- 1Department of Fisheries and Aquatic Sciences, University of Eldoret, Eldoret, Kenya
- 2Department of Fisheries and Aquatic Sciences, Mzuzu University, Mzuzu, Malawi
- 3Aquatic Science and Ecosystems Group, University of KwaZulu-Natal, Durban, South Africa
Expansion of agriculture is particularly worrying in tropical regions of the world, where native forests have been replaced by croplands and grasslands, with severe consequences for biodiversity conservation and ecosystem functioning. However, limited data exist on the effects of agriculture on the functioning of tropical streams. We conducted a leaf litter decomposition experiment in coarse- and fine-mesh litterbags using the three species of leaves (Eucalyptus globulus [non-native], Vernonia myriantha, and Syzygium cordatum [indigenous]) in three forested and agricultural streams to determine the effect of agriculture on instream leaf litter decomposition in headwater stream sites. We also examined the functional composition of macroinvertebrates in the streams through the contents of benthic kick samples. Agricultural streams had a less dense riparian canopy and smaller abundance of coarse organic particulate matter, and higher electric conductivity and suspended solids than forested streams. In terms of the effects of litter quality on decomposition rates, Vernonia had the fastest decomposition rates while Eucalyptus had the slowest in both forested and agricultural sites. Shredder invertebrates were less abundant in agricultural streams, and in both stream types, they were less diverse and abundant than other functional groups. Overall, leaf litter decomposition rates did not respond to agricultural land-use. The hypothesized negative effects of agriculture on organic matter processing were minimal and likely modulated by intact riparian zones along agricultural streams.
Introduction
Land-use change from native forestry to other uses is a major concern in many parts of the world because of its negative influence on water quality and quantity, biodiversity, and the structure and functioning of streams and rivers (Allan, 2004; Boyero et al., 2016; Masese et al., 2017; Jun et al., 2019). Land-use change led to shifts in the functional and structural organization of aquatic assemblages and an overall loss of ecological integrity of streams (Masese et al., 2009; Wantzen and Mol, 2013; Hepp et al., 2016). For example, conversion of native forests to agriculture tends to degrade streams through nutrient enrichment, soil erosion and sedimentation, increase in mean water temperature, and loading of toxic substances such as pesticides and herbicides (Osano et al., 2003; Matthaei et al., 2010; Jinggut et al., 2012).
Apart from agricultural land-use, plantation forestry is another common practice along riparian zones of streams and rivers. This has often introduced non-native trees with notable effects on aquatic and terrestrial ecosystems (Reinhart and Vandevoort, 2006). Monocultures of non-native tree species in riparian zones, such as Eucalyptus, frequently correspond with changes in organic matter standing stocks, modified food webs and flow regimes, reduced leaf processing by shredders, and changes in the composition of aquatic communities in streams (Cooper et al., 2013; Silva-junior et al., 2014). Changes in land-use and alteration of riparian vegetation can have both distal and proximal controls on abiotic and biotic factors that drive different pathways of litter decomposition in streams. For instance, the efficiency of shredders to convert leaf organic matter to secondary production greatly depends on the quality of the leaves (Reinhart and Vandevoort, 2006; C et al., 2012). An increase in mean water temperature as a result of clearance of riparian vegetation is known to promote microbial decomposition over shredder-mediated decomposition (Boyero et al., 2011; Fugère et al., 2020). Similarly, nutrient enrichment and sediments from agricultural lands can stimulate microbial decomposition (Ferreira et al., 2006)Tank et al., 2010 and impair shredder assemblages and their role in leaf litter decomposition (Danger et al., 2012; Woodward et al., 2012; Mustonen et al., 2016), respectively.
Macroinvertebrates play a major role in ecosystem-level processes and their functional composition in terms of functional feeding groups are indicators of ecosystem attributes and the health of streams and rivers (Boyero et al., 2015; Merritt et al., 2017; Sitati et al., 2021). This approach has been extended to leaf litter decomposition rates (Gessner and Chauvet, 2002; Young and Matthaei, 2008). Several studies have investigated the influence of agriculture on the composition of macroinvertebrates functional feeding groups and leaf litter decomposition in tropical streams (Jinggut et al., 2012; Boyero et al., 2015; Fugère et al., 2018; Cornejo et al., 2020). Compared to temperate streams, higher mean water temperature and low diversity of shredders in tropical streams have been suggested as the main reasons why litter decomposition may be driven mainly by microorganisms (Irons et al., 1994; Boyero et al., 2011; Tiegs et al., 2019). Although some studies have reported a diverse shredders guild in tropical streams (Cheshire et al., 2005; Yule et al., 2009; Masese et al., 2014a; Boyero et al., 2015), other studies have also reported a depauperate diversity of shredders (Dobson et al., 2002; Watnzen et al., 2008; Li et al., 2009). This implies that a loss in shredder diversity, which is very sensitive to poor water quality and habitat degradation (Yule et al., 2009; Masese et al., 2014a; Masese et al., 2014b), would increase further microbially-driven leaf litter decomposition rates in tropical streams (Boyero et al., 2021).
Another important factor influencing the decomposition rates of leaf litter is its quality (Webster and Benfield, 1986; Martínez et al., 2013). The quality of leaf litter is defined by toughness, the concentration of chemical constituents such as lignins, tannins, alkaloids, nitrogen, and phosphorus (Gessner and Chauvet, 2002; Li et al., 2009; MacKenzie et al., 2013). Litter quality in turn controls the activity and abundance of microbial decomposers and shredders associated with the litter and hence litter decomposition rate (Gessner and Chauvet, 2002; Hladyz et al., 2009). High-quality leaf litter that has low concentrations of lignins, tannins, and alkaloids but with high N and P concentration (low C: N and C: P stoichiometry) generally show faster decomposition rates than more recalcitrant litter (Gessner et al., 2010; Boyero et al., 2015, 2016). Studies have shown that there is a latitudinal gradient in leaf litter quality whereby the quality of leaf litter in tropical streams is poorer than in temperate streams because of the high toughness and content of inhibitory compounds that protect leaves against herbivory (Wantzen and Wagner, 2006; Boyero et al., 2017).
In this study, we investigated the effects of agricultural land-use on shredder diversity and leaf litter decomposition in Afrotropical headwater streams in western Kenya. We quantified and compared water quality physico-chemistry, habitat quality, and the proportion of different land-use types between forested and agricultural streams. We then compared decomposition rates of leaf litter in forested and agricultural streams using fine-mesh and coarse-mesh litterbags, which allowed the distinction between microbial and shredder-mediated decomposition pathways. We further investigated the use of decomposition rates as a measure of the ecological health of the streams. We used litter of three riparian tree species (1 non-native [Eucalyptus globulus] and 2 indigenous [Syzygium cordatum, Vernonia myriantha]) to explore differences due to leaf quality and replacement of riparian vegetation by non-native species. Additionally, we examined shredder assemblages through the contents of coarse-mesh litterbags and benthic kick samples. We tested the following hypotheses: 1) there are declined in water and habitat quality, and organic matter standing stocks in agricultural streams, 2) shredder-mediated decomposition rates are higher in forested streams because of higher diversity and abundance of shredders, 3) microbial decomposition of leaf litter is higher in agricultural streams because of higher nutrient concentrations, and 4) leaf litter quality influence decomposition rates with higher quality leaf litter having higher shredder and microbial decomposition than poor quality leaf litter.
Materials and Methods
Study Area
This study was conducted in three agricultural (Kipsinende, Mlango, and Seger) and three forested (Sabor, Kipkaren, and Chepkoilel) stream sites in the headwaters of the Sosiani River in western Kenya (Figure 1). The Sosiani River is a tributary of the Nzoia River and originates from the western slopes of the Kerio Escarpment. The climate of the area is mainly tropical humid, with mean annual rainfall ranging from 900 to 2,200 mm and mean monthly temperature ranging from 13°C to 25°C which varies strongly with elevation and season. The annual rainfall pattern is bimodal, with long rains from March to June and short rains from August to October.
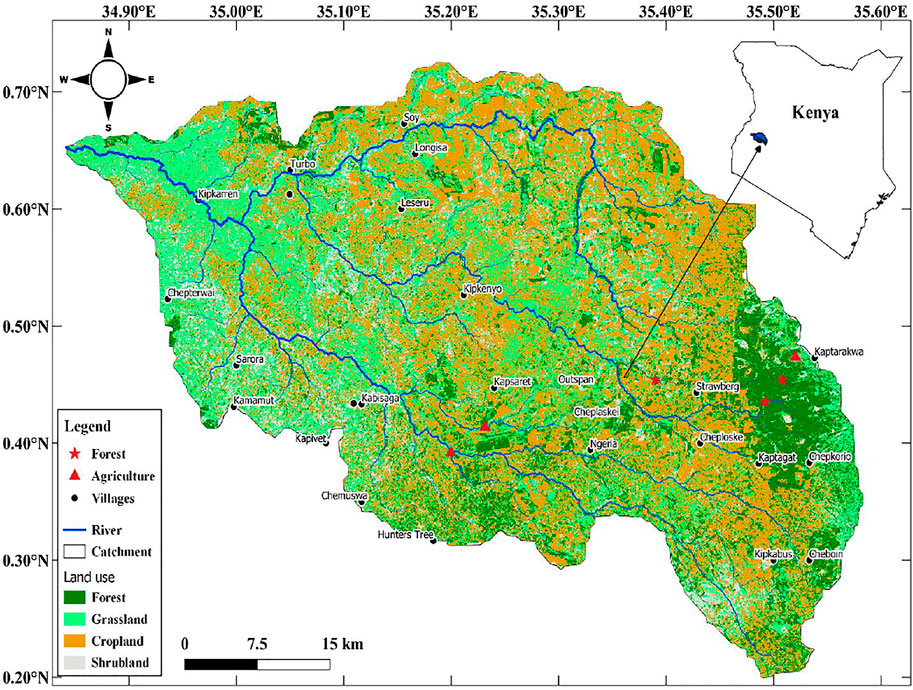
FIGURE 1. Map of Sosiani River Basin showing the position of forested (n = 3) and agricultural (n = 3) streams used for the study.
The upper Nzoia River Basin (NRB) has experienced rapid population growth over the years, which has exerted pressure on available land resources as the demand for food and fiber has increased leading to deforestation, crop farming in the catchments and along river banks, draining of wetlands for horticulture and livestock grazing, harvesting of sand from streams and rivers, and brick-making (Masese et al., 2009; Wabusya et al., 2015). Among the multiple stressors afflicting the Nzoia River basin, agriculture is of major concern due to increasing human population growth and the resultant demand for agricultural land for crops and livestock production (Masese et al., 2009; Wabusya et al., 2015). The headwater of the Sosiani River is dominated by native forest but land-use is steadily changing from forest cover to mixed croplands (mainly maize, wheat, and potatoes) and grazing areas for improved breeds of cattle. However, as opposed to other areas in western Kenya, agriculture is still mechanized and large-scale, and most streams have a well-protected riparian zone.
Analysis of Catchment Land-Use
Land-use classification and catchment maps were generated using QGIS 3.14. The semi-automatic classification plugin was used to download sentinel-2 images on April 10, 2020 for the six catchments of the streams used in this study according to Congedo (2020a, b). The images were downloaded in April 2021. The satellite images were pre-processed and processed for four categories of predominating land-use in the study area (forest, grassland, cropland, and shrubland). For all sampling sites, catchments were delineated as the entire landscape contributing surface water to the site. The area of each land use category in the catchments of each sampling site was calculated.
The six catchments of streams used in this study were categorized as either agricultural (n = 3) or forested (n = 3) depending on the proportion of land-use under the respective uses (Figure 2). Forest sites and agricultural sites were defined as those that drained catchments with the percentage of land use >50% for forest and agriculture, respectively. Agricultural land included cropland and grassland cover because the latter (grasslands) represent grazing areas for livestock which are part of agricultural activities in the area. Similarly, forested land included forests (both natural and plantation) and shrubland cover. Shrublands are part of native vegetation in the area that are remnants of natural forests that have been cleared in patches and some are regenerative.
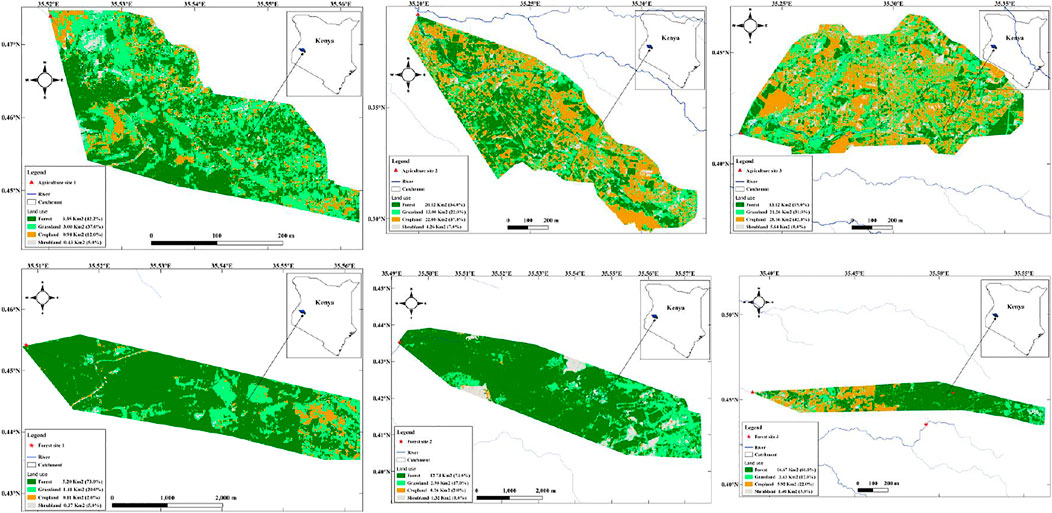
FIGURE 2. Land-use classified maps of the six catchments of the streams in the upper reaches of the Sosiani River used for this study: Upper panel = Agricultural streams (n = 3), and lower panel = forested (n = 3). Sampling sites for each stream catchment are located at the most downstream tip of each map (indicated in red).
Physical and Chemical Variables
At each site, water depth, velocity, stream width, percent canopy cover, and discharge were determined within a 100 m long stream reach. A mechanical flow meter (General Oceanics; 2030 Flow-meter, Miami, Florida) was used to measure water velocity. Stream width was measured at transects spaced at 10 m intervals along a 100-m stream reach while water depth was measured on each transect at least at 5 points across the river using a 1-m ruler. The velocity-area method was used to measure stream discharge for each study reach (Wetzel and Likens, 2000). Percentage coverage of the streambed with coarse particulate organic matter (CPOM; mainly leaf litter, sticks, seeds, flowers, and fruits) was estimated at each study reach in triplicates using a quadrat (0.5*0.5 m2). Percent canopy cover in each study reach was also estimated visually (Masese et al., 2014a).
We characterized the instream and riparian habitat conditions by scoring the variables for each study reach using the qualitative habitat evaluation index by Rankin (1995) modified for the Lake Victoria Basin (Masese et al., 2009; Raburu and Masese, 2012). The index is based on six variables, namely; substrate composition, instream cover, channel morphology, riparian zone, and bank erosion, pool/glide and riffle/run quality, and riffle/run depth. The qualitative habitat scoring was done thrice; during deployment of the litter bags and during each of the two retrieval periods (day 14 and day 28). Visual characterization of substrate composition was employed as the proportion of various types of streambed substrate (boulder, cobble, pebbles, hardpan, muck, gravel, sand, mud and detritus) (Barbour, 1999). Riparian width (m) and percent canopy cover were determined for each stream (100 m reach). Because the guidelines for maintenance of riparian zones along Kenyan streams and rivers specify a minimum of 30 m, we treated this (30 m) as the maximum size for all streams for standardization.
Measurements of pH, temperature, dissolved oxygen (DO), electrical conductivity (EC) and salinity were done in situ using a YSI multi-probe water quality meter (556 MPS, Yellow Springs Instruments, Ohio, United States). Water samples were collected from the thalweg and immediately filtered on-site for total suspended solids (TSS) and particulate organic matter (POM) using pre-combusted and pre-weighed Whatman GF/F filters (0.7 µm pore size, 47 mm diameter). Filters with amended sediments were wrapped in aluminum envelopes and preserved in a cooler at 4°C and transported to the laboratory for analysis. Unfiltered water samples were also collected using acid-washed HDPE bottles and preserved in a cooler for nutrient analysis.
In the laboratory, water samples were analyzed for soluble reactive phosphorus (SRP), nitrate (NO3), nitrite (NO2), and ammonium (NH4) following standard methods for concentration (APHA, 2005). Filters for TSS were dried at 105°C for 48 h and reweighed using an analytical balance (Citizen, Model CY220, 0.001 g). The concentration of TSS was determined gravimetrically (APHA, 2005). TSS filters were then ashed at 450°C for 5 h and re-weighed for determination of POM as the difference between TSS and ash-free-dry mass (APHA, 2005). CPOM samples were dried to a constant weight at 60°C for 48 h where various fractions of leaves, flowers, sticks and seeds were weighed independently using an analytical balance (0.001 g).
Leaf Litter Breakdown Experiment
To determine decomposition rates of the three species of leaves, an experiment was conducted during the dry months of February—March 2020. Three common riparian tree species (Supplementary Plate S1) were: an indigenous tough species, Syzygium cordatum Hochst ex. Krauss (Family Myrtaceae) [hereafter Syzygium]; a soft indigenous species, Vernonia myriantha Hook. f (Family Asteraceae) [henceforth Vernonia], and a tough non-native species Eucalyptus globulus Labill (Family Myrtaceae) [henceforth Eucalyptus]. Vernonia exhibits very soft leaves, and thus, represented the highest quality of the three leaves. In comparison, Syzygium has tough and smooth leaves whose breakdown rate was assumed to be comparable to that of the non-native Eucalyptus within the same family (Mathooko et al., 2001; Masese et al., 2014b).
Recently abscised leaves were air-dried for 2 weeks under a shade at room temperature until a constant mass was attained. Then, 4 g of each species were weighed and put into fine-mesh (0.5 mm mesh size) and coarse-mesh (10 mm mesh size) litterbags. Fine-mesh litterbags were used to exclude macroinvertebrate access and allow only microbial decomposition, whereas coarse-mesh litterbags were used to allow both macroinvertebrate and microbial-mediated processing of leaf litter. A total of 228 litterbags were used in the experiment from which 216 litterbags were divided into 3 replicates per litterbag type (fine-vs coarse-mesh) per plant species (Eucalyptus, Syzygium, and Vernonia) in each site (3 forested and 3 agriculture), for two retrieval dates (Day 14 and Day 28). The extra 12 litterbags were used to determine the initial ash-free-dry mass (AFDM) conversion factor for each leaf species (4 replicates each). Before deployment, the litterbags were tied along nylon lines to avoid any overlaps and for easy retrieval. Litterbags were secured on metal stakes hammered into the streambed in pools and riffles. The riffles and pools in each study site were selected more than 20 m apart to avoid sharing of resources. Riffles were shallower than pools, but selected pools were less than 0.5 m deep. While pools were dominated by detritus and soft sediments (gravel, sand, and mud), riffles were dominated by stable substrate (boulders, cobbles and pebbles) and embedded detritus.
During retrieval, litterbags were carefully enclosed in a 300 mm mesh net and placed in zip-lock plastic bags to avoid loss of invertebrates and leaf fragments. Litterbag samples were placed in zip-lock bags and preserved using 5% formalin until further processing. In the laboratory, the litterbags were thoroughly washed with tap water and sieved (250 μm mesh size) to separate macroinvertebrates from leaf fragments. Leaf fragments were rinsed and oven-dried at 105°C for 48 h to obtain dry mass remaining, then ashed at 550°C for 4 h and reweighed to calculate Ash-Free Dry Mass (AFDM). One set of litterbags was lost at Seger, Kipkaren and Kipsinende streams due to human tampering and sedimentation. This reduced the number of analyzed replicates in these streams to five per leaf species per mesh size, but we considered this a minor infraction that would not affect the results.
Macroinvertebrates in Kick Samples and Litterbags
Macroinvertebrate kick sampling was conducted using a semi-quantitative kick-net sampling method (Dickens and Graham, 2002). The following biotopes were delineated and sampled within each site: 1) GSM: gravel, sand and mud; 2) STONES: bedrock, boulders, cobbles, and pebbles, either under flowing or non-flowing conditions; 3) VEG: submerged and marginal vegetation (Masese et al., 2021; Sitati et al., 2021). The sampling process involved disturbing/kicking the bottom upstream of the net (500-μm mesh size), so that dislodged invertebrates are washed into the net by water currents. The attached macroinvertebrates were sampled by jabbing the sampling net repeatedly against marginal vegetation. Kicking was carried out on an area of approximately 1 m2 for a standard 1 min per biotope on two sampling occasions; day 1 of litterbag deployment and day 28 of final litterbags retrieval. Large substrate types such as boulders and cobbles were disturbed by hand and washed into the net.
Sampled macroinvertebrates were preserved separately per biotope using 75% ethyl ethanol in zip-lock bags for further processing in the laboratory. After sorting, macroinvertebrates from coarse-mesh litterbags and kick samples were preserved in vials in 75% ethyl ethanol until identification and enumeration. Identifications were done to the lowest taxon level possible, mainly genus, using several keys in guides (Day and de Moor, 2002a, b; de Moor et al., 2003a, b; Merritt et al., 2008). Macroinvertebrates were classified into four major functional feeding groups (FGGs; scrapers, shredders, collectors and predators) according to Merritt et al. (2008) and Masese et al. (2014a).
Data Analysis
We used a two-sample t-test to compare water quality variables, nutrients, organic matter and stream size variables between forested and agricultural sites. The structural and functional composition of macroinvertebrate assemblages in kick samples and coarse-mesh litterbags were described in terms of abundance and taxon richness. Two-sample t-tests were also used to test for differences in taxon richness (number of taxa) and abundance of all taxa, shredders and non-shredders in kick-samples and coarse-mesh litterbags between the two land-uses. Testing for significant differences in multivariate water and habitat quality and organic matter characteristics was done using permutational analysis of variance (PERMANOVA, 999 iterations) in the R package vegan (Oksanen et al., 2020).
Statistical differences in macroinvertebrate assemblage structure between land use types (forested vs agricultural sites) in litterbags and kick samples were tested with a one-way analysis of similarity (ANOSIM). Non-metric multidimensional scaling (nMDS) was used to display macroinvertebrate assemblages between forested and agricultural sites utilizing Bray–Curtis dissimilarities calculated from Ln-log transformed abundances. In each case, we overlay the taxa onto the nMDS plot to indicate associations of each taxon or FFG to each other and sampling sites.
Leaf decomposition rates were estimated using an exponential decay model (Boulton and Boon, 1991; Benfield, 1996):
Differences in decomposition rates (−k) were tested using three-way ANOVA whereby leaf species (Vernonia, Syzygium, and Eucalyptus), land-use (forested and agricultural), and mesh size (coarse- and fine-mesh), including interactions, were treated as the main factors and leaf litter decomposition rates (−k) as the response variable. Where there was no significant interaction between land-use and both leaf species and mesh sizes, two-way ANOVA was re-run separately for each land-use with leaf species and mesh size including interactions, as the main factors affecting decomposition rates.
We further analyzed how overall leaf litter decomposition, diversity (number) of shredder taxa and abundance of shredder individuals responded to agricultural land-use using generalized additive models (GAMs) (Wood, 2017). GAMs included land-use, the width of the riparian zone, habitat quality and percent canopy cover as fixed effects, and individual stream sites as a random effect. GAMs were fitted using the R-package mgcv (Wood and Wood, 2015) in the R platform (Team, R. C, 2017).
Statistical analyses were performed with PAST (version 3.21) software (Hammer et al. 2001) and R version 4.0.2. (Team, R. C, 2017). Figures were created in SigmaPlot (Version 12), MS Office Excel (2016).
Results
Catchment Land-Use and Habitat Quality
Agricultural streams had the combined proportion of land-use under cropland and grassland that ranged from 52 to 73% while forested streams had the combined proportion of land-use under forests and shrubland that ranged from 66 to 82% (Figure 2). There were no significant differences in habitat quality variables between forested and agricultural streams, except for the composition of the soft substrate (fine gravel, sand, and mud) whose percentage coverage of streambed was higher in agricultural streams (Table 1). The three agricultural streams in the study area had well-maintained riparian zones (Supplementary Plate S2). There were no obvious human activities in the vicinity of the study sites, except road crossings and livestock watering points at one of the agricultural sites, but study sites were selected upstream of these influences.
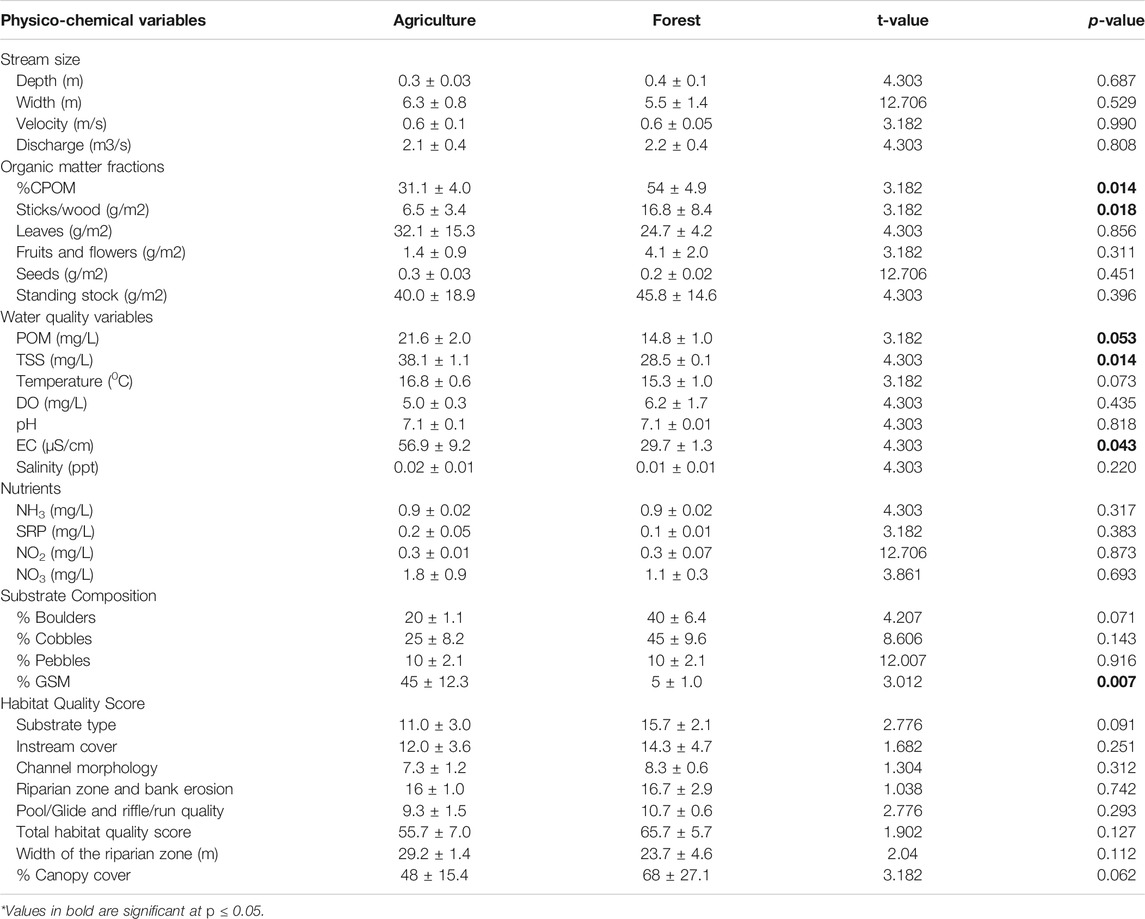
TABLE 1. Mean (±SD) physico-chemical water quality and stream size variables, organic matter and nutrients concentrations and habitat quality scores for agricultural and forested sites in the headwaters of the Sosiani River, western Kenya.
Physico-chemical Water Quality Parameters
There were significant differences (p = 0.014) in the percentage of CPOM coverage of the streambed (%CPOM) between forested and agricultural sites, with forested sites recording higher values (Table 1). Although insignificantly different between the two land-uses, mean CPOM standing stocks were higher in forested sites and different fractions of organic matter (e.g., sticks) had higher standing stocks in forested sites. Significant differences were also recorded in TSS (p = 0.014), EC (p = 0.043) and %GSM (p = 0.007) between forested and agricultural sites, with agricultural sites recording higher values (Table 1). Multivariate water quality (PERMANOVA, F = 8.4, df = 3, p = 0.107), habitat quality and organic matter (PERMANOVA, F = 1.7, df = 1, p = 0.209) did not differ between forested and agricultural sites.
Macroinvertebrate Assemblages
Non-metric multidimensional scaling (NMDS) ordination of macroinvertebrate composition indicated that most forested and agricultural sites were more clearly separated in litterbag samples than in kick samples (Figure 3). These differences were further confirmed by ANOSIM which indicated that there were no significant differences in macroinvertebrate assemblage in kick samples between forested and agricultural sites (R-statistic = 0.11, p = 0.391). However, there were significant differences in macroinvertebrate composition in coarse-mesh litterbags between forested and agricultural sites (R-statistic = 0.21, p = 0.028).
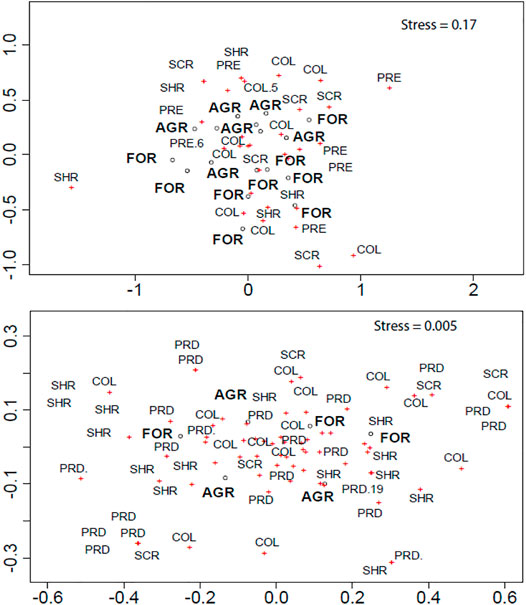
FIGURE 3. Non-metric multidimensional scaling (NMDS) ordination of macroinvertebrate functional feeding groups (FFGs) in coarse-mesh litterbags (upper panel) and kick samples (lower panel). The same names of overlapping sites and FFGs have been deleted for clarity. FOR, forested sites; AGR, agricultural sites; COL, collectors; PRE, predators; SCR, scrapers; SHR, shredders.
In kick samples, a total of 17,114 macroinvertebrate individuals were collected from the six sites, of which 7,999 were from agricultural sites while 9,115 were from forested sites. The macroinvertebrate samples comprised of 14 orders, 51 families, and 75 genera (Supplementary Table S2). About 119 taxa were identified of which seven (Neoperla sp., Rheoptilum sp., Orthotrichia sp., Crambidae sp., Sciomyzidae, Chrysomelidae, and Oligoneuriopsis sp.) were restricted to forested sites, while six (Mesovelia, Musca, Ranatra, Gerris, Planorbi,zs, and Aranaea sp.) were restricted to agricultural sites. The macroinvertebrates comprised of 30 predators, 19 collectors, 17 shredders, and 9 scrapers. Three of the shredder taxa (Crambidae, Chrysomelidae and Potamonautes sp.) occurred only in forested sites. Collectors (forested = 47.5% and agricultural = 45.3%) were the most abundant while shredders (forested = 7.3% and agricultural = 7.5%) were the least abundant. Predators were more abundant in agricultural (21%) than in forested (4.64%) sites, while scrapers were more abundant in forested (42%) than in agricultural (24%) sites. Even though insignificant, the number of taxa and non-shredder taxa were higher in agricultural sites (Figure 4). Shredder abundance and taxon richness were significantly higher in forested sites than in agricultural sites (Figure 4).
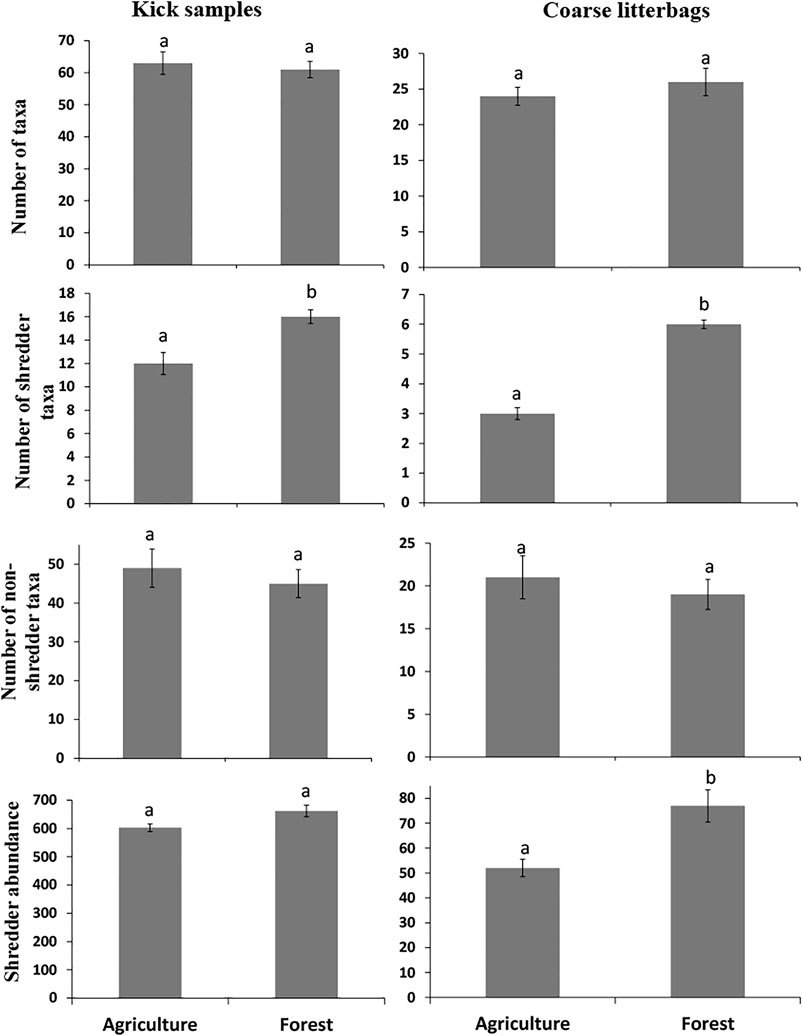
FIGURE 4. Mean (±SE, n = 3) number of taxa, number of shredder taxa, number of non-shredder taxa and shredder abundance in agriculture and forest sites for kick samples and coarse-mesh litterbags. The different letters indicate significant differences among land-uses (two-sample t-test). Note the different values in y-axes.
A total of 1884 macroinvertebrate individuals were collected from coarse-mesh litterbags, of which 14.6% were classified as shredders (Supplementary Table S1). Both taxon richness and abundance of shredders were higher in forested sites compared to agricultural sites (Figure 4) with Potamonaute sp. and Acanthiops sp. dominating in both land-use types, while Lepidostoma sp. dominated in forested sites. Vernonia recorded the highest number of macroinvertebrate individuals (1,306), followed by Syzygium 1,138) and lastly Eucalyptus (903).
Leaf Litter Decomposition
After 28 days of deployment, there were minor differences in instream decomposition rates between forested and agricultural streams for all three species of leaves (Vernonia, Syzygium, and Eucalyptus) (Figure 5; Table 2). This implies that land-use change from forestry to agriculture have a minor influence on the role of shredders in the decomposition of leaf litter in the studied streams which depended on the leaf species; only Eucalyptus had a higher decomposition rate mediated by shredders (coarse-mesh litterbags) in forested than agricultural streams. However, there was a strong response in microbial decomposition with Vernonia and Syzygium recording lower decomposition rates in agricultural streams. Overall, the decomposition rate (−k/day) was highest in Vernonia in both forested and agricultural streams, followed by Eucalyptus and Syzygium (Figure 5). Interestingly, overall decomposition rates in Eucalyptus and Syzygium showed similar trends, indicating that shredders did not discriminate against the non-native Eucalyptus leaves.
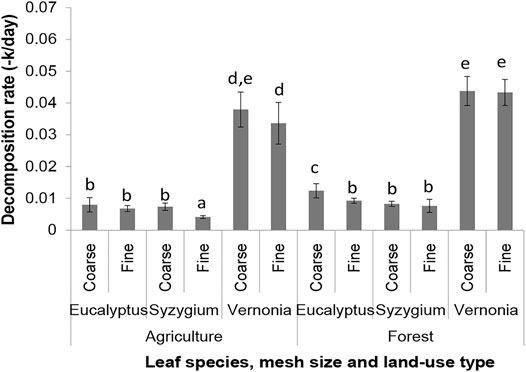
FIGURE 5. Decomposition rates (−k/day) of Eucalyptus, Syzygium and Vernonia leaves in coarse- and fine-mesh litterbags in forested and agricultural streams after 28 days of deployment. Different letters on bars represent post hoc mean differences (two-sample t-tests) in decomposition rates among treatments.
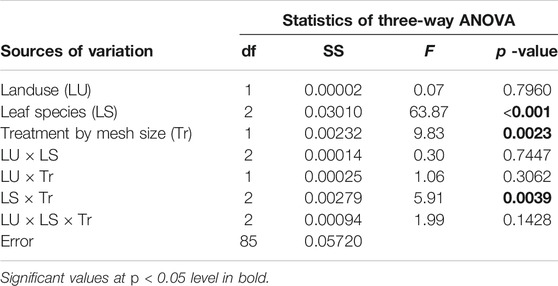
TABLE 2. Results of three-way ANOVA exploring variation in leaf litter decomposition rates (−k) between land use types (forest and agriculture), leaf species (Vernonia, Syzygium, and Eucalyptus), and treatment by mesh size (coarse and fine mesh) after 28 days of deployment. Degrees of freedom (df), sums of squares (SS), F - statistic and p -values are shown.
GAMs did not identify significant influences of land-use, the width of the riparian zone and habitat quality on mean decomposition rates, the number of shredder taxa, and the abundance of shredder individuals (Table 3). Percent canopy cover influence the number of shredder taxa, but not leaf litter decomposition or abundance of shredders. Overall, the role of agriculture on proximate drivers of leaf litter decomposition in streams (nutrients concentrations, shredder diversity, and abundance) seemed to be minimal.
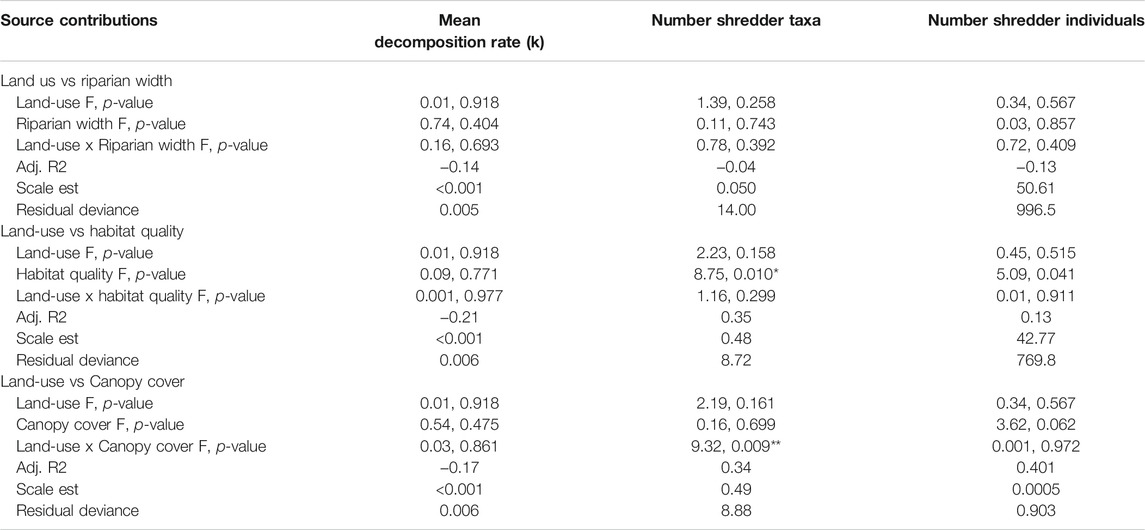
TABLE 3. Summary of generalized additive mixed models (GAM) of the effects of land-use (forest vs agriculture), riparian width, and percent canopy cover over the streams on mean decomposition rates (k) of the three species of leaves, number of shredder taxa, and abundance of shredder individuals in the study sites. In all cases, d.f. = 1. *p < 0.05, **p < 0.01.
The role of shredder in decomposition rates depended on leaf species (litter quality). For all leaf species, decomposition rates were slightly higher in coarse- (shredder + microbial decomposition) than in fine-mesh (microbial decomposition only) litterbags (kc/kf > 1) (Table 4). Decomposition rates in coarse-mesh litterbags were similar for Vernonia in both forested and agricultural streams and were either highest or second highest. In forested streams, the mean kc/kf coefficient was highest for Vernonia (kc/kf = 1.38) (Table 4). However, in agricultural streams, kc/kf coefficients were highest for Syzygium (kc/kf = 1.84). Eucalyptus had the lowest decomposition rates attributable to shedders (lowest kc/kf values) in the agricultural streams while Syzygium had the lowest in forested streams.
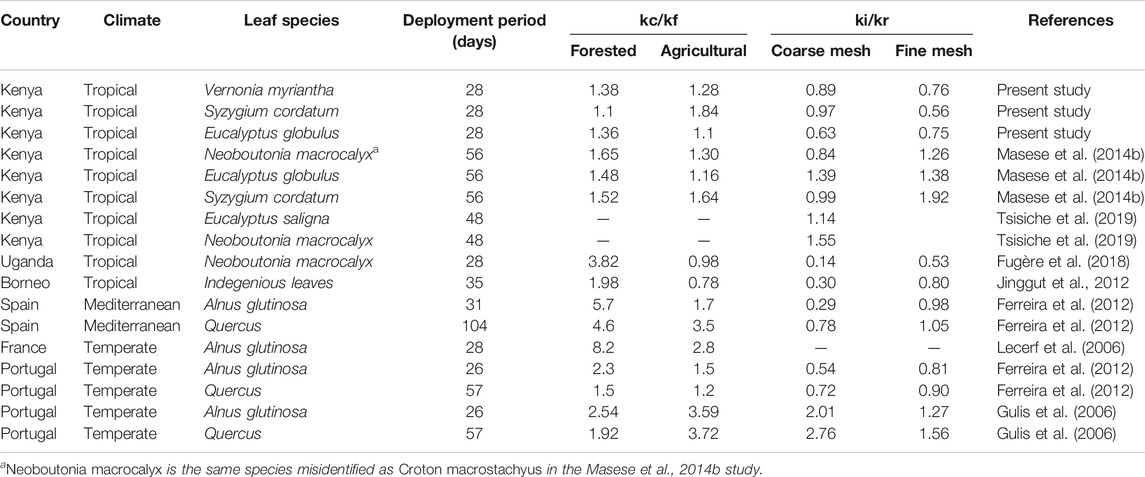
TABLE 4. Means of kc/kf coefficients in leaf litter decomposition rates between coarse-mesh (kc) and fine-mesh (kf) litterbags and ki/kr coefficients of decomposition rates in impacted (ki) and reference (kr) streams in coarse- and fine-mesh litterbags across different streams with varying days of deployment. The coefficients show the contribution of shredders (coarse-mesh) relative to microbes (fine-mesh) in leaf litter decomposition rates.
The effect of agriculture (ki/kr) on decomposition rates was less than that of shredders (kc/kf) (Table 4). Overall decomposition rates were higher in forested (reference) streams (ki/kr < 1) than in agricultural streams for all leaf species and litterbag types (Table 4). In comparison with other studies in Kenya and other tropical, Mediterranean and temperate streams, kc/kf coefficients were much higher in the Mediterranean and most temperate streams irrespective of land-use (reference and impacted) (Table 4). For ki/kr, most values in the Mediterranean and temperate streams were within the range of tropical values in both coarse- and fine-mesh litterbags, although values were much higher (>2) in coarse-mesh litterbags for two species of leaves in Portugal (Table 4).
Discussion
Land-use, particularly the proportion of agricultural land, has recently increased in many parts of the world, with increasing effects on the structure and functioning of riverine ecosystems. Here, we investigated the effects of agricultural land-use on water and habitat quality, the composition of macroinvertebrates, with a focus on shredders, and leaf litter decomposition rates of three species of leaves (two indigenous and one exotic) in headwater streams in western Kenya. There were minimal effects of agriculture on water and habitat quality in streams. Overall decomposition rates by shredders and microbes (coarse- and fine-mesh litterbags, respectively), did not change with land-use as much as it differed among leaf species, implying that decomposition rates were driven by leaf quality and not differences in the diversity and abundance of shredders between forested and agricultural streams. Thus, the hypothesized increased role of shredders in forested streams and microbes in agricultural streams on leaf litter decomposition was not supported in this study.
Lack of land-use effects on decomposition rates in this study is intriguing and contrary to similar studies on the effects of agriculture on organic matter processing in tropical (Jinggut et al., 2012; Masese et al., 2014b; Silva-junior et al., 2014; Fugère et al., 2020) and temperate (Woodward et al., 2012; Ferreira et al., 2015) streams. Although our agricultural sites had significantly elevated levels of total suspended solids and electrical conductivity, which are indicators of anthropogenic activities on water quality (Minaya et al., 2013; Ontumbi et al., 2015; Masese et al., 2017; Kroese et al., 2020), these were deemed within tolerable limits of most sensitive taxa, including shredders, in these sites. Riparian zones in agricultural streams were well maintained, and this increased the quality of instream habitats, canopy cover and standing stocks of organic matter (Table 1) that are depended upon by shredders. Indeed, multivariate water and habitat quality and organic matter standing stocks did not differ between forested and agricultural sites and all major shredder taxa such as Potamonutes sp., Acanthiops sp., and Lepidostoma sp. were widely distributed in all land-uses.
The hypothesized increased role of nutrients in leaf litter decomposition in agricultural streams was not recorded in this study. Nutrient concentrations, which have been reported in some studies to increase organic matter processing rates in streams (Woodward et al., 2012; Ferreira et al., 2015; Rosemond et al., 2015), did not differ between forested and agricultural sites in this study (Table 1). However, nutrient concentrations in this study were higher than values that have been shown to stimulate leaf litter decomposition in streams. For instance, Woodward et al. (2012) showed that p values around 30 μg/L and N values around 500 μg/L stimulated the decomposition rates of both oak (Quercus robur) and black alder (Alnus glutinosa) leaves. Most of the studies that have reported stimulation of leaf litter decomposition by nutrient concentrations have been in cases where concentrations of dissolved inorganic N and/or P have been increased above background levels (Rosemond et al., 2002; Gulis and Superkropp, 2003; Richarson et al., 2004; Rosemond et al., 2015). In contrast, when nutrient concentrations are not limited, studies have reported that decomposition rates are not stimulated by nutrient enrichment (Chadwick and Huryn, 2003; Albelho and Graça, 2006; Baldy et al., 2007). In our study, nutrient concentrations were high in both forested and agricultural streams implying that other factors beyond nutrient concentrations potentially influenced the decomposition rates.
A lack of a strong influence of catchment-scale agricultural land use on nutrient concentration and organic matter processing in this study raises the question of the scale at which ecosystem processes in Afrotropical upland-montane streams and most responsive. Considering that agricultural land use in the three catchments ranged from 52 to 73%, it is likely that the proportion of agricultural land use was not high enough to have a significant effect on the streams that would make them differ significantly from forested streams, especially considering that riparian zones in these agricultural streams were fairly maintained. The study area falls within the former white highlands where farm sizes are much larger and the use of fertilizers and pesticides is higher compared to smallholder farming areas in Kenya (Osano et al., 2003; Jindo et al., 2020). This partly explains the higher levels of nutrient concentrations in agricultural streams in this study that are higher than values found in streams draining smallholder farmlands (e.g., Masese et al., 2014b, 2017; Jacobs et al., 2017), but are in the range of values recorded in streams draining catchments dominated by commercial tea plantations (Maghanga et al., 2012; Jacobs et al., 2017). Many studies have addressed the issue of whether land use near streams and rivers is a better predictor of water quality, biotic diversity and ecosystem processes than land use over the entire catchment. (Hunsaker and Levine, 1995; Johnson et al., 1997; Sliva and Williams, 2001). While results have largely been incongruent, there is increasing evidence that reach-scale or riparian zone land use plays an equally important role as catchment scale land use in determining water quality and ecosystem properties. For instance, several studies have found that land cover of the riparian zone is better correlated to total suspended solids (turbidity) and nutrient concentrations in rivers compared to the land cover of the whole catchment (Johnson et al., 1997; Chang, 2008; Uriarte et al., 2011). Similarly, studies have shown that the composition of macroinvertebrate communities is better predicted by factors operating at the reach scale (Minaya et al., 2013; Villeneuve et al., 2018). These findings are significant for stream management and biodiversity conservation as they reinforce the important role of maintaining intact riparian zones and habitat quality in modulating the negative effects of agriculture on water quality, biodiversity, and ecosystem functioning in streams (Hughes and Vadas, 2021).
Contrary to a lack of significant agriculture effects on leaf litter decomposition in our study, there were significant mesh-size and leaf species interactions, implying that the role of shredder- and microbial-mediated breakdown of leaf litter depended on leaf quality/species. Vernonia, which was considered to be of the highest quality and palatability among the three species of leaves, recorded the highest decomposition rate across agricultural and forested sites in both coarse- and fine-mesh litterbags. Syzygium and the non-native Eucalyptus are tougher; both belong to the family Myrtaceae, and have been reported to have higher levels of tannins and secondary compounds, which reduce palatability for shredders (Gonçalves et al., 2006; Reis et al., 2018). In a similar study, decomposition rates in Syzygium cordatum and Eucalyptus globulus leaves were much lower than in Neoboutonia macrocalyx, an indigenous soft-leafed riparian species, in which shredder-mediated breakdown was also higher (Masese, et al., 2014b). In yet another study, the decomposition rate in Neubotonia macrocalyx was 59% faster than in Eucalyptus saligna leaves in forested sites and more than twice as fast (111%) in agricultural sites (Tsisiche et al., 2019).
The relative roles of shredders and microbes in leaf litter decomposition depended on leaf species (litter quality), more than land-use. There were minor relationships between microbially driven leaf litter decomposition rates and litter quality, with reduced microbially driven decomposition rates in Syzygium in agricultural streams and Eucalyptus in forested streams. Leaf litter decomposition rates were similar for Eucalyptus and Syzygium (Figure 5), implying that shredders did not discriminate against the non-native Eucalyptus leaves. However, the lower decomposition rates in Eucalyptus and Syzygium compared to Vernonia can be attributed to the slow conditioning of the tough leaves before they became palatable for shredders (Wantzen et al., 2008; Gessner et al., 2010). The kc/kf coefficients indicated that shredders played an important role in all the leaf species, but their relative roles decreased with leaf quality (Table 4).
The kc/kf coefficients we report in this study are within the range of values reported in tropical streams, but much lower than values reported in the Mediterranean and temperate streams (Table 3). In temperate streams, shredders play a major role in leaf litter decomposition because of their higher abundance and diversity (Dobson et al., 2002; Gonçalves et al., 2006; Gessner et al., 2010; Medina-villar et al., 2015). However, a scarcity of macroinvertebrate shredders has been reported in some tropical studies (Camacho et al., 2009; Yule et al., 2009; Blanco and Gutiérrez-isaza, 2014), although in others a diverse shredder assemblage has been reported (e.g., Cheshire et al., 2005; Masese et al., 2014a; Sitati et al., 2021). The low number and abundance of shredders found in this study (only 7% of macroinvertebrates in coarse-mesh litterbags) demonstrate the potentially dominant role of microbial breakdown of leaf litter in tropical streams (Mathuriau and Chauvet, 2002; Wantzen et al., 2008; Boyero et al., 2015).
Despite the minimal negative effects of agriculture on organic matter processing we report in this study, there is growing evidence of the negative effects of changing land-use from forest to agriculture in other Afrotropical studies (Masese et al., 2014a; Masese et al., 2014b; Fugère et al., 2018). In this study, the differences in leaf litter decomposition rates did not differ between land-uses, and most of the ki/kr values were slightly below 1 (range 0.56–0.97), which implies that agricultural land-use has not severely compromised organic matter processing in the study area. As per the criteria for assessing ecosystem functional integrity by Gessner and Chauvet (2002), breakdown coefficients (ki/kr) within the limits of 0.75–1.33 around the mean of a local reference system reveal no clear evidence of an impact on ecosystem functioning. Agricultural land-use often increases rates of microbial decomposition of leaf litter in streams because of increased water temperature and nutrient concentrations (Young and Matthaei, 2008; Tank et al., 2010). Although our agricultural sites had higher water temperatures than forested sites, the level of nutrient concentrations did not differ from levels in forested streams, which is contrary to what is often recorded (Allan, 2004; Woodward et al., 2012). Interestingly, the potential stimulating effects of breakdown rates by warmer temperature and high nutrient concentrations (Graça et al., 2015) were not recorded in this study. Warmer tropical biomes have been noted to display remarkable heterogeneity in organic matter processing rates than colder high-latitude biomes (Tiegs et al., 2019; Boyero et al., 2021). However, studies that study the combined role of shredders, litter quality, and environmental factors, such as nutrient concentrations, are limited, making it difficult to disentangle the relative importance of these factors.
Management Implications of This Study
Land-use plays a major role in determining stream ecosystem characteristics and function (Fisher et al., 2000; Fugère et al., 2020). Although agriculture was hypothesized to influence organic matter processing through the loss of macroinvertebrate shredders and increased microbial processing through increased nutrient availability and mean water temperature (e.g., Silva-junior et al., 2014; Fugère et al., 2020), the findings of this study are to the contrary. Land-use effects were a minor incursion to differences in decomposition rates, which were more dependent on litter quality (leaf species) and inclusion of macroinvertebrate shredders in the decomposition process. Although there are minor differences in the species diversity and composition of riparian vegetation between forested and agricultural streams in the study area, riparian vegetation along forested and agricultural streams are mainly indigenous, but agricultural streams have higher densities of Syzygium spp., while forested streams have a more diverse and species richness of vegetation.
Understanding how stream ecosystems respond to land-use changes is critical for stream management and conservation. Forest cover in the Afrotropical catchments has been declining over the years with potential effects for stream ecosystems (Fugère et al., 2016, 2018). Studies have reported negative effects of land-use changes from forestry to agriculture and urbanization in tropical streams, ranging from declines in water quality and quantity, loss of biodiversity, mainly the sensitive shredder taxa, and changes in ecosystem structure and functioning (Masese et al., 2014a, b; Fugère et al., 2016, 2018; Masese et al., 2017; Yegon et al., 2021). However, the findings of this study show that agriculture does not necessarily have negative effects on streams if riparian zones and habitat quality are well protected. Given observed minimal changes in stream water physico-chemistry, habitat quality, diversity, and distribution of macroinvertebrates, and organic matter processing between forested and agricultural streams, conservation and management efforts need to clearly understand how land-use is impacting tropical stream ecosystems so that management measures can be put in place. Many countries in the region, including Kenya (Government of Kenya, 2012; Matunda et al., 2015), have regulations in place for the protection of the riparian zone along streams and rivers. These regulations should be enforced as there are demonstrated benefits arising from the maintenance of riparian zones ads evidenced in this and similar studies (e.g., (Masese et al., 2012; Njue et al., 2016).
Conclusion
This study reports the interactions between environmental variables, leaf quality and the role of shredders vs microbiota in the processing of organic matter in streams. The hypothesized effects of land-use on leaf litter decomposition, potentially mediated by warmer water temperature, elevated nutrient concentration and limited shredders in agricultural streams, were not supported in this study. Instead, there were limited differences in water and habitat quality, nutrient concentrations, and organic matter standing stocks between forested and agricultural sites which favored the widespread distribution of shredders in agricultural sites although in low abundances. Responses in the role of both shredders and microbes in the decomposition of leaf litter to land-use were minimal, and the potential negative effects of agriculture that have been reported elsewhere seemed muted and were potentially modulated by the maintenance of intact riparian zones and habitat quality in agricultural streams. Although the replicate number of sites (three) we used in this study for agricultural and forested streams was low, the effects of agriculture on macroinvertebrates and litter decomposition were markedly low compared to similar studies in the region. Future studies are needed to evaluate aspects of the riparian zones, such as riparian width and length, that are playing a role in minimizing the negative effects of agriculture on streams and rivers.
Data Availability Statement
The raw data supporting the conclusion of this article will be made available by the authors, without undue reservation.
Author Contributions
EK, FM, DM, and EC: conceptualization, experimental design; EK, FM, BK, and AS: data collection and data analysis; EK, FM, and AS: drafting of the manuscript; All authors commented on the manuscript and approved it for publication.
Funding
This work was supported by the Intra-Africa Academic Mobility Scheme (COTRA-project) (grant 2017/2929) funded by the European Union through a fellowship to EK. Additional support came from the NRF Kenya (grant FY 2017/2018) funded project “development of a biomonitoring protocol for Kenyan streams and rivers”.
Conflict of Interest
The authors declare that the research was conducted in the absence of any commercial or financial relationships that could be construed as a potential conflict of interest.
The handling Editor declared a past co-authorship with one of the authors FM, AS.
Publisher’s Note
All claims expressed in this article are solely those of the authors and do not necessarily represent those of their affiliated organizations, or those of the publisher, the editors and the reviewers. Any product that may be evaluated in this article, or claim that may be made by its manufacturer, is not guaranteed or endorsed by the publisher.
Acknowledgments
We appreciate the assistance by Henry Lubanga and Brian Keya, University of Eldoret, during field sampling and sample analysis in the laboratory. We are grateful to Alfred Achieng, University of Eldoret, for generation of the maps of the study area.
Supplementary Material
The Supplementary Material for this article can be found online at: https://www.frontiersin.org/articles/10.3389/fenvs.2021.794525/full#supplementary-material
References
Albelho, M., and Graça, M. A. S. (2006). Effects of Nutrient Enrichment on Decomposition and Fungal Colonization of Sweet Chestnut Leaves in an Iberian Stream (Central Portugal). Hydrobiologia 560, 239–247. doi:10.1007/s10750-005-9414-2
Allan, J. D. (2004). Landscapes and Riverscapes: The Influence of Land Use on Stream Ecosystems. Annu. Rev. Ecol. Evol. Syst. 35, 257–284. doi:10.1146/annurev.ecolsys.35.120202.110122
APHA (American Public Health Association) (2005). Standard Methods for the Examination of Water and Wastewater. 21st Edn. USA: APHA, American Water Works Association, Water Pollution Control Federation.
Baldy, V., Gobert, V., Guerold, F., Chauvet, E., Lambrigot, D., and Charcosset, J.-Y. (2007). Leaf Litter Breakdown Budgets in Streams of Various Trophic Status: Effects of Dissolved Inorganic Nutrients on Microorganisms and Invertebrates. Freshw. Biol 52, 1322–1335. doi:10.1111/j.1365-2427.2007.01768.x
Barbour, M. T. (1999). Rapid Bioassessment Protocols for Use in Wadeable Streams and Rivers: Periphyton, Benthic Macroinvertebrates and Fish. Office of Water: US Environmental Protection Agency.
Benfield, E. F. (1996). “Leaf Breakdown in Stream Ecosystems, ” Methods in Stream Ecology. Editors F. R. Hauer, and G. A. Lamberti (Academic Press), 579–589.
Boyero, L., López-Rojo, N., Tonin, A. M., Pérez, J., Correa-Araneda, F., Pearson, R. G., et al. (2021). Impacts of Detritivore Diversity Loss on Instream Decomposition Are Greatest in the Tropics. Nat. Commun. 12, 3700. doi:10.1038/s41467-021-23930-2
Boyero, L., Graça, M. A. S., Tonin, A. M., Pérez, J., J. Swafford, A., Ferreira, V., et al. (2017). Riparian Plant Litter Quality Increases with Latitude. Sci. Rep. 7, 10562. doi:10.1038/s41598-017-10640-3
Boyero, L., Pearson, R. G., Dudgeon, D., Graça, M. A. S., Gessner, M. O., Albariño, R. J., et al. (2011). Global Distribution of a Key Trophic Guild Contrasts with Common Latitudinal Diversity Patterns. Ecology 92 (9), 1839–1848. doi:10.1890/10-2244.1
Boyero, L., Pearson, R. G., Gessner, M. O., Dudgeon, D., Ramírez, A., Yule, C. M., et al. (2015). Leaf-litter Breakdown in Tropical Streams: Is Variability the Norm? Freshw. Sci. 34 (2), 759–769. doi:10.1086/681093
Boyero, L., Pearson, R. G., Hui, C., Gessner, M. O., Pérez, J., Alexandrou, M. A., et al. (2016). Biotic and Abiotic Variables Influencing Plant Litter Breakdown in Streams: a Global Study. Proc. R. Soc. B. 283, 20152664. doi:10.1098/rspb.2015.2664
Camacho, R., Boyero, L., Cornojo, A., Ibanez, A., and Pearson, R. G. (2009). Local Variation in Shredder Distribution Can Explain Their Oversight in Tropical Streams. Biotrop 11 (11), 1–8. doi:10.1111/j.1744-7429.2009.00519.x
Chadwick, M. A., and Huryn, A. D. (2003). Effect of a Whole-Catchment N Addition on Stream Detritus Processing. J. North Am. Benthological Soc. 22, 194–206. doi:10.2307/1467992
Chang, H. (2008). Spatial Analysis of Water Quality Trends in the Han River basin, South Korea. Water Res. 42, 3285–3304. doi:10.1016/j.watres.2008.04.006
Cheshire, K., Boyero, L., and Pearson, R. G. (2005). Food Webs in Tropical Australian Streams: Shredders Are Not Scarce. Freshw. Biol 50 (5), 748–769. doi:10.1111/j.1365-2427.2005.01355.x
Congedo, L. (2020b). Semi-Automatic Classification Plug-In Document. Available online at: https://www.researchgate.net/publication/344876862_Semi-Automatic_Classification_Plugin_Documentation_Release_7001_Luca_Congedo.
Cooper, S. D., Lake, P. S., Sabater, S., Melack, J. M., and Sabo, J. L. (2013). The Effects of Land Use Changes on Streams and Rivers in Mediterranean Climates. Hydrobiologia 719, 383–425. doi:10.1007/s10750-012-1333-4
Cornejo, A., Pérez, J., López-Rojo, N., Tonin, A. M., Rovira, D., Checa, B., et al. (2020). Agriculture Impairs Stream Ecosystem Functioning in a Tropical Catchment. Sci. Total Environ. 745, 140950. doi:10.1016/j.scitotenv.2020.140950
Danger, M., Cornut, J., Elger, A., and Chauvet, E. (2012). Effects of Burial on Leaf Litter Quality, Microbial Conditioning and Palatability to Three Shredder Taxa. Freshw. Bio. 57 (5), 1017–1030. doi:10.1111/j.1365-2427.2012.02762.x
Day, J. A., and de Moor, I. J. (2002a). Volume 5: Non-arthropods (The Protozoans, Porifera, Cnidaria, Platyhelminthes, Nemertea, Rotifera, Nematoda, Nematomorpha, Gastrotrichia, Bryozoa, Tardigrada, Polychaeta, Oligochaeta and Hirudinea). WRC Report No.TT 167/02. Pretoria, South Africa: Water Research Commission.Guides to the Freshwater Invertebrates of Southern Africa
Day, J. A., and de Moor, I. J. (2002b). Guides to the Freshwater Invertebrates of Southern Africa. in Volume 6: Arachnida and Mollusca (Araneae, Water Mites and Mollusca). WRC Report No.TT 182/02. Pretoria, South Africa: Water Research Commission.
de Moor, I. J., Day, J. A., and de Moor, F. C. (2003a). Volume 7: Insecta I: Ephemeroptera, Odonata and Plecoptera. WRC Report No. TT 207/03. Pretoria, South Africa: Water Research Commission.Guides to the Freshwater Invertebrates of Southern Africa
de Moor, I. J., Day, J. A., and de Moor, F. C. (2003b). Volume 8: Insecta II. Report No. TT 214/03. Pretoria, South Africa: Water Research Commission.Guides to the Freshwater Invertebrates of Southern Africa
Dobson, M., Magana, A., Mathooko, J. M., and Ndegwa, F. K. (2002). Detritivores in Kenyan highland Streams: More Evidence for the Paucity of Shredders in the Tropics? Freshw. Bio. 47, 909–919. doi:10.1046/j.1365-2427.2002.00818.x
Ferreira, V., Chauvet, E., and Canhoto, C. (2015). Effects of Experimental Warming, Litter Species, and Presence of Macroinvertebrates on Litter Decomposition and Associated Decomposers in a Temperate Mountain Stream. Can. J. Fish. Aquat. Sci. 72 (2), 206–216. doi:10.1139/cjfas-2014-0119
Ferreira, V., Encalada, A. C., and Graça, M. A. S. (2012). Effects of Litter Diversity on Decomposition and Biological Colonization of Submerged Litter in Temperate and Tropical Streams. Freshw. Sci. 31 (3), 945–962. doi:10.1899/11-062.1
Ferreira, V., Gulis, V., and Graça, M. A. S. (2006). Whole-stream Nitrate Addition Affects Litter Decomposition and Associated Fungi but Not Invertebrates. Oecologia 149 (4), 718–729. doi:10.1007/s00442-006-0478-0
Fisher, D. S., Steiner, J. L., Endale, D. M., Stuedemann, J. A., Schomberg, H. H., Franzluebbers, A. J., et al. (2000). The Relationship of Land Use Practices to Surface Water Quality in the Upper Oconee Watershed of Georgia. For. Ecol. Manag. 128, 39–48. doi:10.1016/S0378-1127(99)00270-4
Fugère, V., Jacobsen, D., Finestone, E. H., and Chapman, L. J. (2018). Ecosystem Structure and Function of Afrotropical Streams with Contrasting Land Use. Freshw. Biol. 63 (12), 1498–1513. doi:10.1111/fwb.13178
Fugère, V., Kasangaki, A., and Chapman, L. J. (2016). Land Use Changes in an Afrotropical Biodiversity Hotspot Affect Stream Alpha and Beta Diversity. Ecosphere 7 (6). doi:10.1002/ecs2.1355
Fugère, V., Lostchuck, E., and Chapman, L. J. (2020). Litter Decomposition in Afrotropical Streams: Effects of Land Use, home-field Advantage, and Terrestrial Herbivory. Freshw. Sci. 39 (3), 497–507. doi:10.1086/709807
Gessner, M. O., and Chauvet, E. (2002). A Case for Using Litter Breakdown to Assess Functional Stream Integrity. Ecol. Appl. 12 (2), 498–510. doi:10.1890/1051-0761(2002)012[0498:acfulb]2.0.co;2
Gessner, M. O., Swan, C. M., Dang, C. K., McKie, B. G., Bardgett, R. D., Wall, D. H., et al. (2010). Diversity Meets Decomposition. Trends Ecol. Evol. 25 (6), 372–380. doi:10.1016/j.tree.2010.01.010
Gonçalves, J. F., Graça, M. A., and Callisto, M. (2006). Leaf-litter Breakdown in 3 Streams in Temperate, Mediterranean, and Tropical Cerrado Climates. J. N. Am. Benthol. Soc. 25 (2), 344–355. doi:10.1899/0887-3593(2006)25[344:LBISIT]2.0.CO;2
Government of Kenya (2012). Water Act, Laws of Kenya. Chapter 372. 2nd ed. Nairobi, Kenya: National Council for Law Reporting with the Authority of the Attorney‐General, 1–245.
Graça, M. A. S., Ferreira, V., Canhoto, C., Encalada, A. C., Guerrero-Bolaño, F., Wantzen, K. M., et al. (2015). A Conceptual Model of Litter Breakdown in Low Order Streams. Internat. Rev. Hydrobiol. 100, 1–12. doi:10.1002/iroh.201401757
Gulis, V., Ferreira, V., and Graca, M. A. S. (2006). Stimulation of Leaf Litter Decomposition and Associated Fungi and Invertebrates by Moderate Eutrophication: Implications for Stream Assessment. Freshw. Biol 51 (9), 1655–1669. doi:10.1111/j.1365-2427.2006.01615.x
Gulis, V., and Suberkropp, K. (2003). Leaf Litter Decomposition and Microbial Activity in Nutrient-Enriched and Unaltered Reaches of a Headwater Stream. Freshw. Biol. 48, 123–134. doi:10.1046/j.1365-2427.2003.00985.x
Gutiérrez Isaza, N., and Blanco, J. F. (2014). Leaf Litter Breakdown Rates and Associated Fauna of Native and Exotic Trees Used in Neotropical Riparia Reforestation. Acta Biol. Colomb. 19 (1), 91–100. doi:10.15446/abc.v19n1.38155
Hammer, Ø., Harper, D. A. T., and Ryan, P. D. (2001). PAST: Paleontological Statistics Software Package for Education and Data Analysis. Palaeontol. Electronica 4, 1. Avaiable at: http://palaeo-electronica.org/2001_1/past/issue1_01.htm.
Hepp, L. U., Urbim, F. M., Tonello, G., Loureiro, R. C., Sausen, T. L., Fornel, R., et al. (2016). Influence of Land-Use on Structural and Functional Macroinvertebrate Composition Communities Associated on Detritus in Subtropical Atlantic Forest Streams. Acta Limnol. Bras. 28, 1–9. doi:10.1590/S2179-975X0616
Hughes, R. M., and Vadas, R. L. (2021). Agricultural Effects on Streams and Rivers: A Western USA Focus. Water 13 (14), 1901. doi:10.3390/w13141901
Hunsaker, C. T., and Levine, D. A. (1995). Hierarchical Approaches to the Study of Water Quality in Rivers. BioScience 45 (3), 193–203. doi:10.2307/1312558
Irons, J. G., Oswood, M. W., Stout, R. J., and Pringle, C. M. (1994). Latitudinal Patterns in Leaf Litter Breakdown: Is Temperature Really Important? Freshw. Biol 32, 401–411. doi:10.1111/j.1365-2427.1994.tb01135.x
Jacobs, S. R., Breuer, L., Butterbach-Bahl, K., Pelster, D. E., and Rufino, M. C. (2017). Land Use Affects Total Dissolved Nitrogen and Nitrate Concentrations in Tropical Montane Streams in Kenya. Sci. Total Environ. 603-604, 519–532. doi:10.1016/j.scitotenv.2017.06.100
Jindo, K., Schut, A. G. T., and Langeveld, J. W. A. (2020). Sustainable Intensification in Western Kenya: Who Will Benefit? Agric. Syst. 182, 102831. doi:10.1016/j.agsy.2020.102831
Jinggut, T., Yule, C. M., and Boyero, L. (2012). Stream Ecosystem Integrity Is Impaired by Logging and Shifting Agriculture in a Global Megadiversity center (Sarawak, Borneo). Sci. Total. Environ. 437, 83–90. doi:10.1016/j.scitotenv.2012.07.062
Johnson, L., Richards, C., Host, G., and Arthur, J. (1997). Landscape Influences on Water Chemistry in Midwestern Stream Ecosystems. Freshw. Biol. 37, 193–208. doi:10.1046/j.1365-2427.1997.d01-539.x
Jun, Y.-C., Kim, N.-Y., Kwon, S.-J., Han, S.-C., Hwang, I.-C., Park, J.-H., et al. (2011). Effects of Land Use on Benthic Macroinvertebrate Communities: Comparison of Two Mountain Streams in Korea. Ann. Limnol. - Int. J. Lim. 47, S35–S49. doi:10.1051/limn/2011018
Lecerf, A., Usseglio-Polatera, P., Charcosset, J.-Y. L., Bracht, B., Chauvet, E., and Chauvet, E. (2006). Assessment of Functional Integrity of Eutrophic Streams Using Litter Breakdown and Benthic Macroinvertebrates. archiv_hydrobiologie 165 (1), 105–126. doi:10.1127/0003-9136/2006/0165-0105
Li, A. O. Y., Ng, L. C. Y., and Dudgeon, D. (2009). Effects of Leaf Toughness and Nitrogen Content on Litter Breakdown and Macroinvertebrates in a Tropical Stream. Aquat. Sci. 71, 80–93. doi:10.1127/0003-9136/2006/0165-0105
MacKenzie, R. A., Wiegner, T. N., Kinslow, F., Cormier, N., and Strauch, A. M. (2013). Leaf-litter Inputs from an Invasive Nitrogen-Fixing Tree Influence Organic-Matter Dynamics and Nitrogen Inputs in a Hawaiian River. Freshw. Sci. 32, 1036–1052. doi:10.1899/12-152.110.1899/12-152.1
Maghanga, J. K., Kituyi, J. L., Kisinyo, P. O., and Ng’etich, W. K. (2013). Impact of Nitrogen Fertilizer Applications on Surface Water Nitrate Levels within a Kenyan tea Plantation. J. Chem. 2013 (4), 1. doi:10.1155/2013/196516
Martínez, A., Larrañaga, A., Pérez, J., Basaguren, A., and Pozo, J. (2013). Leaf-litter Quality Effects on Stream Ecosystem Functioning: a Comparison Among Five Species. fal 183 (3), 239–248. doi:10.1127/1863-9135/2013/0514
Masese, F. O., Achieng, A. O., O’Brien, G. C., and McClain, M. E. (2021). Macroinvertebrate Taxa Display Increased Fidelity to Preferred Biotopes Among Disturbed Sites in a Hydrologically Variable Tropical River. Hydrobiologia 848 (2), 321–343. doi:10.1007/s10750-020-04437-1
Masese, F. O., Kitaka, N., Kipkemboi, J., Gettel, G. M., Irvine, K., and Mcclain, M. E. (2014b). Litter Processing and Shredder Distribution as Indicators of Riparian and Catchment Influences on Ecological Health of Tropical Streams. Ecol. Indicators 46, 23–37. doi:10.1016/j.ecolind.2014.05.032
Masese, F. O., Kitaka, N., Kipkemboi, J., Gettel, G. M., Irvine, K., and McClain, M. E. (2014a). Macroinvertebrate Functional Feeding Groups in Kenyan highland Streams: Evidence for a Diverse Shredder Guild. Freshw. Sci. 33 (2), 435–450. doi:10.1086/675681
Masese, F. O., Raburu, P. O., Mwasi, B. N., and Etiégni, L. (2012). Effects of Deforestation on Water Resources: Integrating Science and Community Perspectives in the Sondu‐Miriu River Basin Kenya. New Adv. Contrib. Res. InTechnology, 3–18.
Masese, F. O., Salcedo-Borda, J. S., Gettel, G. M., Irvine, K., and McClain, M. E. (2017). Influence of Catchment Land Use and Seasonality on Dissolved Organic Matter Composition and Ecosystem Metabolism in Headwater Streams of a Kenyan River. Biogeochemistry 132 (1-2), 1–22. doi:10.1007/s10533-016-0269-6
Masese, F., Raburu, P., and Muchiri, M. (2009). A Preliminary Benthic Macroinvertebrate index of Biotic Integrity (B-IBI) for Monitoring the Moiben River, Lake Victoria Basin, Kenya. Afr. J. Aquat. Sci. 34 (1), 1–14. doi:10.2989/AJAS.2009.34.1.1.726
Mathooko, J. M., Morara, G. O., and Leichtfried, M. (2001). Leaf Litter Transport and Retention in a Tropical Rift Valley Stream: an Experimental Approach. Hydrobiologia 443 (1-3), 9–18. doi:10.1023/A:1017542617696
Mathuriau, C., and Chauvet, E. (2002). Breakdown of Leaf Litter in a Neotropical Stream. J. North Am. Benthological Soc. 21 (3), 384–396. doi:10.2307/1468477
Matthaei, C. D., Piggott, J. J., and Townsend, C. R. (2010). Multiple Stressors in Agricultural Streams: Interactions Among Sediment Addition, Nutrient Enrichment and Water Abstraction. J. Appl. Ecol. 47 (3), 639–649. doi:10.1111/j.1365-2664.2010.01809.x
Matunda, J. M. (2015). “Sustainable Management of Riparian Areas in Kenya:a Critique of the Inadequacy of the Legislative Framework Governing the protection of Sustainable Management of Riparian Zones in Kenya,”. MSc Thesis (Kenya: University of Nairobi).
Medina-villar, S., Alonso, Á., Aldana, B. R. V., Pérez-corona, E., and Castro-díez, P. (2015). Decomposition and Biological Colonization of Native and Exotic Leaf Litter in a Central Spain Stream. Limnet 34 (2), 293–310.
Merritt, R. W., Cummins, K. W., and Berg, M. B. (2008). An Introduction to the Aquatic Insects of North America. 4th (Edition. USA: Kendall Hunt Publishing. Dubuque, Iowa.
Merritt, R. W., Cummins, K. W., and Berg, M. B. (2017). Trophic Relationships of Macroinvertebrates. Met. Str. Ecol. 1, 413–433. doi:10.1016/B978-0-12-416558-8.00020-2
Minaya, V., McClain, M. E., Moog, O., Omengo, F., and Singer, G. A. (2013). Scale-dependent Effects of Rural Activities on Benthic Macroinvertebrates and Physico-Chemical Characteristics in Headwater Streams of the Mara River, Kenya. Ecol. Indicators 32, 116–122. doi:10.1016/j.ecolind.2013.03.011
Moses, W., Nyongesa, H., Konje, M., and Agevi, H. M. T. (2015). Effects of Land Use Practices on Soil Organic Carbon, Nitrogen and Phosphorus in River Nzoia Drainage basin, Kenya. Aff 4 (4), 153–158. doi:10.11648/j.aff.20150404.11
Mustonen, K.-R., Mykrä, H., Louhi, P., Markkola, A., Tolkkinen, M., Huusko, A., et al. (2016). Sediments and Flow Have Mainly Independent Effects on Multitrophic Stream Communities and Ecosystem Functions. Ecol. Appl. 26 (7), 2116–2129. doi:10.1890/15-1841.1
Njue, N., Koech, E., Hitimana, J., and Sirmah, P. (2016). Influence of Land Use Activities on Riparian Vegetation, Soil and Water Quality: An Indicator of Biodiversity Loss, South West Mau Forest, Kenya. Ojf 06, 373–385. doi:10.4236/ojf.2016.65030
Oksanen, J., Blanchet, F. G., Friendly, M., Kindt, R., Legendre, P., McGlinn, D., ., et al. (2020). Vegan: Community Ecology Package, R package version 2.5-5. 2019.
Ontumbi, G., Obando, J., and Ondieki, C. (2015). The Influence of Agricultural Activities on the Water Quality of the River Sosiani in Uasin Gishu County, Kenya. Int. J. Res. Agric. Sci. 2 (1), 2348–3997.
Osano, O., Nzyuko, D., Tole, M., and Admiraal, W. (2003). The Fate of Chloroacetanilide Herbicides and Their Degradation Products in the Nzoia Basin, Kenya. AMBIO: A J. Hum. Environ. 32 (6), 424–427. doi:10.1579/0044-7447-32.6.424
Raburu, P. O., and Masese, F. O. (2012). Development of a Fish-Based index of Biotic Integrity (FIBI) for Monitoring Riverine Ecosystems in the Lake Victoria Drainage Basin, Kenya. River Res. Applic. 28 (1), 23–38. doi:10.1002/rra.1428
Reinhart, K. O., and VandeVoort, R. (2006). Effect of Native and Exotic Leaf Litter on Macroinvertebrate Communities and Decomposition in a Western Montana Stream. Divers. Distrib. 12 (6), 776–781. doi:10.1111/j.1472-4642.2006.00252.x
Reis, D. F., Machado, M. M. D., Coutinho, N. P., Rangel, J. V., Moretti, M. S., and Morais, P. B. (2018). Feeding Preference of the Shredder Phylloicus Sp. For Plant Leaves of Chrysophyllum Oliviforme or Miconia Chartacea after Conditioning in Streams from Different Biomes. Braz. J. Biol. 79 (1), 22–28. doi:10.1590/1519-6984.170644
Richarson, J. S., Shaughnessy, C. R., and Harrison, P. G. (2004). Litter Breakdown and Invertebrate Association with Three Types of Leaves in a Temperate Rainforest Stream. Archiv fur Hydrobiologie 159, 309–325. doi:10.1127/0003-9136/2004/0159-0309
Rosemond, A. D., Benstead, J. P., Bumpers, P. M., Gulis, V., Kominoski, J. S., Manning, D. W. P., et al. (2015). Experimental Nutrient Additions Accelerate Terrestrial Carbon Loss from Stream Ecosystems. Science 347, 1142–1145. doi:10.1126/science.aaa1958
Rosemond, A. D., Pringle, C. M., Ramírez, A., Paul, M. J., and Meyer, J. L. (2002). Landscape Variation in Phosphorus Concentration and Effects on Detritus-Based Tropical Streams. Limnol. Oceanogr. 47, 278–289. doi:10.4319/lo.2002.47.1.0278
Silva-junior, E. F., Moulton, T. P., Boëchat, I. G., and Gücker, B. (2014). Leaf Decomposition and Ecosystem Metabolism as Functional Indicators of Land Use Impacts on Tropical Streams. Ecol. Indicators 36, 195–204. doi:10.1016/j.ecolind.2013.07.027
Sitati, A., Raburu, P. O., Yegon, M. J., and Masese, F. O. (2021). Land-use Influence on the Functional Organization of Afrotropical Macroinvertebrate Assemblages. Limnologica 88, 125875. doi:10.1016/j.limno.2021.125875
Sliva, L., and Dudley Williams, D. (2001). Buffer Zone versus Whole Catchment Approaches to Studying Land Use Impact on River Water Quality. Water Res. 35 (14), 3462–3472. doi:10.1016/S0043-1354(01)00062-8
Stenfert Kroese, J., Jacobs, S. R., Tych, W., Breuer, L., Quinton, J. N., and Rufino, M. C. (2020). Tropical Montane forest Conversion Is a Critical Driver for Sediment Supply in East African Catchments. Water Resour. Res. 56, e2020WR027495. doi:10.1029/2020WR027495
Tank, J. L., Rosi-marshall, E. J., Griffiths, N. A., Entrekin, S. A., and Stephen, M. L. (2010). A Review of Allochthonous Organic Matter Dynamics and Metabolism in Streams. J. North Am. Benthological Soc. 29 (1), 118–146. doi:10.1899/08-170.1
Team, R. C (2017). R: A Language and Environment for Statistical Computing. Vienna, Austria: R Foundation for Statistical Computing. Computer software, Version 3.4. 2.
Tiegs, S. D., Costello, D. M., Isken, M. W., Woodward, G., McIntyre, P. B., Gessner, M. O., et al. (2019). Global Patterns and Drivers of Ecosystem Functioning in Rivers and Riparian Zones. Sci. Adv. 5 (1), eaav0486. doi:10.1126/sciadv.aav0486
Tsisiche, A., M'merimba, C. M., and Mbaka, J. G. (2019). Effect of Land-Use on Leaf Litter Decomposition in Upper Mara Streams, Kenya. Eg. J. Sci. Tech. 16, 1–139.
Uriarte, M., Yackulic, C. B., Lim, Y., and Arce-Nazario, J. A. (2011). Influence of Land Use on Water Quality in a Tropical Landscape: a Multi-Scale Analysis. Landscape Ecol. 26, 1151–1164. doi:10.1007/s10980-011-9642-y
Villeneuve, B., Piffady, J., Valette, L., Souchon, Y., and Usseglio-Polatera, P. (2018). Direct and Indirect Effects of Multiple Stressors on Stream Invertebrates across Watershed, Reach and Site Scales: A Structural Equation Modelling Better Informing on Hydromorphological Impacts. Sci. Total Environ. 612, 660–671. doi:10.1016/j.scitotenv.2017.08.197ff
Wantzen, K., and Mol, J. (2013). Soil Erosion from Agriculture and Mining: a Threat to Tropical Stream Ecosystems. Agriculture 3 (4), 660–683. doi:10.3390/agriculture3040660
Wantzen, K. M., and Wagner, R. (2006). Detritus Processing by Invertebrate Shredders: a Neotropical-Temperate Comparison. J. North Am. Benthological Soc. 25, 216–232. doi:10.1899/0887-3593(2006)25[216:dpbisa]2.0.co;2
Wantzen, K. M., Yule, C. M., Mathooko, J. M., and Pringle, C. M. (2008). Organic Matter Processing in Tropical Streams. Trop. Str. Ecol. 3, 43–64. doi:10.1016/B978-012088449-0.50005-4
Webster, J. R., and Benfield, E. F. (1986). Vascular Plant Breakdown in Freshwater Ecosystems. Annu. Rev. Ecol. Syst. 17, 567–594. doi:10.1146/annurev.es.17.110186.003031
Wetzel, R. G., and Likens, G. E. (2000). Limnological Analysis. 3rd edition. New York: Springer-Verlag. doi:10.1007/978-1-4757-3250-4
Woodward, G., Gessner, M. O., Giller, P. S., Gulis, V., Hladyz, S., Lecerf, A., et al. (2012). Continental-scale Effects of Nutrient Pollution on Stream Ecosystem Functioning. Science 336 (6087), 1438–1440. doi:10.1126/science.1219534
Young, R. G., Matthaei, C. D., and Townsend, C. R. (2008). Organic Matter Breakdown and Ecosystem Metabolism: Functional Indicators for Assessing River Ecosystem Health. J. North Am. Benthological Soc. 27, 605–625. doi:10.1899/07-121.1
Keywords: agricultural effects, non-native species, land-use, litter decomposition, macroinvertebrates, water quality
Citation: Kadeka EC, Masese FO, Lusega DM, Sitati A, Kondowe BN and Chirwa ER (2021) No Difference in Instream Decomposition Among Upland Agricultural and Forested Streams in Kenya. Front. Environ. Sci. 9:794525. doi: 10.3389/fenvs.2021.794525
Received: 13 October 2021; Accepted: 22 November 2021;
Published: 08 December 2021.
Edited by:
José Francisco Gonçalves Júnior, University of Brasilia, BrazilReviewed by:
Luiz Ubiratan Hepp, Federal University of Mato Grosso do Sul, BrazilArturo Elosegi, University of the Basque Country, Spain
Copyright © 2021 Kadeka, Masese, Lusega, Sitati, Kondowe and Chirwa. This is an open-access article distributed under the terms of the Creative Commons Attribution License (CC BY). The use, distribution or reproduction in other forums is permitted, provided the original author(s) and the copyright owner(s) are credited and that the original publication in this journal is cited, in accordance with accepted academic practice. No use, distribution or reproduction is permitted which does not comply with these terms.
*Correspondence: Frank O. Masese, Zi5tYXNlc2VAZ21haWwuY29t, Zm1hc2VzZUB1b2VsZC5hYy5rZQ==