD), (D) microbe biomass carbon (MBC), (E) microbe biomass nitrogen (MBN), (F) annual mean precipitation (AMP). (G) annual mean temperature (AMT), (H) soil organic matter (SOM), (I) belowground biomass (BGB), (J) aboveground biomass (AGB), (K) community diversity of Shannon–Wiener (Shannon),and (L) plant diversity of Gleason (Gleason) in different patterns. “*” indicates significant differences between different patterns.
Relationships Between DON and Environmental Factors in Different Patterns
In the LDON pattern, the variation of DON was significantly correlated with AGB, MBC, SBD, AMP, and Gleason (Figure 3A, p < 0.05). In the HDON pattern (Figure 3B), SOM, SBD, BGB, AMT, SWC, MBC, and MBN were the dominant factors that correlated with DON (p < 0.01). Taken together, these results indicated that variation in DON in different patterns was jointly controlled by climate, soil, microbe (MBC in particular), and plant.
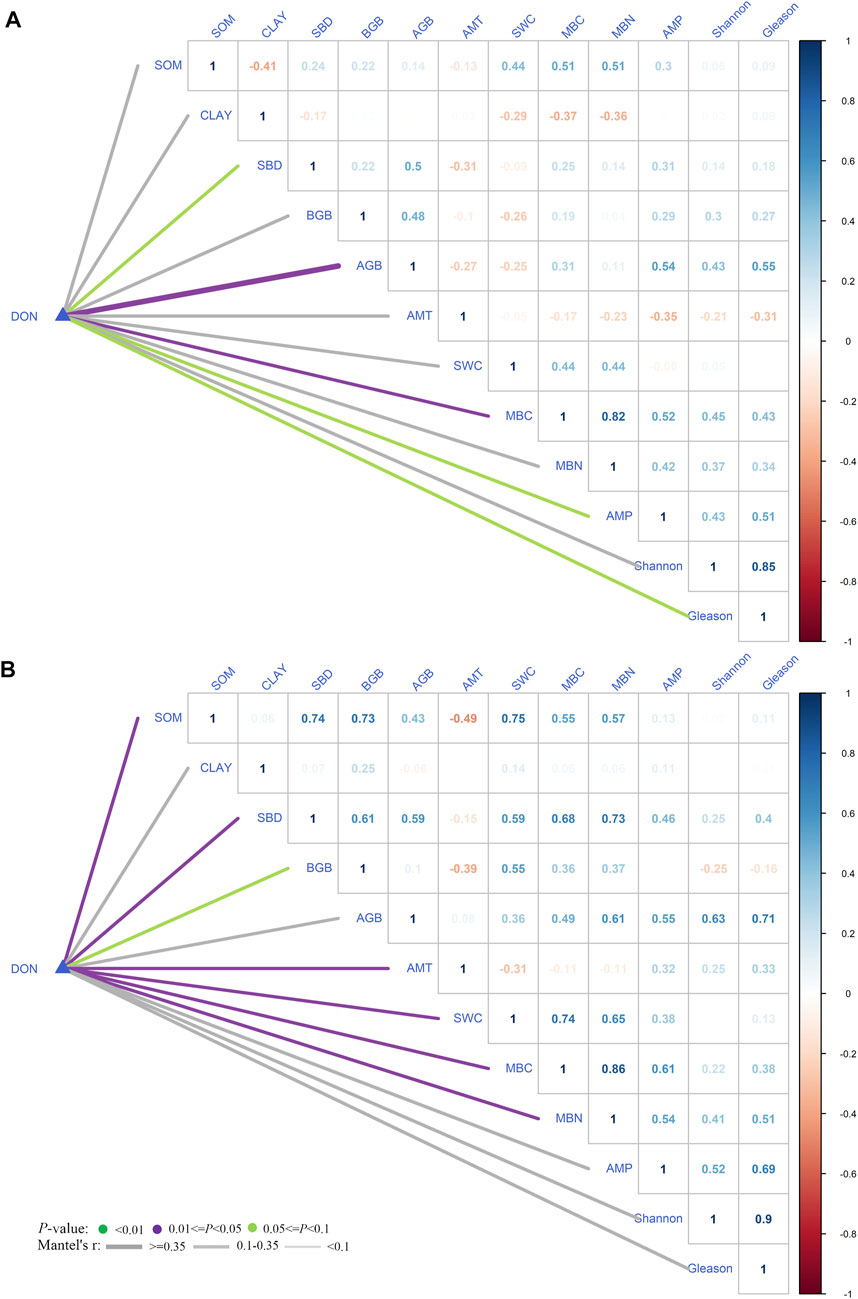
FIGURE 3. Relationships between dissolved organic nitrogen (DON) and environment and biological factors in the LDON pattern (A) and the HDON pattern (B), respectively. Environment and biological factors include soil clay (CLAY), annual mean temperature (AMT), annual mean precipitation (AMP), aboveground biomass (AGB), belowground biomass (BGB) community diversity of Shannon–Wiener (Shannon), plant diversity of Gleason (Gleason), soil water content (SWC), soil organic matter (SOM), microbe biomass carbon (MBC), and microbe biomass nitrogen (MBN).
Control Factors of DON in Different Patterns
Variation partitioning analysis showed that DON in the LDON pattern was mainly explained by the plant (22.0%), followed by microbe (14.1%), climate (11.6%), and soil (8.07%) factors. In the HDON pattern, soil factors (18.4%) explained a greater proportion of the variance in DON than microorganisms (14.5%), climate (11.4%), and plants (7.1%) did.
To better explain the relationship between DON and environment factors in the two patterns, we introduced SEM analysis. In the LDON pattern (Figure 5A), plant and microorganism properties are key factors affecting DON concentrations because AGB (scored at 0.52) and microbes (scored at 0.26) have significantly (p < 0.05) positive effects on DON. Meanwhile, AI also mediates DON concentrations through AGB (scored at 0.52) and microbe (scored at 0.46). In the HDON pattern (Figure 5B), AI affects BGB (scored at 0.26), microbe (scored at 0.67), and water availability (scored at 0.68) through positive effects. BGB (scored at 0.41) and microbe (scored at 0.53) affect SOM through positive response and mediate DON.
Discussion
Biotic Factors Dominate the DON Concentration in the LDON Pattern
In the LDON pattern, plant and microbial factors played dominant roles in influencing DON (Figure 4A). In addition, AGB and microbe directly affected DON (Figure 5A). The dominance of biologic factors over abiotic factors in regulating DON can be attributed to the following two aspects. First, biotic factors regulating both the input and output of DON. To be specific, soil microbial biomass, root metabolites, and secretions are the main sources of DON in soil (Schiff et al., 1992; Xu et al., 2011). Meanwhile, as a “raw material” for mineralization, DON can also be decomposed into inorganic nitrogen by microorganisms for plant growth (Jiang et al., 2016). Second, compared with biotic factors, abiotic factors do not play a decisive role in the low mode. In details, water availability is often the key element to measure the response of DON to abiotic factors (Mao et al., 2020). But in our study, SWC had no correlation with DON, AMT, or AMP (Figure 3A). In the LDON, low AMP (Figure 2F) and high SBD (Figure 2C) make the soil’s water retention ability poor, resulting in faster soil compaction. Therefore, biotic factors (microbial turnover and plant residues) rather than abiotic factors regulate the DON in the LDON pattern.
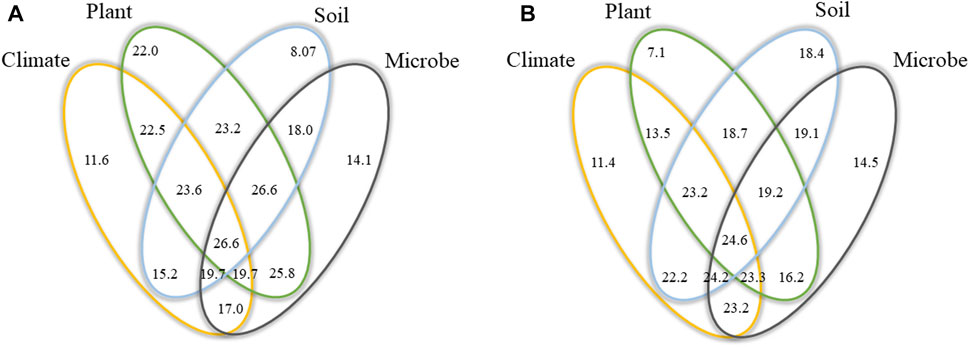
FIGURE 4. Relative contributions of environmental and biological factors to dissolved organic nitrogen (DON) (e.g., climate, plant, soil, and microbial properties that significantly correlated with DON in Figure 3A) in the LDON pattern (A) and relative contributions of environment and biological to DON (e.g., climate, plant, soil, and microbial properties that significantly correlated with DON in Figure 3B) in the HDON pattern (B).
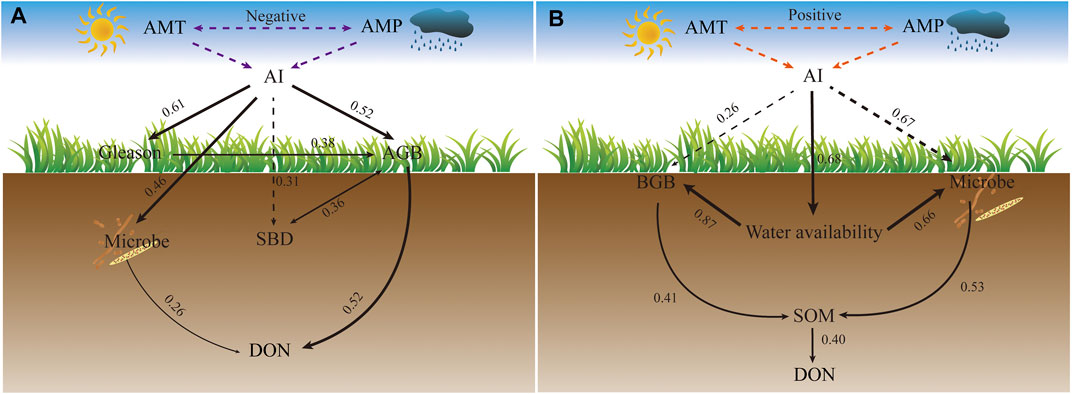
FIGURE 5. Structure equation modeling (SEM) examining the standard total effects of environment and biological factors (soil clay (CLAY), annual mean temperature (AMT), annual mean precipitation (AMP), aboveground biomass (AGB), belowground biomass (BGB) community diversity of Shannon–Wiener (Shannon), plant diversity of Gleason (Gleason), soil water content (SWC), soil organic matter (SOM), microbe biomass carbon (MBC), and microbe biomass nitrogen (MBN)) on dissolved organic nitrogen (DON) in the LDON pattern (A) and HDON pattern (B). Black line and its thickness represent positive relationships and their total effect value. The solid and dashed lines represent direct and indirect effects, respectively. Purple and orange dotted lines represent only the connection. Microbial factors in Figure 5A include MBC and include MBC and MBN in Figure 5B. Water availability represents SBD and SWC in Figure 5B. “Microbe” and “water availability” were the selected factors for the principal component analysis.
Our results also showed that a low AGB (Figure 2J) and the microbe level (Figure 2D) have a direct positive effect on DON (Figure 5A). From the AGB perspective, previous studies revealed that litter is the main source of DON (Vogt et al., 1983; Casals et al., 1995). The low AGB results in a low litter input, which reduces the return of nutrients to the soil and consequently limits the DON concentration. From the microbe perspective, consistent with previous studies, microbial metabolism is one of the key factors affecting soil nutrient availability (Zandt et al., 2019). In the LDON with a low litter input and return and a slow nitrogen cycle, microbes and plants compete fiercely for DON, but because microbes can cover a larger area of soil, they have faster turnover and adsorption (Owen and Jones, 2001). As a result, microorganisms are easier to use and absorb DON than plants (Owen and Jones, 2001).
In the LDON pattern, our results showed that AI indirectly regulated the DON concentration through a positive effect on aboveground biomass and plant diversity (Gleason diversity index) (Figure 5A). It is probably because environment stress (low AI (Supplementary Figure S3), high SBD, and hydrothermal asynchronization (Figures 2A,F)) decreased community diversity and richness, which greatly slowed down litter decomposition (Walter et al., 2020). Finally, this low level of litter return capacity limits DON concentrations. Taken together, in the LDON, low levels of litter decomposition and competitive relationships of plant and microorganisms lead to low concentrations of DON.
Abiotic Factors Dominate the DON Concentration in the HDON Pattern
In the HDON pattern with the warm and wet climate (high AI (Supplementary Figure S3) and hydrothermal synchronization) conditions, our results demonstrated that soil factors (SBD, SWC, and SOM) made greater contributions to DON (Figure 4B). This result suggested that the DON concentration in the HDON pattern is driven by abiotic factors: high water availability (low SBD and high SWC) in particular. At the same time, the microorganisms mediated the DON through the positive effect on SOM (Figure 5B). Nitrogen in soil organic matter mainly exists in the form of polymers and cannot be directly utilized by plants and microorganisms (Monreal and McGill, 1985). Soil microorganisms including mycorrhizas can secrete specific extracellular enzymes (such as proteases and chitinases involved in nitrogen-containing molecular hydrolysis) (Kandeler et al., 1999). These enzymes then degrade the complex organic compounds into dissolved subunits that can be utilized by plants and microorganisms (Cepeda et al., 2000). In addition, our study also found that water availability increased SOM through a positive effect on BGB and microbes (Figure 5B; Supplementary Figure S4). This suggested that high water availability enhances the depolymerization of microorganisms and active and passive absorption of nutrients by the roots. On the one hand, soil nutrient cycles, gross nitrogen mineralization, and microbial immobilization rates are strongly driven by the availability of water (Lange et al., 2019; Mao et al., 2020). High water availability could increase the accumulation of substrate for microorganisms (Mao et al., 2020). As the primary substrate source of microbial nitrogen mineralization and immobilization, DON was mainly derived from the plant litter or root exudate input (Khalid et al., 2007; Murphy et al., 2014). On this basis, the high water availability promoted the good growth of plant roots and the entry of organic matter into the soil (Yang et al., 2009; Reid et al., 2011), thus expediting the subsequent accumulation of substrates in the process of soil nitrogen transformation. On the other hand, high water availability could also directly or indirectly increase the abundance and activity of microorganisms. As the main control on substrate diffusion, rich water resources could strongly decrease the diffusion limitation and increase the amount of microbially accessible dissolve substrate, and further directly contribute to the increase of microbial activity (Hernández and Hobbie, 2010) and lead to soil microorganisms secreting adequate protease into the soil, which complicates the breakdown of proteins and peptides into their constituent amino acid units (HMW-DON) (Kandeler et al., 1999).
Notably, in the HDON pattern, SOM mediated DON by joint positive effects of BGB and microbes (Figure 5B); BGB, MBC, and MBN have a significant positive correlation (Supplementary Figure S4, p < 0.01). Because in superior climatic conditions (high AI and water availability, low SBD, and hydrothermal synchronization), plant and microbial cooperation predominates (Zhou et al., 2021). Previous studies have shown that plant residues are an important source of SOM and the main driving force of microbial activity (Matsuyama et al., 2007). Plant residues in soil can be decomposed by microbes into a soluble organic matter (e.g., DON). At the same time, the decomposition of plant residues is greatly affected by water and heat conditions (Tian et al., 2007). In our study, warm-wet climate facilitated the degradation of plant residues in BGB by increasing microbial biomass and activity, thus consequently resulting in high DON concentrations (Feng and Simpson, 2009; Wetterstedt et al., 2010). Moreover, plants secrete DON (e.g., amino acids) through exudation, forming root sedimentation, which produces a rhizosphere effect and provides abundant nutrition and energy for microorganisms (Marschner, 2012). In this way, the input of DON can be explained by the secretion of a large amount of small molecular organic matter from the root system under moderate hydrothermal conditions in the HDON pattern. In summary, cooperation of plants and microorganisms in warm and humid climates is a key factor leading to high DON concentration.
Moreover, we explored the correlation between water and heat, and found a significant positive correlation between AMP and AMT (Figure 6B, p < 0.05). Previous studies have shown that hydrothermal control of DON may be as important as biological factors (Kalbitz et al., 2000). This hydrothermal synchrony relationship between water and heat can greatly promote the accumulation of soil nutrients (Melillo et al., 2003), so the concentration of DON also increases. In addition, there is no correlation between AGB and DON in HDON, possibly because of low litter accumulation in the region with strong winds (Sun et al., 2018). Therefore, in the HDON pattern, favorable environment (high water availability and SOM) and plants–microorganism cooperation lead to more nutrient accumulation in the soil and higher depolymerization of soil organic matter, resulting in the production of large amounts of DON.
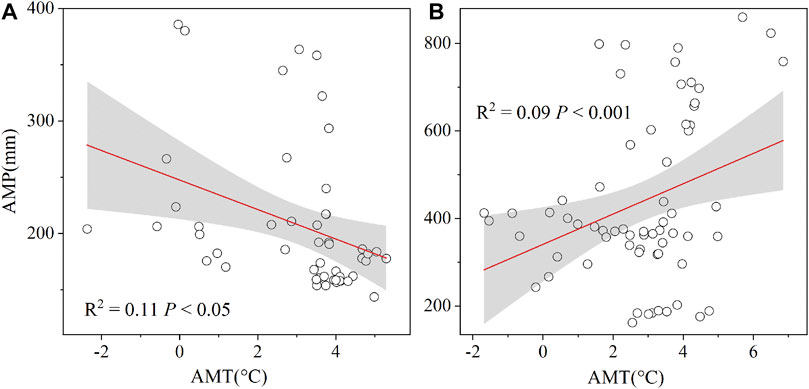
FIGURE 6. Relationship between the annual mean temperature (AMT) and annual mean precipitation (AMP) in low (A) and (B) high patterns, respectively.
Conclusion
Our results suggested that soil organic matter is the key factor in determining the size of the dissolved organic nitrogen pool on the Tibetan Plateau. The dissolved organic nitrogen pool can be divided into two modes: low concentration pattern (5.43 g/mg) and high concentration pattern (16.36 g/mg) in our study region. In the low concentration pattern, plant microbial competition and low litter return (biologic factors) limited the DON concentration. In the high concentration pattern, large amounts of DON were produced by plant–microorganism cooperation and a high level of depolymerization under warm and humid climate conditions (abiotic factors mediate biologic factors).
Data Availability Statement
The original contributions presented in the study are included in the article/Supplementary Material; further inquiries can be directed to the corresponding authors.
Author Contributions
SL, TZ, and JS proposed scientific problems and design experiments. SL and TZ conducted the experiments and analyzed the experimental data. SL, TZ, JS, MZ, and LZ wrote the manuscript. All authors discussed the results and approved the final manuscript and contributed substantially to this research work.
Funding
Funding was provided by the Second Tibetan Plateau Scientific Expedition and Research (Grant No.2019QZKK0405), Joint Research Project of Three-River-Resource National Park funded by Chinese Academy of Sciences and Qinghai Provincial People’s Government (LHZX-2020-08), and the National Science Foundation of China (Grant Nos. 41871040 and 31901198).
Conflict of Interest
The authors declare that the research was conducted in the absence of any commercial or financial relationships that could be construed as a potential conflict of interest.
Supplementary Material
The Supplementary Material for this article can be found online at: https://www.frontiersin.org/articles/10.3389/fenvs.2021.702713/full#supplementary-material
References
Aitkenhead-Peterson, J. A., McDowell, W. H., and Neff, J. C. (2003). Sources, Production, and Regulation of Allochthonous Dissolved Organic Matter Inputs to Surface Waters. Aquat. Ecosyst. 19 (1), 25–70. doi:10.1016/B978-012256371-3/50003-2
Casals, P., Romanyà, J., Cortina, J., Fons, J., Bode, M., and Vallejo, V. R. (1995). Nitrogen Supply Rate in Scots pine (Pinus Sylvestris L.) Forests of Contrasting Slope Aspect. Plant Soil 168 (1), 67–73. doi:10.1007/BF0002931410.1007/978-94-011-0455-5_7
Casson, N. J., Eimers, M. C., and Watmough, S. A. (2012). Impact of winter Warming on the Timing of Nutrient export from Forested Catchments. Hydrol. Process. 26 (17), 2546–2554. doi:10.1002/hyp.8461
Cepeda, C. T., Leirós, M. C., and Sotres, F. G. (2000). Biochemical Properties of Acid Soils under climax Vegetation (Atlantic oakwood) in an Area of the European Temperate–Humid Zone (Galicia, NW Spain): Specific Parameters. Soil Biol. Biochem. 32, 747–755. doi:10.1016/S0038-0717(99)00196-0
Chapin, F., Matson, P., and Mooney, H. A. (2002). Principles of Terrestrial Ecosystem Eology. Berlin: Springer, doi:10.1007/978-1-4419-9504-9
Chapin, F. S., Moilanen, L., and Kielland, K. (1993). Preferential Use of Organic Nitrogen for Growth by a Non-mycorrhizal Arctic Sedge. Nature 361, 150–153. doi:10.1038/361150a0
Chen, L., Liu, L., Mao, C., Qin, S., Wang, J., Liu, F., et al. (2018). Nitrogen Availability Regulates Topsoil Carbon Dynamics after Permafrost Thaw by Altering Microbial Metabolic Efficiency. Nat. Commun. 9, 1. doi:10.1038/s41467-018-06232-y
Dunn, R. M., Mikola, J., Bol, R., and Bardgett, R. D. (2006). Influence of Microbial Activity on Plant-Microbial Competition for Organic and Inorganic Nitrogen. Plant Soil 289 (1-2), 321–334. doi:10.1007/s11104-006-9142-z
Feng, X., and Simpson, M. J. (2009). Temperature and Substrate Controls on Microbial Phospholipid Fatty Acid Composition during Incubation of Grassland Soils Contrasting in Organic Matter Quality. Soil Biol. Biochem. 41 (4), 804–812. doi:10.1016/j.soilbio.2009.01.020
Hernández, D. L., and Hobbie, S. E. (2010). The Effects of Substrate Composition, Quantity, and Diversity on Microbial Activity. Plant Soil 335 (1-2), 397–411. doi:10.1007/s11104-010-0428-9
Jaeger, C. H., Monson, R. K., Fisk, M. C., and Schmidt, S. K. (1999). Seasonal Partitioning of Nitrogen by Plants and Soil Microorganisms in an Alpine Ecosystem. Ecology 80 (6), 1883–1891. doi:10.1890/0012-9658(1999)080[1883:sponbp]2.0.co;2
Jahnel, J. B., Mahlich, B., and Frimmel, F. H. (1994). Beeinflussung der Enzymaktivität einer Protease durch Huminstoffe Influence of Humic Substances on the Activity of a Protease. Acta Hydrochim. Hydrobiol. 22, 109–116. doi:10.1002/aheh.19940220302
Jiang, L., Wang, S., Luo, C., Zhu, X., Kardol, P., Zhang, Z., et al. (2016). Effects of Warming and Grazing on Dissolved Organic Nitrogen in a Tibetan alpine Meadow Ecosystem. Soil Tillage Res. 158, 156–164. doi:10.1016/j.still.2015.12.012
Jiang, L., Wang, S., Pang, Z., Wang, C., Meng, F., Lan, Z., et al. (2020). Abiotic and Biotic Controls of Soil Dissolved Organic Nitrogen along a Precipitation Gradient on the Tibetan Plateau. Plant Soil 459, 65–78. doi:10.1007/s11104-020-04613-1
Joergensen, R. G. (1996). The Fumigation-Extraction Method to Estimate Soil Microbial Biomass: Calibration of the kEC Value. Soil Biol. Biochem. 28 (1), 25–31. doi:10.1016/0038-0717(95)00102-6
Jones, D. L., Shannon, D., V. Murphy, D., and Farrar, J. (2004). Role of Dissolved Organic Nitrogen (DON) in Soil N Cycling in Grassland Soils. Soil Biol. Biochem. 36 (5), 749–756. doi:10.1016/j.soilbio.2004.01.003
Kalbitz, K., Glaser, B., and Bol, R. (2010). Clear-cutting of a Norway spruce Stand: Implications for Controls on the Dynamics of Dissolved Organic Matter in the forest Floor. Eur. J. Soil Sci. 55 (2), 401–413. doi:10.1111/j.1351-0754.2004.00609.x
Kalbitz, K., Solinger, S., Park, J.-H., Michalzik, B., and Matzner, E. (2000). Controls on the Dynamics of Dissolved Organic Matter in Soils: A Review. Soil Sci. 165 (4), 277–304. doi:10.1097/00010694-200004000-00001
Kandeler, E., Luxhi, J., Tscherko, D., and Magid, J. (1999). Xylanase, Invertase and Protease at the Soil-Litter Interface of a Loamy Sand. Soil Biol. Biochem. 31 (8), 1171–1179. doi:10.1016/S0038-0717(99)00035-8
Khalid, M., Soleman, N., and Jones, D. L. (2007). Grassland Plants Affect Dissolved Organic Carbon and Nitrogen Dynamics in Soil. Soil Biol. Biochem. 39 (1), 378–381. doi:10.1016/j.soilbio.2006.07.007
Killick, R., and Eckley, I. A. (2015). Changepoint: AnRPackage for Changepoint Analysis. J. Stat. Soft. 58, 3. doi:10.18637/jss.v058.i03
Lange, M., Koller-France, E., Hildebrandt, A., Oelmann, Y., Wilcke, W., Gerd, G., et al. (2019). How Plant Diversity Impacts the Coupled Water, Nutrient and Carbon Cycles. Adv. Ecol. Res. 61, 185-219. doi:10.1016/bs.aecr.2019.06.005
Lu, X., Yan, Y., Sun, J., Zhang, X., Chen, Y., Wang, X., et al. (2015). Carbon, Nitrogen, and Phosphorus Storage in alpine Grassland Ecosystems of Tibet: Effects of Grazing Exclusion. Ecol. Evol. 5, 4492–4504. doi:10.1002/ece3.1732
Luo, C., Xu, G., Chao, Z., Wang, S., Lin, X., Hu, Y., et al. (2010). Effect of Warming and Grazing on Litter Mass Loss and Temperature Sensitivity of Litter and Dung Mass Loss on the Tibetan Plateau. Glob. Change Biol. 16, 1606–1617. doi:10.1111/j.1365-2486.2009.02026.x
Mao, C., Kou, D., Chen, L., Qin, S., Zhang, D., Peng, Y., et al. (2020). Permafrost Nitrogen Status and its Determinants on the Tibetan Plateau. Glob. Change Biol. 26, 5290–5302. doi:10.1111/gcb.15205
Matsuyama, T., Nakajima, Y., Matsuya, K., Ikenaga, M., Asakawa, S., and Kimura, M. (2007). Bacterial Community in Plant Residues in a Japanese Paddy Field Estimated by RFLP and DGGE Analyses. Soil Biol. Biochem. 39 (2), 463–472. doi:10.1016/j.soilbio.2006.08.016
Mcdowell, W. H. (2003). Dissolved Organic Matter in Soils—Future Directions and Unanswered Questions. Geoderma 113 (3-4), 179–186. doi:10.1016/S0016-7061(02)00360-9
Melillo, J. M., Steudler, P. A., Aber, J. D., Newkirk, K., and Morrisseau, S. (2003). Soil Warming and Carbon-Cycle Feedbacks to the Climate System. Science 298 (5601), 2173–2176. doi:10.1126/science.1074153
Monreal, C. M., and McGill, W. B. (1985). Centrifugal Extraction and Determination of Free Amino Acids in Soil Solutions by TLC Using Tritiated 1-Fluoro-2,4-Dinitrobenzene. Soil Biol. Biochem. 17 (4), 533–539. doi:10.1016/0038-0717(85)90021-5
Murphy, C. J., Baggs, E. M., Morley, N., Wall, D. P., and Paterson, E. (2014). Rhizosphere Priming Can Promote Mobilisation of N-Rich Compounds from Soil Organic Matter. Soil Biol. Biochem. 81, 236–243. doi:10.1016/j.soilbio.2014.11.027
Owen, A. G., and Jones, D. L. (2001). Competition for Amino Acids between Wheat Roots and Rhizosphere Microorganisms and the Role of Amino Acids in Plant N Acquisition. Soil Biol. Biochem. 33, 651–657. doi:10.1016/S0038-0717(00)00209-1
Raab, T. K., Lipson, D. A., and Monson, R. K. (1996). Non-mycorrhizal Uptake of Amino Acids by Roots of the alpine Sedge Kobresia Myosuroides: Implications for the alpine Nitrogen Cycle. Oecologia 108 (3), 488–494. doi:10.1007/BF00333725
Reid, M. A., Ogden, R., and Thoms, M. C. (2011). The Influence of Flood Frequency, Geomorphic Setting and Grazing on Plant Communities and Plant Biomass on a Large Dryland Floodplain. J. Arid Environments 75 (9), 815–826. doi:10.1016/j.jaridenv.2011.03.014
Schiff, S. L., Aravena, R., and Elgood, R. (1992). Sources and Transformation of Dissolved Organic Carbon in the Harp Lake Forested Catchment: The Role of Soils. Radiocarbon 34, 3. doi:10.1017/S0033822200063918
Sebestyen, S. D., Boyer, E. W., Shanley, J. B., Kendall, C., Doctor, D. H., Aiken, G. R., et al. (2008). Sources, Transformations, and Hydrological Processes that Control Stream Nitrate and Dissolved Organic Matter Concentrations during Snowmelt in an upland forest. Water Resour. Res. 44, 12. doi:10.1029/2008WR006983
Stepanauskas, R., Edling, H., and Tranvik, L. J. (1999). Differential Dissolved Organic Nitrogen Availability and Bacterial Aminopeptidase Activity in Limnic and Marine Waters. Microb. Ecol. 38 (3), 264–272. doi:10.1007/s002489900176
Streeter, T. C., Bol, R., and Bardgett, R. D. (2015). Amino Acids as a Nitrogen Source in Temperate upland Grasslands: the Use of Dual Labelled (13C, 15N) glycine to Test for Direct Uptake by Dominant Grasses. Rapid Commun. Mass. Spectrom. 14 (15), 1351–1355. doi:10.1002/1097-0231(20000815)14:15<1351::AID-RCM23>3.0.CO;2-9
Sun, J., Liu, B., You, Y., Li, W., Liu, M., Shang, H., et al. (2019). Solar Radiation Regulates the Leaf Nitrogen and Phosphorus Stoichiometry across alpine Meadows of the Tibetan Plateau. Agric. For. Meteorology 271, 92–101. doi:10.1016/j.agrformet.2019.02.041
Sun, J., Zhou, T. C., Liu, M., Chen, Y. C., Liu, G. H., Xu, M., et al. (2020). Water and Heat Availability Are Drivers of the Aboveground Plant Carbon Accumulation Rate in alpine Grasslands on the Tibetan Plateau. Glob. Ecol Biogeogr 29 (1), 50–64. doi:10.1111/geb.13006
Sun, J., Zhou, T., Liu, M., Chen, Y., Shang, H., Zhu, L., et al. (2018). Linkages of the Dynamics of Glaciers and Lakes with the Climate Elements over the Tibetan Plateau. Earth-Science Rev. 185, 308–324. doi:10.1016/j.earscirev.2018.06.012
Tian, G., Badejo, M. A., Okoh, A. I., Ishida, F., Kolawole, G. O., Hayashi, Y., et al. (2007). Effects of Residue Quality and Climate on Plant Residue Decomposition and Nutrient Release along the Transect from Humid forest to Sahel of West Africa. Biogeochemistry 86 (2), 217–229. doi:10.2307/2045657010.1007/s10533-007-9158-3
Tollefson, J. (2018). IPCC Says Limiting Global Warming to 1.5 °C Will Require Drastic Action. Nature 562, 172–173. doi:10.1038/d41586-018-06876-2
Ueda, M. U., Muller, O., Nakamura, M., Nakaji, T., and Hiura, T. (2013). Soil Warming Decreases Inorganic and Dissolved Organic Nitrogen Pools by Preventing the Soil from Freezing in a Cool Temperate forest. Soil Biol. Biochem. 61, 105–108. doi:10.1016/j.soilbio.2013.02.016
Vance, E. D., Brookes, P. C., and Jenkinson, D. S. (1987). An Extraction Method for Measuring Soil Microbial Biomass C. Soil Biol. Biochem. 19 (6), 703–707. doi:10.1016/0038-0717(87)90052-6
Vanderbilt, K. L., Lajtha, K., and Swanson, F. J. (2003). Biogeochemistry of Unpolluted Forested Watersheds in the Oregon Cascades: Temporal Patterns of Precipitation and Stream Nitrogen Fluxes. Biogeochemistry 62 (1), 87–117. doi:10.1023/a:1021171016945
Vestgarden, L. S., Austnes, K., and Strand, L. T. (2010). Vegetation Control on DOC, DON and DIN Concentrations in Soil Water from a Montane System, Southern Norway. Boreal Environ. Res. 15 (6), 565–578. doi:10.1029/2010WR009092
Vogt, K. A., Grier, C. C., Meier, C. E., and Keyes, M. R. 1983). Organic Matter and Nutrient Dynamics in Forest Floors of Young and Mature Abies Amabilis Stands in Western Washington, as Affected by Fine‐Root Input. Ecol. Monogr. 53, 2, 139–157. doi:10.2307/1942492
Walter, J., Buchmann, C. M., and Schurr, F. M. (2020). Shifts in Plant Functional Community Composition under Hydrological Stress Strongly Decelerate Litter Decomposition. Ecol. Evol. 10, 5712–5724. doi:10.1002/ece3.6310
Wang, S., Duan, J., Xu, G., Wang, Y., Zhang, Z., Rui, Y., et al. (2012). Effects of Warming and Grazing on Soil N Availability, Species Composition, and ANPP in an alpine Meadow. Ecology 93 (11), 2365–2376. doi:10.1890/11-1408.1
Warren, C. R. (2013). Quaternary Ammonium Compounds Can Be Abundant in Some Soils and Are Taken up as Intact Molecules by Plants. New Phytol. 198, 476–485. doi:10.1111/nph.12171
Warren, C. R. (2009). Uptake of Inorganic and Amino Acid Nitrogen from Soil by Eucalyptus Regnans and Eucalyptus Pauciflora Seedlings. Tree Physiol. 29, 401–409. doi:10.1093/treephys/tpn037
Wetterstedt, J. Å. M., Persson, T., and Ågren, G. I. (2010). Temperature Sensitivity and Substrate Quality in Soil Organic Matter Decomposition: Results of an Incubation Study with Three Substrates. Glob. Change Biol. 16, 1806–1819. doi:10.1111/j.1365-2486.2009.02112.x
Xu, X., Ouyang, H., Richter, A., Wanek, W., Cao, G., and Kuzyakov, Y. (2011). Spatio-temporal Variations Determine Plant-Microbe Competition for Inorganic Nitrogen in an alpine Meadow. J. Ecol. 99 (2), 563–571. doi:10.1111/j.1365-2745.2010.01789.x
Yang, Y., Fang, J., Ji, C., and Han, W. (2009). Above- and Belowground Biomass Allocation in Tibetan Grasslands. J. Veg. Sci. 20, 177–184. doi:10.1111/j.1654-1103.2009.05566.x
Yu, Z., Zhang, Q., Kraus, T. E. C., Dahlgren, R. A., Anastasio, C., Zasoski, R. J., et al. (2002). Contribution of Amino Compounds to Dissolved Organic Nitrogen in Forest Soils. Biogeochemistry 61 (2), 173–198. doi:10.1023/A:1020221528515
Zandt, D. I., Brink, A. V. D., Kroon, H. D., and Visser, E. J. W. (2019). Plant-soil Feedback Is Shut Down when Nutrients Come to Town. Plant Soil 439, 541–551. doi:10.1007/s11104-019-04050-9
Zhang, Y., Huo, S., Zan, F., Xi, B., and Zhang, J. (2015). Dissolved Organic Nitrogen (DON) in Seventeen Shallow Lakes of Eastern China. Environ. Earth Sci. 74 (5), 4011–4021. doi:10.1007/s12665-015-4185-1
Zhou, T., Liu, M., Sun, J., Li, Y., Shi, P., Tsunekawa, A., et al. (2020a). The Patterns and Mechanisms of Precipitation Use Efficiency in alpine Grasslands on the Tibetan Plateau. Agric. Ecosyst. Environ. 292, 106833. doi:10.1016/j.agee.2020.106833
Zhou, T., Sun, J., Liu, M., Shi, P., Sun, W., Yang, G., et al. (2020b). Coupling between Plant Nitrogen and Phosphorus along Water and Heat Gradients in alpine Grassland. Sci. Total Environ. 701, 134660. doi:10.1016/j.scitotenv.2019.134660
Keywords: Tibetan Plateau, biologic and abiotic factors, soil, plant–microbe interaction, soil dissolved organic nitrogen
Citation: Li S, Sun J, Zhou T, Zhao M, Cong N and Zhang L (2021) Biologic and Abiotic Factors Regulate Dissolved Organic Nitrogen With Low and High Nutrient Concentrations on Tibetan Plateau, Respectively. Front. Environ. Sci. 9:702713. doi: 10.3389/fenvs.2021.702713
Received: 06 May 2021; Accepted: 21 June 2021;
Published: 20 July 2021.
Edited by:
Rosa Francaviglia, Council for Agricultural and Economics Research, ItalyReviewed by:
Liu Lei, Nanjing University of Information Science and Technology, ChinaJihui Fan, Institute of Mountain Hazards and Environment (CAS), China
Copyright © 2021 Li, Sun, Zhou, Zhao, Cong and Zhang. This is an open-access article distributed under the terms of the Creative Commons Attribution License (CC BY). The use, distribution or reproduction in other forums is permitted, provided the original author(s) and the copyright owner(s) are credited and that the original publication in this journal is cited, in accordance with accepted academic practice. No use, distribution or reproduction is permitted which does not comply with these terms.
*Correspondence: Jian Sun, c3VuamlhbkBpdHBjYXMuYWMuY24=; Mengli Zhao, bm1nbWx6aEAxMjYuY29t