- 1Ecosystem Research Group, Institute of Geography, Faculty of Mathematics and Natural Sciences, University of Cologne, Cologne, Germany
- 2German Federal Institute of Hydrology, Koblenz, Germany
Accumulation of microplastics in aquatic environments is an issue of emerging concern. Initially, research focused on marine systems. However, recent studies also investigate the abundance of microplastics in freshwater environments. Rivers connect terrestrial with marine ecosystems and contribute a considerable share of macro- and microplastics to the oceans. A previous study found a large amount of micro-spheres in Dessau downstream the river mouth of the Mulde. Therefore, the objective of this research was to examine whether the Mulde river with its highly industrialized catchment contributes to the microplastic pollution of the Elbe. Sediment (Van Veen grab sampler) and water samples (filter cascade with the smallest mesh size 50 μm and nets with the smallest mesh size 150 μm) were taken from the Elbe river up- and downstream the confluence with the Mulde. After extensive sample preparation, we examined the samples under a digital microscope and determined polymer types by pyrolysis Gas Chromatography/Mass Spectrometry (pyr-GC-MS). The amount of primary microplastics increased in sediment and water samples just downstream the confluence. Those microplastics originate probably from the Mulde. We measured larger amounts and different shapes of microplastics in filter cascades that have a smaller mesh size compared to the nets.
1 Introduction
Plastic became a mass product in the second half of the 20th century and has changed almost all areas of our everyday life since then. Littering and uncontrolled disposal of discarded plastic products threaten the environment because plastic is resistant and poorly biodegradable (Thompson et al., 2004; Geyer et al., 2017). Studies on macroplastics and plastic waste are common in the scientific literature. In contrast, microplastics, usually defined as plastic particles smaller than 5 mm (Arthur et al., 2009), has only become a major research topic since the early 2000s (Thompson et al., 2004). Microplastics is an umbrella term and encompasses different categories, e.g., polymer types, shapes (amorphous, fibres, spheres, films, and foams) and origins (primary and secondary microplastic). While primary microplastics are intentionally produced, for example as spheres for industrial purposes, secondary microplastics originate from fragmentation of macroplastic objects by exposure to light, heat, mechanical friction or organisms (Geyer et al., 2017; Kataoka et al., 2019; Meides et al., 2021; Petersen and Hubbart, 2021). So far, researchers studied mostly marine environments, while the contamination of freshwater and terrestrial systems gained far less attention (Dris et al., 2015; Wagner and Lambert, 2018; Scherer et al., 2020). Additionally, the research on microplastics has focused mainly on their abundance and distribution in the environment, while their transport and pathways have remained rather understudied (Horton et al., 2017; Hurley and Nizzetto, 2018; Rochman, 2018; Rillig and Lehmann, 2020). Consequently, we know much less about the sources, transport ways and sinks of microplastics in fluvial environments than in the oceans (Dris et al., 2015; Klein et al., 2015; Wagner and Lambert, 2018).
Rivers transport microplastics to the oceans (Lebreton et al., 2017; Rochman, 2018; Weber and Opp, 2020) and an estimated 80% of marine plastic debris originates from inland sources (Meijer et al., 2021). Several studies have identified urban regions and most notably industrial areas as major sources of microplastic pollution in rivers (Mani et al., 2016; Schmidt et al., 2018; Tibbetts et al., 2018) and therefore as a threat to fluvial and marine environments (Eerkes-Medrano et al., 2015; Blair et al., 2017; Petersen and Hubbart, 2021). The sources of microplastics are manifold, and so are their pathways to rivers. Indeed, microplastics can be transported by surface run-off from agricultural areas, aerial emission from industries or application of sewage sludge, and be released from discharge of waste water treatment plants (WWTP) (Horton and Dixon, 2018; Wagner and Lambert, 2018; Sun et al., 2019; Brandes et al., 2021). Although WWTPs filter more than 90% of microplastics, they still contribute a certain share of microplastic particles to fluvial systems (Murphy et al., 2016; Horton et al., 2017; Kay et al., 2018; Schmidt et al., 2020; Haberstroh et al., 2021; Schell et al., 2021).
Rivers are not only transport systems of microplastics to the oceans, they can also function as sinks themselves (Horton and Dixon, 2018; Frei et al., 2019; Scherer et al., 2020; Waldschläger and Schüttrumpf, 2020). Depending on their density and shape, microplastics tend to float on or close to the water surface or, if denser, can sink (Waldschläger and Schüttrumpf, 2019a). Erosion during flooding events, for example, may re-mobilise the particles (Hurley et al., 2018; Lechthaler et al., 2021). In particular, due to their low density, microplastic particles are re-mobilised more easily than natural sediments (Waldschläger and Schüttrumpf, 2019a). The residence time of microplastic particles in river water affects biofouling, which forms biofilms and changes the particles’ surface characteristics and their density (Horton et al., 2017; Horton and Dixon, 2018).
Consequently, the occurrence and distribution of microplastics in fluvial systems is rather complex and depends on the distribution of sources and pollutants (Kay et al., 2018; Haberstroh et al., 2021) as well as on fluvial dynamics, such as seasonality (Mani and Burkhardt-Holm, 2020) and flood events (Hurley et al., 2018). In this context, recent studies suggest that smaller and medium-sized rivers are of crucial importance for the microplastic distribution in the whole catchment. They point out that the abundance of microplastics in smaller rivers might be far higher than in larger rivers due to the proximity of point sources (Heß et al., 2018; Constant et al., 2020). Therefore, tributaries are more and more seen as relevant contributors of microplastics to larger rivers (Mani et al., 2016; Scherer et al., 2020).
Our study focuses on the confluence of the Mulde (major tributary) and the Elbe rivers (Junge, 2020). In a previous study, Scherer et al. (2020) showed that the site of Dessau along the Elbe was highly contaminated with microbeads. By sampling both, sediment and water of the Elbe, upstream and downstream of the confluence, we intend to 1) clarify if the Mulde contributes to a substantial share of microplastic to the Elbe and 2) analyse the spatial distribution of microplastic contamination of the area on a local scale. Furthermore, we 3) quantify and characterize the microplastic particles by optical microscopy and pyrolysis Gas Chromatography/Mass Spectrometry (pyr-GC-MS).
2 Materials and Methods
2.1 Research Area and Sampling
The Elbe river is one of the largest rivers in Central Europe. It originates in the Giant Mountains in the Czech Republic and debouches after 1094 km course close to Cuxhaven into the North Sea in Germany. Its catchment covers an area of approximately 148,000 km2 with roughly 24.5 million inhabitants. The Elbe can be separated in the Upper Elbe (Czech Republic and German Elbe Sandstone Mountains until Castle Hirschstein), the Middle Elbe (from Castle Hirschstein until the barrage at Geesthacht close to Hamburg) and the tide influenced Lower Elbe from Geesthacht to the open North Sea at Cuxhaven-Kugelbake (Scherer et al., 2020).
The Mulde river is the fourth main tributary of the Elbe, after Vltava, Saale and Havel, and covers a catchment of approximately 7,400 km2 (Schneider and Reincke, 2006) (Figure 1). Intense and long-term anthropogenic activities of ore mining, smelting and metalworking industries led to continuous inputs of trace metals to the river, that are detectable in both, water and sediments of the Mulde (Junge, 2020). Additionally, plastic-processing industries in the surroundings of the cities of Bitterfeld and Dessau could potentially contribute microplastics to the river (Scherer et al., 2020).
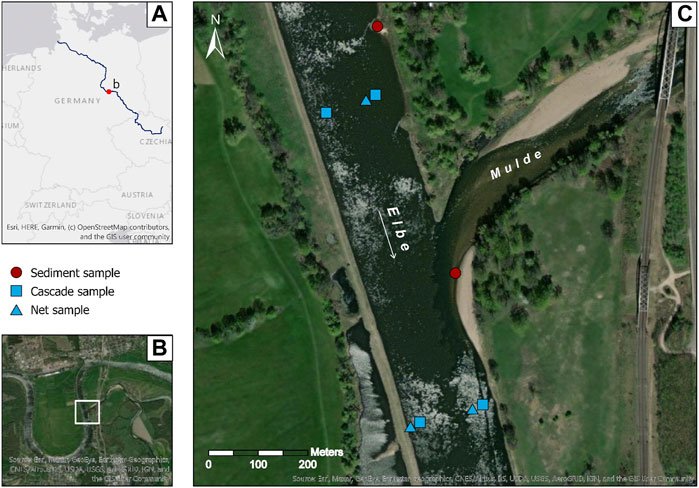
FIGURE 1. (A,B) Location of the Elbe–Mulde confluence in Dessau-Roßlau (Germany). (C) One sediment sample and three water samples were taken upstream of the confluence, and four water samples and one sediment sample were taken downstream.
In January 2020 samples were taken in the Elbe nearby the city of Dessau-Roßlau upstream and downstream of the confluence with the Mulde river, close to both riversides (Figure 1). Seven water samples were retrieved with two different methods. For smaller particles, a filter cascade with mesh sizes of 100 and 50 μm was used for four samples (fractionated pressure filtration) (Klein et al., 2018; Stock et al., 2019). The filter cascade was connected with a pump which was placed in a depth of 30 cm below the water surface filtering 530–680 L per sample. For larger particles, an Apstein plankton net (opening: 0.022 m2, diameter 17 cm, length 110 cm) with two connected nets of 150 and 300 μm mesh size was used for three samples. For measuring the water volume, a flow meter was fixed on the plankton net (40 and 53 m3 per sample) (Klein et al., 2018; Stock et al., 2019). Samples were stored in glass jars until further processing.
Additionally, two sediment samples were taken at the left shoreline of the Elbe, from the river banks upstream and downstream of the Mulde confluence with a Van Veen grab sampler (1.2 kg of sediment upstream and 1.23 kg of sediment downstream, respectively) (Figure 1). Sediments were transferred into glass jars until further processing.
2.2 Sample Preparation
In the laboratory, the sediment samples were dried in an oven at 40°C for 5 days. All subsequent sample preparation steps in the laboratory were done under a laminar flow box to avoid contamination. The samples were dry-sieved on a vibrating shaker using a five-sieves cascade (Microtrac Retsch GmbH, Haan, Germany) with the mesh sizes of 1,000, 500, 100, 50 and 20 μm (Enders et al., 2019). Subsequently, they were placed on a transversal cross-shaker and treated with 35% hydrogen peroxide (H2O2) to dissolve the organic matter (Mai et al., 2018). After all visible reaction has ceased, the samples were transferred to glass separation funnels. A saturated potassium formate [K(HCOO)] solution was added for a density separation to remove the inorganic sediment material (Mai et al., 2018; Enders et al., 2019; Stock et al., 2019). Finally, the supernatant containing the microplastics was vacuum-filtered onto glass microfibre filters (Fisherbrand MF 100 by Fisher Scientific, Waltham, Massachusetts, United States) (Pagter et al., 2018).
The water samples were vacuum-filtered with stainless-steel sieves with mesh sizes of 50 and 100 μm for the samples of the filter cascade, and mesh sizes of 150, 300 and 500 μm for the samples of the nets. Similarly to the sediment samples, the organic matter was destroyed by adding hydrogen peroxide (H2O2) and the samples were filtered on glass microfibre filters.
2.3 Identification of Microplastics in Water and Sediment Samples
2.3.1 Visual Identification
Each filter was photographed under a digital microscope (VHX-2000 by Keyence Corporation, Osaka, Japan) equipped with 200 × magnifying lenses. Presumable microplastic particles were counted and their shape determined (Hidalgo-Ruz et al., 2012). The number of particles was related to the dry weight of the sediment samples (number of particles per kg dry sediment) or the volume of the water samples (number of particles per m3 of water), respectively (Mai et al., 2018). Then, 121 particles were picked, photographed and their size measured in the same digital microscope. Kindly note that the 100 μm water sample downstream on the left side was pyrolysed before pictures could be taken.
2.3.2 Pyr-GC-MS Analysis
The photographed single particles were analysed to identify the polymer type of frequently occurring particles (e.g., spheres, fibres etc.). The remaining particles were extracted via pressurized liquid extraction similar to sample preparation published by Dierkes et al. (2019). Instead of the described equipment (ASE-350, Dionex, Sunnyvale, CA, United States) an alternative extraction system was used (EDGE, CEM, Matthews, NC, United States) with parameters listed in Table 1.
Briefly, filters were transferred to aluminium-coated cups (Q-cups; CEM, Matthews, NC, United States) covered with calcined (600°C, 2.5 h) sea sand and automatically extracted with methanol (MeOH, LC-MS grade; Merck, Darmstadt, Germany) to reduce disturbing organic matrix effects. Subsequently, microplastic particles were extracted with tetrahydrofuran (THF, HPLC grade, unstabilised; Sigma-Aldrich, Schnelldorf, Germany). While MeOH-extracts were discarded, THF-extracts were collected in 60 ml-vials previously filled with 200 mg calcined silica gel. Fluorinated polystyrene [poly(4-fluorstyrene), PFS; PolymerSource, Montreal, Canada) was used as internal standard (10 ml of 1 mg ml−1 in dichloromethane (DCM, picograde; Sigma-Aldrich, Schnelldorf, Germany)] (Lauschke et al., 2021) (Table 1; Supplementary Table S1). Calcined silica gel was used to capture precipitating synthetic polymers as THF was subsequently evaporated for Pyr-GC-MS analysis. Adhered microplastics were manually rinsed off vial walls with DCM for at least three times. Then, silica gel was manually homogenised in an agate mortar and aliquots of 20 mg were weighted into 80 μl pyrolysis cups (Eco-Cup LF, Frontier Laboratories, Saikon, Japan) and pyrolysed at 600°C. Pyr-GC-MS analysis was conducted as described by Dierkes et al. (2019), except that a DB-5ms capillary separation column (Agilent, Santa Clara, CA, United States) was used. For the single particles, scan mode was used (qualitative analysis), while for the remaining mass-based quantification selected ion monitoring (SIM) mode was applied. Therefore, pyrolysis products and indicator ions, respectively, were monitored as shown in Table 2. Kindly note that the utilised pyr-GC-MS method is currently limited to determine mass concentrations of the three polymers PE, PP and PS.
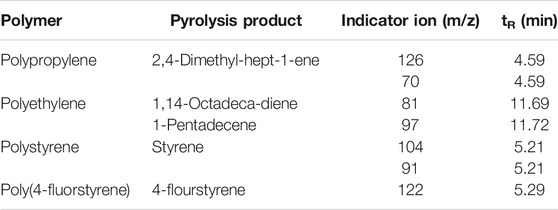
TABLE 2. Indicator compounds and selected indicator ions in the pyr-GC-MS analysis (m/z, mass/charge; tR, retention time).
2.3.3 Validation and Quality Control
To estimate the recovery rates of microplastic particles during the sample preparation, artificial quartz sand-silt mixture (approx. 1,500 g with a ratio of 60% sand and 40% silt) were spiked with PS, PET, LDPE, PP and PVC (30 particles of 200–2,000 μm each). This artificial validation sample was treated equally to the sediment samples. The total recovery rate for the whole extraction process equals 71.3%.
In addition, two blank samples were used to assess the influence of airborne contamination in the laboratory (Klein et al., 2018; Mai et al., 2018). In one of the blank samples, we detected two, in the other six fibres. Furthermore, blank filters were put next to the microscope during visual identification to quantify contamination during the analysis (Scherer et al., 2020). Here, one fibre was found on the filter. Therefore, the airborne contamination during sample preparation and visual analysis can be considered as low and no correction of the concentration of microplastics was done.
3 Results
3.1 Visual Identification
In total, 1,782 presumable microplastics were counted and categorized under the microscope, 393 in sediment samples and 1,389 in water samples. Within the sediment samples, spheres occurred most frequently with a notable difference of 35 presumable microplastic particles kg−1 upstream and 208 presumable microplastic particles kg−1 downstream of the Mulde confluence (Figure 2; Supplementary Table S1). In contrast, the numbers of fibres, films, and fragments remained rather low in both locations (27 presumable microplastic particles kg−1 or less). Most spheres were in the size fraction between 500 and 1,000 μm and between 50 and 100 μm.
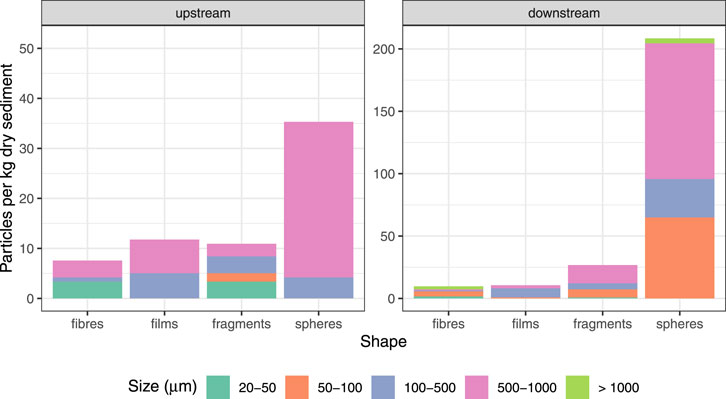
FIGURE 2. Number of presumable microplastics in sediment samples by shape. Note different scales in the ratio 1:4 on the y axis.
Due to the different sampling methods of the water samples, either with the Apstein plankton net or the filter cascade, comparisons need to be considered carefully. Indeed, the particle sizes differ between the sampling techniques because of different mesh sizes of the cascade and the net, respectively. We found substantially more fibres in the cascade than in the plankton net (188 versus 15 presumable microplastic particles m−3 Figure 3 and Supplementary Figure S1). While the number of fibres, especially in the cascade samples, remained large in all samples, the occurrence of spheres seems to be related to the sampling sites. Upstream of the confluence, we found a few spheres only. In contrast, the number of larger spheres in the net increased downstream and especially on the left riverside that is adjacent to the Mulde.
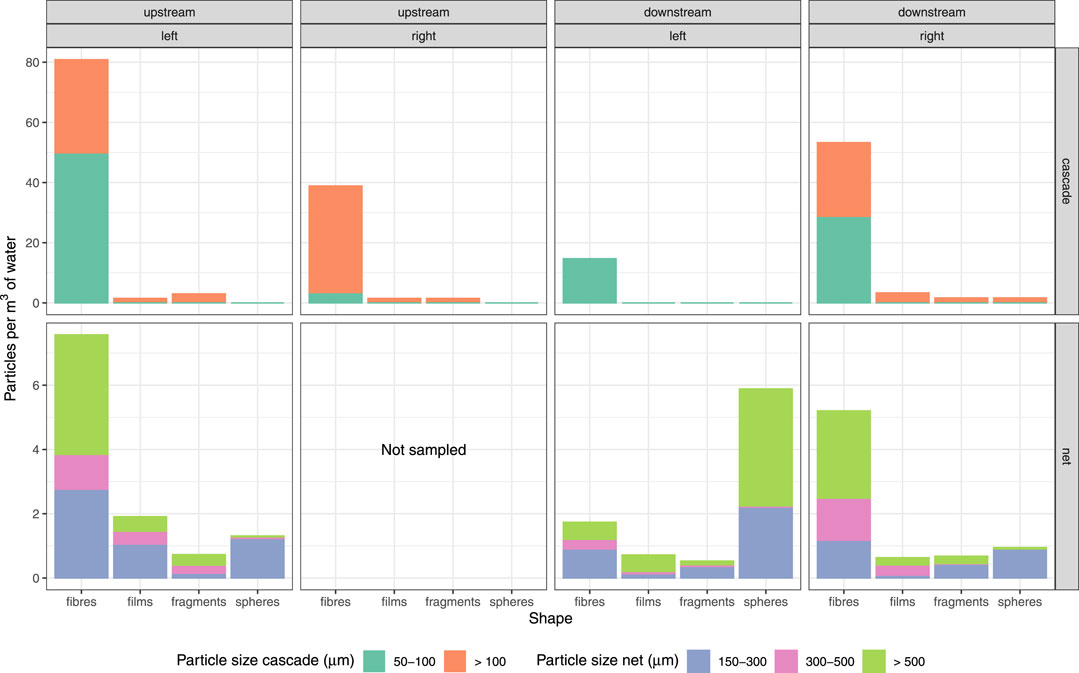
FIGURE 3. Number of microplastics in water samples by shape. Note different scales on the y axes for cascade and net samples.
3.2 Pyr-GC-MS Analysis
Altogether, 121 single presumable microplastic particles were picked, 87 from water samples (20 upstream and 67 downstream) and 34 particles from sediment samples (18 upstream and 16 downstream). More than half of these 121 isolated presumable microplastic particles were spheres, from which almost all (62 out of 68) were identified as PS or PS-DVB, while a certain number of fragments consisted of PE, PP or PS. 36 particles could not be identified (Supplementary Table S1).
In the remaining sediment sample upstream of the confluence, we found PE almost exclusively (Figure 4). Especially in the coarser fractions (500–1000 μm and
The concentrations of microplastics (all polymers taken together) in the water samples varied between 0.33 mg m−3 (downstream, left side, net sample) and 1.19 mg m−3 (upstream, left side, cascade sample). PE and PS were roughly equally common in the cascade samples, with maxima of 0.60 mg m−3 and 0.56 mg m−3, respectively (upstream, left side, all fractions summed up) while the net samples indicated higher shares of PE. The concentration of PP remained below 0.05 mg m−3 (Figure 5).
4 Discussion
4.1 Microplastic Abundance in Water and Sediments
The sediment and water samples which we took upstream of the Elbe–Mulde confluence show that–regardless of the sampling site and type–the Elbe carries a certain load of microplastics. They are mainly composed of fibres; however, the water also contains small amounts of spheres, fragments, and films. Whether these values truly reflect the average microplastic load remains open, since our samples provide a snapshot at the moment of sampling only. Indeed, the number of microplastics in the river water can vary over the seasons and with flood events (Constant et al., 2020; Mani and Burkhardt-Holm, 2020; Napper et al., 2021). This also holds true for the sediment samples that were taken at the riverside. There, the occurrence of microplastics depends mostly on deposition and re-mobilisation, driven by the water level, flooding, flow dynamics and exposure to the atmosphere during low discharge (Hurley et al., 2018; Constant et al., 2020; Mani and Burkhardt-Holm, 2020).
The remarkable amount of (PS) spheres downstream the confluence originates most likely from the Mulde river. The largest concentrations of spheres can be found in the water samples closest to the confluence downstream on the left river side (Figure 3). With increasing distance to the confluence at the sampling sites of the cascade and net samples on the right sight of the Elbe, the influence of the Mulde decreases and the abundance of microplastics is comparable to that found upstream of the confluence. Similarly, a larger number of spheres on the Mulde-dominated left river bank supports the hypothesis of spheres originating from the Mulde (Figure 4). Therefore, the influence of the Mulde as one of possible sources for microplastic spheres in the Elbe is very likely. On the other hand, the Mulde seems to contribute few fibres only. While upstream at both sides and downstream on the right side fibres abound, the strongly Mulde-influenced water sample downstream on the right side contains far lower amounts of fibres (Figure 3).
The pyr-GC-MS analysis of the isolated particles reveal on the one hand that these spheres consists of PS or PS-DVB. This accords with the high PS content of the sediment sample downstream of the Mulde confluence (Figure 4). However, water samples with a large content of spheres do not show a higher concentration of PS. This indicates a certain degree of incomparability between visual and chemical analyses, and also between water and sediment samples. A possible explanation for this discrepancy could be that the spheres do not consist of 100% PS, but are made of another polymer and eventually only coated with PS. A clear categorization of single particles based on pyr-GC-MS remains challenging, especially because the differentiation between PS and PS-DVB is complicated due to the weak DVB signal. Therefore, PS-DVB is often identified as PS (Mani et al., 2019; Scherer et al., 2020).
A potential source and original function of these spheres could be so-called ion-exchange resin (IER) beads that are commonly used e.g., in industrial waste water treatment plants for softening and desalination of water (or aqueous solutions), a phenomenon known from several other German rivers (Mani et al., 2019). An increased occurrence of the spheres in the environment is commonly attributed to one or several point sources (Mani et al., 2019), which are often formed by the discharge of industrial (micro-)plastic production plants (Lechner and Ramler, 2015) or the above-mentioned wastewater treatment plants (Browne et al., 2011; Kay et al., 2018; Schmidt et al., 2020). However, our data set is too limited to clearly trace the origin of these particles. Further studies are in preparation.
Because the utilised pyr-GC-MS method is limited to the quantification of PS, PP and PE, only those polymers could be identified in our study. Other frequently used polymers such as PMMA, PET or PBT remained undetected. Furthermore, due to sieving no particles smaller than 20 μm could be analysed in the sediments. However, small microplastics are often abundant in river waters, sediments and the hyporheic zone and therefore of great relevance for microplastic research (Frei et al., 2019).
Additionally, the sampling methods and possible local peculiarities of the sampling site affect the detection of microplastic particles (Hidalgo-Ruz et al., 2012; Klein et al., 2018; Lenaker et al., 2019; Stock et al., 2019). For example, the smallest size of detectable particles in the water samples is limited by the mesh size and varies between the cascades (fractions of 50–100 and
Moreover, the sampling depth of 30 cm below the water surface might have an impact on the abundance of detected polymers and particle shapes (Eriksen et al., 2013; Löder et al., 2017). Due to the specific density of the polymers, the particles’ size and shape and the turbulent flow in the river, higher concentrations might have been detected at a greater water depth or directly at the surface (Waldschläger and Schüttrumpf, 2019a; Lenaker et al., 2019; Wurpts and Shiravani, 2019). Therefore, the total load of the Elbe might still be underestimated and a comprehensive sampling over the entire water column across the river would be desirable.
Furthermore, it should be noted that the sediment samples were dry-sieved. Compared to wet-sieved samples, microplastic particles could be distributed differently between the size fractions. However, the total number of particles remains the same. Additionally, the relatively high total recovery rate indicates a certain accuracy of our sample preparation and analysis.
4.2 Comparison With Other Parts of the Elbe and European Rivers
The high share of fibres in all water samples accords well with the results of several other studies on abundance of microplastics in the Elbe (Scherer et al., 2020) and other European rivers (Frei et al., 2019; Lenaker et al., 2019; Napper et al., 2021). Due to their low density, small fibres tend to occur rather close to the surface, which is reflected especially in our cascade samples. Numerous potential sources can contribute microplastics to the river, e.g., urban and industrial areas within the catchment in general and waste water treatment plants as typical effluents for fibres in particular (Kay et al., 2018; Haberstroh et al., 2021).
The abundance of the most frequent polymers also reflects, to a certain extent, the findings of other studies. Especially the large share of PP and PS in the single analysed particles agrees with studies, e.g., on Swiss lakes (Faure et al., 2015), different sites from the Rhine and Main areas (Klein et al., 2015; Mani et al., 2016), the United Kingdom (Sadri and Thompson, 2014) and other places across Europe and Asia (Browne et al., 2011). Although the amounts of PP measured by pyr-GC-MS in our samples were small, one should keep in mind that the mass-based pyrolysis results give no information on the number of particles, which is well shown by Scherer et al. (2020) for the Elbe.
Scherer et al. (2020) worked on several sites along the Elbe. In their study, the amounts of PP also remained quite low in all sediment samples which were taken along the Middle, Lower and Outer Elbe (between 1.7 and 7.8 mg kg−1). However, the share of PE varied between ca. 2 and 80 mg kg−1. The concentration of PS varied even stronger. It remained mainly low (between 0 and 2 mg kg−1), but increased to over 150 mg kg−1 in Geesthacht, where the Lower Elbe begins (Scherer et al., 2020). This sudden change in microplastic concentrations can be related to tidal influence and the barrage in Geesthacht. Close to our research area (further downstream), Scherer et al. (2020) measured concentration of 34 mg kg−1 PE, 3.25 mg kg−1 PP and 1 mg kg−1 PS. While the latter one accords roughly with our findings, the concentrations of PE and PP are both several times larger in the study by Scherer et al. (2020) compared to our samples.
Local conditions at sampling sites restrict the comparison of the absolute abundance of microplastics in the Elbe with other sites or rivers. We analysed one sample upstream and downstream of the confluence, respectively. The exact location of the samples (possibility of sedimentation of microplastics) might strongly influence the results. Indeed, flow patterns have a major impact on the transport, settling, deposition and remobilization of microplastics (Waldschläger and Schüttrumpf, 2019b). A larger transport capacity, for example, might impede deposition at riversides or in the hyporheic zone (Boano et al., 2014; Frei et al., 2019). Furthermore, a larger transport energy might (re-)mobilize coarser sediment that could crush microplastic particles and contribute to their physical degradation (Ding et al., 2019). The difference in sample preparation, especially for the sediment samples with dry-sieving, must also be considered. However, our results accord well with Scherer et al. (2020) who found comparably large amounts of primary PS-DVB spheres close to the Mulde confluence.
Scherer et al. (2020) estimated the concentration of microplastics in the Elbe water samples to be rather low compared to rivers that contribute a large global share of microplastics to the oceans (Meijer et al., 2021). On a regional scale, the studies by Mani et al. (2016) in the Rhine and Wagner et al. (2014) in the Elbe, Moselle, Neckar, and Rhine rivers, Weber and Opp (2020) in the Lahn, Horton et al. (2017) in the Thames, Constant et al. (2020) in the Rhone and Lechner and Ramler (2015), Pojar et al. (2021) in Austrian and Romanian parts of the Danube would be some of only a few eligible comparisons. Compared to these rivers, the microplastic concentrations of the Elbe estimated by Scherer et al. (2020) are lower in the water samples, while the concentrations in the sediments are comparable. However, the microplastic concentrations that were measured in this study remain at remarkably low levels.
A comparison with rivers on a global scale remains challenging as well. Besides the complexity of fluvial dynamics, different climatic and geomorphological conditions, the occurrence and distribution of microplastics may vary strongly (Kay et al., 2018; Haberstroh et al., 2021). Nevertheless, several studies showed that rivers draining large, densely populated and industrialized catchments carry considerable loads of microplastics (Lebreton et al., 2017; Gerolin et al., 2020; Napper et al., 2021) and discharge an amount of approximately 1.15–2.41 million tonnes of (micro and macro) plastic per year into the world oceans (Lebreton et al., 2017). Because Southeast Asia, e.g., in India and People’s Republic of China, are such densely populated regions with an advancing industrialization, it is not surprising to find particularly large microplastic loads and concentrations there (Zhao et al., 2014; Lebreton et al., 2017; Yan et al., 2019; Napper et al., 2021).
5 Conclusion
We detected microplastics in all sediment and water samples taken from the Elbe close to the Mulde confluence by optical microscopy and pyr-GC-MS. In all water samples, we found numerous fibres. Although it is challenging to compare the results of the visual and chemical analyses, this large number of fibres roughly coincides with high PE concentrations. In contrast, we detected large numbers of PS (or PS-DVB) spheres in the sediment sample directly downstream of the Mulde confluence only. Although a clear identification of possible source area(s) remains challenging, the distribution pattern suggest that the Mulde contributes microplastics (especially spheres) to the Elbe. These findings reinforce the argument that tributaries may be important sources of microplastics in larger rivers, and might be applied to other catchments as well. However, a comparison with other sampling sites along the Elbe and other (European) rivers remains tentative due to different sampling and analytical approaches. In our study, the differences between water samples collected with Apstein nets and the filter cascades confirm this challenge. Nevertheless, our results may serve to better understand the different contributors and microplastic occurrence in a fluvial catchment.
Data Availability Statement
Data for the calibration of the pyr-GC-MS analysis is provided in the Supplementary Material. Data and code necessary to reproduce the analysis presented in the study are published on Zenodo, https://doi.org/10.5281/zenodo.5691239. Original photographs of filtered water and sediment samples can be obtained from the first authors upon request.
Author Contributions
HL, FS, JK, CB, and GR conceived the study; field work was performed by FS, DS, and CS; JK prepared the water and sediment samples for analysis with contribution by HL, FS, GD, and CF; CF and GD measured and analysed the pyr-GC-MS; JK and CB provided the graphics; HL, CF, FS, JK, and CB wrote the manuscript; all authors critically discussed the results, commented and reviewed the manuscript.
Funding
This study is embedded in the Project “Micro- and macroplastic in federal water ways of Germany” (Project 4.1.12, Project No. M39620304028) that intends to analyse the occurrence, transport mechanism, ecological risks and management options. This project was funded by the Deutsche Forschungsgemeinschaft (DFG, German Research Foundation), Project Number 391977956, SFB 1357, subproject B06.
Conflict of Interest
The authors declare that the research was conducted in the absence of any commercial or financial relationships that could be construed as a potential conflict of interest.
Publisher’s Note
All claims expressed in this article are solely those of the authors and do not necessarily represent those of their affiliated organizations, or those of the publisher, the editors and the reviewers. Any product that may be evaluated in this article, or claim that may be made by its manufacturer, is not guaranteed or endorsed by the publisher.
Acknowledgments
We thank the staff of the BfG laboratory for their support during the pyr-GC-MS analysis. Julia Horn helped with geochemical and sedimentological measurements in the laboratory of the Institute of Geography at the University of Cologne.
Supplementary Material
The Supplementary Material for this article can be found online at: https://www.frontiersin.org/articles/10.3389/fenvs.2021.794895/full#supplementary-material
Abbreviations
PS, Polystyrene; PP, Polypropylene; PE, Polyethylene; PS-DVB, Polystyrene divinylbenzene; PMMA, Polymethyl methacrylate; PET, Polyethylene terephthalate; PBT, Polybutylene terephthalate.
References
Blair, R. M., Waldron, S., Phoenix, V., and Gauchotte-Lindsay, C. (2017). Micro- and Nanoplastic Pollution of Freshwater and Wastewater Treatment Systems. Springer Sci. Rev. 5, 19–30. doi:10.1007/s40362-017-0044-7
Boano, F., Harvey, J. W., Marion, A., Packman, A. I., Revelli, R., Ridolfi, L., et al. (2014). Hyporheic Flow and Transport Processes: Mechanisms, Models, and Biogeochemical Implications. Rev. Geophys. 52, 603–679. doi:10.1002/2012RG000417
Brandes, E., Henseler, M., and Kreins, P. (2021). Identifying Hot-Spots for Microplastic Contamination in Agricultural Soils-A Spatial Modelling Approach for Germany. Environ. Res. Lett. 16, 104041. doi:10.1088/1748-9326/ac21e6
Browne, M. A., Crump, P., Niven, S. J., Teuten, E., Tonkin, A., Galloway, T., et al. (2011). Accumulation of Microplastic on Shorelines Woldwide: Sources and Sinks. Environ. Sci. Technol. 45, 9175–9179. doi:10.1021/es201811s
C. Arthur, J. Baker, and H. Bamfort (Editors) (2009). Proceedings of the International Research Workshop on the Occurrence, Effects, and Fate of Microplastic Marine Debris (United States: National Ocean Service., Office of Response and Restoration). Technical Memorandum NOS-OR&R-30.
Constant, M., Ludwig, W., Kerhervé, P., Sola, J., Charrière, B., Sanchez-Vidal, A., et al. (2020). Microplastic Fluxes in a Large and a Small Mediterranean River Catchments: The Têt and the Rhône, Northwestern Mediterranean Sea. Sci. Total Environ. 716, 136984. doi:10.1016/j.scitotenv.2020.136984
Dierkes, G., Lauschke, T., Becher, S., Schumacher, H., Földi, C., and Ternes, T. (2019). Quantification of Microplastics in Environmental Samples via Pressurized Liquid Extraction and Pyrolysis-Gas Chromatography. Anal. Bioanal. Chem. 411, 6959–6968. doi:10.1007/s00216-019-02066-9
Ding, L., Mao, R. f., Guo, X., Yang, X., Zhang, Q., and Yang, C. (2019). Microplastics in Surface Waters and Sediments of the Wei River, in the Northwest of China. Sci. Total Environ. 667, 427–434. doi:10.1016/j.scitotenv.2019.02.332
Dris, R., Imhof, H., Sanchez, W., Gasperi, J., Galgani, F., Tassin, B., et al. (2015). Beyond the Ocean: Contamination of Freshwater Ecosystems with (Micro-)plastic Particles. Environ. Chem. 12, 539. doi:10.1071/EN14172
Eerkes-Medrano, D., Thompson, R. C., and Aldridge, D. C. (2015). Microplastics in Freshwater Systems: A Review of the Emerging Threats, Identification of Knowledge Gaps and Prioritisation of Research Needs. Water Res. 75, 63–82. doi:10.1016/j.watres.2015.02.012
Enders, K., Käppler, A., Biniasch, O., Feldens, P., Stollberg, N., Lange, X., et al. (2019). Tracing Microplastics in Aquatic Environments Based on Sediment Analogies. Sci. Rep. 9, 15207. doi:10.1038/s41598-019-50508-2
Eriksen, M., Mason, S., Wilson, S., Box, C., Zellers, A., Edwards, W., et al. (2013). Microplastic Pollution in the Surface Waters of the Laurentian Great Lakes. Mar. Pollut. Bull. 77, 177–182. doi:10.1016/j.marpolbul.2013.10.007
Faure, F., Demars, C., Wieser, O., Kunz, M., and de Alencastro, L. F. (2015). Plastic Pollution in Swiss Surface Waters: Nature and Concentrations, Interaction with Pollutants. Environ. Chem. 12, 582. doi:10.1071/EN14218
Frei, S., Piehl, S., Gilfedder, B. S., Löder, M. G. J., Krutzke, J., Wilhelm, L., et al. (2019). Occurence of Microplastics in the Hyporheic Zone of Rivers. Sci. Rep. 9, 15256. doi:10.1038/s41598-019-51741-5
Gerolin, C. R., Pupim, F. N., Sawakuchi, A. O., Grohmann, C. H., Labuto, G., and Semensatto, D. (2020). Microplastics in Sediments from Amazon Rivers, Brazil. Sci. Total Environ. 749, 141604. doi:10.1016/j.scitotenv.2020.141604
Geyer, R., Jambeck, J. R., and Law, K. L. (2017). Production, Use, and Fate of All Plastics Ever Made. Sci. Adv. 3, e1700782. doi:10.1126/sciadv.1700782
Haberstroh, C. J., Arias, M. E., Yin, Z., and Wang, M. C. (2021). Effects of Urban Hydrology on Plastic Transport in a Subtropical River, 1. ACS ES&T Water, acsestwater, 1714–1727. doi:10.1021/acsestwater.1c00072Effects of Urban Hydrology on Plastic Transport in a Subtropical RiverACS EST Water
Heß, M., Diehl, P., Mayer, J., Rahm, H., Reifenhäuser, W., Stark, J., et al. (2018). Mikroplastik in Binnengewässern Süd- und Westdeutschlands. Teil 1: Kunststoffpartikel in der oberflächennahen Wasserphase. Tech. Rep.
Hidalgo-Ruz, V., Gutow, L., Thompson, R. C., and Thiel, M. (2012). Microplastics in the Marine Environment: A Review of the Methods Used for Identification and Quantification. Environ. Sci. Technol. 46, 3060–3075. doi:10.1021/es2031505
Horton, A. A., and Dixon, S. J. (2018). Microplastics: An Introduction to Environmental Transport Processes. WIREs Water 5, e1268. doi:10.1002/wat2.1268
Horton, A. A., Walton, A., Spurgeon, D. J., Lahive, E., and Svendsen, C. (2017). Microplastics in Freshwater and Terrestrial Environments: Evaluating the Current Understanding to Identify the Knowledge Gaps and Future Research Priorities. Sci. Total Environ. 586, 127–141. doi:10.1016/j.scitotenv.2017.01.190
Hurley, R. R., and Nizzetto, L. (2018). Fate and Occurrence of Micro(nano)plastics in Soils: Knowledge Gaps and Possible Risks. Curr. Opin. Environ. Sci. Health 1, 6–11. doi:10.1016/j.coesh.2017.10.006
Hurley, R., Woodward, J., and Rothwell, J. J. (2018). Microplastic Contamination of River Beds Significantly Reduced by Catchment-wide Flooding. Nat. Geosci 11, 251–257. doi:10.1038/s41561-018-0080-1
Junge, F. W. (2020). Aquatische Makrophyten als Indikatoren für die Elementverteilung im Fließgewässer Mulde. Mitteldeutschland. doi:10.5675/HYWA_2020.3_2
Kataoka, T., Nihei, Y., Kudou, K., and Hinata, H. (2019). Assessment of the Sources and Inflow Processes of Microplastics in the River Environments of Japan. Environ. Pollut. 244, 958–965. doi:10.1016/j.envpol.2018.10.111
Kay, P., Hiscoe, R., Moberley, I., Bajic, L., and McKenna, N. (2018). Wastewater Treatment Plants as a Source of Microplastics in River Catchments. Environ. Sci. Pollut. Res. 25, 20264–20267. doi:10.1007/s11356-018-2070-7
Klein, S., Dimzon, I. K., Eubeler, J., and Knepper, T. P. (2018). “Analysis, Occurrence, and Degradation of Microplastics in the Aqueous Environment,” in Freshwater Microplastics. Editors M. Wagner, and S. Lambert (Cham: Springer International Publishing), 58, 51–67. doi:10.1007/978-3-319-61615-5_3
Klein, S., Worch, E., and Knepper, T. P. (2015). Occurrence and Spatial Distribution of Microplastics in River Shore Sediments of the Rhine-Main Area in Germany. Environ. Sci. Technol. 49, 6070–6076. doi:10.1021/acs.est.5b00492
Lauschke, T., Dierkes, G., Schweyen, P., and Ternes, T. A. (2021). Evaluation of Poly(styrene-D5) and Poly(4-Fluorostyrene) as Internal Standards for Microplastics Quantification by Thermoanalytical Methods. J. Anal. Appl. Pyrolysis 159, 105310. doi:10.1016/j.jaap.2021.105310
Lebreton, L. C. M., van der Zwet, J., Damsteeg, J.-W., Slat, B., Andrady, A., and Reisser, J. (2017). River Plastic Emissions to the World's Oceans. Nat. Commun. 8, 15611. doi:10.1038/ncomms15611
Lechner, A., and Ramler, D. (2015). The Discharge of Certain Amounts of Industrial Microplastic from a Production Plant into the River Danube Is Permitted by the Austrian Legislation. Environ. Pollut. 200, 159–160. doi:10.1016/j.envpol.2015.02.019
Lechthaler, S., Esser, V., Schüttrumpf, H., and Stauch, G. (2021). Why Analysing Microplastics in Floodplains Matters: Application in a Sedimentary Context. Environ. Sci. Process. Impacts 23, 117–131. doi:10.1039/D0EM00431F
Lenaker, P. L., Baldwin, A. K., Corsi, S. R., Mason, S. A., Reneau, P. C., and Scott, J. W. (2019). Vertical Distribution of Microplastics in the Water Column and Surficial Sediment from the Milwaukee River Basin to Lake Michigan. Environ. Sci. Technol. 53, 12227–12237. doi:10.1021/acs.est.9b03850
Löder, M. G. J., Imhof, H. K., Ladehoff, M., Löschel, L. A., Lorenz, C., Mintenig, S., et al. (2017). Enzymatic Purification of Microplastics in Environmental Samples. Environ. Sci. Technol. 51, 14283–14292. doi:10.1021/acs.est.7b03055
Mai, L., Bao, L.-J., Shi, L., Wong, C. S., and Zeng, E. Y. (2018). A Review of Methods for Measuring Microplastics in Aquatic Environments. Environ. Sci. Pollut. Res. 25, 11319–11332. doi:10.1007/s11356-018-1692-0
Mani, T., and Burkhardt-Holm, P. (2020). Seasonal Microplastics Variation in Nival and Pluvial Stretches of the Rhine River - from the Swiss Catchment towards the North Sea. Sci. Total Environ. 707, 135579. doi:10.1016/j.scitotenv.2019.135579
Mani, T., Hauk, A., Walter, U., and Burkhardt-Holm, P. (2016). Microplastics Profile along the Rhine River. Sci. Rep. 5, 17988. doi:10.1038/srep17988
Mani, T., Primpke, S., Lorenz, C., Gerdts, G., and Burkhardt-Holm, P. (2019). Microplastic Pollution in Benthic Midstream Sediments of the Rhine River. Environ. Sci. Technol. 53, 6053–6062. doi:10.1021/acs.est.9b01363
Meides, N., Menzel, T., Poetzschner, B., Löder, M. G. J., Mansfeld, U., Strohriegl, P., et al. (2021). Reconstructing the Environmental Degradation of Polystyrene by Accelerated Weathering. Environ. Sci. Technol. 55, 7930–7938. doi:10.1021/acs.est.0c07718
Meijer, L. J. J., van Emmerik, T., van der Ent, R., Schmidt, C., and Lebreton, L. (2021). More Than 1000 Rivers Account for 80% of Global Riverine Plastic Emissions into the Ocean. Sci. Adv. 7, eaaz5803. doi:10.1126/sciadv.aaz5803
Murphy, F., Ewins, C., Carbonnier, F., and Quinn, B. (2016). Wastewater Treatment Works (WwTW) as a Source of Microplastics in the Aquatic Environment. Environ. Sci. Technol. 50, 5800–5808. doi:10.1021/acs.est.5b05416
M. Wagner, and S. Lambert (Editors) (2018). Freshwater Microplastics: Emerging Environmental Contaminants? The Handbook of Environmental Chemistry (Cham: Springer International Publishing). doi:10.1007/978-3-319-61615-5
Napper, I. E., Baroth, A., Barrett, A. C., Bhola, S., Chowdhury, G. W., Davies, B. F. R., et al. (2021). The Abundance and Characteristics of Microplastics in Surface Water in the Transboundary Ganges River. Environ. Pollut. 274, 116348. doi:10.1016/j.envpol.2020.116348
Pagter, E., Frias, J., and Nash, R. (2018). Microplastics in Galway Bay: A Comparison of Sampling and Separation Methods. Mar. Pollut. Bull. 135, 932–940. doi:10.1016/j.marpolbul.2018.08.013
Petersen, F., and Hubbart, J. A. (2021). The Occurrence and Transport of Microplastics: The State of the Science. Sci. Total Environ. 758, 143936. doi:10.1016/j.scitotenv.2020.143936
Pojar, I., Stănică, A., Stock, F., Kochleus, C., Schultz, M., and Bradley, C. (20212000). Sedimentary Microplastic Concentrations from the Romanian Danube River to the Black Sea. Sci. Rep. 11, 2000. doi:10.1038/s41598-021-81724-4
Prata, J. C., da Costa, J. P., Duarte, A. C., and Rocha-Santos, T. (2019). Methods for Sampling and Detection of Microplastics in Water and Sediment: A Critical Review. Trac Trends Anal. Chem. 110, 150–159. doi:10.1016/j.trac.2018.10.029
Rillig, M. C., and Lehmann, A. (2020). Microplastic in Terrestrial Ecosystems. Science 368, 1430–1431. doi:10.1126/science.abb5979
Rochman, C. M. (2018). Microplastics Research-From Sink to Source. Science 360, 28–29. doi:10.1126/science.aar7734
Sadri, S. S., and Thompson, R. C. (2014). On the Quantity and Composition of Floating Plastic Debris Entering and Leaving the Tamar Estuary, Southwest England. Mar. Pollut. Bull. 81, 55–60. doi:10.1016/j.marpolbul.2014.02.020
Schell, T., Hurley, R., Nizzetto, L., Rico, A., and Vighi, M. (2021). Spatio-temporal Distribution of Microplastics in a Mediterranean River Catchment: The Importance of Wastewater as an Environmental Pathway. J. Hazard. Mater. 420, 126481. doi:10.1016/j.jhazmat.2021.126481
Scherer, C., Weber, A., Stock, F., Vurusic, S., Egerci, H., Kochleus, C., et al. (2020). Comparative Assessment of Microplastics in Water and Sediment of a Large European River. Sci. Total Environ. 738, 139866. doi:10.1016/j.scitotenv.2020.139866
Schmidt, C., Kumar, R., Yang, S., and Büttner, O. (2020). Microplastic Particle Emission from Wastewater Treatment Plant Effluents into River Networks in Germany: Loads, Spatial Patterns of Concentrations and Potential Toxicity. Sci. Total Environ. 737, 139544. doi:10.1016/j.scitotenv.2020.139544
Schmidt, L. K., Bochow, M., Imhof, H. K., and Oswald, S. E. (2018). Multi-temporal Surveys for Microplastic Particles Enabled by a Novel and Fast Application of SWIR Imaging Spectroscopy - Study of an Urban Watercourse Traversing the City of Berlin, Germany. Environ. Pollut. 239, 579–589. doi:10.1016/j.envpol.2018.03.097
Schneider, P., and Reincke, H. (2006). “Contaminated Sediments in the Elbe basin and its Tributary Mulde,” in Uranium in the Environment: Mining Impact and Consequences. Editors B. J. Merkel, and A. Hasche-Berger (Berlin, Heidelberg: Springer Berlin Heidelberg), 655–662.
Stock, F., Kochleus, C., Bänsch-Baltruschat, B., Brennholt, N., and Reifferscheid, G. (2019). Sampling Techniques and Preparation Methods for Microplastic Analyses in the Aquatic Environment - A Review. Trac Trends Anal. Chem. 113, 84–92. doi:10.1016/j.trac.2019.01.014
Sun, J., Dai, X., Wang, Q., van Loosdrecht, M. C. M., and Ni, B.-J. (2019). Microplastics in Wastewater Treatment Plants: Detection, Occurrence and Removal. Water Res. 152, 21–37. doi:10.1016/j.watres.2018.12.050
Thompson, R. C., Olsen, Y., Mitchell, R. P., Davis, A., Rowland, S. J., John, A. W. G., et al. (2004). Lost at Sea: Where Is All the Plastic? Science 304, 838. doi:10.1126/science.1094559
Tibbetts, J., Krause, S., Lynch, I., and Sambrook Smith, G. (2018). Abundance, Distribution, and Drivers of Microplastic Contamination in Urban River Environments. Water 10, 1597. doi:10.3390/w10111597
Wagner, M., Scherer, C., Alvarez-Muñoz, D., Brennholt, N., Bourrain, X., Buchinger, S., et al. (2014). Microplastics in Freshwater Ecosystems: What We Know and what We Need to Know. Environ. Sci. Eur. 26, 12. doi:10.1186/s12302-014-0012-7
Waldschläger, K., and Schüttrumpf, H. (2019a). Effects of Particle Properties on the Settling and Rise Velocities of Microplastics in Freshwater under Laboratory Conditions. Environ. Sci. Technol. 53, 1958–1966. doi:10.1021/acs.est.8b06794
Waldschläger, K., and Schüttrumpf, H. (2019b). Erosion Behavior of Different Microplastic Particles in Comparison to Natural Sediments. Environ. Sci. Technol. 53, 13219–13227. doi:10.1021/acs.est.9b05394
Waldschläger, K., and Schüttrumpf, H. (2020). Infiltration Behavior of Microplastic Particles with Different Densities, Sizes, and Shapes-From Glass Spheres to Natural Sediments. Environ. Sci. Technol. 54, 9366–9373. doi:10.1021/acs.est.0c01722
Weber, C. J., and Opp, C. (2020). Spatial Patterns of Mesoplastics and Coarse Microplastics in Floodplain Soils as Resulting from Land Use and Fluvial Processes. Environ. Pollut. 267, 115390. doi:10.1016/j.envpol.2020.115390
Wurpts, A., and Shiravani, G. (2019). Numerische Modellierung der Ausbreitung von Mikroplastik im Weser-Ästuar und angrenzenden Wattenmeer. Wasser Abfall 21, 12–17. doi:10.1007/s35152-019-0135-7
Yan, M., Nie, H., Xu, K., He, Y., Hu, Y., Huang, Y., et al. (2019). Microplastic Abundance, Distribution and Composition in the Pearl River along Guangzhou City and Pearl River Estuary, China. Chemosphere 217, 879–886. doi:10.1016/j.chemosphere.2018.11.093
Keywords: microplastics, rivers, Elbe, Mulde, sediments, pyr-GC-MS
Citation: Laermanns H, Reifferscheid G, Kruse J, Földi C, Dierkes G, Schaefer D, Scherer C, Bogner C and Stock F (2021) Microplastic in Water and Sediments at the Confluence of the Elbe and Mulde Rivers in Germany. Front. Environ. Sci. 9:794895. doi: 10.3389/fenvs.2021.794895
Received: 14 October 2021; Accepted: 16 November 2021;
Published: 17 December 2021.
Edited by:
Tanveer Adyel, Deakin University, AustraliaReviewed by:
Muhammad Reza Cordova, Indonesian Institute of Sciences, IndonesiaVeerasingam S., Qatar University, Qatar
Copyright © 2021 Laermanns, Reifferscheid, Kruse, Földi, Dierkes, Schaefer, Scherer, Bogner and Stock. This is an open-access article distributed under the terms of the Creative Commons Attribution License (CC BY). The use, distribution or reproduction in other forums is permitted, provided the original author(s) and the copyright owner(s) are credited and that the original publication in this journal is cited, in accordance with accepted academic practice. No use, distribution or reproduction is permitted which does not comply with these terms.
*Correspondence: Hannes Laermanns, aC5sYWVybWFubnNAdW5pLWtvZWxuLmRl; Friederike Stock, c3RvY2tAYmFmZy5kZQ==
†ORCID: Hannes Laermanns, orcid.org/0000-0002-6272-2688; Georg Reifferscheid, orcid.org/0000-0001-6134-8236; Georg Dierkes, orcid.org/0000-0002-2561-1866; Dirk Schaefer, orcid.org/0000-0002-0641-1471; Christina Bogner, orcid.org/0000-0003-4495-0676; Friederike Stock, orcid.org/0000-0003-4182-3778
‡These authors share senior authorship