Trace metal fate in soil after application of digestate originating from the anaerobic digestion of non-source-separated organic fraction of municipal solid waste
- 1CIIMAR—Interdisciplinary Centre of Marine and Environmental Research, University of Porto, Porto, Portugal
- 2Chemistry and Biochemistry Department, Faculty of Sciences, University of Porto, Porto, Portugal
- 3Molecular Chemistry Institute of Reims, ICMR UMR 7312 CNRS, University of Reims Champagne-Ardenne, Reims, France
- 4Department of Mathematics and Applications Renato Caccioppoli, University of Naples Federico II, Napoli, Italy
- 5TratoLixo—Tratamento de Resíduos Sólidos, E.I.M. S.A., Trajouce, São Domingos de Rana, Portugal
- 6Biology Department, Faculty of Sciences, University of Porto, Porto, Portugal
Introduction: Digestate originating from anaerobic digestion of non-source-separated organic fraction of municipal solid waste (OFMSW) is produced abundantly worldwide and generally discarded in landfills. However, it can be a valuable resource for many bioeconomy strategies as land restoration, only if a consolidated understanding of the contaminants’ presence and behaviour in digestate-amended soil is achieved. This study aimed to investigate the fate of trace metals, namely Zn, Cu, Pb, and Cr found in the digestate, along the soil profile after digestate application on soil, and the influence that other contaminants as pharmaceutical compounds can have on their behaviour in the soil system.
Methods: For that, a 90-day soil column experiment was conducted using a fine loamy sand soil topped with a layer of digestate-amended soil. Digestate-amended soil had a soil to digestate proportion of 14 to 1 (dry weight). Two experimental conditions were tested: soil amended with digestate, and soil amended with digestate spiked with the antidiabetic drug metformin. Soil samples were taken at 4 depths on days 1, 7, 21, 35 and 90, and total trace metals concentrations and fractionation were determined via atomic absorption spectroscopy.
Results: Results showed that Zn, Cu, Pb ad Cr initially present in the digestate were transferred from the digestate-amended soil layer to the underlying soil layer over time, although in low amounts. Nevertheless, no transfer was detected to the deeper soil layers. Trace metals in soil were predominantly in immobile and less bioavailable forms associated with clay and silicate mineral groups, whereas in the digestate-amended soil they were in more bioavailable forms, which could be related to metals’ migration in the soil layers below. Results also show that the presence of metformin had no influence on trace metal behaviour, giving insight also on possible interactions with other potentially present contaminants as microplastics.
Conclusion: The current study showed that OFMSW digestate can be a promising organic nutrient-rich matrix for land restoration even if it may contain high metals’ concentrations because low metal mobility along the soil profile can be expected.
Introduction
Attention towards anaerobic digestion (AD) is growing as global demand for renewable energy and sustainable practices increases. Apart from biogas, the AD process produces also digestate (Fermoso et al., 2019) that is treated frequently as a waste. This waste is then discarded in landfills because it does not meet the quality standards imposed by legislations. However, digestate can be transformed into a resource for different industrial and agricultural processes strengthening local circular bioeconomies (Kaur et al., 2020). Digestate can be used directly or indirectly, for instance as soil amender, for element recovery, or for biomolecules production (Cesaro, 2021). Digestate is typically separated in two components, the liquid and the solid fraction, for more efficient resource use and management. Recently, for example, non-conventional strategies utilizing these two fractions have been developed such as microalgae biomass cultivation using the liquid fraction and production of biochar using the solid fraction for CO2 capture, nutrients and contaminants sorption (Thi Nguyen et al., 2019; Kisielewska et al., 2020, 2022; Qiao et al., 2020; Lee et al., 2021; Rodríguez Alberto et al., 2021; Liu et al., 2022). However, with increasing degradation of land and soil due to intensive agriculture and poor farming practices, digestate fractions have been re-evaluated also as a potential resource for sustainable bioenergy crop cultivation and land restoration (Saveyn and Eder, 2014). In fact, it is a semi-stabilized organic matrix containing macronutrients as nitrogen, phosphorous and potassium, and micronutrients as trace metals that can promote and restore soil quality (Peng and Pivato, 2019). Nevertheless, there are strict regulations at the EU level that define the permitted soil amendments originating from AD and their applications (European Parliament and Council, 2019). These legislations impose quality standards to ensure the safety and value of the digestate, and the protection of the environment in which they are applied (Logan and Visvanathan, 2019).
Digestate originating from municipal source-separated organic waste and agricultural organic waste are considered of higher quality compared to digestate originating from non-source-separated OFMSW, due to a possibly greater biotic and abiotic contamination level (Svensson et al., 2004; Ward et al., 2008; Makádi et al., 2012). OFMSW digestate is still generally classified as waste, but it can be transformed into a product after appropriate treatment providing the necessary quality standards to safeguard society, ecosystems, and the environment (European Parliament and Council of the European Union, 2008; European Parliament and Council, 2019). About 46 million tons out of 180 million tons of yearly produced digestate in the EU originate from non-source-separated OFMSW, while only 7 million originate from source-separated organic waste (Corden et al., 2019). Thus, it is important to further investigate the properties and potential applications of OFMSW digestate to support its transformation into a resource promoting its use as was done for compost, and digestate originated from sludge and other organic wastes (Logan and Visvanathan, 2019). A simple and feasible circular bioeconomy strategy involving OFMSW digestate (whole or solid fraction) is its application on marginal lands for soil restoration (Peng and Pivato, 2019) coupled with bioenergy crop cultivation. This strategy would allow to cultivate energy crops needed as feed material for AD reactors simultaneously remediating the soil and decreasing the competition between land for food and land for energy (Nabel et al., 2014; Pastorelli et al., 2021).
A concern regarding the use of OFMSW digestate is the presence of contaminants such as metals, inorganic and organic impurities (as plastics), micro-organic contaminants not degraded during AD process and greenhouse gases (Kupper et al., 2014; Fermoso et al., 2019). All these compounds can pose a threat to ecosystems and society. In fact, they can have a degree of toxicity; they can be degraded into by-products with even higher toxicity; they can leach to groundwater; they can accumulate in the environment and enter the food chain (Teglia et al., 2011). Most of these compounds are priority substances as metals (European Parliament and Council of the European Union, 2013), or contaminants of emerging concern (CEC) (Cortes et al., 2020) as pharmaceutical and personal care products (PPCPs) and microplastics (Pathan et al., 2020; Petersen and Hubbart, 2021).
Trace metals concentration in digestate varies according to the feed material and the addition of essential micronutrients (Fe, Ni, Co, Zn, Mo, Se, W) to sustain the efficiency of the AD process. Usually, metal concentrations are within legislation limits, but they may be higher due to waste content and its season variability (Kupper et al., 2014; Peng and Pivato, 2019). It is a well-known fact that high trace metal concentrations in soil pose an environmental and health risk (Dragicevic et al., 2017; Sogn et al., 2018; Azam et al., 2022) leading to loss of biodiversity and ecosystem functioning (Hattab-Hambli et al., 2020; Jia et al., 2022). For this reason, there are strict regulations that define maximum trace metals concentration at the EU level for all soil improvers and growing media (European Commission and Directorate-General for Environment, 2022).
Microplastics are plastic debris with <5 mm size that can enter the soil system through many pathways, prevalently the application of biosolids and sludges that intrinsically contain plastic particles (Mahon et al., 2017; Watteau et al., 2018). Microplastics may interact with other contaminants as metals and organic compounds through adsorption/desorption reactions contributing to pollutant transport and pollutant leaching (Davranche et al., 2019; Qi et al., 2020; Zhang et al., 2020; Chen et al., 2021). They may also cause disrupting effects of soil biogeochemical cycles and soil microbial community activities (De Souza MacHado et al., 2018; Wang et al., 2021). CEC are organic compounds that have raised concern in recent years (Verlicchi and Zambello, 2015), most of them having still poorly studied environmental risks. The concentration of CEC in digestate depends on the CEC content in the waste, the origin of the waste and the applied waste treatment technologies (Dubey et al., 2021). The AD process can successfully remove/degrade many CEC but is inefficient towards persistent and recalcitrant compounds as chemical fertilizers, flame-retardants and some PPCPs (Ali et al., 2019; Krzeminski et al., 2019; Dubey et al., 2021). Once introduced into the soil, these contaminants are subject to adsorption/desorption and transformation processes as a result of abiotic and biotic reactions with biodegradation being one of the most dominant (Wu et al., 2015). CEC also have ecotoxicological effects: they can be uptook by microorganisms and plants (parent compounds and transformation products), bioaccumulated and translocated through the soil’s macrofauna and up the trophic chain (Carter et al., 2014; Carter et al., 2014; Prosser et al., 2014; Wu et al., 2015).
It is critical to understand the behaviour of contaminants along the soil profile once digestate is applied to soil. Soil physico-chemical properties play a key role in contaminant distribution and in the interactions between contaminants and soil constituents (Fermoso et al., 2019). One of the main interaction processes was found to be sorption, including adsorption, absorption and desorption (Adriano, 2001; Kabata-Pendias and Pendias, 2001). Trace metals as Cd, Ni, Zn, Cu, Pb at low pH and redox-potential have shown to have higher solubility in the soil leading to higher mobility and bioavailability (Chuan et al., 1996; Bradl, 2004; Cipullo et al., 2018). Biosolid amendment can also induce a downward movement of trace metals in soil and their redistribution amongst soil fractions. In fact, it may increase trace metals bioavailability and their concentration in soil leachate due to complexation reactions between trace metals and dissolved organic carbon (DOC) (Zheljazkov and Warman, 2004; Gu and Bai, 2018). This phenomenon was found to be lower in amendments originating from OFMSW due to the decrease of DOC with organic matter mineralization (Fang et al., 2017) and an increased adsorption onto OM present in the soil (Kaschl et al., 2002; Weng et al., 2002; Shaheen et al., 2013). Combined with lignocellulosic waste, OFMSW digestate was shown to meet quality requirements as soil fertilizer, while mitigating the risk of metal leaching, immobilizing metals in soil and valorising nutrient content (Pecorini et al., 2020). It was also found to have presumably good agronomical characteristics and higher biomass yield when compared to mineral fertilizers (Tampio et al., 2016).
Some studies have been conducted on evaluating the possibility to use solid OFMSW digestate as a marginal soil amendment (Carabassa et al., 2020), but contradicting results have been found. Thus, the application of solid OFMSW digestate as soil amendment is a subject that deserves further research and understanding to elucidate the effects that it may have on contaminant transport in the soil system. To our knowledge there is no study that focuses on the potential contamination of soils with trace metals, persistent micro-organic contaminants and anthropogenic impurities (as microplastics) originating from the solid fraction of the OFMSW digestate, and their possible interactions and transformations within the soil system. As so, the aim of this study was to investigate the fate and mobility of trace metals, mainly Zn, Cu, Pb, Cr, in marginal land soils after application of solid OFMWS digestate contaminated with trace metals, metformin (proxy for micro-organic pollutants) and microplastics. It is hypothesis that metals present in the digestate could transfer to the soil and migrate through the soil profile affecting the environment, and that the presence of different types of contaminants could influence their mobility. Zn, Cu, Pb and Cr were selected because (i) they were present in the solid OFMSW digestate potentially posing an environmental risk after digestate application on soil; (ii) Zn and Cu are considered essential trace elements for plant growth that can also be toxic when present in high levels; and (iii) Pb and Cr are known as toxic but they can be uptook and accumulated by specific plant species (Dragicevic et al., 2017; Zeremski et al., 2021). The study’s results will give more insight on the effects that solid OFMSW digestate has on trace metal dynamics in the soil system, which will contribute to develop safe, simple and sustainable strategies to strengthen the circular bioeconomy centred around AD.
Materials and methods
Materials and reagents
All chemical compounds used in the study were of the highest available purity. The chemical compounds used were ethanol puriss. p. a. > 98% (603-002-00-5, Honeywell), analytical grade hydrogen peroxide >30% w/v (H/1800/15, Fischer Scientific), nitric acid puriss. p. a. 65% (84380-M, Sigma-Aldrich), acetic acid glacial 100% p. a. (100063, Merck), hydroxylamine hydrochloride ACS reagent 98.0% (255580, Sigma-Aldrich), ammonium acetate ACS reagent grade >97% (238074, Sigma-Aldrich) and metformin hydrochloride (PHR1084, Sigma-Aldrich). Metal stock standard solutions were also acquired from Sigma-Aldrich. Deionized water (conductivity <0.1 ms cm−1) was used throughout the experiments for solution preparation and dilutions. Before utilization, all standard laboratory glassware and plastic equipment was washed in a nitric acid bath [20% (v/v)] and thoroughly rinsed with deionised water.
Soil and OFMSW digestate
The soil was collected from an excavation site for building construction in a residential area in Ermesinde, located in the Porto city district (NW Portugal; 41°12’25.7’’N, 8°32’26.7’’W). Before being used in the experiments, the soil was homogenized and sieved to <2 mm. The soil texture was identified through INFOSOLO, the Portuguese online database for soil profile data (Ramos et al., 2017). Three soil profile data points were used to identify the soil texture around the sampling area (INFOSOLO soil profiles identification codes: 355V, 351V and 27762200). The soil was classified as a Cambisol deriving from metamorphic Schist parent rock. The soil texture was determined to be a fine loamy sand with a proportion of sand, silt, and clay of 78.7%, 14.8% and 6.5%, respectively (Supplementary Material S1, Supplementary Figure S1). Soil pH was on average 8.1 ± 0.5 and cation exchange capacity was 4.45 cmol+/kg. Initial water content was 11.9 ± 0.6 wt%, organic matter (OM) content was 3.02 ± 0.06 dw%, total carbon content was 0.40% with 0.20% being total organic carbon and 0.20% inorganic carbon. Furthermore, the predominant phases of the soil were found to be quartz and kaolinite, with minor phases of muscovite and calcite.
The solid OFMSW digestate (referred to as digestate from now on) was collected at Tratolixo, a Portuguese full-scale municipal solid waste treatment facility equipped with an AD unit for the treatment of the non-source-separated OFMSW (details of the process are reported in Supplementary Material S1). The solid fraction of the digestate was collected at the outlet of the centrifugation unit at the end of the solid-liquid separation process line. Digestate pH was on average 8.16 ± 0.05, dry matter content was 50 ± 6 wt% and OM content was 24 ± 4 dw%. Digestate mass density was equal to 1.06 g/cm3 and inert material content was 18.0 dw%.
The soil and digestate were characterized for trace metals’ total concentrations and trace metals fractionation which are reported in Results (paragraph Soil, Digestate and Digestate Amended Soil Characterization). In addition, elemental composition of soil and digestate was carried out and is presented in Supplementary Material S1 together with the quantification and characterization of microplastics found in soil and digestate.
Soil mesocosm column experiment
The experiment was a short-term experiment with a duration of 90 days. The experimental conditions defined were: (E1-Dig) soil amended with digestate and (E1-Dig-Met) soil amended with digestate spiked with metformin. For each experimental condition two columns were assembled, being conducted in duplicate. The soil column experiments were carried out in transparent acrylic plastic soil columns designed and constructed in the facilities of the Physics Department of the Faculty of Science of the University of Porto, Portugal. The columns, 60 cm in height and 19.4 cm in diameter, had 6 evenly spaced lateral sampling ports, and a bottom sampling port positioned at the centre of the column base (Figure 1) for leachate collection. Each column was assembled with three layers: (i) a 2 cm bottom layer of inert gravel (diameter <1 cm) to allow drainage and prevent clogging, (ii) a 37 cm middle layer of soil, and (iii) a 15 cm top layer of digestate amended soil. The digestate amended soil was prepared by thoroughly mixing the soil and the digestate for 30 min with a soil to digestate proportion of 14 to 1 (dry weight). This was done to simulate the scenario of marginal land digestate application followed by plowing and tillage operations for energy crop planting. The different materials were packed in the columns at 5 cm layers at a time, compacting them manually and scarifying their surface to ensure hydraulic conductivity (Lewis and Sjöstrom, 2010). For E1-Dig-Met, the digestate-amended soil was spiked with a 2 mg/L metformin aqueous solution (by thoroughly mixing it with the amended soil) before placing it on top of the soil of the soil column. The concentration chosen simulated metformin concentration found in digestates and AD sludges (Haiba et al., 2018; Briones and Sarmah, 2019). The columns were completely covered with aluminium foil to prevent sunlight exposure (with exception of the top base). They were kept in an indoor open-space with natural sunlight exposure at a constant ambient temperature of 22°C ± 1°C. The water content of the soil columns was kept constant at 80% water holding capacity by watering each column once a week with 200 ml of deionized water.
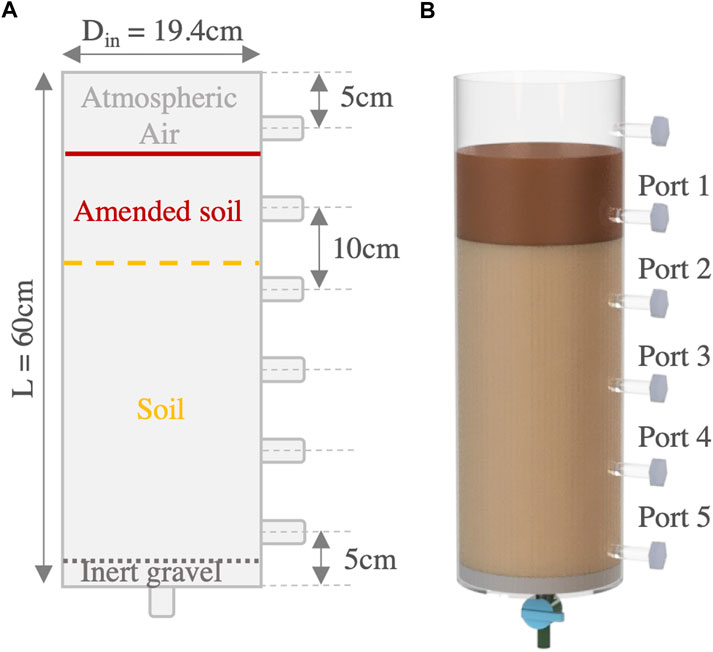
FIGURE 1. (A) Schematic of soil columns with column dimensions and packing layers; (B) 3D representation of soil column with indication of the digestate amended soil layer (dark brown) and the soil layers (light brown).
Soil samples were collected along the soil profile, through the column lateral sampling ports from 1 to 4 (Figure 1) on days 1, 7, 21, 35, and 90. The samples collected from each port were immediately stored at −20°C for sample preservation until further analysis. All solid samples were analysed for trace metals total and fractionation concentrations. During experiment duration, leachate from the base port of each column was also collected, acidified with HNO3 and analysed for total metal concentrations.
Analytical determinations
Water content was determined by heating solid samples at 105°C until constant weight was reached, and subsequently calculating the difference of mass between the initial sample and the dried one. Organic matter content was determined through Loss on Ignition (LOI) method by heating a 2 g dry solid sample in a muffle furnace at 500°C for 4 h, and then calculating the difference of mass between the initial sample and the dried one. The soil pH was measured following the ISO method for Soil quality—pH determination (NF ISO 10390). For all the mentioned parameters, analyses were carried out in triplicate for each sample.
Total metal concentrations (Fe, Mn, Ni, Zn, Cu, Pb, Cr, Cd and Co) in the solid samples were analysed by atomic absorption spectroscopy (AAS), after a high-pressure digestion in an advanced microwave system (ETHOS 1, Milestone Inc.). The method applied followed a laboratory procedure previously optimised and validated with certified reference materials (Estuarine Sediment BCR 277, certified for total metal content), as described in Almeida et al. (2004). Acceptable results were obtained in all cases. For soil samples, 0.25 g of sample was weighted into a microwave Teflon vessel and 5 ml HNO3 were added; while for digestate and digestate-amended soil samples, 0.50 g of sample was weighed and 1 ml of HNO3 along with 5 ml of H2O2 were added. After the microwave digestion, the vessels were allowed to cool down to room temperature; the solutions were transferred into 50 ml falcon tubes and volumes were made up to 15 ml with deionized water.
The solutions were analysed either via AAS with flame atomisation (AAS-F), using an AAnalysit 200 AA spectrometer system (PerkinElmer), or via AAS with electrothermal atomisation (AAS-ET), using a PinAAcle 900Z AA spectrometer (PerkinElemer), depending on metal concentration values and instrument operation rage. The concentrations were obtained by external calibrations with aqueous metal standard solutions conducted prior to each analysis. Acidified leachate samples were analysed directly either with AAS-F or AAS-ET. Analytical methodology was also previously optimised and validated with the mention certified reference material as described in Almeida et al. (2004). Blank solutions were prepared similarly to samples, with no significant signal being detected. AAS precision was lower than 5% for triplicate measurements. The analytical quality parameters for AAS-F and AAS-ET methods are reported in Supplementary Table S2 (Supplementary Material S1).
Initial samples were analysed in triplicate, whereas only one replicate was analysed for column samples.
For metal fractionation, the sequential extraction BCR procedure described in Rauret et al. (1999) was used with few minor modifications. Briefly, 0.50 g of each sample was weighed and placed into 50 ml falcon tubes. The first extraction was carried out with 20 ml of a 0.11 mol/L acetic acid solution for the determination of the soluble and exchangeable metal fraction. The second sequential extraction was carried out with 20 ml of a 0.5 mol/L hydroxylamine hydrochloride solution for the determination of the reducible iron/manganese oxides fraction. The third sequential extraction, for the determination of the oxidizable organic matter and sulphide fraction, was carried out with a 1 mol/L ammonium acetate solution after sample oxidation with hydrogen peroxide aqueous solution 30% (v/v). The extractions were carried out in an end-over-end shaker (Unitronic Reciprocating Shaking Bat, JP Selecta) at room temperature. Metal concentrations in the extract solutions were determined by AAS-F or AAS-ET as described above. The methodology was previously optimised and validated in the laboratory using the certified reference material BCR 701, certified for the extractable metal content, as described in Almeida et al. (2004). Acceptable results were obtained in all cases. Blank solutions were prepared and analysed as samples, with no significant signal being detected.
Fractionation of Fe, Mn, Zn, Cu, Pb and Cr for initial soil, digestate and digestate-amended soil samples was carried out in triplicate; whereas the fractionation for soil column samples was carried out only for Zn, Cu, Pb and Cr with one replicate per sample.
Data analysis
For each initial solid sample, the metals total and fractionation concentrations were represented as the mean and standard deviation of the three replicates. For samples collected during the soil column experiment, mean and error were calculated with values of replicate columns. Statistical analyses were performed using RStudio, version 1.4.1103 (R Core Team, 2021). Data collected from the soil column experiment was analysed via two-way ANOVA statistical analysis to evaluate significant differences in trace metals’ total and fractionation concentrations through time and between experimental conditions. Trace metals’ concentrations were defined as the dependent variable, while time and experimental conditions as the independent variables. The significant results (p-value <0.05) were further assessed by pairwise comparison with post hoc Tukey’s Honestly Significant-Difference (THSD) test to understand which groups were significantly different from one another.
Results
Soil, digestate and digestate amended soil characterization
The initial soil, digestate and digestate-amended soil Fe, Mn, Ni, Zn, Pb, Cu, Cr, Co, and Cd concentrations are reported in Figure 2. The results showed that in general the trace metals’ concentrations were higher in the digestate than in the soil. The concentrations of Mn, Zn, Cu and Pb in the digestate were significantly higher than those in the soil, potentially posing a risk of metal transport along the soil profile. These high concentrations are probably due to the origin of the digestate which derives from non-source-separated OFMSW. Cr and Ni concentrations were also slightly higher in digestate than in soil, but lower than those of the other mentioned trace metals. Only Fe, considered as a major element, had a higher concentration in the soil due to its mineral origin. Concentrations for Co were low in both soil and digestate, while Cd concentrations were below the limit of detection (5.8 μg/g) in all samples analysed. Thus, these two metals were not considered and analysed in the soil column experiments. The trace metals’ concentrations in the digestate-amended soil showed the dilution effect that the soil had on the digestate, although some trace metals’ concentrations (Zn, Cu and Pb) continued to be more than double of those found in the soil. This difference in trace metals’ concentrations between soil and digestate-amended soil led to the use of digestate with no addition of trace metals, allowing to simulate a real case scenario of digestate application onto soil.
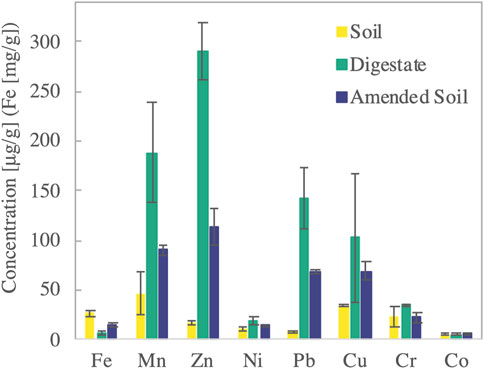
FIGURE 2. Total trace metals concentrations in initial soil (yellow), digestate (green), and digestate amended soil (blue) from the soil column experiment (mean ± standard deviation, n = 2).
The soil, digestate and digestate amended soil metal fractionation analysis allowed to evaluate trace metals’ concentrations within different operationally defined chemical forms of the solid samples: the exchangeable, the reducible (bound to Fe and Mn oxides), the oxidizable (bound to organic) and the residual fraction. It was decided to combine the oxidizable and residual fractions because the quantity of trace metals in the oxidizable fraction (bound to organic matter) was generally very low compared to the quantity present in the other fractions (Supplementary Material S1, Supplementary Figure S2). The fractionation results are reported in Figures 3A–C as percentage of the total trace metal concentration measured. The results for soil showed that Fe, Pb, Cu and Cr were found mainly in the residual + oxidizable fraction at 99%, 93%, 95%, and 98%, respectively. Zn and Mn were found in all fractions with Zn mainly in the residual + oxidizable (50%) and the exchangeable (30%) fraction, and Mn mostly in the reducible fraction (81%). The digestate showed similar results with Pb, Cu, Cr and Zn found mainly in the residual + oxidizable fraction at 99, 90, 99, and 58%, respectively. Fe was mostly found in the reducible (55%) and in the residual fraction (39%); whereas Mn was found in all fractions with 30% being in the exchangeable fraction, 22% in the reducible fraction, and 47% in the residual fraction. In the digestate-amended soil Pb, Cu and Cr were found mostly in the residual + oxidizable fraction at 98, 81% and 91%, respectively. Cu and Cr were also found in a small percentage in the reducible fraction. Furthermore, Fe, Mn and Zn were found in all fractions: in the residual + oxidizable (52, 27% and 49%) fraction, bound to Fe/Mn oxides (12, 29% and 30%), and in the exchangeable fraction (35, 43% and 21%). The distribution of trace metals in the digestate-amended soil was influenced by the mixing between the soil and the digestate. Overall, it can be estimated that the initial substrates contained Pb, Cu and Cr mainly in immobile forms, and Zn in both readily bioavailable and immobile forms. As previously mentioned, trace metal fractionation was not carried out for Co, Cd and Ni as their total concentrations were lower than the limit of detect or were too low to determine any significant variation.
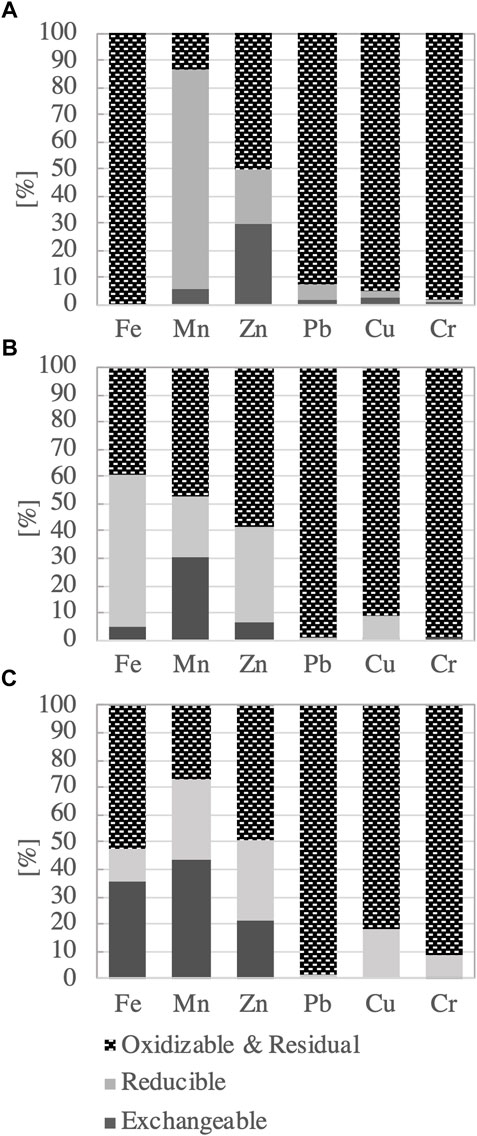
FIGURE 3. Trace metals fractionation in initial soil (A), digestate (B) and digestate amended soil (C) from the soil column experiment (mean ± standard deviation, n = 2).
Soil column experiments: pH and leachate trace metals concentrations
pH of the different soil column layers was measured at the beginning (day 1) and at the end of the experiment (day 90). The average pH of the soil layers over time varied between 8.1 ± 0.2 (on day 1) and 8.4 ± 0.1 (on day 90), indicating a slight non-significant increase. The average pH of the digestate-amended soil layer over time varied between 9.1 ± 0.5 (on day 1) and 8.2 ± 0.7 (on day 90), indicating a slight decrease.
Regarding soil leachate samples, no trace metals were detected or quantified in the collected leachate solutions.
Soil column experiments: Total trace metal concentrations
Total Zn, Pb, Cu and Cr concentrations along the column profile throughout the experiment duration for both experimental conditions (E1-Dig and E1-Dig-Met) are reported in Figure 4. Total Fe, Mn and Ni concentrations are reported in Supplementary Material S1 (Supplementary Figure S3). For both experimental conditions, metal concentrations measured in the deeper soil layers (port 3 and 4, Figure 1B) were in the same range of those of the initial soil, indicating that metals from the digestate-amended soil layer (port 1, Figure 1B) were not able to reach the deeper soil layers throughout the experiment duration. Additionally, trace metals’ concentrations overall had a decreasing trend in the first layer (port 1, Figure 1B) and an increasing trend in the second layer (port 2, Figure 1B), except for Fe which was present in higher quantity in the soil and Cr (Figures 4G, H) whose concentrations in the soil (24 ± 10 μg/g) and in the digestate-amended soil 23 ± 5 μg/g were statistically identical. Moreover, no statistically significant difference between the trend of total trace metals’ concentrations in the soil columns of the two experimental conditions (E1-Dig and E1-Dig-Met) were observed.
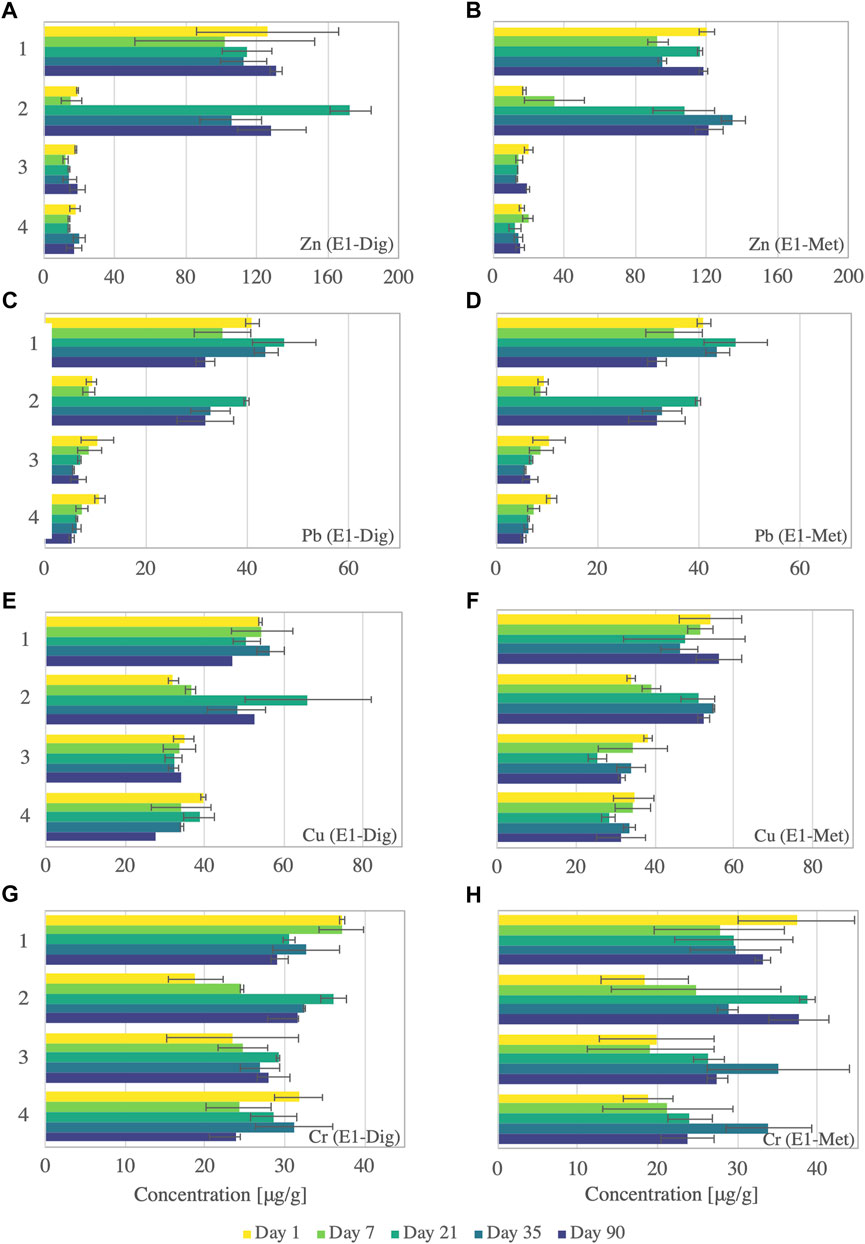
FIGURE 4. Zn, Pb, Cu and Cr total concentrations in the soil of the four column layers (ports 1, 2, 3 and 4) collected throughout time, for the two experimental conditions: E1-Dig [soil amended with digestate (A,C,E,G)] and E1-Dig-Met [soil amended with digestate doped with metformin (B,D,F,H)] (mean ± standard deviation, n = 2).
The results of the statistical analysis (independence of variables, normality, and homoscedasticity tested and verified) are reported in Supplementary Material S1 (Supplementary Table S4). The statistical analysis evaluated the effect of time and experimental condition (presence/absence of metformin) on the total trace metals’ concentrations along the soil column profile. The statistical analysis was carried out only for Zn, Cu, Pb and Cr as these were considered trace metals of concern due to their concentrations in the digestate, that could lead to ecotoxicological effects in the soil system. The analysis was not conducted for Fe and Mn because they were considered major elements commonly present in soils; while Ni was found in similar concentrations in both soil and digestate-amended soil not allowing to assess its behaviour through the soil column profile. Overall, the statistical analysis revealed that no statistically significant interaction between the effects of time and those of experimental condition occurred, with exception for Zn concentration in the second column layer (port 2 Figure 1B).
The simple main effects analysis showed that time had a statistically significant effect on total trace metals’ concentrations, especially on those in the second soil column layer. For experimental condition E1-Dig, the concentration of Zn in the second layer on days 1 and 7 was significantly lower than the concentration of Zn on days 21, 35 and 90, with a similar (non-significant) trend being observed for condition E1-Dig-Met. The variation of Cu concentration in the second column layer was significant only for experimental condition E1-Dig-Met, which showed concentrations on days 1 and 7 significantly lower than those on days 21, 35 and 90. This trend (not statistically significant) was observed also for condition E1-Dig. Similarly, Pb in the second column layer on day 1 and day 7 was significantly lower than on days 21, 35 and 90 for experimental condition E1-Dig. Pb, in condition E1-Dig-Met, in the second column layer on day 1 was significantly lower than on day 21, while Pb in the fourth layer (port 4, deepest) on day 1 was significantly higher than on days 21, 35 and 90. Lastly, Cr in the second column layer on day 1 and 7 for condition E1-Dig were significantly lower than Cr on days 21, 35 and 90. In summary, these results showed that time had an effect on the variation of trace metals’ concentration in the soil profile: a significant increase in concentrations was determined in the second soil column layer on day 21 of the experiment (week 3). In addition, the simple main effects analysis showed that experimental condition (presence/absence of metformin) did not have a statistically significant effect on the variation of total trace metals’ concentrations along the soil profile throughout experiment duration.
Soil column experiments: Trace metals fractionation
Trace metals’ fractionation along the soil column profile over time (90 days) are reported in Figures 5, 6 for experimental condition E1-Dig and E1-Dig-Met, respectively. Trace metals’ fractionation was carried out only for Zn, Cu, Pb and Cr as mentioned above. As previously case, the oxidizable and residual fractions are represented together as the metals bound to the organic fraction were in very low quantities compared to those found in the other fractions. Trace metals’ fractionation showed that Zn was mostly found in an immobile form (residual + oxidizable fraction) in the lower soil layers (ports 3 and 4), while it was distributed amongst all fractions in the first and second layer (ports 1 and 2). Cr, Cu and Pb were mostly found in an immobile form in all the soil column layers with a small percentage also being found in the reducible fraction (between 5%–20%) and exchangeable fraction (<5%). Over time, the exchangeable and reducible fractions of Zn were subject to some variation resulting in a slight increase in the more bioavailable fractions and a consequent decrease in the residual fraction. Similarly, the quantities of Pb and Cu within the different fractions varied over time, but no clear trend was observed. On the other hand, the quantity of Cr within the different fractions showed no variation, remaining prevalently in the residual fraction over time. Additionally, no evident difference between E1-Dig and E1-Dig-Met was observed.
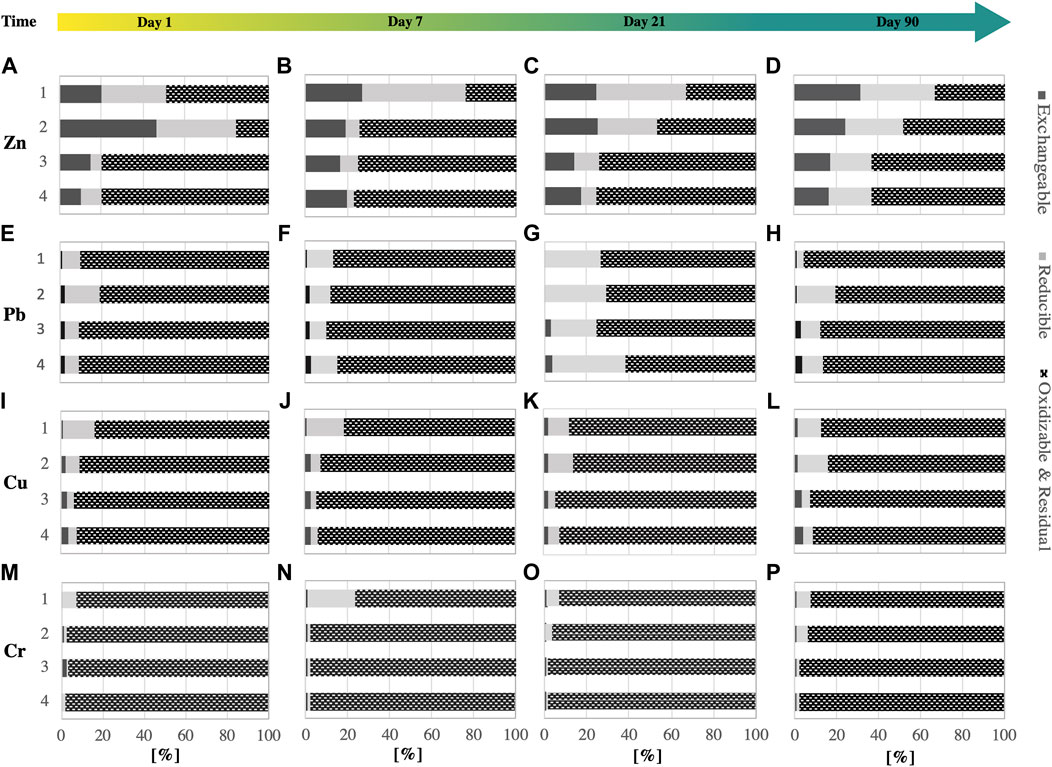
FIGURE 5. Zn (A–D), Pb (E–H), Cu (I–L), and Cr (M–P) fractionation in the soil of the four column layers collected over time for experimental condition E1-Dig (soil amended with digestate) (mean ± standard deviation, n = 2).
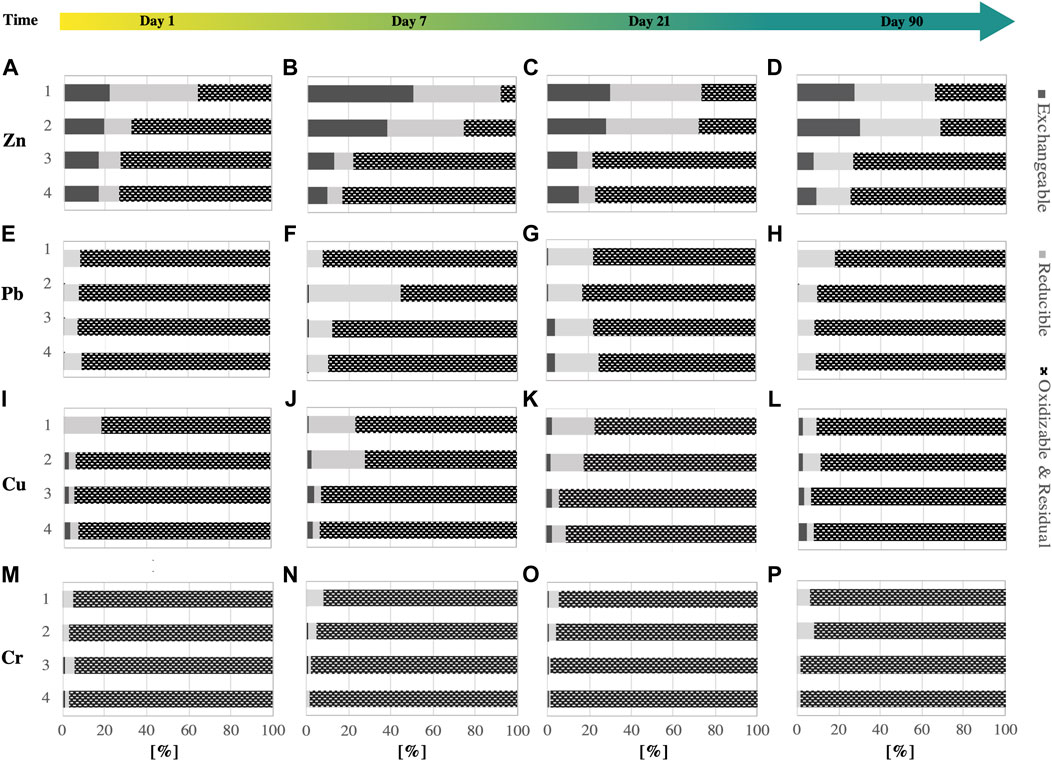
FIGURE 6. Zn (A–D), Pb (E–H), Cu (I–L), and Cr (M–P) fractionation in the soil of the four column layers collected over time for experimental condition E1-Dig-Met (soil amended with digestate doped with metformin) (mean ± standard deviation, n = 2).
The statistical analysis on the effect of time and experimental condition (presence/absence of metformin) on trace metals’ fractionation are reported in Supplementary Material S1 (Supplementary Table S5). Overall, the analysis revealed that there was no statistically significant interaction between the effect of time and experimental condition on trace metal fractionation in the soil column. The only exception was encountered for Zn in the fourth layer, where both time and experimental condition resulted having a significant effect on the exchangeable and oxidizable-residual fractions. The simple main effects analyses showed that both time and experimental condition had a significant effect on trace metals’ fractionation for specific trace metals and in specific soil column layers.
Specifically, the exchangeable fraction of Zn in the second layer on day 1 for experimental condition E1-Dig-Met was significantly lower than that measured on days 21 and 90. Also, the oxidizable-residual fraction of Zn in the fourth layer on day 90 for condition E1-Dig was statistically lower than that measured on all other days.
Cu fractionation varied significantly in the first and second soil column layer only for condition E1-Dig-Met. In fact, in the first layer, the analysis determined that the exchangeable fraction of Cu measured on day 1 and 7 was significantly lower than that of day 21; Cu reducible fraction measured on day 7 and 21 was significantly greater than that of day 90; and Cu oxidizable-residual fraction increased significantly from day 21 to day 90. While, in the fourth layer, the reducible and oxidizable-residual fractions of Cu measured on day 1 were significantly different compared to those measured on day 21 showing an increase and decrease, respectively.
For Pb, the analysis determined that its fractionation varied significantly in all column layers throughout time. In the first layer, the simple effect analysis showed that experimental condition had a significant effect on the reducible fraction measured on day 90: experimental condition E1-Dig-Met had greater quantity of Pb bound to Fe/Mn oxides compared to experimental condition E1-Dig. In the second column layer of condition E1-Dig, the exchangeable fraction measured on day 21 and 90 was significantly greater than that of day 1 and 7. In third layer, neither time nor experimental condition had a significant effect on Pb fractionation. In the fourth layer of condition E1-Dig-Met, the reducible and oxidizable-residual fractions measured on day 1 and 7 were significantly greater than those of day 21; while the reducible and oxidizable-residual fractions measured on day 90 showed a significant increase and decrease compared to those of day 21, respectively.
Cr fractionation varied significantly only in the second layer of experimental condition E1-Dig: the exchangeable fraction measured on day 90 significantly decreased compared to that of day 1; the reducible fraction measured on day 90 significantly increased compared to that of day 1 and 7; and the oxidizable-residual fraction measured on day 90 significantly decreased compared to that of day 1, 7 and 21.
Discussion
First and foremost, all trace metals’ concentrations measured in the digestate resulted below the maximum metal concentration limits defined by EU legislation (European Parliament and Council, 2019), with exception of Pb (measured concentration = 142 mg/kgdw and limit concentration = 120 mg/kgdw). Even so, trace metals’ concentrations were more than double of those measured in the selected soil, allowing to simulate a real-case scenario of solid OFMSW digestate application onto marginal land soil with no addition of trace metals. The digestate application onto the selected soil (fine loamy sand) led to an alkaline pH throughout the entire soil column profile with values ranging from 8.3 to 8.5. Metal sorption, complexation, precipitation and other reaction mechanisms governing metal mobility in the soil system are highly dependent on pH: in fact, as pH becomes more alkaline the retention of metal cations increases due to an increase of the global negative soil surface charge (Shaheen et al., 2013). Generally, Pb exhibited the strongest adsorption interactions, followed by Zn, Cu, and Cd, while Ni presented the weakest (Teglia et al., 2011).
Overall, the soil mesocosm column experiment results showed that total trace metals’ concentrations increased in the soil layer beneath the digestate-amended one (top layer), during the 90-day experiment. This result indicated a downward movement of trace metals from the first soil column layer to the second one throughout time. This behaviour could be attributed either to a seepage of digestate from the top layer to the underlying one, or to the soil solution and colloidal particle transport (convective and diffusive transport) as was observed by Gu and Bai (2018). As illustrated in Figure 4, no movement of trace metals was observed within the deeper soil column layers most likely for the trace metals’ forms present.
Trace metals fractionation analysis in the deeper soil column layers showed that the trace metals were mainly found in the residual fraction (including also the oxidizable fraction which was considered negligible). This suggests that the trace metals were not subject to a redistribution amongst the different defined fractions through time, remaining in an immobile form. This can be attributed to the strong bounds generated between the metals and the soil’s mineral groups as kaolinite (clay mineral) and muscovite (silicate mineral). In fact, clay and silicate minerals not only favour the formation of high microporosities in the soil matrix causing greater compound diffusion, but also enhance the adsorption of metallic cations due to their negative surface charge (Adriano, 2001).
Specifically, the fractionation results of this study determined that Zn proportion in the deeper soil layers followed the order: residual > exchangeable > reducible fraction, which changed over time resulting in the reducible fraction having a greater proportion than the exchangeable fraction by day 90. The proportion of Cu throughout experiment time followed the order: residual > reducible > exchangeable fraction. Cu is known to have a high affinity towards stable soil organic matter (Clemente et al., 2006). Even though the soil used in the experiments had an OM content of 3.02% dw, the percentage found in the oxidizable fraction for all metals was very low (<4%) and considered negligible compared to the residual fraction. The proportion of Pb followed the same order as the proportion of Cu: residual > reducible > exchangeable fraction, with a slight increase of the reducible fraction over time.
The results for Pb are in accordance with those reported in other studies, observing the high affinity of Pb to Fe-, Mn-, Al-oxides and clay mineral groups (Ryan et al., 2008). All these compounds are present in high amounts in the studied soil (Supplementary Material S1, Supplementary Table S1), leading to Pb low mobility and bioavailability (Gu and Bai, 2018). In contrast, Cr was found primarily in the residual fractions during the experiment, with the exchangeable and reducible fractions being less than 1% and 5%, respectively. This could be attributed to the fast sorption kinetics in alkaline soils predominantly governed by precipitation of Cr hydroxide onto the soil (Xu et al., 2020).
Trace metals fractionation of the first and second soil column layer showed that trace metals were distributed amongst all the defined fractions. This is in contrast with what was determined for the deep soil column layers, as discussed above. In fact, the proportion of Zn was on average reducible = residual > exchangeable. This is in agreement with previous studies, focusing on source-separated OFMSW digestate amendments, that observed greater quantities of Zn bound to Fe/Mn oxides in the amended soils (Zheljazkov and Warman, 2004; Rossi and Beni, 2018). These interactions are favoured by the affinity between Zn and Fe/Mn oxides which grows with increasing pH (Shuman, 1999). Nevertheless, this is in contradiction with the study showing Zn as the least mobile and bioavailable metal in mudflats amended with sludge (Gu and Bai, 2018). Pb, Cu and Cr fractionation in the first and second column layers followed the same proportion order of the deep soil layers with slightly greater quantity of trace metals found in the reducible fraction. As mentioned above, this is most likely attributed to trace metals’ high affinity towards silicate and clay mineral groups, metal oxides and stable organic matter leading to their low bioavailability (Bradl, 2004). This agrees with another study that determined the lower mobility of Cu, Ni, and Zn originating from digestate compared to those originating from animal manure or mineral fertilizers (Dragicevic et al., 2017). This shows that, in certain conditions, digestate can have a lower environmental impact than traditional accepted soil amendments/fertilizers, resulting in leachate metal concentrations lower than those imposed by legislation. As to leachate solutions, this study showed that no metal was detected in leachate samples collected during the entire experiment.
Additionally, attention must be given to anthropogenic impurities (inert materials as small DIY debris, glass, aluminium, and plastics) present in the non-source-separated OFMSW digestate because they may increase the sorption surface for metals contributing to the increase of the proportion of metals found in the oxidizable-residual fraction. In the present study, a considerable number of microplastics were found in the digestate used (data in Supplementary Material S1, Supplementary Table S3). Recent studies have shown that the presence of microplastics in the soil can affect the soil’s metal adsorption capacity by increasing metal desorption and mobility as a consequence of the weak attraction forces between microplastics and metals easily altered by environmental condition changes (Zhang et al., 2020; Wang et al., 2021). We can hypothesise that part of the trace metals found in the exchangeable fraction of the digestate-amended soil originates from microplastics. This could lead to an increased environmental risk for metal transfer and leaching due to the microplastics’ metal desorption behaviour influenced by environmental conditions, and the transport of plastic-metal complexes within the soil-groundwater system (Wang et al., 2021).
Organic contaminants, as CEC, are also compounds that may affect the behaviour and the mobility of trace metals in the digestate-amended soil and in the soil profile. Even though the soil column experiments determined that metformin (pharmaceutical compound selected as proxy for polar organic contaminants), did not have a statistically significant effect on the mobility of trace metals during the experiment, it cannot be concluded that the presence of metformin did not affect metal speciation and metal behaviour with soil constituents. In fact, in the presence of organic compounds trace metals may undergo complexation, forming coordination complexes that modify their speciation and their behaviour in the soil system (Sayen et al., 2019). The formation of complexes may lead to an increase or decrease in the transfer of contaminants within the soil system, posing additional environmental risks.
In general, this study showed that metal mobility and transfer along the soil column profile was most likely due to (i) the application of digestate amendment on the top of the column that promoted ad/desorption mechanisms in the digestated-amended soil layer, and (ii) the alkaline nature of the loamy sand soil which favoured specific bounding and interaction mechanisms.
This study was conducted over a short time period (90-day) looking at the immediate response of digestate amendment application on soil; however, it would be important to conduct a similar experiment over a longer time period to study the effects of metal aging in the digestate-amended soil. In addition, a more in-depth analysis should be conducted on the influence of emerging micro-organic contaminants on metal sorption mechanisms in the soil system. While this study looked at the macroscopic effect of a specific pharmaceutical compound (metformin) on trace metal behaviour along the soil profile through time, it is important to conduct a study at the microscopic scale to evaluate the formation of coordination complexes and the influence they may have on soil sorption and transfer. More research should also be conducted on microplastics in the soil system to understand their role in soil contaminant transfer and their effects on soil ecosystems. This is important especially due to their increasing environmental concentrations, and the lack of knowledge on their behaviour and toxicity.
Conclusion
In this study, the fate of trace metals in marginal land soil after application of solid biogas digestate, originating from non-source separated OFMSW, was assessed. This work showed that total metal concentrations increased in the soil layer directly beneath the digestate amended-soil layer (top layer) throughout the experiment period, indicating a downward movement of the metals through the first column layers. Nevertheless, the metals were not able to reach the deeper soil layers most likely due to their speciation. In fact, metals fractionation showed that over time, in the deeper soil layers, trace metals were mainly found in the residual fraction, suggesting that they were not subject to redistribution amongst the other operationally defined fractions due to the strong bounds between metals and soil mineral groups (especially silicates and clays). On the other hand, in the first few layers, trace metals were found significantly also in the exchangeable and the reducible (bound to Fe/Mn oxides) fractions, suggesting a greater bioavailability and possible metal mobility through the soil profile, which may also increase due to the presence and interaction with micro-organic pollutants and anthropogenic impurities present in the digestate amended soil. Overall, the results showed a potential for the application of non-source-separated OFMSW digestate as marginal-land amendment due to the high metal sorption and low mobility of trace metals over time. Thus, this application would allow to increase marginal land soil quality through the supply of stable organic matter and nutrients, which coupled with the cultivation of energy crops would allow to further immobilize and accumulate the mobile and bioavailable fractions of metals present in the soil.
Data availability statement
The raw data supporting the conclusion of this article will be made available by the authors, without undue reservation.
Author contributions
VB: Conceptualization, methodology, investigation, data collection, formal analysis (data interpretation and analysis), writing—original draft. NBG: Investigation, data collection. SS: Conceptualization, writing—review and editing. EG: Conceptualization, writing—review and editing. LF: Conceptualization, writing—review and editing, funding acquisition. CRG: Conceptualization, methodology, writing—review and editing, Supervision. MA: Methodology, data information. RC; Methodology, data information. APM: Conceptualization, methodology, writing—review and editing, supervision, funding acquisition. CMRA: Conceptualization, methodology, writing—review and editing, supervision, funding acquisition. All authors have given approval to the final version of the manuscript.
Funding
This project has received funding from the European Union’s Horizon 2020 research and innovation programme under the Marie Skłodowska-Curie grant agreement No. 861088.
Acknowledgments
The authors would like to thank E2Lim laboratory of the University of Limoges (France) for the soil and digestate chemical characterization; the physics department of the Faculty of Science of the University of Porto (Portugal) for the manufacturing of the soil column systems; Mafalda Flores from Faculty of Science of the University of Porto for her assistance during AAS analysis. FCT is acknowledge by the Strategic Funding UIDB/04423/2020 and UIDP/04423/2020 of CIIMAR.
Conflict of interest
Authors MJA and RC were employed by company TratoLixo—Tratamento de Resíduos Sólidos, E.I.M. S.A.
The remaining authors declare that the research was conducted in the absence of any commercial or financial relationships that could be construed as a potential conflict of interest.
Publisher’s note
All claims expressed in this article are solely those of the authors and do not necessarily represent those of their affiliated organizations, or those of the publisher, the editors and the reviewers. Any product that may be evaluated in this article, or claim that may be made by its manufacturer, is not guaranteed or endorsed by the publisher.
Supplementary material
The Supplementary Material for this article can be found online at: https://www.frontiersin.org/articles/10.3389/fenvs.2022.1007390/full#supplementary-material
References
Adriano, D. C. (2001). “Biogeochemical processes regulating metal behavior,” in Trace elements in terrestrial environments: Biogeochemistry, bioavailability, and risks of metals (New York, NY: Springer New York), 29–59. doi:10.1007/978-0-387-21510-5_2
Ali, A. M., Nesse, A. S., Eich-Greatorex, S., Sogn, T. A., Aanrud, S. G., Aasen Bunæs, J. A., et al. (2019). Organic contaminants of emerging concern in Norwegian digestates from biogas production. Environ. Sci. Process. Impacts 21 (9), 1498–1508. doi:10.1039/c9em00175a
Almeida, C. M. R., Mucha, A. P., and Vasconcelos, M. T. S. D. (2004). Influence of the Sea rush juncus maritimus on metal concentration and speciation in estuarine sediment colonized by the plant. Environ. Sci. Technol.38, 3112–3118. doi:10.1021/es049932j
Azam, M., Khan, M. R., Wabaidur, S. M., Al-Resayes, S. I., and Islam, M. S. (2022). Date pits waste as a solid phase extraction sorbent for the analysis of lead in wastewater and for use in manufacturing brick: An eco-friendly waste management approach. J. Saudi Chem. Soc. 26, 5. doi:10.1016/j.jscs.2022.101519
Bradl, H. B. (2004). Adsorption of heavy metal ions on soils and soils constituents. J. Colloid Interface Sci. 277 (1), 1–18. doi:10.1016/j.jcis.2004.04.005
Briones, R. M., and Sarmah, A. K. (2019). Sorption and mobility of metformin and guanylurea in soils as affected by biosolid amendment: Batch and column tests. Environ. Pollut. 244, 19–27. doi:10.1016/j.envpol.2018.10.025
Carabassa, V., Domene, X., and Alcañiz, J. M. (2020). Soil restoration using compost-like-outputs and digestates from non-source-separated urban waste as organic amendments: Limitations and opportunities. J. Environ. Manag. 255, 109909. doi:10.1016/j.jenvman.2019.109909
Carter, L. J., Garman, C. D., Ryan, J., Dowle, A., Bergstrom, E., Thomas-Oates, J., et al. (2014). Fate and uptake of pharmaceuticals in soil-earthworm systems. Environ. Sci. Technol. 48 (10), 5955–5963. doi:10.1021/es500567w
Cesaro, A. (2021). The valorization of the anaerobic digestate from the organic fractions of municipal solid waste: Challenges and perspectives. J. Environ. Manag. 280, 111742. doi:10.1016/j.jenvman.2020.111742
Chen, X., Gu, X., Bao, L., Ma, S., and Mu, Y. (2021). Comparison of adsorption and desorption of triclosan between microplastics and soil particles. Chemosphere 263, 127947. doi:10.1016/j.chemosphere.2020.127947
Chuan, M. C., Shu, G. Y., and Liu, J. C. (1996). Solubility of heavy metals in a contaminated soil: Effects of redox potential and pH. Water, Air, Soil Pollut. 90 (3–4), 543–556. doi:10.1007/BF00282668
Cipullo, S., Snapir, B., Tardif, S., Campo, P., Prpich, G., and Coulon, F. (2018). Insights into mixed contaminants interactions and its implication for heavy metals and metalloids mobility, bioavailability and risk assessment. Sci. Total Environ. 645, 662–673. doi:10.1016/j.scitotenv.2018.07.179
Clemente, R., Escolar, Á., and Bernal, M. P. (2006). Heavy metals fractionation and organic matter mineralisation in contaminated calcareous soil amended with organic materials. Bioresour. Technol. 97 (15), 1894–1901. doi:10.1016/j.biortech.2005.08.018
Corden, C., Bougas, K., Cunningham, E., Tyrer, D., KreiBig, J., Zetti, E., et al. (2019). Digestate and compost as fertilisers: Risk assessment and risk management options. Wood Environment & Infrastructure Solutions UK Limited.
Cortes, L. G., Marinov, D., Sanseverino, I., Cuenca, A. N., Niegowska, M., Porcel, E., et al. (2020) Selection of substances for the 3rd watch list under the water framework directive. JRC Technical Report. doi:10.2760/194067
Davranche, M., Veclin, C., Pierson-Wickmann, A. C., El Hadri, H., Grassl, B., Rowenczyk, L., et al. (2019). Are nanoplastics able to bind significant amount of metals? The lead example. Environ. Pollut. 249, 940–948. doi:10.1016/j.envpol.2019.03.087
De Souza MacHado, A. A., Lau, C. W., Till, J., Kloas, W., Lehmann, A., Becker, R., et al. (2018). Impacts of microplastics on the soil biophysical environment. Environ. Sci. Technol. 52 (17), 9656–9665. doi:10.1021/acs.est.8b02212
Dragicevic, I., Eich-Greatorex, S., Sogn, T. A., Linjordet, R., and Krogstad, T. (2017). Fate of copper, nickel and zinc after biogas digestate application to three different soil types. Environ. Sci. Pollut. Res. 24 (14), 13095–13106. doi:10.1007/s11356-017-8886-8
Dubey, M., Mohapatra, S., Tyagi, V. K., Suthar, S., and Kazmi, A. A. (2021). Occurrence, fate, and persistence of emerging micropollutants in sewage sludge treatment. Environ. Pollut. 273, 116515. doi:10.1016/j.envpol.2021.116515
European Commission and Directorate-General for Environment (2022). Commission Decision (EU) 2022/1244 of 13 July 2022 establishing the EU Ecolabel criteria for growing media and soil improvers. Available at: http://data.europa.eu/eli/dec/2022/1244/oj (Accessed: December 2, 2022).
European Parliament and Council of the European Union (2008). Directive 2008/98/EC of the European Parliament and of the Council of 19 November 2008 on waste and repealing certain Directives (Text with EEA relevance). Official J. Eur. Union L 312, 3–30. Available at: http://data.europa.eu/eli/dir/2008/98/oj (Accessed July 11, 2022).
European Parliament and Council of the European Union (2013). Directive 2013/39/EU of the European Parliament and of the Council of 12 August 2013 amending Directives 2000/60/EC and 2008/105/EC as regards priority substances in the field of water policy (text with EEA relevance). Official J. Eur. Union L 226, 1–17. Available at: http://data.europa.eu/eli/dir/2013/39/oj (Accessed July 05, 2022).
European Parliament and Council (2019). Regulation (EU) 2019/1009 of the European Parliament and of the Council of 5 June 2019 laying down rules on the making available on the market of EU fertilising products and amending Regulations (EC) No 1069/2009 and (EC) No 1107/2009 and repealing Regulation (EC) No 2003/2003 (Text with EEA relevance). Official J. Eur. Union 170, 1–114. Available at: http://data.europa.eu/eli/reg/2019/1009/oj (Accessed June 28, 2022).
Fang, W., Delapp, R. C., Kosson, D. S., van der Sloot, H. A., and Liu, J. (2017). Release of heavy metals during long-term land application of sewage sludge compost: Percolation leaching tests with repeated additions of compost. Chemosphere 169, 271–280. doi:10.1016/j.chemosphere.2016.11.086
Fermoso, F. G., Hullebusch, E. V., Collins, G., Roussel, J., Mucha, A. P., and Esposito, G. (2019). Trace elements in anaerobic Biotechnologies. Available at:. doi:10.2166/9781789060225
Gu, C., and Bai, Y. (2018). Heavy metal leaching and plant uptake in mudflat soils amended with sewage sludge. Environ. Sci. Pollut. Res. 25 (31), 31031–31039. doi:10.1007/s11356-018-3089-5
Haiba, E., Lembit, N., Koit, H., Mari, I., and Merike, L. (2018). On the degradation of metformin and carbamazepine residues in sewage sludge compost. Agron. Res. 16 (3), 696–707. doi:10.15159/AR.18.123
Hattab-Hambli, N., Lebrun, M., Miard, F., Le Forestier, L., Bourgerie, S., and Morabito, D. (2020). Preliminary characterization of a post-industrial soil for long-term remediation by phytomanagement: Mesocosm study of its phytotoxicity before field application. Int. J. Environ. Res. 14 (1), 93–105. doi:10.1007/s41742-019-00241-5
Jia, J., Bai, J., Xiao, R., Tian, S., Wang, D., Wang, W., et al. (2022). Fractionation, source, and ecological risk assessment of heavy metals in cropland soils across a 100-year reclamation chronosequence in an estuary, South China. Sci. Total Environ. 807, 151725. doi:10.1016/j.scitotenv.2021.151725
Kabata-Pendias, A., and Pendias, H. (2001). Trace elements in soils and plants, 3rd edn. Boca Raton, FL: CRC Press.
Kaschl, A., Römheld, V., and Chen, Y. (2002). The influence of soluble organic matter from municipal solid waste compost on trace metal leaching in calcareous soils. Sci. Total Environ. 291 (1–3), 45–57. doi:10.1016/S0048-9697(01)01091-9
Kaur, G., Wong, J. W. C., Kumar, R., Patria, R. D., Bhardwaj, A., Uisan, K., et al. (2020). Value addition of anaerobic digestate from biowaste: Thinking beyond agriculture. Curr. Sustainable/Renewable Energy Rep. 7 (2), 48–55. doi:10.1007/s40518-020-00148-2
Kisielewska, M., Debowski, M., Zielinski, M., Kazimierowicz, J., Quattrocelli, P., and Bordiean, A. (2022). Effects of liquid digestate treatment on sustainable microalgae biomass production. BioEnergy Res. 15, 357–370. doi:10.1007/s12155-021-10251-x/Published
Kisielewska, M., Zielinski, M., Debowski, M., Kazimierowicz, J., Romanowska-Duda, Z., and Dudek, M. (2020). Effectiveness of Scenedesmus sp. Biomass grow and nutrients removal from liquid phase of digestates. Energies 13 (6), 1432. doi:10.3390/en13061432
Krzeminski, P., Tomei, M. C., Karaolia, P., Langenhoff, A., Almeida, C. M. R., Felis, E., et al. (2019). Performance of secondary wastewater treatment methods for the removal of contaminants of emerging concern implicated in crop uptake and antibiotic resistance spread: A review. Sci. Total Environ. 648, 1052–1081. doi:10.1016/j.scitotenv.2018.08.130
Kupper, T., Burge, D., Bachmann, H. J., Gusewell, S., and Mayer, J. (2014). Heavy metals in source-separated compost and digestates. Waste Manag. 34 (5), 867–874. doi:10.1016/j.wasman.2014.02.007
Lee, J. T. E., Ok, Y. S., Song, S., Dissanayake, P. D., Tian, H., Tio, Z. K., et al. (2021). Biochar utilisation in the anaerobic digestion of food waste for the creation of a circular economy via biogas upgrading and digestate treatment. Bioresour. Technol. 333, 125190. doi:10.1016/j.biortech.2021.125190
Lewis, J., and Sjöstrom, J. (2010). Optimizing the experimental design of soil columns in saturated and unsaturated transport experiments. J. Contam. Hydrology 115 (1–4), 1–13. doi:10.1016/j.jconhyd.2010.04.001
Liu, J., Zhang, W., Mei, M., Wang, T., Chen, S., and Li, J. (2022). A Ca-rich biochar derived from food waste digestate with exceptional adsorption capacity for arsenic (III) removal via a cooperative mechanism. Sep. Purif. Technol. 295, 121359. doi:10.1016/j.seppur.2022.121359
Logan, M., and Visvanathan, C. (2019). Management strategies for anaerobic digestate of organic fraction of municipal solid waste: Current status and future prospects. Waste Manag. Res. 37, 27–39. doi:10.1177/0734242X18816793
Mahon, A. M., O’Connell, B., Healy, M. G., O’Connor, I., Officer, R., Nash, R., et al. (2017). Microplastics in sewage sludge: Effects of treatment. Environ. Sci. Technol. 51 (2), 810–818. doi:10.1021/acs.est.6b04048
Makádi, M., Tomócsik, A., and Orosz, V. (2012). “Digestate: A new nutrient source - review,” in Biogas. [Preprint]. doi:10.5772/31355
Nabel, M., Barbosa, D. B., Horsch, D., and Jablonowski, N. D. (2014). Energy crop (Sida hermaphrodita) fertilization using digestate under marginal soil conditions: A dose-response experiment. Energy Procedia 59, 127–133. doi:10.1016/j.egypro.2014.10.358
Pastorelli, R., Valboa, G., Lagomarsino, A., Fabiani, A., Simoncini, S., Zaghi, M., et al. (2021). Recycling biogas digestate from energy crops: Effects on soil properties and crop productivity. Appl. Sci. Switz. 11 (2), 750–770. doi:10.3390/app11020750
Pathan, S. I., Arfaioli, P., Bardelli, T., Ceccherini, M. T., Nannipieri, P., and Pietramellara, G. (2020). Soil pollution from micro-and nanoplastic debris: A hidden and unknown biohazard. Sustain. Switz. 12 (18), 7255–7286. doi:10.3390/su12187255
Pecorini, I., Peruzzi, E., Albini, E., Doni, S., Macci, C., Masciandaro, G., et al. (2020). Evaluation of MSW compost and digestate mixtures for a circular economy application. Sustain. Switz. 12 (7), 3042. doi:10.3390/su12073042
Peng, W., and Pivato, A. (2019). Sustainable management of digestate from the organic fraction of municipal solid waste and food waste under the concepts of back to earth alternatives and circular economy. Waste Biomass Valorization 10 (2), 465–481. doi:10.1007/s12649-017-0071-2
Petersen, F., and Hubbart, J. A. (2021). The occurrence and transport of microplastics: The state of the science. Sci. Total Environ. 758, 143936. doi:10.1016/j.scitotenv.2020.143936
Prosser, R. S., Trapp, S., and Sibley, P. K. (2014). Modeling uptake of selected pharmaceuticals and personal care products into food crops from biosolids-amended soil. Environ. Sci. Technol. 48 (19), 11397–11404. doi:10.1021/es503067v
Qi, R., Jones, D. L., Li, Z., Liu, Q., and Yan, C. (2020). Behavior of microplastics and plastic film residues in the soil environment: A critical review. Sci. Total Environ. 703, 134722. doi:10.1016/j.scitotenv.2019.134722
Qiao, Y., Zhang, S., Quan, C., Gao, N., Johnson, C., and Wu, C. (2020). One-pot synthesis of digestate-derived biochar for carbon dioxide capture. Fuel 279, 118525. doi:10.1016/j.fuel.2020.118525
R Core Team (2021). R: A language and environment for statistical computing. Vienna, Austria: R Foundation for Statistical Computing.
Ramos, T. B., Horta, A., Goncalves, M. C., Pires, F. P., Duffy, D., and Martins, J. C. (2017). The INFOSOLO database as a first step towards the development of a soil information system in Portugal. Catena 158, 390–412. doi:10.1016/j.catena.2017.07.020
Rauret, G., Lopez-Sanchez, J. F., Sahuquillo, A., Rubio, R., Davidson, C., Ure, A., et al. (1999). Improvement of the BCR three step sequential extraction procedure prior to the certification of new sediment and soil reference materials. J. Environ. Monit. 1, 57–61. doi:10.1039/A807854H
Rodríguez Alberto, D., Tyler, A. C., and Trabold, T. A. (2021). Phosphate adsorption using biochar derived from solid digestate. Bioresour. Technol. Rep. 16, 100864. doi:10.1016/j.biteb.2021.100864
Rossi, G., and Beni, C. (2018). Effects of medium-term amendment with diversely processed sewage sludge on soil humification—Mineralization processes and on Cu, Pb, Ni, and Zn bioavailability. Plants 7 (1), 16. doi:10.3390/plants7010016
Ryan, P. C., Hillier, S., and Wall, A. J. (2008). Stepwise effects of the BCR sequential chemical extraction procedure on dissolution and metal release from common ferromagnesian clay minerals: A combined solution chemistry and X-ray powder diffraction study. Sci. Total Environ. 407 (1), 603–614. doi:10.1016/j.scitotenv.2008.09.019
Saveyn, H., and Eder, P. (2014). End-of-waste criteria for biodegradable waste subjected to biological treatment (compost & digestate): Technical proposals, Joint Research Center Scientific and Policy Reports.
Sayen, S., Rocha, C., Silva, C., Vulliet, E., Guillon, E., and Almeida, C. M. R. (2019). Enrofloxacin and copper plant uptake by Phragmites australis from a liquid digestate: Single versus combined application. Sci. Total Environ. 664, 188–202. doi:10.1016/j.scitotenv.2019.01.134
Shaheen, S. M., Tsadilas, C. D., and Rinklebe, J. (2013). A review of the distribution coefficients of trace elements in soils: Influence of sorption system, element characteristics, and soil colloidal properties. Adv. Colloid Interface Sci. 201-202, 43–56. doi:10.1016/j.cis.2013.10.005
Shuman, L. M. (1999). Organic waste amendments effect on zinc fractions of two soils. J. Environ. Qual. 28 (5), 1442–1447. doi:10.2134/jeq1999.00472425002800050008x
Sogn, T. A., Dragicevic, I., Linjordet, R., Krogstad, T., Eijsink, V. G. H., and Eich-Greatorex, S. (2018). Recycling of biogas digestates in plant production: NPK fertilizer value and risk of leaching. Int. J. Recycl. Org. Waste Agric. 7 (1), 49–58. doi:10.1007/s40093-017-0188-0
Svensson, K., Odlare, M., and Pell, M. (2004). The fertilizing effect of compost and biogas residues from source separated household waste. J. Agric. Sci. 142 (4), 461–467. doi:10.1017/S0021859604004514
Tampio, E., Salo, T., and Rintala, J. (2016). Agronomic characteristics of five different urban waste digestates. J. Environ. Manag. 169, 293–302. doi:10.1016/j.jenvman.2016.01.001
Teglia, C., Tremier, A., and Martel, J. L. (2011). Characterization of solid digestates: Part 1, review of existing indicators to assess solid digestates agricultural use. Waste Biomass Valorization 2 (1), 43–58. doi:10.1007/s12649-010-9051-5
Thi Nguyen, M. L., Lin, C. Y., and Lay, C. H. (2019). Microalgae cultivation using biogas and digestate carbon sources. Biomass Bioenergy 122, 426–432. doi:10.1016/j.biombioe.2019.01.050
Verlicchi, P., and Zambello, E. (2015). Pharmaceuticals and personal care products in untreated and treated sewage sludge: Occurrence and environmental risk in the case of application on soil - a critical review. Sci. Total Environ. 538, 750–767. doi:10.1016/j.scitotenv.2015.08.108
Wang, Y., Wang, X., Li, Y., Li, J., Liu, Y., Xia, S., et al. (2021). Effects of exposure of polyethylene microplastics to air, water and soil on their adsorption behaviors for copper and tetracycline. Chem. Eng. J. 404, 126412. doi:10.1016/j.cej.2020.126412
Ward, A. J., Hobbs, P. J., Holliman, P. J., and Jones, D. L. (2008). Optimisation of the anaerobic digestion of agricultural resources. Bioresour. Technol. 99 (17), 7928–7940. doi:10.1016/j.biortech.2008.02.044
Watteau, F., Dignac, M. F., Bouchard, A., Revallier, A., and Houot, S. (2018). Microplastic detection in soil amended with municipal solid waste composts as revealed by transmission electronic microscopy and pyrolysis/GC/MS. Front. Sustain. Food Syst. 2. doi:10.3389/fsufs.2018.00081
Weng, L., Temminghoff, E. J. M., Lofts, S., Tipping, E., and Van Riemsdijk, W. H. (2002). Complexation with dissolved organic matter and solubility control of heavy metals in a sandy soil. Environ. Sci. Technol. 36 (22), 4804–4810. doi:10.1021/es0200084
Wu, X., Dodgen, L. K., Conkle, J. L., and Gan, J. (2015). Plant uptake of pharmaceutical and personal care products from recycled water and biosolids: A review. Sci. Total Environ. 536, 655–666. doi:10.1016/j.scitotenv.2015.07.129
Xu, T., Nan, F., Jiang, X., Zeng, Y., Zhang, W., and Shi, B. (2020). Effect of soil pH on the transport, fractionation, and oxidation of chromium(III). Ecotoxicol. Environ. Saf. 195, 110459. doi:10.1016/j.ecoenv.2020.110459
Zeremski, T., Randelovic, D., Jakovljevic, K., Jeromela, A. M., and Milic, S. (2021). Brassica species in phytoextractions: Real potentials and challenges. plants 10, 2340. doi:10.3390/plants10112340
Zhang, S., Han, B., Sun, Y., and Wang, F. (2020). Microplastics influence the adsorption and desorption characteristics of Cd in an agricultural soil. J. Hazard. Mater. 388, 121775. doi:10.1016/j.jhazmat.2019.121775
Keywords: trace metals, municipal solid waste digestate, metal bioavaibility, soil-metal interaction, metal dynamics
Citation: Baldasso V, Bonet-Garcia N, Sayen S, Guillon E, Frunzo L, Gomes CAR, Alves MJ, Castro R, Mucha AP and Almeida CMR (2023) Trace metal fate in soil after application of digestate originating from the anaerobic digestion of non-source-separated organic fraction of municipal solid waste. Front. Environ. Sci. 10:1007390. doi: 10.3389/fenvs.2022.1007390
Received: 30 July 2022; Accepted: 08 December 2022;
Published: 04 January 2023.
Edited by:
Chuan Wu, Central South University, ChinaReviewed by:
Marcin Debowski, University of Warmia and Mazury in Olsztyn, PolandMohammad Rizwan Khan, King Saud University, Saudi Arabia
Copyright © 2023 Baldasso, Bonet-Garcia, Sayen, Guillon, Frunzo, Gomes, Alves, Castro, Mucha and Almeida. This is an open-access article distributed under the terms of the Creative Commons Attribution License (CC BY). The use, distribution or reproduction in other forums is permitted, provided the original author(s) and the copyright owner(s) are credited and that the original publication in this journal is cited, in accordance with accepted academic practice. No use, distribution or reproduction is permitted which does not comply with these terms.
*Correspondence: Veronica Baldasso, veronica.baldasso@fc.up.pt