- 1Department of Environmental Science, Faculty of Life Sciences and Informatics, Balochistan University of Information Technology, Engineering and Management Sciences, Quetta, Pakistan
- 2School of Environmental Studies, China University of Geosciences, Wuhan, Hubei Province, China
Due to high toxicity, arsenic is regarded as a major global environmental pollutant. The present study is investigated the potential factors influencing to elevate concentration of arsenic in groundwater, surface water, and soil of the Dongting basin. The arsenic contamination potential prediction map and categories were developed using various GIS techniques such as Ordinary Kriging and the Quantile method. Then the “Raster calculator” tool was applied to verify the impact of the factors on arsenic. Eighty-four single-factor, bi-factor, and multi-factor models were established to investigate effective combinations among factors of each phase. Additionally, statistical tests were computed to evaluate arsenic between classes and factors. The arsenic value varies in groundwater from 0.0001 to 0.1582 mg/l, while in surface water between 0.0001–0.0287 mg/l and soil sediments range from 1.8–45.69 mg/kg. JunShan and GongAn groundwater resources have been identified as posing a high risk to human health. The single factors showed the best match frequency of arsenic with a population density of 66.86% in water and land use depicted match frequency of arsenic 73.19% in soil. The statistical calculations with percentage frequency factors also depicted positive trends. The correlation of the factors with arsenic in soil and water showed slow oxidation and reduction in the groundwater system. Treated portable water could be the best option to reduce the health risk of the local community.
1 Introduction
Contaminated natural sources are a constant threat to the healthy survival of the living organisms (Chormare and Kumar, 2022), but due to human activities and natural factors, the natural composition of the soil, surface water, and groundwater has been changed (Malik Muhammad and Zhonghua, 2014). Groundwater arsenic is known as a high-toxicity environmental pollutant globally due to its carcinogenic and non-carcinogenic health effects on living organisms (human and animal) health (Zhang et al., 2015a; Waqas et al., 2017) and skin cancer (Lamm et al., 2007). Last few decades, heavy metal pollutants in water and soil have seriously affected China’s ecological environment (Wu et al., 2014). Elevated value of arsenic in groundwater has been observed in many countries, especially Bangladesh, Cambodia, Chile, China, India, Pakistan, Vietnam, and the United States (Polizzotto et al., 2008; Fendorf et al., 2010; Muhammad et al., 2016). A high arsenic ratio was reported in China’s continental lithosphere (CCL) and natural water (Che et al., 2020). However, Bangladesh, Pakistan, and China are facing serious issues related to waterborne endemic arsenic poison (Zhang et al., 2015b; Muhammad et al., 2016). Between 2008 and 2009, five major arsenic contamination incidents were reported from providences: Guizhou, Hunan, Guangxi, Yunnan, and Henan (Wu et al., 2014). In recent decade, environmentalists have focused their research on pollution issues in the Dongting basin (Chen et al., 2018). Exacerbated human activities, reclaimed agriculture, and deforestation have dominated its catchment and adjacent area of the Dongting basin (Du et al., 2001). Different researchers conducted studies and concluded that the Dongting area contained a high arsenic and other heavy metals in water resources (Yao et al., 2009; Qian et al., 2005). China is facing high pressure on its natural resources due to urbanization and industrialization, which has many challenges related to arsenic pollution (Zhang et al., 2009; Li et al., 2021). During a national survey, it was found that 2.7% of collected soil showed elevated concentration of arsenic (http://www.mep.gov.cn/gkml/hbb/qt/201404/W020140417558995804588.pdf). Song investigated 109 regions of China and found a 9.48 mg/kg average arsenic value in soil (Song et al., 2013). During a survey of 45 counties in nine provinces of China, nineteen contained elevated arsenic in portable water higher than WHO permissible limit (0.01 mg/l) and China Standard (0.05 mg/l) (He and Charlet, 2013). It was observed that 0.01–0.05 mg/l arsenic value was exposed in the local community (He et al., 2020). The present research is a comprehensive study of arsenic distribution in water and soil in the Dongting basin of China, including various factors such as groundwater level, population density, heavy metal contamination, pumping rate, land use, rain, and surface elevation. The influence of these factors to high arsenic in the area was investigated. Remote sensing techniques, GIS applications and statistical methods are new ways to find out what controls the amount of arsenic in three different media.
2 Location, climate, and hydrogeology of Dongting plain
Dongting Lake Plain is located in the northeast of Hunan Province, in the south of the two lake plains (also known as the Hubei basin), and in the north, it is connected with the Jianghan Plain in Hubei Province. It is mainly composed of the sediment imported from the Yangtze River through the four ports of Songzi, Taiping, Ouchi, and Tiaoxuan, and the sediment from the Xiangjiang, Zijiang, Yuanjiang, and Lishui rivers. It covers an area of 10,000 km2 with an altitude of 34.5 m and a total capacity of 17.8 BCM. The whole plain covers a total area of 18,780 km2, of which 15,200 km2 are part of Hunan Province, accounting for 81% of the total area; 3,580 km2 are part of Hubei Province, accounting for 19%. Most of the main lakes and depressions are on the southern margin. Dongting Lake produces fish, water chestnuts, lotus, reeds, etc. It is an ideal base for grain, cotton, hemp, aquatic products, and silk. The lake area is mostly surrounded by lakes to form polder fields. China’s commodity grain base and important freshwater fish areas the output has reached the forefront of the country.
3 Data collection and methodology
3.1 Sampling
Total 300 groundwater samples were collected from Donating Plain while 125 samples and 75 samples were collected from surface water (Rivers and Lakes) and soil, respectively. The groundwater depth is shallow in the study area, generally 0.5–3.5 m deep. The samples were collected after more than 10 min of pumping until the physical parameters [temperature, electrical conductivity (EC), and pH] were stable. Four 50 ml HDPE bottles were used for each sample point and filtered on site (using 0.45 m membrane filters). For the total dissolved analysis, HCL was used to acidify the bottle and wrap it with tinfoil. The second bottle was acidified (PH2 using ultra-pure HNO3) for chemical analysis of dissolved ions and trace elements, and the remaining two bottles were not acidified for the analysis of anions and H/O isotopes. All samples were immediately stored at 4°C until the analyses were completed. Our study area is seriously affected by arsenic contamination of groundwater, so investigating arsenic levels in the soil is important to develop their relationship. Soil samples were collected at 20 cm in depth. The soil samples were collected from a boring core and capped with PTFE lids, and after that, they were stored at 4°C in an opaque anaerobic box.
3.2 Analytical methods for waters
Temperature, dissolved oxygen (DO), pH, and EC were measured on the sampling spot using a portable meter (HQ40D Field Case, cat. No. 58258-00, HACH, Colorado, United States). The portable gel-filled ORP probe (MTC10103, HACH) was used to measure Eh. The total concentration value was determined for dissolved ions (Mg and Fe) using an inductively coupled plasma atomic emission spectrometer (ICP-AES) (IRIS Intrepid II XSP, United States). For trace elements, inductively coupled plasma mass spectrometry (ICP-MS) (ELAN 9000/DRC-e, PerkinElmer) was used. Verification of the accuracy of major elements analyzed by ICPAES and ICP-MS was found within 4% and 5%, respectively. Anions (NO3, and SO4) were measured using an ion chromatograph (Dionex 2500, United States). The analytical precision was better than 5%. The total organic carbon analyzer (multi N/C 3100, Germany) was used to measure dissolved organic carbon (DOC) and the detected value of 0.004 mg/l with a precision of 8%. Total As of groundwater and surface water were measured using a hydride generation-atomic fluorescence spectrometer (HG-AFS, 930, Titan, China).
3.3 Soil analyst method
To measure the mineralogical compositions of the soil samples, an automated powder X-Ray Diffractometer (Cu–K radiation and a graphite monochromator) (X'Pert PRO DY2198, PANalytical) was used with a detection limit of 2%. Chemical elements were determined by X-Ray Fluorescence Spectroscopy (Spectro Xepos HE XRF Spectrometer). The rest of the chemical tests were done at the China University of Geosciences in Wuhan at the State Key Laboratory of Biogeology and Environmental Geology and the State Key Laboratory of Geological Processes and Mineral Resources.
3.4 GIS models development
The study area’s topographic map (1:50,000) was used to create a thematic map that contains comprehensive information about the boundaries and land use in Figure 1. The simple Kriging method was used in the ArcGIS Geostatistical analyst toolbar to develop arsenic distribution zones in groundwater, surface water, and soil for the study area. The 2014 arsenic levels of pumping wells were used to fulfill this aim. Kriging and co-kriging are parts of the geostatistical techniques, which are found to be superior in several functions for data analysis. For example, they are included in exploratory spatial data analysis tools to handle statistical properties and to create various types of maps such as probability, prediction, quantile, simple and ordinary. Kriging can be divided into two other sub-methods: ordinary kriging and indicator kriging, which provide two types of information. Ordinary kriging is useful for the prediction and development of contamination potential maps, whereas indicator kriging is important for the identification of probabilities. To design the distribution map, the 2012 arsenic value were categorized into five levels using the Quantile method as shown in Table 1. Then, six different factors were tested to examine their influence on arsenic levels in groundwater, surface water, and soil in the study area, such as:
3.4.1 Groundwater level
Since local groundwater level is the main factor for infiltration and transport of various pollutants, the simple kriging method in the geostatistical analysis was used again to create groundwater level zones for the study area. Pumping Ratio: Total pumping quality per annum was calculated for each county located in the Dongting Plan. ArcGIS was used for further analysis. Finally, the result was converted from vector data format to raster data format for the final analysis process.
3.4.2 Rain
Rain data for the year 2014 was collected from the website of the Hydrology and Water Resources Survey in Hunan Province, and a total of 90 meteorological stations’ data was used to show rainfall tendency through the geostatistical analyst tool in GIS.
3.4.3 Heavy metal contamination
A heavy metal concentration distribution map was developed based on data available in a report titled “The Ecological Geochemical Assessment Report of Dongting Lake in Hunan Province” published by the Geological Research Institute of Hunan Province in the year 2007. A geostatistical analyst tool was applied to show the final contamination elevated concentration in GIS.
3.4.4 Landuse
Landuse is an important factor for generating and infiltrating arsenic. A recent Landsat-8 image was used to create a supervised land use classification for the study area in the ENVI software environment. Seven major categories (Woodland, Dryland, Paddy Field, Surface Water, Construction Area, Bareland, and Greenland) were exploited to understand real local land use.
3.4.5 Topography
Elevation is a factor that could control water flow while low elevation produces more chances of water infiltration. An ASTER Digital Elevation Model (DEM) was used to create a contour map for the study area using 3D-analyst tools in the ArcGIS environment. The results from each factor were categorized into five groups according to the quantile method using the reclassify tool included in the spatial analysis toolset. The reclassifies were applied to modify the values in the raster, and the input raster was classified into different class ranges for the reclassification. The “Raster calculator” tool was used to determine the most important factor influencing arsenic distribution in the study area. It allows for creating and executing an algebraic expression that outputs a raster. Spatial Analyst operators were employed to transform two inputs into a single expression. The Raster Calculator is designed to execute a single-line algebraic expression. It is developed using multiple tools and operators included in the simple calculator tool interface. Forty different models were developed using a raster calculator. The models were built by combining different factors to find the most effective combination among them. Single and multi-factor models were carefully established by assigning coefficients 1 to 6 as rank because each model must contain unique values. Then, the quantile method included in the Reclassify tool was repeated for each model to categorize the results into five classes. To evaluate the eighty-four models, the “Equal to frequency” tool was applied based on a cell-by-cell match with the Arsenic distribution model result, and then the best frequency match combination models were selected. Additionally, the frequency range was computed with the “Band Collection Statistic” tool that provides statistics results from a multivariate analysis of a set of raster bands. The frequency range was also used to count the matched and unmatched pixels in each of the forty models. Finally, statistical analysis was computed to investigate the effect of factors (groundwater, surface water, and soil arsenic) with high equal frequency ratio factors. The collected data was first analyzed using Microsoft Office Excel 2007 spreadsheets. Several classes were built for each factor to evaluate their variation in arsenic. Correlation analysis was applied to verify arsenic value in water with other factors (population density and water level) and arsenic distribution in soil with land use and pumping. Results and discussion are presented in detail in the next sections based on the described methodology.
4 Results and discussions
4.1 Arsenic presence in surface and subsurface water
4.1.1 Chemical analysis of water samples
Three hundred groundwater samples were collected from different drinking water sources such as wells, hand-pump, and motor pumps. Minimum and maximum concentration of arsenic was computed during the analysis process as 0.0001 and 0.1582 mg/l, respectively. The statistical results showed a variance range of 0.00026 and the standard deviation was 0.0159. However, arsenic distribution in groundwater showed local variations within the distribution pattern. Figure 2A displayed that high concentration of arsenic areas were on the east side (higher than the Chinese permissible limit) and the north area showed slightly lower but higher than the WHO limit. However, the south and west have fewer pollution ratios. In Figure 2A, the red color represented arsenic concentration of over 0.05 mg/l, which is believed to be higher than WHO (0.01 mg/l) and Chinese (0.05 mg/l), and water in the corresponding area is supposed to be harmful to the community’s health. Based on available data from different residential areas, commercial, industrial, and agricultural areas, the pumping wells were scattered throughout the whole area, but the northern part of the counties contained low risk, where the average concentration was less than 0.02 mg/l, and water in these towns is considered safe for drinking. The highest concentration of arsenic was observed in Junshan county in the east and the surrounding areas of Anxiang city in the north. During a study on groundwater quality, the results showed that 76 cities were seriously polluted, while 39 cities were slightly polluted, and the groundwater pollution trend is from urban to suburban areas in China (Mei and Feng, 1993). China Daily (2006) reported that Yueyang County, located in Hunan Province, has contained dangerous levels of concentration of arsenic in drinking water.
Surface water samples were collected from rivers and lakes at Dongting Plain. On the basis of the chemical analysis results of 125 samples, low and high values were determined to be 0.0001 and 0.0287 mg/l, respectively, while the calculated variance was 9.5 × 10−5 and standard derivative 0.0098. The surface water concentration of arsenic developed map was depicted with spatial variation. The eastern and central parts had the highest concentrations of more than 0.02 mg/l, while the northwest had the lowest values compared to WHO and Chinese standards. Previous researchers have identified a lot of surface water resources in the form of lakes, rivers, and open water bodies, but the quality of water is not good (He and Charlet, 2013). In Figure 2B, Dongting lake, located in the east, exhibits a high arsenic value. It was observed that the contamination level of river waters was higher than that of waters in lakes at Dongting Plain. Economic development pressures have accelerated human activities to rise in production (industrial as well as agricultural) and urbanization is a major source of surface water pollution in the Dongting plan. BI observed that in China, domestic sewage has polluted lakes (Akhtar et al., 2015; Bi et al., 2018), while another researcher identified agricultural activities caused by watersheds (Gao and Mucci, 2003). Similarly, due to untreated industrial effluents and wastes, various pollutants values in river waters did not meet standards. In the year 2005, toxic arsenic events were reported in branches and reaches of the Li River and Xiang River (Hunan Water Administration, 2006). According to statistics, the amount of untreated polluted water drained into rivers is 3.42 × l010T, with 2.565 × l010T (7.5 percent) from industrial sewage and 8.85 × l03T (25 percent) from domestic sewage (Guogang et al., 1991). It can be concluded that polluted surface water affects groundwater quality. Controlling fresh watershed pollution in China is important to integrate issues related to population, economic development, and future planning. It will help to address human health-related issues.
4.1.2 GIS base spatial distribution model of water
The permissible level of arsenic in drinking water in China is 0.05 mg/l (50 ppb) in groundwater and surface water, and 40 mg/kg in soil (Ministry of Health of ChinaStandardization Administration of China, 2006), which is safe enough for human health. The distribution of concentration of arsenic in waters was compared using similar frequency values of a single factor (7 models), two factors (4 models), three factors (9 models), and multi-factors (42 models), which are included in raster models. The results of the estimated frequency values, according to the models, are displayed in Table 1. The single model’s frequencies of population density (Model 53 with 66.86%) and water level (Model 51 with 64.47%) were greater than the other single and combined factor model frequencies. The model results showed that high matching related to population density and water level individually greatly affects the concentration of arsenic in the Dongting basin. Furthermore, for the single models, the population density model gave the highest matching frequency with arsenic, while rainfall (Model 56, with 31.26%) provided the lowest frequency. The observed moderate frequency values were 64.33% and 60.32% for land use and pumping, respectively. However, this model frequency in a single factor was lower than that of its combination in the multi-factor models (Table 1). The best matching frequency for the multi-factor models was observed for the 2WL*1Pop (model 50 with an equal frequency of 65.49%) and the 1WL+2Pop+3LU (model 47 with an equal frequency of 63.61%). The highest matching frequency percentages were 65.49% among two factor combination models, 63.61% between three factor models, and the lowest, 55.91%, while the four and five factor values ranged from 10.9% to 55.66%. The most effective factors were population density and water level, which have the two highest matching frequencies between the models. This result was confirmed by the frequency maps of the best four models shown in Figure 3. The analysis with an equal frequency tool explored the various factors contributing to the decreasing water quality in the Dongting basin. This result helped to identify the affectivity of single factors as well as the different groups of multi-factors. Human activities based on water demand impacting the water level through pumping were diagnosed as major factors enriching the water resources with arsenic.
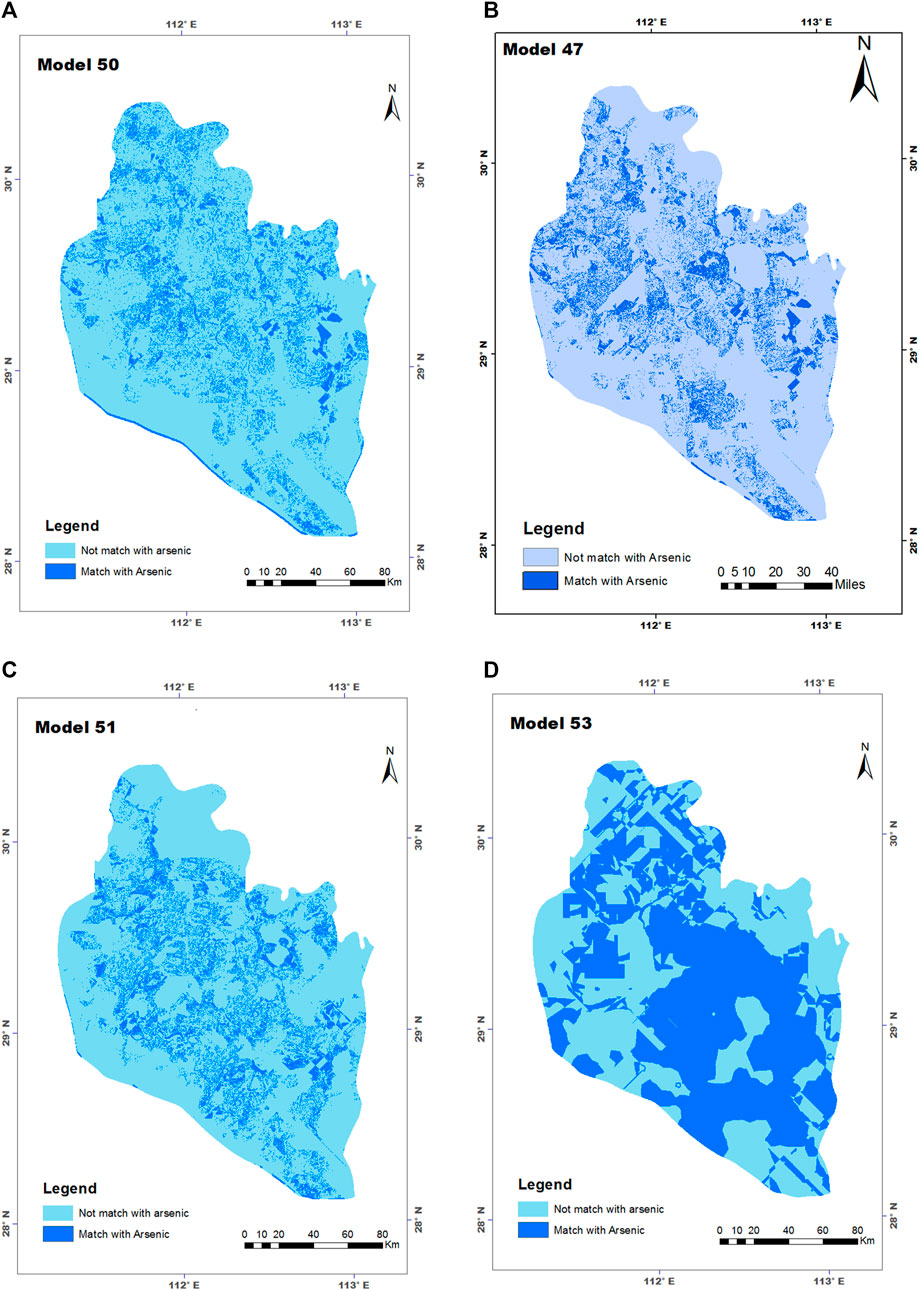
FIGURE 3. Final equal frequency map of best models with groundwater and surface water concentration of arsenic: (A) (1*WL+3*LU+2*Pop), (B) (2*WL+1*Pop), (C) WL, and (D) Pop.
4.1.3 High equal frequency factors in water
4.1.3.1 Population density vs. arsenic distribution
According to the National Bureau of Statistics, the annual national population growth rate was 0.49% between 2010 and 2013, and in Hunan Province, it was 0.62% in the last 3 years. The population strength was between 1 × 105 and 120 × 105, as displayed in the results of the population distribution in Table 2. The population distribution map (Figure 4A) also showed a wide spatial dispersion of the population in the available area. The central area had medium density compared to other parts of the Dongting Basin. Additionally, high spatial distribution was found in the northern part, and four districts such as GongAn, Ning Xiang, and Changsha showed the highest population capacity, while in the south (HaoShan and WangCheng), east (JunShan), and west (LinLi and Anxiang) contained the lowest population. However, the medium population densities were located in the east and central parts of the study area. China’s population growth will undoubtedly affect and increase these distractions over time. Therefore, population pressures could involve an increase in solid waste production, urban non-point runoff, and a decline in water quality. In a case study of the Dongting basin, population distribution showed a low relationship with concentration of arsenic distribution. Figure 3D depicts the effect of population on the average concentration of arsenic of distractions in the Dongting basin. The statistical results are based on the average arsenic of each district, and the population density in the study area has a significant impact on the concentration of arsenic and its distribution with the value of the calculated correlation coefficient of +0.442. The average concentration of arsenic in distracts were divided into five classes, where the lowest population class (1–10 × 105) showed a 0.0164 mg/l concentration of arsenic under China’s permissible standards. A high arsenic (0.026 mg/l) value in the densely populated class (90–120 × 105) indicates that human activities are affecting contaminated groundwater resources. It is reported worldwide that groundwater resources in highly populated areas have elevated concentration of arsenic (Van Geen et al., 2002), with similar results in Dongting plain. Gaus founded a strong correlation between concentration of arsenic and population density in many areas of Dhaka (Gaus et al., 2003). China occupies only 7% of the total world’s land but feeds about 25% of its population. Therefore, water scarcity and quality are significant issues for a huge population (Impending Water Crisis in China, Nina Brooks). Concentration of arsenic in surface water resources was found to be significantly high, with concentrations of 0.02 while lowest value mg/l 0.005 mg/l. A mixed-correlation between arsenic range and population strength was observed with regard to classes. Therefore, it can be concluded that human activities have an influence on the control of pollution concentration in surface water resources. A comprehensive study was conducted to explore the population under arsenic risk, which showed that in highly populated areas of China, a greater percentage of inhabitants are affected by toxic arsenic (Rodriguez-Lado et al., 2013).
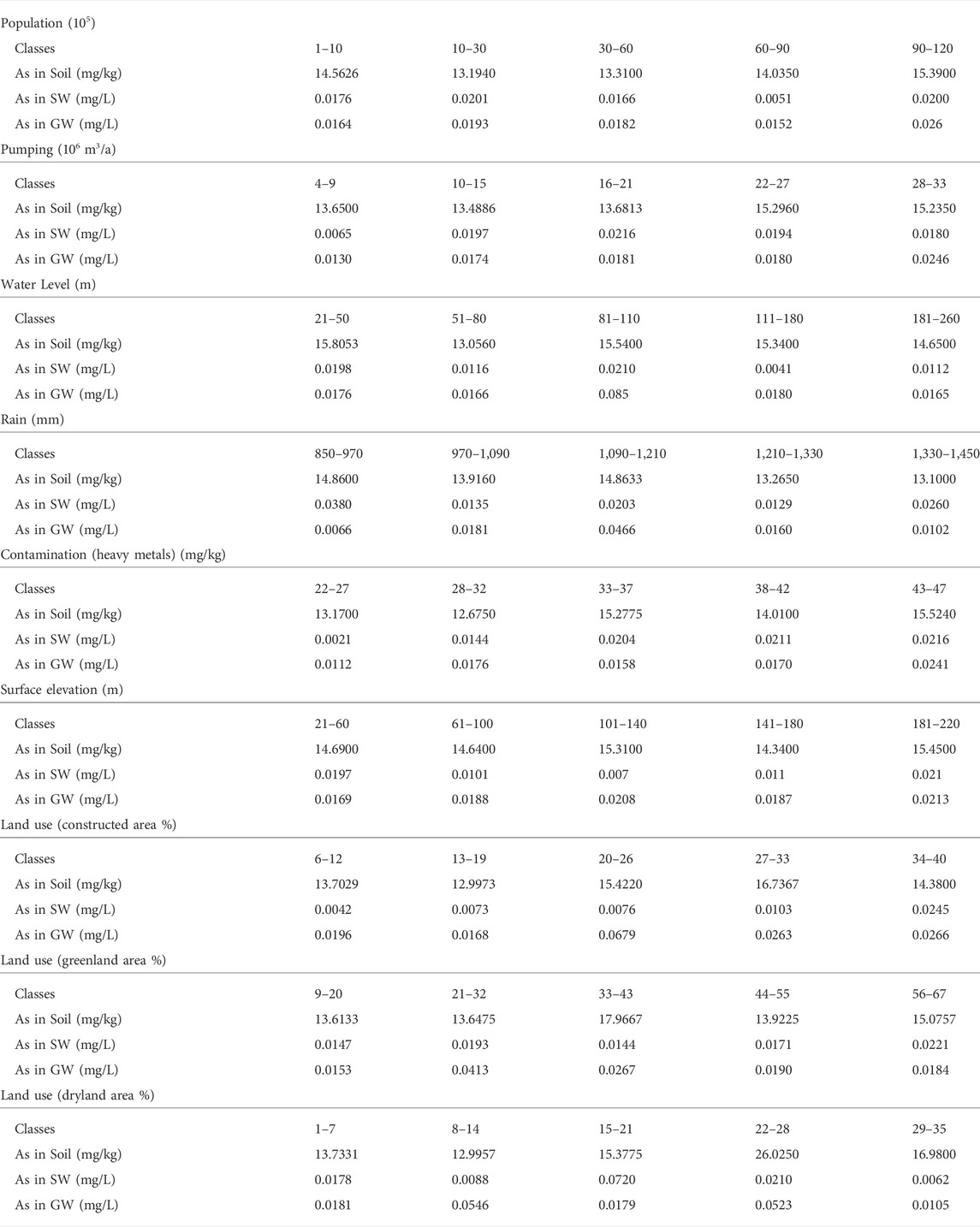
TABLE 2. Seven factors classifications and average arsenic concentration of each class in waters and soil.
4.1.3.2 Water table vs. arsenic distribution
The unconfined nature of the Dongting plain contributes to its high vulnerability to pollution. Figure 4B depicts the water levels in Dongting Plain. From Figure 4B, it was found that the west-south and west-north boundary areas showed the highest water table levels due to the presence of the mountains, and the whole central and east parts showed low water levels linked to plenty of natural lakes and other surface water resources. The groundwater level in the study area is gradually rising from east to west (Figure 4B). Joseph reported that concentration of arsenic has no significant relation to changing water levels in California, while New England shows a strong impact (Ayotte et al., 2015). Anthropogenic factors may affect concentration of arsenic by including land development and solutes in the aquifer (Harte et al., 2012), or by artificially changing the flow system, well development, and over-exploitation, which affect aquifer storage and recovery capacity (Ayotte et al., 2015; Katz et al., 2009; Price and Pichler, 2006). Excessive groundwater exploitation can activate the Fe-reducing process and mobilize arsenic in the aquifer system (Berg et al., 2008). The results on the impact of water level on arsenic distribution are displayed in Table 2. The results are in the Table 2 presented the average concentration of arsenic in groundwater, where a high value was observed at the middle class of water level (81–110 m) with 0.085 mg/l and low in deep water (Class 181–260). Surface water also contained high and low arsenic values in the same classes. Water levels less than 50 m and between 81–110 m in both surface and groundwater was affected, and arsenic in the soil was also significantly high. Therefore, the general trend showed that concentration of arsenic decreased with increasing groundwater depth in the Dongting basin. Yanhua’s study in central China’s Jianghan plain confirmed that concentration of arsenic variation has a highly positive correlation with groundwater level changes (Gan et al., 2014). The lowest water quality observed in the shallow aquifer is found to be due to surface water, urban water, and sanitation system, high urban density, poor solid waste, and agriculture activities, while high arsenic in the deep aquifer is related to the high pumping for different sectors such as urban, industrial, etc., (Farooqi et al., 2003). Additionally, Moqbul Hossain concluded in his study that the deep aquifer can be protected against arsenic percolation by shallow pumping (Hossain and Piantanakulchai, 2013). Our findings show that a high water table has more concentration of arsenic due to contaminated recharge, while the high concentration of arsenic in deep groundwater has a valid reason for high pumping for years.
4.1.3.3 Groundwater extration vs. arsenic distribution
Groundwater is a major source for drinking and domestic purposes in the Dongting plan. Groundwater exploitation is associated with seasonal water demand and may vary with seasons. Here, we evaluated the effect of the pumping rate on the concentration of arsenic of groundwater in the Dongting plain. The estimated total groundwater for various purposes of each district of the study area lies between 1.4 m3/a × 106 m3/a and 4.48 m3/a × 106 m3/a. Ravenscroft showed that the operational age of a well and the period of pumping have a serious link with groundwater quality and can elevate its concentration of arsenics (Erickson et al., 2019). The north and south parts show low groundwater exploitation as compared to other areas, while the results show that Linyi has low pumping and TaoYuan flows large amounts of groundwater. The distribution map of soil arsenic showed a significant close relationship with total groundwater exploitation; the central parts had elevated arsenic (Figure 2C) and similar areas had high pumping intensity (Figure 5B). The vulnerability of water supply wells to natural and anthropogenic contaminants depends on the pumping stress level of the groundwater system. Cuthbert et al. (2002) report that arsenic breakthroughs may occur within to 20 years of pumping, which depends on various hydraulic parameters. Regarding the concentration of arsenic and pumping quantity relationship, total groundwater exploitation has been divided into five classes to verify soil concentration of arsenic variation with increasing pumping quantity. The statistical findings investigated the general trend of positive correlation between two factors (r2 = +0.5782). The elevated arsenic value in soil is 22–27 m3/a × 106 m3/a, while the lowest concentration of arsenic in the second class is 16.63 mg/kg (10–15 m3/a × 106 m3/a). The soil arsenic value is slightly higher in the first class, but it gradually increases with pumping in subsequent classes. Researchers observed that redox conditions rapidly change due to exploitation, and concentration of arsenic varies with changes in pumping rate. Therefore, infiltration of high-arsenic induced water and increasing discharge rates into aquifers indicate the possible risks of arsenic contamination in groundwater.
4.2 Arsenic presence in soil
4.2.1 Chemical analysis of soil samples
Arsenic in soil and its impact on other natural resources and human health are gaining researchers’ attention worldwide (Zhang et al., 2015a; Biswas et al., 2020). Chemical analysis of 75 soil samples revealed a wide variation in a concentration range between 1.8 and 45.69 mg/kg. Weng explored arsenic levels of 2.5–33.5 mg/kg in the soil of China, where mountain soil (16 mg/kg) had comparatively higher levels than siallitic soil (4 mg/kg) (Wang and Shpeyzer, 2000). According to the researchers, a general baseline of arsenic value in soil should be 5–10 mg/kg (Firdaus et al., 2018). According to chemical analysis, 18% of soil samples have higher concentrations than the Chinese upland contamination standard (20 mg/kg) and 3% have higher concentrations than the WHO (50 mg/kg) limit. Li found that 58% of soil sediment samples exceeded PEL (probable effect level, dry weight (Smith et al., 1998) arsenic value (Fei et al., 2013). Additionally, the variance and standard derivative values were computed at 200.376 and 14.155, respectively. Fei et al. (2013) did an analysis of sediment samples at Dongting Lake and got an average arsenic value of 23.03 mg/kg (Fei et al., 2013). Figure 2C was developed by using an arsenic level in soil and showing the presence of the pollutant in the whole area. The north and west parts exhibited low concentrations, while the central area (with low surface elevation) showed a high-risk value. NanXian, YuanJiang, and Ziyang are the most affected counties in the central part, where Guo has identified the same location with high As content in soil due to agricultural activities, surface water flow (Guo et al., 2012), industrialization, and urbanization (Mu et al., 2019). Surface elevation may be a factor that enhances pollutants’ mobility towards low-elevation counties. Additionally, surface water quality impacts on sediments cannot be ignored, as previous research showed that rivers, lakes, and other bodies of water contain high arsenic quantities (He and Charlet, 2013), which infiltrate the aquifer through upland.
4.2.2 GIS base spatial distribution model of soil
The sections that follow will show the results of developed single and multi-factor models, as well as their correlation with elevated soil concentration of arsenic. It is extremely important to explore the impacts of local factors which increase arsenic value, their causes, and their relationship. The soil arsenic distribution model was compared with a total of 64 models (single and multi-factor). The highest equal frequency multi-factor models were used to develop gradually low factor models, but single, two, and three-factor models with high equal frequency are presented in Table 1. Among seven single model frequencies, land use (model_152) and pumping quantity (model_154) were depicted as equal frequency percentages of 73.19 and 73.07, respectively, while the lowest similar frequency could be seen with rainfall factor (model_156) with 13.62% in Table 1. Individual models of groundwater pumping and land use in the Dongting basin revealed a high equal frequency with soil concentration of arsenic. The highest and lowest equal frequency values among developed models were model-149 (1Pumping*2LU) and model-156 (rain) with 74.45% and 13.6% arsenic, respectively. The results of these models indicated that local anthropological activities had a negative impact on soil, which was eventually transmitted to the groundwater system. In the GIS “Raster Calculator” tool used to develop multi-factor models, a ranking technique is also applied to verify the highest and lowest impact of a factor. Table 1 represents the best model results and shows the most effective factor for pumping and land among all selected factors. However, the four highest equal frequency model diagrams are displayed in Figure 6.
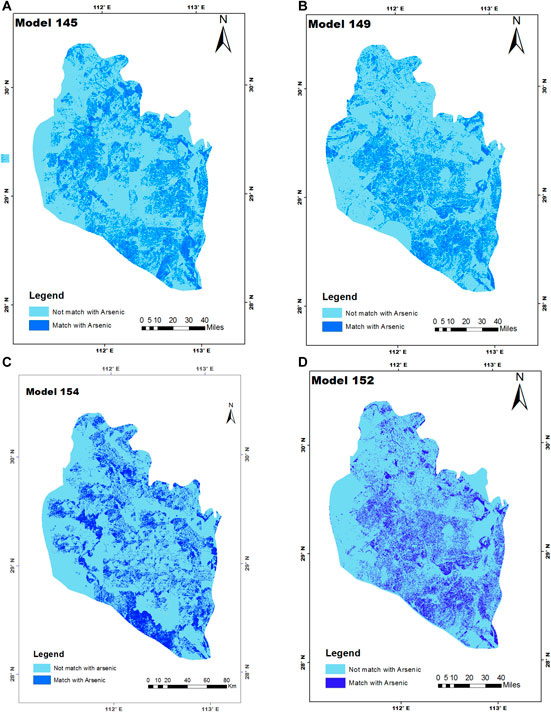
FIGURE 6. Final equal frequency map of best models with soil arsenic concentration: (A) (2*Pump+3*LU+1*SE), (B) (1*Pump+1*LU), (C) Pump, and (D) LU.
4.2.3 High equal frequency factors in soil
Land use is regarded as a major factor that directly generates various wastes and pollutants. The variation of groundwater concentration of arsenic within seven groups of land use classes is represented in Figure 5A. The land use map (Figure 5A) showed that greenland, dryland, surface water, and urbanization (domestic and commercial areas) were the most intense, followed by agricultural land use and industrial activities (others). As shown in Figure 5A, urban settlements and other construction cover nearly 15% of the study area. A large quantity of agrochemical use and the industrial revolution have subjected the soil to stress due to the rise in pollution levels (Zhong et al., 2012; Patle et al., 2019) worldwide. Human activities are regarded as an important factor, involved in elevating different pollutants’ ratios in the topsoil of the Dongting basin. Heavy metal distribution causes industrialization and farmland in the Dongting region. Additionally, Xiangjiang river water used for irrigation is suspected as a source of pollutants in the soil around Dongting Lake, which is a constant risk for local food production and the general community (Zhong et al., 2012; Yao et al., 2009). The aquifers beneath urban, industrial, and agricultural areas may become polluted by toxic metals, organic compounds, and major anions and cations that have the potential to deteriorate the water quality and the environment (Vidal et al., 2000; Akhtar et al., 2014). However, the correlation value (r2 = 0.338) calculated the effect of human activities on the concentration of arsenic in the Dongting Basin. Table 2 shows that concentration of arsenic in soil increased with increased landuse frequency. Less than 19% of landuse (construction base) areas were indicated as having an average concentration of arsenic of between 12.9973 and 13.7029 mg/kg, the lowest among five classes, while the 4th class (27%–33%) covered areas had the highest concentration of 16.7367 mg/kg. However, the high percentage of construction distracts from the high arsenic in soil caused by solid waste and effluents. Table 2 revealed that with more agricultural and dry land percentages could affect pollutants’ concentrations, while low arsenic quantity (13.61 mg/kg) was noted in areas with less than 20% vegetated land. By comparing Table 2 and Figure 2C, high population density areas showed a good correlation with elevated arsenic in the soil. The atmospheric arsenic impact on raising the arsenic level in aqueous systems and soils is significant (He and Charlet, 2013).
4.3 Compare concentration of arsenic in three water cycle media
Comparing three areas (SW, Soil, and GW) that are involved in the water cycle can give a deep understanding of toxic arsenic’s presence and mobility. The interaction process may clarify complex pollutant transport concepts at the soil-surface-water-aquifer interface (Shao et al., 2006). Figure 2 shows the significant relationship among concentration of arsenic levels in soil, surface water, and groundwater, where SW and GW contained high concentrations in the east part and low in the west. Other parts of both diagrams (Figures 2A,B) depicted deep closeness regarding concentration of arsenic. This significant resemblance of spatial arsenic distribution identified SW’s impact on GW at Dongting. Fei investigated heavy metal pollutants in the Dongting Plan and observed that cities located near Dongting Lake and the Xiangjiang River contained high concentrations of them because of the close relationship between surface and groundwater (Fei et al., 2013). Concentration of arsenic in GW and soil showed a strong association in some parts in the west, south, and west, while the east part represented a negative relationship. Groundwater systems and topsoil chemistry depend on different hydrogeological parameters and factors which can affect their movement and pollutants’ flow direction. Previous studies observed that anthropogenic factors such as sewage discharge, industrial wastewater discharge, agricultural fertilizer leaching, etc., are major sediment contamination sources (Fei et al., 2013). Heavy metals naturally sink to the bottom of lakes and river sediments and eventually become part of the groundwater system (Yu et al., 2008). When surface water (Figure 2B) and soil (Figure 2C) were compared, a weaker relationship was found between concentration of arsenic s in both interfaces than in others. However, the west and some extended north parts of the study area depicted a high correlation, while the central and eastern regions had contrary arsenic content. Due to the direct interaction of surface water and soil particles, contamination particle exchange is very convenient between both faces. It is normal practice worldwide to deposit solid waste and effluent into soil or surface water resources. Various pollutants from anthropogenic factors have changed soil composition in China (Sodango et al., 2018). Over 80% rivers of China have different level of contamination in surface water (Wong et al., 2002) and elevated levels of arsenic (Sun et al., 2019), which have a direct impact on soil, sediments, and, indirectly, local groundwater systems. From Table 2, population classes showed a significant relationship with arsenic in surface water and groundwater, and the arsenic contamination level in the soil had a trend to increase with population density. Arsenic in three media [soil (15.39 mg/kg), SW (0.2 mg/l), and GW (0.26 mg/l)] contained the highest arsenic values in class 90–120 × 105, whereas the first class (1–10 × 105) contained slightly higher values in soil as compared to other classes. The statistical analysis revealed a high pumping class (28–33) with a good correlation with soil and GW with concentrations of, respectively, 15.2350 mg/kg and 0.0246 mg/l. SW in the middle class (16–22) with an elevated arsenic value of 0.0216 mg/l and a low concentration of 0.0065 mg/l in the first class. Groundwater exploitation appears to be an effective factor in the Dongting Plain for increasing and mobile arsenic in soil and aquifer systems. WL (81–110 m from surface) in soil had a high arsenic impact at 15.54 mg/kg, SW 0.21 mg/l, and GW 0.085 mg/l among the five water level classes. The arsenic trend in soil was mixed with the trend in both water media, and the middle class contained the most elevated concentration of arsenic in the middle class as compared to low and high water levels. The deep aquifer had good quality water in Dongting Plain. Rain is regarded as a major source of groundwater recharge and also affects arsenic transportation from the surface to the aquifer through the water cycle process. Areas were observed where rain intensity between 1,090 and 1,210 mm/year had a high concentration of arsenic in soil (14.8633 mg/kg), SW (0.0203 mg/l), and GW (0.0466 mg/l). Statistical comparison and analyst results revealed that rain’s impact on surface water resources are much higher than on soil and groundwater. Areas with heavy metal contamination with a range of 43–47 mg/kg perceived high arsenic in soil, groundwater, and surface water with values of 15.5240 mg/kg, 0.0216 mg/l, and 0.0241 mg/l, respectively (Table 2). The first and last classes (21–60 m) showed significant variation in concentration of arsenic in three different media. From statistical results, it can be concluded that mountain areas contained high arsenic values while plain surfaces had low arsenic levels. There may be geogenic effects on soil and water. The highest arsenic range of 0.0168–0.0679 mg/l was observed, but the surface water contamination level was slightly lower when compared with other factors. The land use factor was observed in the results of GIS models (Table 1) that had a high equal frequency percentage with soil concentration of arsenic. Table 2 displayed three parameters that identified additional land use activity sources to increase arsenic levels in the soil. Land use had a low or high impact in different classes, but it had a low impact in low percentage range classes. Counties with a construction density of more than 20% gradually increased concentration of arsenic in soil (14.3800 mg/kg) and groundwater (0.0263 mg/l), while surface water had an arsenic pollution level of 0.0245 mg/l. Similarly, the area with green land where agricultural activities and forests are on a large scale is also regarded as a major contamination source of surface water (0.0221 mg/l). A region with a low percentage of dry land had lower concentration of arsenics in three media, but where there was more than 15% of dry land, the arsenic impact was greater. From the above discussion, it can be concluded that arsenic is present in three phases in the Dongting plain, and naturally, these interfaces have their own interaction systems based on various parameters which can transport pollutants from one to another interface. As a result, surface water and soil pollutants have an impact on groundwater quality in the Dongting plain.
4.4 Correlation of arsenic with hydro-geochemical parameters
•A summary of laboratory analysis results for hydro-chemical data and their correlation with concentration of arsenic in soil, surface water, and groundwater from the Dongting plain is presented in Table 2. Maximum arsenic levels in soil (45.69 mg/kg) and groundwater (0.1582 mg/l) were above WHO and Chinese standards, respectively, while a 0.0287 mg/l level in surface water was below the Chinese standard (>0.05 mg/l). However, average concentration of arsenic values in three phases were found within the range of both standards. Variable concentrations of trace amounts (Cr, Fe, Cu, Co, Mg, F, Cu, Mn, and Pb) in soil, surface water, and groundwater were detected (Table 2). The statistical results showed a high correlation in soil (As-Mn = 0.7), SW (As-pH = 0.341), and GW (As-Fe = 0.0792). Arsenic’s correlation with other hydro-geochemical parameters in three phases had significant variation based on concentration range. pH values in groundwater are near acidic to alkaline (pH 5.34–8.7 with an average of 7.239) and in surface water are neutral to alkaline (pH 7.23–8.7 with an average of 7.974). Overall, pH values were within the standard limit suitable for human use. Often, high pH indicates anions’ presence and causes the reduction process to control arsenic mobility (Source: USGS). Arsenic levels were found to be high in waters with pH values greater than 8. pH (8) may be indicative of arsenic desorption due to oxidation of the surface (Smedley and Kinniburgh, 2002). The maximum concentration of Pb was 0.0385 mg/l in groundwater and 0.0144 mg/l in surface water, which were higher than the WHO value of 0.01 mg/l. Furthermore, Pb concentrations in soil were found to be significantly high, at 21.932 mg/kg. The correlation between As-Pb in groundwater was computed at 0.311, while in surface water and soil it was 0.135 and 0.2, respectively. (Put-As-Pb influence) Due to redox-sensitive elements, lower pH conditions can enhance Pb mobility (Mapoma et al., 2014). Arsenic’s correlation with Fe (r2 = 0.16) in soil indicates that arsenic has no significant association with minerals (Xie et al., 2008). The correlation values of Fe with arsenic were computed at 0.792 and 0.01 in GW and SW. Based on the results of geochemical analysis of collected soil samples and statistical calculations, a significant correlation was presented between arsenic with Fe (r2 = 0.16), and SO4 (r2 = −0.25). The correlation of other geochemical parameters was higher than iron, indicating that As had no strong association with iron minerals. The relationship between arsenic and SO4 is consistent with the process of reduction dissolution of iron oxides/oxyhydroxides, as has been shown in other case studies (Pathak et al., 2022), similar findings in Datong Basin, China (Xie et al., 2008). A study in Jianghan Plain show that the spatial heterogeneity of arsenic and iran has good correlation with the local features of geological, hydrological and lithology under the anthropogenic influence and redox conditions (Duan et al., 2015; Ying et al., 2017). Under oxidizing conditions, correlation between Fe-As is common. The reduction of As-bearing Fe oxides can cause the release of arsenic in the long term in a good borehole (Jiang et al., 2022). Interestingly, high concentrations of SO4 (GW: max = 194.063 mg/l; SW: max = 81.85 mg/l) indicated that the slow occurrence of reduction processes in the system for the production of sulfide minerals precipitates (Xie et al., 2008). The arsenic correlation with SO4 in three phases did not have a significant trend. The low concentration of Fe in the phases may be linked to the reduction processes that identify the primary source of groundwater arsenic rather than the Fe/SO4 cycle in Dongting plain. The correlation of Fe and alkalinity with groundwater and surface water demonstrates a strong influence of redox processes on elevated concentration of arsenic. Many studies have proved that elevated Fe concentrations could be due to the reduction process in water (Nur et al., 2019). The suboxic to anoxic conditions of chemical reactions and the correlation of NO3 and SO4 with arsenic could not support a reduction mechanism for the dissolution of Fe in soil. Fe concentration levels in sediments and groundwater are used to predict arsenic mobility and sources. Groundwater statistical results depicted significant correlations between As and Fe (r2 = 0.792), and As with Mn (r2 = 0.382), while the concentration of arsenic in surface water showed a low relationship with Fe (r2 = 0.01), but with Mn (r2 = −0.318). It was observed that a positive correlation between Fe and As indicated reductive dissolution of arsenic linked with Fe oxides under reductive conditions. However, the statistical analysis also indicated a weak correlation between Fe and As, with a significant correlation with Mn as in the Table 2. Reductive dissolution of As-correlated Fe oxides/hydroxides is regarded as the major arsenic mobility controlling process in groundwater (Kumar et al., 2020). The value of r2 with arsenic in surface water and groundwater also provided a low relationship with arsenic mobility. Thus, a consortium of mechanisms such as reductive dissolution and pH-dependent desorption can influence the mineralization of As in the aquifer system of the Dongting plain. The abovementioned arsenic correlation with other hydro-geochemical data proved that there may be some other factors affecting concentration of arsenic in the groundwater system of Dongting basin because the oxidation/reduction mechanism was observed to have a weak relationship. Therefore, other factors were considered to investigate arsenic’s elevated concentration in the groundwater system.
5 Conclusion
In this paper, a study was carried out concerning concentration of arsenic in groundwater, surface water, and soil and investigated their relationships with seven factors in the Dongting basin. Arsenic was diagnosed in three media of the water cycle with a variation in concentration (some higher than the WHO and Chinese prescribed limit). Thus, direct use of groundwater is not recommended for drinking purposes because the shallow water is highly contaminated. The major factors to elevate arsenic in groundwater and surface water in the single, bi-, and triple combined factor models were population and groundwater level, while land use and groundwater pumping in soil. Low value indicates a slow reduction process and the correlation of As-Fe (r2 = 0.16) depicted a weak association of soil with mineralization. The relationship between arsenic and SO4 is consistent with the process of reduction dissolution of iron oxides/oxyhydroxides. So, the arsenic relationship with other hydrochemical parameters revealed that geogenic sources have a minor influence on concentration of arsenic in groundwater, but groundwater and soil have a positive relationship. Modeling techniques on factors and statistical analyses of hydrogeochemical parameters, it was indicated that both anthropogenic and geogenic factors are active in contaminating groundwater. To control high concentration of arsenic in potable water, local water resource managers and planners in the Dongting basin should develop water treatment and alternative water resources. Thus, the urban planning, industrial, environmental and water departments must solve groundwater, surface water, and soil contamination problems by sharing information and future planning.
Data availability statement
The original contributions presented in the study are included in the article/supplementary material, further inquiries can be directed to the corresponding author.
Author contributions
All authors listed have made a substantial, direct, and intellectual contribution to the work and approved it for publication.
Acknowledgments
Authors would like to thank the Geology Survey Bureau of China, which has provided financial support for this research work through project No. 1212011121142.
Conflict of interest
The authors declare that the research was conducted in the absence of any commercial or financial relationships that could be construed as a potential conflict of interest.
Publisher’s note
All claims expressed in this article are solely those of the authors and do not necessarily represent those of their affiliated organizations, or those of the publisher, the editors and the reviewers. Any product that may be evaluated in this article, or claim that may be made by its manufacturer, is not guaranteed or endorsed by the publisher.
References
Akhtar, M. M., Tang, Z., and Mohamadi, B. (2014). Contamination potential assessment of potable groundwater in Lahore. Pakistan. Pol. J. Environ. Stud. 23 (6), 1905–1916.
Akhtar, M. M., Zhonghua, T., Sissou, Z., and Mohamadi, B. (2015). Assess arsenic distribution in groundwater applying GIS in the capital of Punjab, Pakistan. Nat. Hazards Earth Syst. Sci. Discuss. 3 (3), 2119–2147. doi:10.5194/nhessd-3-2119-2015
Ayotte, J., Belaval, M., Olson, S., Burow, K., Flanagan, S., Hinkle, S., et al. (2015). Factors affecting temporal variability of arsenic in groundwater used for drinking water supply in the United States. Sci. Total Environ. 505, 1370–1379. doi:10.1016/j.scitotenv.2014.02.057
Berg, M., Trang, P., Stengel, C., Buschmann, J., Viet, P., Van Dan, N., et al. (2008). Hydrological and sedimentary controls leading to arsenic contamination of groundwater in the Hanoi area, Vietnam: The impact of iron-arsenic ratios, peat, river bank deposits, and excessive groundwater abstraction. Chem. Geol. 249 (1-2), 91–112. doi:10.1016/j.chemgeo.2007.12.007
Bi, B., Liu, X., Guo, X., and Lu, S. (2018). Occurrence and risk assessment of heavy metals in water, sediment, and fish from Dongting Lake, China. Environ. Sci. Pollut. Res. 25 (34), 34076–34090. doi:10.1007/s11356-018-3329-8
Biswas, J. K., Warke, M., Datta, R., and Sarkar, D. (2020). Is arsenic in rice a major human health concern? Curr. Pollut. Rep. 6, 37–42. doi:10.1007/s40726-020-00148-2
Che, F., Jiang, X., Yao, C., Zhao, L., and Wang, K. (2020). Arsenic distribution and speciation in multiphase media of a lake basin, Tibet: The influences of environmental factors on arsenic biogeochemical behavior in the cold arid plateau lake. Sci. Total Environ. 714, 136772. doi:10.1016/j.scitotenv.2020.136772
Chen, Y., Wang, L., Liang, T., Xiao, J., Li, J., Wei, H., et al. (2018). Major ion and dissolved heavy metal geochemistry, distribution, and relationship in the overlying water of Dongting Lake, China. Environ. Geochem. Health 41 (3), 1091–1104. doi:10.1007/s10653-018-0204-y
Chormare, R., and Kumar, M. A. (2022). Environmental health and risk assessment metrics with special mention to biotransfer, bioaccumulation and biomagnification of environmental pollutants. Chemosphere 302, 134836–36. doi:10.1016/j.chemosphere.2022.134836
Cuthbert, M., Burgess, W., and Connell, L. (2002). Constraints on sustainable development of arsenic-bearing aquifers in southern Bangladesh. Part 2: Preliminary models of arsenic variability in pumped groundwater. Geol. Soc. Lond. Spec. Publ. 193 (1), 165–179. doi:10.1144/gsl.sp.2002.193.01.13
Du, Y., Cai, S., Zhang, X., and Zhao, Y. (2001). Interpretation of the environmental change of Dongting Lake, middle reach of Yangtze River, China, by 210Pb measurement and satellite image analysis. Geomorphology 41 (2-3), 171–181. doi:10.1016/s0169-555x(01)00114-3
Duan, Y. H., Gan, Y. Q., Wang, Y. X., Deng, Y. M., Guo, X. X., and Dong, C. J. (2015). Temporal variation of groundwater level and arsenic concentration at Jianghan Plain, central China. J. Geochem. Explor. 149, 106–119. doi:10.1016/j.gexplo.2014.12.001
Erickson, M., Malenda, H., Berquist, E., and Ayotte, J. (2019). Arsenic concentrations after drinking water well installation: Time-varying effects on arsenic mobilization. Sci. Total Environ. 678, 681–691. doi:10.1016/j.scitotenv.2019.04.362
Farooqi, A., Firdous, N., Masuda, H., and Haider, N. (2003). Fluoride and arsenic poisoning in ground water of Kalalanwala area, near Lahore, Pakistan. Geochimica Cosmochimica Acta 67 (18).
Fei, D., Koestler, D., Li, Z., Giambelli, C., Sanchez-Mejias, A., Gosse, J., et al. (2013). Association between in utero arsenic exposure, placental gene expression, and infant birth weight: a US birth cohort study. Environ. Health 12 (1), 58. doi:10.1186/1476-069x-12-58
Fendorf, S., Michael, H., and van Geen, A. (2010). Spatial and temporal variations of groundwater arsenic in south and southeast asia. Science 328 (5982), 1123–1127. doi:10.1126/science.1172974
Firdaus, F., Zafeer, M., Anis, E., Ahmad, M., and Afzal, M. (2018). Ellagic acid attenuates arsenic induced neuro-inflammation and mitochondrial dysfunction associated apoptosis. Toxicol. Rep. 5, 411–417. doi:10.1016/j.toxrep.2018.02.017
Gan, Y., Wang, Y., Duan, Y., Deng, Y., Guo, X., and Ding, X. (2014). Hydrogeochemistry and arsenic contamination of groundwater in the Jianghan Plain, central China. J. Geochem. Explor. 138, 81–93. doi:10.1016/j.gexplo.2013.12.013
Gao, Y., and Mucci, A. (2003). Individual and competitive adsorption of phosphate and arsenate on goethite in artificial seawater. Chem. Geol. 199 (1-2), 91–109. doi:10.1016/s0009-2541(03)00119-0
Gaus, I., Kinniburgh, D., Talbot, J., and Webster, R. (2003). Geostatistical analysis of arsenic concentration in groundwater in Bangladesh using disjunctive kriging. Environ. Geol. 44 (8), 939–948. doi:10.1007/s00254-003-0837-7
Guo, H., Zhong, Z., Lei, M., Xue, X., Wan, X., Zhao, J., et al. (2012). Arsenic uptake from arsenic-contaminated water using hyperaccumulator pteris vittata L.: Effect of chloride, bicarbonate, and arsenic species. Water Air Soil Pollut. 223 (7), 4209–4220. doi:10.1007/s11270-012-1185-6
Guogang, H., Fenglan, J., and Jimin, Y. (1991). 2000 AD: Water environment problems of China. Int. J. Soc. Econ. 18 (8/9/10), 174–179. doi:10.1108/03068299110139224
Harte, P. T., Ayotte, J. D., Hoffman, A., Révész, K. M., Belaval, M., Lamb, S., et al. (2012). Heterogeneous redox conditions, arsenic mobility, and groundwater flow in a fractured-rock aquifer near a waste repository site in New Hampshire, USA. Hydrogeol. J. 20 (6), 1189–1201.
He, J., and Charlet, L. (2013). A review of arsenic presence in China drinking water. J. Hydrol. 492, 79–88.
He, X., Li, P., Ji, Y., Wang, Y., Su, Z., and Elumalai, V. (2020). Groundwater arsenic and fluoride and associated arsenicosis and fluorosis in China: Occurrence, distribution and management. Expo. Health 12 (3), 355–368. doi:10.1007/s12403-020-00347-8
Hossain, M., and Piantanakulchai, M. (2013). Groundwater arsenic contamination risk prediction using GIS and classification tree method. Eng. Geol. 156, 37–45. doi:10.1016/j.enggeo.2013.01.007
Jiang, Z., Zhong, S., Shen, X., Cui, M., Wang, Y., and Li, J. (2022). Microbially mediated arsenic mobilization in the clay layer and underlying aquifer in the Hetao Basin, Inner Mongolia, China. Sci. Total Environ. 836, 155597. doi:10.1016/j.scitotenv.2022.155597
Katz, B., Griffin, D., and Davis, J. (2009). Groundwater quality impacts from the land application of treated municipal wastewater in a large karstic spring basin: Chemical and microbiological indicators. Sci. Total Environ. 407 (8), 2872–2886. doi:10.1016/j.scitotenv.2009.01.022
Kumar, M., Goswami, R., Patel, A., Srivastava, M., and Das, N. (2020). Scenario, perspectives and mechanism of arsenic and fluoride Co-occurrence in the groundwater: A review. Chemosphere 249, 126126. doi:10.1016/j.chemosphere.2020.126126
Lamm, S., Luo, Z., Bo, F., Zhang, G., Zhang, Y., Wilson, R., et al. (2007). An epidemiologic study of arsenic-related skin disorders and skin cancer and the consumption of arsenic-contaminated well waters in huhhot, inner Mongolia, China. Hum. Ecol. Risk Assess. Int. J. 13 (4), 713–746. doi:10.1080/10807030701456528
Li, Y., Bi, Y., Mi, W., Xie, S., and Ji, L. (2021). Land-use change caused by anthropogenic activities increase fluoride and arsenic pollution in groundwater and human health risk. J. Hazard. Mater. 406, 124337–337. doi:10.1016/j.jhazmat.2020.124337
Malik Muhammad, A., and Zhonghua, T. (2014). Municipal solid waste and its relation with groundwater contamination in lahore, Pakistan. Res. J. Appl. Sci. Eng. Technol. 7 (8), 1551–1560. doi:10.19026/rjaset.7.431
Mapoma, H. W., and Xie, X. (2014). Basement and alluvial aquifers of Malawi: An overview of groundwater quality and policies. Afr. J. Environ. Sci. Technol. 8 (3), 190–202.
Mei, Y., and Feng, S. (1993). Water pollution in China: Current status, future trends and countermeasures. Chin. Geogr. Sci. 3 (1), 22–33. doi:10.1007/bf02664590
Ministry of Health of China, Standardization Administration of China (2006). Standards of drinking water quality (GB 5749-2006). China: National Standard of the People’s Republic of China.
Mu, T., Wu, T., Zhou, T., Li, Z., Ouyang, Y., Jiang, J., et al. (2019). Geographical variation in arsenic, cadmium, and lead of soils and rice in the major rice producing regions of China. Sci. Total Environ. 677, 373–381. doi:10.1016/j.scitotenv.2019.04.337
Muhammad, A., Zhonghua, T., Sissou, Z., Mohamadi, B., and Ehsan, M. (2016). Analysis of geological structure and anthropological factors affecting arsenic distribution in the Lahore aquifer, Pakistan. Hydrogeol. J. 24 (7), 1891–1904. doi:10.1007/s10040-016-1453-4
Nur, T., Loganathan, P., Ahmed, M., Johir, M., Nguyen, T., and Vigneswaran, S. (2019). Removing arsenic from water by coprecipitation with iron: Effect of arsenic and iron concentrations and adsorbent incorporation. Chemosphere 226, 431–438. doi:10.1016/j.chemosphere.2019.03.142
Pathak, P., Ghosh, P., Swaraj, A., Yu, T., and Shen, C. (2022). Role of carbon and sulfur biogeochemical cycles on the seasonal arsenic mobilization process in the shallow groundwater of the Bengal aquifer. Appl. Geochem. 141, 105322. doi:10.1016/j.apgeochem.2022.105322
Patle, P. N., Kadu, P. R., Gabhane, A. R., Pharande, A. L., Bhagat, A. P., Bhoyar, S. M., et al. (2019). Consequences provoked due to excess application of agrochemical on soil health deterioration–A review for Sustainable Agriculture. J. Pharmacogn. Phytochemistry 2, 63–66.
Polizzotto, M., Kocar, B., Benner, S., Sampson, M., and Fendorf, S. (2008). Near-surface wetland sediments as a source of arsenic release to ground water in Asia. Nature 454 (7203), 505–508. doi:10.1038/nature07093
Price, R. E., and Pichler, T. (2006). Abundance and mineralogical association of arsenic in the Suwannee Limestone (Florida): Implications for arsenic release during water–rock interaction. Chem. Geol. 228 (1–3), 44–56.
Qian, Y., Zheng, M. H., Gao, L., Zhang, B., Liu, W., Jiao, W., et al. (2005). Heavy metal contamination and its environmental risk assessment in surface sediments from Lake Dongting, People’s Republic of China. Bull Environ. Contam. Toxicol. 75 (1), 204–210.
Rodríguez-Lado, L., Sun, G., Berg, M., Zhang, Q., Xue, H., Zheng, Q., et al. (2013). Groundwater arsenic contamination throughout China. Science 341 (6148), 866–868. doi:10.1126/science.1237484
Shao, M., Tang, X., Zhang, Y., and Li, W. (2006). City clusters in China: air and surface water pollution. Front. Ecol. Environ. 4 (7), 353–361. doi:10.1890/1540-9295(2006)004[0353:ccicaa]2.0.co;2
Smith, E., Naidu, R., and Alston, A. M. (1998). Arsenic in the soil environment. Adv. Agron. 64, 149–195.
Smedley, P. L., and Kinniburgh, D. G. (2002). A review of the source, behaviour and distribution of arsenic in natural waters. Appl. Geochem. 17 (5), 517–568.
Sodango, T., Li, X., Sha, J., and Bao, Z. (2018). Review of the spatial distribution, source and extent of heavy metal pollution of soil in China: Impacts and mitigation approaches. J. Health Pollut. 8 (17), 53–70. doi:10.5696/2156-9614-8.17.53
Song, K., Kim, W., Suh, C., Shin, D., Ko, K., and Ha, K. (2013). Magnetic iron oxide nanoparticles prepared by electrical wire explosion for arsenic removal. Powder Technol. 246, 572–574. doi:10.1016/j.powtec.2013.06.023
Sun, X., Li, B., Han, F., Xiao, E., Xiao, T., and Sun, W. (2019). Impacts of arsenic and antimony Co-contamination on sedimentary microbial communities in rivers with different pollution gradients. Microb. Ecol. 78 (3), 589–602. doi:10.1007/s00248-019-01327-5
Van Geen, A., Ahsan, H., Horneman, A. H., Dhar, R. K., Zheng, Y., Hussain, I., et al. (2002). Promotion, of well-switching to mitigate the current arsenic crisis in Bangladesh. Bull. World Health Organ. 80, 732–737. doi:10.1590/S0042-96862002000900010
Vidal, M., López, A., Santoalla, M., and Valles, V. (2000). Factor analysis for the study of water resources contamination due to the use of livestock slurries as fertilizer. Agric. Water Manag. 45 (1), 1–15. doi:10.1016/s0378-3774(99)00073-6
Wang, Y. X., and Shpeyzer, G. (2000). Hydrogeochemistry of mineral waters from rift systems on the east asia continent case studies in shanxi and baikal. Beijing: China Environmental Science Press. (in Chinese with English abstract).
Waqas, H., Shan, A., Khan, Y., Nawaz, R., Rizwan, M., Rehman, M., et al. (2017). Human health risk assessment of arsenic in groundwater aquifers of Lahore, Pakistan. Hum. Ecol. Risk Assess. Int. J. 23 (4), 836–850. doi:10.1080/10807039.2017.1288561
Wong, S., Li, X., Zhang, G., Qi, S., and Min, Y. (2002). Heavy metals in agricultural soils of the pearl river delta, south China. Environ. Pollut. 119 (1), 33–44. doi:10.1016/s0269-7491(01)00325-6
Wu, Q., Tam, N., Leung, J., Zhou, X., Fu, J., Yao, B., et al. (2014). Ecological risk and pollution history of heavy metals in Nansha mangrove, South China. Ecotoxicol. Environ. Saf. 104, 143–151. doi:10.1016/j.ecoenv.2014.02.017
Xie, X., Wang, Y., Su, C., Liu, H., Duan, M., and Xie, Z. (2008). Arsenic mobilization in shallow aquifers of Datong Basin: Hydrochemical and mineralogical evidences. J. Geochem. Explor. 98 (3), 107–115. doi:10.1016/j.gexplo.2008.01.002
Yao, Z., Bao, Z., Zhou, L., and Gao, P. (2009). A statistical approach for determining the environment impact of surface sediments from the Dongting Lake area, central China. Chin. J. Chem. 28 (1), 97–104.
Ying, S. C., Schaefer, M. V., Cock-Esteb, A., Li, J., and Fendorf, S. (2017). Depth stratification leads to distinct zones of manganese and arsenic contaminated groundwater. Environ. Sci. Technol. 51 (16), 8926–8932. doi:10.1021/acs.est.7b01121
Yu, G., Sun, D., and Zheng, Y. (2007). Health effects of exposure to natural arsenic in groundwater and coal in China: An overview of occurrence. Environ. Health Perspect. 115 (4), 636–642.
Zhang, G., Liu, H., Liu, R., and Qu, J. (2009). Adsorption behavior and mechanism of arsenate at Fe–Mn binary oxide/water interface. J. Hazard. Mater. 168 (2-3), 820–825. doi:10.1016/j.jhazmat.2009.02.137
Zhang, X., Ren, B., Wu, S., Sun, Y., Lin, G., and Chen, B. (2015a). Arbuscular mycorrhizal symbiosis influences arsenic accumulation and speciation in Medicago truncatula L. in arsenic-contaminated soil. Chemosphere 119, 224–230. doi:10.1016/j.chemosphere.2014.06.042
Zhang, X., Zhong, T., Chen, D., Cheng, M., Liu, L., Zhang, X., et al. (2015b). Assessment of arsenic (As) occurrence in arable soil and its related health risk in China. Environ. Geochem. Health 38 (3), 691–702. doi:10.1007/s10653-015-9751-7
Keywords: GIS, remote sensing, geostatistical analyst, arsenic, Dongting plain
Citation: Malik Muhammad A, Tang Z and Xiao T (2022) Evaluation of the factors affecting arsenic distribution using geospatial analysis techniques in Dongting Plain, China. Front. Environ. Sci. 10:1024220. doi: 10.3389/fenvs.2022.1024220
Received: 21 August 2022; Accepted: 22 September 2022;
Published: 10 October 2022.
Edited by:
Muhammad Tayyab Sohail, Xiangtan University, ChinaReviewed by:
Ammar Dawood, University of Basrah, IraqDevanita Ghosh, Delft University of Technology, Netherlands
Copyright © 2022 Malik Muhammad, Tang and Xiao. This is an open-access article distributed under the terms of the Creative Commons Attribution License (CC BY). The use, distribution or reproduction in other forums is permitted, provided the original author(s) and the copyright owner(s) are credited and that the original publication in this journal is cited, in accordance with accepted academic practice. No use, distribution or reproduction is permitted which does not comply with these terms.
*Correspondence: Akhtar Malik Muhammad, ZHJtYWxpa21hMjFAZ21haWwuY29t; Zhonghua Tang, emhodGFuZ0BjdWcuZWR1LmNu