- 1Department of Chemistry, Université de Montréal (UdeM), Montréal, QC, Canada
- 2Centre d’expertise en analyse environnementale du Québec (CEAEQ), Ministère de l’Environnement et de la Lutte contre les changements climatiques, Québec, QC, Canada
- 3Department of Civil Engineering, McGill University, Montréal, QC, Canada
- 4Département des Sciences de l’Environnement, Université du Québec à Trois-Rivières (UQTR), Trois-Rivières, QC, Canada
We examined the influence of Montreal wastewater treatment plant (WWTP) effluents on two top predators, Walleye (Sander vitreus) and Sauger (Sander canadensis), with a focus on δ15N isotopic signatures and per- and polyfluoroalkyl substances (PFAS). These two fish species were collected in the summer 2013 in the St. Lawrence River upstream and downstream from a major WWTP, as well as in background sites (semi-remote lakes). Most of the δ15N variations for Sauger and Walleye are attributable to 1) δ15N values of the primary producers and sewage-derived particulate organic matter (SDPOM) at the base of the trophic food chain, 2) agricultural activities combined with biogeochemical processes, and 3) food web length. δ15N was significantly lower in fish collected in the effluent-mixed water masses than other sites of the St. Lawrence River, attributed to the SDPOM of the WWTP effluent. Relative to the background sites, certain PFAS were present at much higher levels in the St. Lawrence River, with profiles dominated by perfluoroalkyl sulfonates (PFSA). However, PFSA profiles generally remained consistent along the St. Lawrence River. PFOS levels in fish from the St. Lawrence exceeded the current Federal Environmental Quality Guidelines for protecting piscivorous mammals or birds. However, the human chronic daily intake of PFOS remained below current thresholds suggested by national agencies.
Introduction
Per- and polyfluoroalkyl substances (PFAS) encompass thousands of man-made chemicals, with some compounds having high persistence and toxicity. In recent years, worldwide contamination with PFAS has attracted extensive research interests (Cordner et al., 2021). PFAS are ubiquitous and found in the global environment (e.g., including arctic ecosystems) and humans, but there are still data gaps in fully understanding their impacts on humans and environmental health (Zhu et al., 2021). Initial reports on PFAS have essentially targeted perfluoroalkyl acids (PFAA), including perfluorooctane sulfonate (PFOS) and perfluorooctanoic acid (PFOA). Considerable efforts have been engaged to understand the environmental fate of legacy and current-use PFAS. These include, for instance, environmental baseline studies (Shi et al., 2010; Stahl et al., 2014; Akerblom et al., 2017; Kaboré et al., 2022) and monitoring studies at or downstream from impacted sites (Malinsky et al., 2011; Gewurtz et al., 2014; Bhavsar et al., 2016; Munoz et al., 2017; Nickerson et al., 2021; Liu et al., 2022).
Data gaps remain concerning the fate of PFAS in major hydrosystems, such as the St. Lawrence River. The St. Lawrence is a complex ecosystem impacted by dense population centers and industrial activities along its shores and those of the Laurentian Great Lakes that feed into it (Marcogliese et al., 2015). Since 1999, Environment and Climate Change Canada (ECCC), Québec’s Ministère de l’Environnement et de la Lutte contre les changements climatiques (MELCC), and academic collaborators have evaluated the impact of municipal wastewaters on the St. Lawrence River. Targeted contaminants included trace metals (e.g., Cd, Cr, Pb, and Hg), polychlorinated biphenyls, combustion-derived polycyclic aromatic hydrocarbons, surfactants (e.g., nonylphenols), flame retardants (e.g., polybrominated diphenyl ethers), and pharmaceutical drugs (Marcogliese et al., 2015). PFAS have also been the focus of a few previous studies in the St. Lawrence River. For instance, Houde et al. (2013) reported the induction of gene responses in St. Lawrence River Northern Pike (Esox lucius) environmentally exposed to 12 PFAS, including the newly detected perfluoroethylcyclohexane sulfonate (PFEtCHS). Significant relationships were found between perfluorotridecanoate (PFTrDA), perfluorotetradecanoate (PFTeDA) and perfluorodecane sulfonate (PFDS) plasma concentrations and vitellogenin (Vtg) gene expression in liver, Vtg activity in plasma, suggesting possible endocrine effects (Houde et al., 2013). Long-chain perfluoroalkane sulfonates (C8,C10 PFSA), long-chain perfluorocarboxylic acids (C9-C13 PFCA), and PFEtCHS were also detected in whole-body homogenates of St. Lawrence River Yellow Perch (Perca flavescens) (Houde et al., 2014).
The St. Lawrence River receives substantial loadings of sewage-derived particulate organic matter (SDPOM, 100 mg L−1) with total phosphorus of 0.5 mg L−1 and total nitrogen of 9 mg L−1 (deBruyn et al., 2003). The sewage is an important contributor to the nitrogen budget of the St. Lawrence River ecosystem, and also exerts influences on fish body size and isotopic distributions (deBruyn et al., 2002; deBruyn et al., 2003). Stable isotope analyses of carbon (δ13C) and nitrogen (δ15N) revealed that macroinvertebrates within the effluent plume downstream of Montreal WWTP effluent from the St. Lawrence River consumed sewage-derived resources, whereas those outside the effluent plume ingested seston and epiphytic resources (deBruyn and Rasmussen, 2002; deBruyn et al., 2003). Fish species feeding in the effluent plume also had a distinct δ15N isotopic signature vs. those feeding outside the effluent plume (Pelletier et al., 2022). How sewage-derived resources may influence δ15N and PFAS levels of piscivorous fishes in the St. Lawrence effluent-impacted area remains to be confirmed.
Walleye and Sauger are two sympatric species regularly found in large rivers and lakes of North America (Haxton and Friday, 2019). Segregation by depth, temperature and turbidity enables the two species to coexist, with distinct habitats and food resources (Haxton and Friday, 2019). No information is currently available on the occurrence of PFAS in these two species and how their δ15N are distributed before/after the WWTP effluent. Here, we studied the occurrence of PFAS and the distribution of δ15N isotopic signatures in Walleye and Sauger from the St. Lawrence River in a longitudinal gradient impacted by sewage effluents. Several sites along this large, temperate river were targeted to understand how PFAS occurred from upstream-downstream of the Montreal WWTP effluent relative to background sites. Both liver and muscle tissues were considered for analysis, considering the organotropism of PFAS in select organs (Ahrens et al., 2015) and the relevance of edible tissues for human dietary exposure. Key objectives were to 1) assess the occurrence and distribution of some legacy and newly identified PFAS across the river water masses; 2) understand how top predatory fish δ15N signatures are distributed as a result of bottom-up food source uptake in different water masses; and 3) characterize exposure to targeted PFAS and the risks PFOS may pose to the fish themselves, piscivorous mammals, birds and humans.
Materials and Methods
Chemicals and Materials
Details on solvents, chemicals, and other materials are provided in the Supporting Information (Supplementary Text S1). Native PFAS standards were obtained from Wellington Labs, Inc. (Guelph, ON, Canada) at chemical purities >98%. Full details on native analytes, including compound name, acronym, and exact m/z, are provided in Supplementary Table S1. In addition, cationic and zwitterionic PFAS synthesized at the Fluobon Surfactant Institute (Beijing University, China) were used to provide a reference for the semi-quantification of a range of newly identified PFAS (Barzen-Hanson et al., 2017; Mejia-Avendaño et al., 2017). Isotope-labelled internal standards were obtained from Wellington Labs, Inc. (Guelph, ON, Canada) at chemical purities >98% and isotopic purities >99% per 13C or >94% per 18O.
Study Site Description
The St. Lawrence River is exposed to a multitude of anthropogenic stresses and the Québec section hosts nearly 70% of Quebec’s population (∼5.5 million) living on its shores along with 75% of all industry in the province (Desrosiers et al., 2008). This study was carried out on the Lac des Deux-Montagnes, the fluvial portion, and Montreal-Sorel corridor of the St. Lawrence River (Québec, Canada), covering a 160-km tract of the St. Lawrence from the inlet of Lac Saint-Louis to the Lac Saint-Pierre outlet (see Figure 1). At the Island of Montreal, the river has three main water masses: the clear, hard, “green water” of the Great Lakes (GL), the humic, soft, “brown water” of Ottawa River (OR), and the Montreal WWTP effluent. The Montreal WWTP is the primary treatment facility serving more than two million people and thousands of commercial establishments on the Island of Montreal (deBruyn et al., 2002; deBruyn et al., 2003). Wastewater undergoes primary physical treatment (coagulation plus settling) to result in 85% reduction of suspended solids by and then is directly discharged into the St. Lawrence River via an underwater pipe at a depth of 7 m. The effluent is discharged at an average rate of 2 × 106 m3 day−1 and contains approximately 100 mg L−1 particulate organic matter of wet mass, 0.5 mg L−1 total phosphorus, and 9 mg L−1 total nitrogen. This discharge contributes approximately 450 t year−1 total phosphorus, 8,000 t year−1 total nitrogen, and 90,000 t year−1 of SDPOM to the River (deBruyn et al., 2002; deBruyn et al., 2003; Houde et al., 2013).
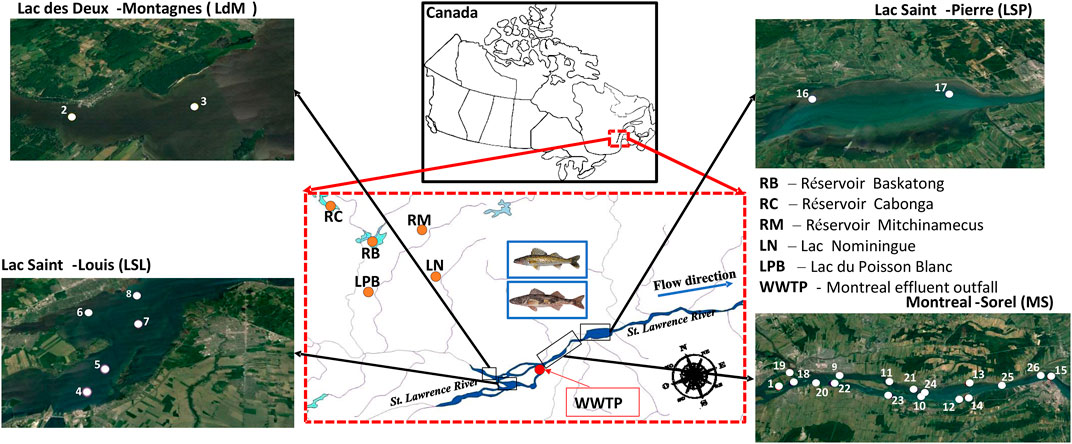
FIGURE 1. Maps showing the spatial distribution of Sauger and Walleye sampling locations from background sites (LPB, LN, RM, RC, RB), and St. Lawrence River in Quebec, Canada (sample collection stations 1–17 for Walleye at sectors LdM, LSL, MS, and LSP and 1,2,18–26 for Sauger at sectors LdM, MS). Further details on sample collections are also provided in SI Supplementary Table S2.
Collection of Field Samples
Adult fish including Sauger (SACA: Sander canadensis) and Walleye (SAVI: Sander vitreus) were collected along the St. Lawrence River and background sites by rod and reel and tissues were preserved frozen (−20°C) in plastic bags. Their geographical distribution is illustrated in Figure 1. These sites were selected to investigate the impact of Montreal WWTP effluents on PFAS and δ15N signature distribution in the two sporting fish. Sample collections were performed in the summer 2013 along the St. Lawrence River for Walleye (n = 31 specimens; from −79.22 km upstream up to +93.84 km downstream of the Montreal effluent) and for Sauger (n = 15 specimens; from −79.22 km upstream up to +48.98 km downstream of the Montreal effluent). Twenty-one (21) representative Walleye samples were also collected in the summer 2013 from background sites at Lac du Poisson Blanc, Lac Nominingue, Réservoir Mitchinamecus, Réservoir Cabonga and Réservoir Baskatong (Kaboré et al., 2022). The surveyed background areas are located between 60 and 250 km north of major urban centers along the St. Lawrence River, and are also spots of recreational fishing activities. Table 1 summarizes the sampling design including sectors, water masses where the fish were collected, number of specimens, fish size, and δ15N (muscle and liver). Additional details on fish habitats, samples ID, sampling stations, and latitude/longitude coordinates are provided in Supplementary Table S2. The different water masses were assigned different groups (Table 1) using geographical positions combined with the total water hardness.
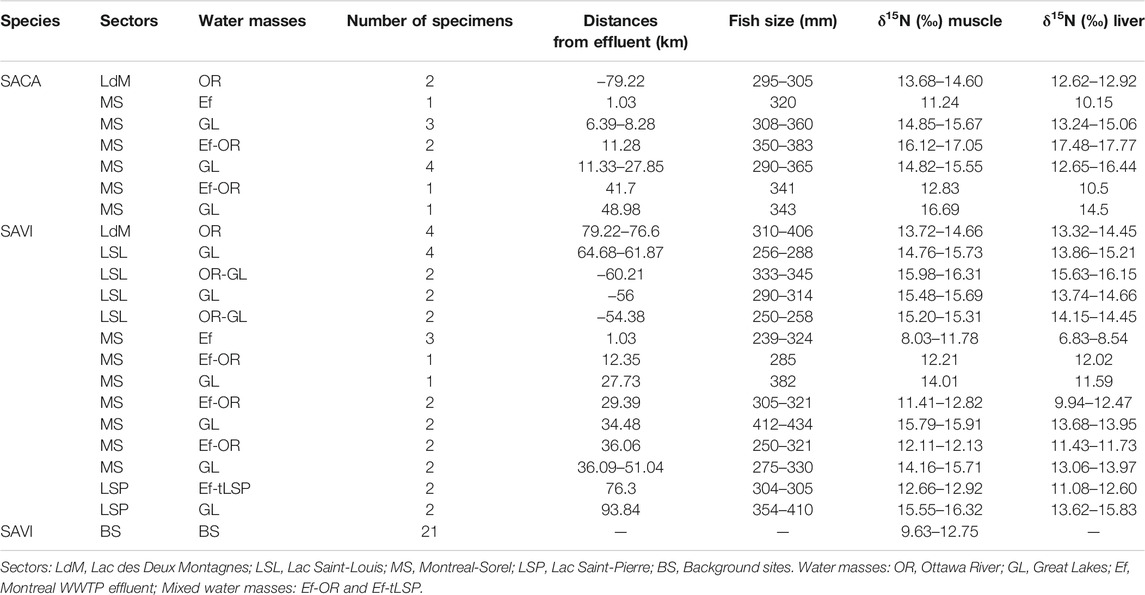
TABLE 1. Sauger (Sander canadensis: SACA) and Walleye (Sander vitreus: SAVI) summer 2013 sampling design: sectors, water masses, number of specimens analyzed for PFAS in available muscle tissues, distance from Montreal WWTP effluent, fish size (mm) and stable isotopes (δ15N) in muscle and liver.
Chemical Analyses
The sample preparation and instrumental methods were based on previous studies (Munoz et al., 2017; Kaboré et al., 2022), and details on PFAS chemical analyses are provided in SI (Supplementary Text S2). Briefly, freeze-dried and ground fish muscle samples (250 mg dry weight) were spiked with surrogate internal standards and extracted with organic solvent (0.1% NH4OH in MeOH) in an ultrasonication bath (two cycles). Following concentration, aqueous-diluted extracts were submitted to solid-phase extraction (SPE) using Strata X-AW cartridges (Phenomenex, 200 mg/6 ml). After sample loading, the Strata X-AW cartridges were dried for 1 h under vacuum and connected above ENVI-Carb cartridges (Supelco, 250 mg/6 ml). Analytes were then eluted using 2 × 4 ml of 0.2% NH4OH in MeOH. Fish muscle extracts were concentrated to a final volume of 300 μl using N2 evaporation with mild heating (40°C). Following brief vortex mixing and centrifugation (5,000 rpm, 5 min), a 200-μl aliquot was transferred to a 250-μl LC-MS polypropylene injection vial and stored at −20°C until LC-MS analysis.
Concentrated biota extracts were analyzed by ultra-high-performance liquid chromatography coupled to high-resolution mass spectrometry (UHPLC-HRMS Q-Exactive Orbitrap) through a polarity-switching electrospray ionization source. A resolution setting of 70,000 fwhm at m/z 200 was applied. The quantified PFAS were all expressed in the ng g−1 wet weight (ww) concentration unit (abbreviated as ng g−1).
For δ15N analysis, fish muscle and liver tissues were dry-ground with a mortar and pestle to a homogeneous powder and kept in tightly sealed glass vials. Approximately 1.0 mg of dried material was weighted and sealed in tin capsules and analyzed using an Isotope Ratio Mass Spectrometer (Delta V Plus; Thermo Scientific) at the Laboratory for analyses in Aquatic Ecology and Sedimentology, UQTR (Québec, Canada). The values for δ15N presented in Table 1 in parts per thousand (‰) were expressed relative to air nitrogen.
Quality Assurance and Quality Control
The identification of quantitatively targeted analytes relied on matching retention times with authentic standards, peak intensity higher than the set threshold (1e4), and mass accuracy of extracted chromatograms within a ± 5 ppm window of their theoretical exact mass (Kaboré et al., 2018). Method blanks were performed for each preparation batch of samples. Method limits of detection (LODs) were derived from the standard deviation of the blanks or using the calibration curve method (Araujo, 2009). LODs were in the range of 0.005–0.3 ng g−1 in fish muscle. Matrix-matched calibration curves were constructed, and a linear fit with inverse-weighting was applied with suitable determination coefficients (R2 > 0.99). Trueness and precision were also verified upon analysis of a NIST standard reference material (SRM 1947 Lake Michigan Fish Tissue). The determined PFOS concentration was 6.96 ± 0.18 ng g−1 (n = 5), within ± 20% of the consensus value (Reiner et al., 2012).
Suspect Screening
Four classes of suspect PFAS in Supplementary Table S3 were identified and semi-quantified among the screened suspect PFAS (Mejia-Avendaño et al., 2017; Munoz et al., 2017; Kaboré et al., 2018; Kaboré et al., 2022): cyclic perfluoroalkyl sulfonate (PFEtCHS), perfluoroalkyl sulfonates (PFHpS, PFNS), perfluoroalkyl sulfonamide (FBSA, FHxSA), and fluorotelomer carboxylic acid (9:3 FTCA). Identification and semiquantification confidence levels (Schymanski et al., 2014) are discussed elsewhere (Kaboré et al., 2018; Kaboré et al., 2022).
Statistical Analyses
Statistical analyses were conducted with the R statistical software (R-Core-Team, 2018). For statistical analysis, non-detects were replaced by ½ LOD. One-way ANOVA or Kruskal-Wallis rank sum tests were used for PFAS mean concentration comparison among sites, Sauger and Walleye or between δ15N liver or muscle tissues or among water masses. Tukey’s HSD was used as a post-hoc test if the null hypothesis (H0) of ANOVA was rejected. Pearson correlation coefficients were calculated between δ15N (muscle or liver) and individual PFAS or summed PFAS (ΣPFAS). Statistical significance was set at p < 0.05.
Results
Stable Isotopes in Fish Impacted by Montreal WWTP Effluents
Walleye and Sauger exhibited similar δ15N levels across the St. Lawrence River, with more variability noted for downstream sites (Supplementary Figure S1). For instance, δ15N muscle values were 13.09 ± 2.37‰ (Walleye) and 15.03 ± 1.54‰ (Sauger) at downstream sites, vs. 15.08 ± 0.79‰ (Walleye) and 14.14 ± 0.65‰ (Sauger) at upstream sites. A similar trend was observed for δ15N liver values. Because the fish habitats pattern added no information beyond the water masses pattern, the results for Walleye and Sauger will be described using the latter (Supplementary Table S2).
As illustrated in Figure 2, δ15N exhibited distinct ranges when grouped by water masses, particularly downstream from the Montreal WWTP effluents outfall. Lower δ15N values were typically observed in the WWTP effluent or effluent-mixed water masses, compared to other sites in the St. Lawrence. For instance, Walleye δ15N ranged between 6.83 and 13‰ in the effluent plume (MS_Ef +1.03 km) and effluent-mixed water masses (MS_Ef-OR +12.35/+36.06 km and LSP_Ef-tLSP +76.30 km); in contrast, δ15N ranged from 14.01 to 16.32‰ in water masses unrelated to the effluent plume (e.g., MS_GL +27.73/+51.04 km and LSP_GL +93.84 km). Walleye δ15N values 50–80 km upstream of the effluent outfall (i.e., Lac des Deux Montagnes, LdM, and Lac Saint-Louis, LSL) are also comparable to the δ15N values downstream the Montreal Island but unrelated to the effluent plume. The δ15N deviations in the effluent plume relative to the sites unrelated to the effluent plume are attributed to a mass transfer from St. Lawrence River water masses.
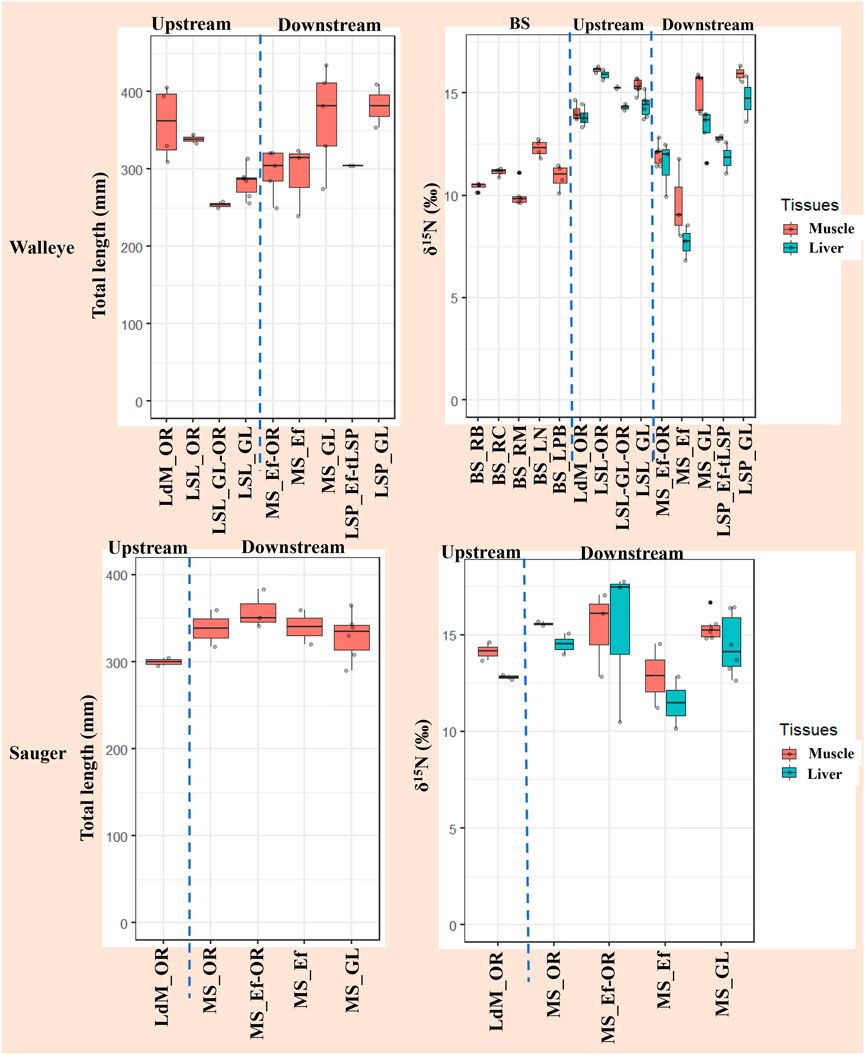
FIGURE 2. Total length (mm) and δ15N (‰) muscle and liver of adults Sauger (Sander canadensis) and Walleye (Sander vitreus) by St. Lawrence River water masses and background sites. Sauger fish’s samples were only collected upstream and downstream from Montreal WWTP and Walleye fish’s samples were collected both at upstream-downstream and background sites (BS: RB, RC, RM, LN, LPB). Legend of sectors: Lac des Deux-Montagnes (LdM), Montreal-Sorel (MS), Lac Saint-Louis (LSL), Lac Saint-Pierre (LSP). Legend of water masses: Ottawa River (OR), Montreal WWTP effluent (Ef), Great Lakes (GL), tributary of LSP (tLSP), Réservoir Baskatong (RB), Réservoir Cabonga (RC), Réservoir Mitchinamecus (RM), Lac du Poisson-Blanc (LPB), Lac Nominingue (LN). Sectors are associated to specific water masses by « _ » symbol and mixed water masses by “-” symbol. The blue vertical dash line delineates the background sites (BS) vs. upstream water masses vs. downstream water masses of St. Lawrence River.
PFAS in Walleye and Sauger From the St. Lawrence Relative to Background Sites
PFAS occurrence and concentration data will be described for the following major classes based on the detected PFAS origin and synthesis (Buck et al., 2011): fluorotelomer-based precursors, ECF-based precursors, and perfluoroalkyl acids (PFAA). Figure 3 illustrates PFAS concentrations by water masses for Sauger and Walleye from the St. Lawrence River and background sites, while Supplementary Table S3 provides the detection frequencies and concentration ranges of targeted (T) and suspect (S) PFAS in both adult Sauger and Walleye.
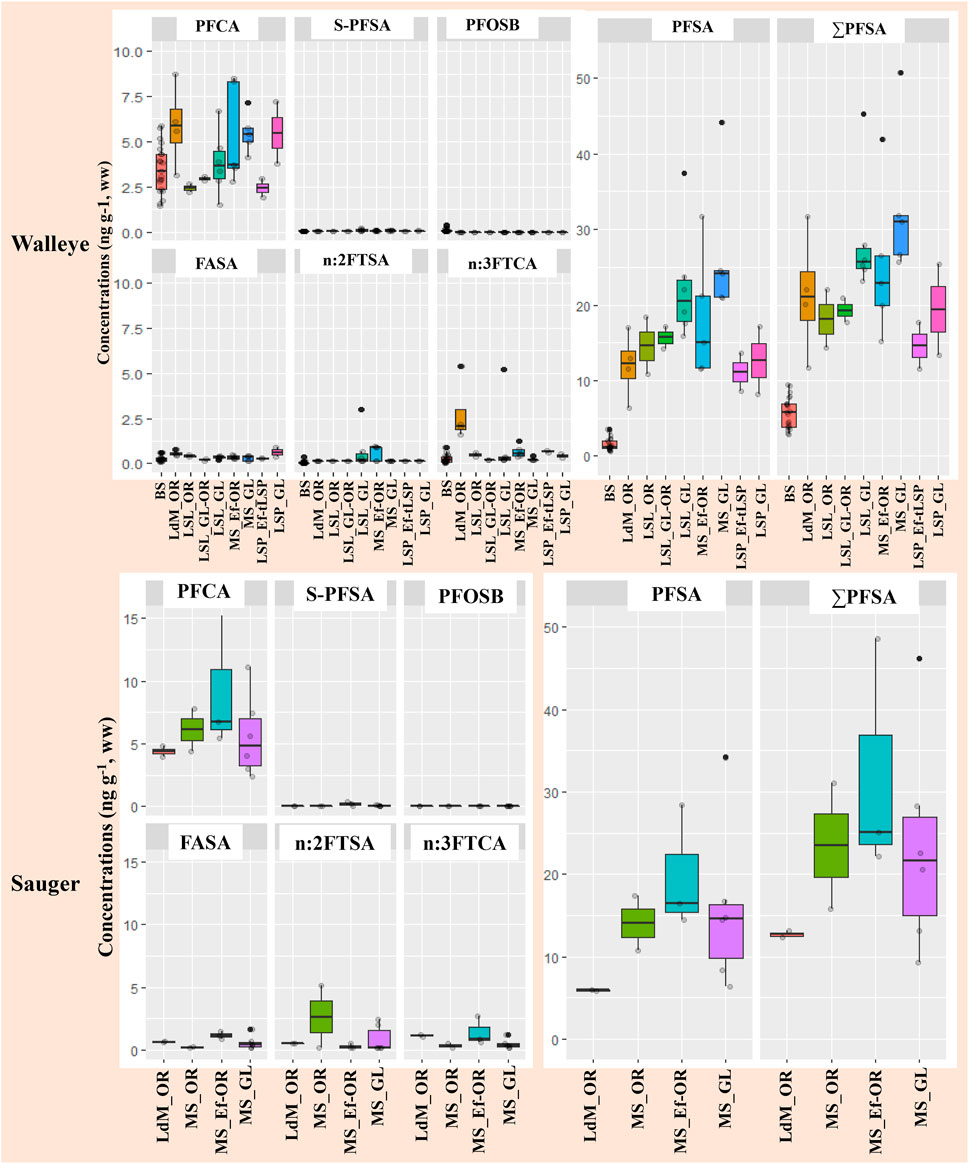
FIGURE 3. PFCA (C8-14), Suspect-PFSA (S-PFSA (PFEtCHS, PFHpS, PFNS)), PFOSB, FASA (FBSA, FHxSA, FOSA), n:2FTSA (6:2 FTSA), n:3FTCA (7:3 FTCA, 9:3 FTCA), PFSA (C6,8,10) and ∑PFAS muscle concentrations by water masses for Sauger from St. Lawrence River and for Walleye from St. Lawrence River and background sites (BS). Legend of sectors: Lac des Deux-Montagnes (LdM), Montreal-Sorel (MS), Lac Saint-Louis (LSL), Lac Saint-Pierre (LSP). Legend of water masses: Ottawa River (OR), Montreal WWTP effluent (Ef), Great Lakes (GL), a tributary of LSP (tLSP). Sectors are associated to specific water masses by « _ » symbol and mixed water masses are associated by “-“ symbol.
Fluorotelomer-Based Precursors
Fluorotelomer sulfonate (6:2 FTSA) and fluorotelomer carboxylates (7:3 FTCA, 9:3 FTCA) were the two important fluorotelomer-based classes detected. 7:3 FTCA and 6:2 FTSA (Supplementary Table S3) were detected in less than 50% of fish (muscle) samples, at typically low concentrations (generally below 1 ng g−1). Concentrations higher than 5 ng g−1 were observed at a few specficic locations, for instance, Montreal-Sorel (Ottawa River water mass MS_OR +6.39km, 6:2 FTSA: 5.2 ng g−1), Lac des Deux Montagnes (LdM_OR −79.22 km, n:3 FTCA: 5.4 ng g−1), and Lac Saint-Louis (LSL_GL −64.68 km, n:3 FTCA: 5.2 ng g−1). Suspect-target 9:3 FTCA was detected in 77% of Sauger and 68% of Walleye samples from the St. Lawrence River (<LOD-5.1 ng g−1), while the detection frequency was 48% in Walleye from background sites with 0.70 ng g−1 as maximum concentration.
ECF-Based Precursors
Two ECF-based classes, perfluoroalkane sulfonamidoalkyl betaine (PFOSB) and perfluoroalkyl sulfonamides (FBSA, FHxSA, FOSA), were detected in fish muscle from the present survey. FOSA (C8) was detected in 92% of Sauger samples from the St. Lawrence River (<LOD-1.1 ng g−1). FOSA was also systematically detected (100%) in Walleye from the St. Lawrence River (0.088–0.64 ng g−1) and background sites (0.05–0.43 ng g−1). Two short-chain sulfonamides that are also precursors to PFSAs, FBSA (C4) and FHxSA (C6), were detected at high detection frequencies in fish from the St. Lawrence River (95–100 and 71–77%, respectively). Comparatively, lower detection rates were observed for semi-remote lakes (e.g., 19% for FHxSA). Summed perfluoroalkyl sulfonamides (FASA: FBSA, FHxSA, FOSA) remained at levels lower than 1 ng g−1 at all sites except for Sauger (1.2–1.4 ng g−1) in the effluent-mixed water mass (MS_Ef-OR +11.28 km). PFOSB was detected in 38% of Walleye from background sites (Kaboré et al., 2022), but was not detected in fish from the St. Lawrence.
PFAA
Legacy PFAS such as PFCA and PFSA were detected in fish from the St. Lawrence River and semi-remote background sites. PFOA and short-chain PFCAs (C4,6,7) were occasionally detected in fish from the St. Lawrence River but not background sites. Some long-chain PFCA (C10-12) and PFSA (C8,10) were detected in all Sauger and Walleye from the St. Lawrence River. Other long-chain homologs, including PFCA (C9,13,14) and PFSA (C6), were also frequently detected (85–92%) in fish from the St. Lawrence River. Comparatively, some PFSAs (C6,10) were reported at much lower frequencies at background sites (<50%). Suspect-target PFEtCHS and PFHpS were detected at relatively high frequency (62–86%), while PFNS was detected in less than 20% of fish samples.
When water masses or sites are classified based on δ15N signatures, the ΣPFCA (sum of C8−14 homologs) was similar (p-value > 0.4) for Sauger (2.4–15 ng g−1) and Walleye (1.5–8.7 ng g−1) along the St. Lawrence River and Walleye (1.4–5.7 ng g−1) from background sites (see Figure 4). Similar to fluorotelomer-based precursors, levels of individual PFCAs were equivalent across upstream-downstream sites in the St. Lawrence River and remained generally lower than 5.0 ng g−1. PFEtCHS was mostly related to the water masses corresponding to the Great Lakes inputs, regardless of the longitudinal position (upstream/downstream the effluent). High detection rates of PFEtCHS were thus observed for fish collected in the St. Lawrence River in Lac Saint-Louis (LSL) and downstream the Montreal Island in the effluent plume and effluent-mixed water. No detections of PFEtCHS were reported in fish from Lac des Deux Montagnes (Ottawa River), nor at certain downstream locations close to the north shores of the St. Lawrence, such as in the Lake St. Pierre (sites #16–17 in Figure 1, corresponding to the brown waters originated from the Ottawa River). Based on these observations and earlier reports of PFEtCHS in Lake Ontario predator fish and surface water (De Silva et al., 2011), we hypothesize that the main sources of this cyclic PFSA may be located upstream from the surveyed area, in the Great Lakes Basin.
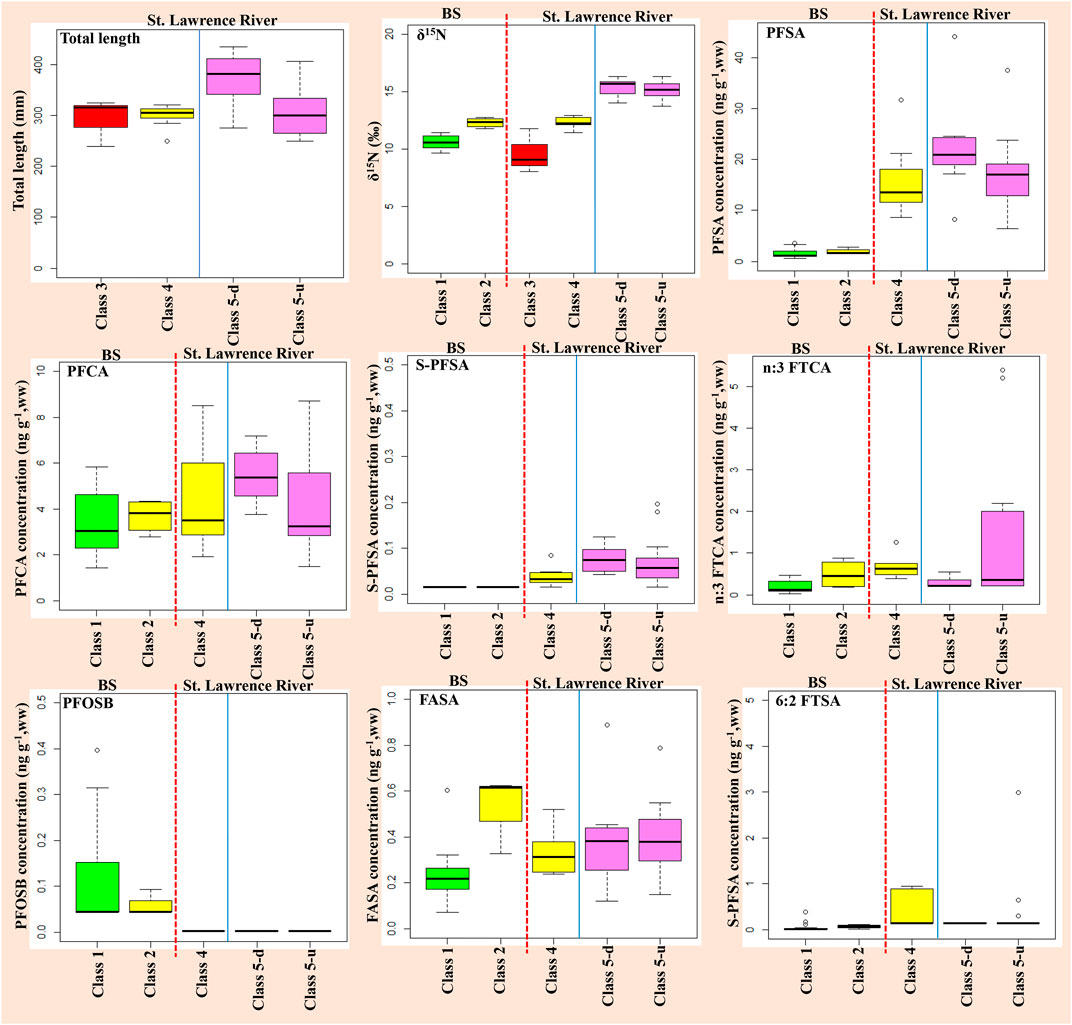
FIGURE 4. ∑PFAS, PFCA, PFSA, S-PFSA, PFOSB, 6:2 TFSA, n:3 FTCA concentrations and total length from St. Lawrence River and background sites (BS) classified based on the Walleye δ15N muscle. Class compositions: Class 1 (LPB, RC, RM, RB), Class 2 (LN), Class 3 (MS_Ef), Class 4 (MS_Ef-OR, LSP_Ef-tLSP), Class 5-d (MS_GL, LSP_GL) with “d” meaning downstream from effluent outfall and Class 5-u (LdM_RdO, LSL_RdO, LSL_GL-OR, LSL_GL) with “u” meaning upstream from effluent outfall. The red vertical dash line delineates background sites (BS) vs. the St. Lawrence River, while the vertical blue line separates from St. Lawrence River sites within vs. outside the effluent plume.
Significant differences (χ2 (8) = 40.2, p-value < 0.000003) were noted for summed PFSA (PFHxS, PFOS, PFDS) between semi-remote background sites and those of the St. Lawrence River (see Figures 3, 5), with 91.2% of the variance explaining the variability. For instance, PFSA levels ranged between 0.66 and 3.5 ng g−1 at background sites versus much higher levels for St. Lawrence River sites: 6.4–17 ng g−1 at Lac des Deux Montagnes, 11–38 ng g−1 at Lac Saint-Louis, 12–44 ng g−1 at Montreal-Sorel, and 8.2–17 ng g−1 at Lac Saint-Pierre. Interestingly, Sauger and Walleye had slightly higher levels of perfluorodecane sulfonate (PFDS) in the Lac des Deux Montagnes (1–2.62 ng g−1), compared to other locations in the St. Lawrence (0.21–1.17 ng g−1) or semi-remote background sites (<LOD-0.35 ng g−1). PFDS is a possible additive in technical products used in the metal plating, textile and upholstery industry, in polishing/cleaning agents, and impregnation/proofing agents (Boucher et al., 2018); industrial sources located in the Ottawa River watershed upstream from the sampling location may explain this pattern.
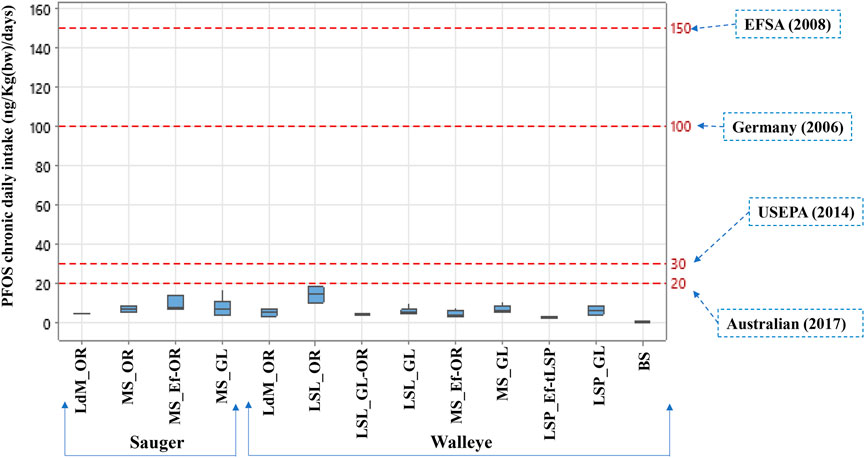
FIGURE 5. PFOS chronic daily intake (CDI) for Walleye and Sauger collected along St. Lawrence River and background sites. The red dashed line at 150 ng/kg bw/day (EFSA (European Food Safety Authority), 2008), 100 ng/kg bw/day (German-BfR, 2006), 30 ng/kg bw/day (US EPA, 2014), and 20 ng/kg bw/day (FSANZ, 2017) represented the TDI proposed by several regulatory agencies. Legend of sectors: Lac des Deux-Montagnes (LdM), Montreal-Sorel (MS), Lac Saint-Louis (LSL), Lac Saint-Pierre (LSP). Legend of water masses: Ottawa River (OR), Montreal WWTP effluent (Ef), Great Lakes (GL), tributary of LSP (tLSP). Sectors are associated to specific water masses by « _ » symbol and mixed water masses are associated by “-” symbol.
PFOS dominated the PFSA profiles (see Supplementary Figure S2) with concentrations gradually increasing (χ2(17) = 52.3, p-value < 0.00002) from background sites (0.66–3.2 ng g−1) to Lac des Deux Montagnes (4.3–14 ng g−1) and the St. Lawrence River (8.0–43 ng g−1). Along the St. Lawrence, PFOS levels remained consistent between the Lac Saint-Louis (15–23 ng g−1) and Montreal-Sorel (11.0–30.0 ng g−1) areas. Lower PFOS concentrations were observed in the Lac Saint-Pierre area (8.04–12.7 ng g−1), which may reflect increased dilution of the Great Lakes inputs and reduced exposure for fish foraging partly near the north shore within the brown water masses (sites #16–17 in Figure 1).
PFOS concentrations in St. Lawrence River fish analyzed in this study are similar to Walleye (5.2–100 ng g−1) from the Welland River watershed (Canada) (Gewurtz et al., 2014), freshwater fish from South Carolina impacted by multiple sources (Fair et al., 2019), and freshwater fish from U.S urban rivers and Greats Lakes (Stahl et al., 2014). Walleye and other fish collected in Minnesota adjacent to 3M cottage grove site (Malinsky et al., 2011) and urban lakes (Xiao et al., 2013) exhibited higher PFOS concentrations than those reported here. As illustrated in Supplementary Figure S3, the scope of concentrations observed for fish from the St. Lawrence is higher than fish from pristine Swedish lakes (Akerblom et al., 2017), high mountain lakes (Shi et al., 2010), and deciduous forest lakes (Kaboré et al., 2022).
Risk Assessment Related to Fish Consumption
A risk quotient (RQ) approach was used to assess ecological risks associated with PFOS. ECCC has developed federal environmental quality guidelines (FEQGs) for PFOS to protect fish health (8,100 ng g−1), and for the protection of piscivorous mammalian (4.6 ng g−1) and avian (8.1 ng g−1) consumers of aquatic biota (ECCC 2018; Longpré et al., 2020). In Lac des Deux Montagnes, RQs for Sauger were 1.9–2.0 and 1.0–1.2 respectively for fish-eating mammals and birds; RQs above unity were also noted for Walleye (RQs: 2.3–6.2 and 1.3–3.5). Along the St. Lawrence River, RQs above unity were observed at Lac Saint-Louis (Walleye, RQs: 2.4–15.9 and 2.4–8.9), Montreal-Sorel (Sauger, RQs: 3.5–14.5 and 1.5–8.1; Walleye, RQs: 4.8–18.9 and 2.7–10.6), and Lac Saint-Pierre (Walleye, RQs: 3.4–7.2 and 1.9–4.0). PFOS concentrations in fish from the present study remained between 93 and 6,152 times lower than the FEQG for the protection of fish themselves.
The human chronic daily intake (CDI) values are summarized in Supplementary Table S4. PFOS showed the highest chronic daily intake for Walleye and Sauger from different sectors of the present study at Lac des Deux Montagnes (2.7–7.3 ng/kg bw/day), Lac Saint-Louis (3.5–18.2 ng/kg bw/day), Montreal-Sorel sector (2.8–17.0 ng/kg bw/day), Lac Saint-Pierre (2.1–8.4 ng/kg bw/day) and background sites (0.17–0.81 ng/kg bw/day). Due to the lack of tolerable daily intake (TDI) for fish consumption from Canada, the human health effect was assessed by compiling TDI data of PFOS from different regulatory agencies to evaluate the hazard quotient. US-EPA advisory benchmark of 30 ng/kg bw/day (US EPA, 2014), the European Food Safety Authority criterion of 150 ng/kg bw/day (EFSA (European Food Safety Authority), 2008), the German criterion of 100 ng/kg bw/day (German-BfR, 2006), and Australian criterion of 20 ng/kg bw/day (FSANZ, 2017) were used. Overall, CDI values were 2–178 times lower than the US EPA benchmark, 5–523 times lower than the German TDI, 11–892 times lower than the European Food Safety Authority TDI, and 1–119 times lower than the Australian TDI (Figure 5).
Discussion
Spatial Variations of δ15N in Fish
δ15N is an important parameter generally used for modeling or comparing food chain structure and pelagic food web (Cabana and Rasmussen 1994; Vander Zanden and Rasmussen 1996; Vander Zanden et al., 1997), as well as a descriptor of contaminant bioaccumulation (Rasmussen et al., 1990; Cabana and Rasmussen 1994; Vander Zanden and Rasmussen 1996; Lapointe et al., 2020; Pelletier et al., 2022). δ15N can also be used to assess the influence of sewage on large river food webs (deBruyn et al., 2003) and nitrogen inputs from agricultural watersheds as resources of littoral food webs (Anderson and Cabana 2005).
Implications of a differential fractionation of δ15N among fish muscle and liver tissues were previously discussed (Pinnegar and Polunin 1999). δ15N in the present study were generally higher in muscle than liver either for Sauger or Walleye. This may reflect the presence of different types of amino acids in muscle (taurine) and liver (essential amino acid) tissues (Gaebler et al., 1966; Pinnegar and Polunin 1999), and differences in metabolic effects (Pinnegar and Polunin 1999). Additionally, this can be related to different short-term exposures. The greater enrichment of 15N in muscle might be the general feature of muscle tissue relative to other tissues (Pinnegar and Polunin 1999). δ15N liver of fishes can provide insight on recent (few weeks) fish feeding behaviors, while δ15N muscle would inform the longer-term trends (Paradis et al., 2008). Both δ15N muscle and δ15N liver are useful for testing the effects of effluent on the distribution and occurrence of PFAS of these two mobile fish using field-based data.
Considering the relatively similar sizes of the collected specimens and despite distinct preying habits, Walleye and Sauger had similar δ15N isotopic signatures across different water masses of the St. Lawrence. The pattern of δ15N values of two top mobile predators is attributed to water masses or site-specific effects. For instance, Walleye individuals of similar size had δ15N values lower in the effluent plume compared to other sites (non effluent-related) within the St. Lawrence. These observations are consistent with previous studies on macroinvertebrates impacted by primary WWTP effluents (deBruyn et al., 2003) and planktivorous fish influenced by primary sewage (Gaston and Suthers 2004). Our data are also in agreement with a recent study on Walleye and Northern Pike collected near the Montreal effluent (Pelletier et al., 2022). We hypothesized that the lower δ15N values of fish collected in the effluent plume relative to other sites reflected the difference in local interactions and cascading effects from bottom-up food source uptake. The variability observed in δ15N may also support the idea that fish frequented more than one water mass for their food uptake.
Similar to our observations for fish, δ15N values of resources at the bottom of the St. Lawrence food web were generally higher in sites located outside the effluent plume, e.g., seston (δ15N = +4.9 ± 0.2‰ or +4.5 ± 0.2‰)) or epiphytic biofilm (δ15N = +6.6 ± 0.9‰), compared to those located in the effluent plume including SDPOM (δ15N = −1.3 ± 1.3‰) and epiphytic biofilm (δ15N = +0.8 ± 2‰) (deBruyn et al., 2003). In general, particulate organic matter from untreated sewage has a low δ15N signature (Anderson and Cabana 2006), while the greater δ15N at the base of the food chain in sites outside the effluent could be explained by the microbiological decay of animal or plant matter (Estep and Vigg 1985). The variation in δ15N isotopic signature at the base of the food chain (seston, epiphytic biofilm, and SDPOM) can produce variations in δ15N within the same species of predator through cascading effects.
The higher δ15N outside the effluent plume may also be attributable to agricultural activities (uses of fertilizers and manures) combined with biogeochemical processes. The N transformation processes by volatilization and denitrification (after N application and transportation via runoff) can then propagate throughout the food chain (Anderson and Cabana 2005). The targeted fluvial lakes (LSL, LdM, and LSP) and the Montreal-Sorel corridor are known as agricultural zones, which could support this hypothesis (La Violette, 2004; Anderson and Cabana, 2005).
Another potential hypothesis relates to the food chain length, caused by the presence/absence of biological stressors such as invasive species (see Supplementary Figure S4) resulting in an increase of the food chain length. Aquatic invasive species have been shown to cause major changes in the native fish community structures and among them the Round Goby (Neogobius melanostomus) has possibly become one of the most successful invader of freshwater ecosystems in the Quebec section of the St. Lawrence (Lapointe et al., 2020). In the local food chain, Walleye and Sauger (both piscivorous species) have shifted their diet, generally based on Yellow Perch, Trout Perch, Cyprinids, and minor contribution of crustaceans and insects (Swenson and Smith, 1976; Vander Zanden et al., 1997), to the invasive species Round Goby. The Round Goby now represents the major prey item of Walleye (46% of diet) and Sauger (64% of diet) in the freshwater portion of the St. Lawrence River (Reyjol et al., 2010; Brodeur et al., 2011). The Round Goby feeds on mussels and other invertebrates (Supplementary Figure S4) that are microsestonic feeders (Cabana and Rasmussen, 1994). The higher δ15N values (see Figure 4) observed in fish from St. Lawrence water masses unrelated to the effluent (larger trophic chain), in contrast to background sites (shorter trophic chain), could thus be partially explained by the recent modification of the food web structure of the St. Lawrence River (Lapointe et al., 2020). In summary, the wastewater effluent of Montreal had a significant effect on isotopic signatures by lowering δ15N values, especially for Walleye specimens collected in the effluent plume or mixed effluent with other water masses.
Spatial Variations of PFAS in Fish
Since 2002, the major global producers of PFCA, fluoropolymers and fluorotelomer-based substances have engaged efforts to reduce their industrial emissions and also replaced long-chains PFCA and their precursors with shorter-chain homologues (Wang et al., 2014a). This may explain the relatively low levels of long-chain PFCA observed here. Precursor degradation may be the dominant source of PFCA in remote environments (Wang et al., 2014b), such as boreal freshwater ecosystems (Kaboré et al., 2022). For instance, fluorotelomer carboxylic acids (n:3 FTCA) are known biodegradation intermediates of fluorotelomer alcohols (FTOH) (Myers and Mabury, 2010) and other fluorotelomer-based PFAS (Weiner et al., 2013; Munoz et al., 2017). The greater n:3 FTCA concentrations in Walleye observed at Lac des Deux Montagnes water mass (LdM_OR) may be related to the local influence of scattered industries (foods, textiles) and inputs from the Ottawa River. 6:2 FTSA, for instance, is a key active ingredient in Capstone products, marketed for use in coatings, paints, and industrial cleaning products (Field and Seow, 2017). It can also be generated at elevated levels from fluorotelomer-based aqueous film-forming foam (AFFF) components via environmental transformation processes (Harding-Marjanovic et al., 2015; D'Agostino and Mabury, 2017; Mejia-Avendaño et al., 2017; Munoz et al., 2017; Shaw et al., 2019). Fluorotelomer precursors can ultimately degrade to short-chain or long-chain PFCA, depending on the length of the fluorocarbon chain involved. ECF-based precursors such as FASA (FBSA, FHxSA, FOSA) were detected both at semi-remote background sites and in the St. Lawrence River. FBSA and FOSA levels in the present study are comparable to FBSA and FOSA concentrations reported for Lake Trout from the Great Lakes region and northern Canada (Chu et al., 2016).
PFOS aggregated relative abundances (Supplementary Figure S6) ranked as follows: background sites (27.4 ± 6.1%), Lac des Deux Montagnes (47.4 ± 7.8%), and St. Lawrence River (71.4 ± 9.0%). Background sites were located between 60 and 250 km north of major urban centers along the St. Lawrence River, which can explain lower PFOS levels and abundance. Despite high dilution capability, the St. Lawrence receives domestic and industrial wastes, runoff waters, and the major inputs of the Laurentian Great Lakes and Ottawa River. Different fish habitats (Haxton and Friday, 2019) with different PFOS sources can explain the observed pattern. Reyjol et al. (2010) and Brodeur et al. (2011) also showed that the variables related to space and fish feeding habits were linked to the occurrence of Round Goby in stomach contents of Walleye and Sauger. The consumption of Round Goby, a bottom-dwelling species, was higher in benthic-feeding Sauger (64% of diet) than in Walleye (46% of diet) feeding more in the pelagic zone and subsurface. Overall, similar/different habitats of Walleye and Sauger, their feeding habits, and the modification of St. Lawrence River food web structure could have contributed to the observed PFOS patterns.
The cyclic PFAS perfluoroethylcyclohexane sulfonate (PFEtCHS) was solely detected in fish from the St. Lawrence River upstream and downstream of the effluent outfall, but not in Lac des Deux Montagnes (Ottawa River) nor in semi-remote lakes and reservoirs. PFEtCHS is used in aircraft hydraulic fluids, with continued usage in Canada based on the lack of alternatives and the critical aspect of aircraft safety performance (De Silva et al., 2011). PFEtCHS was previously reported in Northern Pike (plasma and liver) and Yellow Perch (whole-body homogenates) from the St. Lawrence River (Houde et al., 2013; Houde et al., 2014). It was also previously identified in the upper source of the St. Lawrence River, i.e., in the Great Lakes surface waters and biota (De Silva et al., 2011). Based on these observations, we hypothesize that the main source of PFEtCHS in the St. Lawrence may be related to upstream sources located in the Laurentian Great Lakes (and long-range transport), in addition to the possible local contamination sources (e.g., Montreal effluents).
Because of fishes’ high mobility, diversity of food sources (Lapointe et al., 2020) and highly diversified sources of PFAS, we would not expect the levels of individual PFAS to be much influenced by δ15N within species (except perhaps for juveniles). However, certain PFAS such PFTrDA and PFTeDA were negatively correlated to Walleye δ15N liver (Supplementary Figure S5). PFTrDA and PFTeDA concentrations were also positively related to distance from the effluent, while δ15N liver (Walleye) was negatively related to this parameter. However, interpretations should be made with caution due to the possible coincidental nature of the relationship between PFAS concentration and δ15N or trophic position, as discussed in a companion paper (Kaboré et al., 2022).
PFOS Ecological and Human Risk Assessment
The discrepancies between TDI for protecting fish themselves, humans and other piscivorous mammalian and avian are related to the methods used to derive them. Guidelines are established based on the surface water guidelines derived from species sensitivity distribution with fifth percentile, bioaccumulation factors, no observed adverse effects level (NOAELs) or lowest observed adverse effects level (LOAELs), and applying uncertainty factors for humans, piscivorous mammals, and birds. The NAOELs and LOAELs are thus divided by uncertainty factors of 10 or 100 based on the extrapolations from laboratory to field conditions to produce a set of TDI values for whole fish consumption by wildlife predators (ECCC, 2018). In the present study, we used the conversion factor of ∼2 proposed elsewhere (de Vos et al., 2008; Munoz et al., 2017; Simmonet-Laprade et al., 2019) to convert muscle concentrations into whole-body PFOS concentrations. The criteria for protecting piscivorous mammals and birds are all surpassed in Walleye and Sauger collected from the St. Lawrence River. Although human health risk quotients remained lower than unity across all target sites, RQPFOS would be higher for human consumers feeding on fish fillets from the St. Lawrence River compared to background sites. This can be cause for concern considering the long PFOS human half-life of ca. 5 years (Olsen et al., 2007).
Conclusion
The distribution of δ15N isotopic signatures and PFAS were assessed in the fish predators Walleye and Sauger from the St. Lawrence River. Isotopic signature variations were observed from the dataset, explained by site-specific effects. Notably, fish collected within the effluent plume had significantly lower δ15N compared to other sites, attributed to ingestion of sewage-derived particulate organic matter. PFAS were confirmed to be present in fish from the St. Lawrence River, and their concentrations remained similar across upstream-downstream water masses, but significantly higher than semi-remote inland lakes and reservoirs. The PFAS profile was mainly dominated by PFOS and a meta-analysis of PFOS concentration revealed that PFAS contamination is mainly explained by urbanization and site-specific impacts. Piscivorous mammals or birds are at risk considering the PFOS levels, while these levels would not pose health risks to human fish consumers, according to current guidelines.
Data Availability Statement
The original contributions presented in the study are included in the article/Supplementary Material, further inquiries can be directed to the corresponding author.
Ethics Statement
The animal study was reviewed and approved by Comité de bons soins aux animaux de l’UQTR.
Author Contributions
HK wrote the initial manuscript and data interpretation. The PFAS analysis was done by HK with help from KG, SVD, and GM. SVD supervised the mass spectrometry laboratory work. GC realized the fish sampling along with the δ15N measurements. MD, GM, and SS helped with data interpretation and statistics. SS supervised the research coordination and SS and JL have secured funding for this project. HK, SS, MD, GM, and GC have contributed to the research design. All authors have contributed to the writing of the final version of the manuscript.
Funding
The authors thank the Natural Sciences and Engineering Research Council of Canada (RGPIN-2015–05729), the Fonds de Recherche Nature et Technologies du Québec (2015-PR-183278) and the Canadian Foundation for Innovation (31090) for their financial support. We acknowledge technical support from Thermo Fisher Scientific and Phytronix Technologies.
Conflict of Interest
The authors declare that the research was conducted in the absence of any commercial or financial relationships that could be construed as a potential conflict of interest.
Publisher’s Note
All claims expressed in this article are solely those of the authors and do not necessarily represent those of their affiliated organizations, or those of the publisher, the editors and the reviewers. Any product that may be evaluated in this article, or claim that may be made by its manufacturer, is not guaranteed or endorsed by the publisher.
Supplementary Material
The Supplementary Material for this article can be found online at: https://www.frontiersin.org/articles/10.3389/fenvs.2022.833164/full#supplementary-material
References
Ahrens, L., Norström, K., Viktor, T., Cousins, A. P., and Josefsson, S. (2015). Stockholm Arlanda Airport as a Source of Per- and Polyfluoroalkyl Substances to Water, Sediment and Fish. Chemosphere 129, 33–38. doi:10.1016/j.chemosphere.2014.03.136
Åkerblom, S., Negm, N., Wu, P., Bishop, K., and Ahrens, L. (2017). Variation and Accumulation Patterns of Poly- and Perfluoroalkyl Substances (PFAS) in European Perch (Perca F) across a Gradient of Pristine Swedish Lakes. Sci. Total Environ. 599-600, 1685–1692. doi:10.1016/j.scitotenv.2017.05.032
Anderson, C., and Cabana, G. (2005). δ15N in Riverine Food Webs: Effects of N Inputs from Agricultural Watersheds. Can. J. Fish. Aquat. Sci. 62, 333–340. doi:10.1139/f04-191
Anderson, C., and Cabana, G. (2006). Does delta15N in River Food Webs Reflect the Intensity and Origin of N Loads from the Watershed? Sci. Total Environ. 367, 968–978. doi:10.1016/j.scitotenv.2006.01.029
Araujo, P. (2009). Key Aspects of Analytical Method Validation and Linearity Evaluation. J. Chromatogr. B 877, 2224–2234. doi:10.1016/j.jchromb.2008.09.030
Barzen-Hanson, K. A., Roberts, S. C., Choyke, S., Oetjen, K., McAlees, A., Riddell, N., et al. (2017). Discovery of 40 Classes of Per- and Polyfluoroalkyl Substances in Historical Aqueous Film-Forming Foams (AFFFs) and AFFF-Impacted Groundwater. Environ. Sci. Technol. 51, 2047–2057. doi:10.1021/acs.est.6b05843
Bhavsar, S. P., Fowler, C., Day, S., Petro, S., Gandhi, N., Gewurtz, S. B., et al. (2016). High Levels, Partitioning and Fish Consumption Based Water Guidelines of Perfluoroalkyl Acids Downstream of a Former Firefighting Training Facility in Canada. Environ. Int. 94, 415–423. doi:10.1016/j.envint.2016.05.023
Boucher, J. M., Cousins, I. T., Scheringer, M., Hungerbühler, K., and Wang, Z. (2018). Toward a Comprehensive Global Emission Inventory of C4-C10 Perfluoroalkanesulfonic Acids (PFSAs) and Related Precursors: Focus on the Life Cycle of C6- and C10-Based Products. Environ. Sci. Technol. Lett. 6, 1–7. doi:10.1021/acs.estlett.8b00531
Brodeur, P., Reyjol, Y., Mingelbier, M., Rivière, T., and Dumont, P. (2011). Prédation du gobie à taches noires par les poissons du Saint-Laurent : Contrôle potentiel d’une espèce exotique? Nat. Can. 135, 4–11.
Buck, R. C., Franklin, J., Berger, U., Conder, J. M., Cousins, I. T., de Voogt, P., et al. (2011). Perfluoroalkyl and Polyfluoroalkyl Substances in the Environment: Terminology, Classification, and Origins. Integr. Environ. Assess. Manag. 7, 513–541. doi:10.1002/ieam.258
Cabana, G., and Rasmussen, J. B. (1994). Modelling Food Chain Structure and Contaminant Bioaccumulation Using Stable Nitrogen Isotopes. Nature 372, 255–257. doi:10.1038/372255a0
Chu, S., Letcher, R. J., McGoldrick, D. J., and Backus, S. M. (2016). A New Fluorinated Surfactant Contaminant in Biota: Perfluorobutane Sulfonamide in Several Fish Species. Environ. Sci. Technol. 50, 669–675. doi:10.1021/acs.est.5b05058
Cordner, A., Goldenman, G., Birnbaum, L. S., Brown, P., Miller, M. F., Mueller, R., et al. (2021). The True Cost of PFAS and the Benefits of Acting Now. Environ. Sci. Technol. 55 (14), 9630–9633. doi:10.1021/acs.est.1c03565
D'Agostino, L. A., and Mabury, S. A. (2017). Aerobic Biodegradation of 2 Fluorotelomer Sulfonamide-Based Aqueous Film-Forming Foam Components Produces Perfluoroalkyl Carboxylates. Environ. Toxicol. Chem. 36, 2012–2021. doi:10.1002/etc.3750
De Silva, A. O., Spencer, C., Scott, B. F., Backus, S., and Muir, D. C. G. (2011). Detection of a Cyclic Perfluorinated Acid, Perfluoroethylcyclohexane Sulfonate, in the Great Lakes of North America. Environ. Sci. Technol. 45, 8060–8066. doi:10.1021/es200135c
de Vos, M. G., Huijbregts, M. A. J., van den Heuvel-Greve, M. J., Vethaak, A. D., Van de Vijver, K. I., Leonards, P. E. G., et al. (2008). Accumulation of Perfluorooctane Sulfonate (PFOS) in the Food Chain of the Western Scheldt Estuary: Comparing Field Measurements with Kinetic Modeling. Chemosphere 70, 1766–1773. doi:10.1016/j.chemosphere.2007.08.038
deBruyn, A. M. H., Marcogliese, D. J., and Rasmussen, J. B. (2002). Altered Body Size Distributions in a Large River Fish Community Enriched by Sewage. Can. J. Fish. Aquat. Sci. 59, 819–828. doi:10.1139/f02-056
deBruyn, A. M. H., Marcogliese, D. J., and Rasmussen, J. B. (2003). The Role of Sewage in a Large River Food Web. Can. J. Fish. Aquat. Sci. 60, 1332–1344. doi:10.1139/f03-114
deBruyn, A. M. H., and Rasmussen, J. B. (2002). Quantifying Assimilation of Sewage-Derived Organic Matter by Riverine Benthos. Ecol. Appl. 12, 511–520. doi:10.1890/1051-0761(2002)012[0511:qaosdo]2.0.co;2
Desrosiers, M., Gagnon, C., Masson, S., Martel, L., and Babut, M. P. (2008). Relationships Among Total Recoverable and Reactive Metals and Metalloid in St. Lawrence River Sediment: Bioaccumulation by Chironomids and Implications for Ecological Risk Assessment. Sci. Total Environ. 389, 101–114. doi:10.1016/j.scitotenv.2007.08.019
ECCC (2018). Canadian Environmental Protection Act, 1999 - Federal Environmental Quality Guidelines Perfluorooctane Sulfonate (PFOS). National Guidelines and Standards Office. ECCC, Gatineau, QC. Available at: https://www.canada.ca/en/environment-climate-change/services/evaluating-existing-substances/federal-environmental-quality-guidelines-perfluorooctane-sulfonate.html (Accessed July 6, 2021).
EFSA European Food Safety Authority (2008). Opinion of the Scientific Panel on Contaminants in the Food Chain on Perfluorooctane Sulfonate (PFOS), Perfluorooctanoic Acid (PFOA) and Their Salts. EFSA J. 653, 1–131.
Estep, M. L. F., and Vigg, S. (1985). Stable Carbon and Nitrogen Isotope Tracers of Trophic Dynamics in Natural Populations and Fisheries of the Lahontan Lake System, Nevada. Can. J. Fish. Aquat. Sci. 42, 1712–1719. doi:10.1139/f85-215
Fair, P. A., Wolf, B., White, N. D., Arnott, S. A., Kannan, K., Karthikraj, R., et al. (2019). Perfluoroalkyl Substances (PFASs) in Edible Fish Species from Charleston Harbor and Tributaries, South Carolina, United States: Exposure and Risk Assessment. Environ. Res. 171, 266–277. doi:10.1016/j.envres.2019.01.021
Field, J. A., and Seow, J. (2017). Properties, Occurrence, and Fate of Fluorotelomer Sulfonates. Crit. Rev. Environ. Sci. Technol. 47, 643–691. doi:10.1080/10643389.2017.1326276
FSANZ (2017). Health Based Guidance Values for PFAS. Available at: https://www1.health.gov.au/internet/main/publishing.nsf/Content/2200FE086D480353CA2580C900817CDC/$File/HBGV-Factsheet-20190911.pdf (Accessed July 08, 2021).
Gaebler, O. H., Vitti, T. G., and Vukmirovich, R. (1966). Isotope Effects in Metabolism of 14N and 15N from Unlabeled Dietary Proteins. Can. J. Biochem. 44, 1249–1257. doi:10.1139/o66-142
Gaston, T. F., and Suthers, I. M. (2004). Spatial Variation in δ13C and δ15N of Liver, Muscle and Bone in a Rocky Reef Planktivorous Fish: the Relative Contribution of Sewage. J. Exp. Mar. Biol. Ecol. 304, 17–33. doi:10.1016/j.jembe.2003.11.022
German-BfR (2006). High Levels of Perfluorinated Organic Surfactants in Fish Are Likely to Be Harmful to Human Health. Available at: https://www.bfr.bund.de/en/press_information/2006/21/high_levels_of_perfluorinated_organic_surfactants_in_fish_are_likely_to_be_harmful_to_human_health-8172.html#attachments (Accessed July 8, 2021).
Gewurtz, S. B., Bhavsar, S. P., Petro, S., Mahon, C. G., Zhao, X., Morse, D., et al. (2014). High Levels of Perfluoroalkyl Acids in Sport Fish Species Downstream of a Firefighting Training Facility at Hamilton International Airport, Ontario, Canada. Environ. Int. 67, 1–11. doi:10.1016/j.envint.2014.02.005
Harding-Marjanovic, K. C., Houtz, E. F., Yi, S., Field, J. A., Sedlak, D. L., and Alvarez-Cohen, L. (2015). Aerobic Biotransformation of Fluorotelomer Thioether Amido Sulfonate (Lodyne) in AFFF-Amended Microcosms. Environ. Sci. Technol. 49, 7666–7674. doi:10.1021/acs.est.5b01219
Haxton, T., and Friday, M. (2019). Interaction of Sauger Sander canadensis and Walleye Sander V in a Large, Shallow Northern River. J. Fish. Biol. 95, 847–854. doi:10.1111/jfb.14080
Houde, M., Douville, M., Despatie, S.-P., De Silva, A. O., and Spencer, C. (2013). Induction of Gene Responses in St. Lawrence River Northern Pike (Esox L) Environmentally Exposed to Perfluorinated Compounds. Chemosphere 92, 1195–1200. doi:10.1016/j.chemosphere.2013.01.099
Houde, M., Giraudo, M., Douville, M., Bougas, B., Couture, P., De Silva, A. O., et al. (2014). A Multi-Level Biological Approach to Evaluate Impacts of a Major Municipal Effluent in Wild St. Lawrence River Yellow Perch (Perca F). Sci. Total Environ. 497-498, 307–318. doi:10.1016/j.scitotenv.2014.07.059
Kaboré, H. A., Goeury, K., Desrosiers, M., Vo Duy, S., Liu, J., Cabana, G., et al. (2022). Novel and Legacy Per- and Polyfluoroalkyl Substances (PFAS) in Freshwater Sporting Fish from Background and Firefighting Foam Impacted Ecosystems in Eastern Canada. Sci. Total Environ. 816, 151563. doi:10.1016/j.scitotenv.2021.151563
Kaboré, H. A., Vo Duy, S., Munoz, G., Méité, L., Desrosiers, M., Liu, J., et al. (2018). Worldwide Drinking Water Occurrence and Levels of Newly-Identified Perfluoroalkyl and Polyfluoroalkyl Substances. Sci. Total Environ. 616-617, 1089–1100. doi:10.1016/j.scitotenv.2017.10.210
La Violette, N. (2004). Les lacs fluviaux du Saint-Laurent : Hydrologie et modifications humaines. Nat. Can. 128, 98–104.
Lapointe, D., Pelletier, M., Paradis, Y., Armellin, A., Verreault, J., Champoux, L., et al. (2020). Trophic Transfer of Polybrominated Diphenyl Ethers in a Recently Modified Freshwater Food Web from the St. Lawrence River, Canada. Chemosphere 255, 126877. doi:10.1016/j.chemosphere.2020.126877
Liu, M., Munoz, G., Vo Duy, S., Sauvé, S., and Liu, J. (2022). Per- and Polyfluoroalkyl Substances in Contaminated Soil and Groundwater at Airports: A Canadian Case Study. Environ. Sci. Technol. 56, 885–895. doi:10.1021/acs.est.1c04798
Longpré, D., Lorusso, L., Levicki, C., Carrier, R., and Cureton, P. (2020). PFOS, PFOA, LC-PFCAS, and Certain Other PFAS: A Focus on Canadian Guidelines and Guidance for Contaminated Sites Management. Environ. Technol. Innov. 18, 100752. doi:10.1016/j.eti.2020.100752
Malinsky, M. D., Jacoby, C. B., and Reagen, W. K. (2011). Determination of Perfluorinated Compounds in Fish Fillet Homogenates: Method Validation and Application to Fillet Homogenates from the Mississippi River. Analytica Chim. Acta 683, 248–257. doi:10.1016/j.aca.2010.10.028
Marcogliese, D. J., Blaise, C., Cyr, D., de Lafontaine, Y., Fournier, M., Gagné, F., et al. (2015). Effects of a Major Municipal Effluent on the St. Lawrence River: A Case Study. Ambio 44, 257–274. doi:10.1007/s13280-014-0577-9
Mejia-Avendaño, S., Munoz, G., Vo Duy, S., Desrosiers, M., Benoı̂t, P., Sauvé, S., et al. (2017). Novel Fluoroalkylated Surfactants in Soils Following Firefighting Foam Deployment during the Lac-Mégantic Railway Accident. Environ. Sci. Technol. 51 (15), 8313–8323. doi:10.1021/acs.est.7b02028
Munoz, G., Budzinski, H., Babut, M., Drouineau, H., Lauzent, M., Menach, K. L., et al. (2017). Evidence for the Trophic Transfer of Perfluoroalkylated Substances in a Temperate Macrotidal Estuary. Environ. Sci. Technol. 51, 8450–8459. doi:10.1021/acs.est.7b02399
Munoz, G., Desrosiers, M., Duy, S. V., Labadie, P., Budzinski, H., Liu, J., et al. (2017). Environmental Occurrence of Perfluoroalkyl Acids and Novel Fluorotelomer Surfactants in the Freshwater Fish Catostomus Commersonii and Sediments Following Firefighting Foam Deployment at the Lac-Mégantic Railway Accident. Environ. Sci. Technol. 51, 1231–1240. doi:10.1021/acs.est.6b05432
Myers, A. L., and Mabury, S. A. (2010). Fate of Fluorotelomer Acids in a Soil-Water Microcosm. Environ. Toxicol. Chem. 29, 1689–1695. doi:10.1002/etc.211
Nickerson, A., Rodowa, A. E., Adamson, D. T., Field, J. A., Kulkarni, P. R., Kornuc, J. J., et al. (2021). Spatial Trends of Anionic, Zwitterionic, and Cationic PFASs at an AFFF-Impacted Site. Environ. Sci. Technol. 55, 313–323. doi:10.1021/acs.est.0c04473
Olsen, G. W., Burris, J. M., Ehresman, D. J., Froehlich, J. W., Seacat, A. M., Butenhoff, J. L., et al. (2007). Half-Life of Serum Elimination of Perfluorooctanesulfonate, Perfluorohexanesulfonate, and Perfluorooctanoate in Retired Fluorochemical Production Workers. Environ. Health Perspect. 115, 1298–1305. doi:10.1289/ehp.10009
Paradis, Y., Bertolo, A., and Magnan, P. (2008). What Do the Empty Stomachs of Northern Pike (Esox L) Reveal? Insights from Carbon (δ13C) and Nitrogen (δ15N) Stable Isotopes. Environ. Biol. Fish. 83, 441–448. doi:10.1007/s10641-008-9366-2
Pelletier, M., Isabel, L., Armellin, A., McDaniel, T., Martin, P., McGoldrick, D., et al. (2022). Influence of Wastewater Effluents on the Bioaccumulation of Volatile Methylsiloxanes in the St. Lawrence River. Sci. Total Environ. 806, 151267. doi:10.1016/j.scitotenv.2021.151267
Pinnegar, J. K., and Polunin, N. V. C. (1999). Differential Fractionation of δ13C and δ15N Among Fish Tissues: Implications for the Study of Trophic Interactions. Funct. Ecol. 13, 225–231. doi:10.1046/j.1365-2435.1999.00301.x
R-Core-Team (2018). R: A Language and Environment for Statistical Computing. Vienna, Austria: R Foundation for Statistical Computing. Available at: https://www.R-project.org/.
Rasmussen, J. B., Rowan, D. J., Lean, D. R. S., and Carey, J. H. (1990). Food Chain Structure in Ontario Lakes Determines PCB Levels in Lake Trout (Salvelinus N) and Other Pelagic Fish. Can. J. Fish. Aquat. Sci. 47, 2030–2038. doi:10.1139/f90-227
Reiner, J. L., O’Connell, S. G., Butt, C. M., Mabury, S. A., Small, J. M., De Silva, A. O., et al. (2012). Determination of Perfluorinated Alkyl Acid Concentrations in Biological Standard Reference Materials. Anal. Bioanal. Chem. 404, 2683–2692. doi:10.1007/s00216-012-5943-5
Reyjol, Y., Brodeur, P., Mailhot, Y., Mingelbier, M., and Dumont, P. (2010). Do native Predators Feed on Non-Native Prey? the Case of Round Goby in a Fluvial Piscivorous Fish Assemblage. J. Great Lakes Res. 36, 618–624. doi:10.1016/j.jglr.2010.09.006
Schymanski, E. L., Jeon, J., Gulde, R., Fenner, K., Ruff, M., Singer, H. P., et al. (2014). Identifying Small Molecules via High Resolution Mass Spectrometry: Communicating Confidence. Environ. Sci. Technol. 48, 2097–2098. doi:10.1021/es5002105
Shaw, D. M. J., Munoz, G., Bottos, E. M., Duy, S. V., Sauvé, S., Liu, J., et al. (2019). Degradation and Defluorination of 6:2 Fluorotelomer Sulfonamidoalkyl Betaine and 6:2 Fluorotelomer Sulfonate by Gordonia Sp. Strain NB4-1Y under Sulfur-Limiting Conditions. Sci. Total Environ. 647, 690–698. doi:10.1016/j.scitotenv.2018.08.012
Shi, Y., Pan, Y., Yang, R., Wang, Y., and Cai, Y. (2010). Occurrence of Perfluorinated Compounds in Fish from Qinghai-Tibetan Plateau. Environ. Int. 36, 46–50. doi:10.1016/j.envint.2009.09.005
Simmonet-Laprade, C., Budzinski, H., Babut, M., Le Menach, K., Munoz, G., Lauzent, M., et al. (2019). Investigation of the Spatial Variability of Poly- and Perfluoroalkyl Substance Trophic Magnification in Selected Riverine Ecosystems. Sci. Total Environ. 686, 393–401. doi:10.1016/j.scitotenv.2019.05.461
Stahl, L. L., Snyder, B. D., Olsen, A. R., Kincaid, T. M., Wathen, J. B., and McCarty, H. B. (2014). Perfluorinated Compounds in Fish from U.S. Urban Rivers and the Great Lakes. Sci. Total Environ. 499, 185–195. doi:10.1016/j.scitotenv.2014.07.126
Swenson, W. A., and Smith, L. L. (1976). Influence of Food Competition, Predation, and Cannibalism on Walleye (Stizostedion Vitreum Vitreum) and Sauger (S. Canadense) Populations in Lake of the Woods, Minnesota. J. Fish. Res. Bd. Can. 33, 1946–1954. doi:10.1139/f76-248
US EPA (2014). Proceedings of the 2014 National Forum on Contaminants in Fish. Available at: https://www.epa.gov/sites/production/files/2015-02/documents/2014proceedings.pdf (Accessed January 22, 2020).
Vander Zanden, M. J., Cabana, G., and Rasmussen, J. B. (1997). Comparing Trophic Position of Freshwater Fish Calculated Using Stable Nitrogen Isotope Ratios (δ15N) and Literature Dietary Data. Can. J. Fish. Aquat. Sci. 54, 1142–1158. doi:10.1139/f97-016
Vander Zanden, M. J., and Rasmussen, J. B. (1996). A Trophic Position Model of Pelagic Food Webs: Impact on Contaminant Bioaccumulation in Lake Trout. Ecol. Monogr. 66, 451–477. doi:10.2307/2963490
Wang, Z., Cousins, I. T., Scheringer, M., Buck, R. C., and Hungerbühler, K. (2014a). Global Emission Inventories for C4-C14 Perfluoroalkyl Carboxylic Acid (PFCA) Homologues from 1951 to 2030, Part I: Production and Emissions from Quantifiable Sources. Environ. Int. 70, 62–75. doi:10.1016/j.envint.2014.04.013
Wang, Z., Cousins, I. T., Scheringer, M., Buck, R. C., and Hungerbühler, K. (2014b). Global Emission Inventories for C4-C14 Perfluoroalkyl Carboxylic Acid (PFCA) Homologues from 1951 to 2030, Part II: the Remaining Pieces of the Puzzle. Environ. Int. 69, 166–176. doi:10.1016/j.envint.2014.04.006
Weiner, B., Yeung, L. W. Y., Marchington, E. B., D'Agostino, L. A., and Mabury, S. A. (2013). Organic Fluorine Content in Aqueous Film Forming Foams (AFFFs) and Biodegradation of the Foam Component 6 : 2 Fluorotelomermercaptoalkylamido Sulfonate (6 : 2 FTSAS). Environ. Chem. 10 (6), 486–493. doi:10.1071/en13128
Xiao, F., Gulliver, J. S., and Simcik, M. F. (2013). Perfluorooctane Sulfonate (PFOS) Contamination of Fish in Urban Lakes: A Prioritization Methodology for Lake Management. Water Res. 47, 7264–7272. doi:10.1016/j.watres.2013.09.063
Keywords: δ 15 N, PFAS, wastewater, effluent, SDPOM, St. Lawrence River, walleye, sauger
Citation: Kaboré HA, Goeury K, Desrosiers M, Vo Duy S, Liu J, Cabana G, Munoz G and Sauvé S (2022) Fish Exhibit Distinct Fluorochemical and δ15N Isotopic Signatures in the St. Lawrence River Impacted by Municipal Wastewater Effluents. Front. Environ. Sci. 10:833164. doi: 10.3389/fenvs.2022.833164
Received: 10 December 2021; Accepted: 03 February 2022;
Published: 23 February 2022.
Edited by:
Weilan Zhang, University at Albany, United StatesReviewed by:
Yinghao Wen, Texas A&M University, United StatesMandana Barghi, Korea University, South Korea
Copyright © 2022 Kaboré, Goeury, Desrosiers, Vo Duy, Liu, Cabana, Munoz and Sauvé. This is an open-access article distributed under the terms of the Creative Commons Attribution License (CC BY). The use, distribution or reproduction in other forums is permitted, provided the original author(s) and the copyright owner(s) are credited and that the original publication in this journal is cited, in accordance with accepted academic practice. No use, distribution or reproduction is permitted which does not comply with these terms.
*Correspondence: Sébastien Sauvé, c2ViYXN0aWVuLnNhdXZlQHVtb250cmVhbC5jYQ==