- 1Bat Biology and Conservation Laboratory, Department of Zoology, University of Brasilia, Brasília, Brazil
- 2Graduate Program in Ecology, Department of Ecology, Institute of Biological Sciences, University of Brasilia, Brasília, Brazil
- 3Graduate Program in Zoology, Department of Zoology, Institute of Biological Sciences, University of Brasilia, Brasília, Brazil
Increasing anthropization is detrimental to the natural environment and the quality of life, affecting populations, communities, and the relationships between organisms. One of the most unique relationships in the animal world is parasitism, which often involves tightly specialized interactions between pairs of species. Bat flies, for example, are obligate ectoparasites represented by two highly adapted dipteran families that usually parasite a single bat species or genus. Recent studies have shown that bat flies could carry pathogens such as bacteria and viruses, transmitting them among bat individuals in a colony. Because host roost characteristics can influence bat-fly parasitism, we aimed to assess whether the ecological networks between parasites and their host bats are influenced by the degree of habitat anthropization. Our hypothesis was that bat-fly interaction networks would be less specialized and more nested in highly anthropized sites. We collected bat fly individuals from bats captured at 21 sampling sites located in the Federal District of Brazil and quantified the amount of natural and anthropized area within a 3-km buffer from the sampling site. Areas consisting of agriculture, construction, mining, roads, or any man-made structure were considered anthropized. Sites presented different degrees of anthropization, with areas ranging from 100% anthropized to areas retaining full natural cover. We built bat-bat fly networks for each of the sites and excluded those with sampling completeness values smaller than 0.7. We calculated key weighted structural metrics for each network, such as nestedness, specialization, and modularity. The effect of the reduction in natural cover on structural metrics was assessed through GLMMs, controlling for network size and ectoparasite diversity. Nestedness increased with the amount of anthropization, while specialization and modularity did not change and were overall high in all networks. This result suggests that anthropization may influence the assembly of bat-bat fly networks, leading to the emergence of a hierarchical assembly of interactions as parasites become less specialized and interact with a wider variety of hosts. Less specialized relationships could influence parasite fitness or even increase the likelihood of transmitting pathogens between populations of different bat species.
Introduction
Increasing anthropization is one of the most ecologically threatening human activities worldwide as it occurs to the detriment of the natural environment and the quality of life of many species (Fahrig 2003; Haddad et al., 2015). Anthropization brings consequences such as habitat loss, increased pollution, and habitat degradation (Haddad et al., 2015; Russo and Ancillotto 2015), leading to different effects on biodiversity, such as abrupt changes in community composition (Caughley 1994; Willig et al., 2007; Mbora and McPeek 2009; Russo and Ancillotto 2015). Bats, for example, usually display shifts in species abundances, with increases in the abundance of species resistant to anthropized habitats while those with more ecologically specialized requirements are severely hampered (Willig et al., 2007; Bobrowiec and Gribel 2010; Russo and Ancillotto 2015). This shift in species abundances, coupled with environmental alterations derived from anthropization, may also affect relationships between bats and other organisms, such as prey, predators, and parasites (Russo and Ancillotto 2015). In urban areas, insect availability is lower, limiting the amount of prey for insectivorous bat species (Threlfall et al., 2011; Russo and Ancillotto 2015). Conversely, a higher abundance and diversity of predators can be found in urban areas, posing great danger to bats in those habitats (Threlfall et al., 2013; Russo and Ancillotto 2015). Moreover, recent studies have reported that bats inhabiting anthropized areas present a greater diversity of zoonotic parasites, including fungi, bacteria, and viruses (Mühldorfer et al., 2011; Nunes et al., 2017).
Parasitism is a unique relationship in the animal world as it often involves tightly specialized interactions between pairs of species (Combes, 2001). Bat flies, for example, are obligate bat ectoparasites represented by two highly adapted dipteran families: Streblidae and Nycteribiidae (Marshall, 1982). Bat flies’ morphology reflects their role as ectoparasites, with many species having absent or reduced wings, with long limbs that facilitate locomotion on the host body, claws on the distal region of the limbs or ctenia on the ventral lower end of the head to help individuals to hold onto the fur, and specialized buccal structures for piercing the skin (Peterson and Wenzel 1987; Whitaker Jr. 1988). Both bat fly families are usually highly specific parasites, with each species of bat fly parasitizing a single bat species or genus (Wenzel et al., 1966; Marshall 1982). Although there is no evidence that bat flies could affect the health condition of the host, recent studies showed that those flies harbor potentially pathogenic microorganisms such as bacteria, viruses, and fungi (Morse et al., 2012; Dick and Dittmar 2013; Gay et al., 2014; Abundes-Gallegos et al., 2018; Martínez et al., 2021).
Bat-fly parasitism can be influenced by different characteristics of the host, such as age, sex, body size, reproductive status, activity, and abundance (Muñoz et al., 2003, Bertola et al., 2005, Patterson et al., 2008a,b, Presley and Willig 2008, Esbérard et al., 2012, Fagundes et al., 2017). This interaction can also be shaped by the type and characteristics of the roost used by the host, with larger and more enclosed colonies exhibiting higher parasitic rates, including higher prevalence, mean intensity of infestation, and parasite richness (Patterson et al., 2007). Habitat degradation can also influence parasitism dynamics by affecting ectoparasitic survival and reproduction rates or by causing stress in the host population (Patterson et al., 2007; Mbora and McPeek 2009; Pilosof et al., 2012; Ramalho et al., 2018). Moreover, in degraded areas, decreased roost availability could force bats to roost in colonies with different species, again affecting parasitism as well as facilitating horizontal transmission (Urbieta et al., 2020), even between different bat species. Because of the high degree of specialization of bat flies to bats (Wenzel et al., 1966; Marshall 1982), the relationship between them is considered an excellent model for studies of host-parasite association.
Network science is a valuable framework for investigating interspecific and community-wide assemblies of interactions, as it can yield quantitative information on species specialization and shared dependence on interacting partners (Dormann et al., 2009; Ings et al., 2009). This tool has become increasingly popular for describing several types of interaction at community-wide levels, including host-parasite networks (Vázquez et al., 2005; Löwenberg-Neto 2008; Canard et al., 2014). Bat-bat fly interaction networks have recently gained much attention, and research has shown that interactions in these systems tend to be highly specialized, with networks often being compartmentalized (Zarazúa-Carbajal et al., 2016; Saldaña-Vázquez et al., 2019; Hiller et al., 2021), pointing to tight coevolution of certain parasites with their host bats. However, the effect of land-use change on the assembly of such networks has been poorly explored. Hernández-Martinez et al. (2018) observed that bat-bat fly networks were more specialized in less fragmented areas, while Urbieta et al. (2020) have shown that bat-ectoparasite networks preserve their structural characteristics even in areas with differences in land use. However, no study to date has performed a formal analysis to assess how the assembly of bat-ectoparasite networks changes along an anthropization continuum. We aimed to reconstruct several interaction networks between ectoparasites and their host bats at sites within and outside protected areas in central Brazil, encompassing a wide range of anthropized land cover, to assess the effect of the reduction of natural habitats on network structure and assembly rules. We tested the hypothesis that bat-fly interaction networks are affected by the degree of anthropization surrounding the studied sites, leading to less specialized and more nested networks in highly anthropized areas.
Materials and Methods
Study Area
We conducted this study in the Federal District of Brazil, located in the core area of the Cerrado Biosphere Reserve (Figure 1). The area comprises a mosaic of different vegetation types, with the dominant typess consisting of savanna habitats and forested riparian areas. The climate in the study area is classified as Tropical Savanna (Aw) and consists of two well defined seasons, a rainy season from September to April and a dry season from May to August (Ratter et al., 1997).
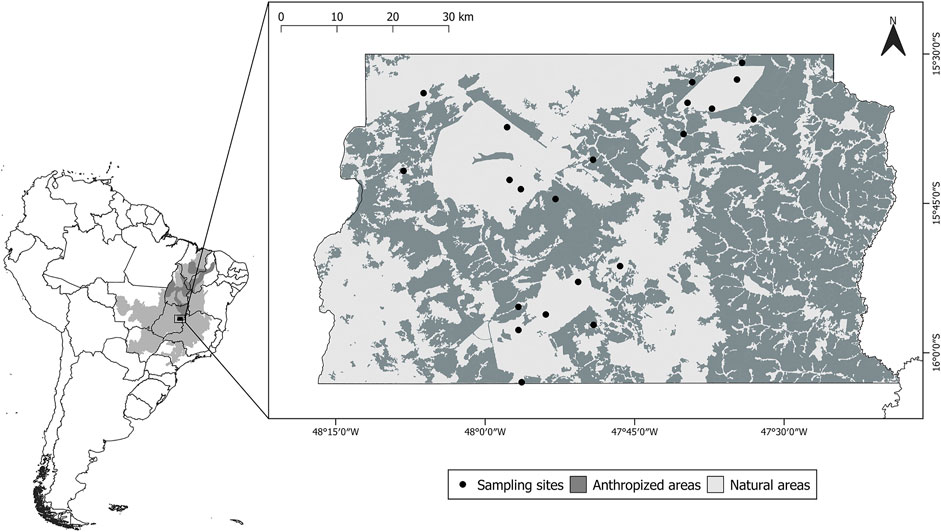
FIGURE 1. Location of the study area within South America. In small map light grey represents the Cerrado biome and dark grey represents the Cerrado Biosphere Reserve. In large map light grey represents natural areas, dark grey represents anthropized areas, and black dots represent sampling sites.
In order to assess information from different communities of bats and their ectoparasites, we conducted captures in areas within and in the proximity of the three main protected areas in the Federal District: Brasília National Park (PNB) (42,389 ha, 15°41′42″S, 48°08′10″W), Gama-Cabeça de Veado Environmental Protection Area (AGCV) (25,000 ha, 15°52′29″S, 47°50′48″W), and Águas Emendadas Ecological Station (ESECAE) (10,547 ha, 15°36′32″S, 47°33′03″W) (Figure 1). We defined 21 sampling sites with different degrees of anthropization in the study area to characterize the effects of habitat loss on bat-bat fly assemblies. The minimum and maximum distances between sampling sites were 2.6 and 64.8 km, respectively.
Quantification of Anthropization
For each sampling site, we defined a 3-km buffer and quantified the percentage of natural and anthropized area within that radius. The distance was defined based on previous studies that registered a flight distance between 1 and 3 km for bats in Neotropical savanna areas (Bernard and Fenton 2003; Aguiar et al., 2014). We used Mapbiomas Collections classification maps (Souza et al., 2020, https://mapbiomas.org/) to identify land use within each buffer in QGIS 3.6 Noosa (QGIS Development Team 2021). Areas consisting of agriculture, construction, mining, roads, or any man-made structure were considered anthropized, while areas with natural vegetation of any type (e.g., savanna, grasslands, and gallery forests) were considered natural. Each site received an anthropization value corresponding to the ratio of anthropized cover in relation to natural vegetation within the 3-km buffer.
Capture of Bats and Bat Flies
We captured bats during 89 capturing sessions, with each of the 21 sites being sampled 4 or 5 times between April 2012 and August 2013. Bats were captured with six to ten mist nets (12 × 3 m) installed along natural paths through the vegetation or near trees located in the sampling sites. Nets were opened at sunset and remained open for 6 h per night. Bats were identified upon capture under the criteria of Díaz et al. (2016). Shortly after removing the bats from the nets, we inspected each individual for the presence of ectoparasites with brushes and tweezers, placing collected flies inside Eppendorf® microtubes containing 70% ethanol. Bat flies were identified under a stereomicroscope (Motic K-series) at the Bat Biology and Conservation Lab at the University of Brasília using specialized identification keys (Guerrero, 1993, 1994a,b, 1995a,b, 1996). Species identification was confirmed by Prof. Dr. Gustavo Graciolli from the Federal University of Mato Grosso do Sul. After manipulation, all bats were tagged with numbered rings and released at the location of capture.
Network Analysis and Effect of Anthropization
We used interactions between bats and ectoparasites at each of the sampling sites to build weighted adjacency matrices. Pairwise interactions (cell values) corresponded to the number of individuals of a bat species carrying a parasite species. With each matrix, we built a weighted bipartite interaction network. We assessed the sampling completeness of each network through individual-based rarefaction curves (Colwell et al., 2012) of ectoparasites found on bats, using the nonparametric Chao1 estimator of asymptotic species richness (Chao et al., 2009). We calculated the completeness of each network as the ratio between observed ectoparasite richness and the estimated asymptotic richness (Chacoff et al., 2012). We set a cutoff of 0.7 of sampling completeness, below which networks were discarded from further analysis.
From each network, we calculated three structural metrics that describe different aspects of network assembly and distribution of interactions: nestedness, through the weighted NODF (wNODF) metric (Almeida-Neto and Ulrich 2011); weighted modularity (Qw) (Schleuning et al., 2014); and complementary specialization (H2′ metric) (Blüthgen, 2010). Nestedness refers to whether the interactions of specialist species form a subset of the interactions of generalists (Bascompte et al., 2003). The wNODF index varies from zero to 100, and a fully nested network is a highly asymmetric network, where specialists always interact with generalists that have a larger pool of partners. Modularity assesses the existence of subgroups of species within the network that interact more among themselves than with the rest of the species and reveals patterns of affinity between the two levels of the network (Olesen et al., 2007). The index Qw varies from zero to one, and networks with high modularity have conspicuous and tightly knit subgroups of species. Finally, specialization is calculated as the average niche breadth of species within the network. The index H2′ corrects for network size and varies from zero to one, and species in a highly specialized network have narrower niches and interact strongly with one or few partners and thus leading to high niche complementarity (Blüthgen, 2010). Analyses were conducted in R Studio 3.6.0 (R Core Team, 2015). Network and sampling completeness analyses were performed using the bipartite (Dormann et al., 2008) and vegan (Oksanen et al., 2020) packages, respectively.
We ran three distinct mixed-effect generalized linear models (GLMMs) to assess the effect of anthropization on network structural metrics. The proportion of anthropized area within each site’s buffer was set as an explanatory variable, and each of the three metrics was each set as a response variable. We ran all models with a logit link function and quasibinomial error distribution to account for the proportion values of response variables. Network size and ectoparasite diversity were set as random variables. The metric wNODF was standardized to range from zero to one to be consistent with the other metrics (values were divided by 100). All models were checked a posteriori for overdispersion.
Moreover, we constructed three meta-networks grouping individual networks according to their degree of anthropization to synthesize interactions: fully preserved (100% natural cover within buffer), moderately anthropized (99.9–50% natural cover) and highly anthropized (49.9–0% natural cover). The three aforementioned structural metrics were calculated for each of the meta-networks. Meta-networks were constructed with Gephi 0.9.2 (Bastian et al., 2009).
Results
We captured 2,243 bats of 36 species in the 21 sampling sites, of which 899 bats of 23 species were carrying bat flies, which comprised 1,721 individuals of 38 species (Supplementary Table S1). After excluding sites with low sampling completeness (<0.7), 18 sites remained, leaving 21 bat species, 33 ectoparasite species and 711 pairwise interactions between 611 bats and 1,389 flies (Supplementary Table S1). Sites showed a broad variation in the percentage of natural cover within the buffer, from fully preserved sites within protected areas (100% natural cover) to fully anthropized sites outside of the national parks (0% natural cover) (Supplementary Table S2).
Networks were overall highly specialized (H2′ = 0.90 ± 0.14), modular (Qw = 0.65 ± 0.08), and showed a remarkable lack of nestedness (wNODF = 2.41 ± 4.68). Anthropization did not affect network specialization (β = −0.001, t = −0.105; p = 0.917) nor modularity (β = −0.004; t = −1.452; p = 0.166), but nestedness showed a positive response to an increase in anthropic cover (β = 0.034; t = 3.45; p < 0.005) (Figure 2). The most anthropized sites (81.6 and 100% of anthropic cover) showed the most nested networks among the pool (10.50 and 18.75 wNODF, respectively) (Figure 2).
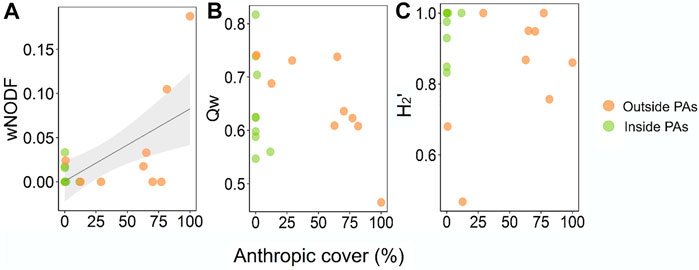
FIGURE 2. Distribution of structural metrics from bat-bat fly interaction networks along an anthropization continuum in Central Brazil. (A): nestedness; (B): modularity; (C): complementary specialization. Networks are divided according to their position in the landscape (inside or outside Protected Areas). Points with denser colors indicate overlapping values.
Regarding the meta-networks, the highly anthropized one exhibited the highest nestedness value, followed by the fully preserved and moderately anthropized meta-networks. The highly anthropized meta-network was also the least modular and the least specialized among the three meta-networks, and consisted of only two separated compartments, contrasting with three and eight compartments in the fully preserved and moderately anthropized networks, respectively (Figure 3).
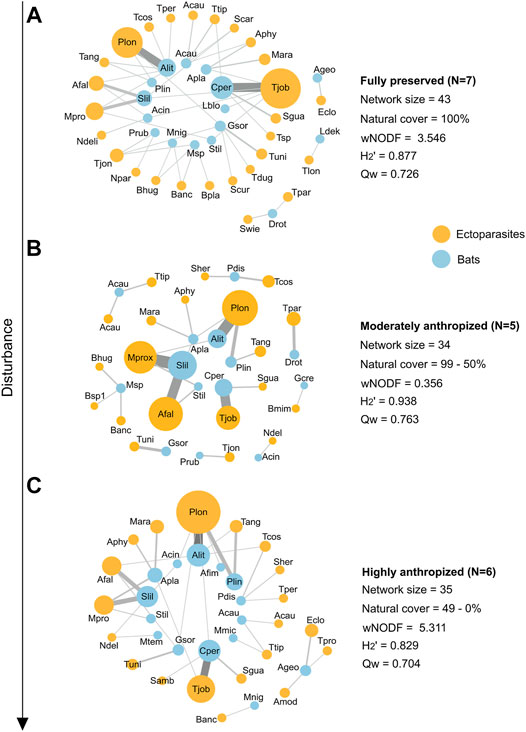
FIGURE 3. Bat-bat fly interaction meta-networks and their structural metrics from natural and disturbed areas in Central Brazil. The 18 individual interaction networks were grouped according to the degree of anthropization of their areas of origin: (A): fully preserved (100% natural cover within buffer), (B): moderately anthropized (99.9–50% natural cover), and (C): highly anthropized (49–0% natural cover). Node size is associated with species abundance and link width pairwise interaction frequency. Bat labels: Acau: Anoura caudifer; Ageo: Anoura geoffroyi; Acin: Artibeus cinereus; Afim: Artibeus fimbriatus; Alit: Artibeus lituratus; Apla: Artibeus planirostris; Cper: Carollia perspicillata; Drot: Desmodus rotundus; Gcre: Gardnerycteris crenulatum; Gsor: Glossophaga soricina; Lblo: Lasiurus blossevillii; Ldek: Lonchophylla dekeyseri; Mmic: Micronycteris microtis; Mtem: Molossops temminckii; Mnig: Myotis nigricans; Msp: Myotis sp.; Pdis: Phyllostomus discolor; Plin: Platyrrhinus lineatus; Prub: Pteronotus rubiginosus; Slil: Sturnira lilium; Stil: S. tildae. Parasite labels: Acau: Anastrebla caudiferae; Amod: A. modestini; Afal: Aspidoptera falcata; Aphy: A. phyllostomatis; Banc: Basilia anceps; Bhug: B. hughscotti; Bmim: B. mimoni; Bsp.: Basilia sp. 1; Bsp1: Basilia sp. 2; Eclo: Exastinion clovisi; Mara: Megistopoda aranea; Mpro: M. proxima; Ndel: Neotrichobius delicatus; Npar: Nycterophylia parnelii; Plon: Paratrichobius longicrus; Samb: Speiseria ambigua; Scar: Strebla carvalhoi; Scur: S. curvata; Sgua: S. guajiro; Sher: S. hertigi; Swie: S. wiedermannii; Tper: Trichobioides perscpicillatus; Tang: Trichobius angulatus; Tcos: T. costalimai; Tdug: T. dugesii; Tjob: T. joblingi; Tjon: T. johnsonae; Tlon: T. lonchophyllae; Tlon: T. longipes; Tpar: T. parasiticus; Tpro: T. propinquus; Tsp: Trichobius sp.; Ttip: T. tiptoni; Tuni: T. uniformis.
Discussion
We evaluated how anthropization affects network assembly between bats and their ectoparasitic bat flies. Network’s specialization and modularity remained similar across all the sampling sites in the study, while anthropization positively affected nestedness across sites. Therefore, we rejected our hypothesis that specialization would be lower in highly anthropized sites and accepted the hypothesis that networks were more nested in those sites. Previous studies considering anthropic effects on bat-fly interactions have considered only specialization (Hernández-Martinez et al., 2018) or specialization and modularity (Urbieta et al., 2020) in their analysis, with different responses for specialization. Similar to our results, Urbieta et al. (2020) observed that modularity and specialization remained consistent among three sites with different degrees of urbanization in an area of Cerrado in central Brazil. On the other hand, Hernández-Martinez et al. (2018) recorded more specialized networks in areas with lower degrees of fragmentation in a dry forest habitat in western Mexico.
The decreased specialization of bat-fly interaction networks in degraded areas could result from the smaller number of roosts in those areas, which would lead to increased aggregation of different species in the same roost, thus facilitating intraspecific transmission and leading to accidental infestations (Urbieta et al., 2018, 2020). However, in our study area, roost availability can be considered high, as the Brazilian Federal District is a largely green city, with over 140 recorded tree species, most of them exotic (Vale et al., 2005; Alencar 2008; Guimarães 2020). Moreover, the presence of expansion joints on many of the buildings in the city also facilitates the presence of house-dwelling species, especially insectivores. The high availability of roosts allows each species to roost separately, thus avoiding the type of accidental infestations observed in previous studies (Urbieta et al., 2020) and resulting in the highly specialized networks observed throughout our study area, regardless of the degree of anthropization. It’s important to note that even in natural areas where species share roosts the specificity of Streblidae infestation is usually high, probably as a result of co-evolutionary processes between flies and hosts (Marshall, 1982; Patterson et al., 2007; Fagundes et al., 2017).
Even though we recorded no effect of anthropization on network specialization, we observed a higher level of nestedness and a reduced number of network compartments in sites located in highly anthropized areas. Increasingly nested networks indicate that, although parasites retain their host specificity, some bats tend to be more frequently parasitized by a richer ectoparasite assemblage, becoming hub species and approaching the role of keystone species often present in mutualistic networks (Mello et al., 2015). Thus, the most anthropized networks exhibit the emergence of a hierarchical assembly of interactions, as interaction asymmetries start to become apparent, that is, more generalist bats harboring specialized ectoparasites.
The increased level of nestedness and reduction of network compartments observed along the anthropization continuum in our study area suggest that specialization is not the best metric for evaluating how bat-fly networks respond to habitat degradation. Our results suggest that, even in anthropized areas, bat flies still retain their specialized host selection, possibly because of adaptations and evolutionary constraints that prevent them from parasitizing other bat species (Petersen et al., 2007; Dick and Dittmar 2013; Dittmar et al., 2015). For bats, on the other hand, anthropization may lead to a higher diversity of ectoparasites per host, possibly due to increased stress or worsened health conditions (Pilosof et al., 2012; Russo and Ancillotto 2015).
Regarding the meta-networks, we observed that the fully preserved meta-network behaved similarly to the highly anthropized one in terms of nestedness, probably because the fully preserved meta-network is larger, which may influence nestedness (Freitas Júnior et al., 2020). Such an outcome was expected, as more preserved areas tend to have richer species assemblages (Russo and Ancillotto 2015) potentially sharing roosts more often. A more informative comparison can be made between the moderately anthropized and the highly anthropized networks, which have similar sizes but behave very differently in terms of specialization and nestedness. The less anthropized meta-network is much more fragmented into compartments and is highly specialized, with fewer bats connected by common ectoparasites. Meanwhile, species previously occurring in compartments were incorporated into the network core in highly anthropized areas. Due to a lower availability of natural roosts in anthropized sites, different bat species may share man-made roosts more often or share the same natural roosts encroached upon by rural or urban environments (Kunz, 1982). This scenario may lead to the exchange of ectoparasites between bat species that were not previously in contact (Urbieta et al., 2018, 2020), which leads to a more structured network. Such exchanges may have important epidemiological implications.
Recent studies indicate that ectoparasitic bat flies can carry disease agents, such as bacteria of the genus Bartonella (Morse et al., 2012) and viruses from families Flaviviridae, Rhabdoviridae, Reoviridae, and Peribunyaviridae (Abundes-Gallegos et al., 2018; Martínez et al., 2021). Moreover, Gay et al. (2014) observed a positive correlation between ectoparasite and virus richness in Southeast Asia, which is especially concerning considering that both the prevalence of infectious diseases and the diversity of zoonotic parasites in bats are higher in urban areas (Mühldorfer et al., 2011; Nunes et al., 2017). Therefore, an increased number of accidental infestations of ectoparasites in different bat species could facilitate the spread of such disease agents in anthropized areas, leading to public health issues.
Many studies have indicated that environmental degradation, including anthropization and habitat suppression, could be responsible for the increased occurrence of emerging diseases (Daszak et al., 2001; Nabi et al., 2020). Nevertheless, the role of bat flies in microorganism transmission and the extent to which environmental degradation affects bats and their ectoparasites still requires better elucidation.
Data Availability Statement
The raw data supporting the conclusions of this article will be made available by the authors, without undue reservation.
Ethics Statement
The studies involving animals were reviewed and approved by Ethics Commission on Animal Use from University of Brasília (CEUA/UnB) (process #116319/2011). Capture in Protected Areas was permitted by Chico Mendes Institute for Biodiversity Conservation from Brazilian Ministry of the Environment (ICMBio/MMA) (license #39296-1).
Author Contributions
DR captured and identified individuals, conducted statistical analysis, and contributed to the writing of the paper; UD conducted network and statistical analysis and contributed to the writing of the paper; LA contributed to the writing of the paper and experimental design of the study.
Funding
This work was funded by CNPq (processes #130876/2013-5, #309299/2016-0, and #166314/2017-0) and CAPES (process #1562713).
Conflict of Interest
The authors declare that the research was conducted in the absence of any commercial or financial relationships that could be construed as a potential conflict of interest.
Publisher’s Note
All claims expressed in this article are solely those of the authors and do not necessarily represent those of their affiliated organizations, or those of the publisher, the editors and the reviewers. Any product that may be evaluated in this article, or claim that may be made by its manufacturer, is not guaranteed or endorsed by the publisher.
Acknowledgments
We thank SISBIOTA/ICMBio for permission to study in the research area. We also thank all the team from Bat Biology and Conservation Lab for the help during field work. We especially thank Dr. Gustavo Graciolli for the help on the identification of the fly specimens.
Supplementary Material
The Supplementary Material for this article can be found online at: https://www.frontiersin.org/articles/10.3389/fenvs.2021.752412/full#supplementary-material
References
Abundes-Gallegos, J., Salas-Rojas, M., Galvez-Romero, G., Perea-Martínez, L., Obregón-Morales, C. Y., Morales-Malacara, J. B., et al. (2018). Detection of Dengue Virus in Bat Flies (Diptera: Streblidae) of Common Vampire Bats,Desmodus rotundus, in Progreso, Hidalgo, Mexico. Vector-Borne Zoonotic Dis. 18 (1), 70–73. doi:10.1089/vbz.2017.2163
Aguiar, L. M. S., Bernard, E., and Machado, R. B. (2014). Habitat Use and Movements of Glossophaga soricina and Lonchophylla Dekeyseri (Chiroptera: Phyllostomidae) in a Neotropical savannah. Zoologia (Curitiba) 31 (3), 223–229. doi:10.1590/S1984-46702014000300003
Alencar, F. O. C. C. (2008). Arborização urbana no Distrito Federal: história e espécies do Cerrado. Brasília: NOVACAP.
Almeida-Neto, M., and Ulrich, W. (2011). A Straightforward Computational Approach for Measuring Nestedness Using Quantitative Matrices. Environ. Model. Softw. 26 (2), 173–178. doi:10.1016/j.envsoft.2010.08.003
Bascompte, J., Jordano, P., Melián, C. J., and Olesen, J. M. (2003). The Nested Assembly of Plant-Animal Mutualistic Networks. Proc. Natl. Acad. Sci. 100 (16), 9383–9387. doi:10.1073/pnas.1633576100
Bastian, M., Heymann, S., and Jacomy, M. (2009). “Gephi: an Open Source Software for Exploring and Manipulating Networks,” in Proceedings of the Third International Conference on Weblogs and Social Media, San Jose, California, USA, May 17-20, 2009 (ICWSM 2009).
Bernard, E., and Fenton, M. B. (2003). Bat Mobility and Roosts in a Fragmented Landscape in Central Amazonia, Brazil. Biotropica 35 (2), 262–277. doi:10.1111/j.1744-7429.2003.tb00285.x
Bertola, P. B., Aires, C. C., Favorito, S. E., Graciolli, G., Amaku, M., and Pinto-da-Rocha, R. (2005). Bat flies (Diptera: Streblidae, Nycteribiidae) parasitic on bats (Mammalia: Chiroptera) at Parque Estadual da Cantareira, São Paulo, Brazil: parasitism rates and host-parasite associations. Mem. Inst. Oswaldo Cruz 100 (1), 25–32. doi:10.1590/S0074-02762005000100005
Blüthgen, N. (2010). Why Network Analysis Is Often Disconnected from Community Ecology: A Critique and an Ecologist's Guide. Basic Appl. Ecol. 11 (3), 185–195. doi:10.1016/j.baae.2010.01.001
Bobrowiec, P. E. D., and Gribel, R. (2010). Effects of Different Secondary Vegetation Types on Bat Community Composition in Central Amazonia, Brazil. Anim. Conserv. 13, 204–216. doi:10.1111/j.1469-1795.2009.00322.x
Canard, E. F., Mouquet, N., Mouillot, D., Stanko, M., Miklisova, D., and Gravel, D. (2014). Empirical Evaluation of Neutral Interactions in Host-Parasite Networks. The Am. Naturalist 183, 468–479. doi:10.1086/675363
Caughley, G. (1994). Directions in Conservation Biology. J. Anim. Ecol. 63, 215–244. doi:10.2307/5542
Chacoff, N. P., Vázquez, D. P., Lomáscolo, S. B., Stevani, E. L., Dorado, J., and Padrón, B. (2012). Evaluating Sampling Completeness in a Desert Plant-Pollinator Network. J. Anim. Ecol. 81 (1), 190–200. doi:10.1111/j.1365-2656.2011.01883.x
Chao, A., Colwell, R. K., Lin, C.-W., and Gotelli, N. J. (2009). Sufficient Sampling for Asymptotic Minimum Species Richness Estimators. Ecology 90 (4), 1125–1133. doi:10.1890/07-2147.1
Colwell, R. K., Chao, A., Gotelli, N. J., Lin, S.-Y., Mao, C. X., Chazdon, R. L., et al. (2012). Models and Estimators Linking Individual-Based and Sample-Based Rarefaction, Extrapolation and Comparison of Assemblages. J. Plant Ecol. 5 (1), 3–21. doi:10.1093/jpe/rtr044
Combes, C. (2001). Parasitism: The Ecology and Evolution of Intimate Interactions. Chicago: The University of Chicago Press Ltd.
Daszak, P., Cunningham, A. A., and Hyatt, A. D. (2001). Anthropogenic Environmental Change and the Emergence of Infectious Diseases in Wildlife. Acta Tropica 78, 103–116. doi:10.1016/s0001-706x(00)00179-0
Díaz, M. M., Solari, S., Aguirre, L. F., Aguiar, L. M. S., and Barquez, R. M. (2016). Clave de identificación de los murciélagos de sudamérica. Tucumán: Programa de Conservación de los Murciélagos de Argentina PCMA.
Dick, C. W., and Dittmar, K. (2013). “Parasitic Bat Flies (Diptera: Streblidae and Nycteribiidae): Host Specificity and Potential as Vectors,” in Bats (Chiroptera) as Vectors of Diseases and Parasites. Editors S. Klimpel, and H. Mehlhorn (Berlin: Springer), 131–155. doi:10.1007/978-3-642-39333-4_6
Dittmar, K., Morse, S. F., Dick, C. W., and Patterson, B. D. (2015). “Bat Fly Evolution from the Eocene to the Present (Hippoboscoidea, Streblidae and Nycteribiidae),” in Parasite Diversity and Diversification: Evolutionary Ecology Meets Phylogenetics. Editors S. Morand, B. R. Krasnov, and D. T. J. Littlewood (Cambridge: Cambridge University Press), 246–264.
Dormann, C. F., Gruber, B., and Fründ, J. (2008). Introducing the Bipartite Package: Analysing Ecological Networks. R. News 8 (2), 8–11. http://www.biom.uni-freiburg.de/Dateien/PDF/dormann2008rnews.pdf.
Dormann, C. F., Fründ, J., Blüthgen, N., and Gruber, B. (2009). Indices, Graphs and Null Models: Analyzing Bipartite Ecological Networks. Toecolj 2, 7–24. doi:10.2174/1874213000902010007
Esbérard, C., Astúa, D., Geise, L., Costa, L., and Pereira, L. (2012). Do young Carollia perspicillata (Chiroptera: Phyllostomidae) Present Higher Infestation Rates of Streblidae (Diptera). Braz. J. Biol. 72 (3), 617–621. doi:10.1590/S1519-69842012000300027
Fagundes, R., Antonini, Y., and Aguiar, L. M. (2017). Overlap in Cave Usage and Period of Activity as Factors Structuring the Interactions between Bats and Ectoparasites. Zool. Stud. 56, e22. doi:10.6620/ZS.2017.56-22
Fahrig, L. (2003). Effects of Habitat Fragmentation on Biodiversity. Annu. Rev. Ecol. Evol. Syst. 34, 487–515. doi:10.1146/annurev.ecolsys.34.011802.132419
Júnior, L. D. F., De Araújo, W. S., and Falcão, L. A. D. (2020). Structure of the Interaction Networks between Bats (Mammalia: Chiroptera) and Ectoparasite Flies (Diptera: Streblidae, Nycteribiidae) on a Latitudinal Gradient. Acta Chiropterologica 22 (1), 187–196. doi:10.3161/15081109ACC2020.22.1.018
Gay, N., Olival, K. J., Bumrungsri, S., Siriaroonrat, B., Bourgarel, M., and Morand, S. (2014). Parasite and Viral Species Richness of Southeast Asian Bats: Fragmentation of Area Distribution Matters. Int. J. Parasitol. Parasites Wildl. 3, 161–170. doi:10.1016/j.ijppaw.2014.06.003
Guerrero, R. (1993). Catalogo de los Streblidae (Diptera: Pupipara) parasitos de murciélagos (Mammalia: Chiroptera) del Nuevo Mundo. I. Clave para los géneros y Nycterophillinae. Acta Biol. Venez. 14, 61–75.
Guerrero, R. (1994a). Catalogo de los Streblidae (Diptera: Pupipara) parasitos de murciélagos (Mammalia: Chiroptera) del Nuevo Mundo. II. Los grupos: pallidus, caecus, major, uniformis y longipes del género Trichobius Gervais, 1844. Acta Biol. Venez. 15, 1–18.
Guerrero, R. (1994b). Catalogo de los Streblidae (Diptera: Pupipara) parasitos de murciélagos (Mammalia: Chiroptera) del Nuevo Mundo. IV. Trichobiinae com alas desarrolladas. Bol. Entomol. Venez. 9, 161–192.
Guerrero, R. (1995a). Catalogo de los Streblidae (Diptera: Pupipara) parasitos de murciélagos (Mammalia: Chiroptera) del Nuevo Mundo. III. Los gupos: dugesii, dunni y phyllostomae del género Trichobius Gervais, 1844. Acta Biol. Venez. 15, 1–27.
Guerrero, R. (1995b). Catalogo de los Streblidae (Diptera: Pupipara) parasitos de murciélagos (Mammalia: Chiroptera) del Nuevo Mundo. V. Trichobiinae con alas reducidas o ausentes y miscelaneos. Bol. Entomol. Venez. 10, 135–160.
Guerrero, R. (1996). Catalogo de los Streblidae (Diptera: Pupipara) parasitos de murciélagos (Mammalia: Chiroptera) del Nuevo Mundo. VI. Streblinae. Acta Biol. Venez. 16, 1–25.
Guimarães, M. M. (2020). A influência da arborização urbana e Do ruído sobre a avifauna Do Plano Piloto de Brasília. Master’s thesis. Brasília, DF, Brazil: University of Brasília.
Haddad, N. M., Brudvig, L. A., Clobert, J., Davies, K. F., Gonzalez, A., Holt, R. D., et al. (2015). Habitat Fragmentation and its Lasting Impact on Earth's Ecosystems. Sci. Adv. 1 (1), e1500052. doi:10.1126/sciadv.1500052
Hernández-Martínez, J., Morales-Malacara, J. B., Alvarez-Añorve, M. Y., Amador-Hernández, S., Oyama, K., and Avila-Cabadilla, L. D. (2018). Drivers Potentially Influencing Host-Bat Fly Interactions in Anthropogenic Neotropical Landscapes at Different Spatial Scales. Parasitology 146, 74–88. doi:10.1017/S0031182018000732
Hiller, T., Vollstädt, M. G. R., Brändel, S. D., Page, R. A., and Tschapka, M. (2021). Bat-bat Fly Interactions in Central Panama: Host Traits Relate to Modularity in a Highly Specialised Network. Insect Conserv Divers. 14, 686–699. doi:10.1111/icad.12508
Ings, T. C., Montoya, J. M., Bascompte, J., Blüthgen, N., Brown, L., Dormann, C. F., et al. (2009). Review: Ecological Networks - Beyond Food Webs. J. Anim. Ecol. 78, 253–269. doi:10.1111/j.1365-2656.2008.01460.x
Kunz, T. H. (1982). “Roosting Ecology of Bats,” in Ecology of Bats. Editor T. H. Kunz (New York, NY: Plenum Press), 1–55. doi:10.1007/978-1-4613-3421-7_1
Löwenberg-Neto, P. (2008). The Structure of the Parasite-Host Interactions between Philornis (Diptera: Muscidae) and Neotropical Birds. J. Trop. Ecol. 24 (5), 575–580. doi:10.1017/S0266467408005348
Marshall, A. G. (1982). “Ecology of Insects Ectoparasitic on Bats,” in Ecology of Bats. Editor T. H. Kunz (New York, NY: Plenum Press), 369–401. doi:10.1007/978-1-4613-3421-7_10
Martínez, M. M. R., Lopez, M. P. I., Iñiguez-Dávalos, L. I., Yuill, T., Orlova, M. V., and Reeves, W. K. (2016). New Records of Ectoparasitic Acari (Arachnida) and Streblidae (Diptera) from Bats in Jalisco, Mexico. J. Vector Ecol. 41 (2), 309–313. doi:10.1111/jvec.12228
Mbora, D. N. M., and McPeek, M. A. (2009). Host Density and Human Activities Mediate Increased Parasite Prevalence and Richness in Primates Threatened by Habitat Loss and Fragmentation. J. Anim. Ecol. 78, 210–218. doi:10.1111/j.1365-2656.2008.01481.x
Mello, M. A. R., Rodrigues, F. A., Costa, L. d. F., Kissling, W. D., Şekercioğlu, Ç. H., Marquitti, F. M. D., et al. (2015). Keystone Species in Seed Dispersal Networks Are Mainly Determined by Dietary Specialization. Oikos 124 (8), 1031–1039. doi:10.1111/oik.01613
Morse, S. F., Olival, K. J., Kosoy, M., Billeter, S., Patterson, B. D., Dick, C. W., et al. (2012). Global Distribution and Genetic Diversity of Bartonella in Bat Flies (Hippoboscoidea, Streblidae, Nycteribiidae). Infect. Genet. Evol. 12, 1717–1723. doi:10.1016/j.meegid.2012.06.009
Mühldorfer, K., Speck, S., Kurth, A., Lesnik, R., Freuling, C., Müller, T., et al. (2011). Diseases and Causes of Death in European Bats: Dynamics in Disease Susceptibility and Infection Rates. PLoS One 6, e29773. doi:10.1371/journal.pone.0029773
Muñoz, L., Aguilera, M., and Casanueva, M. E. (2003). Prevalencia e intensidad de ectoparasitos asociados a Tadarida brasiliensis (Geoffroy Saint-Hilaire, 1824) (Chiroptera: Molossidae) en Concepcion. Gayana (Concepc.) 67 (1), 1–8. doi:10.4067/S0717-65382003000100001
Nabi, G., Siddique, R., Ali, A., and Khan, S. (2020). Preventing Bat-Born Viral Outbreaks in Future Using Ecological Interventions. Environ. Res. 185, 109460. doi:10.1016/j.envres.2020.109460
Nunes, H., Rocha, F. L., and Cordeiro-Estrela, P. (2017). Bats in Urban Areas of Brazil: Roosts, Food Resources and Parasites in Disturbed Environments. Urban Ecosyst. 20, 953–969. doi:10.1007/s11252-016-0632-3
Oksanen, J., Blanchet, F. G., Friendly, M., Kindt, R., Legendre, P., McGlinn, D., et al. (2020). Vegan: Community Ecology Package. R Package Version. 2.5-7. Available at: https://cran.r-project.org/web/packages/vegan/vegan.pdf (Accessed June 10, 2021).
Olesen, J. M., Bascompte, J., Dupont, Y. L., and Jordano, P. (2007). The Modularity of Pollination Networks. Proc. Natl. Acad. Sci. 104 (50), 19891–19896. doi:10.1073/pnas.0706375104
Patterson, B. D., Dick, C. W., and Dittmar, K. (2007). Roosting Habits of Bats Affect Their Parasitism by Bat Flies (Diptera: Streblidae). J. Trop. Ecol. 23 (2), 177–189. doi:10.1017/S0266467406003816
Patterson, B. D., Dick, C. W., and Dittmar, K. (2008a). Sex Biases in Parasitism of Neotropical Bats by Bat Flies (Diptera: Streblidae). J. Trop. Ecol. 24 (4), 387–396. doi:10.1017/S0266467408005117
Patterson, B. D., Dick, C. W., and Dittmar, K. (2008b). Parasitism by Bat Flies (Diptera: Streblidae) on Neotropical Bats: Effects of Host Body Size, Distribution, and Abundance. Parasitol. Res. 103, 1091–1100. doi:10.1007/s00436-008-1097-y
Petersen, F. T., Meier, R., Kutty, S. N., and Wiegmann, B. M. (2007). The Phylogeny and Evolution of Host Choice in the Hippoboscoidea (Diptera) as Reconstructed Using Four Molecular Markers. Mol. Phylogenet. Evol. 45, 111–122. doi:10.1016/j.ympev.2007.04.023
Peterson, B. V., and Wenzel, R. L. (1987). “Nycteribiidae,” in Manual of Neartics Diptera. Editors J. F. Mcalpine, B. V. Peterson, G. E. Shewell, H. J. Teskey, J. R. Vockeroth, and D. M. Wood (Ottawa: Minister of Supply and Services), 2, 1283–1301.
Pilosof, S., Dick, C. W., Korine, C., Patterson, B. D., and Krasnov, B. R. (2012). Effects of Anthropogenic Disturbance and Climate on Patterns of Bat Fly Parasitism. PLoS ONE 7 (7), e41487. doi:10.1371/journal.pone.0041487
Presley, S. J., and Willig, M. R. (2008). Intraspecific Patterns of Ectoparasite Abundances on Paraguayan Bats: Effects of Host Sex and Body Size. J. Trop. Ecol. 24 (1), 75–83. doi:10.1017/S0266467407004683
QGIS Development Team (2021). QGIS Geographic Information System. Open Source Geospatial Foundation Project. Available at: http://qgis.osgeo.org. (Accessed April 27, 2021).
R Core Team (2015). R: A Language and Environment for Statistical Computing. Vienna, Austria: R Foundation for Statistical Computing. Available at: www.R-project.org/(Accessed June 10, 2019).
Ramalho, D. F., Graciolli, G., and Aguiar, L. M. S. (2018). Bat Fly (Diptera: Streblidae) Parasitism in Degraded and Preserved Areas in a Neotropical Savanna. Mastozool. Neotrop 25 (1), 245–250. doi:10.31687/saremmn.18.25.1.0.21
Ratter, J., Ribeiro, J. F., and Bridgewater, S. (1997). The Brazilian Cerrado Vegetation and Threats to its Biodiversity. Ann. Bot. 80, 223–230. doi:10.1006/anbo.1997.0469
Russo, D., and Ancillotto, L. (2015). Sensitivity of Bats to Urbanization: A Review. Mamm. Biol. 80, 205–212. doi:10.1016/j.mambio.2014.10.003
Saldaña-Vázquez, R. A., Sandoval-Ruiz, C. A., Veloz-Maldonado, O. S., Durán, A. A., and Ramírez-Martínez, M. M. (2019). Host Ecology Moderates the Specialization of Neotropical Bat-Fly Interaction Networks. Parasitol. Res. 118, 2919–2924. doi:10.1007/s00436-019-06452-1
Schleuning, M., Ingmann, L., Strauß, R., Fritz, S. A., Dalsgaard, B., Matthias Dehling, D., et al. (2014). Ecological, Historical and Evolutionary Determinants of Modularity in Weighted Seed-Dispersal Networks. Ecol. Lett. 17 (4), 454–463. doi:10.1111/ele.12245
Souza, C. M., Z. Shimbo, J., Rosa, M. R., Parente, L. L., A. Alencar, A., Rudorff, B. F. T., et al. (2020). Reconstructing Three Decades of Land Use and Land Cover Changes in Brazilian Biomes with Landsat Archive and Earth Engine. Remote Sensing 12 (17), 2735. doi:10.3390/rs12172735
Threlfall, C., Law, B., Penman, T., and Banks, P. B. (2011). Ecological Processes in Urban Landscapes: Mechanisms Influencing the Distribution and Activity of Insectivorous Bats. Ecography 34, 814–826. doi:10.1111/j.1600-0587.2010.06939.x
Threlfall, C., Law, B., and Banks, P. B. (2013). Odour Cues Influence Predation Risk at Artificial Bat Roosts in Urban bushland. Biol. Lett. 9, 20121144. doi:10.1098/rsbl.2012.1144
Urbieta, G. L., Torres, J. M., Anjos, E. A. C. D., Carvalho, C. M. E., and Graciolli, G. (2019). Parasitism of Bat Flies (Nycteribiidae and Streblidae) on Bats in Urban Environments: Lower Prevalence, Infracommunities, and Specificity. Acta Chiropterologica 20, 511–518. doi:10.3161/15081109ACC2018.20.2.021
Urbieta, G. L., Graciolli, G., and Vizentin-Bugoni, J. (2020). Modularity and Specialization in Bat-Fly Interaction Networks Are Remarkably Consistent across Patches within Urbanized Landscapes and Spatial Scales. Curr. Zool. 67 (4), 403–410. doi:10.1093/cz/zoaa072
Vázquez, D. P., Poulin, R., Krasnov, B. R., and Shenbrot, G. I. (2005). Species Abundance and the Distribution of Specialization in Host-Parasite Interaction Networks. J. Anim. Ecol. 74 (5), 946–955. doi:10.1111/j.1365-2656.2005.00992.x
Vale, A. T. d., Sarmento, T. R., and Almeida, A. N. (2005). Caracterização e uso de madeiras de galhos de árvores provenientes da arborização de Brasília, DF. Ciênc. Florest. 15 (4), 411–420. doi:10.5902/198050981878
Wenzel, R. L., Tipton, V. J., and Kiewlicz, A. (1966). “The Streblid Bat Flies of Panama (Diptera: Calyptera: Streblidae),” in Ectoparasites of Panama. Editors R.L. Wenzel, and V.J. Tipton (Chicago, IL: Field Museum of Natural History), 405–675.
Whitaker, J. O. (1988). “Collecting and Preserving Ectoparasites for Ecological Study” in Ecological and Behavioral Methods for the Study of Bats. Editor J.H. Kunz (Washington: Smithsonian Institution Press), 459–474.
Willig, M. R., Presley, S. J., Bloch, C. P., Hice, C. L., Yanoviak, S. P., Díaz, M. M., et al. (2007). Phyllostomid Bats of Lowland Amazonia: Effects of Habitat Alteration on Abundance. Biotropica 39, 737–746. doi:10.1111/j.1744-7429.2007.00322.x
Keywords: Brazil, Cerrado (Brazilian savanna), Chiroptera, habitat degradation, Hippoboscoidea, Streblidae
Citation: Ramalho DF, Diniz UM and Aguiar LMS (2021) Anthropization Affects the Assembly of Bat-Bat Fly Interaction Networks. Front. Environ. Sci. 9:752412. doi: 10.3389/fenvs.2021.752412
Received: 03 August 2021; Accepted: 22 September 2021;
Published: 08 October 2021.
Edited by:
Alvaro Soutullo, Universidad de la República, UruguayReviewed by:
Valeria Salinas-Ramos, University of Naples Federico II, ItalyEder Barbier, Federal University of Pernambuco, Brazil
Copyright © 2021 Ramalho, Diniz and Aguiar. This is an open-access article distributed under the terms of the Creative Commons Attribution License (CC BY). The use, distribution or reproduction in other forums is permitted, provided the original author(s) and the copyright owner(s) are credited and that the original publication in this journal is cited, in accordance with accepted academic practice. No use, distribution or reproduction is permitted which does not comply with these terms.
*Correspondence: Daniel F. Ramalho, ZGFuaWVsLmYucmFtYWxob0BnbWFpbC5jb20=