- 1Department of Environmental Science, Faculty of Basic and Applied Sciences, International Islamic University, Islamabad, Pakistan
- 2Pakistan Council of Research in Water Resources, Islamabad, Pakistan
- 3Research Center for Environment and Society, Hohai University, Nanjing, China
- 4School of Public Administration, Hohai University, Nanjing, China
- 5National Institute of Maritime Affairs, Bahria University, Islamabad, Pakistan
- 6Department of Sociology, University of Chakwal, Chakwal, Pakistan
- 7College of Engineering, IT & Environment, Charles Darwin University, Darwin, NT, Australia
- 8College of Engineering and Science, Victoria University, Melbourne, VIC, Australia
This study applied a nuclear technique in conjunction with a classical monitoring tool to characterize the origin, fate, and behavior of metal pollutants in groundwater of Islamabad-Rawalpindi Metropolitans, which are also known as the “twin cities.” In total, 122 groundwater samples were collected and analyzed in accordance with standard methods. GIS and multivariate statistical analysis were employed for the groundwater vulnerability assessment and source apportionment. The results of the aesthetic parameters indicated that the majority of groundwater sources were tested and were colorless, odorless and tasteless in the “twin cities.” In addition, the findings of this study indicated that the concentration of pH, phosphates, copper, manganese, and zinc were within the drinking water standards in the “twin cities” as stipulated by the World Health Organization (WHO) and Pakistan Standard and Quality Control Authority (PSQCA) at all sampling points in the study area. The groundwater quality was found unsuitable for consumption due to elevated levels of electrical conductivity and total dissolved solids at 9.83% and 4.09% of samples, respectively. The contents of arsenic and fluoride were well within the allowable range at almost all points except at one location. However, iron and lead contents were above permissible limits. A statistical analysis revealed that trace metals originated from both geogenic and anthropogenic sources such as enhanced rock-water interaction, over abstraction, evaporation enrichment, improper waste disposal, discarded batteries, cross contamination of water supply and sewerage lines, active recharge from Lie drain, and domestic, industrial, and agricultural effluents. The computed water quality index (WQI) based on heavy metals elucidated that groundwater quality was poor in most of the study area due to elevated electrical conductivity, total dissolved solids, lead, iron, arsenic, and fluoride values. A highly depleted isotopic composition of 13C provides clues about the aquifer’s vulnerability from miscellaneous sources such as domestic, urban, construction, and agricultural sites and the dissolution of carbonate minerals. This study clearly indicates that a rapidly growing population, unplanned urbanization, industrialization, improper waste disposal, over abstraction, and a lack of water abstraction policies are significantly contributing toward the impairment of groundwater quality in the study area. The study strongly emphasized the need to regulate groundwater abstraction by improving water treatment and the supply system for the provision of safe water to the urban populace. These results will help in designing remedial strategies for improving water quality in the “twin cities.”
Introduction
Groundwater constitutes an elementary source of the water supply as approximately 99.97% of total freshwater that is readily available is found in aquifers across the globe (Khatri et al., 2020). Nearly 50% of the urban water supply in developing countries is obtained from groundwater (open wells, tube wells, springs, and bore wells). Roughly 1000 million people in Asia and 150 million in Latin America meet their water demands from such resources (Rizwan et al., 2009). Reliance on groundwater systems is significantly escalating in most of the arid and semi-arid areas in the world as a consequence of variations in rainfall patterns, climate-induced changes (extended droughts and high evaporation rates), and the irregularity in surface water flows (Kumar Sundaray et al., 2006; Sharma et al., 2021). This also holds true for Pakistan, which is cataloged as a highly arid and water-stressed country and is expected to become water scarce in the near future (Rizwan et al., 2009).
The occurrence of heavy metals has become a global contemporary dilemma since these potential toxicants have wide sources, a very long biological half-life, and possess indestructible properties and an accumulative nature (Boateng et al., 2015; Mashiatullah et al., 2016; Rahman et al., 2020; Ganiyu et al., 2021; Varol et al., 2022). Additionally, metals cannot be eliminated from the groundwater system as a result of self-cleaning and can lead to geo-accumulation, bioaccumulation, and bio-magnification (Ward, 1995; Loska and Wiechuła, 2003; Ustaoğlu and Aydın, 2020). For this reason, the occurrence of heavy metals in urban groundwater supplies above permissible limits is extremely undesirable due to their toxic effects (Malassa et al., 2014) and creates high ecological apprehension for environmentalists, health practitioners, and decision-makers (Vetrimurugan et al., 2017). Furthermore, there are more than fifty elements that are cataloged as heavy metals, but seventeen elements are of particular concern due to extreme toxicity and accessibility even at low concentrations (Rahman et al., 2020). Metals are trace constitutes of the natural environment and some metals make up essential components of the diet and are considered indispensable for maintaining good health. However, heavy metals at elevated levels can lead to acute or chronic toxicity. Anthropogenic activities have significantly increased heavy metal contents in groundwater systems, predominantly from industries, agricultural and domestic effluents, landfill sites, improper solid waste disposal, and mining (Varol et al., 2022). The consumption of such water sources for domestic and drinking purposes can pose health risks for humans and the ecosystem (Sharma et al., 2021). Since 1900 AD, over 90% of metal pollution has been reported from anthropogenic sources due to industrial and technological progress (Nriagu, 1992).
Heavy metals and hazardous trace elements can enter the human body through a variety of routes including inhalation, dermal contact, and ingestion. Drinking water polluted with toxic metals can pose numerous carcinogenic and non-carcinogenic risks to the human populace (Asim and Rao, 2021). For example, elevated levels of heavy metals like chromium, cadmium, mercury, lead, zinc, copper, cobalt, fluoride, arsenic, and nickel in drinking water may result in diseases like tremors, impaired vision and hearing, paralysis, insomnia, emotional instability, developmental deficits, nerve damage, forgetfulness, gastrointestinal distress, blood disorders, muscular pain, liver problems, renal dysfunction, lung cancer, and bone degeneration (Duruibe et al., 2007; WHO, 2017). Groundwater with a high fluoride content is found in different parts of Pakistan such as the Salt Range, Kasur, and Mianwali. There are also reports of high fluoride content ranging from 65 to 12 mg/L in groundwater of Bahawalpur. An analysis of groundwater in Jhelum, Gujarat, and Sargodha districts have shown concentrations of arsenic well above the WHO permissible limit of 10 μg/L (PCRWR, 2016). A recent study conducted in 11 cities of Punjab demonstrated an excess of arsenic and fluoride concentrations in the water supply systems of six cities (Multan, Bahawalpur, Sheikhupura, Kasur, Gujranwala, and Lahore). Alarmingly, over 2 million people in these cities are consuming arsenic-contaminated groundwater (PCRWR, 2016). Currently, the presence of elevated levels of nitrates, microbes, arsenic, and fluoride ions in the water supply are considered major pollutants in most parts of the country. Moreover, different research studies indicate that various physiochemical parameters of water supply obtained from major cities are not apt for drinking purpose as per WHO/PSQCA standards (PCRWR, 2016). A water quality monitoring survey conducted by the Pakistan Council of Research on Water Resources (PCRWR) in 2016 covering 23 major cities of Pakistan, indicated that out of a total of 369 monitored sites, only 31% (116) of sources were found safe and the remaining 69% (253) were unsafe for drinking purposes. Findings also revealed that in the capital city of Islamabad, only 32% of sources were supplying safe water while 68% were found unsafe for drinking purposes. Similarly, in Rawalpindi, 62% of sources were found unfit for drinking (PCRWR, 2016).
As per WHO reports, 80% of diseases were associated with the consumption of contaminated water in third world countries (Rahman et al., 2018; Töre et al., 2021). Groundwater utilized for drinking purposes should be devoid of hazardous substances and aesthetically pleasing to ensure good health (Iqbal et al., 2009). Accurate estimation of parameters, such as aesthetic, pH, EC, TDS, and metals, can offer valuable clues about groundwater quality. Therefore, periodic monitoring of public and private groundwater sources in rapidly growing urban areas is essential to ensure their suitability for drinking purposes (Khatri et al., 2020; Yüksel et al., 2021).
Environmental isotopes (carbon, oxygen, and hydrogen) are an extremely valuable tool in hydrological studies for the estimation of water quality, recharge mechanisms, rock-water interactions, and source apportionment (Mashiatullah et al., 2016; Ogrinc et al., 2019). Carbon-13 (13C) is a natural, stable isotope of carbon that makes up to 1.1% of the total carbon on Earth. In environmental analysis, stable carbon isotopes are used as a natural tracer of domestic and industrial pollution (Qureshi et al., 1998) (see Supplementary Material S2). The δ13CDIC values can identify different sources of carbon that contribute to the aquifers’ carbon pools including organic and inorganic carbon species (Nowak et al., 2017). The stable isotope of carbon offers a complementary tool to infer reliable information about the sources, distribution, and fate of hydro-pollutants. Therefore, the stable carbon isotope (13C) has the potential to generate pollution inventories in the aquatic environment including aquifer systems (Ahmad, 2002).
Previously, attempts were undertaken to determine the groundwater pollution status in Rawalpindi and Islamabad (which are referred to as the “twin cities” due to adjacent location and interconnectivity) and were mostly limited to the use of conventional techniques and there has been no integrated study covering greater sampling size using a heavy metal-based water quality index, statistical, and GIS tools (Sajjad, 1998; Munir et al., 2011; Mehmood et al., 2013; Shabbir & Ahmad, 2015). Therefore, this study was undertaken to apply a nuclear technique (stable isotopic technique) in conjunction with a classical monitoring tool to characterize the origin, fate, and behavior of metal pollutants in the groundwater system of the metropolitan area of Islamabad and Rawalpindi. The spatial distribution maps of metals and stable carbon isotopes highlighted hotspot areas to accurately evaluate groundwater vulnerability in the “twin cities.” Moreover, multivariate statistical analysis, i.e., the correlation coefficient (r2), cluster analysis (CA), and factor analysis (FA), were performed in MS Statistica (version 7.0) for source apportionment and provided valuable information about the potential sources of pollution within the studied basin. The monitoring results led to remedial measures for improving the existing water quality situation in the “twin cities.”
Materials and methods
Study area description
Islamabad-Rawalpindi Metropolitan, which is commonly known as the “twin cities,” was selected as the study area for this research. The “twin cities” of Islamabad and Rawalpindi are located in the northernmost part of Potwar Plateau that encompasses an area of approximately 906.50 km2 and 259 km2, respectively (Ghaffar et al., 2017). Geographically, it is located at a latitude of 33.662883° N and a longitude of 73.086373° E (Figure 1). Islamabad is the capital city while Rawalpindi is situated in the Punjab Province of Pakistan (Mannan et al., 2019; Atif Bokhari et al., 2022). The city of Islamabad is divided into numerous sectors that cover a total area of two square kilometers and each sector is further subdivided into four sub-sectors. For administrative purposes, Islamabad is divided into eight basic zones (administrative, diplomatic enclave, residential areas, educational sectors, industrial sectors, commercial areas, rural areas, and green areas). However, Rawalpindi is administratively divided into 46 union councils (Jamil Butt et al., 2012). The total estimated population of the third largest metropolitan region of Pakistan is more than 5.0 million with nearly 2.01 million and 3.00 million people dwelling in Islamabad and Rawalpindi, respectively (GoP, 2021).
The climate of the “twin cities” is humid to semi-arid with moderate summers and winters. The temperature rises to a maximum of 45 °C during the summer season and drops to a minimum of −3 °C during the winter season (Mannan et al., 2019). The annual rainfall in the “twin cities” occurs due to a prevailing summer monsoon and a western depression during winters and usually fluctuates between 900 and 1000 mm (Ghaffar et al., 2017). The surface hydrology of the study area is characterized by the Korang and Soan Rivers that are fed through numerous perennial and non-perennial streams (Sajjad, 1998; Mashiatullah et al., 2010). Lei Nullah is the longest natural drain originating from Margallah Hills, Islamabad, and then meanders through the center of Rawalpindi (Abbas et al., 2014). Geologically, the study area is composed of sedimentary rocks (limestone, sandstone, conglomerate, maroon, and red shale) and sediments (gravel, alluvial fill, and wind-blown silt), which represent the Eocene to the current age. These consolidated deposits do not function as an aquifer and infiltrated water can only travel through the joints, cracks, and fissures present in rocks. For this reason, groundwater resources in the Islamabad-Rawalpindi area are mostly found in uncemented conglomerate beds (terrace gravel, alluvial, and fractured hard rock) (Sheikh et al., 2007). A large number of deep tube wells (200 m or above) mostly extract groundwater from the quaternary alluvial gravel aquifer at a rate of 18–22 h per day (Ahmad et al., 2016). The majority of the study area falls into the semi-arid category; therefore, residents of the “twin cities” depend on the groundwater supply (Sajjad, 1998; Munir et al., 2011; Ghaffar et al., 2017). The main industries of the “twin cities” include petroleum and petrochemical, textiles, food and beverages, chemical products, marble factories, steel and re-rolling mills, edible ghee and oil, flour mills, a cement factory, basic metals, and electrical machinery (UN-Habitat, 2018). The study area map is shown in Figure 1.
Sampling procedure
Systematic grid sampling was conducted to collect groundwater samples from the “twin cities” during two sampling campaigns (Feb-March and July-September 2020). Groundwater samples were collected in a pre-cleaned 1500 ml plastic bottle for physiochemical and metal analysis. Groundwater samples were collected in opaque 1000 ml doubled capped HDPE bottles for stable isotopic analysis. Moreover, HgCl2 was added in groundwater samples to inhibit microbial activity and were completely filled with source water to eliminate headspace. In total, 122 groundwater samples were collected from purged tube wells and bore wells using standard field sample collection/preservation methods.
All samples were properly sealed, labeled, and transported to the National Water Quality Laboratory (NWQL)-PCRWR, Islamabad, and properly stored. The geographical coordinates of each sampling site were recorded with a hand-held Global Positioning System (GPS) (GPSmap 62s GARMIN). Field notes were collected on a performa and records were further maintained to establish a detailed sample inventory. Basic physicochemical parameters like pH, temperature, total dissolved solids (TDS), electrical conductivity (EC), and aesthetic parameters were measured within 24 h of sampling in accordance with standard procedures (APHA, 2012).
For stable environmental isotopic analysis of δ13C in groundwater, samples were properly stored in accordance with an International Atomic Energy Agency (IAEA) prescribed protocol. Further analysis was conducted at the Environmental Lab of Isotope Application Division (IAD), Pakistan Institute of Nuclear Science and Technology (PINSTECH), Islamabad.
Lab analysis
The pH of all water samples was measured using a digital pH meter (Hanna Model 210, Italy). Electrical conductivity (EC) and total dissolved solids (TDS) of all water samples were measured with a digital EC meter (Hach-44600, United States, Jenway 4320). Aesthetic parameters (color, taste, and odor) of all groundwater samples were recorded using human subjective evaluation during the field survey (Uzaira et al., 2005). The fluoride concentration was measured in all groundwater samples through the SPANDS method (HACH DR 2800) at a wavelength of 195. The iron concentration was analyzed through iron pillows using a colorimeter (HACH DR 2800).
The phosphate content was assessed using phosphate pillows and using a colorimeter (HACH, DR/890 Colorimeter). Prior to analysis, all instruments were duly calibrated in accordance with the manufacturer’s instructions. The arsenic concentration was measured in the groundwater of the study area through an Atomic Absorption Spectrophotometer (AAS) (Analytik Jena Zeenit 700, Germany) equipped with a Hydride Generation Mode using Argon gas. The contents of the copper, zinc, and manganese were also determined in groundwater samples collected from the “twin cities” through an Atomic Absorption Spectrophotometer (Analytik Jena Zeenit 700, Germany) by direct aspiration into Air-Acetylene flame (See Supplementary Material S1). Lead in the groundwater was also analyzed using the AAS graphite mode equipped with an auto-sampler.
All metals were analyzed under standard operating conditions with r > 0.999 (See Supplementary Material S1, S2 for details). Prior to analysis, AAS was calibrated with standard solutions for each metal as described in the manufacturer’s manual. All reagents used during lab analysis were of analytical grade. Double deionized water (DDW) was used throughout the experimental work. Moreover, the overall accuracy of the measurement technique was maintained through analyzing standards and a blank after every fifteen samples. Results of Cu, Mn, and Zn were reported in mg/L and As and Pb in µg/L.
The stable isotope of carbon offers a complementary tool to infer reliable information about the sources, distribution, and fate of hydro-pollutants. Therefore, stable carbon isotopes (13C) have a powerful potential to generate pollution inventories in the aquatic environment including aquifer systems. Stable carbon isotope (13C) analysis of total dissolved inorganic carbon (TDIC) was carried out by converting inorganic carbon to carbon dioxide (CO2) with 100% phosphoric acid (H3PO4), followed by cryogenic separation and further analysis through a modified gas source Mass Spectrometer (Varian Mat GD-150). All values of the stable carbon isotope (13C) were reported as δ (delta) per mil (‰) against international carbonate standard, namely, Pee Dee Belemnite (PDB) (Dassi and Tarki, 2014). The overall analytical error for each measurement of the stable carbon isotope (δ13 C) was ±0.1‰.
where R = 13C/12C ratio, S = unknown sample, and St = known standard or reference material.
Statistical analysis was employed to present the analytical findings of the study. All descriptive statistical analyses (maximum (Max), minimum (Min), mean, variance (var), kurtosis, skewness, standard deviation (SD), and standard error (SE)) were performed in XL stat (Windows 7). Multivariate statistical analysis, viz., correlation coefficient (r2), cluster analysis (CA), and factor analysis (FA), were performed in MS Statistica (Version 7.0) for source apportionment. The water quality index (WQI) based on heavy metal was computed to provide a comprehensive picture of the geochemical alterations in groundwater of the “twin cities.” Box and Whiskers of all parameters were plotted using MS Statistica software (Stat Soft Inc., 2020). Spatial maps of all studied parameters were produced through GIS.
Geostatistical analysis
Point data interpolation was conducted for all the given parameters. The similarity of the nearby points helps in the estimation of unsampled points of the study area and is the key for all interpolation methods. In this study, a kriging method was applied, which assumes that nearby points are similar to each other compared to those that are far apart. This model is based on a univariate linear regression model for points. This linear weighted averaging method not only depends on the distance between the points but also on the direction and orientation of the adjacent data on non-sampled locations.
Results and discussion
All groundwater samples collected from Rawalpindi and Islamabad (“twin cities”) were analyzed statistically and the results are presented in Table 1.
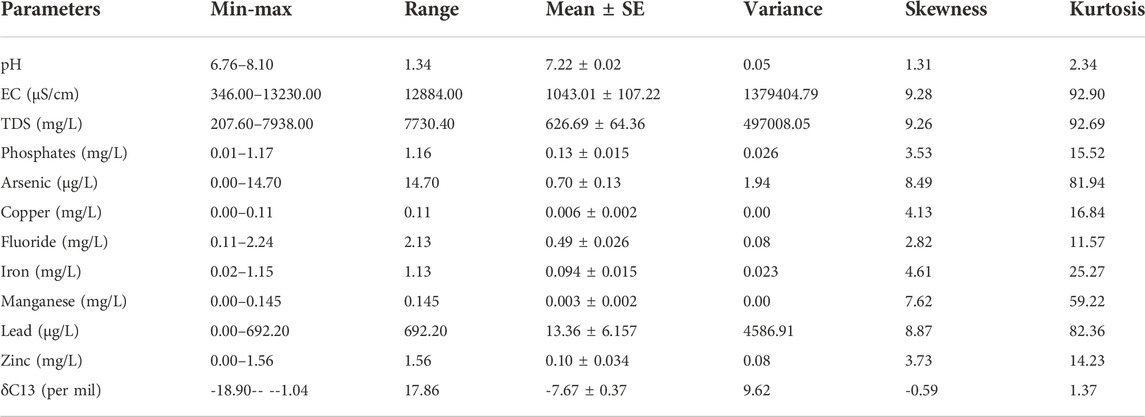
TABLE 1. Statistical summary of the physiochemical, heavy metal, and stable carbon isotope composition in groundwater samples of the “twin cities.”
Groundwater characterization
Aesthetic parameters are the basic indicators of environmental pollution and help protect the water system. The supply of aesthetically unpleasant water can lead to the use of less safe but more appealing water resources. There are three main aesthetic parameters used for water quality assessment, i.e., color, taste, and odor. The color of the water is one of the most important and conveniently observed indicators of water quality. Naturally occurring water is colorless. Dissolved organic matter, such as humic acid and fulvic acid, imparts a dull yellow color. Colored water may be harmless but can make the water detrimental for various uses (Asthana and Asthana, 2003). The majority of the groundwater sources (79.50%) collected from the “twin cities” were found to be colorless, while 20.49% of sources had colored water.
The major colors monitored in groundwater sources were slightly muddy, muddy, turbid having white suspended particles, cloudy having black particles, slightly yellow, and yellowish brown and were due to the geologic conditions, prevailing seasons, and depth of the water table. Moreover, most color was detected in groundwater sources collected from bore wells as compared to tube wells which is correlated well with the seasons and water table depth. A number of organic and inorganic substances contribute to the odor and taste in groundwater. Moreover, iron (Fe) and manganese (Mn) also impart an objectionable color and smell in water (Kahlown et al., 2008a).
The findings of this study indicate that the majority of the tested groundwater sources (84.42%) were odorless. Conversely, 19 (15.57%) groundwater sources had different odors like chlorinated, earthy, grassy, and muddy during the study period. The results of this study indicated that most of the tested groundwater sources (88.52%) were tasteless. In contrast, only a few groundwater sources (11.47%) reported objectionable tastes like chlorinated, earthy, grassy, salty, and metallic.
The pH water quality parameter is a measure of the intensity of the acidic or basic character of water and roughly represents the concentration of hydrogen ions (H+) (APHA, 2012). The pH values of all groundwater samples ranged between 6.76–8.12 with a mean value of 7.21 in the “twin cities” of Rawalpindi and Islamabad. The lowest pH value was recorded in Afshan Colony Misrial Road and the highest was in IIUI Hostel near Faisal Mosque. The results indicate that the groundwater in the “twin cities” is slightly acidic to alkaline in nature and corresponds to the geologic conditions.
Lower and near neutral pH values were reported due to the buffering of carbonate ions, while higher pH values in groundwater samples were due to either anthropogenic influence or the leaching of anions (Shabbir and Ahmad, 2015; Ghaffar et al., 2017). Moreover, pH values at all localities lie within safe limits, i.e., neutral and slightly alkaline (Figure 2) as prescribed by the WHO, 2017, and PSQCA, 2008 (6.5–8.5).
The EC values vary significantly from 346 to 13230.0 μS/cm with a mean value of 1045.74 μS/cm in Rawalpindi and Islamabad (Table 1). The elevated EC values indicate enrichment of soluble salts in the groundwater of the “twin cities.” The recharge mechanism of Lie Nullah, domestic and industrial effluents, and high abstraction rates coupled with semi-arid climatic conditions and high evaporation rates are the most probable source for the enrichment of EC (Islam-ul-haq et al., 2008; Krishna Kumar et al., 2015; Shabbir and Ahmad, 2016). The most desirable range prescribed for EC in drinking water is 1400 μS/cm (WHO, 2017). Accordingly, 9.83% of the total samples collected from the “twin cities” were beyond the permissible range and approximately 90% of the area had good quality groundwater for drinking that lies in the permissible and desirable range (Figure 2). Moreover, a classification based on the EC/enrichment of salt developed by Prasanth et al. (2012) and Dey (2012) is presented in Table 2. In accordance with this interpretation, 91.80% of the total groundwater samples fall under Type I (low enrichment), 7.37% of the samples under Type II (medium enrichment), and 0.82% (high enrichment) under Type III (RC-49) classifications. These results agree well with previous findings (Shabbir and Ahmad, 2015; Ghaffar et al., 2017). Type I and Type II of EC relates to the desirable and permissible limits while Type III relates to the unsuitable limit as shown in the spatial distribution map (Figure 2).
The TDS values ranged from 207.60 to 7938 mg/L (Table 1) with a mean value of 628.92 mg/L in Rawalpindi and Islamabad metropolitan. The high TDS content examined in groundwater has been associated with natural sources (rock-water interaction and recharge conditions) as well as anthropogenic influences such as sewage and urban, agriculture, and industrial runoff and chemicals used in the water treatment process (Irfan et al., 2020). The WHO (WHO, 2017) guideline value is 1000 mg/L for TDS, which is shown in the low and medium range in the spatial distribution map, while 4.09% of samples deviate from this standard as shown with the high range in Figure 2. A water quality assessment based on TDS (mg/L), as proposed by Prasanth et al. (2012) and Dey (2012), was carried out. The interpretation of groundwater quality based on TDS explains that the majority of the samples are categorized as fresh (95.90%) and only a few samples are categorized as brackish (4.09%) (Table 3).
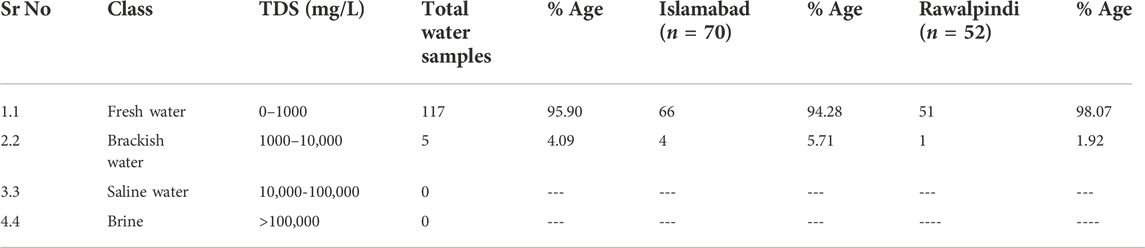
TABLE 3. Water quality assessment based on total dissolved solids (TDS) (mg/L) (Todd, 1980) n = 122.
Phosphates are natural constituents of groundwater derived from the dissolution of P-containing rocks and often exist solely as phosphates. Phosphate ions are cataloged as an “essential nutrient” for plant growth. It is considered a limiting factor and its content is usually found below 0.03 mg/L due to low solubility and the adherence capability to soil particles (Todd, 1980). Therefore, phosphates are vital components of synthetic fertilizers that are extensively used in agriculture. For this reason, the elementary anthropogenic sources of PO4−3 in groundwater are percolation and runoff from agricultural lands and cattle feedlots (Kahlown, et al., 2008b). Additional inputs come from sewage and industrial effluents (Shah, 2007; Mehmood et al., 2013; Srinivasamoorthy et al., 2014; Cüce et al., 2022). The phosphate contents significantly varied between 0.010 and 1.170 mg/L with a mean value of 0.131 mg/L in Rawalpindi and Islamabad. The maximum concentration for phosphates was noted in the groundwater of Bander Stop, Japan Road, and a minimum concentration was found at seven different locations in the “twin cities” (I-8/4, Convention Center, Capital Enclave, Rawal Town, Sports Complex, Mangral Town, and Khayaban-e-Saryad). The results clearly revealed that all groundwater samples recorded lower phosphates (<1 mg/L) except one site due to geological and aerobic conditions in aquifers of the Rawalpindi and Islamabad region. Nevertheless, the health risk associated with the intake of phosphate ions is not well understood in humans. However, elevated levels are most commonly associated with cultural eutrophication (Dohare et al., 2014). No guideline value is prescribed by the World Health Organization (WHO) for this parameter in drinking water. In some regions across the globe, natural geology or soil may have elevated background concentrations for total phosphates such as the Tualatin River in Oregon, United States (Salma, 2011; Khatri et al., 2020; Tokatlı et al., 2022). Somewhat lower values for phosphate were reported by Mehmood et al. (2013) in the groundwater of Rawalpindi and Islamabad (0.02 mg/L) compared to this study. However, in other studies in India, higher values were reported (Srinivasamoorthy et al., 2014; Töre et al., 2021). The spatial distribution of phosphates is plotted in Figure 3. The spatial interpolation of heavy metals is depicted in Figure 4.
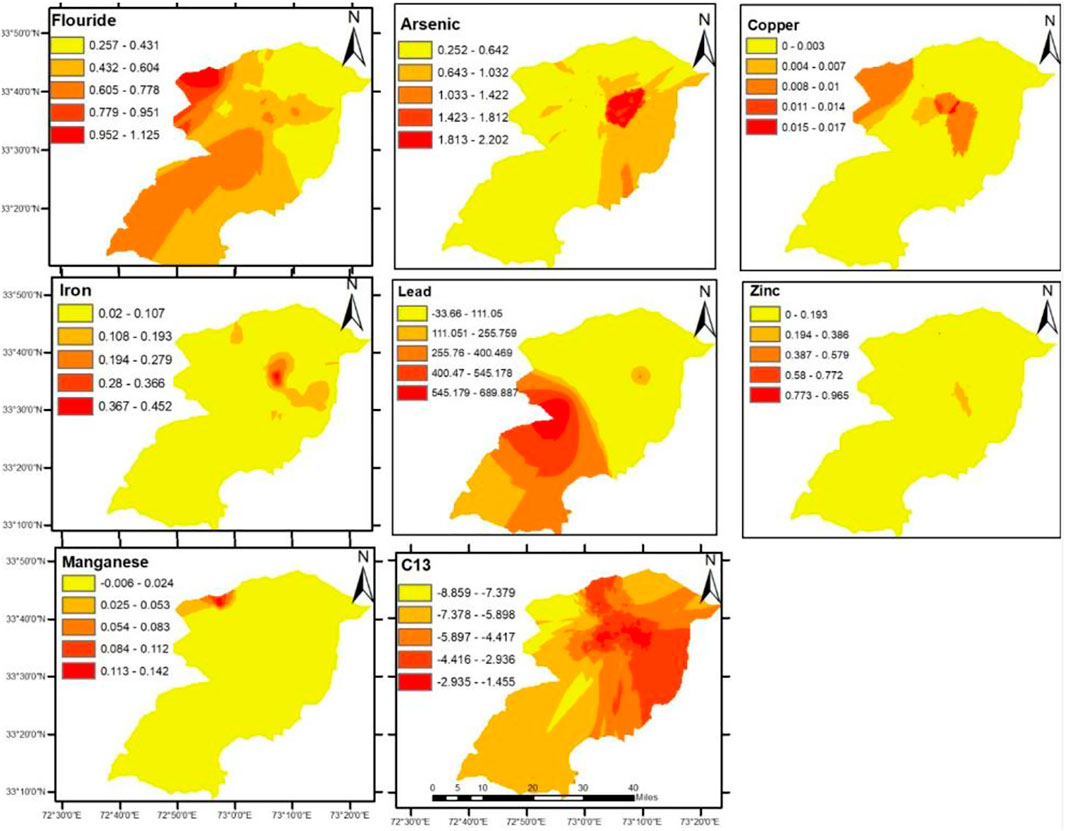
FIGURE 4. Spatial distribution of heavy metals and δC13 in the groundwater of the “twin cities” of Rawalpindi and Islamabad.
Heavy metal distribution in groundwater of Islamabad-Rawalpindi
Arsenic is a naturally occurring element found in the earth’s crust. It is extensively distributed in the ground and surface water system as a consequence of erosion and weathering. Arsenic is commonly present in different rocks such as shale, clay, coal, iron, and manganese ores as well as in active geothermal areas. Arsenic does not result in an undesirable color, taste, or odor in water (Kahlown and Majeed, 2005). In addition to the dissolution of As-bearing rocks, another dominant source of ‘As’ leaching in aquifers in arid and semi-arid regions is the presence of an evaporative climate. Apart from geogenic sources, ‘As’ pollution is also the result of anthropogenic activities such as over abstraction of groundwater. It is widely used in the manufacturing of industrial products such as agrochemicals like pesticides and insecticides, alloying agents, paints, wood preservatives, glass processing, and pharmaceuticals. Therefore, leaching of As from agricultural, industrial, and urban settings can be another potential source in groundwater (Sharma et al., 2021).
Exposure to elevated levels can cause serious health risks like stomach ache, nausea, vomiting, diarrhea, coma, shock, cancer, and even death. In addition, humans with suppressed immune systems and infants are more vulnerable to arsenic toxicity (Khalown and Majeed, 2005; Tokatlı et al., 2022). The concentration of arsenic ranged from 0 to 14.74 μg/L with a mean value of 0.70 μg/L in the Rawalpindi and Islamabad metropolitan areas. The results revealed that ‘As’ concentrations were found below detectable limits (BDL) at 12 sites (9.83%) in the “twin cities.” The WHO has a prescribed guideline value of 10 μg/L for ‘As’ in drinking water. All groundwater samples had ‘As’ well below the permissible limit except one sample. Groundwater obtained from the Judicial Colony had anonymously high ‘As’ content (14.74 μg/L) above the WHO permissible range but well within the limit prescribed by PSQCA, 2008 (50.0 μg/L). The occurrence of As-containing underlain rocks and its dissolution in groundwater may be the prime source of elevated arsenic contents at this site.
These findings are in agreement with previous studies conducted by PCRWR (2001-2016) in the “twin cities” and by Jabeen et al. (2014) and Anwar et al. (2014) in Haripur Basin, Pakistan. However, groundwater obtained from Punjab (Bahawalpur, Multan, and Sheikhupura) was found to be highly polluted with As (PCRWR, 2016). However, higher values for As (200–1260 μg/L) were reported by Sharma et al. (2021) in the Mansa District, India, and (Rahman et al., 2018) in Gopalganj, Bangladesh, during the pre- (5–198 μg/L) and post-monsoon (5–102.5 μg/L) timeframes compared to this study.
Copper is a trace metal that occurs naturally in the environment. The concentration of copper is usually low in ground and surface water but the quantities can be significantly increased due to anthropogenic inputs such as mining, farming, municipal, industrial, and urban wastewater discharge in water channels. Copper enters groundwater supplies either directly through infiltration or indirectly through corrosion of pipelines due to acidic water. Exposure to an elevated copper dose can damage vital organs like the kidneys, liver, and intestines (Ammara and Nawaz, 2014). Drinking waters with Cu contents above permissible limits can cause the mental syndrome Alzheimer’s disease (Jabeen et al., 2014). It is also toxic to living organisms even at low concentrations. The concentration of Cu in the study area varied between 0.00 and 0.106 mg/L with a mean value of 0.006 mg/L. Cu levels were not detected at the majority of sampling sites (85.33%). The maximum Cu contents were observed at Chah Sultan (RC-18) near the Rawalpindi Institute of Cardiology (RIC), Rawalpindi, probably due to the presence of automobile workshops and the breaking of old automobiles and scrap markets in the vicinity of the sampling location. In addition, results highlighted that Cu concentrations were recorded at 18.66% in groundwater obtained from areas impacted by industrial, urban, and agricultural activities. The WHO guideline value of 1 mg/L in drinking water and all groundwater samples occurred within safe limits. The results revealed that copper was below detectable limits at most of the sites and hence not problematic for potable water supply in Rawalpindi and Islamabad metropolitan areas. The findings of this study were in good agreement with a study conducted by Boateng et al. (2015) in Ejisu-Juaben, Ghana (Boateng et al., 2015; Saana et al., 2016), and in Sialkot City (Rizwan et al., 2009).
Fluorides are widely and naturally distributed in both surface and groundwater. However, groundwater aquifers may have elevated fluoride contents above 10 mg/L when located in regions where F-containing rocks and minerals (such as fluorspar, fluorite, triphite, phosphorite, and rock phosphate) are common. Moreover, the fluoride concentration is largely governed by climatic conditions and the nature of accessory minerals present in the host environment through which subsurface water is circulated (British Geological Survey, 2001; Boateng et al., 2015). WHO (2017) reported drinking water as the prime source of daily fluoride intake. Fluorides provide effective protection against dental caries if found in fairly low quantities (<1 mg/L) in the drinking water supply. Conversely, prolonged exposure to elevated doses (>1.5 mg/L) is often related with the prevalence of dental fluorosis and even skeleton fluorosis. In certain cases, chronic ingestion can lead to cancer.
The concentration of fluoride in groundwater varied widely from 0.11 to 2.24 mg/L with a mean value of 0.489 mg/L in the Rawalpindi and Islamabad area. A maximum F concentration was noticed in groundwater obtained from D-12/2, Islamabad, due to erosion, weathering, and leaching of F-bearing rocks as this area is located near the Shah Allah Ditta Caves. The minimum content was recorded in Ratta Amral, Rawalpindi. Results clearly indicate that F contents remain fairly low in groundwater of the “twin cities” (<1.0 mg/L) at most of the tested sites (91.80%). Only a few sites had F above 1.0 mg/L (1.0–1.31 mg/L) and hence occurred very close to the threshold limit. WHO has a recommended permissible limit of 1.5 mg/L for fluoride in drinking water and almost all groundwater samples except one (ICT-38) were found well within permissible limits. The results were in good agreement with the findings of groundwater of northern Ghana (2.9 mg/L) (Lorestani et al., 2020). Comparable results for F were reported in groundwater of Gopalganj, Bangladesh, during the pre- and post-monsoon period.
Manganese (Mn) is a trace metal found in rocks and soil and can serve as an environmental contaminant at elevated thresholds (Maria et al., 2011; Saana et al., 2016). Mn is mostly found in groundwater systems under anaerobic (low oxygen) conditions. Additional sources may include industrial effluents, landfill leachate, and soil. It is principally used in the manufacture of iron and steel alloys and as an oxidant for cleaning, bleaching, and disinfection. High concentrations of manganese mainly damage the brain and leads to hallucinations, nerve damage, forgetfulness, and cause a syndrome known as Manganism. Moreover, Mn-laden groundwater is extremely objectionable to consumers due to the bitter taste, discoloration, and staining.
The concentration of Mn in groundwater ranged between 0.00 and 0.145 mg/L with a mean value of 0.0034 mg/L in the Rawalpindi and Islamabad areas. Results indicate that Mn was observed at only a few sampling sites (seven; 9.33%) located in densely populated areas impacted by industrial, domestic, and agricultural effluents. Moreover, Mn was found below detectable limits at most of the sites (68; 90.66%). The most probable reason may be the geological conditions and redox signatures of aquifers in the “twin cities,” which constrain Mn mobility in groundwater. The WHO has prescribed 0.5 mg/L as a permissible limit for Mn in drinking water and all groundwater samples were within safe limits. Lower Mn content was reported in the groundwater of Ejisu-Juaben, Ghana, compared to this study (Boateng et al., 2015).
Iron is an abundant crustal element. Moreover, it is a redox-sensitive metal and mostly found in groundwater under anaerobic settings like Mn. Iron in groundwater can be derived from both natural and anthropogenic sources like steel pumps, casings, mining, and manufacturing (Saana et al., 2016). However, an elevated concentration of iron in the water supply may not pose a health risk. Fe-laden water could be objectionable to consumers due to the undesirable bitter taste, coloration, and staining (Kahlown and Majeed, 2005). The concentration of Fe fluctuates between 0.020 and 1.15 mg/L with a mean value of 0.094 mg/L in the “twin cities.” The maximum concentration was found in the Faisal Colony bore well, whereas a minimum concentration was measured at eleven different locations. The WHO has established the highest permissible range for Fe in drinking water as 0.3 mg/L and the majority of samples (91.83%) were found within safe limits in the study area. Fe exceeded the permissible range at eight locations (F-9 Park, Main Kahuta Rd, Lohi Bher Park, River Garden, Ghori Garden, Burma Town, Faisal Colony, and Falcon Complex) in the “twin cities.” The most probable reason may be the geological conditions and redox signatures of aquifers in the “twin cities,” which constrain Fe mobility in groundwater at most of the locations. Similar results were obtained from groundwater of Ejisu-Juaben, Ghana, for Fe (Boateng et al., 2015). Conversely, higher values for Fe were reported in the groundwater of Gopalganj, Bangladesh, during the pre- and post-monsoon season.
Lead occurs naturally in water with concentrations greater than 5 μg/L. It is listed among the potentially harmful, highly toxic, persistent, and nondegradable elements (Maria et al., 2011). Moreover, Pb is a good indicator of “urban pollution” as its discharge is commonly from urban activities. Lead can enter into drinking water from multiple sources like mining, smelting, sewage effluents, leaded gasoline, lead discarded batteries, dry cells, mobile oil, car wash activities, paints, pigments, enamels, varnishes, agricultural, and industrial runoff (Kahlown and Majeed, 2005). Indeed, the most common sources of lead in drinking water are lead pipes, faucets, and fixtures.
The values obtained for lead in the current study varied from 0 to 692.20 μg/L with a mean value of 13.35 μg/L. The WHO (2017) has a given guideline value of 10 μg/L for lead in drinking water; Pb above this limit is extremely toxic and shows toxicity to the nervous system, kidneys, and reproductive system. Exposure to elevated lead can cause serious health concerns such as irreversible damage to the brain, nervous system, kidneys, digestive system, blood cells, and hypertension (Rizwan et al., 2009). Results indicate that 82.78% of the total groundwater samples had Pb contents well within the safe limits (<10 μg/L). Conversely, the Pb concentration was found beyond the permissible limit at twenty-one (17.21%) sites in the “twin cities.” Moreover, unexceptionally high values for Pb were observed at two locations (Gulberg green and Chakri). These results highlight that such a high concentration of Pb cannot be the exclusive result of anthropogenic urban activities but may have a geogenic source as well. These results were congruent with previous groundwater studies in Meghna Ghat, Bangladesh (Khattak et al., 2012), Rawalpindi, Islamabad, and Sialkot (Rizwan et al., 2009). Conversely, lower values for Pb were reported in groundwater of Rawalpindi and Islamabad (Mehmood et al., 2013) and Lahore (Bikkad and Mirgane, 2008) compared to this study. Furthermore, comparable results for Pb (<300 μg/L) were reported in groundwater of the Aurangabad Industrial Area, India (Duruibe et al., 2007).
Zinc is a metallic element with a blue-white color used as a protective coating for iron and to make metal alloys like nickel silver. Zinc can be introduced in the air, soil, and water through mining and metallurgical processing of zinc ores, its industrial application, tire industries, and car washing. It also comes from the burning of coal. The intake of Zinc in a large amount causes nausea and vomiting in children. A higher concentration of zinc may cause anemia and cholesterol problems in human beings (Duruibe et al., 2007).
Zinc is a naturally abundant micronutrient and trace element found in groundwater but becomes toxic at elevated concentrations (Saleem et al., 2018). Zn contents were recorded in groundwater samples from a total of 19 (25.33%) study sites. The Zn contents in groundwater obtained from Rawalpindi and Islamabad ranged from 0 to 1.56 mg/L with a mean value of 0.156 mg/L. The zinc content in groundwater samples was below detectable limits in 56 samples (74.66%) in the study area.
All groundwater samples were under the permissible limit (5 mg/L) prescribed by Drinking Water Guidelines (WHO, 2017). These findings were congruent with Zn contents reported in groundwater of Haripur Basin and Sialkot City, Pakistan. Furthermore, comparable results were reported in groundwater of the Aurangabad Industrial Area, India (Bikkad and Mirgane, 2008).
Stable carbon isotope analysis (δ13C)
The stable isotope of carbon offers a complementary tool to infer reliable information about the sources, distribution, and fate of hydro-pollutants. Therefore, stable carbon isotope (13C) has a powerful potential to generate pollution inventories in the aquatic environment including groundwater aquifer systems. In environmental analysis, stable carbon isotopes are used as a natural tracer of domestic and industrial pollution. Isotopic abundances are commonly reported using the δ notation in per mil (‰). Calcium carbonate from a cretaceous belemnite from the Pee Dee Belemnite formation in South Carolina (United States) is used as the reference for measuring relative concentrations (13C–12C) of δ13carbon in different environmental components including groundwater (Malik et al., 2019). Seventy-three samples were analyzed for their 13C contents to gauge biogeochemical reactions and human interventions occurring within the aquifer systems of the “twin cities.” The measured delta carbon-13 composition of groundwater obtained from the metropolitan areas of Islamabad and Rawalpindi was within the range of −18.90 to −1.04 per mil. The mean value of δ13CDIC was −7.67 per mil (parts per thousand). The results obtained in this study are in accordance with groundwater DIC (-20 to 0) prescribed by Vienna Pee Dee Belemnite (VPDB). However, the International Atomic Energy Agency (IAEA) defines the δ13C value of VPDB as 0 and any deviation from this standard is indicated toward groundwater pollution from natural/geogenic, anthropogenic, or mixed sources.
The lowest value (most depleted) δ 13C was recorded in Atfaq Colony, G-12/4 ICT, and the highest value (least depleted) in Liaquat Bagh, Rawalpindi. The more depleted (negative) values of the stable isotope of carbon (13C) in groundwater samples indicates anthropogenic pollution by inorganic matter (Qureshi et al., 1998). The groundwater samples contaminated with a high amount of sewage and industrial waste water should have high depleted values of C-13 and normally range from −12 to −22‰. The results of this study demonstrates that nearly 10.95% sites (G-12/4, Ojhri Khurd Near Pervaiz Mussharaf Farm House, Millat Colony, Chakra Road, Gulshan-e-Jinnah, F-5/1, G-11/2, Lohi Bher Park Opposite Orchid Marquee, Dhoke Saydian Chowk and Chakri, Rawalpindi) in the “twin cities” had 13C content fall within the range of −18.90 to −12.00‰. These sites are considered the most vulnerable to pollution and receive active recharge from domestic, urban, and agricultural effluents. In addition, nearly nine sites (PIDE, Pirwadhai, G-7 Markez, Aabpara Market, G-6/4, Dhoke Chaudrian, Murghuzar Zoo, Pindora, Shalimar Recording Company, H-9/3 and G-5) had 13C contents very close to the threshold, and if same trend continues, these sites will become potentially polluted sites in the future. These findings clearly confirm that shallow and deep groundwater in the “twin cities” is vulnerable to pollution through the infiltration of urban recharge (Qureshi et al., 1998; Sajjad, 1998). Moreover, approximately 19.17% of site 13C contents ranged between 8.98 and -8.05‰ and can be derived from DIC from rock-water interaction, whereas groundwater is in equilibrium with soil CO2 or minute mixing from urban wastewater. Moreover, the values of 13C (−6.97 to −6.06‰) at a few sites (13.69%) suggest a normal atmospheric CO2 presence in the study area. As pH values ranged between 6.76 and 8.12 (<8.5), the TDIC was found as HCO3- at all groundwater sampling locations. More depleted isotopic compositions of 13C clearly provides clues about the aquifer’s vulnerability from multiple sources including both natural and anthropogenic. Based on the C-13 values, it can be concluded that there is a significant mixing of urban effluents (industrial, domestic, and agricultural wastewater) in groundwater samples of the study sites. Moreover, groundwater is polluted from leaked sewer pipelines and is unfit for drinking and needs further treatment. In general, wells located near drains, rivers, industrial, and agricultural sites reported more depleted 13C values. Moreover, wells located in parks and greenbelts also recorded depleted 13C values as these sites derived their isotopic signature from land plants and incorporated it in the total carbon pool of the aquifer. As per earlier studies in the literature, the isotopic contents of stable carbon (δ13C DIC) varied between -12.0 and -6.5 per mil in urban streams of South Korea. These findings clearly suggest that detergents could be a probable source of depleting DIC values in the urban surface and groundwater systems. In addition to urban activities, δ13C content is likely derived from the dissolution of carbonate minerals (increased rock-water interaction) that constitute the predominant parts of limestone, calcite, and dolomite that are common in most bedrock within the study area (Glok-Galli et al., 2020). The negative values were noted for stable 13C isotopes at all sampling locations within the “twin cities” and are most likely due to the presence of bicarbonate ions below 500 mg/L at most of the studied points (Clark and Fritz, 1997). Conversely, Ahmed and Aggarwal (2017) reported positive values for δ13C at some points (+1.87 to +14.02 per mil) in groundwater of the Bagerhat coastal area, Bangladesh, due to the presence of elevated bicarbonate contents (660–738 mg/L).
Figure 5 presents the physicochemical parameters, heavy metals, and C-13 isotopes in the “twin cities.”
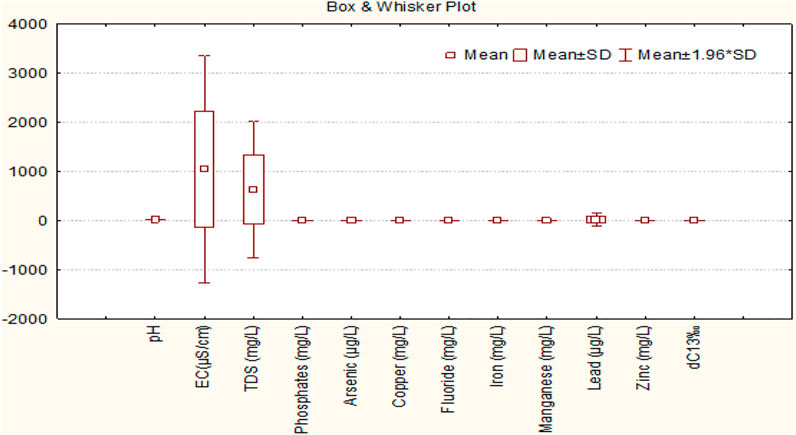
FIGURE 5. Box and whisker plots of physicochemical parameters, heavy metals, and δC13 in the groundwater of the “twin cities” of Rawalpindi and Islamabad.
Statistical analysis
Factor analysis
A factor analysis was performed on groundwater quality parameters to identify major sources of pollution in the study area and the results are presented in Table 4. Factor 1 accounted for 26.20% of the total variance and shows a strong positive loading of EC, TDS, and Pb. This factor explains that EC, TDS, and Pb in groundwater of the “twin cities” is derived from domestic, agriculture, urban, and industrial effluents, untreated and partially treated solid waste, paints, pigments, discarded batteries, atmospheric deposition, and partly from the dissolution of Pb-containing rocks (PbS-essential mineral in shale) and bedrock. This factor clearly points toward an anthropogenic influence on groundwater quality. Factor 2 accounts for 40.82% of the total variance and shows strong positive loadings of phosphates and copper and weak loading of iron. This factor suggests that Cu and PO4−3 ions derived from a common source, most likely from urban, industrial, and agricultural runoff. In addition, factor 3 accounts for 54.09% of the total variance and shows a positive loading for carbon-13 and pH and a weak loading of Mn, As, and F. This factor clearly suggests that C-13 in groundwater comes from both geogenic and anthropogenic sources and is predominantly controlled by the pH of groundwater. Factor 4 accounts for 40.82% of the total variance and shows a strong negative loading of ‘As’ and a weak loading of Zn. This factor explains the presence of ‘As’ in groundwater of the “twin cities” from the dissolution of rocks, withdrawal beyond recharge rates, higher evaporation rates and municipal, industrial, and agricultural runoff.
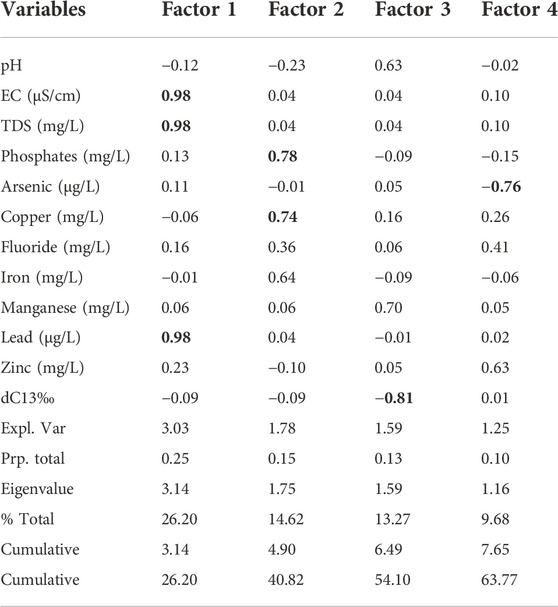
TABLE 4. Factor Analysis of heavy metals and stable carbon isotopes in groundwater of the “twin cities.”
Cluster analysis
CA was performed on the raw dataset to discriminate pollution sources and confirm the results obtained from FA. The results of CA are given in Figure 6. The findings revealed that the cluster analysis produced four classes. Cluster-I is composed of EC and TDS, which explain that groundwater quality in the “twin cities” is largely influenced by these two parameters. Cluster-II linked EC, TDS, Pb, δ13C, and As, and points toward a common origin and miscellaneous sources such as enhanced rock-water interaction, over abstraction, evaporation enrichment, improper waste disposal, discarded batteries, cross contamination of the water supply and sewerage lines, active recharge from Lie drain, and domestic, industrial, and agricultural effluents. Cluster-III is composed of Pb, δ13C, As, and F. Cluster-IV linked together pH, phosphate ions, Cu, Mn, Fe, Zn, F, As, and δ13C indicated an anthropogenic input. Moreover, this factor also demonstrated that phosphate ions, Cu, Mn, Fe, Zn, F, As, and δ13C contents in groundwater are pH dependent. Cluster-III and cluster-IV clearly elucidated the occurrence of these pollutants in the groundwater system from both lithogenic and anthropogenic emissions.
Correlation coefficient
In addition, a correlation matrix was performed on the dataset to evaluate the interrelationships among studied groundwater quality parameters and heavy metals in the “twin cities.” The results of the correlation coefficient are presented in Table 5. The results signify that pH has a strong negative relationship with carbon-13. Significant correlations were observed between EC and TDS, which indicates a common source. Phosphate showed a strong association with Cu (r2 = 0.34) and F (r2 = 0.33) and indicated miscellaneous sources. ‘As’ did not show any strong association with other water quality parameters. This indicates that the presence of ‘As’ in groundwater is from erosion and weathering of As-containing bedrock in the “twin cities” of Islamabad and Rawalpindi. TDS is strongly associated with EC, Pb, and Zn. These findings revealed that groundwater quality is strongly influenced by anthropogenic emissions (improper solid waste disposal, evaporated environment, domestic, urban, industrial, and agricultural wastewater) along with geogenic sources (dissolution of rocks and enhanced rock-water interactions) in the study area. Cu is linked with Fe (r2 = 0.31) and F (r2 = 0.28) and is most likely derived from industries located in the study area. A strong negative interrelationship was noticed between Mn and δ13C (r2 = -0.39) and clearly pointed toward different sources in urban groundwater.
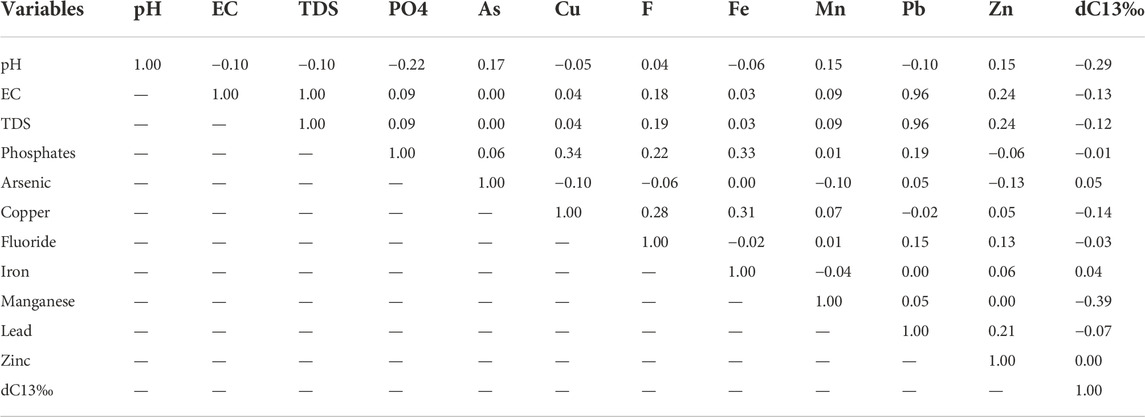
TABLE 5. Correlation coefficient between heavy metals and water quality parameters measured in groundwater samples of the “twin cities.”
Water quality index
One of the most efficient, easiest, commonly employed means of communicating valuation information about water quality of an area to the community, concerned departments, and decision-makers is through the water quality index (Iqbal et al., 2009). The water quality index (WQI) based on heavy metals was computed for groundwater obtained from Islamabad and Rawalpindi to provide a comprehensive picture of the groundwater quality. For this purpose, groundwater samples were evaluated for eight water quality parameters (TDS, As, Cu, Fe, F, Mn, Pb, and Zn). The details are summarized in Tables 6 and 7.
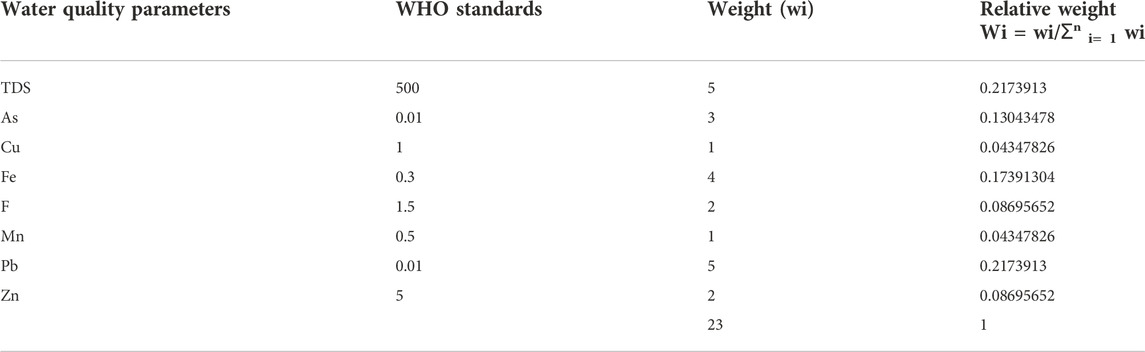
TABLE 6. Water Quality Index based on heavy metal concentration in groundwater of the “twin cities.”
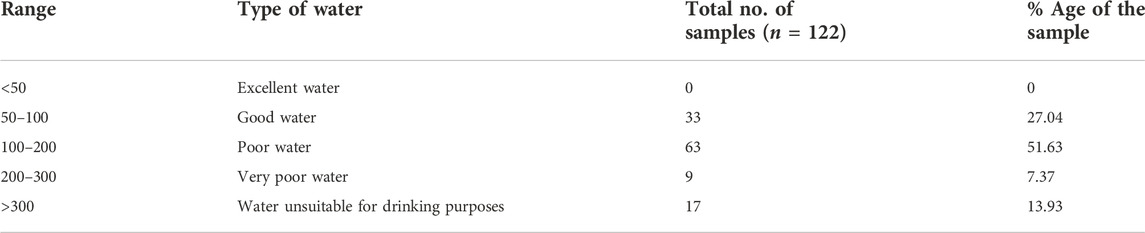
TABLE 7. Water quality classification ranges and types of water based on WQI values in the study area.
The findings of this study indicate that not a single site within the study area had excellent water quality. Out of a total of 122, only 33 groundwater samples (27.04%) had good quality water and therefore were fit for drinking. Moreover, 63 (51.63%) and 9 (7.37%) groundwater samples had poor and very poor water quality, respectively. However, 17 sites (13.93%) in Islamabad and Rawalpindi were identified in the range of “water unsuitable for drinking purpose” (see Figures 7 and 8).
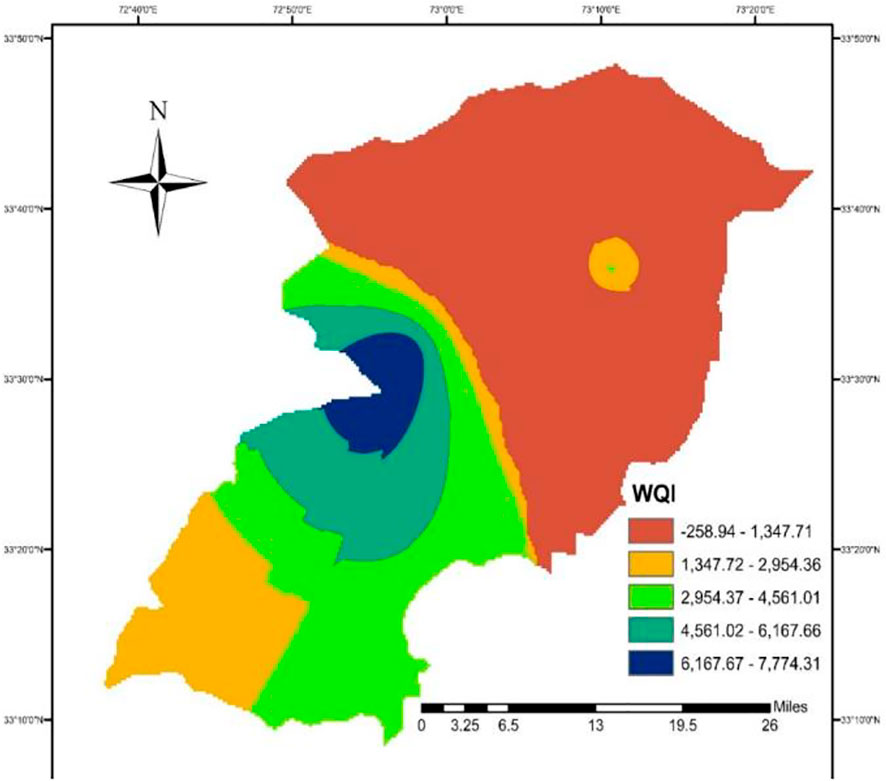
FIGURE 8. Spatial distribution of the groundwater quality index based on heavy metals in the “twin cities.”
The findings highlight that the overall water quality situation in the Rawalpindi-Islamabad metropolitan is very alarming. The analytical findings clearly indicate that groundwater resources are vulnerable to pollution risk at most of the studied sites, and therefore, either pretreatment of the water supply or provision of an alternate water source to the local populace is required and urgently needed. Furthermore, the rapidly growing population, unplanned urbanization, industrialization, improper waste disposal, over abstraction of groundwater, and a lack of water abstraction policies are all significantly contributing to the impairment of groundwater quality in the study area. Therefore, there is a dire need to urgently devise and implement appropriate water management strategies.
Conclusions
Groundwater constitutes an elementary source of the water supply in Islamabad-Rawalpindi. Groundwater quality in the “twin cities” is at risk due to natural and anthropogenic activities. Hence, there is a dire need for a comprehensive understanding of the origin, distribution, fate, and behavior of urban groundwater systems from a sustainability perspective. The findings of this study revealed that among the studied parameters, only pH and phosphates occurred within a desirable range as stipulated by WHO and PSQCA in the groundwater of the “twin cities.” However, other studied parameters such as taste, color, odor, EC, and TDS were found to be above permissible limits at a few localities, which indicated groundwater mineralization and anthropogenic inputs. Heavy metals like Cu, Zn, and Mn were within permissible limits at all pf the studied locations. The contents of both As and F were within prescribed limits at most of the sampling locations except at one location each. Iron exceeded the permissible range at eight studied locations in the “twin cities.” The lead concentration was found beyond permissible limits at twenty-one studied sites. Elevated Fe and Pb levels rendered groundwater unfit for drinking and urgently need to be considered for their treatment. δ13C confirmed that pollution is coming from both natural and anthropogenic sources. The more depleted isotopic composition of 13C clearly gives clues about an aquifer’s vulnerability from miscellaneous sources. Depleted δ13C values indicated that most of the area in the “twin cities” is vulnerable to pollution and received active recharge from domestic, urban, and agricultural effluents. In general, the wells located near drains, rivers, industrial, and agricultural sites reported more depleted 13C values. Moreover, wells located in parks and greenbelts also recorded depleted 13C values as these sites derived their isotopic signature from land plants and incorporated it into the total carbon pool of the aquifer. Apart from urban activities, δ13C content is likely to derive from the dissolution of carbonate minerals (increased rock-water interaction) that constitute a predominant part of limestone, calcite, and dolomite, which are common in most bedrock within the study area. The computed WQI, based on heavy metals, elucidated that groundwater quality was poor in most of the study area due to elevated EC, TDS, Pb, Fe, As, and F values. The findings highlight that the overall water quality situation in the Rawalpindi-Islamabad metropolitan area is very alarming. The multivariate statistical analysis revealed that groundwater quality is influenced by both lithogenic and anthropogenic inputs. Furthermore, the groundwater chemistry of the “twin cities” is largely influenced by pH, EC, and TDS contents. Based on the presented water quality data, the following specific suggestions were made to improve existing water supply conditions in the “twin cities;” it is recommended to maintain the water quality in the “twin cities” through periodic testing and monitoring of groundwater by concerned authorities like CDA, RCB, RDA (Rawalpindi Development Authority), PCRWR (Pakistan Council for Research for Water Resources), and WASA (Water and Sanitation Agency). Furthermore, leaking sewer pipelines must be repaired as a first priority to prevent domestic waste intrusion into the groundwater. A low cost and efficient water filtration plant should be installed to ensure the provision of safe and healthy drinking water to the public. Effective methods should be developed to improve aesthetic water quality. The findings of this study will assist concerned decision-makers in urgently devising and implementing appropriate water management strategies for the Islamabad-Rawalpindi metropolitan area.
Data availability statement
The raw data supporting the conclusion of this article will be made available by the authors without undue reservation.
Author contributions
SR: conceptualization, developed methodology, data collection, formal analysis, validation, writing-original draft; SA: supervision, assist in data analysis, writing—review and editing; MA: conceptualization, co-supervision, resources, funding and overseeing administration during research work; AS: writing—review and editing, help in generating discussion; KI: formal analysis, resources, writing—review and editing; WU: writing—review and editing, proofread and submission to journal; MT: helped in methodology, writing—review and editing, formal analysis, resources; NI: assisted in development of study design, assisted during field surveys and overseeing administration on behalf of PCRWR during research work; NA: writing—review and editing, referencing work, and proofreading; and QU: developed spatial maps of study area through GIS Arc View.
Funding
This research was fully funded by the Pakistan Council of Research in Water Resources (PCRWR).
Acknowledgments
The first author would like to acknowledge the assistance of Dr. Hifza Rasheed (Director General, Water Quality), NI Gondal (Director, Hydrology), and Saiqa Imran (Senior Research Officer) for providing lab facilities at PCRWR.
Conflict of interest
The authors declare that the research was conducted in the absence of any commercial or financial relationships that could be construed as a potential conflict of interest.
Publisher’s note
All claims expressed in this article are solely those of the authors and do not necessarily represent those of their affiliated organizations, or those of the publisher, the editors, and the reviewers. Any product that may be evaluated in this article, or claim that may be made by its manufacturer, is not guaranteed or endorsed by the publisher.
Supplementary material
The Supplementary Material for this article can be found online at: https://www.frontiersin.org/articles/10.3389/fenvs.2022.1027323/full#supplementary-material
References
Abbas, I., Rafique, H. M., Sohl, M. A., Falak, A., Mahmood, S., Imran, M., et al. (2014). Spatio-temporal analysis of groundwater regime within Rawalpindi municipal jurisdiction, Pakistan. Desalination Water Treat. 52, 1472–1483. doi:10.1080/19443994.2013.786658
Ahmad, Z., Ashraf, A., and Hafeez, M. (2016). “Appraisal of groundwater flow simulation in the sub- himalayan watershed of Pakistan,” in Groundwater. Editor M. S. Javaid (Rijeka: IntechOpen). doi:10.5772/63324
Ahmed, N., and Aggarwal, P. K. (2017). Isotope based assessment of deep groundwater resources for sustainable management in the coastal areas of Bangladesh-crisis in overexploitation and salinization. 1–14.
Ammara, H., and Nawaz, M. (2014). Microbiological and physicochemical assessments of groundwater quality at Punjab, Pakistan. Afr. J. Microbiol. Res. 8, 2672–2681. doi:10.5897/AJMR2014.6701
Anwar, M., Jabeen, M., and Rani, M. (2014). Impact of climate change on the residents of islamabad city. Asian J. Nat. Appl. Sci. 3, 70–76.
Asim, M., and Rao, K. N. (2021). Assessment of heavy metal pollution in Yamuna River, Delhi-NCR, using heavy metal pollution index and GIS. Environ. Monit. Assess. 193, 103. doi:10.1007/s10661-021-08886-6
Asthana, D. K., and Asthana, M. (2003). Environment: Problems and solutions. New Delhi, India: S. Chand & Company.
Atif Bokhari, S., Saqib, Z., Amir, S., Naseer, S., Shafiq, M., Ali, A., et al. (2022). Assessing land cover transformation for urban environmental sustainability through satellite sensing. Sustainability 14, 2810. doi:10.3390/su14052810
Bikkad, S. B., and Mirgane, S. R. (2008). Assessment of heavy metals in ground water of Aurangabad Industrial areas. Curr. World Environ. 3, 131–134. doi:10.12944/CWE.3.1.19
Boateng, T. K., Opoku, F., Acquaah, S. O., and Akoto, O. (2015). Pollution evaluation, sources and risk assessment of heavy metals in hand-dug wells from Ejisu-Juaben Municipality, Ghana. Environ. Syst. Res. 4, 18. doi:10.1186/s40068-015-0045-y
British Geological Survey (2001). Groundwater quality: Pakistan 2001. Nottingham, United Kingdom: British Geological Survey.
Clark, I., and Fritz, P. (1997). Environmental isotopes in hydro-geology. Boca Raton, FL: CRC Press/Lewis.
Cüce, H., Kalıpcı, E., Ustaoğlu, F., Kaynar, İ., Baser, V., and Türkmen, M. (2022). Multivariate statistical methods and GIS based evaluation of the health risk potential and water quality due to arsenic pollution in the Kızılırmak River. Int. J. Sediment Res. 37, 754–765. doi:10.1016/j.ijsrc.2022.06.004
Dassi, L., and Tarki, M. (2014). Isotopic tracing for conceptual models of groundwater hydrodynamics in multilayer aquifer systems of central and southern Tunisia. Hydrological Sci. J. 59, 1240–1258. doi:10.1080/02626667.2014.892206
Dey, P. K. (2012). Project risk management using multiple criteria decision-making technique and decision tree analysis: A case study of Indian oil refinery. Prod. Plan. Control 23, 903–921. doi:10.1080/09537287.2011.586379
Dohare, D., Deshpande, S., and Kotiya, A. (2014). Analysis of ground water quality parameters: A review. Res. J. Eng. Sci. 3, 2278–9472.
Duruibe, J. O., Ogwuegbu, M. O. C., and Egwurugwu, J. N. (2007). Heavy metal pollution and human biotoxic effects. Int. J. Phys. Sci. 2, 112–118. doi:10.1016/j.proenv.2011.09.146
Ganiyu, S. A., Mabunmi, A. A., Olurin, O. T., Adeyemi, A. A., Jegede, O. A., and Okeh, A. (2021). Assessment of microbial and heavy metal contamination in shallow hand-dug wells bordering Ona River, Southwest Nigeria. Environ. Monit. Assess. 0, 126. doi:10.1007/s10661-021-08910-9
Ghaffar, A., Tariq, T., Mashiatullah, A., and Ali, S. M. (2017). Hydrological and environment tritium investigation to evaluate groundwater in capital territory of Pakistan. Water Sci. Technol. Water Supply 17, 433–451. doi:10.2166/ws.2016.149
Glok-Galli, M., Martínez, D. E., Vadillo-Pérez, I., Busso, A. A. S., Barredo, S. P., Londoño, O. M. Q., et al. (2020). Multi-isotope (δ2H, δ18O, δ13C-TDIC, δ18O-TDIC, 87Sr/86Sr) and hydrochemical study on fractured-karstic and detritic shallow aquifers in the Pampean region, Argentina. Isot. Environ. Health Stud. 56, 513–532. doi:10.1080/10256016.2020.1825412
Iqbal, U., Qasim, H., Khan, A. K., Rashid, R., Nasreen, S., Mahmood, Q., et al. (2009). Surface and ground water quality risk assessment in district Attock Pakistan. World Appl. Sci. J. 7, 1029–1036.
Irfan, M., Zhao, Z. Y., Panjwani, M. K., Mangi, F. H., Li, H., Jan, A., et al. (2020). Assessing the energy dynamics of Pakistan: Prospects of biomass energy. Energy Rep. 6, 80–93. doi:10.1016/j.egyr.2019.11.161
Islam-ul-haq, L. C. T., Cheema, W. A., and Naseer Ahmed, C. H. (2008). Multifaceted ground water quality and recharge mechanism issues in a mega-city (Rawalpindi, Pakistan), and mitigation strategies. Secur. Groundw. Qual. Ind. Environ., 2–7.
Jabeen, S., Shah, M. T., Ahmed, I., Khan, S., and Hayat, M. Q. (2014). Physico-chemical parameters of surface and ground water and their environmental impact assessment in the Haripur Basin. Pakistan. J. Geochem. Explor. 138, 1–7. doi:10.1016/j.gexplo.2013.12.004
Jamil Butt, M., Waqas, A., Farooq Iqbal, M., Muhammad, G., K Lodhi, M. A., Butt, M. J., et al. (2012). Assessment of urban sprawl of islamabad metropolitan area using multi-sensor and multi-temporal satellite data. Arab. J. Sci. Eng. 37, 101–114. doi:10.1007/s13369-011-0148-3
Kahlown, M, A., and Majeed, A. (2005). Pakistan water resources. Islamabad: Development and Management.
Kahlown, M. A., Tahir, M. A., and Sheikh, A. A. (2008b). Water quality status in Pakistan: Second Report 2002 – 2003.
Khalown, M. A., and Majeed, A. (2005). Water resources situation in Pakistan: Challenges and future strategies.
Khatri, N., Tyagi, S., Rawtani, D., Tharmavaram, M., and Kamboj, R. D. (2020). Analysis and assessment of ground water quality in Satlasana Taluka, Mehsana district, Gujarat, India through application of water quality indices. Groundw. Sustain. Dev. 10, 100321. doi:10.1016/J.GSD.2019.100321
Khattak, M. A., Ahmed, N., Qazi, M. A., Izhar, A., Ilyas, S., Chaudhary, M. N., et al. (2012). Evaluation of ground water quality for irrigation and drinking purposes of the areas adjacent to Hudiara Industrial Drain, Lahore, Pakistan. Pak. J. Agric. Sci. 49, 549–556.
Krishna kumar, S., Logeshkumaran, A., Magesh, N. S., Godson, P. S., and Chandrasekar, N. (2015). Hydro-geochemistry and application of water quality index (WQI) for groundwater quality assessment, Anna Nagar, part of Chennai City, Tamil Nadu, India. Appl. Water Sci. 5, 335–343. doi:10.1007/s13201-014-0196-4
Kumar Sundaray, S., Chandra Panda, U., Nayak, B. B., and Bhatta, D. (2006). Multivariate statistical techniques for the evaluation of spatial and temporal variations in water quality of the Mahanadi river-estuarine system (India)-a case study. Environ. Geochem. Health 28, 317–330. doi:10.1007/s10653-005-9001-5
Lorestani, B., Merrikhpour, H., and Cheraghi, M. (2020). Assessment of heavy metals concentration in groundwater and their associated health risks near an industrial area. Environ. Health Eng. Manag. 7, 67–77. doi:10.34172/EHEM.2020.09
Loska, K., and Wiechuła, D. (2003). Application of principal component analysis for the estimation of source of heavy metal contamination in surface sediments from the Rybnik Reservoir. Chemosphere 51, 723–733. doi:10.1016/S0045-6535(03)00187-5
Malassa, H., Hadidoun, M., Al-Khatib, M., Al-Rimawi, F., and Al-Qutob, M. (2014). Assessment of groundwater pollution with heavy metals in north west bank/Palestine by ICP-MS. J. Environ. Prot. (Irvine, Calif. 05, 54–59. doi:10.4236/jep.2014.51007
Malik, M. N., Huma Hayat Khan, A. G. C., Goni, F. A., Klemeš, J., and Alotaibi, Y. (2019). Investigating students’ sustainability awareness andthe curriculum of Technology education in Pakistan. Sustainability 11, 2651. doi:10.3390/su11092651
Mannan, A., Liu, J., Zhongke, F., Khan, T. U., Saeed, S., Mukete, B., et al. (2019). Application of land-use/land cover changes in monitoring and projecting forest biomass carbon loss in Pakistan. Glob. Ecol. Conserv. 17, e00535. doi:10.1016/J.GECCO.2019.E00535
Maria, S., Riffat, A., Malik, N., Ali, S. M., and Malik, R. N. (2011). Spatial distribution of metals in top soils of Islamabad City, Pakistan. Environ. Monit. Assess. 172, 1–16. doi:10.1007/s10661-010-1314-x
Mashiatullah, A., Ahmad, N., and Mahmood, R. (2016). Stable isotope techniques to address coastal marine pollution. Appl. Stud. Coast. Mar. Environ. doi:10.5772/62897
Mashiatullah, A., Chaudhary, M. Z., Khan, M. S., Javed, T., and Qureshi, R. M. (2010). Coliform bacterial pollution in rawal lake, islamabad and its feeding streams/river. Nucl 1, 35–40.
Mehmood, S., Ahmad, A., Ahmed, A., Khalid, N., and Javed, T. (2013). Drinking water quality in capital city of Pakistan. Open Access Sci. Rep. 2. doi:10.4172/scientificreports
Munir, S., Mashiatullah, A., Mahmood, S., Javed, T., Khan, M. S., and Zafar, M. (2011). Assessment of groundwater quality using physiochemical and geochemical analysis in the vicinity of lei nala, islamabad, Pakistan. Nucl 48, 149–158.
Nowak, M. E., Schwab, V. F., Lazar, C. S., Behrendt, T., Kohlhepp, B., Totsche, K. U., et al. (2017). Carbon isotopes of dissolved inorganic carbon reflect utilization of different carbon sources by microbial communities in two limestone aquifer assemblages. Hydrol. Earth Syst. Sci. 21, 4283–4300. doi:10.5194/hess-21-4283-2017
Nriagu, J. O. (1992). Toxic metal pollution in Africa. Sci. Total Environ. 121, 1–37. doi:10.1016/0048-9697(92)90304-B
Ogrinc, N., Tamše, S., Zavadlav, S., Vrzel, J., and Jin, L. (2019). Evaluation of geochemical processes and nitrate pollution sources at the ljubljansko polje aquifer (Slovenia): A stable isotope perspective. Sci. Total Environ. 646, 1588–1600. doi:10.1016/J.SCITOTENV.2018.07.245
PCRWR (2016). Water quality status of major cities of Pakistan. Islamabad Capital Territory, Pakistan: PCRWR.
Prasanth, S. V, S., Magesh, N., Jitheshlal, K., Chandrasekar, N., and Gangadhar, K. (2012). Evaluation of groundwater quality and its suitability for drinking and agricultural use in the coastal stretch of Alappuzha District, Kerala, India. Appl. Water Sci. 2, 165–175. doi:10.1007/s13201-012-0042-5
Qureshi, R. M., Mashiatullah, A., Fazil, M., Ahmad, E., Khan, H. A., and Sajjad, M. I. (1998). Seawater pollution studies of the Pakistan coast using stable carbon isotope technique. Nucl, 224–229.
Rahman, M. A. T. M. T., Paul, M., Bhoumik, N., Hassan, M., Alam, M. K., and Aktar, Z. (2020). Heavy metal pollution assessment in the groundwater of the Meghna Ghat industrial area, Bangladesh, by using water pollution indices approach. Appl. Water Sci. 10, 186–215. doi:10.1007/s13201-020-01266-4
Rahman, M. M., Islam, M. A., Bodrud-Doza, M., Muhib, M. I., Zahid, A., Shammi, M., et al. (2018). Spatio-Temporal assessment of groundwater quality and human health risk: A case study in Gopalganj, Bangladesh. Expo. Health 10, 167–188. doi:10.1007/s12403-017-0253-y
Rizwan, U., Malik, R. N., and Abdul, Q. (2009). Assessment of groundwater contamination in an industrial city, Sialkot, Pakistan. Afr. J. Environ. Sci. Technol. 3, 429–446.
Saana, S. B. B. M., Fosu, S. A., Sebiawu, G. E., Jackson, N., and Karikari, T. (2016). Assessment of the quality of groundwater for drinking purposes in the Upper West and Northern regions of Ghana. Springerplus 5, 2001–2015. doi:10.1186/s40064-016-3676-1
Sajjad, M. S. R. S. S. T. (1998). “Chemical quality of groundwater of Rawalpindi/islamabad,” in 24th WEDC Conference: Sanitation and Water For All, Islamabad, Pakistan, 31 August-4 September.
Saleem, S., Ali, W., and Afzal, M. S. (2018). Status of drinking water quality and its contamination in Pakistan. J. Environ. Res. 2, 2–3.
Salma, (2011). “Climate change and variability trends in Pakistan and its environmental effects,”. Doctor of Philosophy (PhD) (Peshawar: University Peshawar), 1–150.
Shabbir, R., and Ahmad, S. S. (2015). Use of geographic information system and water quality index to assess groundwater quality in Rawalpindi and islamabad. Arab. J. Sci. Eng. 40, 2033–2047. doi:10.1007/s13369-015-1697-7
Shabbir, R., and Ahmad, S. S. (2016). Water resource vulnerability assessment in Rawalpindi and islamabad, Pakistan using analytic hierarchy process (AHP). J. King Saud Univ. - Sci. 28, 293–299. doi:10.1016/J.JKSUS.2015.09.007
Shah, T. (2007). The groundwater economy of South Asia: An assessment of size, significance and socio-ecological impacts. Agric. Groundw. Revolut. Oppor. Threat. Dev., 7–36.
Sharma, T., Litoria, P. K., Bajwa, B. S., and Kaur, I. (2021). Appraisal of groundwater quality and associated risks in Mansa district (Punjab, India). Environ. Monit. Assess. 193, 159. doi:10.1007/s10661-021-08892-8
Sheikh, I. M., Pasha, M. K., Williams, V. S., Raza, S. Q., and Khan, K. S. A. (2007). “Environmental geology of the islamabad-rawalpindi area,” in Regional studies of the Potwar Plateau area, northern Pakistan. Editor P. D. W. B. R. Wardlaw.
Srinivasamoorthy, K., Gopinath, M., Chidambaram, S., Vasanthavigar, M., and Sarma, V. S. (2014). Hydrochemical characterization and quality appraisal of groundwater from Pungar sub basin, Tamilnadu, India. J. King Saud Univ. - Sci. 26, 37–52. doi:10.1016/j.jksus.2013.08.001
Tokatlı, C., Towfiqul Islam, A. R. M., Güner Onur, Ş., Ustaoğlu, F., Saiful Islam, M., and Dindar, M. B. (2022). A pioneering study on health risk assessment of fluoride in drinking water in Thrace Region of northwest Türkiye. Groundw. Sustain. Dev. 19, 100836. doi:10.1016/j.gsd.2022.100836
Töre, Y., Ustaoğlu, F., Tepe, Y., and Kalipci, E. (2021). Levels of toxic metals in edible fish species of the Tigris River (Turkey); Threat to public health. Ecol. Indic. 123, 107361. doi:10.1016/j.ecolind.2021.107361
Ustaoğlu, F., and Aydın, H. (2020). Health risk assessment of dissolved heavy metals in surface water in a subtropical rivers basin system of Giresun (North-eastern Turkey). Desalin. Water Treat. 194, 222–234. doi:10.5004/dwt.2020.25900
Uzaira, R., Sumreen, I., and Uzma, R. (2005). Evaluation of drinking water quality in Rawalpindi and Islamabad. Available at: https://inis.iaea.org/search/search.aspx?orig_q=RN:36058285 (Accessed August 24, 2022).
Varol, M., Ustaoğlu, F., and Tokatlı, C. (2022). Ecological risks and controlling factors of trace elements in sediments of dam lakes in the Black Sea Region (Turkey). Environ. Res. 205, 112478. doi:10.1016/j.envres.2021.112478
Vetrimurugan, E., Brindha, K., Elango, L., and Osman Ndwandwe, M. (2017). Human exposure risk to heavy metals through groundwater used for drinking in an intensively irrigated river delta. Appl. Water Sci. 7, 3267–3280. doi:10.1007/s13201-016-0472-6
Ward, N. I. (1995). “Environmental analytical chemistr,” in Trace elements. Editor P. J. Haines (Blackie Academic and Professional), 320–328.
Keywords: heavy metals, trace metals, stable carbon isotopes, groundwater, pollution hotspots, GIS mapping
Citation: Rana SA, Ali SM, Ashraf M, Shah AA, Iqbal KMJ, Ullah W, Tariq MAUR, Iqbal N, Akhtar N and Ulain Q (2022) GIS-based assessment of selective heavy metals and stable carbon isotopes in groundwater of Islamabad and Rawalpindi, Pakistan. Front. Environ. Sci. 10:1027323. doi: 10.3389/fenvs.2022.1027323
Received: 24 August 2022; Accepted: 07 November 2022;
Published: 24 November 2022.
Edited by:
Izharul Haq, Indian Institute of Technology Guwahati, IndiaReviewed by:
Quoc Bao Pham, University of Silesia in Katowice, PolandFikret Ustaoğlu, Giresun University, Turkey
Copyright © 2022 Rana, Ali, Ashraf, Shah, Iqbal, Ullah, Tariq, Iqbal, Akhtar and Ulain. This is an open-access article distributed under the terms of the Creative Commons Attribution License (CC BY). The use, distribution or reproduction in other forums is permitted, provided the original author(s) and the copyright owner(s) are credited and that the original publication in this journal is cited, in accordance with accepted academic practice. No use, distribution or reproduction is permitted which does not comply with these terms.
*Correspondence: Muhammad Atiq Ur Rehman Tariq, YXRpcS50YXJpcUB5YWhvby5jb20=