- 1Laboratorio Universitario de Geofísica Ambiental, Centro de Investigaciones en Geografía Ambiental, Universidad Nacional Autónoma de México, Michoacán, México
- 2Posgrado en Ciencias Biológicas, Universidad Nacional Autónoma de México, Michoacan, Mexico
- 3Laboratorio Universitario de Geofísica Ambiental, Instituto de Geofísica Unidad Michoacán, Universidad Nacional Autónoma de México, Michoacán, México
- 4Instituto Nacional de Investigaciones Forestales, Agrícolas y Pecuarias, Merida, Mexico
- 5Applied Physics Dept Centro de Investigación y de Estudios Avanzados Unidad, Merida, Mexico
Cities occupy a relatively small percentage of the Earth’s surface. However, they influence the entire biosphere, affect biodiversity and environmental conditions, which end up affecting human health and well-being. Therefore, it is necessary to evaluate the level of contamination by heavy metals in urban environments, as well as the possible ecological and human health risks. In this study, the urban dust of six Mexican cities was analyzed and it was found that all studied cities were contaminated, except for Mérida, when soil world background value was used as reference. In contrast, Mérida and Morelia were the most contaminated when a local background was used (decile 1). The concentrations in the cities for the metals Cu, Pb and Zn, decreased in the order CDMX > San Luis Potosí > Toluca > Morelia-Ensenada > Mérida. In the particular case of Cu and Pb, SLP accompanied CDMX as the most polluted city. For Mn and Fe concentrations, the order was CDMX > Toluca > Ensenada > SLP > Morelia-Mérida. No potential ecological risk was found due to contamination by Cu, Pb, and Zn, in the urban dust of the studied cities. However, the higher metal contribution to the potential ecological risk in all the cities was from Pb; and it represented a moderate ecological risk of more than 25% on CDMX, SLP, and Toluca sites. Pb can also be a potential risk for children’s health. In addition, chronic exposure to Fe and Mn could trigger many ailments. In the future, it is important to identify the main sources of Pb in cities and seek mitigation strategies to reduce the possible adverse effects that this metal may be causing.
1 Introduction
Cities occupy a small percentage of the global land surface (∼5%) but can influence the entire biosphere (Angeoletto et al., 2015). Between the multiple challenges of cities, the constant impact of human activities can have unintended repercussions on biodiversity, the functioning of ecosystems, and environmental quality, causing, in turn, a negative impact on human health and well-being (Lawrence 2003).
Urban dust is made up of solid particles deposited on impermeable materials that originate from the interaction of solids, liquids, and gases in the environment (Keshavarzi et al., 2018). Urban dust is a receptor for solid particles from different sources, therefore it becomes a sink for atmospheric particles. At the same time, urban dust can also be considered as a pollutant source into the atmosphere and soils, through the re-suspension of this material. It can also be a source of contaminants for water (Keshavarzi et al., 2018; Safiur Rahman et al., 2019), through rain runoff (Jayarathne et al., 2018).
Among the pollutants present in urban dust, heavy metals can be toxic or harmful to the environment and living beings, even at low concentrations. They are generally associated with relatively high densities (>5 g/cm3) since their density is assumed to be related to toxicity. Additionally, heavy metals are persistent in the environment and bioaccumulate, thus gaining public attention (Lin et al., 2017). The mechanism of toxicity of heavy metals can be explained by their ability to interact with nuclear proteins and DNA, causing deterioration of biological macromolecules (Helaluddin et al., 2016).
Ensenada, San Luis Potosí, Mexico City, Toluca, Morelia, and Mérida are Mexican cities located at different latitudes and with different geological environments. These urban areas are within the 20 Metropolitan Zones with the highest total gross production and number of inhabitants (CONAPO and SEDESOL 2012) and they could highlight the current situation in terms of contamination by heavy metals and let us explore the influence of the city size (number of inhabitants) and geological environment (physiographic province) on the pollution.
Diagnoses of contamination by heavy metals in urban dust have been carried out in some of these cities of Mexico. In Mérida and Ensenada the color of urban dust has proved to be an indicator of heavy metal pollution, dark colors are more polluted than light ones (Cortés et al., 2015; Aguilar et al., 2021). In San Luis Potosí, the highest concentrations of heavy metals in urban dust have been related to the metallurgical complex and the industrial park (Aguilera et al., 2019); and heavy metals in soils could also be an important pathway of exposure (Perez-Vazquez et al., 2015; Pérez-Vázquez et al., 2015), indeed metal concentrations have been identified in children (Flores-Ramírez et al., 2018). Mexico City has been identified as polluted by heavy metals in urban dust (Delgado et al., 2019), and heavy metal concentrations have been measured in mothers and children (Lewis et al., 2018). However, the effects of urbanization on environmental quality vary between regions with different degrees of development, topography, natural resources, and public policies (Liang, Wang, and Li 2019).
In this study, we wonder whether there is heavy metal contamination in the urban dust of six Mexican cities and if this contamination may represent a potential ecological and human health risk. It is expected that the largest and most industrialized cities will have the highest concentrations of heavy metals, however, we do not know if this happens proportionally with size and if this is the case for all metals. We also explore the differences in the pollution between located in the same and different physiographic provinces.
2 Methods
2.1 Data Collection
In previous studies, urban dust samples were collected during the dried season in Mexico City in 2011 (CDMX, n = 89), Ensenada in 2012 (ESE, n = 86), San Luis Potosí in 2017 (SLP, n = 100), Morelia in 2014 (MLM, n = 100), Mérida in 2016 (MID, n = 101) and Toluca in 2013 (TLC, n = 89). For all the cities, a standard sampling procedure was followed which consisted of sweeping 1 m2 of street surface, following a systematic, homogeneously distributed sampling design. The samples were packed in plastic bags and georeferenced (Supplementary Figure S1). Description of the study sites can be seen in the Supplementary material. They were dried in the shade and at room temperature for 2 weeks to avoid any kind of oxidation. Subsequently, they were passed through a number 10 sieve with a 2 mm opening, to remove the coarse fragments.
Chemical analysis of heavy metals in cities was done by X-ray fluorescence energy dispersive (XRF-ED). Only in the case of Morelia inductively coupled plasma optical emission spectroscopy (ICP-OES) was used. The details of the methodology can be consulted in previous studies: CDMX (Delgado et al., 2019), Ensenada (Cortés et al., 2015), SLP (Aguilera et al., 2019), Mérida (Aguilar et al., 2021). We selected the heavy metals that were measured for all the cities, those metals were copper (Cu), lead (Pb), zinc (Zn), manganese (Mn), and iron (Fe).
2.2 Identification of the Most Polluted Cities
To evaluate the level of contamination of each heavy metal, the contamination factor (CF) was used, which is a technique used to find the state of contamination of each element, as well as the pollutant load index (PLI), which is the geometric average of the five metals studied (Tomlinson et al., 1980):
A CF less than 1 indicates insignificant contamination, between 1–3 a moderate contamination, between 3–6 considerable and greater than 6 a high contamination level (Ihl et al., 2015). A PLI close to one indicates that the heavy metal load is close to the background level, while a PLI > 1 indicates contamination (Mehr et al., 2017).
Subsequently, Kruskal-Wallis analyzes were carried out to identify if there were statistically significant differences in the concentrations of heavy metals between cities. To perform the statistical analysis (descriptive statistics, Pearson correlation, and Kruskal-Wallis test) and the figures, the R Project software, version 4.0.4 (2021-02-15) “Lost Library Book” was used.
2.2.1 Ecological Risk Assessment
The ecological risk factor (
where
Toxic response factors (
To obtain the potential ecological risk of several metals (
Where
2.2.2 Human Health Risk Assessment
To estimate the risk of heavy metals, present in urban dust on the health of the population, the USEPA methodology will be used. First, the estimated daily intakes were calculated per ingestion (
CR is the contact or absorption rate. CR = IngR for ingestion, CR = InhR for inhalation, and CR = SA * AF * ABS for dermal contact. We calculated the EDIs for each of the sampling points.
The use of local parameters improves the reliability of the model; however, exposure factors have not been estimated for any Mexican city, therefore those of reference populations were used in this study (Supplementary Table S1).
Hazard ratios for ingestion, inhalation, and dermal contact (
The non-carcinogenic risk index (HI) represents the sum of the
For carcinogenic elements, the risk of developing cancer during life (
The accepted or tolerable risk is in the range of 1E-06 to 1E-04 (USEPA 2001). These values indicate that an additional case in a population of 1,000,000 and 10,000 people is acceptable (Lu et al., 2014).
3 Results and Discussion
Considering all the cities, Mn and Fe had a strong positive correlation (r = 0.9), and a strong negative correlation with Cu, Pb, and Zn (r < −0.7). Cu and Zn were also strongly correlated (r = 0.82), and they had a weaker correlation with Pb (Pb-Cu, r = 0.5; Pb-Zn, r = 0.35). This seems to indicate that Fe and Mn are elements that can share similar sources; in fact, they have been reported as elements of natural or mixed origin (Dehghani et al., 2016), in studies of heavy metals. On the other hand, Zn and Cu may also be sharing similar sources, some of which may be the same as those for Pb, while the latter metal could have other sources, in addition to those shared with Zn and Cu.
In general, the distribution of frequencies of the metals in the different studied cities was asymmetric to the right, this can be seen due to the differences between the median and the mean (Supplementary Table S3). The city with the greatest differences between the median and the mean of Cu and Pb was Morelia, in the case of Mn and Zn it was SLP, and for Fe it was Mérida. Within each city, when comparing the mean and the median among the different metals, Pb had the greatest differences. Such differences have been considered as a qualitative indicator of an anthropic enrichment of metal in the urban environment (Aguilera et al., 2019).
Previously, we did a systematic review to summarize the heavy metal concentrations in urban dust worldwide, considering 39 cities (Aguilera, Bautista, Goguitchaichvili, et al., 2021). This is a more efficient way to compare with multiple studies, instead of doing it one by one. Compared to the median values of that review, Cu (Mexico: 46.83 mg/kg, world: 83.4 mg/kg) and Zn (Mexico: 149.9 mg/kg, world: 280.7 mg/kg) had a lower median in the Mexican cities analyzed in this study. While the median Fe concentration in Mexico was higher than that reported for the world (Mexico: 30,600 mg/kg, world: 22,103 mg/kg).
By cities, the median Fe concentrations in CDMX, Ensenada, Toluca, and SLP were higher than those reported worldwide (Aguilera, Bautista, Goguitchaichvili, et al., 2021). In addition, in CDMX and Toluca the medians of Mn and Pb were also higher than those reported worldwide (Aguilera, Bautista, Goguitchaichvili, et al., 2021). In SLP, the median Pb concentration exceeded the world median While in Morelia and Mérida the median concentrations of all metals were lower than those of the world.
3.1 Most Polluted Cities
When the background value established for soils worldwide was considered (Kabata-Pendias 2011), all cities were contaminated, except for Mérida, since 75% of the data had a PLI greater than one (Figure 1). The median PLI decreased in the order CDMX > SLP > Toluca > Morelia–Ensenada > Mérida. It should be remembered that this indicates a general pattern of contamination by Cu, Pb, Zn, and Mn; Fe was not considered because there is no reported background value. This same order was maintained in the specific case of Cu, Pb, and Zn concentrations, with significant differences; particularly for Cu and Pb, SLP accompanied CDMX as the most polluted city. However, there were variations in the level of contamination for Mn and Fe by the city, the order was CDMX > Toluca > Ensenada > SLP > Morelia-Mérida (Figure 1).
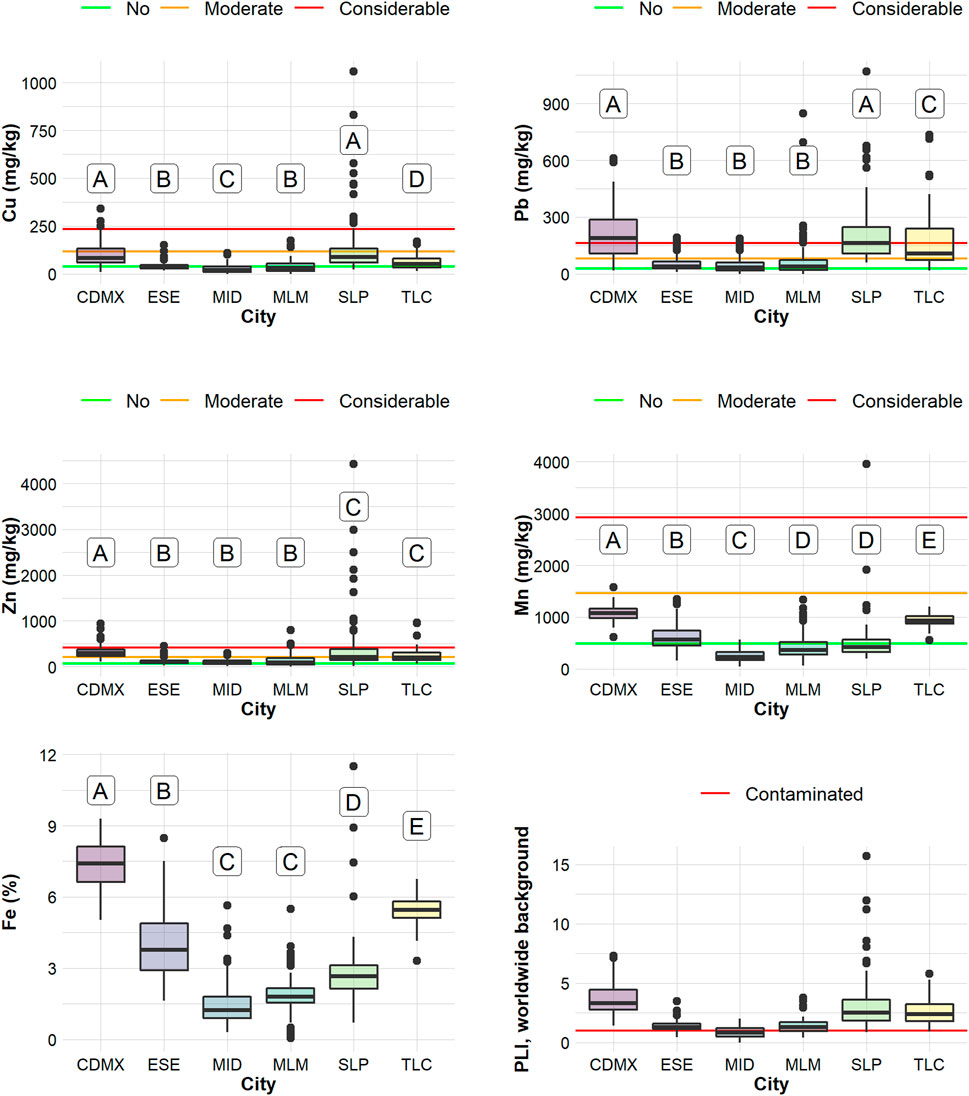
FIGURE 1. Boxplots of the heavy metal concentrations. Different letters indicate significant differences. CDMX: Mexico City, ESE: Ensenada, MID: Mérida, MLM: Morelia, SLP: San Luis Potosí, TCL: Toluca. PLI: pollution load index. Horizontal lines represent the contamination levels using the background value established for soils worldwide (Kabata-Pendias 2011).
On the other hand, when we estimated the level of contamination using decile 1 of each city as background values, the results changed. The cities with the lowest concentrations (Mérida and Morelia), and therefore the least contaminated using as a background value the one established for soils worldwide, became the most polluted (Figure 2). When comparing the results of both background values, we observe that Morelia and Mérida were the most susceptible cities to changes in their level of pollution.
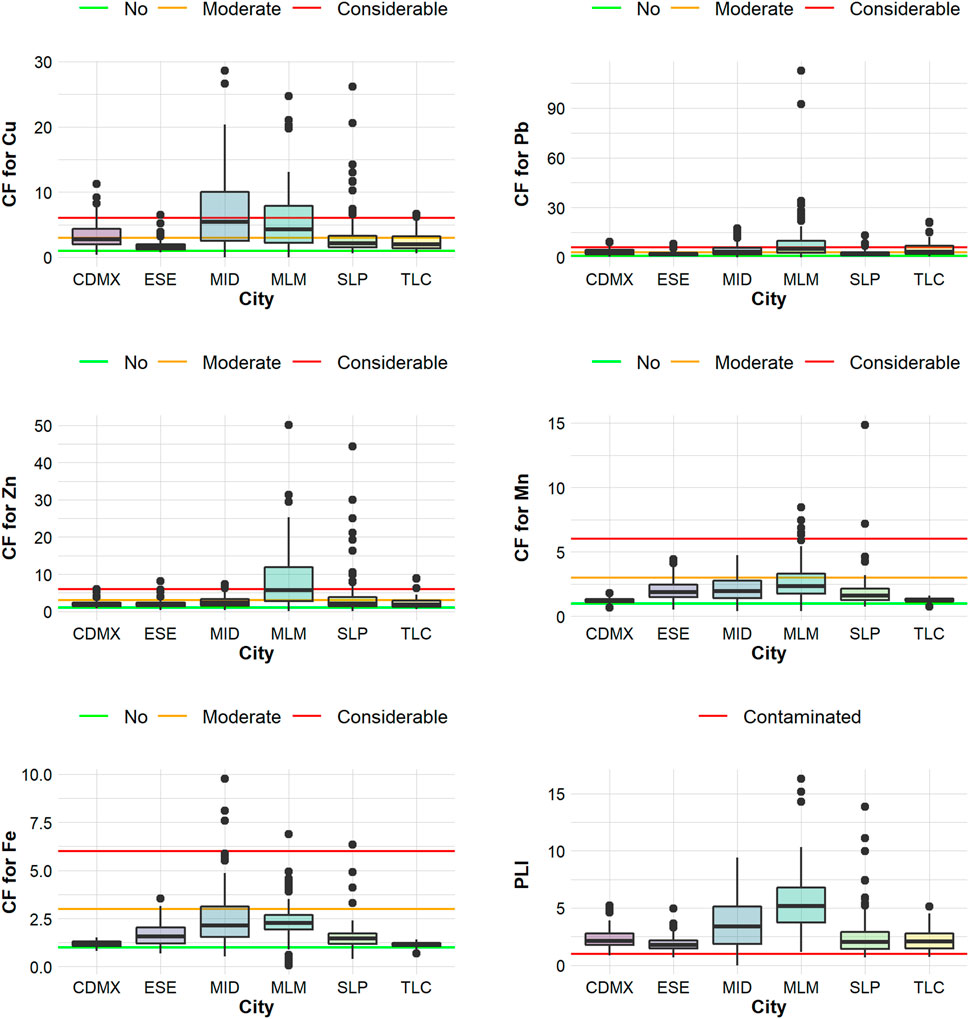
FIGURE 2. Box plots of the contamination factors (CF), using decile 1 of each city as the background value. CDMX: Ciudad de México, ESE: Ensenada, MID: Mérida, MLM: Morelia, SLP: San Luis Potosí, TCL: Toluca. PLI: pollution load index. Horizontal lines represent the contamination levels.
Morelia and Mérida turned out to be the most polluted cities because deciles 1 of heavy metals in both cities were very low (Supplementary Table S3), while deciles 1 of CDMX, Toluca, and SLP were high. The values of the first decile of Morelia were between 3 (the case of Mn) and 5.5 (the case of Cu) times lower than the background values of soils worldwide; while, in CDMX, the values of the first decile were approximately double the background values of soils worldwide, except for Cu, which was very similar to the first decile.
The differences observed by the use of both background values highlight the problem that has been discussed for decades, when a general background value is used all local variations are ignored, while when particular background values are used for each site, all local differences are emphasized (Hakanson 1980). In this study it was clear that the concentrations of CDMX, SLP, and Toluca were higher than those of Morelia or Mérida, however, the local background values of these last two cities were so small that they turned out to be the most contaminated when compared.
Another point to consider was the fact that the analytical technique (ICP-OES) with which Morelia concentrations were obtained was more sensitive than that used in all the other cities (XRF-ED). For this reason, lower values could have been detected in Morelia.
It is noteworthy that the contamination of Pb and Cu in the largest city in Mexico (CDMX) was comparable to that of a metallurgical city (SLP). Metallurgical and mining activities are among the main emitters of heavy metals into the environment (Tapia et al., 2018; Li et al., 2015), however, a large number of minor sources (vehicles, industries, garbage incineration, etc.) in urbanization (CDMX) can lead to a similar level of contamination.
While it is a common idea that the largest or most populated cities should be the most polluted, this is not necessarily true. The population decreased in the order CDMX > Toluca > SLP > Mérida > Morelia > Ensenada (INEGI Instituto Nacional de Estadística y Geografía 2014). Mérida was the fourth most populated city; however, it was the least polluted, considering the global background value. SLP was the third most populated city, but its level of contamination by Pb and Cu was comparable to that of CDMX (the most populated). Ensenada was the least populated city, but its concentrations of Mn and Fe were higher than those of SLP, Morelia, and Mérida. At the global level, Aguilera et al. (2021) did not find a correlation between the number of inhabitants and the concentrations of heavy metals. At the local level, in CDMX, no relationship was found (Aguilera, Bautista-Hernández, et al., 2021). However, other studies have found this relationship (Acosta et al., 2015; Trujillo-González et al., 2016).
Among the cities of the Neovolcanic Belt (Morelia, Toluca, CDMX; Supplementary Material), there were significant differences in the level of contamination, CDMX was the most contaminated city by Cu, Pb, Zn, Mn, and Fe with significant differences from Toluca, while Morelia was the least contaminated; therefore, pollution must be caused by human activities, rather than natural causes.
Fe and Mn may be sharing the same sources, they are considered as elements of natural or mixed origin (Dehghani et al., 2016). Zn and Cu may also be sharing similar sources, some of which may be the same as for Pb, while the latter metal could have other sources.
In CDMX it has been recognized that the main sources of Cu, Pb, and Zn in urban dust could be related to vehicular traffic (Aguilera, Bautista-Hernández, et al., 2021; Aguilera, Bautista, Gutiérrez-Ruiz, et al., 2021). In SLP, the main source of Cu and Zn is the metallurgical complex and to a lesser extent the industrial park, Pb could have the same sources, in addition to vehicular traffic (Aguilera et al., 2019). In Toluca, the main sources of Pb can be the combustion processes of food waste, paper, plastics, textiles, rubber, wood, and metal smelting; other sources, other than combustion, from these industries; as well as the old Pb deposit from leaded gasoline (Ávila-Pérez et al., 2019).
Little information is available on possible sources of heavy metals in the environment in Ensenada and Morelia. In Ensenada, using a bioindicator (mussel Mytilus californianus), it has been observed that Pb concentrations are affected by anthropic activities, in this study it was thought that Pb reached the mussel through the atmospheric deposition (Muñoz-Barbosa, Gutiérrez-Galindo, and Flores-Muñoz 2000). In Morelia, it was found that the highest concentrations of Zn in soils were located in primary roads with significant differences for the other roads. In addition, the concentrations of Mn, Pb, and Fe exceeded the maximum limits of the Mexican regulations for soils (Carranza et al., 2015), called NOM-147 (SEMARNAT 2007).
In Mérida, Cu, Zn, and Pb have been associated with vehicular traffic, because the highest concentrations have been found in the historic center and on primary roads. When observing the dust particles under a microscope, spherical particles of anthropic origin with these associated metals were found (Aguilar et al., 2021).
3.1.1 Potential Ecological Risk
The PER was below 150 for practically all cities; therefore, there is no potential ecological risk due to the concentrations of Cu, Pb, Mn, and Zn, together, in urban dust. Only two sites in SLP had a moderate potential ecological risk (150 < PER ≤ 300) and one more site had a considerable risk (300 < PER ≤ 600). The values of the medians of the PER decreased in the order CDMX > SLP > Toluca > Ensenada > Morelia > Mérida (Figure 3). Pb was the metal that most contributed to the PER in all cities, representing 67.3% of the PER in CDMX, 49.3% in Ensenada, 58.1% in Mérida, 55.1% in Morelia, 66.8.1% in SLP, and 64.1% in Toluca.
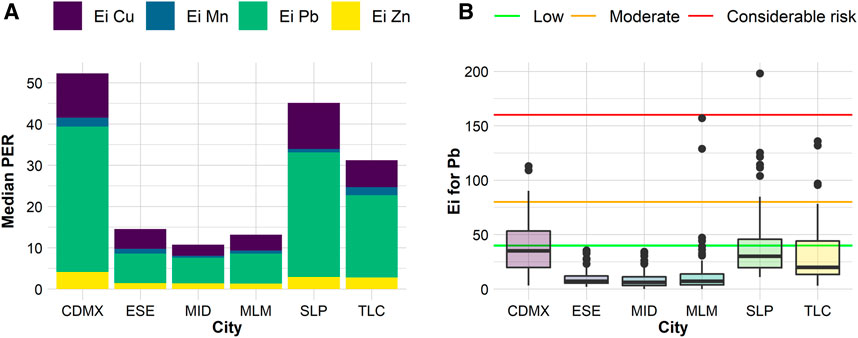
FIGURE 3. In the (A), the value of the median potential ecological risk (PER), divided according to the contribution of each of the metals risk factors (Ei). In the (B), box plots of lead risk factors (Ei) for each studied city. CDMX: Mexico City, ESE: Ensenada, MID: Mérida, MLM: Morelia, SLP: San Luis Potosí, TCL: Toluca.
Even when no potential ecological risk was found due to Cu, Pb, Mn, and Zn, in the urban dust of the studied cities, it is important to remember that this index is the addition of the individual risks of each metal, as in this study only three metals could be analyzed, the risk may be lower than in other cities where more metals were considered (Yesilkanat et al., 2021). Therefore, if more metals were analyzed in Mexican cities, the ecological risk would change.
Pb alone did represent a potential ecological risk, according to the ecological risk factor (Ei). In more than 25% of sites of CDMX, SLP and Toluca a moderate risk was found, in some sites of these cities, a considerable risk was found, and only in one SLP site was a high risk (Figure 3). This result differs from that found in other studies where several cities were analyzed, in those cases different metals contributed the most to the ecological risk (Jahandari 2020; Yesilkanat et al., 2021). In fact, in the present work, Pb alone represents a moderate ecological risk in more than a quarter of the sampling sites of CDMX, SLP, and Toluca.
3.1.2 Human Health Risk
The main route of exposure to heavy metals was ingestion. The risk to human health was 10 times higher for children than for adults. Pb was the only metal that represented a non-carcinogenic risk for the health of children in the cities of CDMX, SLP, and Toluca since its HI was greater than one in ∼25% of the sampling sites (Figure 4). Children are the most susceptible due to their hand-to-mouth habits and rapid growth rates (Kamali, Omidvar, and Kazemzadeh 2013).
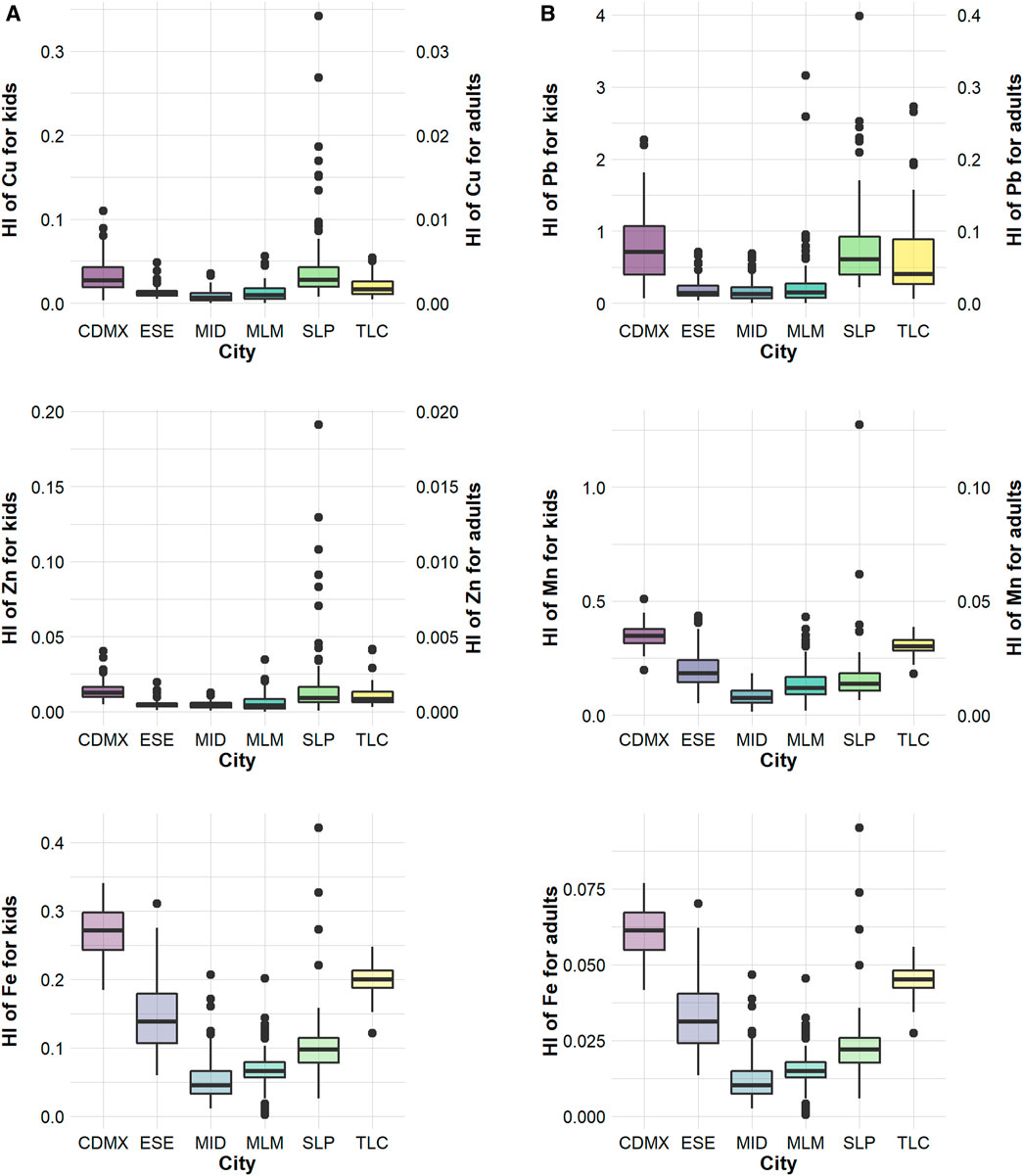
FIGURE 4. Box plots of the human health risk indices (HI) for children (A) and adults (B), of each heavy metal for Mexican cities. CDMX: Mexico City, ESE: Ensenada, MID: Mérida, MLM: Morelia, SLP: San Luis Potosí, TCL: Toluca.
Some points to consider are 1) the fact that the total concentrations of Pb were used to calculate the health risk indices, therefore the risk is being overestimated, it is necessary to use the bioavailable concentrations (Huang. 2016); 2) on the other hand, only the risk represented by urban dust is being considered, while that from other sources such as water, food, air, soil, and glazed ceramic also has its contribution to the total risk of this metal for the human health (Li et al., 2017); and 3) the effect that may have the combination of Pb with other metals and pollutants is still unknown.
Chronic exposure to an HI greater than 0.1 (1E-01) has been reported to trigger many ailments (Jadoon et al., 2018). In this sense, Fe represented a risk of generating ailments in children in all cities, since they all had HI greater than 0.1 (1E-01), in some sampling points; in CDMX and Toluca this happened for the entire city; in Ensenada, it happened in 87% of the cases and in SLP 46% of the cases had an HI greater than 0.1 (1E-01). In Morelia and Mérida, only the extreme values were in this situation. The Mn could also unleash health problems for children in all cities; especially in CDMX and Toluca, since their HI was greater than 0.1 in the entire city; in Ensenada, the HI was higher than 0.1 in 88% of the city, in SLP in 79%, in Morelia in 67% and Mérida in 29% of the city. In the case of Zn HI greater than 0.1 was only found in some sites of SLP; the same for Cu, together with one sampling point in CDMX. Cu and Zn were the metals that represented the least risk to human health.
Iron and manganese are generally not analyzed in heavy metal contamination studies, probably because they are not considered dangerous or relevant in the urban environment, however, that is not true (Kim, Lee, Seok et al., 2015; Kletetschka, Bazala, Takáč et al., 2021). We compared the mean HI for kids and adults in different cities and observed that HI for Fe commonly is higher than the one for other metals such as Cu and Zn (Table 1). In Mexico, the mean Fe HI for kids was higher than those reported in all the other cities. Black magnetite particles can be seen in the air filters of the air monitoring systems of Mexican cities. Mn has been analyzed only at sites where significant sources of this metal are known in advance (Menezes-Filho 2016; Rodrigues et al., 2018). The results of the present work indicated that it is important to include them and monitor their concentrations in cities. 04) (USEPA 2001).
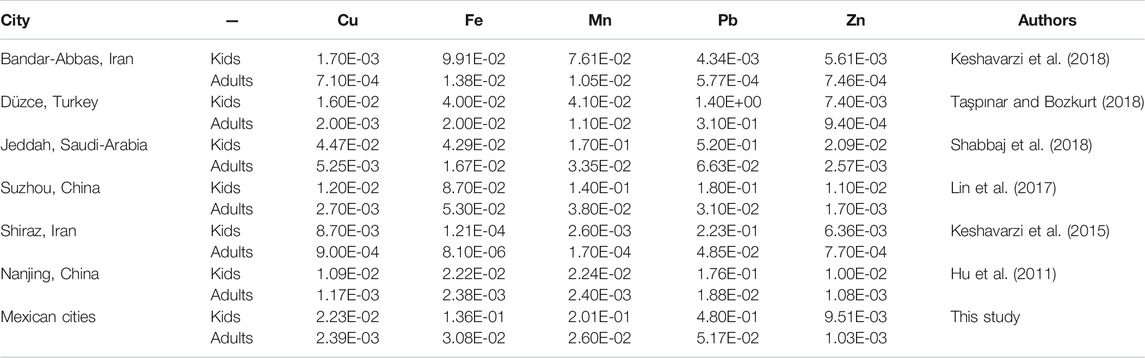
TABLE 1. Comparison of mean Hazard Index (HI) for the studied heavy metals in other cities around the world.
In comparison with other cities (Table 1), the mean HI for Cu for kids was ten times higher than for Bandar-Abbas and Shiraz and the same for the other cities. For Zn were ten to twenty times lesser tan for the other cities. Mean Pb HI for kids in the six Mexican cities was higher than for Bandar-Abbas, lesser than for Düzce, and almost the same magnitude as for the rest of the cities. Mean Fe HI for kids in the six Mexican cities was higher than all the cities in comparison. Mn was higher than four of the six cities in comparison. The HIs for adults were similar but ten times lesser than for kids.
Pb was the only analyzed metal with the potential to generate cancer. However, the carcinogenic risk index (RI) indicated that this metal in urban dust does not represent a risk of developing cancer in any of the cities, the median value was 1.1E-9, which is below the accepted or tolerable risk (1E-06 a 1E-08 After analyzing the pollution and ecological and human health risk indices, we were able to observe that lead was the metal that causes the greatest concern in Mexican cities. Globally, Pb is also one of the metals of greatest concern, along with Cr (Aguilera, Bautista, Goguitchaichvili, et al., 2021). In the case of Mexico, practically in all cities, it is attributed the source of this metal in urban dust to vehicular traffic, however, it is also investigated how much the deposit of old Pb from leaded gasoline contributes (Ávila-Pérez et al., 2019).
On the other hand, it is necessary to establish a monitoring system for heavy metals in the dust of the streets, soils, and plants of Mexican cities, as well as pollution indicators with proxy characteristics (easy analysis and low cost) that allow the analysis of thousands of dust samples to identify the sites of high concentration of heavy metals. In this sense, magnetic (Sánchez-Duque et al., 2015; Aguilera et al., 2020) and colorimetric (Cortés et al., 2015; Sanleandro et al., 2018; Aguilar et al., 2021) techniques are promising.
4 Conclusion
In this study, we highlighted the current situation in terms of heavy metal contamination in Mexican cities. When the proposed value for soils worldwide was considered as the background, all cities were contaminated, except for Mérida. However, Mérida and Morelia were the most polluted cities when a local background value was used (decile 1). For the metals Cu, Pb and Zn, the concentrations in the cities decreased in the order CDMX > San Luis Potosí > Toluca > Morelia-Ensenada > Mérida. In the particular case of Cu and Pb, SLP accompanied CDMX as the city with the highest concentrations. For the Mn and Fe, the order slightly changed: CDMX > Toluca > Ensenada > San Luis Potosí > Morelia-Mérida.
The largest and most industrialized cities were expected to have the highest concentrations of heavy metals; however, we did not know if this was proportional to the size and if this was the case for all metals. The results showed that contamination was not necessarily related to the number of inhabitants, a populated city like Mérida was the least contaminated; on the contrary, a sparsely populated city like Ensenada was more polluted by Mn and Fe than other more populated ones like San Luis Potosí, Morelia, and Mérida.
No potential ecological risk was found due to contamination by Cu, Pb, and Zn, in the urban dust of the studied cities. However, Pb was the metal that contributed the most to potential ecological risk in all cities. Furthermore, Pb alone did represent a moderate ecological risk in more than 25% of the CDMX, San Luis Potosí, and Toluca sites. Pb could also represent a health risk for the children in these cities. Additionally, chronic exposure to Fe and Mn could trigger many ailments or illnesses.
The analysis of the level of contamination and the possible ecological and human health risk of heavy metals in the dust of six Mexican cities, allowed us to identify that Pb is the metal that causes the greatest concern in the urban environment of Mexico. Therefore, it is important to pay attention to this metal and inquire more deeply about its sources and mitigation strategies.
Data Availability Statement
The raw data supporting the conclusion of this article will be made available by the authors, without undue reservation.
Author Contributions
Conceptualization: FB; methodology: CD, YA, DA, JC, AA, and RC; validation: FB, PQ, and AG; formal analysis: AA; resources: FB, PQ, and AG; writing-original draft: AA; writing-review and editing: FB, PQ, and AG; visualization: AA; supervision: FB; Project administration: FB; funding acquisition: FB and AG.
Funding
This research was funded by DGAPA Universidad Nacional Autónoma de México grant number IN208621 and SEP-CONACYT project 283135. The funding sources had no involvement in the design, collection, analysis, and interpretation of the data.
Conflict of Interest
The authors declare that the research was conducted in the absence of any commercial or financial relationships that could be construed as a potential conflict of interest.
Publisher’s Note
All claims expressed in this article are solely those of the authors and do not necessarily represent those of their affiliated organizations, or those of the publisher, the editors and the reviewers. Any product that may be evaluated in this article, or claim that may be made by its manufacturer, is not guaranteed or endorsed by the publisher.
Supplementary Material
The Supplementary Material for this article can be found online at: https://www.frontiersin.org/articles/10.3389/fenvs.2022.854460/full#supplementary-material
References
Ávila-Pérez, P., Ortiz-Oliveros, H. B., Zarazúa-Ortega, G., Tejeda-Vega, S., Villalva, A., and Sánchez-Muñoz, R. 2019. “Determining of Risk Areas Due to Exposure to Heavy Metals in the Toluca Valley Using Epiphytic Mosses as a Biomonitor.” J. Environ. Manage. 241 (2018): 138–148. doi:10.1016/j.jenvman.2019.04.018
Acosta, J. A., Gabarrón, M., Faz, A., Martínez-Martínez, S., Zornoza, R., and Arocena, J. M. (2015). Influence of Population Density on the Concentration and Speciation of Metals in the Soil and Street Dust from Urban Areas. Chemosphere 134, 328–337. doi:10.1016/j.chemosphere.2015.04.038
Aguilar, Y., Bautista, F., Quintana, P., Aguilar, D., Trejo-Tzab, R., Goguitchaichvili, A., et al. (2021). Color as a New Proxy Technique for the Identification of Road Dust Samples Contaminated with Potentially Toxic Elements: The Case of Mérida, Yucatán, México. Atmosphere 12 (4), 483. doi:10.3390/atmos12040483
Aguilera, A., Bautista, F., Gutiérrez-Ruiz, M., Ceniceros-Gómez, A. E., Cejudo, R., and Goguitchaichvili, A. (2021b). Heavy Metal Pollution of Street Dust in the Largest City of Mexico, Sources and Health Risk Assessment. Environ. Monit. Assess. 193 (4), 193. doi:10.1007/s10661-021-08993-4
Aguilera, A., Armendariz, C., Quintana, P., García-Oliva, F., and Bautista, F. (2019). Influence of Land Use and Road Type on the Elemental Composition of Urban Dust in a Mexican Metropolitan Area. Pol. J. Environ. Stud. 28 (3), 1535–1547. doi:10.15244/pjoes/90358
Aguilera, A., Bautista-Hernández, D., Bautista, F., Goguitchaichvili, A., and Cejudo, R. (2021c). Is the Urban Form a Driver of Heavy Metal Pollution in Road Dust? Evidence from Mexico City. Atmosphere 12 (2), 266. doi:10.3390/atmos12020266
Aguilera, A., Morales, J. J., Goguitchaichvili, A., García-Oliva, F., Armendariz-Arnez, C., Quintana, P., et al. (2020). Spatial Distribution of Magnetic Material in Urban Road Dust Classified by Land Use and Type of Road in San Luis Potosí, Mexico. Air Qual. Atmos. Health 13 (8), 951–963. doi:10.1007/s11869-020-00851-5
Aguilera, A., Bautista, F., Goguitchaichvili, Avto., and Garcia-Oliva, F. (2021a). Health Risk of Heavy Metals in Street Dust. Front. Biosci. (Landmark Edition) 26 (9), 327–345. doi:10.2741/4896
Angeoletto, F., Essy, C., Ruiz Sanz, J. P., Silva, F. F. d., Albertin, R. M., and Santos, J. W. M. C. (2015). Ecología Urbana: La Ciencia Interdisciplinaria del Planeta Ciudad. DQuestão 13 (32), 6. doi:10.21527/2237-6453.2015.32.6-20
Carranza, D., Carmen, Ma. del., Israde Alcántara, Isabel., Bautista Zúñiga, Francisco., Gogichaishvili, Avto., Márquez Herrera, Ciro., et al. (2015). “Metales Pesados En Suelos Urbanos de Morelia. Michoacán: Influencia de Los Usos de Suelo y Tipos de Vialidad.” Ciencia Nicolaita 0 (65), 120–138. doi:10.1017/CBO9781107415324.004
CONAPO, and SEDESOL (2012). Catálogo Sistema Urbano Nacional 2012. http://www.conapo.gob.mx/work/models/CONAPO/Resource/1539/1/images/PartesIaV.pdf.
Cortés, J. L., Bautista, Fr., Bautista, F., Quintana, P., Aguilar, D., and Goguichaishvili, A. (2015). “The Color of Urban Dust as an Indicator of Contamination by Potentially Toxic Elements: the Case of Ensenada, Baja California, Mexico,” in Chapingo Serie Ciencias Forestales Y Del Ambiente XXI (3) (Mexico: Baja California), 255–266. doi:10.5154/r.rchscfa.2015.02.003
Dehghani, S., Moore, F., Keshavarzi, B., and HaleHale, B. A. (2016). Health Risk Implications of Potentially Toxic Metals in Street Dust and Surface Soil of Tehran, Iran. Ecotoxicol Environ. Saf. 136, 92–103. doi:10.1016/j.ecoenv.2016.10.037
Delgado, C., Bautista, F., Bautista, F., Gogichaishvili, A., Cortés, J. L., Quintana, P., et al. (2019). Identificación De Las Zonas Contaminadas Con Metales Pesados En El Polvo Urbano De La Ciudad De México. Rev. Int. Contam. Ambie. 35 (1), 81–100. doi:10.20937/RICA
Flores-Ramírez, R., Pérez-Vázquez, F. J., Pérez-Vázquez, S. E., Medellín-Garibay, S. E., Aldrete, A. C., Vallejo-Pérez, M. R., et al. (2018). Exposure to Mixtures of Pollutants in Mexican Children from Marginalized Urban Areas. Ann. Glob. Health 84 (2), 250–256. doi:10.29024/aogh.912
Hakanson, L. (1980). An Ecological Risk index for Aquatic Pollution control.a Sedimentological Approach. Water Res. 14 (8), 975–1001. doi:10.1016/0043-1354(80)90143-8
Helaluddin, A., Khalid, R. S., Alaama, M., and Abbas, S. A. (2016). Main Analytical Techniques Used for Elemental Analysis in Various Matrices. Trop. J. Pharm. Res. 15 (2), 427–434. doi:10.4314/tjpr.v15i2.29
Hu, X., Zhang, Y., Luo, J., Wang, T., Lian, H., and Ding, Z. (2011). Bioaccessibility and Health Risk of Arsenic, Mercury and Other Metals in Urban Street Dusts from a Mega-City, Nanjing, China. Environ. Pollut. 159 (5), 1215–1221. doi:10.1016/j.envpol.2011.01.037
Hua, L., Yang, X., Liu, Y., Tan, X., and Yang, Y. (2018). “Spatial Distributions, Pollution Assessment, and Qualified Source Apportionment of Soil Heavy Metals in a Typical Mineral Mining City in China. Sustainability (Switzerland) 10. doi:10.3390/su10093115
Huang, J.-h., Liu, W.-c., Zeng, G.-m., Li, F., Huang, X.-l., Gu, Y.-l., et al. (2016). An Exploration of Spatial Human Health Risk Assessment of Soil Toxic Metals under Different Land Uses Using Sequential Indicator Simulation. Ecotoxicology Environ. Saf. 129, 199–209. doi:10.1016/j.ecoenv.2016.03.029
Ihl, T., Bautista, F., Rubén Cejudo Ruíz, F., Delgado, M., Quintana Owen, P., Aguilar, Da., et al. (2015). “Concentration of Toxic Elements in Topsoils of the Metropolitan Area of Mexico City: A Spatial Analysis Using Ordinary Kriging and Indicator Kriging.”. Internacional de Contaminacion Ambiental 31 (1), 47–62. http://www.scielo.org.mx/scielo.php?script=sci_abstract&pid=S0188-49992015000100004&lng=en&nrm=iso&tlng=en.
INEGI Instituto Nacional de Estadística y Geografía (2014). “Las Zonas Metropolitanas En México.”. Censos Económicos 2014.
Jadoon, W. A., Khpalwak, W., Chidya, R. C. G., Abdel-Dayem, S. M. M. A., Takeda, K., Makhdoom, M. A., et al. (2018). Evaluation of Levels, Sources and Health Hazards of Road-Dust Associated Toxic Metals in Jalalabad and Kabul Cities, Afghanistan. Arch. Environ. Contam. Toxicol. 74 (1), 32–45. doi:10.1007/s00244-017-0475-9
Jahandari, A. (2020). Pollution Status and Human Health Risk Assessments of Selected Heavy Metals in Urban Dust of 16 Cities in Iran. Environ. Sci. Pollut. Res. 27 (18), 23094–23107. doi:10.1007/s11356-020-08585-8
Jayarathne, A., Egodawatta, P., Ayoko, G. A., and Goonetilleke, A. (2018). Intrinsic and Extrinsic Factors Which Influence Metal Adsorption to Road Dust. Sci. Total Environ. 618, 236–242. doi:10.1016/j.scitotenv.2017.11.047
Kabata-Pendias, Alina. (2011). Trace Elements in Soils and Plants. CRC Press. 4th ed. New York: Taylor & Francis. doi:10.1201/b10158-25
Kamali, M. R., Omidvar, A., Kazemzadeh, E., and Kazemzadeh, Ezatallah. (2013). 3D Geostatistical Modeling and Uncertainty Analysis in a Carbonate Reservoir, SW Iran. J. Geol. Res. 2013, 1–7. doi:10.1155/2013/687947
Keshavarzi, B., Abbasi, S., Moore, F., Mehravar, S., Sorooshian, A., Soltani, N., et al. (2018). Contamination Level, Source Identification and Risk Assessment of Potentially Toxic Elements (PTEs) and Polycyclic Aromatic Hydrocarbons (PAHs) in Street Dust of an Important Commercial Center in Iran. Environ. Manage. 62 (4), 803–818. doi:10.1007/s00267-018-1079-5
Keshavarzi, B., Tazarvi, Z., Rajabzadeh, M. A., and Najmeddin, A. (2015). Chemical Speciation, Human Health Risk Assessment and Pollution Level of Selected Heavy Metals in Urban Street Dust of Shiraz, Iran. Atmos. Environ. 119, 1–10. doi:10.1016/j.atmosenv.2015.08.001
Kim, G., Lee, H.-S., Seok Bang, J., Kim, B., Ko, D., and Yang, M. (2015). A Current Review for Biological Monitoring of Manganese with Exposure, Susceptibility, and Response Biomarkers. J. Environ. Sci. Health C 33 (2), 229–254. doi:10.1080/10590501.2015.1030530
Kletetschka, G., Bazala, R., Takáč, M., and Svecova, E. (2021). Magnetic Domains Oscillation in the Brain with Neurodegenerative Disease. Sci. Rep. 11, 714. doi:10.1038/s41598-020-80212-5
Lawrence, Roderick. J. (2003). “Human Ecology and its Applications.”. Landscape Urban Plann. 65 (1–2), 31–40. doi:10.1016/S0169-2046(02)00235-9
Lewis, R. C., Meeker, J. D., Basu, N., Gauthier, A. M., Cantoral, A., Mercado-GarcíaPetersonMartha Maria Téllez-Rojo, A., et al. (2018). Urinary Metal Concentrations Among Mothers and Children in a Mexico City Birth Cohort Study. Int. J. Hyg. Environ. Health 221 (4), 609–615. doi:10.1016/j.ijheh.2018.04.005
Li, H.-H., Chen, L.-J., Yu, L., Guo, Z.-B., Shan, C.-Q., Lin, J.-Q., et al. (2017). Pollution Characteristics and Risk Assessment of Human Exposure to Oral Bioaccessibility of Heavy Metals via Urban Street Dusts from Different Functional Areas in Chengdu, China. Sci. Total Environ. 586, 1076–1084. doi:10.1016/j.scitotenv.2017.02.092
Li, K., Liang, T., Wang, L., and Yang, Z. (2015). Contamination and Health Risk Assessment of Heavy Metals in Road Dust in Bayan Obo Mining Region in Inner Mongolia, North China. J. Geogr. Sci. 25 (12), 1439–1451. doi:10.1007/s11442-015-1244-1
Liang, L., Wang, Z., and Li, J. (2019). The Effect of Urbanization on Environmental Pollution in Rapidly Developing Urban Agglomerations. J. Clean. Prod. 237, 117649. doi:10.1016/j.jclepro.2019.117649
Lin, M., Gui, H., Wang, Y., and Peng, W. (2017). Pollution Characteristics, Source Apportionment, and Health Risk of Heavy Metals in Street Dust of Suzhou, China. Environ. Sci. Pollut. Res. 24 (2), 1987–1998. doi:10.1007/s11356-016-7934-0
Lu, X., Wu, X., Wang, Y., Chen, H., Gao, P., and Fu, Y. (2014). Risk Assessment of Toxic Metals in Street Dust from a Medium-Sized Industrial City of China. Ecotoxicology Environ. Saf. 106, 154–163. doi:10.1016/j.ecoenv.2014.04.022
Marín Sanleandro, P., Sánchez Navarro, A., Díaz-Pereira, E., Bautista Zuñiga, F., Romero Muñoz, M., and Delgado Iniesta, M. (2018). Assessment of Heavy Metals and Color as Indicators of Contamination in Street Dust of a City in SE Spain: Influence of Traffic Intensity and Sampling Location. Sustainability 10 (11), 4105. doi:10.3390/su10114105
Menezes-Filho, J. A., Souza, K. O. F. d., Rodrigues, J. L. G., Santos, N. R. d., Bandeira, M. d. J., Koin, N. L., et al. (2016). Manganese and lead in Dust Fall Accumulation in Elementary Schools Near a Ferromanganese alloy Plant, Ng Lai Koin, Sérgio S. Do Prado Oliveira, Ana Leonor P. Campos Godoy, and Donna Mergler. Environ. Res. 148, 322–329. doi:10.1016/j.envres.2016.03.041
Muñoz-Barbosa, A., Gutiérrez-Galindo, E. A., and Flores-Muñoz, G. (2000). Mytilus californianus as an Indicator of Heavy Metals on the Northwest Coast of Baja California, Mexico. Mar. Environ. Res. 49 (2), 123–144. doi:10.1016/S0141-1136(99)00052-5
Perez-Vazquez, F. J., Flores-Ramirez, R., Ochoa-Martinez, A. C., Orta-Garcia, S. T., Hernandez-Castro, B., Carrizalez-Yañez, L., et al. (2015). Concentrations of Persistent Organic Pollutants (POPs) and Heavy Metals in Soil from San Luis Potosí, México. Environ. Monit. Assess. 187 (1), 4119. doi:10.1007/s10661-014-4119-5
Pérez-Vázquez, F. J., Flores-Ramírez, R., Catalina Ochoa-Martínez, A., Carrizales-Yáñez, L., Arturo Ilizaliturri-Hernández, Cesar., Moctezuma-González, J., et al. (2015). “Human Health Risks Associated with Heavy Metals in Soil in Different Areas of San Luis Potosí. México.” Hum. Ecol. Risk Assess. Int. J. 7039 (September), 1–14. doi:10.1080/10807039.2015.1064760
Rastegari Mehr, M., Keshavarzi, B., Moore, F., Sharifi, R., Lahijanzadeh, A., Kermani, M., et al. (2017). Distribution, Source Identification and Health Risk Assessment of Soil Heavy Metals in Urban Areas of Isfahan Province, Iran. J. Afr. Earth Sci. 132, 16–26. doi:10.1016/j.jafrearsci.2017.04.026
Rodrigues, J. L. G., Araújo, C. F. S., dos Santosdos Santos, N. R., BandeiraBandeira, M. J., AnjosAnjos, A. L. S., CarvalhoCarvalho, C. F., et al. (2018). Airborne Manganese Exposure and Neurobehavior in School-Aged Children Living Near a Ferro-Manganese alloy Plant. Environ. Res. 167 (May), 66–77. doi:10.1016/j.envres.2018.07.007
Safiur Rahman, M., Khan, M. D. H., Jolly, Y. N., Kabir, J., Akter, S., and Salam, A. (2019). Assessing Risk to Human Health for Heavy Metal Contamination through Street Dust in the Southeast Asian Megacity: Dhaka, Bangladesh. Sci. Total Environ. 660 (April), 1610–1622. doi:10.1016/j.scitotenv.2018.12.425
Sánchez-Duque, A., Bautista, F., Goguitchaichvili, A., Cejudo-Ruiz, R., Alonso Reyes-López, J., Amílcar Solís-Domínguez, F., et al. (2015). “Evaluación de La Contaminación Ambiental a Partir Del Aumento Magnético En Polvos Urbanos. Caso de Estudio Para La Ciudad de Mexicali. México.” Mexicana de Ciencias Geológicas 32 (3), 501–513.
SEMARNAT. (2007). NOM-147-SEMARNAT/SSA1-2004. Diario Oficial de La Federación. http://www.salud.gob.mx/unidades/cdi/nom/147ssa16.html.
Shabbaj, I., Alghamdi, A., Shamy, M, Hassan, S, Alsharif, M, and Khoder, M. 2018. Risk Assessment and Implication of Human Exposure to Road Dust Heavy Metals in Jeddah, Saudi Arabia.” Int. J. Environ. Res. Public Health 15. doi:10.3390/ijerph15010036
Tapia, J. S., Valdés, J., Tchernitchin, A., Dorador, C., Bolados, A., and Harrod, C. (2018). Geologic and Anthropogenic Sources of Contamination in Settled Dust of a Historic Mining Port City in Northern Chile: Health Risk Implications. “Geologic Anthropogenic Sourc. Contam. Settled Dust a Historic Mining Port City North. Chile: Health Risk Implications.” PeerJ 6, e4699. doi:10.7717/peerj.4699
Taşpınar, F., and Bozkurt, Z. (2018). “Heavy Metal Pollution and Health Risk Assessment of Road Dust on Selected Highways in Düzce. Turkey.” Environ. Forensics 19 (4), 298–314. doi:10.1080/15275922.2018.1519736
Tomlinson, D. L., Wilson, J. G., Harris, C. R., and Jeffrey, D. W. (1980). “Problems in the Assessment of Heavy-Metal Levels in Estuaries and the Formation of a Pollution Index.”. Helgoländer Meeresuntersuchungen 33 (1–4), 566–575. doi:10.1007/BF02414780
Trujillo-González, J. M., Torres-Mora, M. A., Keesstra, S., Brevik, E. C., and Jiménez-Ballesta, R. (2016). Heavy Metal Accumulation Related to Population Density in Road Dust Samples Taken from Urban Sites under Different Land Uses. Sci. Total Environ. 553, 636–642. doi:10.1016/j.scitotenv.2016.02.101
USEPA. (2001). U.S. Environmental Protection Agency. Risk Assess. Guidance Superfund (Rags) Volume - A: Process Conducting Probabilistic Risk Assess. Appendix B III, 02–002. http://www.epa.gov/sites/production/files/2015-09/documents/rags3adt_complete.pdf.
Keywords: street dust, pollution load index, risk assessment, lead, Mexico
Citation: Aguilera A, Cortés JL, Delgado C, Aguilar Y, Aguilar D, Cejudo R, Quintana P, Goguitchaichvili A and Bautista F (2022) Heavy Metal Contamination (Cu, Pb, Zn, Fe, and Mn) in Urban Dust and its Possible Ecological and Human Health Risk in Mexican Cities. Front. Environ. Sci. 10:854460. doi: 10.3389/fenvs.2022.854460
Received: 14 January 2022; Accepted: 16 February 2022;
Published: 14 March 2022.
Edited by:
Juan Manuel Trujillo-González, University of the Llanos, ColombiaReviewed by:
Raimundo Jimenez Ballesta, Autonomous University of Madrid, SpainRogelio Flores Ramírez, Universidad Autónoma de San Luis Potosí, Mexico
Copyright © 2022 Aguilera, Cortés, Delgado, Aguilar, Aguilar, Cejudo, Quintana, Goguitchaichvili and Bautista. This is an open-access article distributed under the terms of the Creative Commons Attribution License (CC BY). The use, distribution or reproduction in other forums is permitted, provided the original author(s) and the copyright owner(s) are credited and that the original publication in this journal is cited, in accordance with accepted academic practice. No use, distribution or reproduction is permitted which does not comply with these terms.
*Correspondence: Francisco Bautista, bGVwdG9zb2xAY2lnYS51bmFtLm14