- Department of Civil and Environmental Engineering, Yonsei University, Seoul, South Korea
Multiplicity and complexity in sources account for polycyclic aromatic hydrocarbons (PAHs) in soil and health risk levels in industrial zones. In the present study, cancer risks (CR) for soil-bound carcinogenic PAHs were estimated and compared for the first time in seven different land-use areas adjacent to an industrial zone (Ulsan) in Korea. The entire study area has been recognized as a “low CR” zone (10−6 < value < 10−4). Hence, all land-use areas were found to have significant (>10−6) CR levels, except for an area used to store ore and iron scraps. Estimated CR levels were highest in the railroad area (RA) and traffic area (TA), followed by those in the industrial area (IA). In addition, exposure through dermal absorption (61–70%) and ingestion (21–39%) were the most common factors for CR levels in the study area. Among all health parameters, exposure duration, body weight, and open skin surface area were distinguished as most sensitive to total CR levels. Moreover, among all carcinogenic PAHs, indeno[1,2,3-c,d]pyrene and benzo[a]pyrene were most sensitive to CR levels. Creosote, which was utilized in railroad ties in RA and vehicular exhaust emission in TA, was classified as a source of soil-bound carcinogenic PAHs. Therefore, CR levels resulting from transportation activities were found to be two to three times higher than those obtained from industrial processes. Transportation activities in urban areas mostly serve to provide rapid and comfortable carriage for commuters. However, these facilities were mostly responsible for potential carcinogen exposure. This study directly challenges the conventional perception that industrial zones are the most polluted areas, especially when compared to transportation zones in urban areas. These findings can help local and national governments to better manage resources and maintain an economic balance.
Introduction
Industries are the backbone of a country's economy. It is a common belief that industrial areas (IA) are more polluted than urban areas. Currently, the Korean Government is spending substantial funds to clean up industrial zones. Government policies on industrial disposal management, emission control techniques, and reuse and recycle systems have provided more sustainability within the industrial process system. However, Korean city managements are facing problems due to rapid urbanization, dense population, and waste volume. Although cities have several sources of pollution, people are still migrating toward them. Moreover, cities are surrounded by high traffic density zones. Soils from dense traffic areas (TA) are enriched with more carcinogenic polycyclic aromatic hydrocarbons (PAHs), as compared to soils from other areas (Kim et al., 2019). Growing concentrations of soil-bound carcinogenic PAHs require attention from the scientific community in Korea.
Persistent organic pollutants such as PAHs are notable for their cancer-causing and mutagenic properties because of the fused aromatic rings in the structure (U.S. EPA, 2003). Due to hydrophobicity and stable chemical structure, the accumulation of PAHs onto the soil organic components is rapid (Abdel-Shafy and Mansour, 2016). The soil-bound PAHs are direct and indirectly exposed in the human body (Roy et al., 2017; Tarafdar et al., 2018; Ambade et al., 2021a; Ambade et al., 2021b; Ambade et al., 2021c; Ambade et al., 2021d). Vehicular exhaust emissions, fossil fuel combustions, excessive utilization of manufactured composts for horticulture, and industrial pyrolysis are the major sources of PAHs in soil environments (Bosetti et al., 2007; Arora and Reddy, 2013; Zhang et al., 2015; Ambade et al., 2020; Kumar et al., 2020; Ambade and Sethi, 2021). Moreover, long-term exposures to PAHs are responsible for development of cancerous tumors in human organs (IARC, 2010).
Monte Carlo simulations are a technique to analyze probabilistic uncertainty. The parameter values for exposure equations are randomly selected from distributions generated from the probability density functions for individual input parameters (Roy et al., 2019c; Roy et al., 2019d; Tarafdar et al., 2018). Hence, the role of these parameters in determining the total health risk can be established using sensitivity analysis (Roy et al., 2020). Exposure through ingestion, inhalation, and dermal absorption are the three routes by which PAHs in the soil enter the human body. Deposition and absorption of PAHs carrying particles on open surfaces of human skin, bio-accumulation of soil-bound PAHs in food, and soil particles erosion from the earth surface followed by inhalation are the common ways of human exposures. Apart from the PAHs levels in the soil, other age-specific risk assessment parameters such as body weight (BW), exposure durations (ED), exposure frequency (EF), skin surface area (SA), and inhalation rate are the most important factors for the risk value (Armstrong et al., 2004; Tarafdar et al., 2018; Tarafdar and Sinha, 2017a; Tarafdar and Sinha, 2017b; Roy et al., 2019a; Roy et al., 2019b; Roy et al., 2019c; Roy et al., 2019d). The role of those parameters in the total health risk levels can be determined through sensitivity analysis. Globally, Monte Carlo probabilistic approaches with sensitivity analysis are in common practice to estimate the human health risk levels (Roy et al., 2020; Tarafdar and Sinha, 2017a; Tarafdar and Sinha, 2017b).
Previous studies have focused on the monitoring and distribution of PAHs in agricultural soil, source identification of toxic PAHs, PAH levels in soils and sediments in coastal areas, and PAH levels in coastal fish. Recently, higher PAH levels in roadside soils at traffic-dense regions were observed in Ulsan (Jeon and Oh, 2019; Kim et al., 2019). At present, detailed investigations are required for source identification and health risk levels due to soil-bound carcinogenic PAHs over various land-use area types. Overall, detailed investigations and comparisons of cancer risk (CR) levels are still required for soil-bound PAHs across various land-use areas near industrial zones. The current study aims to reduce research gaps through the following objectives: 1) compare CR levels in soil-bound PAHs for various land-use areas in industrial complex, 2) evaluate the impact of transportation [railroad area (RA) and TA] on CR levels, and 3) identify the most sensitive parameters and significant exposure routes for CR levels.
Methodology
Data Collection and Source Identification of PAHs
This study focused on CR assessment for soil-bound PAHs at various land-use areas near an industrial cluster in Korea. Previous published data (Jeon and Oh, 2019) on soil-bound PAHs were acquired to calculate CR levels: naphthalene (NaP), acenaphthylene (AcPy), acenaphthene (AcP), fluorene (Flu), phenanthrene (PhA), anthracene (AnT), fluoranthene (FluA), pyrene (Pyr), benzo[a]anthracene (BaA), chrysene (Chr), benzo[b]fluoranthene (BbF), benzo[k]fluoranthene (BkF), benzo[a]pyrene (BaP), indeno[1,2,3-c,d]pyrene (InP), dibenz[a,h]anthrathene (DbA), and benzo[g,h,i]perylene (BghiP). The seven different land-use areas near Ulsan city and its IA were taken from the previous study. The land-use areas were as follows: waste treatment facilities area (WA), TA, child playground area (CA), IA, RA, ore and iron scraps area (OA), and residential area (ReA).
Interactions within the source delivering the PAHs directly influence the PAH profile. The diagnostic ratios used to trace PAH sources were generated from pyrolytic or petrogenic combustion, as shown in Table 1. PAHs such as AnT (AnT: 178) and flouranthene (FluA: 202) are commonly used to distinguish between combustion and petroleum sources (Budzinski et al., 1997; Soclo et al., 2000). AnT/(AnT + FluA) ratios <0.1 and >0.1 are generally indicative of a petroleum and combustion source, respectively (Budzinski et al., 1997). For Fl/(Fl + Py) ratios, <0.5, 0.4–0.5, and >0.5 indicate petroleum/combustion, liquid fossil fuel combustion, and coal/wood/grass combustion sources, respectively (Budzinski et al., 1997). Moreover, the IP/[IP + B(ghi)P] ratios of <0.2, 0.2–0.5, and >0.5 represent the petroleum, liquid fossil fuel, and coal/wood/grass combustion sources, respectively. Finally, the BaA/(BaA + Chry) ratios of <0.2 and >0.2 indicate petroleum and pyrogenic/coal combustions, respectively (Budzinski et al., 1997).
Human CR Assessment
Human CRs were assessed from the exposure of the 16 carcinogenic PAHs in soil. The relative potency equivalency factor (PEF) to BaP was used to convert each PAH concentration level into BaP equivalent (BaPequ) (U.S. EPA, 1993). Here, the following assumptions were used: 1)target age groups: 18 (teen) and 70 (adult); 2) exposure path: ingestion (ing), dermal absorption (derm), and inhalation (inh); 3) the BaPequ value was considered for each PAH to calculate CR levels; and 4) risk assessment parameters such as air inhalation rate (HR), ED, BW, and soil ingestion rate (IRd) values were used based on Korean standard values (Table 2). Other model parameter values were taken from the standard values reported by the US Environmental Protection Agency (USEPA) (U.S. EPA, 2011). The chronic daily intake (CDI) of PAHs was calculated as CDIing, CDIinh, and CDIderm. The equations used to calculate CDI, CR levels for individual exposure paths, and total cancer risk [CR(Total)] levels resulting from exposure to soils are shown in Table 3.
The Monte Carlo simulation tool from Oracle's Crystal Ball (11.1.2.4.600) was used to calculate CR levels. Determining CR levels using Monte Carlo simulations for soil-bound PAHs is common in literature (Qu et al., 2015; Roy et al., 2020), as Monte Carlo simulations help in analyzing probabilistic uncertainty. Risk value was calculated with 50,000 iterations along with a sensitivity analysis. Apart from the PAHs levels in the soil, other age-specific risk assessment parameters such as BW, ED, HR, EF, and SA were important considerations in determining risk (Table 2). Monte Carlo simulations and sensitivity analysis are universally used for CR analysis (e.g., Chen and Liao, 2006; Williams et al., 2013; Arora and Reddy, 2014, 2015; Gungormus et al., 2014; Tarafdar and Sinha, 2017a; Tarafdar and Sinha, 2017b; Roy et al., 2020). According to USEPA, CR value >10−6 is considered as a significant level for CR (Wang et al., 2011; Li et al., 2014). The lifetime CR levels can be explained as very low, CR ≤ 10−6; low, 10−6 < CR < 10−4; moderate, 10−4 ≤ CR < 10−3; high, 10−3 ≤ CR < 10−1; and very high, CR ≥ 10−1 (NYSDOH, 2012).
Uncertainty and Variability Analysis
Variability and uncertainty are part of risk assessment processes, and they should be dealt with exclusively. Isolating these ideas in a reenactment drives us to distinguish the variety all the more precisely in the prediction because of limited knowledge and natural variations in the computation. Monte Carlo simulation from Crystal Ball 2D Simulation tool runs an outer loop to simulate the uncertainty values. To simulate the variability, the uncertainty values freeze while it runs an inner loop (of the whole model). This process repeats for hundred times to get the forecast distribution patterns. The 2D simulation output was compared with the previously done one-dimensional simulation results for both variability and uncertainty.
Moreover, deduction of CR induces uncertainties because of varieties in the health hazard evaluation parameters utilized in the estimations. Thus, for the parameters like ED, IRd, BW, and HR, Korean standard values were used to reduce uncertainty. The sample assortment and investigation techniques additionally might have brought about uncertainty in CR assessment; specifically, the disappearance or addition of PAHs in the cooking activity was not taken into account.
Results and Discussion
Concentration of Soil-Bound PAHs and Source Identification
The concentrations of soil-bound PAHs exhibited a wide range. The estimated soil-bound Ʃ 16 PAH concentrations were highest in RA (mean: 13,124 µg/kg), followed by those in IA (mean: 5,130 µg/kg), TA (mean: 3,728 µg/kg), and WA (mean: 3,724 µg/kg), as shown in Supplementary Figure S1A. Korean standards for soil-bound PAH levels are still unpublished. Other countries like Mexican (0–6 µg/g), Polish (0.02–0.05 µg/g), and Dutch (0.02–0.05 µg/g) standards have been compared for this study (Malawska 2000; Ray et al., 2008). As per Polish standards, OA and ReA were classified as low-pollution zones. Meanwhile, RA, IA, TA, and WA were classified as highly polluted sites.
The mean values of Ʃ 16 PAHs ranged from 368.5 to 13,152 µg/kg throughout the study area. At RA, Pyr + BbF levels exhibited a maximum of 16% among all PAHs. BbF was found to be highest in IA and WA, with a mean value of 766 and 472 µg/kg, respectively. In TA, concentration levels of InP were the highest (702 µg/kg) among all PAHs. Total PAHs as BaP toxic equivalent concentration (ƩBaPequ) levels were estimated to exhibit a range of 44–1,930 µg TEQ/kg. The ƩBaPequ value was highest in RA (mean: 1,930 µg TEQ/kg), followed by that in TA (mean: 973 µg TEQ/kg), IA (mean: 781 µg TEQ/kg), and WA (mean: 633 µg TEQ/kg). Moreover, BaPequ values were largest for BaP (mean: 857.5 µg TEQ/kg), followed by those in InP (583.5 µg TEQ/kg), as presented in Supplementary Figure S1B. The (BaP + InP) levels were responsible for 1.5 times higher ƩBaPequ values in TA, although IA exhibited higher Ʃ 16 PAHs levels than those in TA. Furthermore, BaP + InP (as BaPequ) contributed 88%, 80%, 78%, and 75% to ƩBaPequ levels in TA, WA, IA, and RA, respectively. In the previous study higher PAHs levels were reported in Ulsan than those for other cities in Korea, Tokushima (Japan), Bangkok (Thailand), and Tarragona (Spain) (Jeon and Oh, 2019). Previous literature reported that numerous modern industrial units and substantial traffic near the Ulsan IA caused higher than normal ƩPAH (Lee and Dong, 2009; Yoo et al., 2010).
For sources, a diagnostic ratio analysis primarily identified the petrogenic products, petroleum combustion (diesel), coal/soft wood combustion, and petrogenic/coal combustion (gasoline) throughout the study area (Table 1). In RA, sources related to petrogenic products, petroleum combustion (diesel), and petrogenic/coal combustion (gasoline) IP/(IP + BghiP): 0.46; BaA/(BaA + Chr): 0.41; Ant/(Ant + Phe): 0.23; and Fla/(Fla + Pyr): 0.46 were the largest contributors to PAHs in soil. The elevated amount of ƩPAH in RA might be clarified using creosote as a wood additive for railroad ties. In previous research, high ƩPAH levels were also reported for RA (Baek, 2013). Railroad car movement and inadvertent fuel release are potential sources of PAHs in RA (Kim and Shin, 2001). Petroleum combustion (diesel), petrogenic/coal combustion (gasoline), and petrogenic products were also identified as major sources of soil-bound PAHs in TA. Pha, FluA, and BghiP, which are signature compounds in soils of dense TA, were found to be significant in TA (Kim et al., 2019). Except for RA, ƩPAH levels in WA and IA were two to three times higher compared to those in other areas; this was likely due to the wastes generated by petrochemical plants and their modern processing operations. In IA, IP/(IP + BghiP), BaA/(BaA + Chr), Ant/(Ant + Phe), and Fla/(Fla + Pyr) ratios were high (0.6, 0.6, 0.4, and 0.51, respectively). Sources related to industrial activities such as combustion [coal/soft wood combustion and petrogenic/coal combustion (gasoline)] and petrogenic products were mostly observed in IA.
In the Ulsan IA, petrochemical, automobile, shipbuilding, and oil refining are the primary industries that contribute to PAHs in soil. Combustion sources (coal, wood, petroleum) and petrogenic product sources were associated with soil pollution levels in WA. The petrogenic product and combustion sources were also commonly identified in CA, OA, and ReA. Moreover, traffic and industrial sources primarily contributed to soil pollution in ReA and CA. In particular, diesel, gasoline, and heavy oil combustion have been identified as major sources of soil-bound PAHs (Kwon and Choi, 2014). Ulsan is well known for industrial clusters in Korea, containing refineries, non-ferrous metal smelting plants, petrochemical and automobile factories, shipbuilding complexes, and their vendors (Kwon and Choi, 2014).
The combustion of petrochemical products and direct emission of PAHs are possible sources of ƩPAH in the study area (Kim and Shin, 2001; Chung et al., 2006). Two big refinery units are situated in Ulsan, and a lot of raw petroleum is imported to the Port of Ulsan. Therefore, storage, distribution (pipelines), and transportation (ground) processes are potential sources of ƩPAH (Jeon and Oh, 2019).
Human Health Risk Levels
The ƩPAH concentration (BaPequ) was used as an input for the Monte Carlo simulations that calculated the 95% confidence levels for CR in the teen and adult age groups (Figure 1). Seven different land-use areas near the Ulsan industrial complex were considered for the CR assessment. Except for OA, CR(Total) for all other six areas was significant (>10−6), and these areas were classified as low CR zones (Figure 2). The highest CR(Total) was found in RA (95% CL: 4.13E−05 for teen; 3.81E−05 for adult), followed by that in TA (95% CL: 2.10E−05 for teen; 1.89E−05 for adult), IA (95% CL: 1.69E−05 for teen; 1.52E−05 for adult), and WA (95% CL: 1.35E−05 for teen; 1.23E−05 for adult). ReA was identified as a significant CR zone but exhibited the second-lowest CR levels among the seven areas. Compared to ReA, CR levels were estimated to be 19, 10, 8, 6, and 2 times higher in RA, TA, IA, WA, and CA, respectively. OA was not only insignificant (<10−6) but also exhibited a CR level two times lower than that of ReA.
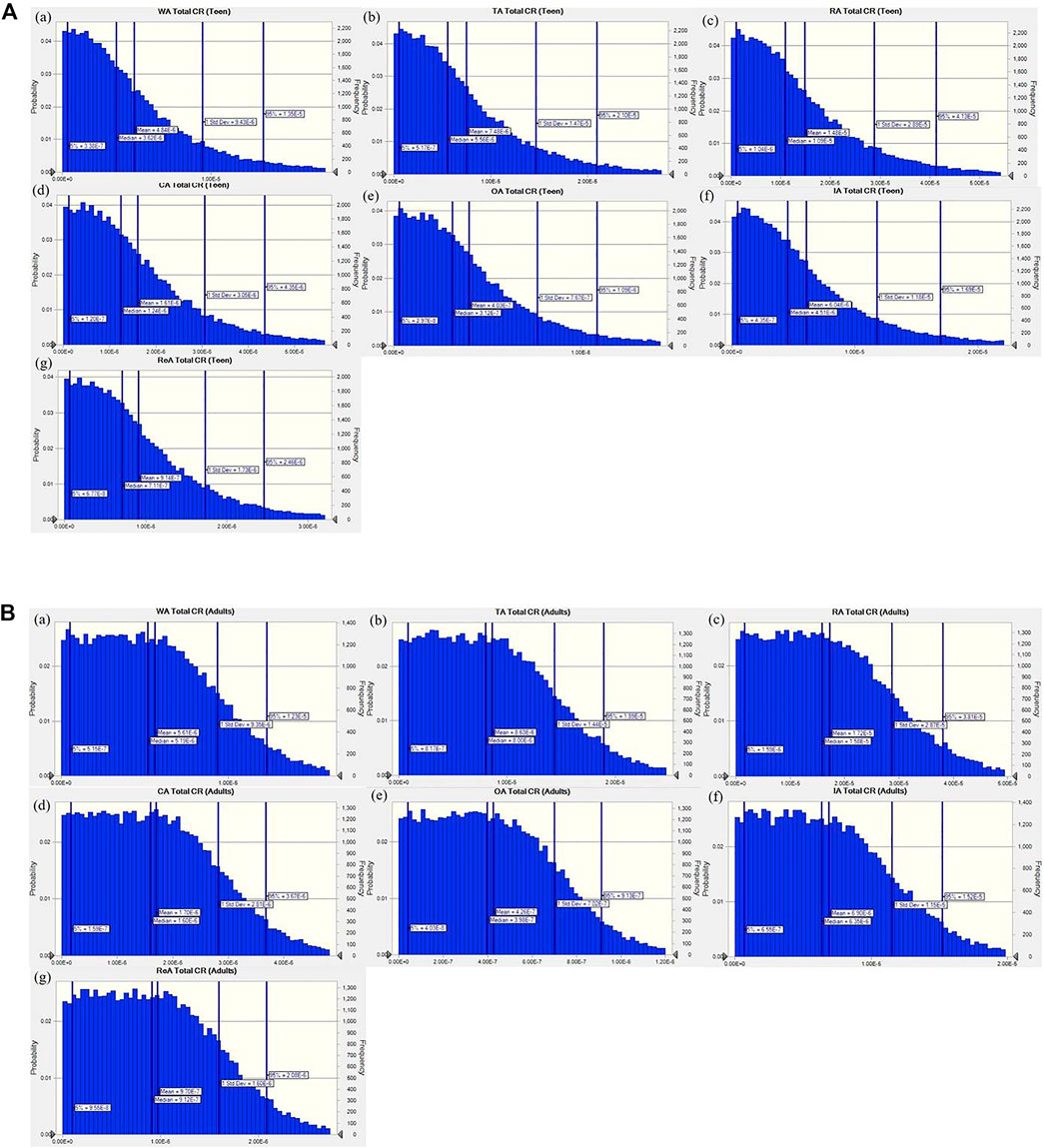
FIGURE 1. (A) Probability density functions from Monte Carlo simulations used to predict CR (95% confidence level) from soil-bound PAHs for the teen group at (a) WA, (b) TA, (c) RA, (d) CA, (e) OA, (f) IA, and (g) ReA. (B) Probability density functions from Monte Carlo simulations used to predict CR (95% confidence level) from soil-bound PAHs for the adult group at (a) WA, (b) TA, (c) RA, (d) CA, (e) OA, (f) IA, and (g) ReA.
Throughout the study area, CR levels through dermal exposure (adult: 79%; teen: 61%) and ingestion (teen: 39%; adult: 21%) were most significant (Supplementary Figure S2). Hence, CR through inhalation exposure was determined to be insignificant (10−10–10−11). Kim et al. (2019) also reported insignificant CR levels through inhalation exposure in Korea. Additionally, the teen group exhibited a higher CR level than the adult group. High concentrations of soil-bound total PAHs and carcinogenic PAHs were responsible for the elevated CR levels in RA. Moreover, the presence of higher carcinogenic PAH (InP and BaP) levels was responsible for higher CR in RA. Likewise, higher InP and BaP levels were identified as the most probable PAHs that explained higher CR levels in TA than in IA. The diagnostic ratio analysis showed that the combustion of petroleum (diesel) and petrogenic (gasoline) were the major contributors other than petrogenic products. A significant correlation between traffic volume and CR levels was reported by Kim et al. (2019).
Overall, industrial activity can still be a major cause for elevated CR levels in IA. Oil refining, automobile manufacturing, petrochemical processes, shipbuilding, and smelting plants can all raise CR levels. Sustainable development with resource recovery and reuse are potential techniques to reduce pollution loads in IA. Moreover, fossil-fuel-free transportation systems can reduce PAH levels in TA. In Seoul, CR levels for soil-bound PAHs were reported in the range of 1.245E−06 to 1.357E−05 for children in playground dust (Tarafdar et al., 2020). This level is three and five times higher than the CA and ReA, respectively in Ulsan. Although Ulsan is a well-known industrial hub in Korea, RA and TA were identified as having the highest CR levels among the seven land-use areas. This outcome can change the conventional perceptions of Korea's residents and policymakers.
Sensitivity Analysis
A Monte Carlo analysis was conducted to identify the factors that are most sensitive to the CR levels (Figure 3). Sensitivity test results are shown in Table 4. The sensitivity to CR levels for all risk assessment parameters was evaluated. For the teen group, total CR levels were estimated to be most sensitive to the following parameters: ED (63%), BW (−26%), SA (6%), cancer slope factor for BaP (CSFing; 3%), and dermal absorption factor (ABS; 2%). For the adult group, total CR levels were most sensitive to ED (87%), ABS (6%), BW (−5%), and SA (1%). BW was determined to be negatively sensitive to CR levels. ED (63–87%) was the most sensitive among all exposure routes (ingestion, dermal absorption, and inhalation) and age groups. The significant role of ED on CR levels has been previously reported (Chen and Liao, 2006; Chiang et al., 2009; Tarafdar and Sinha, 2017a). The negative BW value indicated the negative relationship between BW and total CR levels (Tarafdar and Sinha, 2017b; Chawada et al., 2020). For CRderm and CRing levels, ED + BW was approximately 81% and 75% for the teen and adult groups, respectively. Risk assessment parameters, such as BW, ED, HR, ABS, CSFing, and SA, were identified as the most common factors sensitive to CR levels. In the present study, BW, ED, and HR were based on Korean standards, while the other parameters were taken from the U.S. EPA data. Determination of other risk assessment parameters value could be fruitful in future research. Risk assessment efficiency might also be improved by using more specific health risk parameters.
Uncertainty and Variability Analysis
Two-dimensional simulation plots for variability and uncertainty are shown in Figures 4A, B. The overlay chart of risk curves and trend chart of certainty bands for teen in the case of CR from soil-bound PAHs have been reported in Figures 4A, B, respectively. The 2D simulation result shows that the risk curves are densely clustered near the center, although limited outlier curves are distributed to the Cumulative Frequency axis. The result shows the lesser probability of having a much higher risk than the simulated. Moreover, for soil-bound PAHs at Ulsan area, the 95th percentile CR in two-dimensional study (4.07E−05 for teen) is similar to that of the 95th percentile CR of one-dimensional simulation (4.13E−05 for teen).
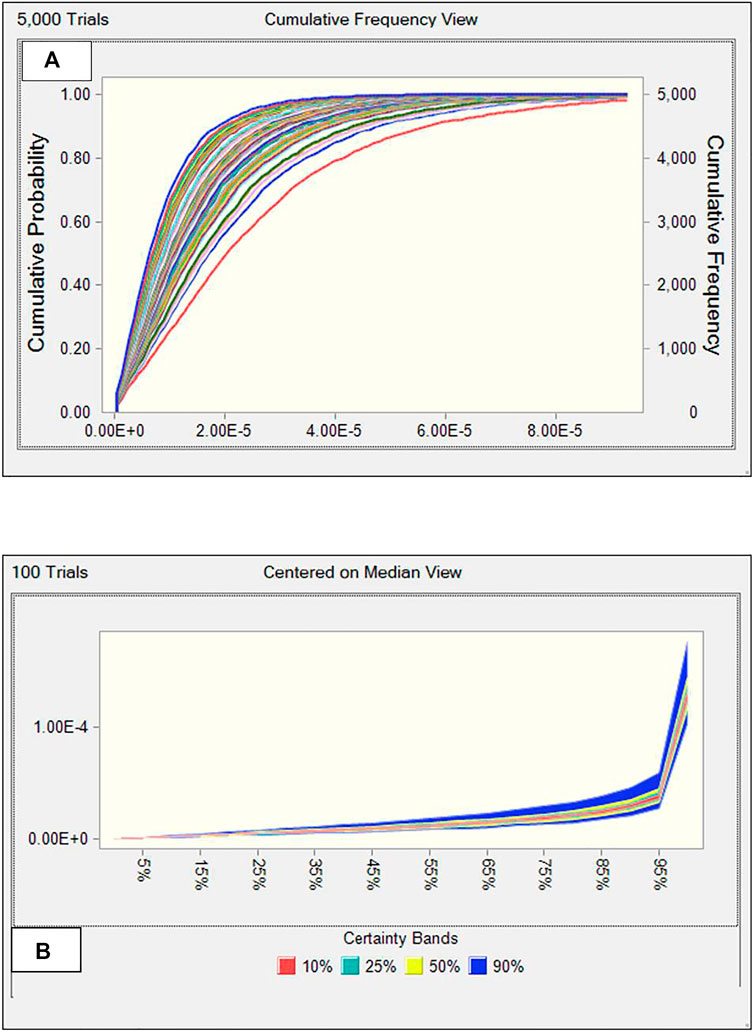
FIGURE 4. Two-dimensional simulation plots of variability and uncertainty (A) overlay chart of risk curves for teen and adults and (B) trend chart of certainty bands for teen and adults at Ulsan.
Conclusion
Ulsan is a well-known industrial city in Korea. In this study, CR levels were estimated for seven different land-use areas, based on soil-bound PAHs. Additionally, the most important exposure routes and sensitive parameters were identified. Although several industries and their associated endeavors (such as petrochemical and automobile factories, non-ferrous metal smelting plants, refineries, and shipbuilding complexes) contributed significant amounts of PAHs to the soil, concentration levels were approximately three times higher in RA than those in IA. CR levels in RA and TA were also higher than those in IA because of the larger amounts of carcinogenic PAHs in the soil. Moreover, exposure through dermal absorption and food ingestion were most significant for soil-bound PAHs. Risk assessment parameters such as ED, AF, and BW were identified as the most sensitive parameters for CR levels. While industrial activities are well-known anthropogenic sources for global pollution and negative human health impacts, carcinogenic PAHs resulting from transportation activities (RA and TA) caused elevated CR levels. Nowadays, cities are surrounded by traffic-intense areas with high vehicle density and railroads. There is a large possibility that the soil in these areas is being contaminated with carcinogenic PAHs, which could result in an unhealthy urban environment. Therefore, detailed investigations on CR levels, with respect to soil-bound PAHs in RA and TA, can be performed in future studies for cities in other areas and countries.
Data Availability Statement
The original contributions presented in the study are included in the article/Supplementary Material, further inquiries can be directed to the corresponding author.
Author Contributions
DR and JP conceived of the study design. DR, JK, WJ, and ML collected and interpreted the data. DR drafted the manuscript. DR and JP revised the manuscript.
Funding
This research was supported by the Basic Science Research Program through the National Research Foundation of Korea (NRF) funded by the Ministry of Education (No. 2018R1A6A1A08025348) and Korea Ministry of Environment (The Subsurface Environment Management, Project No. 2020002480003).
Conflict of Interest
The authors declare that the research was conducted in the absence of any commercial or financial relationships that could be construed as a potential conflict of interest.
Publisher’s Note
All claims expressed in this article are solely those of the authors and do not necessarily represent those of their affiliated organizations or those of the publisher, the editors, and the reviewers. Any product that may be evaluated in this article, or claim that may be made by its manufacturer, is not guaranteed or endorsed by the publisher.
Supplementary Material
The Supplementary Material for this article can be found online at: https://www.frontiersin.org/articles/10.3389/fenvs.2021.744387/full#supplementary-material
References
Abdel-Shafy, H. I., and Mansour, M. S. M. (2016). A Review on Polycyclic Aromatic Hydrocarbons: Source, Environmental Impact, Effect on Human Health and Remediation. Egypt. J. Pet. 25, 107–123. doi:10.1016/j.ejpe.2015.03.011
Akyüz, M., and Çabuk, H. (2010). Gas-particle Partitioning and Seasonal Variation of Polycyclic Aromatic Hydrocarbons in the Atmosphere of Zonguldak, Turkey. Sci. Total Environ. 408, 5550–5558. doi:10.1016/j.scitotenv.2010.07.063
Ambade, B., and Sethi, S. S. (2021). Health Risk Assessment and Characterization of Polycyclic Aromatic Hydrocarbon from Hydrosphere. J. Hazard. Tox. Radioactive Waste 25, 2. doi:10.1061/(ASCE)HZ.2153-5515.0000586
Ambade, B., Kumar, A., Kumar, A., and Sethi, S. S. (2020). Characterization of PAHs and N-Alkanes in Atmospheric Aerosol of Jamshedpur City, India. J. Hazard. Tox. Radioactive Waste 24, 4. doi:10.1061/(ASCE)HZ.2153-5515.0000490
Ambade, B., Kumar, A., Kumar, A., and Sahu, L. K. (2021a). Temporal Variability of Atmospheric Particulate-Bound Polycyclic Aromatic Hydrocarbons (PAHs) over central East India: Sources and Carcinogenic Risk Assessment. Air Qual. Atmos. Health 14, 1–16. doi:10.1007/s11869-021-01089-5
Ambade, B., Kumar, A., and Sahu, L. K. (2021b). Characterization and Health Risk Assessment of Particulate Bound Polycyclic Aromatic Hydrocarbons (PAHs) in Indoor and Outdoor Atmosphere of Central East India. Environ. Sci. Pollut. Res 28 (40), 56269–56280. doi:10.1007/s11356-021-14606-x
Ambade, B., Kurwadkar, S., Sankar, T. K., and Kumar, A. (2021c). Emission Reduction of Black Carbon and Polycyclic Aromatic Hydrocarbons during COVID-19 Pandemic Lockdown. Air Qual. Atmos. Health 14, 1081–1095. doi:10.1007/s11869-021-01004-y
Ambade, B., Sethi, S. S., Kurwadkar, S., Kumar, A., and Sankar, T. K. (2021d). Toxicity and Health Risk Assessment of Polycyclic Aromatic Hydrocarbons in Surface Water, Sediments and Groundwater Vulnerability in Damodar River Basin. Groundwater Sustain. Develop. 13, 100553. doi:10.1016/j.gsd.2021.100553
Armstrong, B., Hutchinson, E., Unwin, J., and Fletcher, T. (2004). Lung Cancer Risk after Exposure to Polycyclic Aromatic Hydrocarbons: a Review and Meta-Analysis. Environ. Health Perspect. 112, 970–978. doi:10.1289/ehp.6895
Arora, A. S., and Reddy, A. S. (2013). Multivariate Analysis for Assessing the Quality of Stormwater from Different Urban Surfaces of the Patiala City, Punjab (India). Urban Water J. 10 (6), 422–433. doi:10.1080/1573062X.2012.739629
Arora, A. S., and Reddy, A. S. (2014). Development of Multiple Linear Regression Models for Predicting the Stormwater Quality of Urban Sub-watersheds. Bull. Environ. Contam. Toxicol. 92, 36–43. doi:10.1007/s00128-013-1160-y
Arora, A. S., and Reddy, A. S. (2015). Conceptualizing a Decentralized Stormwater Treatment System for an Urbanized City with Improper Stormwater Drainage Facilities. Int. J. Environ. Sci. Technol. 12, 2891–2900. doi:10.1007/s13762-014-0655-3
Baek, S. W. (2013). A Research on Actual Condition of Soil Containing PAHs in Area Which Used a Recycled Railroad Tie Wastes. Ann. Rep. Ulsan Instit. Health Environ. 7, 149–187. Written in Korean.
Bosetti, C., Boffetta, P., and La Vecchia, C. (2007). Occupational Exposures to Polycyclic Aromatic Hydrocarbons, and Respiratory and Urinary Tract Cancers: a Quantitative Review to 2005. Ann. Oncol. 18, 431–446. doi:10.1093/annonc/mdl172
Budzinski, H., Jones, I., Bellocq, J., Piérard, C., and Garrigues, P. (1997). Evaluation of Sediment Contamination by Polycyclic Aromatic Hydrocarbons in the Gironde Estuary. Mar. Chem. 58, 85–97. doi:10.1016/s0304-4203(97)00028-5
Cappelletti, N., Astoviza, M., Morrone, M., and Tatone, L. (2018). Urban Geochemistry and Potential Human Health Risks in the Metropolitan Area of Buenos Aires: PAHs and PCBs in Soil, Street Dust, and Bulk Deposition. Environ. Geochem. Health 41, 699–713. doi:10.1007/s10653-018-0163-3
Chawda, S., Tarafdar, A., Sinha, A., and Mishra, B. K. (2020). Profiling and Health Risk Assessment of PAHs Content in Tandoori and Tawa Bread from India. Polycyclic aromatic Compd. 40 (1), 21–32. doi:10.1080/10406638.2017.1349679
Chen, S.-C., and Liao, C.-M. (2006). Health Risk Assessment on Human Exposed to Environmental Polycyclic Aromatic Hydrocarbons Pollution Sources. Sci. Total Environ. 366, 112–123. doi:10.1016/j.scitotenv.2005.08.047
Chen, J.-W., Wang, S.-L., Hsieh, D. P. H., Yang, H.-H., and Lee, H.-L. (2012). Carcinogenic Potencies of Polycyclic Aromatic Hydrocarbons for Back-Door Neighbors of Restaurants with Cooking Emissions. Sci. Total Environ. 417-418, 68–75. doi:10.1016/j.scitotenv.2011.12.012
Chiang, K.-C., Chio, C.-P., Chiang, Y.-H., and Liao, C.-M. (2009). Assessing Hazardous Risks of Human Exposure to temple Airborne Polycyclic Aromatic Hydrocarbons. J. Hazard. Mater. 166, 676–685. doi:10.1016/j.jhazmat.2008.11.084
Chung, H. H., Park, E. H., and Park, S. W. (2006). Compositional Characteristics and Origin of Polycyclic Aromatic Hydrocarbons[PAHs] of Crude Oils and Petroleum Products. J. Environ. Sci. 15, 397–403. doi:10.5322/jes.2006.15.5.397
Dong, T. T., and Lee, B. K. (2009). Characteristics, Toxicity, and Source Apportionment of Polycylic Aromatic Hydrocarbons (PAHs) in Road Dust of Ulsan, Korea. Chemosphere 74, 1245–1253. doi:10.1016/j.chemosphere.2008.11.035
Gungormus, E., Tuncel, S., Hakan Tecer, L., and Sofuoglu, S. C. (2014). Inhalation and Dermal Exposure to Atmospheric Polycyclic Aromatic Hydrocarbons and Associated Carcinogenic Risks in a Relatively Small City. Ecotoxicol. Environ. Saf. 108, 106–113. doi:10.1016/j.ecoenv.2014.06.015
Hussain, M., Rae, J., Gilman, A., and Kauss, P. (1998). Lifetime Health Risk Assessment from Exposure of Recreational Users to Polycyclic Aromatic Hydrocarbons. Arch. Environ. Contam. Toxicol. 35, 527–531. doi:10.1007/s002449900412
IARC (2010). Some Non-heterocyclic Polycyclic Aromatic Hydrocarbons and Some Related Exposures. IARC Monogr. Eval. Carcinog Risks Hum. 92, 1–853.
Jeon, H.-D., and Oh, S.-Y. (2019). Distribution, Toxicity, and Origins of Polycyclic Aromatic Hydrocarbons in Soils in Ulsan, South Korea. Environ. Monit. Assess. 191, 409. doi:10.1007/s10661-019-7558-1
Kim, T. S., and Shin, S. K. (2001). Analytical Methods and Release of Polycyclic Aromatic Hydrocarbons in Environments. Anal. Sci. Techn. 14, 47A. Written in Korean.
Kim, S.-J., Park, M.-K., Lee, S.-E., Go, H.-J., Cho, B.-C., Lee, Y.-S., et al. (2019). Impact of Traffic Volumes on Levels, Patterns, and Toxicity of Polycyclic Aromatic Hydrocarbons in Roadside Soils. Environ. Sci. Process. Impacts 21, 174–182. doi:10.1039/c8em00532j
Kong, S., Shi, J., Lu, B., Qiu, W., Zhang, B., Peng, Y., et al. (2011). Characterization of PAHs within PM10 Fraction for Ashes from Coke Production, Iron Smelt, Heating Station and Power Plant Stacks in Liaoning Province, China. Atmos. Environ. 45, 3777–3785. doi:10.1016/j.atmosenv.2011.04.029
Kumar, A., Sankar, T. K., Sethi, S. S., and Ambade, B. (2020). Characteristics, Toxicity, Source Identification and Seasonal Variation of Atmospheric Polycyclic Aromatic Hydrocarbons over East India. Environ. Sci. Pollut. Res. 27 (1), 678–690. doi:10.1007/s11356-019-06882-5
Kwon, H.-O., and Choi, S.-D. (2014). Polycyclic Aromatic Hydrocarbons (PAHs) in Soils from a Multi-Industrial City, South Korea. Sci. Total Environ. 470-471, 1494–1501. doi:10.1016/j.scitotenv.2013.08.031
Li, H. L., Liu, G. L., and Cao, Y. (2014). Content and Distribution of Trace Elements and Polycyclic Aromatic Hydrocarbons in Fly Ash from a Coal-Fired CHP Plant. Aerosol Air Qual. Res. 14, 1179–1188. doi:10.4209/aaqr.2013.06.0216
Malawska, M. (2000). Contamination with Heavy Metals and Polycyclic. Water Air Soil Pollut. 127, 339–349.
NIER (2016). Korean Exposure Factors Handbook for Children. Korea: National Institute of Environmental Research, Ministry of Environment.
NYSDOH (2012). Health Consultation: hopewell Precision Area Groundwater Contamination Site Town of East Fish Kill, Dutchess County, New York, NY. Appendix C, DOH Procedure for Evaluating Potential Health Risks for Contaminants of Concern. New York: NYSDOH.
Qu, C., Li, B., Wu, H., Wang, S., and Giesy, J. P. (2015). Multi-pathway Assessment of Human Health Risk Posed by Polycyclic Aromatic Hydrocarbons. Environ. Geochem. Health 37, 587–601. doi:10.1007/s10653-014-9675-7
Ray, S., Khillare, P. S., Agarwal, T., and Shridhar, V. (2008). Assessment of PAHs in Soil Around the International Airport in Delhi, India. J. Hazard. Mater. 156, 9–16. doi:10.1016/j.jhazmat.2007.11.099
Roy, D., Seo, Y.-C., Sinha, S., SinghBhattacharya, G., and Biswas, P. K. (2017). Human Health Risk Exposure with Respect to Particulate-Bound Polycyclic Aromatic Hydrocarbons at Mine Fire-Affected Coal Mining Complex. Environ. Sci. Pollut. Res. 26, 19119–19135. doi:10.1007/s11356-017-9202-3
Roy, D., Seo, Y.-C., Kim, S., and Oh, J. (2019a). Human Health Risks Assessment for Airborne PM10-Bound Metals in Seoul, Korea. Environ. Sci. Pollut. Res. 26, 24247–24261. doi:10.1007/s11356-019-05213-y
Roy, D., Seo, Y.-C., Namgung, H.-G., and Kwon, S.-B. (2019b). Inhalation Cancer Risk from PM10 in the Metropolitan Subway Stations in Korea. J. Transport Health 14, 100580. doi:10.1016/j.jth.2019.100580
Roy, D., Singh, G., and Seo, Y.-C. (2019c). Coal Mine Fire Effects on Carcinogenicity and Non-carcinogenicity Human Health Risks. Environ. Pollut. 254, 113091. doi:10.1016/j.envpol.2019.113091
Roy, D., Singh, G., and Seo, Y. C. (2019d). Cancer and Non-cancer Risks from PM10-And PM2.5-bound Metal in a Critically Polluted Coal Mining Complex. Atmos. Pollut. Res. 10, 1964–1975. doi:10.1016/j.apr.2019.09.002
Roy, D., Ahn, S. H., Lee, T. K., Seo, Y.-C., and Park, J. (2020). Cancer and Non-cancer Risk Associated with PM10-Bound Metals in Subways. Transport. Res. D: Transport Environ. 89, 102618. doi:10.1016/j.trd.2020.102618
Soclo, H. H., Garrigues, P. H., and Ewald, M. (2000). Origin of Polycyclic Aromatic Hydrocarbons (PAHs) in Coastal Marine Sediments : Case Studies in Cotonou (Benin) and Aquitaine (France) Areas. Mar. Pollut. Bull. 40, 387–396. doi:10.1016/S0025-326X(99)00200-3
Tarafdar, A., and Sinha, A. (2017a). Cancer Risk Assessment of Polycyclic Aromatic Hydrocarbons in the Soils and Sediments of India: A Meta-Analysis. Environ. Manage. 60, 784–795. doi:10.1007/s00267-017-0920-6
Tarafdar, A., and Sinha, A. (2017b). Estimation of Decrease in Cancer Risk by Biodegradation of PAHs Content from an Urban Traffic Soil. Environ. Sci. Pollut. Res. 24, 10373–10380. doi:10.1007/s11356-017-8676-3
Tarafdar, A., Chawda, S., and Sinha, A. (2018). Health Risk Assessment from Polycyclic Aromatic Hydrocarbons (PAHs) Present in Dietary Components: A Meta-Analysis on a Global Scale. Polycyclic Aromatic Compd. 40, 850–861. doi:10.1080/10406638.2018.1492426
Tarafdar, A., Oh, M.-J., Nguyen-Phuong, Q., and Kwon, J.-H. (2020). Profiling and Potential Cancer Risk Assessment on Children Exposed to PAHs in Playground Dust/soil: a Comparative Study on Poured Rubber Surfaced and Classical Soil Playgrounds in Seoul. Environ. Geochem. Health 42, 1691–1704. doi:10.1007/s10653-019-00334-2
USEPA (2002). Supplemental Guidance for Developing Soil Screening Levels for Superfund Sites. Washington, DC: USEPA.
U.S. EPA (1989). Risk Assessment Guidance for Superfund: Human Health Evaluation Manual (Part A), Vol. I. Washington, DC: USEPA, 291. EPA/540/1-89/002.
U.S. EPA (1993). Provisional Guidance for Quantitative Risk Assessment of Polycyclic Aromatic Hydrocarbons. Washington, DC: USEPA.
U.S. EPA (2003). Integrated Risk Information System. Available at: https://www.epa.gov/iris (Accessed 04 10, 2020).
U.S. EPA (2004). Risk Assessment Guidance for Superfund (RAGS), Vol. I: Human Health Evaluation Manual (Part E, Supplemental Guidance for Dermal Risk Assessment). Washington, DC: USEPA.
U.S. EPA (2009). Risk Assessment Guidance for Superfund, Vol. I: Human Health Evaluation Manual (Part F, Supplemental Guidance for Inhalation Risk Assessment). Washington, DC: USEPA.
Wang, W., Huang, M.-j., Kang, Y., Wang, H.-s., Leung, A. O. W., Cheung, K. C., et al. (2011). Polycyclic Aromatic Hydrocarbons (PAHs) in Urban Surface Dust of Guangzhou, China: Status, Sources and Human Health Risk Assessment. Sci. Total Environ. 409, 4519–4527. doi:10.1016/j.scitotenv.2011.07.030
Wester, R. C., Maibach, H. I., Bucks, D. A. W., Sedik, L., Melendres, J., Liao, C., et al. (1990). Percutaneous Absorption of [14C]DDT and [14C]Benzo[a]pyrene from Soil. Toxicol. Sci. 15, 510–516. doi:10.1093/toxsci/15.3.510
Williams, E. S., Mahler, B. J., and Van Metre, P. C. (2013). Cancer Risk from Incidental Ingestion Exposures to PAHs Associated with Coal-Tar-Sealed Pavement. Environ. Sci. Technol. 47, 1101–1109. doi:10.1021/es303371t
Yoo, E. H., Choi, Y. J., and Kim, M. H. (2010). Pollution Characteristics of Heavy Metal and Polycyclic Aromatic hydrocarbon(PAHs) in Deposited Road Particles of Busan. Annu. Rep. Busan Inst. Health Environ. 20, 178–193. Written in Korean.
Yu, B., Xie, X., Ma, L. Q., Kan, H., and Zhou, Q. (2014). Source, Distribution, and Health Risk Assessment of Polycyclic Aromatic Hydrocarbons in Urban Street Dust from Tianjin, China. Environ. Sci. Pollut. Res. 21, 2817–2825. doi:10.1007/s11356-013-2190-z
Yunker, M. B., Macdonald, R. W., Vingarzan, R., Mitchell, R. H., Goyette, D., and Sylvestre, S. (2002). PAHs in the Fraser River Basin: A Critical Appraisal of PAH Ratios as Indicators of PAH Source and Composition. Org. Geochem. 33, 489–515. doi:10.1016/S0146-6380(02)00002-5
Keywords: cancer risk, soil, PAHs, Monte Carlo, industrial city, South Korea
Citation: Roy D, Jung W, Kim J, Lee M and Park J (2022) Polycyclic Aromatic Hydrocarbons in Soil and Human Health Risk Levels for Various Land-Use Areas in Ulsan, South Korea. Front. Environ. Sci. 9:744387. doi: 10.3389/fenvs.2021.744387
Received: 20 July 2021; Accepted: 04 October 2021;
Published: 04 January 2022.
Edited by:
Bikram Basak, Hanyang University, South KoreaReviewed by:
Amarpreet Singh Arora, Sherpa Space Inc., South KoreaBalram Ambade, National Institute of Technology, Jamshedpur, India
Orish Ebere Orisakwe, University of Port Harcourt, Nigeria
Copyright © 2022 Roy, Jung, Kim, Lee and Park. This is an open-access article distributed under the terms of the Creative Commons Attribution License (CC BY). The use, distribution or reproduction in other forums is permitted, provided the original author(s) and the copyright owner(s) are credited and that the original publication in this journal is cited, in accordance with accepted academic practice. No use, distribution or reproduction is permitted which does not comply with these terms.
*Correspondence: Joonhong Park, cGFya2pAeW9uc2VpLmFjLmty