- 1Jilin Academy of Agricultural Sciences, Changchun, China
- 2College of Horticulture, Shenyang Agricultural University, Shenyang, China
- 3Key Laboratory of Vegetation Ecology, Ministry of Education, Institute of Grassland Science, Northeast Normal University, Changchun, China
- 4Central South Inventory and Planning Institute of National Forestry and Grassland Administration, Changsha, China
Soil salinity is well known heterogeneous and various within natural soil environment. In Songnen grassland of Northeast China, grazing aggravates the saline-alkali heterogeneity in soil habitat, which led to dominant clonal plant species forming a variety of adaptive strategies. However, based on the previous studies of morphological plasticity and clonal integration in clonal plants, there was a lack of mechanism research on the spatial expansion strategy of clonal plants population subjected to clipping in saline-alkali heterogeneity soil patches. To address this knowledge gap, we carried out an experiment by applying different clipping intensities (0%, 35%, 70% of the above-ground biomass removed) to explore the spatial expansion strategy and morphological plasticity of Leymus chinensis and their belowground rhizomes in different heterogeneous saline-alkali patches. We found that, clipping significantly decreased the plant average height and above-ground biomass in homogeneous patches, especially heavy clipping had a significant adverse impact on plant belowground (rhizome, fine root) biomass and leaf area. However, there was no significant difference in the biomass, average height, and leaf area among the clipping treatments in low saline-alkali heterogeneous patches. In addition, the number of leaves, daughter plants and rhizome internode buds were significantly affected by saline-alkali heterogeneity alone, and low saline-alkali heterogeneity had a positive effect on these parameters. Biomass accumulation and rhizome expansion were gradually inhibited by the increasing of clipping intensity in a homogeneous environment, but the inhibitory effect of clipping was not significant in saline-alkali patches soil. We concluded that L. chinensis can respond to grazing or mowing disturbance by increasing tiller and daughter-plant numbers in a saline-alkali heterogeneous environment. The saline-alkali patches promoted clonal plant population spatial expansion more than grazing or mowing disturbance did. This study emphasizes saline-alkali heterogeneity distribution patterns in grassland soil environment were the primary and important driving factor promoting the spatial expansion of L. chinensis.
1 Introduction
In natural communities, resources (i.e., light, water and nutrients) usually show a heterogeneous distribution both spatially and temporally (Hutchings and De Kroon, 1994; Lajtha, 1995). The heterogeneous distribution of resources increases the difficulty for organisms, especially plants, to obtain effective resources (Hutchings and De Kroon, 1994), which has led to the evolution of diverse and effective ecological strategies throughout their long-term evolutionary process (Wang et al., 2011). Clonal integration and morphological plasticity of clonal plants are well known as strategies to adapt heterogeneous environments in a certain time period (De Kroon and Hutchings, 1995; Roiloa and Retuerto, 2006; Liu et al., 2018). Previous studies have shown that connections among ramets of clonal plants by rhizomes or stolons allow the transport of photosynthates, water, and nutrients from established to developing ramets (Alpert, 1999; Gao et al., 2008). Clonal plants exhibit a particularly foraging behavior of rhizome or stolon as a response to resource heterogeneity, such as clones would reduce the length of rhizome (stolon) spacers or increase branching in favorable environments, and place more ramets selectively in nutrient-rich habitats to promote access to heterogeneous resources (Hutchings and De Kroon, 1994; De Kroon and Hutchings, 1995). However, there is still a lack of experimental evidence on the mechanism of clonal plant spatial expansion under heterogeneous environments or interactive stresses. A number of studies have focused on changes in the above-ground parts of clonal plants in different habitats, while few have considered with changes in belowground root system. The clonal expansion of plants is a complex and important process that not only affects their performance, but also determines their mode of access to environmental resources (De Kroon and Hutchings, 1995; Alpert, 1999). The lateral rhizome and rhizome buds of clonal plants grow to form the above-ground ramets, and then the belowground rhizome buds of these ramets continue to repeat this process to achieve clonal expansion. In addition, this expansion process of clonal plants is also affected by both abiotic and biological factors (such as competition, herbivory, mycorrhiza, and environmental pressure) (Gao et al., 2012). Therefore, it can lead to the development of different plant adaptive strategies (Gao et al., 2008; Schiffers et al., 2011). For example, clonal plants reduce the size of the canopy and distribute more biomass to their belowground organs under severe grazing conditions (Wang et al., 2019). However, because of the complexity of root growth in natural grassland plants, belowground clonal growth remains poorly understood.
Environmental heterogeneity is diverse and multifaceted. Saline-alkali heterogeneity is one of the most important soil chemical properties affecting plant growth. Soil salinization has become an increasingly serious global environmental problem because of the large-scale degradation of grasslands (Munns and Tester, 2008; Yang et al., 2011). Salinity is always heterogeneously distributed in local habitats, especially on degraded grasslands. Because of different forms, proportions, and quantities of salt in the soil, soil alkalization often occurs along with salinization, which leads to the complexity of soil salinity heterogeneity (Wallender and Tanji, 2011). Moreover, with climate, topography, grazing, and other factors, soil salinization and patch distribution are intensified (Shi and Sheng, 2005). Researchers have found that saline-alkali stress can slow down plant growth rate and reduce the individual’s size, leaf area, root activity, and induce leaf chlorosis (i.e., yellowing of the leaves), resulting in a decrease in biomass accumulation and various other physiological processes (Wei et al., 2008; Zhao et al., 2008). However, clonal plants have a higher endurance than non-clonal plants in adverse habitats (such as salt, low nutrition, drought, etc.) (Zhao et al., 2008; Gao et al., 2012). A typical grassland clone plant, Leymus chinensis, has a high tolerance to saline-alkali environments, and can distribute its rhizomes or clonal ramets non-randomly in heterogeneous soil types (Ba et al., 2006; Li et al., 2014). Previous studies have shown that the root system of L. chinensis can have a “guerilla” distribution by increasing the rhizome branching angle and spacer length, which is helpful in avoiding the stress damage of saline-alkali soil patches (Shen and Ma, 2013). The heterogeneous distribution of salt and alkali cations in the soil promotes the formation of diversified adaptive strategies in saline-alkali tolerant plants, which is an important driving force for expansion of its population (Zhao et al., 2008; Türkana and Demiral, 2009).
In natural grasslands, plant communities are dominated by perennial herbaceous species. Grazing and mowing play an important role in maintaining grassland ecological health and production stability (Wang et al., 2004; Diaz et al., 2007; Bi et al., 2018). It is generally believed that grazing and mowing affect the plants directly by defoliation and indirectly by modifying their growth allocation and resource availability (Gao et al., 2008; Liu et al., 2015). Plant compensatory growth is an important adaptive response to herbivory, which may alleviate the potential deleterious effects of tissue damage. Many studies have confirmed that moderate clipping has positive effects on the growth of L. chinensis (i.e., increasing above-ground biomass, rhizome tillers and stimulating outgrowth of buds) (Wang, 2004; Zhao et al., 2008; Liu et al., 2012), but its compensation capacity requires both adequate nutrients and meristems to support vegetative regrowth or reproduction. In suitable habitats, clonal plants were able to produce sufficient active rhizome buds when basal meristems were stimulated by clipping. The degree of plant compensation capacity depends on asexual ramet production and expansion (Gao et al., 2012). Thus, a trade-off related to carbon allocation with the potential of morphological plasticity would be an important strategy to cope with herbivory.
Previous studies have shown that clonal growth in a heterogeneous environment is controlled by plasticity in branch production, internode distance, and angle of rhizome growth (Evans and Cain, 1995). A variety of morphological plasticity and differential foraging behaviors affect plant clonal growth and population dynamics (De Kroon and Hutchings, 1995; Kembel et al., 2008; Mcnickle et al., 2009). However, these studies overlooked the spatial clonal expansion strategies and failed to quantify and predict the associational effects, when plants faced contrasting stress and heterogeneous environments. Therefore, we explored population expansion strategies of clonal plants, providing direct evidence for the adaptation patterns of L. chinensis under heterogeneous environmental stress. Specifically, we addressed the following hypotheses: i) Soil saline-alkali heterogeneous patches are beneficial to the spatial expansion of clonal plant; ii) The effect of environmental stress (saline-alkali) on the expansion of plant is more significant than that of external disturbances (grazing or clipping).
2 Materials and methods
2.1 Study site and plant species
This experiment was conducted in a greenhouse located at the Research Station of Shenyang Agricultural University (41°50′N, 123°34′E). The station has a temperate semi-humid continental climate with an average annual temperature of 8.1°C and an average annual precipitation of 721.9 mm. Natural photoperiod, mean air temperature of 26/20°C day/night, 42%/69% day/night relative humidity were maintained throughout the growth stages.
Leymus chinensis (Trin.) Tzvel. is a typical perennial, rhizomatous clonal grass that is dominate in the Northern China, eastern Mongolia, and Siberia grasslands (Wang et al., 2004; Zhang et al., 2012; Li et al., 2014). It generally tolerates the damage caused by salinity, grazing, cold, and drought because of its ability for compensatory growth and well-developed rhizomes system for space expansion colonization (Wang et al., 2001; Ba et al., 2006; Wang et al., 2022).
2.2 Experimental design
In this study, one-meter-long plastic pots (1 m in length, .2 m in width, and .25 m in depth) were used to ensure that the clonal growth of L. chinensis individuals was not limited by the size of the pot, so that the new ramets could have enough space to establish and grow. Each pot was divided into two equal parts using a plastic board inserted at the center (Supplementary Figure S1). The two parts of each pot were filled with washed sand subjected to different saline-alkali stresses, but remain the upper 1 cm of sand from the soil surface was retained without treatment to allow plant roots to grow across heterogeneous patches.
Two neutral salts (NaCl and Na2SO4) and two alkaline salts (NaHCO3 and Na2CO3) were selected for this experiment to simulate the salt components in the soil of the Songnen Plain in Northeast China (Yang et al., 2011). Four salts were mixed at a molar ratio of 1:1:1:1, and according to different concentrations: no saline-alkali (0 mmol L−1, pH = 7.20, EC = 63 μs cm−1), low-saline-alkali (100 mmol L−1, pH = 8.97, EC = 523 μs cm−1), or high-saline-alkali (200 mmol L−1, pH = 9.70, EC = 1209 μs cm−1). Three saline concentrations were used to make different saline-alkali patches: homogeneous patches without saline-alkali conditions in either part of the pot (control, Figure 1A); and heterogeneous patches, which included two levels: 1) no saline-alkali conditions and low saline-alkali conditions in the same pot, separated by the inserted board (low saline-alkali heterogeneity, LS; Figures 1B, C), and 2) low and high saline-alkali conditions in the same pot, separated by the inserted board (high saline-alkali heterogeneity, HS; Figures 1D, E). To avoid differences in planting location of heterogeneous patches, two planting patterns were employed separately for the LS and HS heterogeneity treatments. Three clipping levels were used to defoliate 0% (no clipping, NC), 35% (moderate clipping, MC), and 70% (heavy clipping, HC) of the total above-ground biomass. The linear relationship between biomass and plant height is obtained through pre-test, and the corresponding biomass is calculated by measuring the plant height progress during each clipping treatment. Each treatment had five replicates and was conducted in a randomized block design with a total of 75 pots.
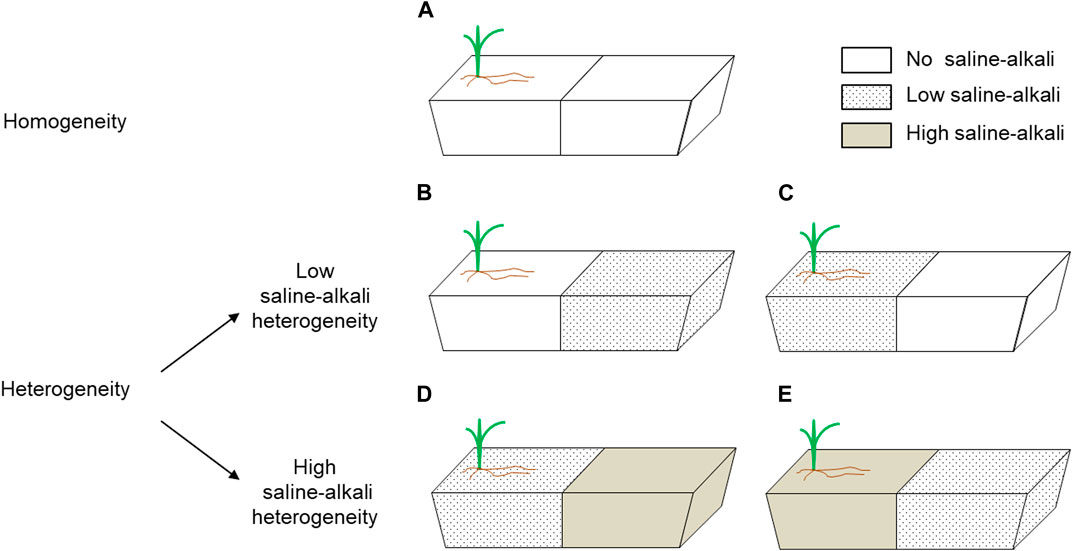
FIGURE 1. The planting configuration of the plants. Each long trough pot has one plant, which is planted on the side of the pot. Schematic representation of saline-alkali stress treatments given to different patches. (A) homogeneous patches without saline-alkali conditions in either part of the pot (homogeneity); (B,C) no saline-alkali conditions and low saline-alkali conditions in the same pot, separated by the inserted board (low saline-alkali heterogeneity); (D,E) low and high saline-alkali conditions in the same pot, separated by the inserted board (high saline-alkali heterogeneity).
L. chinensis seeds collected from the Songnen grassland (44°45′N, 123°45′E) were germinated in trays for 4 weeks. When the plants were approximately 10 cm in height and had three true leaves, 75 seedlings of uniform size and similar weight were transplanted into 75 long pots filled with 20 kg washed sand. Each seedling was positioned on one side of the long pot, approximately 10 cm from the edge. Based on the pre-experiments and the compensatory growth regulation ability of L. chinensis, clipping and saline-alkali treatments were carried out once a month from June to September after 30 days of adaptation, for a total of four times treatments during the experiment. 1.461 g NaCl, 3.551 g Na2SO4, 2.100 g NaHCO3, and 2.649 g Na2CO3 were dissolved in 1 L of distilled water and added to the LS, 2.922 g NaCl, 7.102 g Na2SO4, 4.200 g NaHCO3, and 5.298 g Na2CO3 were dissolved in 1 L of distilled water and added to the HS, while the soil in the control treatment received an equivalent amount of distilled water. Water availability was controlled by the water capacity test (Moisture Meter type HH2, Delta-T Devices Ltd., United Kingdom) of trays, which was conducted every 2 days, and by adding tap water until a predetermined sand water content was reached (75% of field capacity). During the experiment, an equal amount of Hoagland’s nutrient solution was added to each pot every 15 days. The experiment began in mid-May 2018 and ended in mid-September 2018, lasting a total of 120 days.
2.3 Sampling and measurements
After 30 days of clipping, five plants were selected arbitrarily from each pot to measure their height, and less than five plants to measure their full height. Plants were harvested and washed at the end of the growing period (14 September 2018; Supplementary Figure S2). Above- and belowground parts of each plant were separated and kept in individual paper bags. The number of daughter plants, leaves, rhizome apical buds, and internode buds were determined, and the leaf area was measured using a portable laser leaf area meter (CI-202, United States). The above-ground and belowground samples were then oven-dried for 48 h at 65°C and weighed.
The spatial expansion ability of L. chinensis was considered as a linear function of daughter plant growth under the interaction of soil saline-alkali heterogeneity and clipping. Every newborn daughter plant was labeled, and data on its rhizome expansion speed per day was recorded. The vertical distance between the daughter plant and the parent plant was also measured as belowground rhizome expansion distance. The spatial expansion ability was determined by linear regression analysis between daughter plant expansion distance and time using the following equation: Y = kX + b, where X represents the first appearance time of a newborn daughter plant after transplantation, Y represents the vertical distance between the daughter plant and the parent plant, and the slope k represents the extension rate of the daughter plant.
2.4 Statistical analysis
Before data analysis, the data of different planting patterns in the same heterogeneous patches was normalized. The effects of clipping, saline-alkali heterogeneity and their interactions on the biomass accumulation and functional characteristics of Leymus chinensis were analyzed using two-way ANOVA (Tables 1, 2). The least significant difference (LSD) test was performed to determine the difference in the biomass, average height, leaf area, and rhizome apical bud number in the different treatments. One-way ANOVA was also used to analyze the leaf number, daughter plant number, and rhizome internode bud number of plants experiencing saline-alkali heterogeneity stress. Statistical tests were performed using SPSS 22.0 (SPSS, Chicago, IL, United States). The significance level was set at p < .05.

TABLE 1. Statistical summary of two-way ANOVA evaluating the effects of saline-alkali heterogeneity of soil and clipping their combined effects on the above-ground biomass (AGB), belowground biomass (BGB), total biomass (TB), rhizome biomass (RB), fine root biomass (FRB) of L. chinensis. Values are F ratios and their significance for effects. F values and significance levels (**, p < .01; *, p < .05) are given.
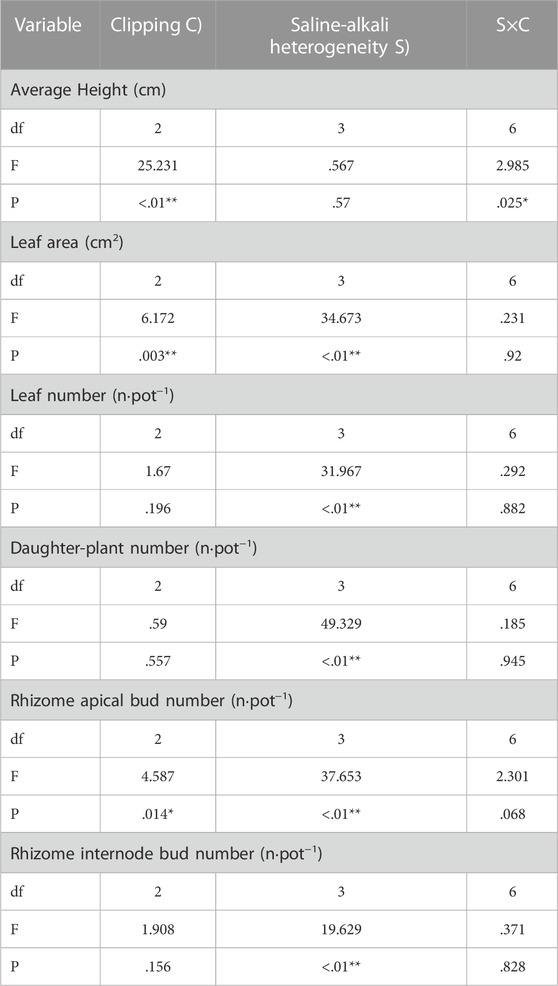
TABLE 2. Statistical summary of two-way ANOVA evaluating the effects of saline-alkali heterogeneity of soil and clipping their combined effects on the average height (AH), leaf area (LA), leaf number (LN), Daughter-plant number (DN), Rhizome apical bud number (RABN) and rhizome internode bud number (RIBN) of L. chinensis. Values are F ratios and their significance for effects. F values and significance levels (**, p < .01; *, p < .05) are given.
3 Results
3.1 Biomass accumulation
Saline-alkali heterogeneity or clipping alone significantly affected the above-ground, belowground (rhizome, fine root), and total biomass of L. chinensis. However, the interaction between saline-alkali heterogeneity and clipping had no significant effect on these parameters (Table 1). In the homogeneous patches, clipping significantly decreased plant above-ground biomass (AGB). Compared with the AGB in homogeneous soil, clipping had no significant effect on AGB under low saline-alkali heterogeneous patches, but heavy clipping significantly reduced the AGB under high saline-alkali heterogeneous patches. Without the clipping treatment, significantly decreased above-ground biomass of L. chinensis was only observed in high saline-alkali patches (Figure 2A).
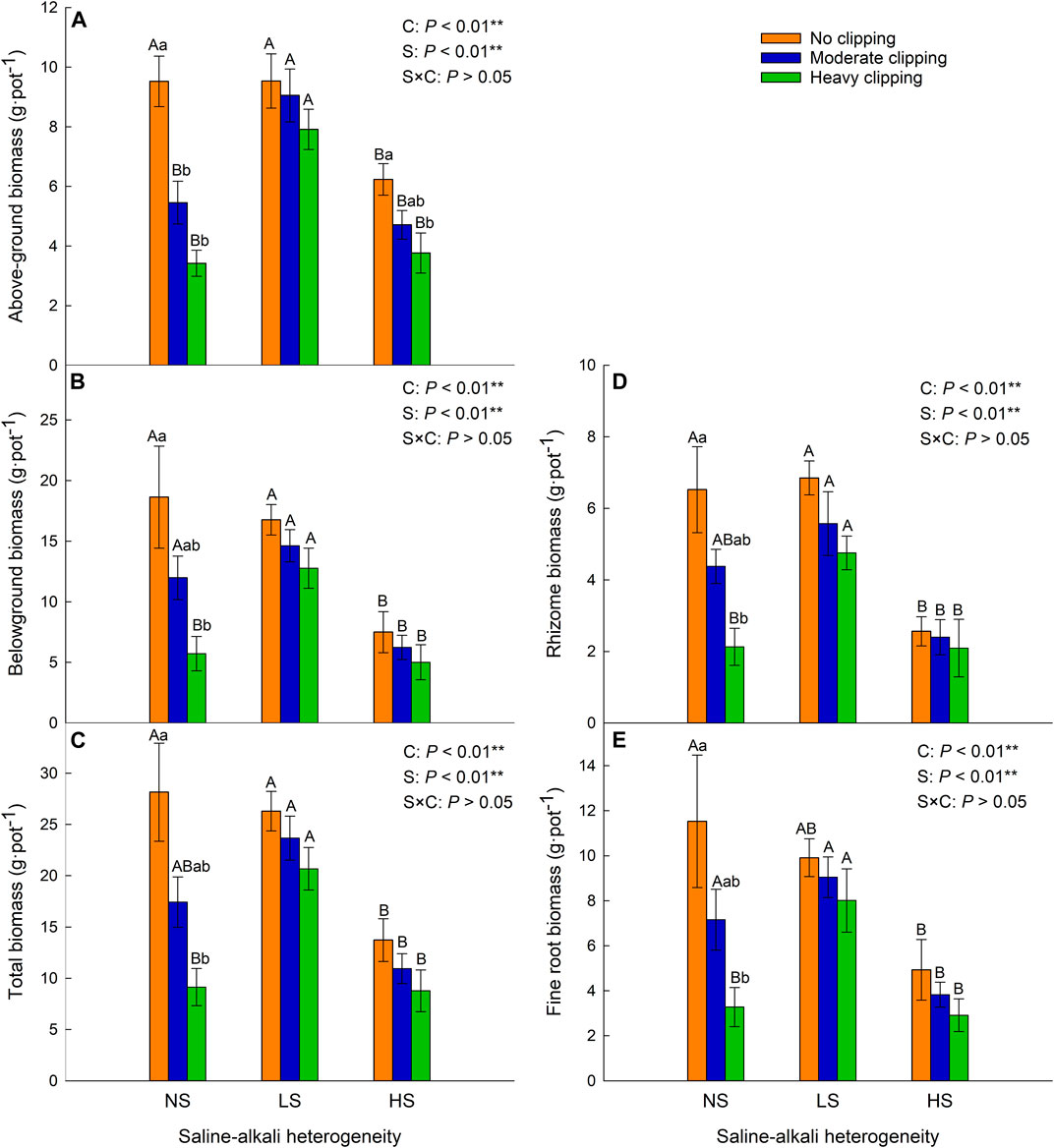
FIGURE 2. Effects of saline-alkali heterogeneity (S), clipping (C) and their interaction (S×C) on the above-ground biomass (A), belowground biomass (B), total biomass (C), rhizome biomass (D) and fine root biomass (E) of L. chinensis. Different lowercase letters represent significant difference (p < .05) of the clipping treatments in the same saline-alkali stress, and different uppercase letters represent significant difference (p < .05) of the saline-alkali heterogeneity in the same clipping treatment.
Belowground (rhizome, fine root) and total biomass decreased with increasing clipping intensity in homogeneous soil (Figures 2B–E). There was no significant difference in these parameters of the whole clones among different clipping intensities, neither at low nor at high saline-alkali heterogeneity levels. However, in high saline-alkali heterogeneous patches, these parameters were significantly decreased in comparison with those in no-clipping and homogeneous soil patches.
3.2 Plant traits
Saline-alkali heterogeneity significantly increased the daughter plant number of L. chinensis (Table 2; Figure 3B), but the number of leaves and rhizome internode buds was significantly increased only under low saline-alkali heterogeneous patches (Table 2; Figures 3A, C).
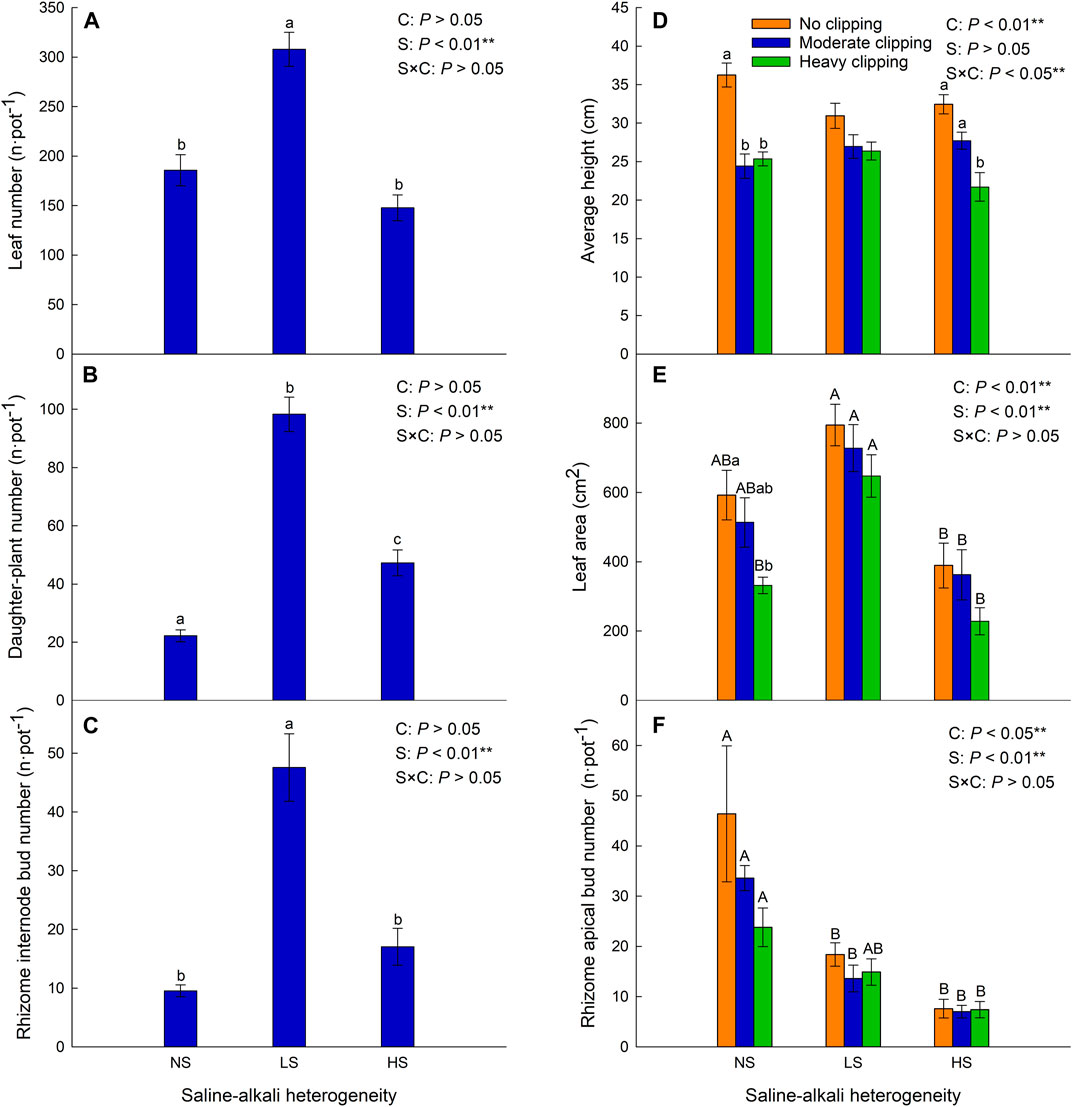
FIGURE 3. Effects of saline-alkali heterogeneity (S), clipping (C) and their interaction (S×C) on the leaf number (A), daughter plant number (B), rhizome internode bud number (C), average height (D), leaf area (E), and rhizome apical bud number (F) of L. chinensis. Different lowercase letters represent significant difference (p < .05) of the clipping treatments in the same saline-alkali stress, and different uppercase letters represent significant difference (p < .05) of the saline-alkali heterogeneity in the same clipping treatment.
Clipping alone or the interaction of saline-alkali heterogeneity and clipping had a significant impact on the average height (Table 2). In the homogeneous patches, clipping significantly decreased the average plant height (AH). Compared with homogeneous soil, clipping had no significant effect on AH in low saline-alkali heterogeneous patches, but high clipping intensity significantly reduced the AH in high saline-alkali heterogeneous patches (Figure 3D).
Saline-alkali heterogeneity or clipping alone significantly affected the leaf area and rhizome apical bud number (Table 2). In the homogeneous patches, heavy clipping significantly decreased the leaf area. Low saline-alkali heterogeneity maintained the leaf area of L. chinensis, but high saline-alkali heterogeneity significantly reduced it, especially in the heavy clipping treatment (Figure 3E).
There was no significant difference in the number of rhizome apical buds by clipping. Rhizome apical bud number significantly decreased in low and high saline-alkali heterogeneous patches compared to that in homogeneous patches, regardless of clipping.
3.3 Expansion rate
In the ideal habitat, homogeneous patches with no clipping disturbance (NS-NC), the daughter plant had the fastest expansion rate (k = .9677). This rate of L. chinensis increased with the increasing of clipping intensity in low saline-alkali heterogeneous patches (Figures 4D–F), but decreased with the increasing of clipping intensity in the high saline-alkali heterogeneous patches (Figures 4G–I). Furthermore, this expansion rate decreased with the increasing of soil saline-alkali concentration in heterogeneous patches under the same clipping intensity.
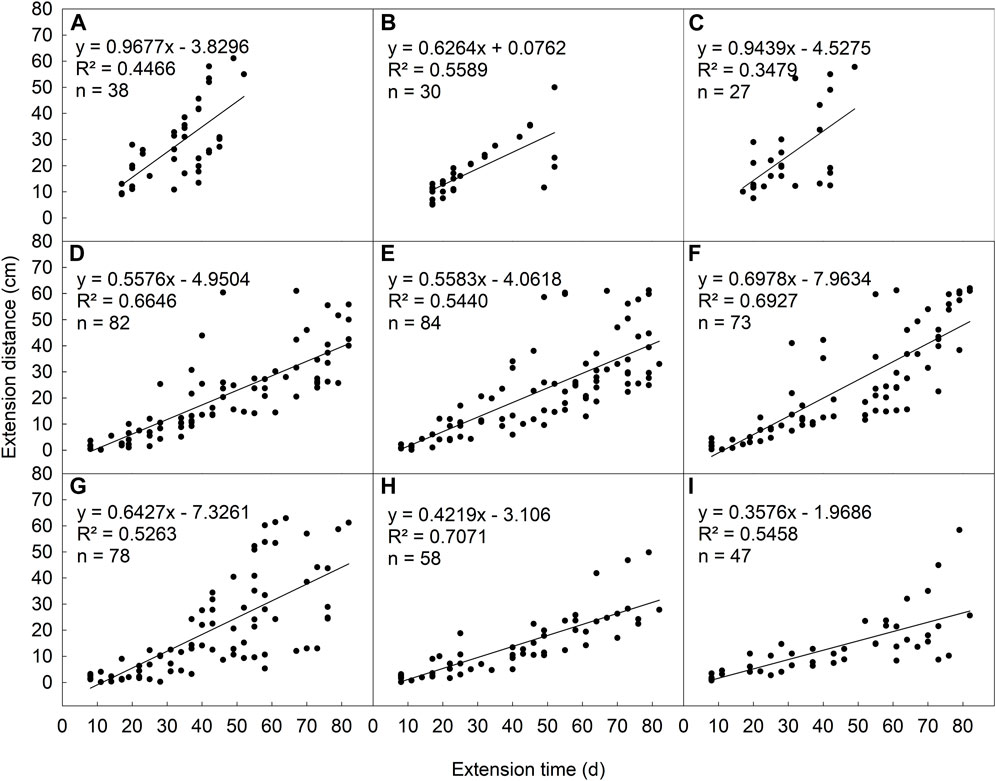
FIGURE 4. The relationship between extension distance and time of L. chinensis rhizome under different saline-alkali and clipping stress: homogeneity and no clipping (A), homogeneity and moderate clipping (B), homogeneity and heavy clipping (C), low saline-alkali heterogeneity and no clipping (D), low saline-alkali heterogeneity and moderate clipping (E), low saline-alkali heterogeneity and heavy clipping (F), high saline-alkali heterogeneity and no clipping (G), high saline-alkali heterogeneity and moderate clipping (H), high saline-alkali heterogeneity and heavy clipping (I).
4 Discussion
Clonal plants exhibit a variety of adaptations in response to environmental heterogeneity and stress, including changes in morphological plasticity, physiological integration, carbon allocation between functioning organs (De Kroon and Mommer, 2006; Kembel et al., 2008; Cahill et al., 2010). In the present study, we tested whether soil environmental heterogeneity may facilitate spatial expansion of clonal plant in Songnen grassland. Our results indicate that leaf areas, daughter plant and rhizome bud numbers of clonal plant were benefited from low saline-alkali heterogeneity patches. The underlying mechanism for the environmental heterogeneity effect might be plays a key role in plant population densities increase and spatial expansion. Although previous studies ascribed the positive relationship between biomass allocation and clonal expansion to mowing or grazing disturbance (Gao et al., 2008; Zhao et al., 2008; Wang et al., 2019), this relationship could also be driven by soil saline-alkali heterogeneity patches. The inter-relationship between herbivores and plants in grassland ecosystems is complex, with factors such as herbivore species, interspecific competition, climatic, and soil physicochemical properties greatly affecting the interspecific balance and ecosystem stability.
Biomass allocation is an important strategy for plants to respond to changes in resource availability and is a key factor in understanding the dynamic changes in plant population in various habitats (Yan et al., 2016; Zhang et al., 2017). In natural grassland, clipping and grazing directly affect the above-ground plant parts. Different frequencies and intensities of mowing or grazing can have different effects on plant dry matter distribution and biomass accumulation. In the present study, we found that clipping significantly decreased the above-ground biomass of L. chinensis in homogeneous patches, which was consistent with the results of previous studies (Bi et al., 2018). Clipping significantly reduced plant height, leaf area and weakened their photosynthetic capacity (Figure 3D), resulting in under-compensatory growth (Gao et al., 2012; Lu et al., 2020). In low saline-alkali heterogeneous patches, clipping had no significant effect on the biomass accumulation (Figures 2A–C), and similar findings were also reported in the study of grazing on Bromus ircutensis and Psammochloa villosa (Liu et al., 2009). This is related to the fact that low saline-alkali heterogeneity significantly increased the number of rhizome internode buds as well as the number of daughter plants (Figures 3B, C), therefore expanding its population size. These results implied that low saline-alkali heterogeneity buffered the negative effects of clipping on plant growth and promoted compensatory growth, which suggested that the compensatory response can increase the grazing tolerance of plant in low saline-alkali patches grassland. However, compensatory growth was not observed in the heavy saline-alkali heterogeneous patches (Figures 2A–C), because high salinity reduced the soil water potential, increase the difficulty of water absorption by plant roots, and caused physiological drought in plants (Munns and Tester, 2008; Geng et al., 2019). At the same time, the entering of salt into plant cells has a toxic effect, leading to a decrease in a series of physiological activities such as photosynthesis and resulting in undercompensated growth (Munns and Tester, 2008; Lu et al., 2020).
Leaves are the primary plant photosynthetic organs and are also considered the main source of dry matter accumulation (Guo et al., 2019). The increase in leaf area and number promoted the photosynthesis of L. chinensis, which increased the plant’s ability to obtain more nutrients and feed back to the belowground organs. We found that low salinity heterogeneity patches could significantly increase leaf area and numbers (Figures 3A, E). This was the reason why L. chinensis able to achieve compensatory growth in a low-saline-alkali heterogeneous environment and induce reasonable biomass allocation patterns between above- and belowground functional organs.
In perennial plant communities, the metabolic and maintenance costs of buds are so low that maintaining a large number of dormant buds is a growth strategy for plants. One such benefit is that plant can quickly respond to environmental changes by producing a large population of tillers or ramets (David et al., 2006). Rhizome buds are critical organs for vegetative reproduction of clonal plants (Munier-Jolain et al., 1996; Costes, 2003). Rhizome internode buds can grow upward to form new ramets, and rhizome apical buds can grow horizontally to form new rhizomes, which contribute to the growth and expansion of plant population (Wang et al., 2022). Several studies have indicated that defoliation can promote the production of buds as a compensation for damage (Wang et al., 2004; Lu et al., 2020), but our results showed that clipping had no significant effects on the rhizome apical or internode buds in saline-alkali heterogeneous patches (Table 2; Figures 3C, F). Moderate clipping was up close to the intercalary meristems and young leaves, and it exerted stimulating effect on axillary bud output and led to the increase in the number of tillers (Liu et al., 2012). Heavy clipping was carried out near the basal meristems, and it produced a signal to stimulate rhizome expansion by increasing rhizome length and quantity. However, the rhizome internode bud number increased significantly in low saline-alkali soil patches (Figure 3C). The heterogeneous distribution patterns of saline-alkali promoted the “development” strategy of L. chinensis, and stimulated the expansion of rhizomes and germination of internode buds to compensate for the serious loss caused by defoliation, then obtain more available resources. In addition, the heterogeneity of saline-alkali soil inhibited the growth of rhizome apical buds (Figure 3F), suggesting that clonal plants change the ratio of rhizome apical buds to internode buds in order to achieve the optimal growth pattern in response to environmental stress.
Expansion through rhizomes (stolons) for greater physical space and resource in heterogeneous environment is important adaptive strategy for regrowth and population expansion of clonal plants after external disturbances (Wang et al., 2019). In the homogeneous environment without stress or disturbance, the expansion rate of L. chinensis was the fastest due to sufficient nutrition supply and growth space (Figure 4A). However, L. chinensis rapidly compensated for the loss of leaves and photosynthesis through tillering rather than rhizome expansion under moderate mowing or grazing. Therefore, rhizome expansion rate was reduced (Figure 4B). Heavy clipping was significantly accelerating the expansion rate (Figure 4C). Although, clipping greatly damaged the above-ground parts of the plant and reduced photosynthesis and material accumulation, but the loss of above-ground plant parts stimulates the expansion of belowground rhizome and production of rhizome buds or daughter plants, thus maintaining plant growth (Lu et al., 2020). In contrast, the expansion rate increased with increasing clipping or grazing intensity in the low-saline-alkali heterogeneous patches, but slowed down with increasing clipping intensity in high saline-alkali soil patches. Our result suggested that the saline-alkali heterogeneous of soil may mediate the effects of mowing or grazing in grasslands. Interactive effects to influence plant population expansion were not consistent at different levels of saline-alkali or patches in habitat. This emphasizes soil saline-alkali heterogeneity plays an important role in driving the spatial expansion of clonal plant, and provided a reference for utilization the ecological restoration of degraded and heterogeneous grassland patches.
5 Conclusion
Our study suggests that environmental pressure was the most important factor limiting the population size of clonal plant. The heterogeneous distribution of saline-alkali in the soil limited the growth, reproduction, and spatial expansion of L. chinensis by regulating the proportion of apical and internode rhizome buds. While grazing or clipping reduced its biomass accumulation, saline-alkali heterogeneity buffered the negative effects and promoted its compensatory growth. Therefore, besides human disturbance factors (grazing or clipping), we believed that saline-alkali heterogeneity distribution pattern in the soil was an important driving factor promoting the spatial expansion of plant. However, other factors affecting the formation and development of L. chinensis populations were not been investigated individually. Therefore, it is suggested that verify the environmental and biological factors affecting the expansion of plant populations in different environments and grassland types, as well as to explore the dynamic changes in L. chinensis populations.
Data availability statement
The original contributions presented in the study are included in the article/Supplementary Material, further inquiries can be directed to the corresponding authors.
Author contributions
JL, LB, ZL, and ZW conceived the idea; LQ and NL collected and analyzed the data; LQ and JL wrote the original draft of the manuscript. JY, YH, and HY contributed to the revision of the manuscript.
Funding
The research was funded by the Agricultural Science and Technology Innovation Engineering Talent Fund Project of Jilin Province (CXGC2021RCG007), the National Natural Science Foundation of China (No. 31600327, 32071858), the Scientific and Technological Innovation Talent Training Program of Science and Technology Department of Jilin Province (20160520056JH).
Acknowledgments
We would like to thank the reviewers for their valuable comments and suggestions. We are also grateful to Changqing Yang, Pengchong Yin, and Hongkun Han for their help in the experimental operation process.
Conflict of interest
The authors declare that the research was conducted in the absence of any commercial or financial relationships that could be construed as a potential conflict of interest.
Publisher’s note
All claims expressed in this article are solely those of the authors and do not necessarily represent those of their affiliated organizations, or those of the publisher, the editors and the reviewers. Any product that may be evaluated in this article, or claim that may be made by its manufacturer, is not guaranteed or endorsed by the publisher.
Supplementary material
The Supplementary Material for this article can be found online at: https://www.frontiersin.org/articles/10.3389/fenvs.2022.1106825/full#supplementary-material
References
Alpert, P. (1999). Effects of clonal integration on plant plasticity in Fragaria chiloensis. Plant Ecol. 141, 99–106. doi:10.1023/A:1009823015170
Ba, L., Wang, D. L., Hodgkinson, K. C., and Xiao, N. Z. (2006). Competitive relationships between two contrasting but coexisting grasses. Plant Ecol. 183, 19–26. doi:10.1007/s11258-005-9002-6
Bi, X., Li, B., Fu, Q., Fan, Y., Ma, L. X., Yang, Z. H., et al. (2018). Effects of grazing exclusion on the grassland ecosystems of mountain meadows and temperate typical steppe in a mountain-basin system in Central Asia's arid regions, China. Sci. Total Environ. 630, 254–263. doi:10.1016/j.scitotenv.2018.02.055
Cahill, J. F., Mcnickle, G. G., Haag, J. J., Lamb, E. G., Nyanumba, S. M., and St. Clair, C. C. (2010). Plants integrate information about nutrients and neighbors. Science 328, 1657. doi:10.1126/science.1189736
Costes, E. (2003). Winter bud content according to position in 3-year-old branching systems of 'Granny Smith' apple. Ann. Bot. 92, 581–588. doi:10.1093/aob/mcg178
David, C. H., Moffat, P. S., and Harmony, J. D. (2006). Bud banks of perennial savanna grasses in Botswana. Afr. J. Ecol. 44, 256–263. doi:10.1111/j.1365-2028.2006.00646.x
De Kroon, H., and Hutchings, M. J. (1995). Morphological plasticity in clonal plants: The foraging concept reconsidered. J. Ecol. 83, 143–152. doi:10.2307/2261158
De Kroon, H., and Mommer, L. (2006). Root foraging theory put to the test. Trends Ecol. Evol. 21, 113–116. doi:10.1016/j.tree.2005.11.021
Diaz, S., Lavorel, S., Mcintyre, S., Falczuk, V., Casanoves, F., Milchunas, D. G., et al. (2007). Plant trait responses to grazing - a global synthesis. Glob. Chang. Biol. 13, 313–341. doi:10.1111/j.1365-2486.2006.01288.x
Evans, J. P., and Cain, M. L. (1995). A spatially explicit test of foraging behavior in a clonal plant. Ecology 76, 1147–1155. doi:10.2307/1940922
Gao, Y., Wang, D. L., Ba, L., Bai, Y. G., and Liu, B. (2008). Interactions between herbivory and resource availability on grazing tolerance of Leymus chinensis. Environ. Exp. Bot. 63, 113–122. doi:10.1016/j.envexpbot.2007.10.030
Gao, Y., Xing, F., Jin, Y. J., Nie, D. D., and Wang, Y. (2012). Foraging responses of clonal plants to multi-patch environmental heterogeneity: Spatial preference and temporal reversibility. Plant Soil 359, 137–147. doi:10.1007/s11104-012-1148-0
Geng, G., Lv, C. H., Stevanato, P., Li, R. R., Liu, H., Yu, L. H., et al. (2019). Transcriptome analysis of salt-sensitive and tolerant genotypes reveals salt-tolerance metabolic pathways in sugar beet. Int. J. Mol. Sci. 20, 5910. doi:10.3390/ijms20235910
Guo, T. T., Wang, D. F., Fang, J. J., Zhao, J. F., Yuan, S. J., Xiao, L. T., et al. (2019). Mutations in the rice OsCHR4 gene, encoding a CHD3 family chromatin remodeler, induce narrow and rolled leaves with increased cuticular wax. Int. J. Mol. Sci. 20, 2567. doi:10.3390/ijms20102567
Hutchings, M. J., and De Kroon, H. (1994). Foraging in plants: The role of morphological plasticity in resource acquisition. Adv. Ecol. Res. 25, 159–238. doi:10.1016/S0065-2504(08)60215-9
Kembel, S. W., De Kroon, H., Cahill, J. F., and Mommer, L. (2008). Improving the scale and precision of hypotheses to explain root foraging ability. Ann. Bot. 101, 1295–1301. doi:10.1093/aob/mcn044
Li, X. Y., Wang, J. F., Lin, J. X., Wang, Y., and Mu, C. S. (2014). Rhizomes help the forage grass Leymus chinensis to adapt to the salt and alkali stresses. Sci. World J. 213401, 1–15. doi:10.1155/2014/213401
Liu, H. D., Yu, F. H., He, W. M., Chu, Y., and Dong, M. (2009). Clonal integration improves compensatory growth in heavily grazed ramet populations of two inland-dune grasses. Flora 204, 298–305. doi:10.1016/j.flora.2008.03.003
Liu, J., Feng, C., Wang, D. L., Wang, L., Wilsey, B. J., and Zhong, Z. W. (2015). Impacts of grazing by different large herbivores in grassland depend on plant species diversity. J. Appl. Ecol. 52, 1053–1062. doi:10.1111/1365-2664.12456
Liu, J. S., Wang, L., Wang, D. L., Bonser, S. P., Sun, F., Zhou, Y. F., et al. (2012). Plants can benefit from herbivory: Stimulatory effects of sheep saliva on growth of Leymus chinensis. PLoS One 7, e29259. doi:10.1371/journal.pone.0029259
Liu, L. L., Du, N., Pei, C. P., Guo, X., and Guo, W. H. (2018). Genetic and epigenetic variations associated with adaptation to heterogeneous habitat conditions in a deciduous shrub. Ecol. Evol. 8, 2594–2606. doi:10.1002/ece3.3868
Lu, N., Qu, L. H., Liu, J., Yang, J. Y., Bai, L., Huang, Y., et al. (2020). Leymus chinensis tolerates mowing disturbance by maintaining photosynthesis in saline-alkali heterogeneous habitats. J. Sensors 2020, 1–10. doi:10.1155/2020/4510275
Mcnickle, G. G., St. Clair, C. C., and Cahill, J. F. (2009). Focusing the metaphor: Plant root foraging behaviour. Trends Ecol. Evol. 24, 419–426. doi:10.1016/j.tree.2009.03.004
Munier-Jolain, N. M., Ney, B., and Duthion, C. (1996). Analysis of branching in spring-sown white lupins (Lupinus albus L.): The significance of the number of axillary buds. Ann. Bot. 77, 123–131. doi:10.1006/anbo.1996.0014
Munns, R., and Tester, M. (2008). Mechanisms of salinity tolerance. Annu. Rev. Plant Biol. 59, 651–681. doi:10.1146/annurev.arplant.59.032607.092911
Roiloa, S. R., and Retuerto, R. (2006). Small-scale heterogeneity in soil quality influences photosynthetic efficiency and habitat selection in a clonal plant. Ann. Bot. 98, 1043–1052. doi:10.1093/aob/mcl185
Schiffers, K., Tielborger, K., Tietjen, B., and Jeltsch, F. (2011). Root plasticity buffers competition among plants: Theory meets experimental data. Ecology 92, 610–620. doi:10.1890/10-1086.1
Shen, Z. R., and Ma, T. (2013). Effect of saline-alkali white muscardine soil and aeolian sandy soil on clonal architecture of Leymus chinensis. Ningxia J. Agri. Fores. Sci. Tech. 54, 46–48.
Shi, D. C., and Sheng, Y. M. (2005). Effect of various salt-alkaline mixed stress conditions on sunflower seedlings and analysis of their stress factors. Environ. Exp. Bot. 54, 8–21. doi:10.1016/j.envexpbot.2004.05.003
Türkana, I., and Demiral, T. (2009). Recent developments in understanding salinity tolerance. Environ. Exp. Bot. 67, 2–9. doi:10.1016/j.envexpbot.2009.05.008
Wallender, W. W., and Tanji, K. K. (2011). Agricultural salinity assessment and management. New York: ASCE.
Wang, J. Y., Abdullah, I., Xu, T. T., Zhu, W. Y., Gao, Y., and Wang, L. (2019). Effects of mowing disturbance and competition on spatial expansion of the clonal plant Leymus chinensis into saline-alkali soil patches. Environ. Exp. Bot. 168, 103890. doi:10.1016/j.envexpbot.2019.103890
Wang, J. Y., Xu, T. T., Feng, X. Y., Zhu, W. Y., Zhang, L., Pan, D. F., et al. (2022). Simulated grazing and nitrogen addition facilitate spatial expansion of Leymus chinensis clones into saline-alkali soil patches: Implications for songnen grassland restoration in Northeast China. Land Degrad. Dev. 33, 710–722. doi:10.1002/ldr.4169
Wang, R. Z. (2004). Responses of Leymus chinensis (Poaceae) to long-term grazing disturbance in the Songnen grasslands of north-eastern China. Grass Forage Sci. 59, 191–195. doi:10.1111/j.1365-2494.2004.00417.x
Wang, R. Z., Ripley, E. A., Zu, Y. G., and Nie, S. Q. (2001). Demography of reproductive and biomass allocation of grassland and dune Leymus chinensis on the Songnen Plain, north-eastern China. J. Arid. Environ. 49, 289–299. doi:10.1006/jare.2000.0787
Wang, Z. W., Li, L. H., Han, X. G., and Dong, M. (2004). Do rhizome severing and shoot defoliation affect clonal growth of Leymus chinensis at ramet population level? Acta Oecol 26, 255–260. doi:10.1016/j.actao.2004.08.007
Wang, Z. W., Li, Y. H., During, H. J., and Li, L. H. (2011). Do clonal plants show greater division of labour morphologically and physiologically at higher patch contrasts? PLoS One 6, e25401. doi:10.1371/journal.pone.0025401
Wei, Y., Dong, M., Huang, Z. Y., and Tan, D. Y. (2008). Factors influencing seed germination of Salsola affinis (Chenopodiaceae), a dominant annual halophyte inhabiting the deserts of Xinjiang. China. Flora 203, 134–140. doi:10.1016/j.flora.2007.02.003
Yan, B. G., Ji, Z. H., Fan, B., Wang, X. M., He, G. X., Shi, L. T., et al. (2016). Plants adapted to nutrient limitation allocate less biomass into stems in an arid-hot grassland. New Phytol. 211, 1232–1240. doi:10.1111/nph.13970
Yang, J. Y., Zheng, W., Tian, Y., Wu, Y., and Zhou, D. W. (2011). Effects of various mixed salt-alkaline stresses on growth, photosynthesis, and photosynthetic pigment concentrations of Medicago ruthenica seedlings. Photosynthetica 49, 275–284. doi:10.1007/s11099-011-0037-8
Zhang, K., Su, Y. Z., and Yang, R. (2017). Biomass and nutrient allocation strategies in a desert ecosystem in the Hexi Corridor, northwest China. J. Plant Res. 130, 699–708. doi:10.1007/s10265-017-0940-6
Zhang, W. J., Zhang, Y. J., Song, J. J., Chen, W. Q., and Yang, G. W. (2012). Clonal Integration for the L. chinensis in a heterogeneous alkaline stress environment. J. Anim. Vet. Adv. 11, 3796–3799. doi:10.3923/javaa.2012.3796.3799
Keywords: saline-alkali heterogeneity, clipping, spatial expansion, rhizome bud, morphological plasticity
Citation: Qu L, Liu J, Yang J, Bai L, Huang Y, Lu N, Yu H, Wang Z and Li Z (2023) Soil saline-alkali heterogeneity is an important factor driving the spatial expansion of clonal plant in grassland. Front. Environ. Sci. 10:1106825. doi: 10.3389/fenvs.2022.1106825
Received: 24 November 2022; Accepted: 30 December 2022;
Published: 11 January 2023.
Edited by:
Xiaoyu Li, Northeast Institute of Geography and Agroecology (CAS), ChinaReviewed by:
Jixiang Lin, Northeast Forestry University, ChinaWentao Luo, Institute of Applied Ecology (CAS), China
Copyright © 2023 Qu, Liu, Yang, Bai, Huang, Lu, Yu, Wang and Li. This is an open-access article distributed under the terms of the Creative Commons Attribution License (CC BY). The use, distribution or reproduction in other forums is permitted, provided the original author(s) and the copyright owner(s) are credited and that the original publication in this journal is cited, in accordance with accepted academic practice. No use, distribution or reproduction is permitted which does not comply with these terms.
*Correspondence: Jun Liu, liuj872@nenu.edu.cn; Zhonghe Li, lizhonghe6@126.com