- Plant Protection Research Institute Guangdong Academy of Agricultural Sciences, Provincial Key Laboratory of High Technology for Plant Protection, Guangzhou, China
A modified-QuEChERS method to determine kresoxim-methyl in banana and soil was developed and validated via high-performance liquid chromatography–tandem mass spectrometry (HPLC-MS/MS). The dissipation behavior, residue distribution, and risk assessment of kresoxim-methyl in banana were further investigated based on this method. The dissipation behavior of kresoxim-methyl in banana and soil was described by using first-order kinetics and its half-life, 4.8–5.7 days and 5.5–6.5 days, respectively. The concentrations of kresoxim-methyl were 0.03–0.08 mg kg−1, 0.06–0.17 mg kg−1, and <0.01 mg kg−1 for a whole banana, peel, and pulp, respectively, on the basis of spraying three times the recommended dosage and pre-harvest interval (PHI, 42 days). The results showed that the risk quotient of kresoxim-methyl in banana to people exhibited an acceptable low dietary risk. This current study could help in guiding the scientific and proper usage of this formulation.
Introduction
Banana is one of the most popular fruits worldwide. It has high nutritional content such as dietary fibers, mineral, vitamins, and phenolic compounds (Facundo et al., 2015; Pereira and Maraschin, 2015; Vu et al., 2018). It has been reported that banana contains bioactive compounds that contribute to physiological defense against oxidative and free-radical-mediated reactions in the biological system (Singh et al., 2016). Hence, banana has great antioxidant potentials. However, diseases are among the most important factors in banana production. Black Sigatoka, which is known for its widespread destruction, could cause significant reductions in leaf area, with yield losses of more than 50% (Castelan et al., 2013; Cook et al., 2013). At present, the use of chemicals remains the main method for plant disease management. Strobilurin fungicides are widely used for disease control in most fruits due to their high efficiency, low toxicity, and broad spectrum.
Kresoxim-methyl (Figure 1) was released by BASF in 1992, which belonged to the first generation of strobilurins (Kolosova et al., 2017). It inhibited mitochondrial respiration by blocking electron transfer between cytochrome b and cytochrome c1, at the ubiquinol oxidizing site (Chen et al., 2015; Li et al., 2016; Lee et al., 2017). As a protective and curative fungicide, kresoxim-methyl shows an obvious effect in controlling black Sigatoka in banana, powdery mildew in apples, and mildew in vegetables (Reuveni et al., 2017).
Previous studies commonly used gas chromatography (GC), high-performance liquid chromatography (HPLC), and GC-mass spectrometry (MS) to determine kresoxim-methyl residues (Abreu et al., 2006; Aguilera et al., 2012; Lefrancq et al., 2013; Liang et al., 2013; Aguilera et al., 2014; Celeiro et al., 2014; Khandelwal et al., 2014; Xue et al., 2015). Few studies have shown the dissipation rates and half-lives of kresoxim-methyl in different crops regarding the dissipation behavior and distribution of kresoxim-methyl in banana. Therefore, this research will be provide an important outlook towards food safety to evaluate the risk of residues to consumers. The maximum residue limit (MRL) in banana has been legislated in some organizations and countries: 0.01 mg kg−1 (temporary limit, European Union, http://ec.europa.eu/sanco_pesticides/public/index.cfm?event=substance.resultat&s=1) and 5 mg kg−1 (Japan, http://www.m5.ws001.squarestart.ne.jp/foundation/agrdtl.php?a_inq=19000). The main objectives of the present study were to (1) establish an effective and sensitive method of kresoxim-methyl based on the LC-ESI-MS/MS technique in banana and soil samples; (2) investigate the dissipation dynamics and terminal residues of kresoxim-methyl in banana and soil by field trial and distribution in a whole banana, pulp, and peel; and (3) evaluate the dietary risk probability of kresoxim-methyl in banana by the value of RQ based on the terminal residue data after spraying three and four times the recommended dosage.
Materials and Methods
Chemicals and Reagents
Standard kresoxim-methyl (98.0% purity) was purchased from Dr. Ehrenstorfer GmbH (Augsburg, Germany). A suspension emulsion (SE) containing 40% kresoxim-methyl was obtained from Shanxi Biaozheng Crop Science Co. Ltd (Shanxi, China). HPLC-grade acetonitrile was purchased from J. T. Baker (Phillipsburg, NJ, United States). MS-grade formic acid was obtained from Aladdin Industrial Corporation (Shanghai, China). Analytical grade activated anhydrous magnesium sulfate and sodium chloride were obtained from Merck (Darmstadt, Germany). Primary secondary amine (PSA; 40–60 μm in size) and octadecylsilane (C18; 40–60 μm in size) were purchased from Agela Technologies Company (Tianjin, China). Wahaha pure water was used (Wahaha Group Co., Ltd., Hangzhou, China).
Field Experiment Design and Sample Collection
Field trials to investigate the dissipation of kresoxim-methyl and residues in banana and soil were carried out in Guangzhou (GZ: 113°E, 22°N, oceanic subtropical monsoon climate) in Guangdong Province, and Fangchenggang (FCG: 108°E, 21°N, oceanic monsoon climate) in Guangxi Province in 2016 according to the “Guidelines for Pesticide Residue Field Trials” (NY/T 788-2004) issued by the Ministry of Agriculture of China. Plots where kresoxim-methyl had never been previously applied were selected. The average rainfall in GZ and FCG was 291 mm and 465 mm, respectively; the average temperature was 23°C and 33°C, respectively, and the average relative humidity was 69% and 78%, respectively. Trials were conducted from September 9 to December 14 in GZ and from May 12 to July 19 in FCG. The characteristic properties of the soil in the fields were as follows: sandy loam in GZ with an organic matter content of 23.0 g kg−1 and a pH of 6.71, and clay in FCG with an organic matter content of 19.9 g kg−1 and a pH of 5.83.
Dissipation Trials
Forty percent kresoxim-methyl suspension emulsion (SE) dissolved in water was sprayed to banana plots and soil plots once, at a dosage of 750 mg/kg (1.5 times the recommended dosage) when the banana reached 50% of the size of a ripe banana. Each banana plot contained three banana trees, and the soil plot measured 30 m2. In addition, there was a 1-m buffer zone between plots, each plot was set in triplicate, and no-pesticide application was treated with clear water. Representative banana and soil samples were randomly collected from each plot to evaluate dissipation at 2 h (calculated as the original concentration) and 1, 3, 7, 10, 14, 21, 28, 35, and 42 days after spraying.
Terminal Trials
The kresoxim-methyl formulation (40% SE) was applied to the final residual experimental plots at a low-dose level of 500 mg/kg (the recommended dosage) and a high-dose level of 750 mg/kg (1.5 times the recommended dosage) three and four times for two treatments. The re-treatment interval was 7 days, which was the recommended minimum re-treatment interval. Terminal residue samples were randomly collected from each plot at different pre-harvest intervals (PHIs; 35 and 42 days) to investigate its distribution in the whole banana, pulp, and peel. Twelve bananas were randomly collected with a knife from each treatment plot.
Sample Preparation
Representative banana samples were cut into smaller pieces according to the four-point method. Samples of 0.5 kg were homogenized in a food processor (Hobart FP-400, United States). Soil samples were thoroughly mixed, and 200 g of subsample was used for kresoxim-methyl analyses. All collected samples were stored in a freezer at −20°C until analysis. There was no need to consider the storage stability problem of analytical samples, because the kresoxim-methyl was stable at −20°C at least 18 months according to the JMPR report 1999.
Pretreatment Procedure
The sample (5 g) was weighed in a 50-ml centrifuge tube, 20 ml of acetonitrile was added, and the screw cap was closed and vigorously shaken for 1 min using a vortex mixer at maximum speed. Next, 1 g of NaCl and 4 g of anhydrous MgSO4 were added, vortexed for 1 min, and centrifuged for 2 min at 5,000 rpm. An aliquot of 2 ml was drawn from the supernatant and added in a pre-prepared 5-ml tube with 50 mg of PSA and 50 mg of C18; then, this tube was immediately shaken by hand, vortexed for 10 s, and centrifuged for 2 min at 5,000 rpm. Finally, the extract was filtered through a filter membrane (0.22 µm, Millex-GV, Millipore, Bedford, MA, United States) and then analyzed by HPLC-MS/MS. A blank sample was collected and subjected to pretreatment using the same procedure described above.
LC-MS/MS Analysis
A high-performance liquid chromatography system (Agilent 1,200, United States) tandem triple quadrupole mass spectrometer (AB SCIEX 4000Q TRAP, United States) was employed to separate and quantify kresoxim-methyl. The analyses were separated by a poroshell-120 EC-C18 column (150 mm × 3.0 mm, 2.7 μm, Agilent, United States). The oven temperature was set at 35°C; the flow rate and injection volume were 0.35 ml/min and 5 μl. The mobile phase consisted of (A) acetonitrile and (B) 0.1% (v/v) formic acid aqueous solution, and the ratio of solvent A to solvent B was 75:25. This current speed was kept constant throughout the 7-min analysis time.
Electrospray ionization was carried out using the positive ion mode (ESI+). The capillary voltage was set at 5.0 kV. The temperature of the source was 550°C. Nitrogen was used as the nebulizer and drying gas at 50 psi and 350°C. Multiple reaction monitoring (MRM) was selected. The selected precursor ion was m/z 314.0 with product quantitative and qualitative ions of m/z 116.1, m/z 222.0, and m/z 235.4, respectively, when the corresponding collision energy levels were set at 17.3 V, 18.9 V, and 24.3 V. Under the above conditions, the kresoxim-methyl retention time was approximately 3.9 min (total run time = 7 min).
Calculations
Dissipation Kinetics
The dissipation rates of kresoxim-methyl were evaluated using the first-order kinetics equations Ct = C0e−kt, where Ct and C0 denote the concentration of the pesticide residue (mg kg−1) and initial concentration (mg kg−1) at time t (days), and k is the rate constant derived from In (C0/Ct) versus t plots by regression analysis. The half-lives of dissipation (DT50) were equal to In2/k.
Risk Evaluation
National estimated daily intake (NEDI), expressed as mg kg−1, is obtained as follows:
Where STMRi (mg kg−1) is the supervised trial median residue in the collected samples, Fi (kg) is the dietary reference intake for a certain kind of food used to assess nutrient intakes of healthy Chinese people, and bw (kg) is the body weight. Based on the report by the China Health and Nutrition Survey, the average body weight for a Chinese adult is 63 kg (Chen et al., 2018).
Risk quotient is calculated for pesticide by dividing the NEDI by the acceptable daily intake (ADI) (mg kg−1 bw) for pesticide.
The ADI of kresoxim-methyl is 0.4 mg kg−1 bw based on the National Food Safety Standard of China (http://www.fao.org/fileadmin/templates/agphome/documents/Pests_Pesticides/JMPR/Evaluation01/11_Kesoxim-methyl.pdf).
The RQ is a measurement of the potential risk of adverse health effects from chemical constituents. A RQ of less than 100% indicates that the exposure does not exceed the level considered to be “acceptable”. In contrast, if it exceeds one, there is a possibility of suffering from adverse effects (Wang et al., 2015).
Results and Discussion
Method Performance
The matrix effect is usually considered to be a major drawback in trace-level analysis. Matrix-matched calibration is an efficient method to avoid matrix effects (ME) (Utture et al., 2011; Wiest et al., 2011; Rahman et al., 2015). ME were calculated using the following equation:
Where mmatrix and msolvent are the slope of the calibration curves in the matrix and in the pure solvent, respectively. An ME with a positive (negative) value indicates that the analyte response is enhanced (suppressed) by the matrix; a zero value indicates that the analyte response is removed by the matrix. The result showed that the ME of the whole banana and peel were all negative values (−3.9% and −36%), which indicated that they caused signal suppression. In contrast, the ME of the pulp caused signal enhancement. The calibration curves were constructed by plotting standard concentrations against the response of the chromatographic peak, and the correlation coefficients for all the calibration curves were greater than 0.999 (Supplementary Table S1).
Recoveries were determined to evaluate the accuracy and precision of this method. Banana and soil samples spiked at three levels of fortification (0.01, 0.1, and 1.0 mg kg−1), with five replicates for each level (Table 1), and the selectivity of the method is illustrated in Figure 2. The average recoveries ranged from 90.3% to 97.7% with RSDs of 2.29%–4.14% in the whole banana, from 90.6% to 96.4% with RSDs of 2.74%–3.03% in pulp, from 85.9% to 96.3% with RSDs of 3.03%–4.56% in peel, and from 94.2% to 99.7% with RSDs of 1.89%–5.77% in soil, respectively. LOQs were defined as the minimum fortified concentrations of analyte in the matrix with a signal-to-noise ratio of 10, which was 0.12 μg kg−1 for the whole banana, pulp, peel, and soil. LODs were 0.04 μg kg−1, which could be calculated at a signal-to-noise ratio of 3.
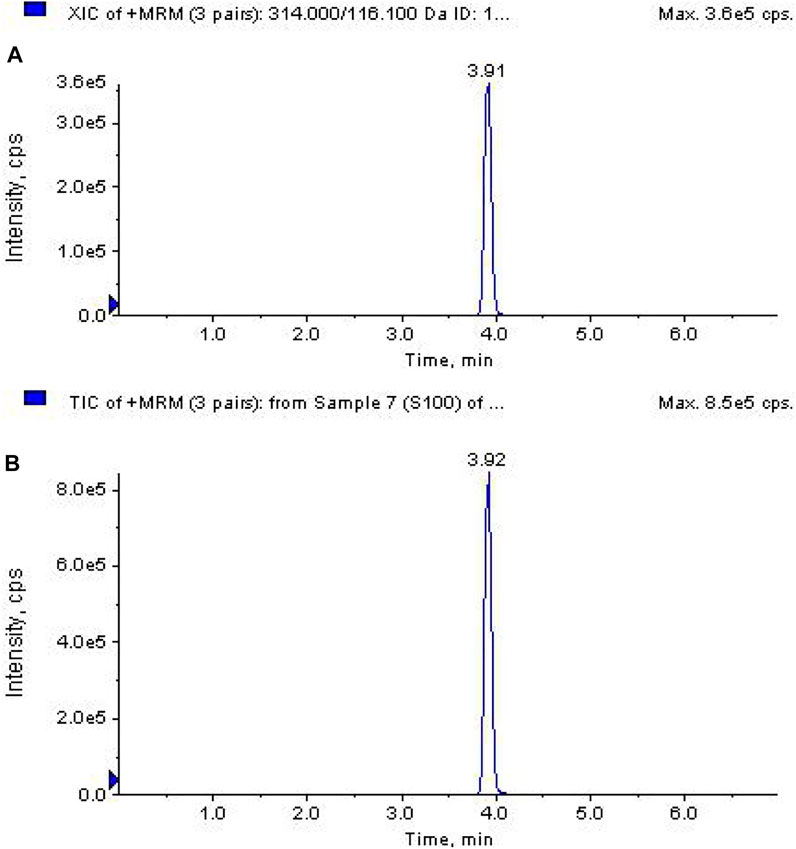
FIGURE 2. The typical chromatograms of standard kresoxim-methyl at 0.1 mg/L (A) and banana sample spiked with fungicides at 0.1 mg/L (B).
Dissipation of Kresoxim-Methyl in Banana and Soil
The initial concentrations of kresoxim-methyl residues in the whole banana were 3.33 mg kg−1 in GZ and 2.84 mg kg−1 in FCG. The half-lives were 5.7 and 4.8 days in GZ and FCG. The degradation rate was gradually decreased with the time elapsed, and the concentrations of kresoxim-methyl reached more than 55% of the initial concentration after 14 days. These values are lower than those observed in other studies, where the half-lives of kresoxim-methyl were 10.6–14.5 days in greenhouse strawberry (Chen et al., 2018) and 5–10 days in wheat (Yu and Cai, 2010). The half-lives of kresoxim-methyl in banana were higher than cucumber (1.5–2.1 days) (Liu et al., 2012) and were similar to the half-lives of 4.58–4.77 days in apple (Malhat et al., 2013). The dissipation rate of kresoxim-methyl in GZ was slower than in FCG. The experiment in GZ was conducted from September to December, and from May to July in FCG; thus, the variety of banana and growth dilution may be major factors during the dissipation. The precipitation could not be an important factor due to the low water solubility of kresoxim-methyl (2 mg/L, 20°C). The temperature and humidity in GZ (15.6°C, 64%) were lower than those in FCG (26.5°C, 78%); hence, it may cause the lower initial concentration and short half-lives in FCG. Some chemical and physical factors like experiment plot, growth speed, chemical properties of pesticide, and light could play a significant role in the degradation of fungicide. The half-life (t1/2) and other statistical parameters of dissipation were calculated from the experimental data, and the results are summarized in Table 2 and Figure 3.
The initial kresoxim-methyl residues in soil were 1.43 mg kg−1 in GZ and 1.23 mg kg−1 in FCG. The half-lives of kresoxim-methyl in soil were 5.5 and 6.5 days in GZ and FCG, respectively. The dissipation rate of kresoxim-methyl in soil was faster than in apple soil (12–13 days) (Zhang et al., 2008), strawberry soil (8–12 days) (Li et al., 2009), and wheat soil (7–13 days) (Yu and Cai, 2010). The results showed that different chemical and physical factors of soil like pH, cation exchange capacity, and microorganism might influence the adsorption and leaching of kresoxim-methyl in soil, which led to different dissipation rates in soil.
Terminal Residues of Kresoxim-Methyl in Banana
The terminal residue concentrations of kresoxim-methyl in bananas at 35 and 42 days after the last application were investigated. When kresoxim-methyl formulation was applied 3 times and 1.5 times the recommended dosage, the residues were 0.12–0.54 mg kg−1 and 0.13–0.79 mg kg−1, respectively, based on PHI (35 days). It was easily observed that the concentrations under different dosages followed the trend that residues increased with the increase in the applied dosage. When kresoxim-methyl was applied three and four times the recommended dosage based on PHI (42 days), the concentrations of kresoxim-methyl were 0.03–0.08 mg kg−1 and 0.12–0.25 mg kg−1, respectively. There were positive relations between residues and application times. When kresoxim-methyl was applied three times the recommended dosage based on different PHI (35 days and 42 days), the kresoxim-methyl residues were 0.12–0.54 mg kg−1 and 0.03–0.08 mg kg−1, respectively. It revealed that the residues were lower with a longer harvest interval.
Residue Distributions of Kresoxim-Methyl in the Whole Banana, Peel, and Pulp
The terminal residues were 0.03–0.08 mg kg−1, 0.06–0.17 mg kg−1, and <0.01 mg kg−1 in the whole banana, peel, and pulp, respectively, when kresoxim-methyl was applied three times the recommended dosage based on PHI (42 days). The residues in peel were much more than the whole banana, in which the concentration in peel was about 2 times that of the whole banana. It revealed that most of the residues were concentrated in the peel (Table 3). The peel accounts for about 35% of the whole fruit weight (Vu et al., 2017). The peel has been traditionally used for the treatment of burns, inflammation, and so on (Pereira and Maraschin, 2015). Thus, high levels of residue in the peel should be paid attention due to its broad use in the medical field and animal forage.
The residues of kresoxim-methyl in the whole banana ranged from 0.03 to 0.08 mg kg−1 (high residue, HR) when kresoxim-methyl was applied three times the recommended dosage based on PHI (42 days), which was higher than the MRL value of EU (0.01 mg kg−1) and lower than Japan (5 mg kg−1). In China, the principle of pesticide maximum residue limit standard is based on at least double the HR value with rounding up; thus, the MRL value for kresoxim-methyl in banana is 0.5 mg kg−1.
The surface of the pulp was covered with peel, and kresoxim-methyl SE was applied to the peel, so the pulp is not directly exposed to the fungicide. First, the fungicide needs to pass through a wax layer of banana before slowly entering the pulp. The kresoxim-methyl is a fat-soluble fungicide due to its Kow logP (3.4, pH 7, 25°C) (the e-pesticide manual, Version 5.0), which indicated that it is adsorbed in the peel. Other factors like wax thickness, rainfall, and temperature will also influence the residue levels in the peel. Hence, the residues that permeated into the pulp were very low. The above results showed that the kresoxim-methyl residues were all below 0.01 mg kg−1 in pulp, which was far below the MRL value in China.
Risk Assessment
The measure of the potential risk to humans due to the presence of pesticide in banana is assessed through the estimation of the risk quotient (RQ) (Tables 4 and 5). The Chinese dietary structure, registered crops in China, and the corresponding MRLs recommended by various countries and organizations were taken into account to accurately assess the NEDI. If there was no STMRi, the corresponding MRLs would be adopted for risk assessment. The selection standards of residue limits followed the principle of China first, CAC second, United States third, Australia fourth, Korea fifth, EU sixth, and Japan last, and at the same time, the selection standards of residue limits needed to satisfy the value with the greatest risk. The STMR of kresoxim-methyl in banana at the PHI of 35 and 42 days were 0.22 mg kg−1 and 0.10 mg kg−1, respectively (Supplementary Table S2). The total NEDI was 0.6232 mg (PHI 35) and 0.6178 mg (PHI 42) in various food classifications, and the RQ was 2.47% and 2.45%. The results showed that the RQ values of kresoxim-methyl in banana were all below 100% of the recommended dosage after being applied three and four times, which indicated that the residual amounts of kresoxim-methyl in banana were not hazardous to people.
Conclusion
A sensitive and efficient HPLC–MS/MS method for the analysis of kresoxim-methyl residues in banana and soil was established. The dissipation and terminal residues of kresoxim-methyl in banana were investigated to ensure the reasonable and safe use of kresoxim-methyl. The results showed that the half-lives of kresoxim-methyl in banana and soil were 4.8–5.7 days and 5.5–6.5 days, respectively. The terminal residues of kresoxim-methyl in banana were below 0.08 mg kg−1 based on spraying three times the recommended dosage, PHI (42 days). The potential dietary risk induced by kresoxim-methyl was negligible for banana consumers. The results of this work with regard to the development of analytical methods and evaluation of residue levels of kresoxim-methyl in banana fields will be useful in keeping agricultural products safe.
Data Availability Statement
The original contributions presented in the study are included in the article/Supplementary Material, further inquiries can be directed to the corresponding authors.
Author Contributions
SW: Methodology, Data curation, and Writing—Original draft preparation. XW: Validation and Data Curation. HC: Investigation and Software. HS: Funding acquisition. YL: Supervision and Data Curation.
Conflict of Interest
The authors declare that the research was conducted in the absence of any commercial or financial relationships that could be construed as a potential conflict of interest.
Publisher’s Note
All claims expressed in this article are solely those of the authors and do not necessarily represent those of their affiliated organizations, or those of the publisher, the editors, and the reviewers. Any product that may be evaluated in this article, or claim that may be made by its manufacturer, is not guaranteed or endorsed by the publisher.
Supplementary Material
The Supplementary Material for this article can be found online at: https://www.frontiersin.org/articles/10.3389/fenvs.2022.853033/full#supplementary-material
References
Abreu, S. M., Caboni, P., Cabras, P., Garau, V. L., and Alves, A. (2006). Validation and Global Uncertainty of a Liquid Chromatographic with Diode Array Detection Method for the Screening of Azoxystrobin, Kresoxim-Methyl, Trifloxystrobin, Famoxadone, Pyraclostrobin and Fenamidone in Grapes and Wine. Analytica Chim. Acta 573-574, 291–297. doi:10.1016/j.aca.2006.01.090
Aguilera, A., Valverde, A., Camacho, F., Boulaid, M., and García-Fuentes, L. (2012). Effect of Household Processing and Unit to Unit Variability of Azoxystrobin, Acrinathrin and Kresoxim Methyl Residues in Zucchini. Food Control 25, 594–600. doi:10.1016/j.foodcont.2011.11.038
Aguilera, A., Valverde, A., Camacho, F., Boulaid, M., and García-Fuentes, L. (2014). Household Processing Factors of Acrinathrin, Fipronil, Kresoxim-Methyl and Pyridaben Residues in green Beans. Food Control 35, 146–152. doi:10.1016/j.foodcont.2013.06.038
Castelan, F. P., Abadie, C., Hubert, O., Chilin-Charles, Y., de Lapeyre de Bellaire, L., and Chillet, M. (2013). Relation between the Severity of Sigatoka Disease and Banana Quality Characterized by Pomological Traits and Fruit green Life. Crop Prot. 50, 61–65. doi:10.1016/j.cropro.2013.02.019
Celeiro, M., Llompart, M., Lamas, J. P., Lores, M., Garcia-Jares, C., and Dagnac, T. (2014). Determination of Fungicides in white Grape Bagasse by Pressurized Liquid Extraction and Gas Chromatography Tandem Mass Spectrometry. J. Chroma A. 1343, 18–25. doi:10.1016/j.chroma.2014.03.057
Chen, L., Song, Y., Tang, B., Song, X., Yang, H., Li, B., et al. (2015). Aquatic Risk Assessment of a Novel Strobilurin Fungicide: A Microcosm Study Compared with the Species Sensitivity Distribution Approach. Ecotoxicol Environ. Saf. 120, 418–427. doi:10.1016/j.ecoenv.2015.06.027
Chen, X., Fan, X., Ma, Y., and Hu, J. (2018). Dissipation Behaviour, Residue Distribution and Dietary Risk Assessment of Tetraconazole and Kresoxim-Methyl in Greenhouse Strawberry via RRLC-QqQ-MS/MS Technique. Ecotoxicology Environ. Saf. 148, 799–804. doi:10.1016/j.ecoenv.2017.11.019
Cook, D. C., Liu, S., Edwards, J., Villalta, O. N., Aurambout, J.-P., Kriticos, D. J., et al. (2013). Predicted Economic Impact of Black Sigatoka on the Australian Banana Industry. Crop Prot. 51, 48–56. doi:10.1016/j.cropro.2013.03.016
Facundo, H. V. D. V., Gurak, P. D., Mercadante, A. Z., Lajolo, F. M., and Cordenunsi, B. R. (2015). Storage at Low Temperature Differentially Affects the Colour and Carotenoid Composition of Two Cultivars of Banana. Food Chem. 170, 102–109. doi:10.1016/j.foodchem.2014.08.069
Khandelwal, A., Gupta, S., Gajbhiye, V. T., and Varghese, E. (2014). Degradation of Kresoxim-Methyl in Soil: Impact of Varying Moisture, Organic Matter, Soil Sterilization, Soil Type, Light and Atmospheric CO2 Level. Chemosphere 111, 209–217. doi:10.1016/j.chemosphere.2014.03.044
Kolosova, A., Maximova, K., Eremin, S. A., Zherdev, A. V., Mercader, J. V., Abad-Fuentes, A., et al. (2017). Fluorescence Polarisation Immunoassays for Strobilurin Fungicides Kresoxim-Methyl, Trifloxystrobin and Picoxystrobin. Talanta 162, 495–504. doi:10.1016/j.talanta.2016.10.063
Lee, S., Kwon, O. S., Lee, C.-S., Won, M., Ban, H. S., and Ra, C. S. (2017). Synthesis and Biological Evaluation of Kresoxim-Methyl Analogues as Novel Inhibitors of Hypoxia-Inducible Factor (HIF) -1 Accumulation in Cancer Cells. Bioorg. Med. Chem. Lett. 27, 3026–3029. doi:10.1016/j.bmcl.2017.05.024
Lefrancq, M., Imfeld, G., Payraudeau, S., and Millet, M. (2013). Kresoxim Methyl Deposition, Drift and Runoff in a Vineyard Catchment. Sci. Total Environ. 442, 503–508. doi:10.1016/j.scitotenv.2012.09.082
Li, H., Zhang, Y. T., Guo, Y. Z., Shao, H., Liu, L., and Li, S. R. (2009). Residual Dynamics of Dry 50%kresoxim-Methyl Suspending Agent in Strawberry and Soil. Anhui Agric. Sci. 37, 9596–9597. doi:10.13989/j.cnki.0517-6611.2009.20.070
Li, L., Li, M., Chi, H., Yang, J., Li, Z., and Liu, C. (2016). Discovery of Flufenoxystrobin: Novel Fluorine-Containing Strobilurin Fungicide and Acaricide. J. Fluorine Chem. 185, 173–180. doi:10.1016/j.jfluchem.2016.03.013
Liang, P., Liu, G., Wang, F., and Wang, W. (2013). Ultrasound-assisted Surfactant-Enhanced Emulsification Microextraction with Solidification of Floating Organic Droplet Followed by High Performance Liquid Chromatography for the Determination of Strobilurin Fungicides in Fruit Juice Samples. J. Chromatogr. B 926, 62–67. doi:10.1016/j.jchromb.2013.02.011
Liu, C. Y., Wang, Y. C., Wan, K., Huang, J. X., and Wang, F. H. (2012). Residue and Risk Assessment of Kresoxim-Methyl in Cucumber under Field Conditions. Chin. J. Pes Sci. 14, 685–688. doi:10.3969/j.issn.1008-7303.2012.06.18
Malhat, F., Kamel, E., Saber, A., Hassan, E., Youssef, A., Almaz, M., et al. (2013). Residues and Dissipation of Kresoxim Methyl in Apple under Field Condition. Food Chem. 140, 371–374. doi:10.1016/j.foodchem.2013.02.050
Pereira, A., and Maraschin, M. (2015). Banana (Musa Spp) from Peel to Pulp: Ethnopharmacology, Source of Bioactive Compounds and its Relevance for Human Health. J. Ethnopharmacology 160, 149–163. doi:10.1016/j.jep.2014.11.008
Rahman, M. M., Abd El-Aty, A. M., Choi, J.-H., Kim, S.-W., Shin, S. C., and Shim, J.-H. (2015). Consequences of the Matrix Effect on Recovery of Dinotefuran and its Metabolites in green tea during Tandem Mass Spectrometry Analysis. Food Chem. 168, 445–453. doi:10.1016/j.foodchem.2014.07.095
Rahman, M. M., Jang, J., Park, J.-H., Abd El-Aty, A. M., Ko, A.-Y., Choi, J.-H., et al. (2014). Determination of Kresoxim-Methyl and its Thermolabile Metabolites in Pear Utilizing Pepper Leaf Matrix as a Protectant Using Gas Chromatography. J. Adv. Res. 5, 329–335. doi:10.1016/j.jare.2013.05.003
Reuveni, M., Gur, L., and Farber, A. (2017). Development of Improved Disease Management of Powdery Mildew on Mango Trees in Israel. Crop Prot. 110, 221–228. doi:10.1016/j.cropro.2017.07.017
Singh, B., Singh, J. P., Kaur, A., and Singh, N. (2016). Bioactive Compounds in Banana and Their Associated Health Benefits - A Review. Food Chem. 206, 1–11. doi:10.1016/j.foodchem.2016.03.033
Utture, S. C., Banerjee, K., Dasgupta, S., Patil, S. H., Jadhav, M. R., Wagh, S. S., et al. (2011). Dissipation and Distribution Behavior of Azoxystrobin, Carbendazim, and Difenoconazole in Pomegranate Fruits. J. Agric. Food Chem. 59, 7866–7873. doi:10.1021/jf200525d
Vu, H. T., Scarlett, C. J., and Vuong, Q. V. (2017). Optimization of Ultrasound-Assisted Extraction Conditions for Recovery of Phenolic Compounds and Antioxidant Capacity from Banana (Musa Cavendish) Peel. J. Food Process. Preservation 41, e13148. doi:10.1111/jfpp.13148
Vu, H. T., Scarlett, C. J., and Vuong, Q. V. (2018). Phenolic Compounds within Banana Peel and Their Potential Uses: A Review. J. Funct. Foods 40, 238–248. doi:10.1016/j.jff.2017.11.006
Wang, Z., Cang, T., Qi, P., Zhao, X., Xu, H., Wang, X., et al. (2015). Dissipation of Four Fungicides on Greenhouse Strawberries and an Assessment of Their Risks. Food Control 55, 215–220. doi:10.1016/j.foodcont.2015.02.050
Wiest, L., Buleté, A., Giroud, B., Fratta, C., Amic, S., Lambert, O., et al. (2011). Multi-Residue Analysis of 80 Environmental Contaminants in Honeys, Honeybees and Pollens by One Extraction Procedure Followed by Liquid and Gas Chromatography Coupled with Mass Spectrometric Detection. J. Chromatogr. A 1218, 5743–5756. doi:10.1016/j.chroma.2011.06.079
Xue, J., Chen, X., Jiang, W., Liu, F., and Li, H. (2015). Rapid and Sensitive Analysis of Nine Fungicide Residues in chrysanthemum by Matrix Extraction-Vortex-Assisted Dispersive Liquid-Liquid Microextraction. J. Chromatogr. B 975, 9–17. doi:10.1016/j.jchromb.2014.10.029
Yu, X. L., and Cai, L. M. (2010). Residues Dynamics of Kresoxim-Methyl 30%SC in Wheat and Soil. Agrichemicals 4, 361–362. doi:10.16820/j.cnki.1006-0413.2010.05.018
Zhang, Y. T., Guo, Y. Z., Shao, H., Liu, L., Li, H., and Song, S. R. (2008). Residue Dynamics of Kresoxim-Methyl in Apple and Soil. Agrochemicals 47, 668–610. doi:10.16820/j.cnki.1006-0413.2008.09.018
Keywords: kresoxim-methyl, banana and soil, dissipation behavior, residue distribution, risk assessment
Citation: Wang S, Sun H, Liu Y, Wang X and Chang H (2022) The Disappearance Behavior, Residue Distribution, and Risk Assessment of Kresoxim-Methyl in Banana (Musa nana Lour.) Based on a Modified QuEChERS Procedure Using HPLC-MS/MS. Front. Environ. Sci. 10:853033. doi: 10.3389/fenvs.2022.853033
Received: 12 January 2022; Accepted: 22 February 2022;
Published: 10 May 2022.
Edited by:
Liangang Mao, Institute of Plant Protection, Chinese (CAAS), ChinaReviewed by:
Bining Jiao, Southwest University, ChinaYiqiang Li, Tobacco Research Institute (CAAS), China
Copyright © 2022 Wang, Sun, Liu, Wang and Chang. This is an open-access article distributed under the terms of the Creative Commons Attribution License (CC BY). The use, distribution or reproduction in other forums is permitted, provided the original author(s) and the copyright owner(s) are credited and that the original publication in this journal is cited, in accordance with accepted academic practice. No use, distribution or reproduction is permitted which does not comply with these terms.
*Correspondence: Haibin Sun, c3VuaGJAZ2RwcHJpLmNu; Yanping Liu, bGl1bGl1eXBAdG9tLmNvbQ==