- 1Department of Agricultural Sciences, University of Sassari, Sassari, Italy
- 2CMCC, Euro-Mediterranean Centre on Climate Change Foundation, IAFES Division, Sassari, Italy
- 3Centre for Ecology, Evolution and Environmental Changes, Faculdade de Ciências, Universidade de Lisboa, Lisbon, Portugal
- 4Department de Biología-IVAGRO, Universidad de Cádiz, Cádiz, Spain
- 5Department de Sistemas Físicos, Químicos y Naturales, Universidad Pablo de Olavide, Sevilla, Spain
- 6Centro Interuniversitário de História Das Ciências e da Tecnologia, Faculdade de Ciências, Universidade de Lisboa, Lisbon, Portugal
- 7Real Jardín Botánico, Consejo Superior de Investigaciones Científicas, Madrid, Spain
- 8Department Biodiversidad, Ecología y Evolución, UD Ecología, Universidad Complutense de Madrid, Madrid, Spain
- 9Department of BioEconomy, National Research Council, Sassari, Italy
Atmospheric nitrogen (N) inputs in the Mediterranean Basin are projected to increase due to fossil fuel combustion, fertilizer use, and the exacerbation of agricultural production processes. Although increasing N deposition is recognized as a major threat to ecosystem functioning, little is known about how local environmental conditions modulate ecosystem function response to N addition, particularly in the context of Mediterranean-Basin ecosystems. Here, we assess how N addition affects important ecosystem properties associated with litter decomposition, soil physical-chemical properties, soil extracellular enzymatic activity and microbial abundance across three long-term N addition experimental sites in the Mediterranean Basin. Sites were located in El Regajal (Madrid, Spain), Capo Caccia (Alghero, Italy), and Arrábida (Lisbon, Portugal) and are all representative of Mediterranean shrublands. No common pattern for litter decomposition process or other studied variables emerged among the control plots of the studied sites. Nitrogen supply only affected soil pH, a major driver of decomposition, in two out of three experimental sites. Moreover, when we explored the role of N addition and soil pH in controlling litter decay, we found that the effects of these factors were site-dependent. Our results point out to local ecosystem features modulating N addition effects in controlling litter decomposition rates in Mediterranean ecosystems, suggesting that the responses of soil functioning to N deposition are site-dependent. These findings provide further knowledge to understand contrasting ecosystem responses to N additions based on a single field experiments.
Introduction
Global change, mainly associated with fossil fuel combustion, fertilizers, and intensive animal farming, are causing large increases in nitrogen (N) deposition in the Mediterranean Basin (Fowler et al., 2013; Galloway et al., 2014). This additional nutrient input is known to strongly impact the structure and function of terrestrial ecosystems (Pardo et al., 2011) resulting in soil acidification (Bobbink et al., 2010), severe eutrophication (Currie, 1999), and changes in the abundance, composition, and activity of soil microbial communities (Ferretti et al., 2014; Vourlitis and Fernandez, 2015; Zeng et al., 2016) with negative consequences for key soil processes such as litter and organic matter decomposition (Hobbie, 2005; Knorr et al., 2005; Vourlitis and Zorba, 2007; Sun et al., 2015). Terrestrial ecosystems in the Mediterranean Basin are projected to experience an increased N deposition from the 7 kg N ha−1 yr−1 of the mid-1990s to 12 kg N ha−1 yr−1 in 2050 (Phoenix et al., 2006). While most previous studies have been performed in mesic ecosystems (Pregitzer et al., 2007; Nave et al., 2009; Hao et al., 2013), we still lack cross-site field experimental evidence of the impact of N additions on important soil processes such as litter decomposition, a major regulator of nutrient and carbon (C) entrance in nutrient-poor Mediterranean ecosystems. Unlike for mesic ecosystems, dry deposition drive the accumulation of N in drier Mediterranean ecosystems, which becomes available to plants and microbes after the first rainfall events (Fenn et al., 2003). As such, the responses of soil processes to N deposition in Mediterranean environments might differ from those in more mesic ecosystems, where wet N deposition dominates and determines a more evenly distributed N input over time (Gerosa et al., 2009).
Increased N deposition can directly or indirectly impact litter decomposition via changes in litter quality, soil N availability, and soil biodiversity, leading to changes in microbial community-level activity (Manning et al., 2008; Zeng et al., 2016). For example, Zhu et al. (2016) found that N addition in N-limited sites allowed decomposers to have sufficient N to allocate more energy to the production of polysaccharide hydrolases during the early stage of litter decay that lead to enhanced litter decomposition. However Vourlitis et al. (2021) recentely demostrated that the addition of N in semiarid ecosystems can reduce the activity of hydrolytic enzymes, while Liu et al. (2010) showed in N-rich sites a reduction of the synthesis of ligninolytic enzymes involved in the late stage of litter decomposition. Nitrogen supply can also reduce soil pH, microbial enzymatic activity, and soil microbial biomass, thus hampering litter decomposition (Waldrop et al., 2004; Treseder, 2008; Liu and Greaver, 2010). Nitrogen-induced changes in soil pH modulate the mineralization process (Curtin et al., 1998) through direct and indirect pathways. Variations of soil pH were shown to have effects on structure and activity of the microbial community (Nicol et al., 2008; Lehtovirta et al., 2009; Jiang et al., 2015), on soil biochemical properties (Kemmitt et al., 2006), and bioavailability of soil nutrients (Robson, 1989; Lucas et al., 2011). Moreover, Kimball et al. (2014) reported that in semiarid ecosystems, N addition combined with drouth influenced the native vegetation cover, and Ochoa-Hueso et al. (2019) proved that vegetation type can be more important than N additions in regulating litter decomposition in mosaic ecosystems. Therefore, the effects of N addition on litter decomposition appears to be specific to the ecosystem characteristics, decomposition stage, and litter quality (Lo Cascio et al., 2017). However, further studies are needed to understand the potential interactions between soil N concentration, ecosystem characteristics and decomposition dynamics, especially in semi-arid ecosystems (Cheng et al., 2019).
The overall aim of this experiment is to identify the most relevant parameters and the direction in which they influence the decomposition rate in Mediterranean ecosystems. To do this, we performed a set of analyses to evaluate the response of litter decomposition to chronic N addition in three long term N manipulation experimental sites (NitroMed network (Lo Cascio et al., 2016; Munzi et al., 2017),) in Mediterranean shrubland ecosystems. We used the Tea Bag Index (TBI) as a standardized metric for litter decomposition (Keuskamp et al., 2013). To understand possible common pathways among the experimental sites, we also examined soil properties, microbial community composition, and soil enzymatic activities. We hypothesized that the effects of N addition on decomposition rates depend on the total amount of added N and the ecosystem specificities. In particular, we expected that physical-chemical and biological soil properties like pH, soil nutrients, and soil microbial composition and activity will modulate the response of decomposition rate to N addition.
Material and Methods
Sites Description and Experimental Designs
This study was performed within the international NitroMed network that was established to find general trends in the response of natural and semi-natural shrubland ecosystems in the Mediterranean Basin to increased atmospheric N deposition. Experimental field sites of the network are: El Regajal (Spain), Capo Caccia (Italy), and Arrábida (Portugal). As N treatments applied in the three sites are different, the aim of the network is to find common trends applicable to ecosystems located in the Mediterranean Basin instead of looking for direct comparison among the sites. The main characteristics of each experimental site are summarized in Table 1.
EL Regajal, Spain
El Regajal is a semiarid Mediterranean shrubland within the Nature Reserve “El Regajal-Mar de Ontígola” (central Spain). The semiarid Mediterranean climate favors rainless and warm conditions in summer and cold and rainy weather in winter. Soils are rich in calcium carbonate with a sandy clayish structure. Vascular vegetation is dominated by evergreen species such as Quercus coccifera L. and Rosmarinus officinalis L. while a well-developed biocrust colonizes the space between shrubs (see Ochoa-Hueso et al., 2011 for detailed community description). The natural oxidized N (NOx) deposition for this area of ca. 1.05 kg NOx ha−1 y−1 for the period from 2000 to 2013 according to the European Monitoring and Evaluation Programme (EMEP Status Report (1/2015)) (EMEP, 2015).
In September 2007, 24 plots (2.5 × 2.5 m) were established following a 6-block design (n = 6). Within each block, four plots were randomly selected: three plots were subjected to N additions of 10, 20, and 50 kg N ha−1 y−1, respectively, and compared to unfertilized control plots. Starting in October 2007 and once per month, 2 L of 0, 19, 37, and 93 mM ammonium nitrate (NH4NO3) solution were added to the whole surface of the plots, except during the typical dry period of this ecosystem (i.e., July and August) to simulate the peak of N availability related to the onset of rains (Fenn et al., 2003). Therefore, a correspondent triple dose was applied in September. The soil surface was only slightly rewetted as a result of the N fertilization treatments and treated soils became dry in few minutes. This procedure fostered N accumulation at the soil surface mimicking the effect of atmospheric N deposition in this area where morning dew frequently wets the soil surface. The same amount of water was added to the control plots. Buffer strips of at least 1 m were set up between the plots. As the main source of N enrichment in these ecosystems is dry deposition that suddenly becomes available with rainfall events leading to high peaks of this nutrient (Fenn et al., 2003), we intended to mimic this effect by fertilizing our experimental sites through wet N addition in high doses which act as a N pulse in the soil.
Capo Caccia, Italy
Capo Caccia is a coastal Mediterranean shrubland ecosystem located in north-western Sardinia (Italy) within the Nature Reserve “Portoconte-Capo Caccia”. The area features a sub-humid Mediterranean climate characterized by dry summers, mild and wet winters, and rainy springs and autumns. Soil is classified as terra rossa (USDA, 1999); more details are reported in Table 1. Juniperus phoenicea L. represents 80% of the vascular vegetation cover, while the remaining 20% is bare soil covered by a biological soil crust community. A detailed description of this biological soil crust can be found in Morillas et al. (2017). According to EMEP Status Report (1/2015), NOx deposition in Capo Caccia was ca. 0.96 kg NOx ha−1 y−1 from 2000 to 2013, whereas Gauss et al. (2016) reported that N natural deposition in 2012 varied from 1.7 to 3.7 kg N ha−1 y−1.
In April 2012, eight plots of 7.5 × 7.5 m were randomly established. To encompass the spatial heterogeneity of the experimental site, each plot contained at least one well-developed J. phoenicea and a section of bare soil. Within each plot two different microsites were analyzed: under the canopy of J. phoenicea (hereafter referred to as Capo Caccia UC) and bare soil (hereafter referred to as Capo Caccia BS). Four randomly selected plots were subjected to the addition of 30 kg N ha−1 y−1 over the natural background N deposition as by Lo Cascio et al. (2017) whereas the other four plots served as unfertilized controls (n = 4). At the beginning of each season, 7.5 kg of NH4NO3 was dissolved in 20 L of water and sprayed on the whole surface of all the treated plots. Control plots received the same amount of water. Like El Regajal, this experimental site was fertilized with wet N addition in high doses to produce a soil N pulse to simulate the natural peaks of N availability associated with the seasonal rains typical of these ecosystems. Following N fertilization treatments, the soil surface was only slightly rewetted and treated soils became dry in few minutes. This allowed N to accumulate at the superficial layers of soil, mirroring the effect of natural atmospheric N deposition in this site where morning dew frequently wets the soil surface.
Arra´bida, Portugal
This experimental site consists of a maquis shrubland ecosystem located in Arrábida Natural Park, south of Lisbon, Portugal. The climate is sub-humid Mediterranean with similar characteristics to those described for Capo Caccia. The soils of this site are terra rossa classified as calcic rhodo-chromic luvisols and calcareous chromic cambiols. The vegetation consists of a dense maquis dominated by Cistus ladanifer L. while a well-developed biocrust colonizes the scarce space between shrubs (see (Dias et al., 2013) for further details on the site description). According to EMEP Status Report (1/2015) the Background N deposition is ca. 5.2 kg ha−1 y−1 (2.9 kg NOx +2.3 kg NHy).
In January 2007, nine plots of 20 × 20 m were established following a 3-block design (n = 3). N availability was manipulated in two plots within each block by adding 40 and 80 kg N ha−1 y−1 in the form of NH4NO3 and compared to unfertilized control plots. The dry fertilizer was homogenously added by hand in three equal applications throughout the year: spring, summer, and mid-autumn/winter (Dias et al., 2013).
Litter Incubation and Soil Sampling
Field activity, encompasing tea bags deployment and soil sampling collection for physico-chemical and biological analysis, was simultaneously performed in all the experimental sites at the beginning of April 2015.
The TBI has been proved to be an efficient estimator to characterize and compare organic matter decomposition dynamics between different ecosystems and soil types (Djukic et al., 2018; Keuskamp et al., 2013 see Supplementary Material for details regarding this method). One randomly placed tea bag of each of the two litter qualities (green tea and rooibos) was labeled and buried at 8 cm depth in each plot at every site. This made a total of 32 tea bags in Capo Caccia (16 in Capo Caccia UC and 16 in Capo Caccia BS), 48 in El Regajal and 18 in Arrábida. Although one unique tea bag of each tea might have constrained our ability to capture the entire spatial variability of the experimental plots, it is important to highlight that plots in El Regajal and Arrábida were mostly homogeneous, whereas the patchy distribution in Capo Caccia was accounted for by deploying tea bags in both microsites. Tea bags were collected from all sites after a field incubation period of 65 days. Subsequently, they were cleaned from roots and soil, oven-dried at 70 C for 48 h, and weighed. As described by Keuskamp et al. (2013), two parameters composing the TBI were computed: TBIk which describes decomposition rate, and TBIS that gives information about the litter stabilization factor.
At the same time the tea bags were installed, one composite soil sample per plot and microsite (Capo Caccia) was collected at each site, making a total of 16 soil samples in Capo Caccia (8 in Capo Caccia UC and eight in Capo Caccia BS), 24 in El Regajal and nine in Arrábida. For each studied site/microsite each plot was defined aiming to be representative of the area, but at the same time reducing at minimum the intra-plot heterogeny, therefore no slope is present, main vegetation cover is included in each plot, and there is an adequate number of plot replications. Composite samples consisted of five soil cores (2 cm in diameter, 0–8 cm depth of the mineral soil) in each plot pooled together to account for spatial variability. A subset of each soil sample was frozen at—20°C to perform the microbial community analyses, while the rest of the soil samples were oven-dried at 40°C for 72 h and sieved through a <2 mm mesh to complete the biogeochemical analyses.
Soil Physical-Chemical and Microbial Analyses
We analyzed soil pH, organic C, total N, inorganic N, total phosphorous (P), and the activities of extracellular soil enzymes (Pritsch et al., 2011) involved in the degradation of cellulose (glucuronidase, cellobiohydrolase, glucosidase), hemicellulose (xylosidase), N-terminus of peptides and proteins (leucine aminopeptidase), chitin (N-acetylglucosaminidase) and phosphate (acid phosphatase). We also measured the total and relative abundance of bacteria, fungi, ammonia-oxidizing bacteria (AOB), and archaea (AOA) using quantitative PCR (qPCR). See Supplementary Material for details on these methods.
Data and Statistical Analyses
All datasets were tested for normality and homogeneity of variance (Shapiro-Wilk and Levene statistics) and were log-transformed when necessary. Statistical significance was defined at the 95% confidence level (p-value <0.05).
We tested for differences among control plots for TBIk and TBIS, soil chemical, physical and microbiological properties among sites with a one-way ANOVA to assess the differences in soil properties among the experimental sites. We also tested for differences among treatments for TBIk and TBIS, soil pH, EEA, soil physico-chemical properties, and microbial community within sites with a one-way ANOVA. Whenever the one-way ANOVA analyses were significant, we conducted a post hoc LSD test to explore differences in our response variables among treatments or sites, respectively. We tested the treatment effect statistically using the total N amount (data not showed) and the annual N doses. We computed the total N amount for each treatment and site as the sum of each N addition from the beginning of each experiment to Spring 2015. Although results showed similar trends for both the annual N doses and cumulative N, we opted for using the annual doses due to their clearer effects.
A principal component analysis (PCA) across sites and treatments was conducted with EEA variables to identify common patterns of response of microbial community activity (Supplementary Figure S1; Supplementary Table S1). The first component of PCA (EEA_1stAxis) explained 65% of the total EEA variance showing higher correlation with the enzymes related to C mineralization activity. The EEA_1stAxis was used for further analyses.
Spearman correlation analyses were conducted between TBIk and TBIS and a number of the studied variables encompassing soil chemical proprieties, soil pH, AI, microbial community, and EEA_first Axis. In addition, we also conducted correlation analyses between pH and EEA for each site and microsite. These analyses were performed by using IBM SPSS, version 23.0 (SPSS Inc. Chicago, IL, United States).
Aridity indexes (AI) were calculated as a numerical indicator of the degree of dryness of the climate at each study site during the tea bag incubation period (Barrow, 1992). Aridity indexes were computed as AI = P/PET, where PET is the monthly average potential evapotranspiration (mm), and P is the monthly average precipitation (mm). Potential evapotranspiration was calculated using the FAO Penman-Monteith method (Jensen et al., 1990).
To examine whether TBIk and TBIS differed between fertilized and control plots, among and within sites, we used generalized linear mixed models (GLMMs). The GLMMs were performed using R (R Core Team, 2014; package = “lme4” for GLMMs and package = “multcomp” to perform post hoc test). The GLMMs for all sites account for the interaction between treatments and sites as fixed factor and pH as a covariate, while the respective experimental designs were included as a random factor. We also analysed all the sites separately. The GLMMs for El Regajal, Capo Caccia BS, Capo Caccia UC, and Arrábida, accounted for the interaction between treatments and pH as fixed factors, while as the previous model, the respective experimental designs were included as random factors on TBIk and TBIS response.
Results
Comparison of Soil Physico-chemical and Biological Properties Among Sites
Control plots of Arrábida showed a significantly lower organic C content compared to Capo Caccia in both microsites and a significantly lower content of total N compared to El Regajal (Table 2). We found no significant differences for inorganic N in control plots among sites. In control plots, C/N content resulted significantly higher in Capo Caccia UC compared to El Regajal (Table 2). Total P content was minimum in the control plots of Arrábida compared to the control plots of the other experimental sites (Table 2). In terms of physical variables, pH and AI showed maximum values in El Regajal, medium in both Capo Caccia microsites, and minimum in Arrábida (Table 2). The EEA_first axis in El Regajal in control plots was significantly higher than the rest of sites (Table 2). The fungi to bacteria (F/B) ratio was significantly lower in El Regajal and Capo Caccia BS than in Capo Caccia UC and Arrábida (Table 2), while the AOA showed a maximum value in El Regajal, medium in both microsites of Capo Caccia and minimum in Arrábida (Table 2). TBIk in control plots of Arrábida was 2-fold higher than El Regajal and Capo Caccia in both microsites (Table 2), while TBIS in control plots of El Regajal was significantly higher compared to both microsites of Capo Caccia (Table 2).
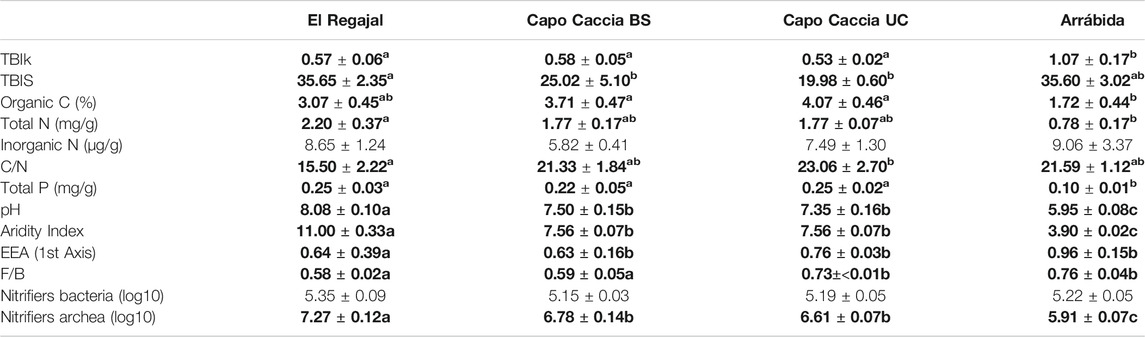
TABLE 2. Soil physico-chemical and biological properties and other studied variables in the top 8 cm of the soil profile (means ± SE) for the control plots in El Regajal (Spain, n = 6), Capo Caccia BS (Italy, n = 4), Capo Caccia UC (Italy, n = 4) and Arrábida (Portugal, n = 3). BS and UC indicate bare soil and under canopy microsites. Soil sampling was performed in April 2015. Statistical differences are highlighted in bold, and letters indicate significant differences (p < 0.05) among the control plots of the study sites (one-way ANOVA, post hoc LSD test). TBIk, TBIS, EEA. TBIk, TBIS, and aridity index results were multiplied by 100.
Nitrogen Treatments Effects on Soil Properties
We found no significant change of TBIk in response to N addition in any of the experimental sites (Figure 1; p > 0.05). Nitrogen addition significantly increased TBIS in Capo Caccia UC (Figure 1, one-way ANOVA; F = 11.752; df = 1, p < 0.014), whereas no effects were detected in the other sites.
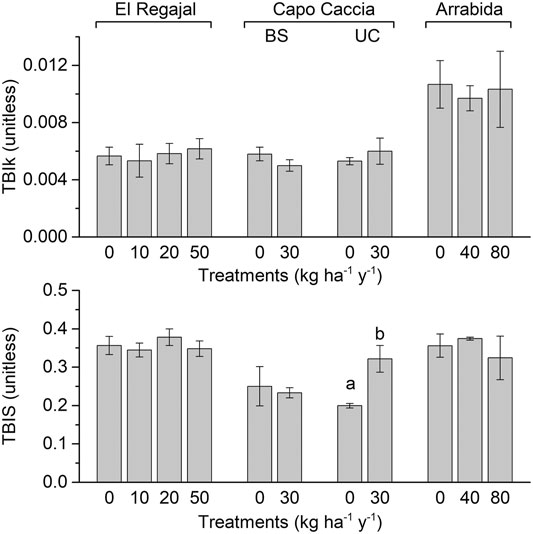
FIGURE 1. Effect of nitrogen (N) addition on soil decomposition rate (TBIk) and stabilisation factor (TBIS) (means ± SE) in the top 8 cm of the soil profile estimated through the tea bag index method for each N treatment in El Regajal (Spain, n = 6), Capo Caccia BS (Italy, n = 4), Capo Caccia UC (Italy, n = 4) and Arrábida (Portugal, n = 3). Capo Caccia BS and UC indicate bare soil and under canopy microsites, respectively. Tea bags incubations were performed for 65 days in spring 2014. Letters indicate significant differences among N treatments within each site (p < 0.05, one-way ANOVA, post hoc LSD test).
Significant differences in soil pH were found among treatments in both Capo Caccia BS (Figure 2, one-way ANOVA; F = 10.714; df = 1, p < 0.017) and Arrábida (Figure 2, one-way ANOVA; F = 6.966; df = 2, p < 0.027). However, in Capo Caccia BS, pH decreased with N addition, whereas in Arrábida pH showed the opposite trend. Soil pH in El Regajal followed the same descending trend as in Capo Caccia BS, but significant differences among treatments were not found (Figure 2; p > 0.05). In contrast, soil pH tended to increase in response to N addition in Capo Caccia UC, although no significant differences among treatments were found (Figure 2; p > 0.05). Inorganic N content significantly increased along the N addition gradient in El Regajal (Table 3, one-way ANOVA; F = 17.654; df = 3, p < 0.001), and a similar not significant trend was showed in Arrábida. No other significant differences in soil chemical properties among treatments were found for any site (Table 3; p > 0.05).
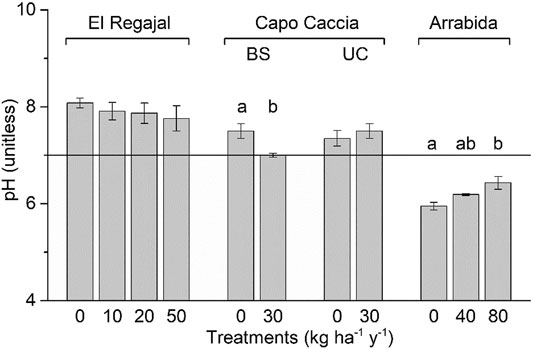
FIGURE 2. Effect of nitrogen (N) addition on soil pH (means ± SE) in the top 8 cm of the soil profile for each N treatment in El Regajal (Spain, n = 6), Capo Caccia BS (Italy, n = 4), Capo Caccia UC (Italy, n = 4) and Arrábida (Portugal, n = 3). Capo Caccia BS and UC indicate bare soil and under canopy microsites, respectively. Letters indicate significant differences among N treatments within each site (p < 0.05, one-way ANOVA, post hoc LSD test). The solid line indicates neutral pH.

TABLE 3. Soil chemical properties (means ± SE) in the top 8 cm of the soil profile for each N treatment in El Regajal (Spain, n = 6), Capo Caccia (Italy, n = 4) and Arrábida (Portugal, n = 3). Capo Caccia BS and Capo Caccia UC indicate bare soil and under canopy microsites, respectively. Statistical differences (one-way ANOVA, post hoc LSD test) are highlighted in bold and letters indicate significant differences (p < 0.05) among N treatments within each site.
Total fungal abundance was significantly lower in treated plots than in control plots in Capo Caccia BS (Table 4, one-way ANOVA; F = 9.458; df = 1, p < 0.022), whereas no differences among treatments were observed for other variables related to the microbial community for the other sites (Table 4; p > 0.05). No differences between treatments were found in Capo Caccia BS or Arrábida for soil EEA, whereas the activity of cellobiohydrolase and glucosidase significantly increased with N addition in Capo Caccia UC (Figure 3, one-way ANOVA; F = 12.800; df = 1, p < 0.012 and F = 10.198; df = 1 p < 0.019, respectively). The same increase in response to N addition was found for cellobiohydrolase in El Regajal (Figure 3, one-way ANOVA; F = 3.740; df = 3, p < 0.031), although significant differences were only found between 50 kg N ha−1 y−1 and the other three treatments.

TABLE 4. Soil microbial community (means ± SE) in the top 8 cm of the soil profile for each N treatment in El Regajal (Spain, n = 6), Capo Caccia BS (Italy, n = 4), Capo Caccia UC (Italy, n = 4) and Arrábida (Portugal, n = 3). Capo Caccia BS and Capo Caccia UC indicate bare soil and under canopy microsites, respectively. Statistical differences (one-way ANOVA, post hoc LSD test) are highlighted in bold and letters indicate significant differences (p < 0.05) among N treatments within each site.
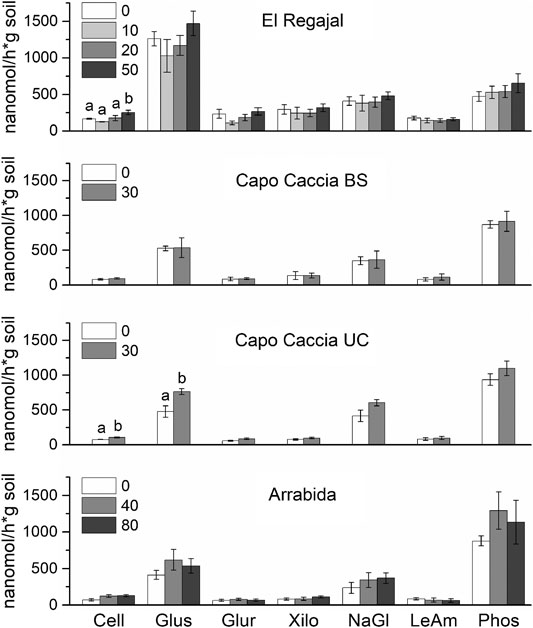
FIGURE 3. Soil extracellular enzymatic activity (EEA, means ± SE) for each N treatment in El Regajal (Spain, n = 6), Capo Caccia BS (Italy, n = 4), Capo Caccia UC (Italy, n = 4) and Arrábida (Portugal, n = 3). Capo Caccia BS and UC indicate bare soil and under canopy microsites, respectively. Letters indicate significant differences among N treatments within each site (p < 0.05, one-way ANOVA, post hoc LSD test). Soil EEA showed are respectively: cellobiohydrolase (Cell), glucosidase (Glus), glucoronidase (Glur), xilosidase (Xilo), N-acetyl glucosaminidase (NaGl), Leucine Aminopeptidase (LeAm), phosphatase (Phos).
Generalized Linear Mixed Model
When analysing all sites together, we found that TBIk and TBIS were not affected by the N treatments, but the model showed a significant site effect. Besides, the interaction between treatments and site was statistically significant for TBIS (Table 5). When looking at the effect of N treatment and soil pH within each experimental site, we found no significant effect of these factors for TBIk or TBIS in El Regajal (Table 5). However, in Capo Caccia BS, N treatments and interaction showed significant effects for TBIk and TBIS (Table 5). In Capo Caccia UC, N treatments, soil pH and their interaction significantly affected TBIk, whereas no effects of these factors were found for TBIS in this site (Table 5). In Arrábida site, we found significant effects of the N treatments and the interaction between treatments and soil pH for TBIS, whereas we detected no effect of these factors on TBIk.
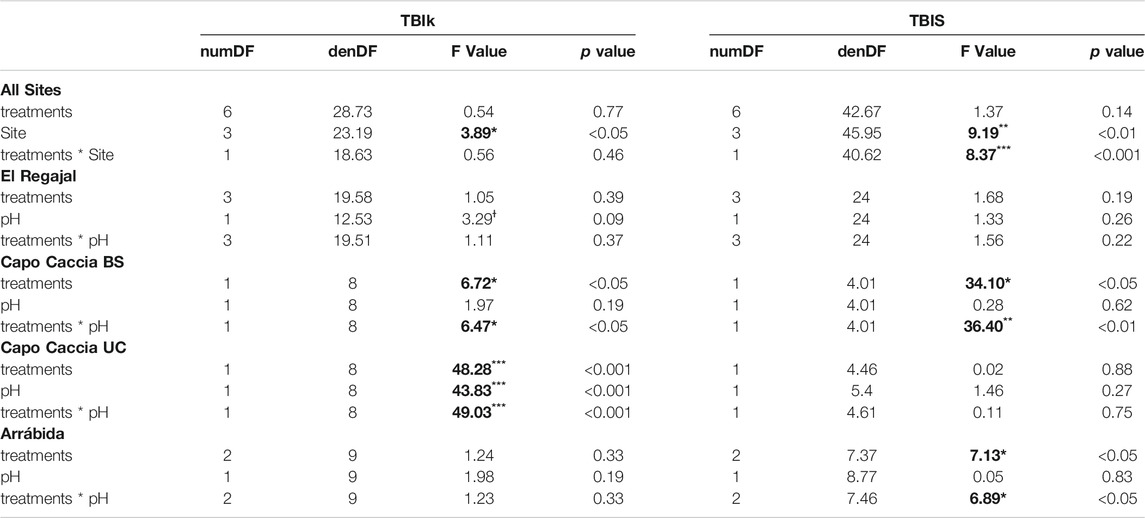
TABLE 5. Results of the generalized linear mixed models (GLMMs). In All Sites (n = 13) the GLMMs accounts for the interaction between treatments and Sites as fixed factors, the pH as covariate and the experimental designs of all sites were included as random factors on the decomposition rate (TBIk) and stabilisation factor (TBIS) response. Capo Caccia BS and Capo Caccia UC indicate bare soil and under canopy microsites, respectively. In El Regajal (Spain, n = 6), Capo Caccia BS (Italy, n = 4), Capo Caccia UC (Italy, n = 4), and Arrábida (Portugal, n = 3), the GLMMs accounts for the interaction between treatments and pH as fixed factors while the respective experimental designs were included as random factors on TBIk and TBIS response. Statistical differences are indicated as *p < 0.05, **p < 0.01, and ***p < 0.001 and are highlighted in bold. Ɨ is used to designate a value with p ≤ 0.1.
Correlations
In El Regajal no significant correlations were found between TBIS and other soil variables, whereas TBIk showed a positive correlation with organic C, C/N, EEA_first Axis, and F/B ratio (Table 6). In Capo Caccia BS, we found no significant correlations for TBIS, whereas TBIk resulted negatively correlated with C/N ratio and EEA_first Axis (Table 6). In Capo Caccia UC, we found a positive correlation between TBIS and the EEA_first Axis, while the TBIk was positively correlated with total N and inorganic N contents (Table 6). In Arrábida, we found no significant correlations with TBIS, whereas TBIk was negatively correlated with the F/B ratio (Table 6). In addition in Capo Caccia BS, Capo Caccia UC and Arrábida we found no significant correlation between pH and the EEA, whereas in El Regajal soil pH resulted positively correlated with xilosidase activity and negatively correlated with leucine aminopeptidase, phosphatase activity (Supplementary Table S2).
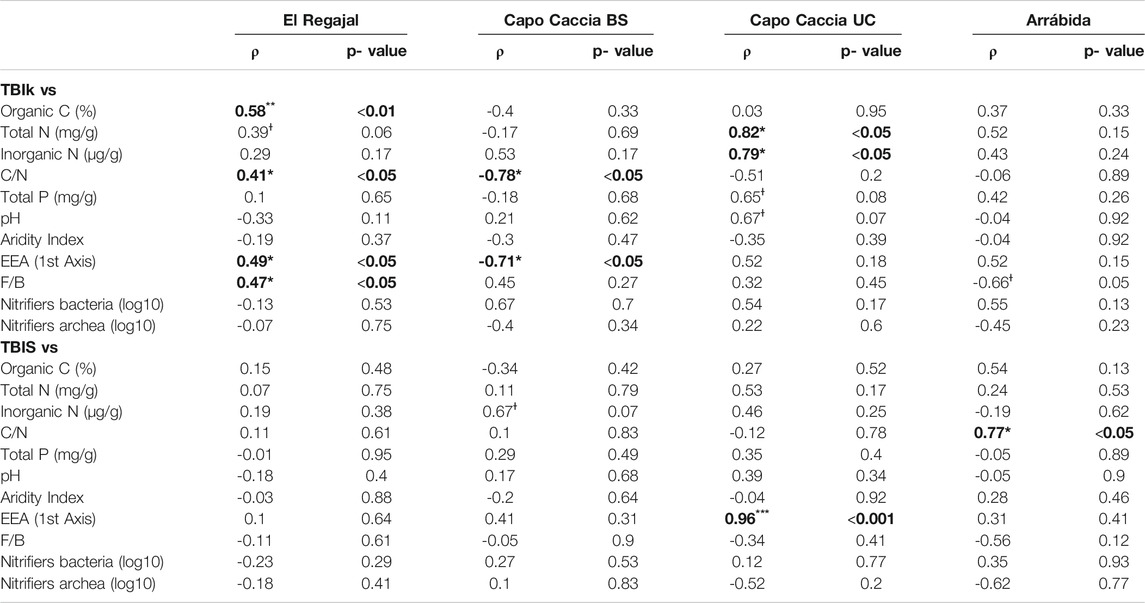
TABLE 6. Results of the Spearman’s rho tests between the decomposition rate (TBIk) and stabilisation factor (TBIS) and the studied variables in El Regajal (Spain, n = 24), Capo Caccia BS (Italy, n = 8), Capo Caccia UC (Italy, n = 8) and Arrábida (Portugal, n = 9). Capo Caccia BS and Capo Caccia UC indicate bare soil and under canopy microsites. EEA and F/B stand for extracellular enzymatic activity and fungi to bacteria ratio, respectively. Statistical differences are indicated as *p < 0.05, **p < 0.01, and ***p < 0.001and are highlighted in bold. Ɨ is used to designate a correlation with p ≤ 0.1. The rho value (ρ) and the level of significance (p value) are shown for each correlation.
Discussion
Results clearly highlight that N addition did not affect decomposition in a consistent manner across the studied experimental sites. Indeed, the effect of N deposition on litter decomposition rates in the selected Mediterranean areas was modulated by a number of site-dependent ecosystem properties such as soil physical-chemical and biological properties, community composition, and climatic conditions. A larger number of sites would have probably highlighted a general effect, but also likely with wide confidence intervals and so of limited use for site specific conservation or restoration practices. These results provide further knowledge to understand contrasting ecosystem responses to N additions based on single field experiments. This will contribute to the establishment of suitable environmental policies and restoration actions to protect threatened Mediterranean areas according to their vulnerability to N deposition, which will depend on these site-dependent ecosystem properties. If we are able to pinpoint what ecosystem characteristics make it more prone to being disrupted by N pollution, policy makers and stakeholders will have the opportunity to dictate particular restoration policies and restoration actions to protect this especially vulnerable areas.
Context-dependent Impacts of N on Soil Chemical and Biological Properties
When studying the response of soil chemical properties to increased N addition in each experimental site, we observed that inorganic N was significantly increased in a dose-dependent manner by fertilization in El Regajal, a similar trend was found in Arrábida although not significant, whereas no effect was reported in Capo Caccia (Table 3). These results could be in part reflecting the different temporal extent of the treatment in each experimental site, which led to accumulating higher inorganic N in the soils amended with N addition for longer periods: El Regajal and Arrábida were treated for 8 years, whereas Capo Caccia was treated only for 3 years (Table 1). On the other hand, these results could also be due to the fact that a unique soil sampling was performed to reveal soil N accumulation, which may have hidden temporal patterns potentially indicating increased soil N accumulation that could have arisen when performing more exhaustive soil samplings.
Fungi are particularly sensitive to fertilization and have been found to be the group of microorganisms most affected by increased N availability (Nilsson and Wallander, 2003; Högberg, 2006; Demoling et al., 2008). Accordingly, it has been reported that fungal biomass decreases with increasing nutrients in natural nutrient gradients in forests (Pennanen et al., 1999; Högberg et al., 2003, 2007), whereas bacteria did not significantly respond to N fertilization in a meta-analysis accounting for 82 field studies (Treseder, 2008). In our study, only total fungi in Capo Caccia BS were significantly reduced by N addition, despite we analyzed a comprehensive set of variables related to the microbial community. Conversely, several studies have observed that the long-term application of N fertilizer can have a positive (He et al., 2007; Shen et al., 2008; Tian et al., 2014) or negative effects (Xu et al., 2012) on the AOA and AOB abundance. This lack of consistency in the microbial responses to N inputs has been reported in other studies (Demoling et al., 2008; Fierer et al., 2012), suggesting that N fertilization on soil microbial diversity is likely site-dependent as well. On the other hand, it is also possible that the observed marginal effect of N addition on the microbial community could be associated to ecosystem N saturation. This potential N-overload may have saturated the ecosystem, disabling it to respond to more N. Thus, the long-term N deposition may induce a nutrient imbalance which interfere with ecosystem functions (Aber et al., 1998), such as microbial resource balance.
Increased N availability has been shown to affect the soil enzymatic functioning in a number of studies in a consistent direction. We found that the unique EEA responding to soil fertilization were cellobiohydrolase in El Regajal and Capo Caccia UC and b-glucosidase in Capo Caccia UC. Previous studies reported that the effects of N availability on EEA were enzyme-specific (Chen et al., 2013). Increased N availability stimulated cellulose-degrading enzymes such as cellobiohydrolase in several previous reports (Sinsabaugh et al., 2002; Frey et al., 2004). Other studies observed that N addition reduced leucine aminopeptidase activity (Sinsabaugh and Moorhead, 1994) and increased b-glucosidase activity (Chen et al., 2013). A possible explanation for the discrepancy is that different litter quality and quantity may lead to the different behavior of hydrolytic enzyme activities (Katsalirou et al., 2010). Also, Tischer et al. (2014) observed that b-glucosidase and cellobiohydrolase activities are related to abiotic factors controlling microbial biomass such as soil pH, N, and P availability, and climate condition (Vourlitis et al., 2021) which dramatically differed among our experimental sites. However, based on data, we can rule out the possibility of pH having a role on these enzymatic activities in our experimental sites (Supplementary Table S2).
Role of Soil pH in Mediating N Effects on Litter Decomposition
Earlier studies reported that soil acidification under increased N deposition is a common consequence of environmental pollution (Bobbink et al., 2003; Oulehle et al., 2008) resulting from indirect processes, such as nitrification. However, we only found a significant reduction of soil pH as a result of N addition in Capo Caccia BS, and this not significant trend also emerged in El Regajal. In contrast, we found a significant increase of soil pH responding to N addition in Arrábida. The contrasting results reported in this site for soil pH could be explained by the high levels of carbonates and bicarbonates found in the calcareous soil featured by this site (Table 1), which is typical from the Mediterranean regions (Dias et al., 2014).
The results of GLMM showed that N addition had no significant effect on decomposition processes when looking at all sites together whereas we found a significant effect of site, supporting the relevance of the site-specificity. When testing the effect of N addition, soil pH, and the interaction of both variables within each experimental site, the GLMM revealed contrasting results. This heterogeneity in the results can be explained by the different response of the soil processes to the N manipulation within each site due to their differences in soil physical-chemical properties as soil pH (Knorr et al., 2005; Vourlitis and Zorba, 2007; Hayakawa et al., 2014), and climatic conditions as aridity (Coùteaux et al., 1995; Aerts, 1997). Unfortunately, in our dataset aridity covaries with soil pH and total N so that the relative importance of climate and soil characteristics cannot be teased apart. It should be also reminded that above-ground community composition may have played a role (Ochoa-Hueso et al., 2019).
Soil pH could also be mediating the effect of N supply on the microbial community. Nitrogen addition reduced soil pH in Capo Caccia BS, which may have contributed to a decrease in the total fungi community in this site responding to N. Nitrogen pollution can cause soil acidification resulting in the mobilization of mineral aluminum (Vitousek et al., 1997; Aber et al., 1998) and changes in calcium and magnesium availability (Lucas et al., 2011). As a result, the soil microbial communities may become calcium or magnesium-limited or be indirectly affected by the aluminum toxicity. Furthermore, the increase in ions such as H+ and NH4+ due to N pollution could change the osmotic potentials in soil solution (Broadbent, 1965), which may directly poison the soil microbes (Kuperman and Edwards, 1997). Despite that N exceedance was not explicitly proved in our experimental sites, this is a plausible explanation for our findings.
Surprisingly, we found no relationship when analysing the correlations between pH and decomposition indexes (Table 6). Our results may not seem to be in line with most of the literature, which often reports a positive relationship between soil pH and decomposition rates because most of the studies have been performed in ecosystems with lower soil pH ranges (e.g., Condron et al., 1993; Motavalli et al., 1995) than those reported in our study. The relationship between soil pH and decomposition may differ depending on the soil pH values: at acidic pH the decomposition is slower with respect to soils with neutral pH; hence, in these margins, the relationship would be positive. This is an interesting finding that calls attention to the value ranges of pH. Besides, it is worth noting that we do not measure native litter decomposition but the TBI which allows to compare the potential decomposition rates of different sites due to soil bio-chemical properties, while eliminating the potential differences due to different litter qualities.
Role of Local Climatic Conditions as Drivers of the Ecosystem Responses to N Additions
Another key factor determining the differences in the TBIk and TBIS values among sites could be the local specific climatic conditions (Zhang et al., 2008). The experimental sites differ in terms of AI index values (Table 2), highlighting an important variability in soil temperature, humidity, and evapotranspiration during the studied decomposition period. Several studies have endorsed the ability of these climatic factors in regulating decomposition (Dyer et al., 1990; Aerts, 1997; O’Neill et al., 2003), indeed water availability becomes a relevant factor in determining litter decomposition in semi-arid regions where water is the main limiting factor (Coùteaux et al., 1995). Even if soil moisture measurements are a more accurate method, the calculated AI could explain the higher TBIk values found in Arrábida compared to the other two sites (Table 2) since this is the site least constrained by water (Tables 1, 2).
Conclusion
Our study provides further knowledge to understand contrasting ecosystem responses to N addition close to forecasted depositions. Our findings suggest that N effects on litter decomposition in very nutrient-poor ecosystems as Mediterranean shrublands depends on ecosystem local conditions, with responses that can be positive, negative, or null. Despite we performed an exhaustive analysis we cannot exclude the possibility of hidden patterns potentially showed by other interpretations of the results. Other soil variables, both biotic and abiotic, showed similar idiosyncratic responses to N addition, with soil pH being affected only in two out of three experimental sites. In the same line, no common pattern for litter decomposition process or other studied variables emerged among the control plots of the study sites. Such a high unpredictability determines a proportional uncertainty on the vulnerability of Mediterranean ecosystems at present and future N deposition rates. Our results demonstrate that responses of soil functioning to N deposition are site-dependent in Mediterranean areas, which contributes to explain the link between ecosystem local conditions and ecological impacts of the effect of N deposition.
Data Availability Statement
The original contributions presented in the study are included in the article/Supplementary Materials, further inquiries can be directed to the corresponding author.
Author Contributions
MLC: Conceptualization, Investigation, Formal analysis, Writing—Original Draft, Visualization, LM: Conceptualization, Writing—Original Draft, Visualization. RO-H: Methodology, Writing—Review and Editing. MD-B: Validation, Writing—Review and Editing. SMu: Validation, Writing—Review and Editing. JR: Validation, Writing—Review and Editing. DS: Resources, Funding acquisition, Review and Editing. CC: Resources, Writing—Review and Editing. AG: Resources, Writing—Review and Editing. EM: Resources, Writing—Review and Editing. MP-C Field activity, Writing—Review and Editing. TD: Editing. CS: Resources, Review and Editing. SMe: Supervision, Conceptualization, Writing—Review and Editing.
Funding
MLC received funding by grant from the Euro-Mediterranean Centre on Climate Change (CMCC) and received an Erasmus scholarship in collaboration with the Autonomous University of Madrid—Madrid, Spain (2016-1-IT02-KA103-022772) and an Ulisse scholarship in collaboration with the Western of Sydney University—Hawkesbury Institute for the Environment–Richmond, Australia (L.R. 3/2009.2015-2016). LM has received funding from the European Union’s Horizon 2020 research and innovation programme under the Marie Sklodowska-Curie Grant agreement No 793965. SM acknowledges financial support from European Union Seventh Framework Programme ((FP7/2007–2013) (FP7/2007–2011)) under grant agreement no. (301785) and FCT Investigator grant.
Conflict of Interest
The authors declare that the research was conducted in the absence of any commercial or financial relationships that could be construed as a potential conflict of interest.
Publisher’s Note
All claims expressed in this article are solely those of the authors and do not necessarily represent those of their affiliated organizations, or those of the publisher, the editors and the reviewers. Any product that may be evaluated in this article, or claim that may be made by its manufacturer, is not guaranteed or endorsed by the publisher.
Acknowledgments
The authors are very thankful to the owners of “Porto Conte Regional Natural Park”, and to “Finca El Regajal” and to “Arrábida Natural Park” for their kind allowance to work in their property.
Supplementary Material
The Supplementary Material for this article can be found online at: https://www.frontiersin.org/articles/10.3389/fenvs.2021.709391/full#supplementary-material
References
Aber, J., McDowell, W., Nadelhoffer, K., Magill, A., Berntson, G., Kamakea, M., et al. (1998). Nitrogen Saturation in Temperate Forest Ecosystems. BioScience 48, 921–934. doi:10.2307/1313296
Aerts, R. (1997). Climate, Leaf Litter Chemistry and Leaf Litter Decomposition in Terrestrial Ecosystems: A Triangular Relationship. Oikos 79, 439. doi:10.2307/3546886
Barrow, C. J. (1992). “World Atlas of Desertification (United Nations Environment Programme), Edited by N. Middleton and D. S. G. Thomas. Edward Arnold, London, 1992. Isbn 0 340 55512 2, £89.50 (Hardback), Ix + 69 Pp. Land Degrad. Dev. 3, 249. doi:10.1002/ldr.3400030407
Bobbink, R., Hicks, K., Galloway, J., Spranger, T., Alkemade, R., Ashmore, M., et al. (2010). Global Assessment of Nitrogen Deposition Effects on Terrestrial Plant Diversity: a Synthesis. Ecol. Appl. 20, 30–59. doi:10.1890/08-1140.1
Bobbink, R., Hornung, M., and Roelofs, J. G. M. (1998). The Effects of Air-Borne Nitrogen Pollutants on Species Diversity in Natural and Semi-natural European Vegetation. J. Ecol. 86, 717–738. doi:10.1046/j.1365-2745.1998.8650717.x
Broadbent, F. E. (1965). Effect of Fertilizer Nitrogen on the Release of Soil Nitrogen. Soil Sci. Soc. Am. J. 29, 692–696. doi:10.2136/sssaj1965.03615995002900060028x
Chen, H., Dong, S., Liu, L., Ma, C., Zhang, T., Zhu, X., et al. (2013). Effects of Experimental Nitrogen and Phosphorus Addition on Litter Decomposition in an Old-Growth Tropical Forest. PLoS One. 8, e84101. doi:10.1371/journal.pone.0084101
Chen, R., Senbayram, M., Blagodatsky, S., Myachina, O., Dittert, K., Lin, X., et al. (2014). Soil C and N Availability Determine the Priming Effect: Microbial N Mining and Stoichiometric Decomposition Theories. Glob. Change Biol. 20, 2356–2367. doi:10.1111/gcb.12475
Cheng, Y., Wang, J., Chang, S. X., Cai, Z., Müller, C., and Zhang, J. (2019). Nitrogen Deposition Affects Both Net and Gross Soil Nitrogen Transformations in forest Ecosystems: A Review. Environ. Pollut. 244, 608–616. doi:10.1016/j.envpol.2018.10.054
Condron, L. M., Tiessen, H., Trasar-Cepeda, C., Moir, J. O., and Stewart, J. W. B. (1993). Effects of Liming on Organic Matter Decomposition and Phosphorus Extractability in an Acid Humic Ranker Soil from Northwest Spain. Biol. Fertil. Soils. 15, 279–284. doi:10.1007/BF00337213
Coùteaux, M.-M., Bottner, P., and Berg, B. (1995). Litter Decomposition, Climate and Liter Quality. Trends Ecol. Evol. 10, 63–66. doi:10.1016/S0169-5347(00)88978-8
Currie, W. S. (1999). The Responsive C and N Biogeochemistry of the Temperate forest Floor. Trends Ecol. Evol. 14, 316–320. doi:10.1016/S0169-5347(99)01645-6
Curtin, D., Campbell, C. A., and Jalil, A. (1998). Effects of Acidity on Mineralization: pH-Dependence of Organic Matter Mineralization in Weakly Acidic Soils. Soil Biol. Biochem. 30, 57–64. doi:10.1016/S0038-0717(97)00094-1
Demoling, F., Ola Nilsson, L., and Bååth, E. (2008). Bacterial and Fungal Response to Nitrogen Fertilization in Three Coniferous forest Soils. Soil Biol. Biochem. 40, 370–379. doi:10.1016/j.soilbio.2007.08.019
Dias, T., Clemente, A., Martins-Loução, M. A., Sheppard, L., Bobbink, R., and Cruz, C. (2014). Ammonium as a Driving Force of Plant Diversity and Ecosystem Functioning: Observations Based on 5 years' Manipulation of N Dose and Form in a Mediterranean Ecosystem. PLoS One. 9, e92517. doi:10.1371/journal.pone.0092517
Dias, T., Oakley, S., Alarcón-Gutiérrez, E., Ziarelli, F., Trindade, H., Martins-Loução, M. A., et al. (2013). N-driven Changes in a Plant Community Affect Leaf-Litter Traits and May Delay Organic Matter Decomposition in a Mediterranean Maquis. Soil Biol. Biochem. 58, 163–171. doi:10.1016/j.soilbio.2012.10.027
Djukic, I., Kepfer-Rojas, S., Schmidt, I. K., Larsen, K. S., Beier, C., Berg, B., et al. (2018). Early Stage Litter Decomposition across Biomes. Sci. Total Environ. 628-629, 1369–1394. doi:10.1016/j.scitotenv.2018.01.012
Dyer, M. L., Meentemeyer, V., and Berg, B. (1990). Apparent Controls of Mass Loss Rate of Leaf Litter on a Regional Scale. Scand. J. For. Res. 5, 311–323. doi:10.1080/02827589009382615
EMEP (2015). EMEP/MSC-W_Reporting. Available at: http://www.emep.int/mscw/.
Fenn, M. E., Haeuber, R., Tonnesen, G. S., Baron, J. S., Grossman-Clarke, S., Hope, D., et al. (2003). Nitrogen Emissions, Deposition, and Monitoring in the Western United States. Bioscience 53, 391–403. doi:10.1641/0006-3568(2003)053[0391:nedami]2.0.co;2
Ferretti, M., Marchetto, A., Arisci, S., Bussotti, F., Calderisi, M., Carnicelli, S., et al. (2014). On the Tracks of Nitrogen Deposition Effects on Temperate Forests at Their Southern European Range - an Observational Study from Italy. Glob. Change Biol. 20, 3423–3438. doi:10.1111/gcb.12552
Fierer, N., Lauber, C. L., Ramirez, K. S., Zaneveld, J., Bradford, M. A., and Knight, R. (2012). Comparative Metagenomic, Phylogenetic and Physiological Analyses of Soil Microbial Communities across Nitrogen Gradients. ISME J. 6, 1007–1017. doi:10.1038/ismej.2011.159
Fowler, D., Coyle, M., Skiba, U., Sutton, M. A., Cape, J. N., Reis, S., et al. (2013). The Global Nitrogen Cycle in the Twenty-First century. Phil. Trans. R. Soc. B. 368, 20130164. doi:10.1098/rstb.2013.0164
Frey, S. D., Knorr, M., Parrent, J. L., and Simpson, R. T. (2004). Chronic Nitrogen Enrichment Affects the Structure and Function of the Soil Microbial Community in Temperate Hardwood and pine Forests. For. Ecol. Manag. 196, 159–171. doi:10.1016/j.foreco.2004.03.018
Galloway, J. N., Winiwarter, W., Leip, A., Leach, A. M., Bleeker, A., and Erisman, J. W. (2014). Nitrogen Footprints: Past, Present and Future. Environ. Res. Lett. 9, 115003. doi:10.1088/1748-9326/9/11/115003
Gauss, M., Nyíri, Á., Benedictow, A., and Klein, H. (2016). Transboundary Air Pollution by Main Pollutants (S, N, O3) and PM in 2014. Country Reports. Spain, Italy: EMEP/MSC-W Data Note 1/2016, 1–23. Available at: https://emep.int/publ/reports/2016/Country_Reports/report_TR.pdf.
Gerosa, G., Finco, A., Mereu, S., Marzuoli, R., and Ballarin-Denti, A. (2009). Interactions Among Vegetation and Ozone, Water and Nitrogen Fluxes in a Coastal Mediterranean Maquis Ecosystem. Biogeosciences 6, 1783–1798. doi:10.5194/bg-6-1783-2009
Hayakawa, C., Funakawa, S., Fujii, K., Kadono, A., and Kosaki, T. (2014). Effects of Climatic and Soil Properties on Cellulose Decomposition Rates in Temperate and Tropical Forests. Biol. Fertil. Soils. 50, 633–643. doi:10.1007/s00374-013-0885-4
He, J.-z., Shen, J.-p., Zhang, L.-m., Zhu, Y.-g., Zheng, Y.-m., Xu, M.-g., et al. (2007). Quantitative Analyses of the Abundance and Composition of Ammonia-Oxidizing Bacteria and Ammonia-Oxidizing Archaea of a Chinese upland Red Soil under Long-Term Fertilization Practices. Environ. Microbiol. 9, 2364–2374. doi:10.1111/j.1462-2920.2007.01358.x
Hobbie, S. E. (2005). Contrasting Effects of Substrate and Fertilizer Nitrogen on the Early Stages of Litter Decomposition. Ecosystems 8, 644–656. doi:10.1007/s10021-003-0110-7
Högberg, M. N., Bååth, E., Nordgren, A., Arnebrant, K., and Högberg, P. (2003). Contrasting Effects of Nitrogen Availability on Plant Carbon Supply to Mycorrhizal Fungi and Saprotrophs - a Hypothesis Based on Field Observations in Boreal forest. New Phytol. 160, 225–238. doi:10.1046/j.1469-8137.2003.00867.x
Högberg, M. N. (2006). Discrepancies between Ergosterol and the Phospholipid Fatty Acid 18:2ω6,9 as Biomarkers for Fungi in Boreal forest Soils. Soil Biol. Biochem. 38, 3431–3435. doi:10.1016/j.soilbio.2006.06.002
Högberg, M. N., Högberg, P., and Myrold, D. D. (2006). Is Microbial Community Composition in Boreal forest Soils Determined by pH, C-To-N Ratio, the Trees, or All Three? Oecologia 150, 590–601. doi:10.1007/s00442-006-0562-5
Jensen, M. E., Burman, R. D., and Allen, R. G. (1990). “Evapotranspiration and Irrigation Water Requirements,” in ASCE Manuals and Reports on Engineering Practice (New York: American Society of Civil Engineers), 360
Jiang, X., Hou, X., Zhou, X., Xin, X., Wright, A., and Jia, Z. (2015). pH Regulates Key Players of Nitrification in Paddy Soils. Soil Biol. Biochem. 81, 9–16. doi:10.1016/j.soilbio.2014.10.025
Katsalirou, E., Deng, S., Nofziger, D. L., and Gerakis, A. (2010). Long-term Management Effects on Organic C and N Pools and Activities of C-Transforming Enzymes in Prairie Soils. Eur. J. Soil Biol. 46, 335–341. doi:10.1016/j.ejsobi.2010.06.004
Kemmitt, S., Wright, D., Goulding, K., and Jones, D. (2006). pH Regulation of Carbon and Nitrogen Dynamics in Two Agricultural Soils. Soil Biol. Biochem. 38, 898–911. doi:10.1016/j.soilbio.2005.08.006
Keuskamp, J. A., Dingemans, B. J. J., Lehtinen, T., Sarneel, J. M., and Hefting, M. M. (2013). Tea Bag Index: a Novel Approach to Collect Uniform Decomposition Data across Ecosystems. Methods Ecol. Evol. 4, 1070–1075. doi:10.1111/2041-210X.12097
Kimball, S., Goulden, M. L., Suding, K. N., and Parker, S. (2014). Altered Water and Nitrogen Input Shifts Succession in a Southern California Coastal Sage Community. Ecol. Appl. 24, 1390–1404. doi:10.1890/13-1313.1
Knorr, M., Frey, S. D., and Curtis, P. S. (2005). Nitrogen Additions and Litter Decomposition: a Meta-Analysis. Ecology 86, 3252–3257. doi:10.1890/05-0150
Kuperman, R. G., and Edwards, C. A. (1997). “Effects of Acidic Deposition on Soil Invertebrates and Microorganisms” in, Effects of Acidic Deposition on Soil Invertebrates and Microorganisms BT - Reviews of Environmental Contamination and Toxicology: Continuation of Residue Reviews. Editors G. W. Ware, H. N. Nigg, and A. Bevenue (New York, NY: Springer New York), 35–138. doi:10.1007/978-1-4612-2264-4_2
Lehtovirta, L. E., Prosser, J. I., and Nicol, G. W. (2009). Soil pH Regulates the Abundance and Diversity of Group 1.1c Crenarchaeota. FEMS Microbiol. Ecol. 70, 367–376. doi:10.1111/j.1574-6941.2009.00748.x
Liu, L., and Greaver, T. L. (2010). A Global Perspective on Belowground Carbon Dynamics under Nitrogen Enrichment. Ecol. Lett. 13, 819–828. doi:10.1111/j.1461-0248.2010.01482.x
Liu, P., Huang, J., Sun, O. J., and Han, X. (2010). Litter Decomposition and Nutrient Release as Affected by Soil Nitrogen Availability and Litter Quality in a Semiarid Grassland Ecosystem. Oecologia 162, 771–780. doi:10.1007/s00442-009-1506-7
Lo Cascio, M., Morillas, L., Ochoa-Hueso, R., Munzi, S., Roales, J., Hasselquist, N. J., et al. (2017). Contrasting Effects of Nitrogen Addition on Soil Respiration in Two Mediterranean Ecosystems. Environ. Sci. Pollut. Res. 24, 26160–26171. doi:10.1007/s11356-017-8852-5
Lo Cascio, M., Ochoa Hueso, R., Lourdes, M., Manrique Reol, E., Munzi, S., Spano, D., et al. (2016). Nitrogen Deposition Impacts on Microbial Abundance and Decomposition in Three Mediterranean Sites: A Coordinated Study Using the NitroMed Network. doi:10.6084/m9.figshare.3554598.v1
Lucas, R. W., Klaminder, J., Futter, M. N., Bishop, K. H., Egnell, G., Laudon, H., et al. (2011). A Meta-Analysis of the Effects of Nitrogen Additions on Base Cations: Implications for Plants, Soils, and Streams. For. Ecol. Manag. 262, 95–104. doi:10.1016/j.foreco.2011.03.018
Manning, P., Saunders, M., Bardgett, R. D., Bonkowski, M., Bradford, M. a., Ellis, R. J., et al. (2008). Direct and Indirect Effects of Nitrogen Deposition on Litter Decomposition. Soil Biol. Biochem. 40, 688–698. doi:10.1016/j.soilbio.2007.08.023
Morillas, L., Bellucco, V., Lo Cascio, M., Marras, S., Spano, D., and Mereu, S. (2017). Contribution of Biological Crust to Soil CO2 Efflux in a Mediterranean Shrubland Ecosystem. Geoderma 289, 11–19. doi:10.1016/j.geoderma.2016.11.012
Motavalli, P. P., Palm, C. A., Parton, W. J., Elliott, E. T., and Frey, S. D. (1995). Soil pH and Organic C Dynamics in Tropical forest Soils: Evidence from Laboratory and Simulation Studies. Soil Biol. Biochem. 27, 1589–1599. doi:10.1016/0038-0717(95)00082-p
Munzi, S., Ochoa-Hueso, R., Gerosa, G., and Marzuoli, R. (2017). (E)merging Directions on Air Pollution and Climate Change Research in Mediterranean Basin Ecosystems. Environ. Sci. Pollut. Res. 24, 26155–26159. doi:10.1007/s11356-017-0688-5
Nave, L. E., Vance, E. D., Swanston, C. W., and Curtis, P. S. (2009). Impacts of Elevated N Inputs on north Temperate forest Soil C Storage, C/N, and Net N-Mineralization. Geoderma 153, 231–240. doi:10.1016/j.geoderma.2009.08.012
Nicol, G. W., Leininger, S., Schleper, C., and Prosser, J. I. (2008). The Influence of Soil pH on the Diversity, Abundance and Transcriptional Activity of Ammonia Oxidizing Archaea and Bacteria. Environ. Microbiol. 10, 2966–2978. doi:10.1111/j.1462-2920.2008.01701.x
Nilsson, L. O., and Wallander, H. (2003). Production of External Mycelium by Ectomycorrhizal Fungi in a norway spruce forest Was Reduced in Response to Nitrogen Fertilization. New Phytol. 158, 409–416. doi:10.1046/j.1469-8137.2003.00728.x
Ochoa-Hueso, R., Delgado-Baquerizo, M., An King, P. T., Benham, M., Arca, V., and Power, S. A. (2019). Ecosystem Type and Resource Quality Are More Important Than Global Change Drivers in Regulating Early Stages of Litter Decomposition. Soil Biol. Biochem. 129, 144–152. doi:10.1016/j.soilbio.2018.11.009
Ochoa-Hueso, R., Hernandez, R. R., Pueyo, J. J., and Manrique, E. (2011). Spatial Distribution and Physiology of Biological Soil Crusts from Semi-arid central Spain Are Related to Soil Chemistry and Shrub Cover. Soil Biol. Biochem. 43, 1894–1901. doi:10.1016/j.soilbio.2011.05.010
O’Neill, E. G., Johnson, D. W., Ledford, J., and Todd, D. E. (2003). Acute Seasonal Drought Does Not Permanently Alter Mass Loss and Nitrogen Dynamics during Decomposition of Red maple (Acer Rubrum L.) Litter. Glob. Chang. Biol. 9, 117–123. doi:10.1046/j.1365-2486.2003.00538.x
Oulehle, F., McDowell, W. H., Aitkenhead-Peterson, J. A., Krám, P., Hruška, J., Navrátil, T., et al. (2008). Long-term Trends in Stream Nitrate Concentrations and Losses across Watersheds Undergoing Recovery from Acidification in the Czech Republic. Ecosystems 11, 410–425. doi:10.1007/s10021-008-9130-7
Pardo, L. H., Fenn, M. E., Goodale, C. L., Geiser, L. H., Driscoll, C. T., Allen, E. B., et al. (2011). Effects of Nitrogen Deposition and Empirical Nitrogen Critical Loads for Ecoregions of the United States. Ecol. Appl. 21, 3049–3082. doi:10.1890/10-2341.1
Pennanen, T., Liski, J., Bååth, E., Kitunen, V., Uotila, J., Westman, C. J., et al. (1999). Structure of the Microbial Communities in Coniferous Forest Soils in Relation to Site Fertility and Stand Development Stage. Microb. Ecol. 38, 168–179. doi:10.1007/s002489900161
Phoenix, G. K., Hicks, W. K., Cinderby, S., Kuylenstierna, J. C. I., Stock, W. D., Dentener, F. J., et al. (2006). Atmospheric Nitrogen Deposition in World Biodiversity Hotspots: the Need for a Greater Global Perspective in Assessing N Deposition Impacts. Glob. Chang. Biol. 12, 470–476. doi:10.1111/j.1365-2486.2006.01104.x
Pregitzer, K. S., Burton, A. J., Zak, D. R., and Talhelm, A. F. (2007). Simulated Chronic Nitrogen Deposition Increases Carbon Storage in Northern Temperate Forests. Glob. Chang. Biol. 14, 142–153. doi:10.1111/j.1365-2486.2007.01465.x
Pritsch, K., Courty, P. E., Churin, J.-L., Cloutier-Hurteau, B., Ali, M. A., Damon, C., et al. (2011). Optimized Assay and Storage Conditions for Enzyme Activity Profiling of Ectomycorrhizae. Mycorrhiza 21, 589–600. doi:10.1007/s00572-011-0364-4
Robson, A. D. (1989). Soil Acidity and Plant Growth. Elsevier. doi:10.1016/B978-0-12-590655-5.X5001-4
Shen, J.-p., Zhang, L.-m., Zhu, Y.-g., Zhang, J.-b., and He, J.-z. (2008). Abundance and Composition of Ammonia-Oxidizing Bacteria and Ammonia-Oxidizing Archaea Communities of an Alkaline sandy Loam. Environ. Microbiol. 10, 1601–1611. doi:10.1111/j.1462-2920.2008.01578.x
Sinsabaugh, R. L., Carreiro, M. M., and Repert, D. A. (2002). Allocation of Extracellular Enzymatic Activity in Relation to Litter Composition, N Deposition, and Mass Loss. Biogeochemistry 60, 1–24. doi:10.1023/A:1016541114786
Sinsabaugh, R. L., and Moorhead, D. L. (1994). Resource Allocation to Extracellular Enzyme Production: A Model for Nitrogen and Phosphorus Control of Litter Decomposition. Soil Biol. Biochem. 26, 1305–1311. doi:10.1016/0038-0717(94)90211-9
Sun, T., Dong, L., and Mao, Z. (2015). Simulated Atmospheric Nitrogen Deposition Alters Decomposition of Ephemeral Roots. Ecosystems 18, 1240–1252. doi:10.1007/s10021-015-9895-4
Tian, X.-F., Hu, H.-W., Ding, Q., Song, M.-H., Xu, X.-L., Zheng, Y., et al. (2014). Influence of Nitrogen Fertilization on Soil Ammonia Oxidizer and Denitrifier Abundance, Microbial Biomass, and Enzyme Activities in an alpine Meadow. Biol. Fertil. Soils. 50, 703–713. doi:10.1007/s00374-013-0889-0
Tischer, A., Blagodatskaya, E., and Hamer, U. (2014). Extracellular Enzyme Activities in a Tropical Mountain Rainforest Region of Southern Ecuador Affected by Low Soil P Status and Land-Use Change. Appl. Soil Ecol. 74, 1–11. doi:10.1016/j.apsoil.2013.09.007
Treseder, K. K. (2008). Nitrogen Additions and Microbial Biomass: a Meta-Analysis of Ecosystem Studies. Ecol. Lett. 11, 1111–1120. doi:10.1111/j.1461-0248.2008.01230.x
USDA (1999). Soil Taxonomy. A Basic System of Soil Classification for Making and Interpreting Soil Surveys. 1975. 754 pp., 12 Coloured Plates. Agriculture Handbook No. 436. Soil Conservation Service, U.S. Department of Agriculture. From Superintendent of Documents. U. Geol. Mag. 114, 492. doi:10.1017/S0016756800045489
Vitousek, P. M., Aber, J. D., Howarth, R. W., Likens, G. E., Matson, P. A., Schindler, D. W., et al. (1997). Human Alteration of the Global Nitrogen Cycle: Sources and Consequences. Ecol. Appl. 7, 737–750. doi:10.1890/1051-0761(1997)007[0737:HAOTGN]2.0.CO;2
Vourlitis, G. L., and Fernandez, J. S. (2015). Carbon and Nitrogen Mineralization of Semi-arid Shrubland Soils Exposed to Chronic Nitrogen Inputs and Pulses of Labile Carbon and Nitrogen. J. Arid Environments. 122, 37–45. doi:10.1016/j.jaridenv.2015.06.007
Vourlitis, G. L., Kirby, K., Vallejo, I., Asaeli, J., and Holloway, J. M. (2021). Potential Soil Extracellular Enzyme Activity Is Altered by Long-Term Experimental Nitrogen Deposition in Semiarid Shrublands. Appl. Soil Ecol. 158, 103779. doi:10.1016/j.apsoil.2020.103779
Vourlitis, G. L., and Zorba, G. (2007). Nitrogen and Carbon Mineralization of Semi-arid Shrubland Soil Exposed to Long-Term Atmospheric Nitrogen Deposition. Biol. Fertil. Soils. 43, 611–615. doi:10.1007/s00374-006-0137-y
Waldrop, M. P., Zak, D. R., and Sinsabaugh, R. L. (2004). Microbial Community Response to Nitrogen Deposition in Northern forest Ecosystems. Soil Biol. Biochem. 36, 1443–1451. doi:10.1016/j.soilbio.2004.04.023
Xu, Y.-g., Yu, W.-t., Ma, Q., and Zhou, H. (2012). Responses of Bacterial and Archaeal Ammonia Oxidisers of an Acidic Luvisols Soil to Different Nitrogen Fertilization Rates after 9 Years. Biol. Fertil. Soils. 48, 827–837. doi:10.1007/s00374-012-0677-2
Zeng, J., Liu, X., Song, L., Lin, X., Zhang, H., Shen, C., et al. (2016). Nitrogen Fertilization Directly Affects Soil Bacterial Diversity and Indirectly Affects Bacterial Community Composition. Soil Biol. Biochem. 92, 41–49. doi:10.1016/j.soilbio.2015.09.018
Zhang, D., Hui, D., Luo, Y., and Zhou, G. (2008). Rates of Litter Decomposition in Terrestrial Ecosystems: Global Patterns and Controlling Factors. J. Plant Ecol. 1, 85–93. doi:10.1093/Jpe/Rtn002
Keywords: air pollution, anthropogenic disturbance, coordinated research networks, mediterranean semiarid ecosystems, soil extracellular enzymatic activity, soil organic matter decomposition, spatial and temporal heterogeneity, tea bag index
Citation: Lo Cascio M, Morillas L, Ochoa-Hueso R, Delgado-Baquerizo M, Munzi S, Roales J, Spano D, Cruz C, Gallardo A, Manrique E, Pérez-Corona ME, Dias T, Sirca C and Mereu S (2021) Nitrogen Deposition Effects on Soil Properties, Microbial Abundance, and Litter Decomposition Across Three Shrublands Ecosystems From the Mediterranean Basin. Front. Environ. Sci. 9:709391. doi: 10.3389/fenvs.2021.709391
Received: 13 May 2021; Accepted: 27 July 2021;
Published: 10 August 2021.
Edited by:
Alexandre Anesio, Aarhus University, DenmarkReviewed by:
Daryl Lee Moorhead, University of Toledo, United StatesGeorge Vourlitis, California State University San Marcos, United States
Copyright © 2021 Lo Cascio, Morillas, Ochoa-Hueso, Delgado-Baquerizo, Munzi, Roales, Spano, Cruz, Gallardo, Manrique, Pérez-Corona, Dias, Sirca and Mereu. This is an open-access article distributed under the terms of the Creative Commons Attribution License (CC BY). The use, distribution or reproduction in other forums is permitted, provided the original author(s) and the copyright owner(s) are credited and that the original publication in this journal is cited, in accordance with accepted academic practice. No use, distribution or reproduction is permitted which does not comply with these terms.
*Correspondence: Mauro Lo Cascio, mlocascio@uniss.it
†These authors contributed equally to this work