- 1Key Laboratory of Urban Stormwater System and Water Environment, Ministry of Education, Beijing University of Civil Engineering and Architecture, Beijing, China
- 2College of Resources and Environment, Chengdu University of Information Technology, Chengdu, China
- 3School of Environment, Beijing Normal University, Beijing, China
As the consumption of Chinese medicine resources increases, the waste of traditional Chinese medicine extraction cannot be disposed of reasonably, which has a serious impact on the environment. Dissolved organic matter (DOM), a crucial fraction in herbal extraction residue, can bond to heavy metals (HMs), creating a potential environmental risk. This study investigated the binding property of herbal extraction residue DOM with Cu(II) via two-dimensional Fourier transform infrared (FTIR) and synchronous fluorescence correlation spectroscopy (2D-COS) in conjunction with parallel factor (PARAFAC) modeling. Through PARAFAC analysis, three kinds of protein components and one kind of fulvic acid can be obtained, and protein-like substances are dominant in the residual DOM of Chinese medicine extracts, becoming the main factor of water quality deterioration. A fluorescence quenching experiment shows that protein-like materials provide the primary binding sites with Cu(II). During the detection, the long-wavelength low-intensity signal will be obscured. The 2D-COS obtained by the 1/9th power transformation can enhance the fluorescence signal so as to get even more information about the binding sites and heterogeneity of DOM and heavy metal ion ligands. The N−H of amine in the protein-like materials could prioritize combining with Cu(II). This study urges that continuous and effective attention be paid to the impact of the herbal extraction residue on the geochemical behavior of HMs.
Introduction
Chinese herbal medicine is considered one of the traditional industries in China. With the rapid development of this industry, the output of herbal extraction residues from the major Chinese medicine pharmaceutical factories increases year by year (Yue et al., 2017; Zhang et al., 2019; Zhang et al., 2021). Herbal extraction residues are rich in cellulose, organic carbon, nitrogen, phosphorus, and other soil nutrients (Wang et al., 2010; Zhou et al., 2018; Ma H et al., 2019); they are a typical biological resource with high utilization value (Huang et al., 2021). The early types of herbal extraction residue treatment included mainly landfill, incineration, and fixed-area stacking—treatments that have not only negatively affected the economy but also caused resource wastage and ecological problems (Ma J et al., 2019; Zhang et al., 2019).
Herbal extraction residues generally have a high moisture content, making them extremely prone to corruption, and consequently, casual discharge of the residue negatively affects the water and soil quality that brings great challenges to the management and resource utilization of herbal extraction residues. Especially in rural areas, weak environmental awareness and lack of environmental infrastructure increase the environmental risks associated with the haphazard stacking and discarding of herbal extraction residues. Dissolved organic matter (DOM) constitutes a significant fraction of herbal extraction residues that can be extracted by water (Gryboset al., 2009). DOM contains abundant active functional groups, including amino, methylene, amide, phenolic hydroxyl, and other functionalities, as an important natural ligand and sorption carrier in the environment, and it is a very active chemical substance, which affects its environmental behavior by linking and interacting with minerals and organic matter (Li et al., 2017; Wei et al., 2019). At the same time, DOM includes humic-, fulvic-, and protein-like elements. Protein-like substances exhibit strong complexation ability with cadmium and even more with copper (Yuan et al., 2015). The complexation of fulvic- and humic-like components with Fe(III), Cu(II), and Cr(III) can also enhance the migration ability of these metal ions (Guoet al., 2020). Thus, deep understanding of the binding characteristics of heavy metals (HMs) with DOM derived from herbal extraction residues is of scientific importance and should be studied (Uchimiyaet al., 2010).
It is well known that spectral techniques present the advantages of rapid and convenient analysis, simple sample pretreatment, and low cost and are regarded as the primary characterization techniques for studying the properties of DOM components, functional groups, chemical bonds, and molecular space configuration (Birdwell and Engel, 2010; Li et al., 2018). For example, the excitation–emission matrix (EEM) technique can directly reflect the intensity of fluorescence peaks and is a quick but sensitive method for exploring the composition of DOM without damaging the sample (Chai et al., 2019). EEM combined with parallel factor (PARAFAC) analysis can be applied to analyze different types of DOM corresponding to independent characteristic fluorescence components, since it can solve some problems of the overlapping peak to a certain extent (Yamashita and Jaffé, 2008). Moreover, two-dimensional correlation spectroscopy (2D-COS) can raise the resolution of the spectral signal and reveal the relationships among the changes of different signal peaks (Noda and Ozaki, 2004). Thus, 2D-COS is able to explore the differences of binding heterogeneity in DOM and HMs, such as the binding sequence of different sites with metal ions in the complexation process (Xu et al., 2013). Finally, two-dimensional Fourier transform infrared (FTIR) and synchronous fluorescence correlation spectroscopy (2D-FTIR-COS and 2D-SFS-COS) have been extensively used to research the binding properties between DOM and metal ions (Hurand Lee, 2011; Huang et al., 2018; Guo et al., 2019; Guo et al., 2020).
The log-transformed 2D-COS analysis has been applied to understand the binding sites of DOM-HM complexes (Tian et al., 2021; Guo et al., 2021). However, we have found many deficiencies in the log-transformed 2D-COS analysis. When fluorescent signals are non-linearly transformed, their physically relevant information will be destroyed. And then, asynchronous spectra and, consequently, the sequential order of events will also be lost. Herein, an alternative approach can avoid these deficiencies by the transformation of the 2D correlation intensities after the 2D-COS analysis but not before. In this work, an alternative approach will be used for identifying small features obscured by strong peaks. Square or cube root transformations (or 1/n-th power in general) after the 2D correlation can preserve the sequential order information.
The current research on Chinese medicine residues mainly focuses on microbial treatment, the extraction of Chinese medicine residues and their resource utilization, etc. Some scholars’ research on DOM mainly focuses on its own migration characteristics, etc. However, in view of the lack of research on DOM in Chinese herbal dregs and its complexation with heavy metals, it is necessary to explore the relationship between the decomposition of DOM in Chinese herbal dregs and the environmental impact of its production of DOM–metal complexes. The aims of this work are to a) analyze the spectral characteristics and components of DOM derived from the herbal extraction residue; b) explore the influence of DOM on the species distribution, mobility, and bioavailability of HMs by fluorescence quenching in couple with the complexation mode, using PARAFAC and 2D-COS analysis; and c) according to the experimental results, analyze the potential influence of consequently casual discharge of the residue on soil and water environment, and the challenges to the management of the herbal extraction residue. This study provides a solid foundation for controlling heavy metal pollution and controlling and regulating the residual pollution of medium- and high-active substance extracts.
Materials and Methods
DOM Extraction
For the preparation of a Chinese herbal medicine, herbal extraction residues were boiled together with all kinds of ingredients. Some of these herbal extraction residues were collected from three pharmacies, and the mixed samples were dried using a freeze dryer, then sieved using a 100-mesh sieve, and ground. The samples and Milli-Q water were mixed at a ratio of 1:10 (w/v) and then shaken horizontally for 24 h at 20°C so as to collect the DOM samples. The DOM extract was centrifuged at 8,000 rpm for 12 min and then filtered to 0.45 microns (Millipore). Dissolved organic carbon concentration (DOC) is a typical DOM concentrate obtained with a total organic carbon analyzer.
Quenching Experiments
To remove the inner filter influence and ensure the comparability of samples, TOC with an absorbance of less than 0.08 at 350 nm was used for the quenching experiments. The temperature of the solution was fixed at 20°C. NaOH and HNO3 were added to the processor, and the pH value of the samples was within the range of 7.5 ± 0.05. Quenching experiments were carried on by adding Cu(II) ion solutions to the DOM derived from the herbal extraction residues. The ultimate Cu(II) concentrations of DOM solutions were controlled at 0, 20, 40, 60, 80, 100, 120, 150, 180, 210, 240, 270, 300, and 350 μmol L−1. To overcome the influence of concentration, the proportion of added metal solution was kept relatively low (less than 5%). All DOM-HM samples were shaken using a horizontal oscillator for 24 h. The reaction equilibrium was maintained at 20°C. To quantitatively describe the complexation reaction between Cu(II) and DOM, a linear model modified Stern–Volmer (S-V) equation was made available to determine the complexation stability constant for the DOM-HM complexes (Lu and Jaffe, 2001), where it was assumed that metal ions form a 1:1 complex with DOM.
where F0 and F indicate the intensity of the fluorescent component without the metal added and with the concentration of CM, respectively; f is the initial fluorescence fraction available to the eliminator; and KM indicates the conditional stability constant (Wu et al., 2004).
Spectral Scanning
The EEM data were collected with an excitation limit of 200–450 nm, the emission extent of 300–550 nm, with a 5-nm gap for both excitation and emission, using a Hitachi F-7000 spectrofluorometer (Hitachi, Japan) at 20°C. To subtract Raman scattering of water, Millie-q water was used for the purpose of a reference for all EEM data. The absorption spectra were applied to correct inner filter effects (Jiang et al., 2017). The acquisition of SFS is constant between the excitation wave and the emission wavelength (Δλ = 60 nm). The solid powder of the DOM sample was collected using vacuum freeze-drying equipment for the measurement of FTIR. The FTIR spectrum was generated from wavelengths of 3,600 to 800 cm−1.
2D-COS Analysis and the PARAFAC Model
The PARAFAC model is based on the trilinear decomposition theory and an iterative three-dimensional matrix decomposition algorithm with the principle of alternating least squares. The essence of PARAFAC is a decomposition algorithm that uses the core consistency test to obtain the most appropriate group fraction (Bro and Kiers, 2003). The preprocessed data are processed in MATLAB 12.0 software (MathWorks Company, the Natick, Massachusetts of United States). At the same time, split-half analysis and defect analysis were applied to verify the effectiveness of the PARAFAC model and determine the optimal DOM component fraction. A detailed theory was introduced in the study (Stedmon and Bro,2008). And in the current study, 56 EEM data collected from DOM-Cu(II) samples were used with the PARAFAC model. The PARAFAC model showed that a four-substance model could be verified with defect analysis, split-half analysis, and loadings. The maximum fluorescence intensity (Fmax) was obtained from the PARAFAC model for all the DOM-HM samples (Stedmon and Bro, 2008).
2D-COS has been applied as an analytical tool for identifying subtle changes in a spectrum caused by adding HMs (Ozaki et al., 2001). Herein, 2D-FTIR-COS was applied to reveal the binding heterogeneity of herbal extraction residue DOM with Cu(II) ions. “2D Shige” software was used to obtain synchronous and asynchronous maps. The synchronous 2D-COS map was symmetric on the leading line; its peaks belonged to auto-peaks and were induced by an external disturbance condition (different Cu(II) concentrations). The asynchronous 2D-COS map was asymmetric around the main diagonal line; these are just diagonal vertices and no auto-peaks (Ozaki et al., 2001). The transformation of the 2D correlation intensities was further treated by 1/n-th power transformation (n = 9). Origin 2018 was used to draw correlation maps after 1/9-th power transformation.
Results and Discussion
Fluorescent Components
Figure 1 reveals the fluorescence components obtained by PARAFAC analysis. Four fluorescence components can be identified from the herbal extraction residue DOM: one humic-like (Component 4) and three protein-like components (Components 1, 2, and 3). Component 1 (C1) included two excitation maxima at 220 and 275 nm, with an emission maximum at 330 nm and a shoulder at 310 nm. It was related to a tryptophan-like fluorophore in conjunction with a tyrosine-like peak, and the tryptophan-like fluorophore was an analog to the traditional tryptophan-like peak B. The presence of Component 1 suggested that the tyrosine-like fluorophore was converted into a tryptophan-like fluorophore. Component 2 (C2) was a typical protein-like material such as a tyrosine-like material and exhibited a main peak at 275 nm and a second peak at 230 nm, with the same maximum excitation wavelength at 310 nm. Component 3 (C3) consisted of three peaks, at 265, 285, and 330 nm, with an emission maximum at 345 nm. This substance was also related to tryptophan-like substances and can be considered a biologically labile substance or an amino acid (Yao et al., 2011; Guo et al., 2020). Similarly, three fluorescent peaks at 240, 290, and 320 nm with a maximum emission wavelength of 435 nm could be found in Component 4 (C4). Component 4 was due to the substance of fluorescent fulvic-like substances.
The maximum fluorescence intensity (Fmax) shows the relative prevalence of fluorescent components in the herbal extraction residue DOM. It can be observed from Figure 2 that the relative abundance of the four substances followed the order of C1>C2>C3>C4, accounting for 54.79, 25.90, 15.95, and 3.36%, respectively. It should be noted that fulvic-like C4 presented an extremely low Fmax value. These results also indicate which protein-like fluorescent components were mainly fluorophores in the herbal extraction residue DOM after long decoction of Chinese medicine. A strong correlation between biochemical oxygen demand (BOD) and protein-like fluorescent components has also been explained in the literature (Hudson et al., 2008). Fluorescent components showed a strong correlation with BOD, chemical total nitrogen (TN), and oxygen demand (COD) concentrations (Hurand Cho, 2012), suggesting that the release of herbal extraction residue DOM can lead to a risk of water quality deterioration. Therefore, it is very important to prevent the discarding of herbal extraction residues to protect the water environment.
2D-SFS-COS Analysis
Two positive peaks could be identified in the synchronous map of DOM-Cu(II) at 275 and 383 nm, respectively (Figure 3). The peak at 275 nm has a bearing on protein-like materials, such as tryptophan- or tyrosine-like, and the peak at 383 nm was indicative of humic-like constituents (Guoet al., 2022). In view of Noda’s rule (Noda and Ozaki, 2004), the protein-like constituents at 275 nm exhibited a high sensitivity of Cu(II) compared to the humic-like constituents at 383 nm. The asynchronous map of DOM-Cu(II) presented one positive and two negative peaks at 286, 301, and 383 nm, separately. The presence of peaks at 286 and 301 nm belonged to the presence of protein-like materials, specifically tryptophan-like materials (Barker et al., 2009; Tan et al., 2021). The protein-like materials identified at a shorter wavelength (286 nm) in Figure 3 indicated the preferential binding of Cu(II) to the herbal extraction residue DOM. In addition, the priority binding with Cu(II) followed the subsequence as 286→ 383→301 nm.
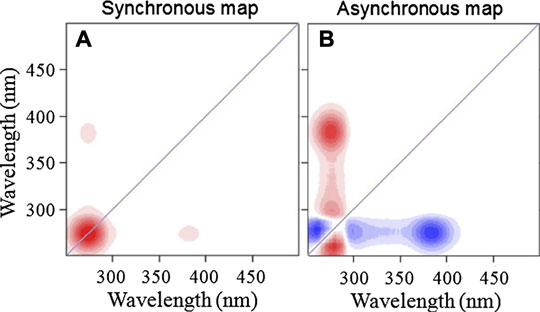
FIGURE 3. 2D-COS analysis obtained by synchronous fluorescence spectra. The red regions show positive correlations, and the blue regions show negative correlations. An increase in the intensity of a particular color represents a stronger positive or negative correlation. (A): Synchronous map; (B): asynchronous map.
Figure 4 shows the asynchronous and synchronous maps of 2D-COS of DOM-Cu(II) obtained by the 1/9-th power transformation. The synchronous map displayed two cross- and two auto-peaks at 383/275 nm, 327/275 nm, 275, and 383 nm, separately. The analysis outcome of the synchronous map was consistent with the result of the 2D-COS that protein-like fluorophores at 275 nm exhibited a high sensitivity to Cu(II) compared to the humic-like components at 383 nm. However, the asynchronous map indicated that eight fluorescent signal regions may be found at 286 nm, 301 nm, 327 nm, 383/275 nm, 383/327 nm, 409 nm, 433 nm, and 463 nm, suggesting that the 1/9-th power transformation was feasible for identifying small features obscured by strong peaks. A peak at 327 nm can be found, which was assigned to fulvic-like components. The peaks at 409 nm, 433 nm, and 463 nm were also concerned with humic-like fluorophores (Guoet al., 2022). According to the results of 2D-SFS-COS obtained by the 1/9-th power transformation, the priority binding of Cu(II) followed the sequence as protein-like (286 nm) → fulvic-like (327 nm) → humic-like (409 nm) → humic-like (433 nm) → humic-like (463 nm) → humic-like (383 nm) → protein-like (301 nm). The results also indicated that the 2D-SFS-COS obtained by the 1/9-th power transformation strengthened shielded long-wavelength and low-intensity fluorescence signals and can obtain more information such as ligand binding characteristics.
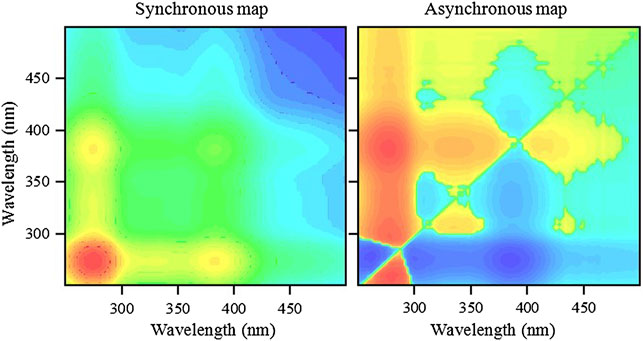
FIGURE 4. Asynchronous and synchronous maps of 2D-COS of DOM-Cu(II) obtained by the 1/9-th power transformation. The red regions show positive correlations, and the blue regions show negative correlations. An increase in the intensity of a particular color represents a stronger positive or negative correlation.
2D-FTIR-COS
2D-COS in conjunction with FTIR spectra has been extensively used to explore information on potential binding sites (Xu et al., 2013; Guo et al., 2020). Figure 5 depicts the asynchronous and synchronous maps of 2D-FTIR-COS of DOM-Cu(II). The synchronous map of DOM-Cu(II) suggests that a large auto-peak and three cross-peaks appeared at about 2,300 and 2,920 cm−1, separately. The peaks at about 2,300 cm−1 were considered to be the C≡C stretching vibration of alkyne, while the band at 2,920 cm−1 was considered to be the C−O vibration of carboxylic acid or the C−H stretching vibration of alkane (Yang et al., 2019). These results reveal a preferential response in the functional group of alkyne due to the increase in Cu(II) concentration.
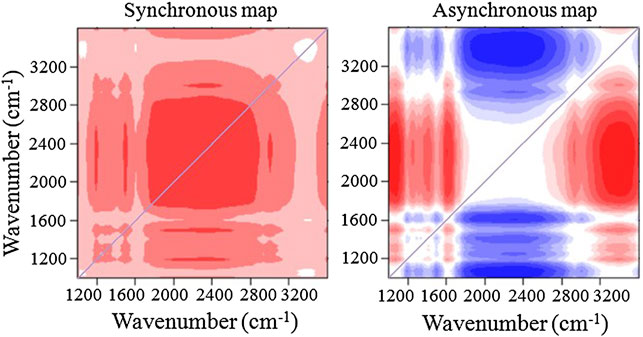
FIGURE 5. 2D-COS analysis obtained by FTIR of DOM-Cu(II). The red regions show positive correlations, and the blue regions show negative correlations. An increase in the intensity of a particular color represents a stronger positive or negative correlation.
The asynchronous map of DOM-Cu(II) exhibits three positive bands (3,370, 2,920, and 1,600 cm−1) and four negative bands (2,270, 1,490, 1,330, and 1,180 cm−1). These bands contain about seventeen binding sites, suggesting that the herbal extraction residue DOM provided a large number of binding sites for Cu(II). According to previous literature (Yu et al., 2012; Huang et al., 2018; Guo et al., 2020), the peaks at 3,370 and 1,600 cm−1 are related to the N−H stretching of amine and the aromatic C=C stretching or N−H bending of primary amide (band II), separately. The two peaks at 1,490 and 1,330 cm−1 were down to the OH deformation of the phenolic hydroxyl group, and the C−O vibration of esters or the C−N stretching of aliphatic amine were identified according to the band at 1,180 cm−1. As a result, the N−H of amine functionalities could preferentially integrate with Cu(II), and the Cu(II) binding with the herbal extraction residue DOM followed the order 3370→2920 →1600→2270→1490→1180→1330 cm−1.
Binding Parameters
Supplementary Figure S1 exhibits the quenching curves of Cu(II) with the herbal extraction residue DOM for the fluorescence substance determined by the PARAFAC model and the 2D-COS analysis. The fluorescence intensity of protein fluorophores declined with the increase in the Cu (II) ion content. Protein-like materials presented better quenching effects in the DOM-Cu(II). 2D-FTIR-COS also revealed that the N−H of amine preferentially combined with Cu(II), suggesting that the N−H of amine mainly originated from the protein-like fluorophore. Fulvic-like fluorophores showed poor quenching effects due to the presence of limited fluorophore which could bind with Cu(II) ion. Clear quenching curves for these peaks with low intensities can be found in Supplementary Figure S1.
Table 1 lists the parameters of DOM-Cu(II) calculated with the revised Stern–Volmer model. The log KM value was in the range of 3.96–4.24 for components 1–4, and 4.14–4.81 for C275, C286, C299, C327, and C433, respectively. These results suggested that the log KM value of different components with Cu(II) increased with an increase in the wavelength of these components. However, the log KM values were generally lower than those of DOM-Cu(II) from other sources, such as digestate (Guoet al., 2020), lake water (Craven et al., 2012), and biochar (Guoet al., 2021; Guo et al., 2022), indicating a relatively chemical stability of DOM-Cu(II). The f% value decreased with the increased wavelength of fluorescent components. However, Component 1 revealed the largest f% value, suggesting that a combination of tyrosine- and tryptophan-like fluorophores can increase the concentration of the total ligand. Humic-like substances showed the largest log KM value, followed by fulvic-like substances, compared with protein-like materials. Although fulvic- and humic-like substances with Cu(II) have high log KM values, their contents were dramatically low in the herbal extraction residue DOM. Therefore, protein-like fluorophores dominate due to their high fluorophore content.
Environmental Impact and Control Measures
Traditional Chinese medicine, including herbal medicine, is still used extensively in China. As mentioned earlier, the haphazard stacking and discarding of herbal extraction residue put tremendous pressure on the environment, especially in rural areas. Protein-like substances dominated in the herbal extraction residue DOM used in this study, and this residue can easily enter rivers via rainwater leaching, resulting in the deterioration of the water environment. The herbal extraction residue DOM and HMs can affect each other, affecting their transformation, biotoxicity, and other properties. With the increase in HM pollution in the soil, herbal extraction residue DOM increases the risk of HMs entering the groundwater from the soil because the complexes formed by DOM-HM have low stability. Therefore, the treatment and utilization of the herbal extraction residues have become an urgent problem, and solving this problem can reduce the environmental deterioration caused by the random stacking and discarding of herbal extraction residues. Biomass energy, papermaking, composites, and re-extraction of bioactive components are promising solutions for herbal extraction residues (Lu and Li, 2021). For example, herbal extraction residues have been used to explore the feasibility of producing biogas for industry (Cheng and Liu, 2010; Li et al., 2011). Herbal extraction residues can also be used to prepare biochar materials for other applications (Wang et al., 2010). In addition, the improvement of environmental protection awareness and environmental infrastructure is also an important way to solve the environmental pollution of the herbal extraction residue in rural areas. The implementation of garbage classification can greatly reduce the environmental risk of the herbal extraction residues.
Conclusion
Chinese medicine residues—especially leached Chinese medicine residues, extraction residues, and DOM—bring huge risks to the environment through the migration, transformation, and biotoxicity of HMs. Through the methods of fluorescence EEM and PARAFAC model, the following conclusions are drawn: (1) A fulvic acid-like component and three protein-like components were identified from the herbal extract residues. This leaching poses a potential contamination risk to the aquatic environment and soil, especially due to the presence of major protein-like components. (2) The use of FTIR and SFS combined with 2D-COS can help explore the information of the binding site, which can shed light on the underlying mechanism of the complexation between DOM and HM. These binding sites are mainly derived from protein-like substances, such as the N-H of amine groups. (3) By the PARAFAC model and 2D-COS analysis, the fluorescence intensity of protein fluorophores decreased with the increase in the Cu(II) ion content. Protein-based materials showed a better quenching effect in DOM-Cu(II). In summary, this work can provide a scientific evaluation for the environmental impact of residual DOM in traditional Chinese medicine extracts, indicating that management and governance are extremely important and worthy of attention.
Data Availability Statement
The original contributions presented in the study are included in the article/Supplementary Material, further inquiries can be directed to the corresponding author.
Author Contributions
DY: writing—editing and review, and funding acquisition. YC: data curation, writing—original draft preparation. YK: writing—editing and review, and funding acquisition. XG: writing—review and visualization. BC: methodology.
Funding
This work was financially supported by the Science and Technology Major Project of Sichuan Province, China (2018SZDZX0024); the Project Funding of Chengdu University of Information Technology, China (KYTZ201744); the Key Research and Development Project of the Science and Technology Department of Sichuan Province, China (2021YFG0267); and the Fundamental Research Funds for Beijing University of Civil Engineering and Architecture (X20137).
Conflicts of Interest
The authors declare that the research was conducted in the absence of any commercial or financial relationships that could be construed as a potential conflict of interest.
The reviewer DF declared a shared affiliation with the author BC.
Publisher’s Note
All claims expressed in this article are solely those of the authors and do not necessarily represent those of their affiliated organizations, or those of the publisher, the editors, and the reviewers. Any product that may be evaluated in this article, or claim that may be made by its manufacturer, is not guaranteed or endorsed by the publisher.
Supplementary Material
The Supplementary Material for this article can be found online at https://www.frontiersin.org/articles/10.3389/fenvs.2022.856308/full#supplementary-material
References
Barker, J. D., Sharp, M. J., and Turner, R. J. (2009). Using Synchronous Fluorescence Spectroscopy and Principal Components Analysis to Monitor Dissolved Organic Matter Dynamics in a Glacier System. Hydrol. Process. 23, 1487–1500. doi:10.1002/hyp.7274
Birdwell, J. E., and Engel, A. S. (2010). Characterization of Dissolved Organic Matter in Cave and spring Waters Using UV-Vis Absorbance and Fluorescence Spectroscopy. Org. Geochem. 41, 270–280. doi:10.1016/j.orggeochem.2009.11.002
Bro, R., and Kiers, H. A. L. (2003). A New Efficient Method for Determining the Number of Components in PARAFAC Models. J. Chemometrics 17, 274–286. doi:10.1002/cem.801
Chai, L., Huang, M., Fan, H., Wang, J., Jiang, D., Zhang, M., et al. (2019). Urbanization Altered Regional Soil Organic Matter Quantity and Quality: Insight from Excitation Emission Matrix (EEM) and Parallel Factor Analysis (PARAFAC). Chemosphere 220, 249–258. doi:10.1016/j.chemosphere.2018.12.132
Cheng, X.-Y., and Liu, C.-Z. (2010). Enhanced Biogas Production from Herbal-Extraction Process Residues by Microwave-Assisted Alkaline Pretreatment. J. Chem. Technol. Biotechnol. 85, 127–131. doi:10.1002/jctb.2278
Craven, A. M., Aiken, G. R., and Ryan, J. N. (2012). Copper(II) Binding by Dissolved Organic Matter: Importance of the Copper-To-Dissolved Organic Matter Ratio and Implications for the Biotic Ligand Model. Environ. Sci. Technol. 46, 9948–9955. doi:10.1021/es301015p
Grybos, M., Davranche, M., Gruau, G., Petitjean, P., and Pédrot, M. (2009). Increasing pH Drives Organic Matter Solubilization from Wetland Soils under Reducing Conditions. Geoderma 154, 13–19. doi:10.1016/j.geoderma.2009.09.001
Guo, X.-J., He, X.-S., Li, C.-W., and Li, N.-X. (2019). The Binding Properties of Copper and lead onto Compost-Derived DOM Using Fourier-Transform Infrared, UV-Vis and Fluorescence Spectra Combined with Two-Dimensional Correlation Analysis. J. Hazard. Mater. 365, 457–466. doi:10.1016/j.jhazmat.2018.11.035
Guo, X., Peng, Y., Li, N., Tian, Y., Dai, L., Wu, Y., et al. (2022). Effect of Biochar-Derived DOM on the Interaction between Cu(II) and Biochar Prepared at Different Pyrolysis Temperatures. J. Hazard. Mater. 421, 126739. doi:10.1016/j.jhazmat.2021.126739
Guo, X., Wu, Y., Li, N., Tian, Y., Peng, Y., and Yuan, D. (2021). Effects on the Complexation of Heavy Metals onto Biochar-Derived WEOM Extracted from Low-Temperature Pyrolysis. Ecotoxicology Environ. Saf. 221, 112456. doi:10.1016/j.ecoenv.2021.112456
Guo, X., Xie, X., Liu, Y., Wang, C., Yang, M., and Huang, Y. (2020). Effects of Digestate DOM on Chemical Behavior of Soil Heavy Metals in an Abandoned Copper Mining Areas. J. Hazard. Mater. 393, 122436. doi:10.1016/j.jhazmat.2020.122436
Huang, C., Li, Z.-X., Wu, Y., Huang, Z.-Y., Hu, Y., and Gao, J. (2021). Treatment and Bioresources Utilization of Traditional Chinese Medicinal Herb Residues: Recent Technological Advances and Industrial prospect. J. Environ. Manage. 299, 113607. doi:10.1016/j.jenvman.2021.113607
Huang, M., Li, Z., Huang, B., Luo, N., Zhang, Q., Zhai, X., et al. (2018). Investigating Binding Characteristics of Cadmium and Copper to DOM Derived from Compost and rice Straw Using EEM-PARAFAC Combined with Two-Dimensional FTIR Correlation Analyses. J. Hazard. Mater. 344, 539–548. doi:10.1016/j.jhazmat.2017.10.022
Hudson, N., Baker, A., Ward, D., Reynolds, D. M., Brunsdon, C., Carliell-Marquet, C., et al. (2008). Can Fluorescence Spectrometry Be Used as a Surrogate for the Biochemical Oxygen Demand (BOD) Test in Water Quality Assessment? an Example from South West England. Sci. Total Environ. 391, 149–158. doi:10.1016/j.scitotenv.2007.10.054
Hur, J., and Cho, J. (2012). Prediction of BOD, COD, and Total Nitrogen Concentrations in a Typical Urban River Using a Fluorescence Excitation-Emission Matrix with PARAFAC and UV Absorption Indices. Sensors 12, 972–986. doi:10.3390/s120100972
Hur, J., and Lee, B.-M. (2011). Characterization of Binding Site Heterogeneity for Copper within Dissolved Organic Matter Fractions Using Two-Dimensional Correlation Fluorescence Spectroscopy. Chemosphere 83, 1603–1611. doi:10.1016/j.chemosphere.2011.01.004
Jiang, T., Skyllberg, U., Björn, E., Green, N. W., Tang, J., Wang, D., et al. (2017). Characteristics of Dissolved Organic Matter (DOM) and Relationship with Dissolved Mercury in Xiaoqing River-Laizhou Bay Estuary, Bohai Sea, China. Environ. Pollut. 223, 19–30. doi:10.1016/j.envpol.2016.12.006
Li, G., Khan, S., Ibrahim, M., Sun, T.-R., Tang, J.-F., Cotner, J. B., et al. (2018). Biochars Induced Modification of Dissolved Organic Matter (DOM) in Soil and its Impact on Mobility and Bioaccumulation of Arsenic and Cadmium. J. Hazard. Mater. 348, 100–108. doi:10.1016/j.jhazmat.2018.01.031
Li, M., Zhang, A., Wu, H., Liu, H., and Lv, J. (2017). Predicting Potential Release of Dissolved Organic Matter from Biochars Derived from Agricultural Residues Using Fluorescence and Ultraviolet Absorbance. J. Hazard. Mater. 334, 86–92. doi:10.1016/j.jhazmat.2017.03.064
Li, Y., Yan, X.-L., Fan, J.-P., Zhu, J.-H., and Zhou, W.-B. (2011). RETRACTED: Feasibility of Biogas Production from Anaerobic Co-digestion of Herbal-Extraction Residues with Swine Manure. Bioresour. Tech. 102, 6458–6463. doi:10.1016/j.biortech.2011.03.093
Lu, Q., and Li, C. (2021). Comprehensive Utilization of Chinese Medicine Residues for Industry and Environment protection: Turning Waste into Treasure. J. Clean. Prod. 279, 123856. doi:10.1016/j.jclepro.2020.123856
Lu, X., and Jaffe, R. (2001). Interaction between Hg(II) and Natural Dissolved Organic Matter: a Fluorescence Spectroscopy Based Study. Water Res. 35, 1793–1803. doi:10.1016/S0043-1354(00)00423-1
Ma, H., Li, H., Zhang, F., Wang, Q., and Tu, M. (2019). Effects of Nitrogen Substitute and Hypericum perforatum Extract on the Ethanol Fermentation of Traditional Chinese Medicine Dregs. Ind. Crops Prod. 128, 385–390. doi:10.1016/j.indcrop.2018.11.021
Ma, J., Chen, Y., Zhao, Y., Chen, D., and Wang, H. (2019). Effects of Traditional Chinese Medicine Residue on Plant Growth and Soil Properties: a Case Study with maize (Zea mays L.). Environ. Sci. Pollut. Res. 26, 32880–32890. doi:10.1007/s11356-019-06322-4
Noda, I., and Ozaki, Y. (2004). Two-dimensional Correlation Spectroscopy: Applications in Vibrational and Optical Spectroscopy. London: John Wiley & Sons.
Ozaki, Y., Czarnik-Matusewicz, B., and Šašic, S. (2001). Two-dimensional Correlation Spectroscopy in Analytical Chemistry. Anal. Sci. 17, i663–i666. doi:10.14891/analscisp.17icas.0.i663.0
Stedmon, C. A., and Bro, R. (2008). Characterizing Dissolved Organic Matter Fluorescence with Parallel Factor Analysis: a Tutorial. Limnol. Oceanogr. Methods 6, 572–579. doi:10.4319/lom.2008.6.57210.4319/lom.2008.6.572b
Tan, F., Zhu, Q., Guo, X., and He, L. (2021). Effects of Digestate on Biomass of a Selected Energy Crop and Soil Properties. J. Sci. Food Agric. 101, 927–936. doi:10.1002/jsfa.10700
Tian, Y., Wu, Y., Peng, Y., Guo, X., Li, Y., Dai, B., et al. (2021). Study on the Complexation of Heavy Metals onto Biogas Slurry DOM Using Two-Dimensional Correlation Spectroscopy Combined with the Log-Transformed Synchronous Fluorescence Spectroscopy. Environ. Sci. Pollut. Res. 28, 22878–22885. doi:10.1007/s11356-021-12401-2
Uchimiya, M., Lima, I. M., Klasson, K. T., and Wartelle, L. H. (2010). Contaminant Immobilization and Nutrient Release by Biochar Soil Amendment: Roles of Natural Organic Matter. Chemosphere 80, 935–940. doi:10.1016/j.chemosphere.2010.05.020
Wang, P., Zhan, S., Yu, H., Xue, X., and Hong, N. (2010). The Effects of Temperature and Catalysts on the Pyrolysis of Industrial Wastes (Herb Residue). Bioresour. Tech. 101, 3236–3241. doi:10.1016/j.biortech.2009.12.082
Wei, J., Tu, C., Yuan, G., Zhou, Y., Wang, H., and Lu, J. (2020). Limited Cu(II) Binding to Biochar DOM: Evidence from C K-Edge NEXAFS and EEM-PARAFAC Combined with Two-Dimensional Correlation Analysis. Sci. Total Environ. 701, 134919. doi:10.1016/j.scitotenv.2019.134919
Wu, F., Cai, Y., Evans, D., and Dillon, P. (2004). Complexation between Hg(II) and Dissolved Organic Matter in Stream Waters: an Application of Fluorescence Spectroscopy. Biogeochemistry 71, 339–351. doi:10.1007/s10533-004-0058-5
Xu, H., Yu, G., Yang, L., and Jiang, H. (2013). Combination of Two-Dimensional Correlation Spectroscopy and Parallel Factor Analysis to Characterize the Binding of Heavy Metals with DOM in lake Sediments. J. Hazard. Mater. 263, 412–421. doi:10.1016/j.jhazmat.2013.09.042
Yamashita, Y., and Jaffé, R. (2008). Characterizing the Interactions between Trace Metals and Dissolved Organic Matter Using Excitation−Emission Matrix and Parallel Factor Analysis. Environ. Sci. Technol. 42, 7374–7379. doi:10.1021/es801357h
Yang, Y.-J., Wang, B., Guo, X.-J., Zou, C.-W., and Tan, X.-D. (2019). Investigating Adsorption Performance of Heavy Metals onto Humic Acid from Sludge Using Fourier-Transform Infrared Combined with Two-Dimensional Correlation Spectroscopy. Environ. Sci. Pollut. Res. 26, 9842–9850. doi:10.1007/s11356-019-04445-2
Yao, X., Zhang, Y., Zhu, G., Qin, B., Feng, L., Cai, L., et al. (2011). Resolving the Variability of CDOM Fluorescence to Differentiate the Sources and Fate of DOM in Lake Taihu and its Tributaries. Chemosphere 82, 145–155. doi:10.1016/j.chemosphere.2010.10.049
Yu, G.-H., Wu, M.-J., Wei, G.-R., Luo, Y.-H., Ran, W., Wang, B.-R., et al. (2012). Binding of Organic Ligands with Al(III) in Dissolved Organic Matter from Soil: Implications for Soil Organic Carbon Storage. Environ. Sci. Technol. 46, 6102–6109. doi:10.1021/es3002212
Yuan, D.-H., Guo, X.-J., Wen, L., He, L.-S., Wang, J.-G., and Li, J.-Q. (2015). Detection of Copper (II) and Cadmium (II) Binding to Dissolved Organic Matter from Macrophyte Decomposition by Fluorescence Excitation-Emission Matrix Spectra Combined with Parallel Factor Analysis. Environ. Pollut. 204, 152–160. doi:10.1016/j.envpol.2015.04.030
Yue, L., Li, L., and Hong, M. (2017). Research on Traditional Chinese Medicine in Dialectical Classification of Acne and Cosmetics Development. J. Light Ind. 32, 33–57. doi:10.3969/j.issn.2096-1553.2017.3.006
Zhang, C., Zhang, Z., Zhang, L., Zhang, H., Wang, Y., Hu, S., et al. (2020). Pyrolysis of Herb Waste: Effects of Extraction Pretreatment on Characteristics of Bio-Oil and Biochar. Biomass and Bioenergy 143, 105801. doi:10.1016/j.biombioe.2020.105801
Zhang, S., Chang, S., Xiao, P., Qiu, S., Ye, Y., Li, L., et al. (2019). Enzymatic In Situ Saccharification of Herbal Extraction Residue by a Medicinal Herbal-Tolerant Cellulase. Bioresour. Tech. 287, 121417. doi:10.1016/j.biortech.2019.121417
Keywords: herbal extraction residue, dissolved organic matter, Cu(II), PARAFAC, environmental risk
Citation: Yuan D, Cui Y, Kou Y, Guo X and Chen B (2022) Environmental Risk and Management of Herbal-Extraction Residues Induced by the Composition and Metal Binding Properties of DOM. Front. Environ. Sci. 10:856308. doi: 10.3389/fenvs.2022.856308
Received: 17 January 2022; Accepted: 08 March 2022;
Published: 06 April 2022.
Edited by:
Ping Wang, Beijing Forestry University, ChinaReviewed by:
Wenchao MA, The University of Tokyo, JapanChuanfu Zang, South China Normal University, China
Delin Fang, Beijing Normal University, China
Copyright © 2022 Yuan, Cui, Kou, Guo and Chen. This is an open-access article distributed under the terms of the Creative Commons Attribution License (CC BY). The use, distribution or reproduction in other forums is permitted, provided the original author(s) and the copyright owner(s) are credited and that the original publication in this journal is cited, in accordance with accepted academic practice. No use, distribution or reproduction is permitted which does not comply with these terms.
*Correspondence: Yingying Kou, a291eXlAYnVjZWEuZWR1LmNu