- 1State Key Laboratory of Simulation and Regulation of Water Cycle in River Basin, China Institute of Water Resources and Hydropower Research, Beijing, China
- 2Department of Water Ecology and Environment, China Institute of Water Resources and Hydropower Research, Beijing, China
- 3Ecological Environment Engineering Research Center of the Yangtze River, China Three Gorges Corporation, Beijing, China
Rivers are important routes for material and energy transport between terrestrial and marine ecosystems. Recent global-scale assessments of carbon (C) have suggested that C emission fluxes to the atmosphere are comparable to the fluvial C fluxes to the ocean. However, many previous studies only collected data from inland rivers in low altitude regions. Therefore, it remains unclear how plateau rivers affect C flux. In this study, 20 monitoring sites were set up along the Yarlung Tsangpo (YT) River on the Tibetan Plateau and detailed observations were carried out in the wet and dry seasons. The riverine CO2 fluxes exhibited significant seasonal patterns which ranged from 597.12 ± 292.63 μatm in the wet season to 368.72 ± 123.50 μatm in the dry season. The CO2 emission flux (FCO2) obtained from floating chamber method, ranging from 8.44 ± 6.94 mmol m−2 d−1 in sunmmer to 3.62 ± 6.32 mmol m−2 d−1 in winter, with an average value of 6.03 mmol m−2 d−1. Generally, the river was a weak carbon source with respect to the atmosphere. However, the pCO2 and FCO2 were much lower than that for other large rivers around the globe, which were obviously restrained by the weak microbial activities due to the low primary productivity and carbonate buffer activities in the carbonate background. Carbon loss via atmosphere exchange in the YT River on the plateau accounted for 2.2% and 10.6% of the riverine dissolved carbon fluxes (67.77 × 109 mol a−1) according to the floating chamber and thin boundary layer methods, respectively. The YT River probably acts as a “pipeline” to transport weathered nutrients from the plateau to downstream areas. Our results demonstrated the characteristics of a “weak outgassing effect and a high transport flux of carbon” for the plateau river, which is different from rivers on plains. Considering the global relevance of Tibetan Plateau, further studies with enhanced spatiotemporal resolution are needed to better understand the important role of plateau rivers on carbon budgets and climate change over both regional and global cycles.
1 Introduction
Rivers are distinct ecosystems, geomorphological agents, sites of biogeochemical transformation, and conduits for material transport across continents and to the oceans (Marcé et al., 2015; Einarsdottir et al., 2017). Furthermore, they are strongly linked with human activities (Aufdenkampe et al., 2011; Liu et al., 2022). Traditionally, rivers have been considered to be passive pipes in the regional and global budgets for carbon (C) and weathering products (i.e., dissolved ions). Recent studies have reported that C emission fluxes to the atmosphere are comparable to the fluvial C fluxes to the ocean (Battin et al., 2009; Teodoru et al., 2015; Lu et al., 2018; Bussmann et al., 2022). Reported values of CO2 emissions for the fluvial network alone ranged from 0.26 Pg C yr−1 to 1.8 Pg C yr−1 (Richey et al., 2002; Cole et al., 2007; Tranvik et al., 2009; Aufdenkampe et al., 2011; Raymond et al., 2013; Regnier et al., 2013), the largest published estimate (1.8 Pg C yr−1, Raymond et al., 2013) being about twice as large as the lateral export of carbon to the coastal ocean from the global river network (Regnier et al., 2013). Thus, carbon remobilization, particularly through water-air interface exchange from freshwaters, including river systems, represents an important component of the global carbon cycle. A large number of studies on river outgassing have been undertaken in typical rivers, such as the Amazon (Richey et al., 2002; Alin et al., 2011; Sawakuchi et al., 2021), the Mississippi (Dubois et al., 2010), the Yangtze River (Zhao et al., 2013; Liu et al., 2016; Ni et al., 2019), the Yellow River (Ran et al., 2015) and the karst river system (Ni et al., 2020; Ni and Li, 2022). However, there is substantial uncertainty in current understanding about global carbon flux, in part due to a lack of direct measurements of rivers in the high-altitude cryosphere (Zhang et al., 2020).
The Tibetan Plateau is sometimes called the “Third pole” of the earth, has an average elevation of over 4,000 m, and is the cradle for 10 large Asian rivers (Immerzeel and Bierkens, 2012). Compared to other inland rivers, rivers on plateaus have higher altitudes and greater erosion and weathering capacities, but their primary productivities are lower (Ye, 2019; Yu et al., 2021). Owing to the negligible impact of anthropogenic activities, the YT River is typical to reflect the geochemical nature of a plateau river and serves as the water vapour channel Tibet (Qu et al., 2017). These factors may considerably influence the basic characteristics of the river carbon balance on plateaus. Only a few studies have investigated the carbon emissions from the rivers on the Tibetan Plateau. Qu et al. (2017) assessed the spatial distributions of CO2 concentration and emission fluxes from headstreams on the Tibetan Plateau and found that all the studied streams released CO2 into the atmosphere. Yan et al. (2018) showed that the littoral zones of lakes on the Tibetan Plateau were supersaturated and acted as sources of greenhouse gases. Research on the alpine riverine dissolved inorganic carbon (DIC) of Nyangqu River found that rock weathering controlled the DIC input into the riverine system and groundwater played a significant role in delivering DIC to the surface water (Ge et al., 2021). However, the degassing effect of plateau rivers might be still unclear. Therefore, it is important to investigate this effect if we are to understand the roles that plateau rivers play in global and regional carbon cycles. Additionally, improved understanding of CO2 emissions from rivers in this climate-sensitive region is critical for forecasting responses to current and future climates.
In this study, we chose the reaches of the Yarlung Tsangpo (YT) River on the plateau as the study region to 1) assess the spatial and seasonal distributions of CO2 partial pressure (pCO2) and CO2 emissions across the river and 2) demonstrate the “outgassing effect” of rivers on the plateau from the perspective of the carbon budget.
2 Materials and methods
2.1 Study area
The Tibetan Plateau is one of the most sensitive areas to climate change in the world and covers approximately 2.5 × 106 km2. The YT River, which originates in the Jiemayangdrung glacier of the Himalayan Mountains, is one of the longest plateau rivers in the world (Huang et al., 2011). It is 2,104 km in length (2,057 km of which is in Tibet) with a total drainage area of 24.048 × 104 km2. The upper part of the river, known as the Maquan River, runs for about 161 km to Lizi village and has an elevation of over 4,400 m. The middle reaches bring together many tributaries, such as the Nimu, Lhasa, and Pailong Zangbu Rivers (Jiang et al., 2015; Tong et al., 2016). The Zangmu reservoir was built in the mainstream part of the middle reaches. The climate in this basin is prominently influenced by the India/South Asia monsoon and about 50%–80% of the precipitation occurs in the wet season, which lasts from July to September (Liu et al., 2007).
Geologically, the catchment of the YT River is composed of different, well-exposed strata. In particular, Mesozoic strata, siliceous rocks, harzburgite, dunite, and basalt are distributed along the YT ophiolite zone (Zhu 2012). Generally, the terranes of the catchment mostly belong to the Tethian Sedimentary Series, which is composed of Palaeozoic-Mesozoic carbonate and clastic sedimentary rocks (Galy and France-Lanord, 1999).
Sampling was carried out along the entire stretch of the middle and upper reaches of YT River (upstream of the Yarlung Zangbo Grand Canyon). Three parallel samples were collected simultaneously at one site in December, 2017 (dry season) and July, 2018 (wet season) for parallel measurements. There were 11 sites in the mainstream part of the river and nine sites in its different tributaries and samples were taken in both the wet and dry seasons. In this study, the upper reaches of Nugesha hydrological station (M1-M3 and T1-T3) were taken as the upstream, and the middle reaches were recognized from Nugesha hydrological station to the Zangmu reservoir Dam (M4-M10 and T4-T6). The low reaches were behind the dam (M11 and T7-T9). Detailed information about the sampling points in the mainstream area and the main tributaries is shown in Figure 1 and Supplementary Schedule S1.
2.2 Hydrochemecal and laboratory analysis
At each sampling site, water temperature (T), pH, dissolved oxygen (DO), electroconductivity (EC), and total dissolved solid (TDS) were measured in situ using a YSI EXO2 multisensor sonde (Yellow Springs Instruments, Yellow Springs, OH, United States). The DIC was calculated by summing up all the inorganic carbon species (HCO3−, CO32-, and H2CO3). The pCO2 was estimated using the pH-alkalinity-temperature method, whereby the alkalinity was titrated in the field with 0.025 M HCl using a Hach digital titrator (Loveland, CO, United States).
The riverine DIC equilibrium conversion can be described by the following equation:
where H2CO3* is the analytical sum of CO2 (aq) and the true value for H2CO3; and KCO2, K1, and K2 are temperature dependent dissociation constants and can be calculated from the empirical expressions according to Clark and Fritz (1997).
The pCO2 was then calculated using alkalinity, in situ pH, and water temperature:
The suspended particulate sediment (SPS) was collected by filtering 2 L of water through Whatman GF/F filters (0.7 μm pore size). The samples were acidified with 2 M HCl to remove the inorganic carbon and then dried at 60°C to complete dryness. The particulate organic carbon (POC) was analyzed using an ECS 4010 CHNSO analyzer (Costech International S.p.A., Milan, Italy). Duplicate samples were taken and the precision was ± 5% for POC.
Water for the dissolved organic carbon (DOC) analysis was first filtered through 0.7 μm Whatman GF/F filters and then 2–3 drops of 2 M HCl were added. The DOC was determined by an Elementar Vario TOC Select Analyzer (Elementar, Langenselbold, Germany) by the high temperature (850°C) combustion method. The triple injections produced a precision of within ± 5%.
The carbon flux (f) was calculated as follows:
where C and Q are the monthly average values for carbon concentration and water discharges from the control sections during the dry and wet seasons, respectively.
2.3 CO2 flues across the water-air interface
2.3.1 Floating chamber method
The CO2 emission flux (FCO2) was measured in situ using a cylindrical floating chamber (FC) method which is an inexpensive and convenient method to measure direct diffusive fluxes at the surface of aquatic ecosystems. The floating chamber had a water surface area of seven dm2, a height of 40 cm, and was attached to an ultra-portable gas analyzer (UPGA, LGR company, United States). The chamber mounted on plastic foam that had streamlined ends to limit artificial disruption due to near-surface turbulence. A vent on the top of the chamber equilibrated the pressure inside the chamber with the atmospheric pressure prior to each measurement. At each site, the chamber walls were lowered 2 cm into the water and installed at the same distance (about 1 m) away from the bank. The gas analyzer automatically recorded at 1 s intervals for about 10 min, and two or consecutive measurements were made.
The CO2 emission flux from the water-air interface (FCO2, mmol m−2d−1) was determined from the slope of a linear regression of the CO2 concentration in the gas analyzer against time (coefficient of determination r2 > 0.90):
where dc/dt is the slope of the CO2 connection in the chamber headspace versus time (ppm s−1), F1 is the conversion factor from ppm to mg m−3, F2 is the conversion factor from seconds to days, and V/A is the height of the floating chamber.
2.3.2 Thin boundary layer method
The CO2 flux across the water–air interface was also calculated using the thin-boundary layer method based on Fick’s law (UNESCO/IHAGHG, 2010).
Where FCO2 is the CO2 flux from water to air (mmol.m−2.d−1), K is the gas transfer velocity (cm.h−1). Cwater is the gas concentration in surface water (mmol L−1), and Ceq-air is the gas concentration in surface water that is in equilibrium with the atmospheric concentration (mmol L−1). We calculated K using the temperature-dependent Schmidt number (SCT) for fresh water (Raymond et al., 2012):
with
Where T is the in situ water temperature (oC), and K600 is the K for CO2 at 20°C in freshwater. K values depend on river size (Alin et al., 2011) and can be 60% greater in rivers with channels <100 m wide than in rivers with channels > 100 m wide. Different equations are available for large and small rivers. Because the YT River’s width is usually greater than 100 m, we estimated K600 using the large rivers formula according to the research of Alin et al. (2011).
Where U10 (m.s−1) is the wind speed at a height of 10 m above the water surface and U1 (m.s−1) is the wind speed over the water body obtained from field monitoring using the wind speed meteorological meter (Kestrel4500, United States).
2.4 Data analysis
The Pearson correlation analysis and Kruskal–Wallis (K-W) test of environment variables were carried out with Statistical Program for Social Sciences 22 (SPSS22, IBM Corporation, Amon, NY, United States) to test the relationship and significant difference in the seasons.
3 Results
3.1 Comparison of floating chamber and TBL methods
As shown in Figures 2, 3, the CO2 fluxes obtained by the TBL in the wet and dry season had greater variation range and higher values in than those obtained by the FC. From the qualitative perspective, about 80% of the results of the two methods in the CO2 sink/source were generally consistent, and five CO2 fluxes showed inconsistencies in the CO2 sink/source which were mainly located in the downstream. The results obtained by the two methods showed relatively good positive correlation (R2 = 0.63, p < 0.01), indicating that the two methods could reflect the general trend of the CO2 fluxes in the monitoring of YT River Basin. The differences between two monitoring results were due to different monitoring principles and error sources which were still not very clear about its main causing environmental factors (Zhang et al., 2019). Since FC method measured the CO2 flux across the water-air surface directly, we use CO2 fluxes estimated from the FC method for further discussion in this study. However, due to the influence of instantaneous meteorological processes (water temperature difference, wind speed, etc.), CO2 fluxes had diurnal changes. In this study, the sampling was conducted at each sampling point in the daytime (mainly from 10:00 to 15:00), so it only represented the CO2 exchange in the daytime of the YT River.
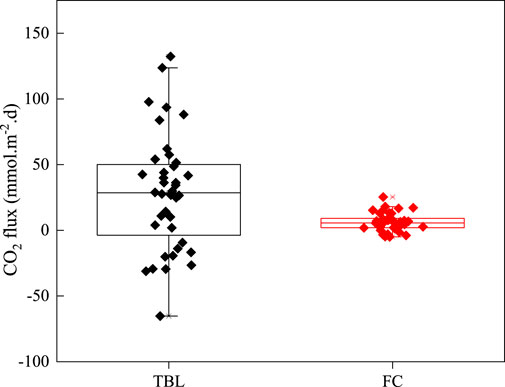
FIGURE 3. Discrete-degree diagrams of the FC method and TBL method (the middle line, lower edge and upper edge, dots in or outside the boxes refer to mean values, 25th and 75th, 5th and 95th, and <5th and >95th percentiles of all data, respectively).
3.2 Physicochemical characteristics
The physicochemical parameters of the YT River are listed in Table 1 and Figure 4. The T, pH, DO showed significant seasonal differences (p < 0.01). The wind speed in rainy season ranged from 0.7 to 2.6 m s−1, with an average of 1.7 ± 1.1 m s−1. A slight high wind speed was observed in dry season, the average was 1.8 ± 1.2 m s−1. Similar K600 averages (19.84 and 20.47 cm h−1, respectively) were observed across the seasons. As expected, T decreased from 16.63 ± 3.26°C in the wet season to 5.59 ± 2.46°C in the dry season. On the contrary, the DO concentration increased from 6.17 ± 0.57 mg L−1 in the wet season to 7.79 ± 0.43 mg L−1. The DO saturations were relatively high (90.6%–110.2%). The pH variation was relatively stable, ranging from 7.95 to 8.98 with a mean value of 8.22 in the wet season and from 8.14 to 8.70 with a mean value of 8.42 in the dry season, indicating the YT River water system was weakly alkaline. This was due to the strongly alkaline and weakly acidic nature of the carbonate rock geology on the plateau (Galy and France-Lanord, 1999). The TDS values increased from 189.34 ± 85.32 mg L−1 in the wet season to 213.64 ± 49.37 mg L−1 in the dry season. The values showed no significance difference for the seasonal pattern. Spatially, TDS decreased along the river, especially in the wet season (p < 0.01). The water vapor channel was transported upward from the downstream area, and thus the precipitation increased from the lower reaches to the upper reaches of the river (Jiang 2020). Therefore, dilution caused by precipitation might be one of the main reasons for the spatial differences of TDS in the wet season.
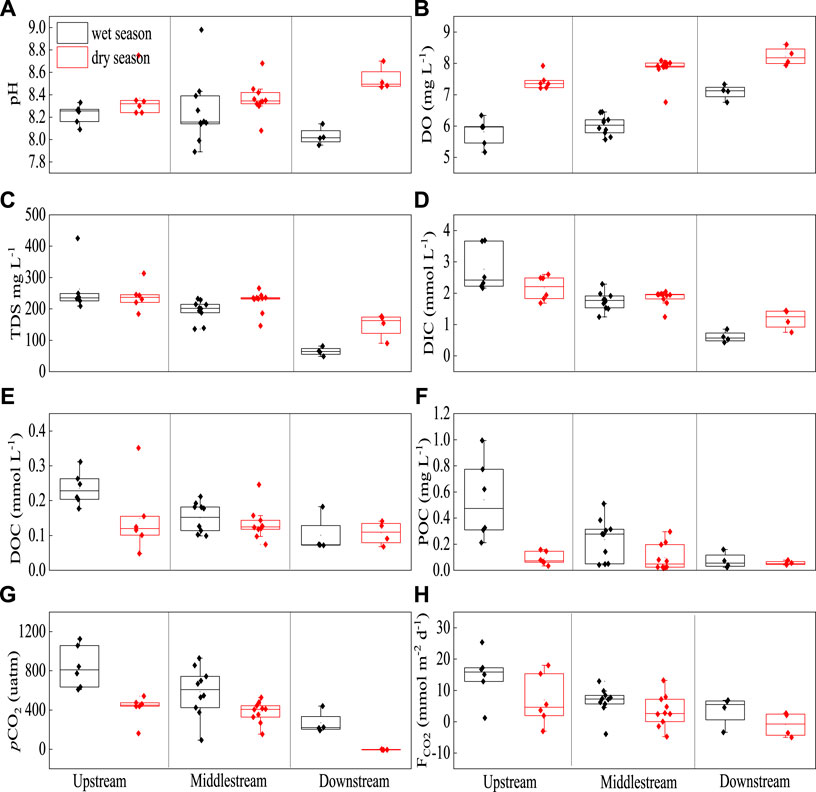
FIGURE 4. Box diagram of pH, DO, TDS, DIC, DOC, POC, pCO2, and FCO2 in different reaches of YT River (the middle line, lower edge and upper edge, dots in or outside the boxes refer to mean values, 25th and 75th, 5th and 95th, and <5th and >95th percentiles of all data, respectively).
3.3 Spatial and temporal variability of carbon species and pCO2
The results of Kruskal–Wallis (K-W) test demonstrated that POC and pCO2 had seasonal variation (p < 0.05), while DIC and DOC had no significant seasonal variations. The DIC varied from 0.23 to 3.69 mmol L−1 and 0.75–2.60 mmol L−1 in the wet season and dry season with the mean values of 1.75 mmol L−1 and 1.81 mmol L−1, respectively. The spatial variation of DIC was similar to the variations in TDS. The DOC concentration varied from 0.07 to 0.31 mmol L−1 with a mean value of 0.17 mmol L−1 in the wet season, whereas, it varied from 0.05 to 0.35 mmol L−1 with an average value of 0.13 mmol L−1 in the dry season. The POC content, ranged from 0.02 to 0.99 mmol L−1 with a mean value of 0.29 mmol L−1 in the wet season, which was much higher than that in the dry season with a mean value of 0.09 mmol L−1 (ranging from 0.02 to 0.30 mmol L−1). The seasonal difference in POC was greater than that for DIC and DOC, which indicated that the POC was more significantly affected by the flush effect. The melting of glaciers and permafrost into the basin meant that more terrigenous organic matter entered into the river, resulting in significant increases in suspended solids and POC concentrations in the river during wet season. The spatial variation in DIC, DOC and POC contents showed a gradually decreasing trend in the YT River during the wet season (p < 0.01), but was relatively stable in the dry season, which might be caused by the increase in precipitation from the upstream part of the river to the downstream part in the wet season.
The pCO2 exhibited spatial and seasonal variations in the YT River (p < 0.05), ranging from 597.12 ± 292.63 μatm in the wet season to 368.72 ± 123.50 μatm in the dry season. The result was lower than the research of Qu et al. (2017) (median value of 864 μatm with headspace balance method) and comparably to the result of Ye (2019) (mean value of 751 μatm). Our results showed about 75% of samplings were supersaturated in CO2 than the atmospheric CO2 (about 400 μatm). The highest pCO2 was measured in the headwater (M1) while the lowest values were found in the lower reaches of the river (M11). In general, a decreasing trend was identified for pCO2 along the mainstream part of the river, especially in the wet season.
4 Discussion
4.1 Controls on pCO2
The riverine pCO2 is closely related to both internal carbon dynamics and external biogeochemical processes. 1) The regional geological background and chemical weathering intensity determine the thermodynamic equilibrium system for carbonate in the river, such as DIC concentration, pH, etc., (Neal et al., 1998; Li et al., 2013). 2) Soil CO2 influxes and the aquatic respiration of organic carbon increases pCO2 and decreases pH. In contrast, photosynthesis will decrease pCO2 levels but increase water pH (Hagedorn and Cartwright, 2010). 3) The above processes changed the pCO2 of the river body and they contributed to degassing or absorption phenomena (Ni et al., 2019).
Seasonal temperature and precipitation influenced the aquatic pCO2, as it was the dominant effect on soil respiration and carbon transportation (Hope et al., 2004) The pCO2 value was significantly higher in the wet season than in the dry season, which was attributed to the enhancement of soil respiration by warm temperature (16.63 ± 3.26°C). More precipitation will flush the soil with relatively a high CO2 content into the river water (Hope et al., 2004; Li et al., 2013), which was also confirmed by the higher POC content in the wet season (Figure 4F). Besides, the concentrated rainfall also promoted rapid kinetics of hydrological cycle (Jung et al., 2012; Zhang et al., 2017; Zhong, 2017), which resulted in low pCO2 in the downstream part of YT River in wet season (Figure 4G). Similar observations were also found in the upper Yangtze in the rainy season (Li et al., 2012). In aquatic ecosystem, the degree of photosynthesis and respiration in the sub-aquatic community will also influence pCO2 content. The organic carbon could be biodegraded into CO2 by respiration of microbes (Guillemette et al., 2013). This was corresponded with the positive Pearson correlation coefficients between DOC and pCO2 (Figure 5). However, the average DOC concentration in the YT River (0.15 mmol L−1) was relatively low compared to other large rivers across the globe (0.479 mmol L−1) (Meybeck, 1982) and it only accounted for about 8.6% of DIC. The internal primary production was a non-essential driver for pCO2 due to low primary productivity on the plateau (Ye, 2019).
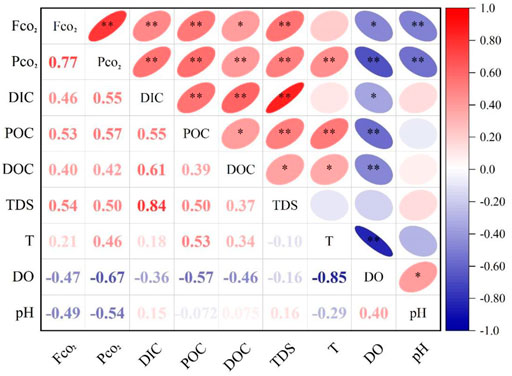
FIGURE 5. Pearson correlation coefficient of FCO2 and environmental factors (*Correlation is significant atp < 0.05 level; **Correlation is significant at p < 0.01 level).
Moreover, the local geological background and water carbonate balance also showed multiple mechanisms influencing pCO2 (Li et al., 2013). Dissolution of carbonate minerals in the YT River basin resulted in high DIC concentrations (1.82 ± 0.69 mmol L−1), and thus potentially contributed to the pCO2 levels. Meanwhile, there are three species of DIC, i.e., CO2* (dissolved CO2 and H2CO3), HCO3−, and CO32–, and their relative activities in water are largely functions of pH (Liu et al., 2010). At a relatively high pH (8.30 ± 0.22), CO2*dissociates into the ionized species HCO3−and CO32–, and by converting CO2* into non-volatile forms, this “carbonate buffering” may reduce pCO2 concentrations (Cai et al., 2008).
4.2 CO2 emission fluxes from the Yarlung Tsangpo river
Differences in FCO2 was also found in YT River between seasons (p < 0.05), with average values varying from 8.44 ± 6.94 mmol m−2 d−1 in wet season to 3.62 ± 6.32 mmol m−2 d−1 in dry season (Table 1 and Figure 4H). Generally, the river acted as “weak carbon source” for atmospheric CO2. The spatial average showed that the largest FCO2 occurred at the upstream of T2 (25.36 mmol m−2 d−1) in wet season and negative flux occurred at the downstream part in dry season which appeared as a CO2 sink, which corresponded with the lower pCO2 in the downstream.
To further understand the degassing CO2 flux in the YT River, it was compared to other large rivers in different climate zones in Table 2. Our result showed that the CO2 emission flux of the YT River was the lowest among other rivers across the globe. For instance, it was much smaller than that of the tropical Amazon River, the temperate Mississippi River, and the subtropical Yangtze and Lancang Rivers. It was relatively close to the Eastmain Quebec River in the boreal zone. According to Aufdenkampe et al. (2011), the median CO2 efflux of large tropical rivers is about 2.2 times greater than that of large temperate rivers and 6.5 times greater than that of large rivers in the cold zone, which suggested that temperature had an important influence on CO2 effluxes. Figure 5 shows the Pearson correlation coefficient of FCO2 and environmental factors. FCO2 was apparently positively dominated by the riverine pCO2 (R = 0.77, p < 0.01) and negatively correlated with pH (R = −0.46, p < 0.01). Obviously, the low FCO2 resulted from the low pCO2 value which was due to the low primary productivity and “carbonate buffering” of the alkaline water body (high pH) in the plateau river.
4.3 Regional carbon flux and worldwide comparation
With large river systems, assessing carbon emission flux is essential when attempting to understand the fate of carbon entering the drainage network and its significance in the global carbon cycle. We took hydrological station Nuxia (M11) as the outflow section of the plateau. It was used to calculate the flux of carbon that was transported from the plateau (altitude > 2000 m) to the downstream plain.
The carbon outgassing load to the atmosphere was calculated as the product between surface area and measured average CO2 flux, and the surface areas of the rivers were estimated from the Landsat images. The average surface plateau areas of the YT River during the wet and dry seasons in 2010 and 2015 were 806.70 km2 and 493.11 km2, respectively. The average CO2 emission values in the wet and dry season were 19.92 × 107 mol month−1 and 5.35 × 107 mol month−1. The annual CO2 release flux was about 1.52 × 109 mol a−1 based on the mean values for the two seasons. The total annual carbon transport recorded at Nuxia was 93.86 × 109 mol a−1, which consisted of 67.77 × 109 mol a−1 DIC, 12.87 × 109 mol a−1 DOC, and 13.22 × 109 mol a−1 POC.
It can be seen that the CO2 degassing effect was relatively weak and only accounted for 2.2% of the annual DIC flux in the river according to the FC method and about 10.6% of the DIC flux according to the TBL method (Table 3). These values were much lower than global average and for rivers such as the Yangtze, Mekong, and Mississippi. For instance, the CO2 emission flux to the atmosphere was 80% and 50% of the DIC for the Yangtze River and the global average value for rivers, respectively. The YT River probably acted as a “pipeline” to transport the nutrient to downstream. Generally, we concluded that plateau rivers were characterized by relatively low degassing fluxes and low carbon transformation intensities and that these were related to weak biological effects and carbonate buffer activities in the carbonate background on the plateau.
It should be noted that while our estimates of carbon losses from the YT River were a significant portion of the carbon budget for the Tibetan Plateau, they may be potentially large uncertainties due to 1) the lack of long-term measurements in the control hydrology section; 2) limiting number of repeated samplings at the same sites and taking repeated samples at a same site over a representative period of time; and 3) the use of different measurement methods for CO2 emission flux. Our data get from the FC methods were measured at the sites which was about 1 m away from the bank at where the flow velocity might be relatively lower. It meant that CO2 emission flux obtained by FC methods might be lower compared to the actual CO2 emission flux in or near the middle part of river. However, our results reflected the basic characteristics of the “weak outgassing effect and a high carbon transport flux” phenomenon shown by the YT River on the Tibetan Plateau. The considerable global relevance of the Tibetan Plateau and its importance to water supply and climate change meant that these topics deserve attention in future research studies.
5 Conclusion
1) The YT River on the plateau was a weak carbon source for the atmosphere. Aquatic pCO2 displayed obvious seasonal variabilities across the river basin, averaging about 597.12 μatm and 368.72 μatm in the wet and dry seasons, respectively. The influx of soil CO2, due to watershed erosion, and the dilution effect may explain the seasonal and spatial variations in pCO2.
2) The pCO2 values for the YT River were much lower than those for other larger rivers in different climate zones. Unlike most rivers and lakes, which are supersaturated with CO2, biological aerobic respiration was not the main driver of CO2 production in the YT River due to its lower primary productivity (low DOC content and weak microbial activity). The regional geological background and its internal carbon dynamics (the carbonate equilibrium system) mainly determined the basic aquatic pCO2 of the YT River.
3) According to the static float method, the CO2 emission fluxes for the YT River were 8.44 mmol m−2 d−1 and 3.62 mmol m−2 d−1 in the wet and dry seasons, respectively. It was estimated that about 1.51 × 109 C mol a−1 was emitted as CO2 to the atmosphere and about 93.86 × 109 mol a−1 carbon was discharged from the plateau to the lower reaches of the river. The carbon mass balance suggested that CO2 emissions by the YT River to the atmosphere represented only 2.2 and 10.6% of the DIC budget. This suggested that rivers on the Tibetan Plateau exhibited the characteristics of “high transport flux and weak outgassing” which was a different pattern to that of other large rivers in the world. Therefore, the carbon cycles of rivers on the Tibetan Plateau should be the focus of future studies to improve global carbon budget and regional environmental predictions.
Data availability statement
The original contributions presented in the study are included in the article/Supplementary Material, further inquiries can be directed to the corresponding authors.
Author contributions
All authors listed have made a substantial, direct, and intellectual contribution to the work and approved it for publication.
Funding
This study was supported by National Natural Science Foundation of China (No. U1802241, 92047204, U2040211), the National key research and development project (2021YFC3201002, 2022YFC3205000), the Project of China ThreeGorges Corporation (No.201903144) and the Open Research Fund of State Key Laboratory of Simulation and Regulation of Water Cycle in River Basin, China Institute of Water Resources and Hydropower Research (SKL2020TS07). The authors declare that this study received funding from China Three Gorges Corporation. The funder was not involved in the study design, collection, analysis, interpretation of data, the writing of this article, or the decision to submit it for publication.
Acknowledgments
We would like to thank all the contributors and the Frontiers staff who have made this research topic possible.
Conflict of interest
Author XW was employed by China Three Gorges Corporation.
The remaining authors declare that the research was conducted in the absence of any commercial or financial relationships that could be construed as a potential conflict of interest.
Publisher’s note
All claims expressed in this article are solely those of the authors and do not necessarily represent those of their affiliated organizations, or those of the publisher, the editors and the reviewers. Any product that may be evaluated in this article, or claim that may be made by its manufacturer, is not guaranteed or endorsed by the publisher.
Supplementary material
The Supplementary Material for this article can be found online at: https://www.frontiersin.org/articles/10.3389/fenvs.2022.1036725/full#supplementary-material
References
Alin, S. R., deFátima, F. L., Rasera, M., Salimon, C. I., Richey, J. E., Holtgrieve, G. W., et al. (2011). Physical controls on carbon dioxide transfer velocity and flux in low-gradient river systems and implications for regional carbon budgets. J. Geophys. Res. 116, G01009. doi:10.1029/2010JG001398
Aufdenkampe, A, K., Mayorga, E., Raymond, P. A., Melack, J. M., Doney, S. C., Alin, S. R., et al. (2011). Riverine coupling of biogeochemical cycles between land, oceans, and atmosphere. Front. Ecol. Environ. 9 (1), 53–60. doi:10.1890/100014
Battin, T. J., Luyssaert, S., Kaplan, L. A., Aufdenkampe, A. K., Richter, A., and Tranvik, L. J. (2009). The boundless carbon cycle. Nat. Geosci. 2 (9), 598–600. doi:10.1038/ngeo618
Bussmann, I., Koedel, U., Schütze, C., Kamjunke, N., and Koschorreck, M. (2022). Spatial. Variability and hotspots of methane concentrations in a large temperate river. Front. Environ. Sci. 10, 833936. doi:10.3389/fenvs.2022.833936
Cai, W. J., Guo, X., Chen, C. T. A., Dai, M., Zhang, L., Zhai, W. D., et al. (2008). A comparative overview of weathering intensity and HCO3− flux in the world’s major rivers with emphasis on the Changjiang, Huanghe, Zhujiang(Pearl) and Mississippi Rivers. Cont. Shelf Res. 28 (12), 1538–1549. doi:10.1016/j.csr.2007.10.014
Clark, I. D., and Fritz, P. (1997). Environmental isotopes in hydrogeology. New York: Lewis Publishers, 328.
Cole, J. J., Prairie, Y. T., Caraco, N. F., McDowell, W. H., Tranvik, L. J., Striegl, R. G., et al. (2007). Plumbing the global carbon cycle: Integrating inland waters into the terrestrial carbon budget. Ecosystems 10, 172–185. doi:10.1007/s10021-006-9013-8
Dubois, K. D., Lee, D., and Veizer, J. (2010). Isotopic constraints on alkalinity, dissolved organic carbon, and atmospheric carbon dioxide fluxes in the Mississippi river. J. Geophys. Res. 115, G02018. doi:10.1029/2009JG001102
Duchemin, E. (2000). Hydroelectricity and greenhouse gases: Emission evaluation and identification of the biogeochemical processes responsible for their production. Master's Thesis. Montréal, Québec, Canada: University of Québec at Montréal.
Einarsdottir, K., Wallin, M. B., and Sobek, S. (2017). High terrestrial carbon load via groundwater to a boreal lake dominated by surface water inflow. J. Geophys. Res. Biogeosci. 122 (1), 15–29. doi:10.1002/2016JG003495
Galy, A., and France-Lanord, C. (1999). Weathering processes in the ganges–brahmaputra basin and the riverine alkalinity budget. Chem. Geol. 159 (1–4), 31–60. doi:10.1016/s0009-2541(99)00033-9
Ge, L., Tan, H. B., Chen, X., Rao, W. B., and Fan, M. T. (2021). Dissolved inorganic carbon isotopes of a typical alpine river on the Tibetan Plateau revealing carbon sources, wetland effect and river recharge. Hydrol. Process. 35 (10), e14402. doi:10.1002/hyp.14402
Guillemette, F., McCallister, S. L., and delGiorgio, P. A. (2013). Differentiating the degradation dynamics of algal and terrestrial carbon within complex natural dissolved organic carbon in temperate lakes. J. Geophys. Res. Biogeosci. 118, 963–973. doi:10.1002/jgrg.20077
Hagedorn, B., and Cartwright, I. (2010). The CO2 system in rivers of the Australian victorian alps: CO2 evasion in relation to system metabolism and rock weathering on multi-annual time scales. Appl. Geochem. 25 (6), 881–899. doi:10.1016/j.apgeochem.2010.03.006
Hope, D., Palmer, S. M., Billett, M. F., and Dawson, J. J. (2004). Variations in dissolved CO2 and CH4 in a first-order stream and catchment: An investigation of soil–stream linkages. Hydrol. Process. 18 (17), 3255–3275. doi:10.1002/hyp.5657
Huang, X., Sillanpää, M., Gjessing, E. T., Peraniemi, S., and Vogt, R. D. (2011). Water quality in the southern Tibetan plateau: Chemical evaluation of the Yarlung Tsangpo (brahmaputra). River Res. Appl. 27 (1), 113–121. doi:10.1002/rra.1332
Immerzeel, W. W., and Bierkens, M. F. P. (2012). Asia’s water balance. Nat. Geosci. 5 (12), 841–842. doi:10.1038/ngeo1643
Jiang, K. (2020). Runoff composition of the Yarlung Zangbo River and its response to climate change. Master's Thesis. Harbin: Harbin Institute of Technology.
Jiang, L. G., Yao, Z. J., Wang, R., Liu, Z. F., Wang, L., and Wu, S. S. (2015). Hydrochemistry of the middle and upper reaches of the Yarlung Tsangpo River system: Weathering processes and CO2, consumption. Environ. Earth Sci. 74 (3), 2369–2379. doi:10.1007/s12665-015-4237-6
Jung, B. J., Lee, H. J., Jeong, J. J., Owen, J., Kim, B., Meusburger, K., et al. (2012). Storm pulses and varying sources of hydrologic carbon export from a mountainous watershed. J. Hydrol. X. 440, 90–101. doi:10.1016/j.jhydrol.2012.03.030
Li, S. Y., Lu, X. X., and Bush, R, T. (2013). CO2 partial pressure and CO2 emission in the lower Mekong river. J. Hydrol. X. 504, 40–56. doi:10.1016/j.jhydrol.2013.09.024
Li, S. Y., Lu, X. X., He, M., Zhou, Y., Li, L., and Ziegler, A. D. (2012). Daily CO2 partial pressure and CO2outgassing in the upper Yangtze River basin: A case study of the longchuan river China. J. Hydrol. X. 466, 141–150. doi:10.1016/j.jhydrol.2012.08.011
Liu, J., Yao, Z. J., and Chen, C. Y. (2007). Evolution trend and causation analysis of the runoff evolution in the Yarlung Zangbo River Basin. J. Nat. Res. 22 (3), 471–477. doi:10.3321/j.issn:1000-3037.2007.03.017
Liu, S. D., Lu, X. X., Xia, X. H., Zhang, S. R., Ran, L. S., Yang, X. K., et al. (2016). Dynamic biogeochemical controls on river pCO2 and recent changes under aggravating river impoundment: An example of the subtropical Yangtze River. Glob. Biogeochem. Cycles 30 (6), 880–897. doi:10.1002/2016GB005388
Liu, S., Maavara, T., Yang, X., and Brown, L. E. (2022). Editorial: Riverine biogeochemistry under increasing damming: Processes and impacts. Front. Environ. Sci. 10, 863255. doi:10.3389/fenvs.2022.863255
Liu, Z., Dreybrodt, W., and Wang, H. (2010). A new direction in effective accounting for the atmospheric CO2 budget: Considering the combined action of carbonate dissolution, the global water cycle and photosynthetic uptake of DIC by aquatic organisms. Earth. Sci. Rev. 99 (3–4), 162–172. doi:10.1016/j.earscirev.2010.03.001
Lu, W. (2018). Geochemical processes of CO2 production and CO2 exchange at the water-atmosphere interface in cascade reservoirs in southwest China. Doctoral thesis. China: Chinese Academy of Geological Sciences.
Lu, W., Wang, S., Yeager, K. M., Liu, F., Huang, Q., Yang, Y., et al. (2018). Importance of considered organic versus inorganic source of carbon to lakes for calculating net effect on landscape C budgets. J. Geophys. Res. Biogeosci. 123 (4), 1302–1317. doi:10.1002/2017JG004159
Marcé, R., Obrador, B., Morguí, J. A., Riera, J. L., Lopez, P., and Armengol, J. (2015). Carbonate weathering as a driver of CO2 supersaturation in lakes. Nat. Geosci. 8 (2), 107–111. doi:10.1038/NGEO2341
Meybeck, M. (1982). Carbon, nitrogen, and phosphorus transport by world rivers. Am. J. Sci. 82 (4), 401–450. doi:10.2475/ajs.282.4.401
Neal, C., House, W. A., and Down, K. (1998). An assessment of excess carbon dioxide partial pressures in natural waters based on pH and alkalinity measurements. Sci. Total Environ. 210 (211), 173–185. doi:10.1016/S0048-9697(98)00011-4
Ni, M. F., and Li, S. Y. (2022). Dynamics and internal links of dissolved carbon in a karst river system: Implications for composition, origin and fate. Water Res. 226, 119289. doi:10.1016/j.watres.2022.119289
Ni, M. F., Li, S. Y., Luo, J. C., and Lu, X. X. (2019). CO2 partial pressure and CO2 degassing in the Daning River of the upper Yangtze River, China. J. Hydrol. X. 569, 483–494. doi:10.1016/j.jhydrol.2018.12.017
Ni, M. F., Luo, J. C., and Li, S, Y. (2020). Dynamic controls on riverine pCO2 and CO2 outgassing in the dry-hot valley region of southwest China. Aquat. Sci. 82, 12. doi:10.1007/s00027-019-0685-5
Qu, B., Aho, K. S., Li, C. L., kang, S. C., Sillanpaa, M., Yan, F. P., et al. (2017). Greenhouse gases emissions in rivers of the Tibetan plateau. Sci. Rep. 7, 16573. doi:10.1038/s41598-017-16552-6
Ran, L., Lu, X, X., Yang, H., Li, L. Y., Yu, R. H., Sun, H. G., et al. (2015). CO2 outgassing from the Yellow River network and its implications for riverine carbon cycle. J. Geophys. Res. Biogeosci. 120 (7), 1334–1347. doi:10.1002/2015JG002982
Raymond, P. A., Hartmann, J., Lauerwald, R., Sobek, S., McDonald, C., Hoover, M., et al. (2013). Global carbon dioxide emissions from inland waters. Nature 503 (7476), 355–359. doi:10.1038/nature12760
Raymond, P. A., Zappa, C. J., Butman, D., Bott, T. L., Potter, J., Mulholland, P., et al. (2012). Scaling the gas transfer velocity and hydraulic geometry in streams and small rivers. Limnol. Oceanogr. 2, 41–53. doi:10.1215/21573689-1597669
Regnier, P., Friedlingstein, P., Ciais, P., Mackenzie, F, T., Gruber, N., Janssens, I, A., et al. (2013). Anthropogenic perturbation of the carbon fluxes from land to ocean. Nat. Geosci. 6 (8), 597–607. doi:10.1038/ngeo1830
Richey, J. E., Melack, J, M., Aufdenkampe, A. K., Ballester, V. M., and Hess, L. L. (2002). Outgassing from amazonian rivers and wetlands as a large tropical source of atmospheric CO2. Nature 416 (6881), 617–620. doi:10.1038/416617a
Sawakuchi, H. O., Bastviken, D., Enrich-Prast, A., Ward, N. D., Camargo, P. B., and Richey, J. E. (2021). Low diffusive methane emissions from the main channel of a large amazonian run-of-the-river reservoir attributed to high methane oxidation. Front. Environ. Sci. 9, 655455. doi:10.3389/fenvs.2021.655455
Striegl, R. G., Dornblaser, M. M., Mcdonald, C. P., Rover, J. R., and Stets, E. G. (2012). Carbon dioxide and methane emissions from the yukon river system. Glob. Biogeochem. Cycles 26, 2012GB004306. doi:10.1029/2012GB004306
Telmer, K., and Verzer, J. (1999). Carbon fluxes, pCO2 and substrate weathering in a large northern River Basin, Canada: Carbon isotope perspectives. Chem. Geol. 159, 61–86. doi:10.1016/S0009-2541(99)00034-0
Teodoru, C. R., Nyoni, F. C., Borges, A. V., Darchambeau, F., Nyambe, I., and Bouillon, S. (2015). Dynamics of greenhouse gases (CO2, CH4, N2O) along the zambezi River and major tributaries, and their importance in the riverine carbon budget. Biogeosciences 12 (8), 2431–2453. doi:10.5194/bg-12-2431-2015
Tong, Y. D., Chen, L., Chi, J., Zhen, G. C., Zhang, Q. Q., Wang, R. N., et al. (2016). Riverine nitrogen loss in the Tibetan Plateau and potential impacts of climate change. Sci. Total Environ. 553, 276–284. doi:10.1016/j.scitotenv.2016.02.099
Tranvik, L. J., Downing, J. A., Cotner, J. B., Loiselle, S. A., Striegl, R. G., Ballatore, T. J., et al. (2009). Lakes and reservoirs as regulators of carbon cycling and climate. Limnol. Oceanogr. 54 (6), 2298–2314. doi:10.4319/lo.2009.54.6_part_2.2298
UNESCO/IHAGHG (2010). Greenhouse gas emissions related to fresh water reservoirs. World Bank Report, 64–127.
Yan, F. P., Sillanpaa, M., Kang, S. C., Aho, K. S., Qu, B., Wei, D., et al. (2018). Lakes on the Tibetan plateau as conduits of greenhouse gases to the atmosphere. J. Geophys. Res. Biogeosci. 123 (7), 2091–2103. doi:10.1029/2017JG004379
Ye, R. C. (2019). Study on greenhouse gas emission characteristics and dissolved inorganic carbon sources in the Yarlung Zangbo river. Master's Thesis. Guiyang: Guizhou University.
Yu, Z. L., Yan, N., Wu, G. J., Xu, T. L., and Li, F. (2021). Chemical weathering in the upstream and midstream reaches of the Yarlung Tsangpo basin, southern Tibetan plateau. Chem. Geol. 559, 119906. doi:10.1016/j.chemgeo.2020.119906
Zhai, W. D., Dai, M. H., and Guo, X. H. (2007). Carbonate system and CO2 degassing fluxes in the inner estuary of changjiang (Yangtze) river, China. Mar. Chem. 107, 342–356. doi:10.1016/j.marchem.2007.02.011
Zhang, L. W., Xia, X. H., Liu, S. D., Zhang, S. B., Li, S. L., Wang, J. F., et al. (2020). Significant methane ebullition from alpine permafrost rivers on the east qinghai-tibet plateau. Nat. Geosci. 13 (5), 349–354. doi:10.1038/s41561-020-0571-8
Zhang, T., Li, J. H., Pu, J. B., Martin, J. B., Khadka, M. B., Wu, F. H., et al. (2017). River sequesters atmospheric carbon and limits the CO2 degassing in karst area, southwest China. Sci. Total Environ. 609, 92–101. doi:10.1016/j.scitotenv.2017.07.143
Zhang, T., Li, J. H., Pu, J. B., and Yuan, D. X. (2019). Carbon dioxide exchanges and their controlling factors in Guijiang River, SW China. J. Hydrol. X. 578, 124073. doi:10.1016/j.jhydrol.2019.124073
Zhao, Y., Wu, B. F., and Zeng, Y. (2013). Spatial and temporal patterns of greenhouse gas emissions from three Gorges reservoir of China. Biogeosciences 10 (2), 1219–1230. doi:10.5194/bg-10-1219-2013
Zhong, J. (2017). Chemical weathering and carbon biogeochemical processes in the upper changjiang basin impacted by the hydrological conditions. Doctoral thesis. Beijing: Chinese Academy of Geological Sciences.
Keywords: partial pressure of CO2, CO2 outgassing, dissolved inorganic carbon, Tibetan Plateau, Yarlung Tsangpo River
Citation: Bao Y, Hu M, Li S, Wang Y, Wen J, Wu X, Sun M and Du P (2023) Carbon dioxide partial pressures and emissions of the Yarlung Tsangpo River on the Tibetan Plateau. Front. Environ. Sci. 10:1036725. doi: 10.3389/fenvs.2022.1036725
Received: 05 September 2022; Accepted: 24 November 2022;
Published: 20 January 2023.
Edited by:
Carlo Camporeale, Politecnico di Torino, ItalyCopyright © 2023 Bao, Hu, Li, Wang, Wen, Wu, Sun and Du. This is an open-access article distributed under the terms of the Creative Commons Attribution License (CC BY). The use, distribution or reproduction in other forums is permitted, provided the original author(s) and the copyright owner(s) are credited and that the original publication in this journal is cited, in accordance with accepted academic practice. No use, distribution or reproduction is permitted which does not comply with these terms.
*Correspondence: Mingming Hu, aHVtaW5nbWluZ0Bpd2hyLmNvbQ==; Yuchun Wang, d2FuZ3ljQGl3aHIuY29t