- 1College of Landscape Architecture and Art Design, Hunan Agricultural University, Changsha, China
- 2College of Horticulture, Hunan Agricultural University, Changsha, China
- 3Hunan Provincial Key Laboratory of Landscape Ecology and Planning & Design in Regular Higher Educational Institutions, Changsha, China
- 4State Key Laboratory of Urban and Regional Ecology, Research Center for Eco-Environmental Sciences, Chinese Academy of Sciences, Beijing, China
The local rise in urban temperature is increasingly exacerbated due to the combined effect of urban heat islands and global climate change. Numerous studies have shown that green roofs (GRs) have great potential for facilitating urban heat mitigation. However, little is known about whether such cooling effects can be achieved under extreme heat conditions. With the expected occurrence of more extreme heat events under climate change, such understanding is crucially important for the effective design of heat mitigation. This study aims to fill this gap by investigating the pedestrian-level cooling effect of GR under two weather conditions (i.e., typical summer weather conditions and extreme heat conditions). This research employed a three-dimensional simulation model, ENVI-met, to simulate pedestrian-level air temperature for three typical residential areas with different roof heights in Beijing. We conducted the simulations in two different roof scenarios, conventional roofs versus green roofs. The results showed that green roofs could provide large cooling exceeding 0.2°C on downwind sides and in the daytime, although the average cooling intensity was small. The pedestrian-level cooling intensity of GR decreased significantly under extreme heat conditions compared to typical summer weather conditions. It varied diurnally following an inverted W-shape for both weather conditions. Results also showed that the pedestrian-level cooling intensity of GR decreased with the increase in roof height in a nonlinear way and became 0 when roof height reached ∼50 m for both weather conditions. The results of our research can provide important insights for cooling-oriented urban design in the future, as we are expecting such extreme weather conditions nowadays may be the new normal in the future.
1 Introduction
The accelerating global warming significantly impacts cities where rapid urbanization is underway at the same time. For example, 68% of the population has been predicted to reside in urban areas by 2050 (UN, 2018). Among the many ecological challenges that cities face, urban heat island (UHI) is one of the most remarked (Francis and Jensen, 2017; Tian et al., 2021). UHI describes a well-known phenomenon that urban areas have higher temperature than the surrounding nonurban areas (Voogt and Oke, 2003; Shafique et al., 2018; Wang J. et al., 2019). Studies have shown that the higher urban temperature has adverse impacts, such as the alteration in species composition and distribution (Niemela, 1999; White et al., 2002), the increase in energy consumption (Castleton et al., 2010; Susca, 2019), and the increased mortality and heat-stress illness (Sailor and Fan, 2002; Knowlton et al., 2004). Thus, effective urban heat mitigation and adaptation strategies are highly desirable for a more comfortable urban thermal environment (Zhou et al., 2021; Wang et al., 2022).
Urban greening has been recognized as an effective means to alleviate extreme urban temperature (Zhou et al., 2011; Kong et al., 2014). However, although many cities have ambitious intentions to increase their urban vegetation, there are limited space and resources for urban planting, especially in cities with high density (Wang et al., 2022). Roofs, commonly accounting for approximately 20–25% of the total urban areas (Izquierdo et al., 2008; Besir and Cuce, 2018), can provide extra and optional surfaces and space for urban greening (Berardi, 2016). Although roofs are typically not close to pedestrians, green roofs (GR) were found to provide significant cooling effects (Shafique et al., 2018; Gao et al., 2019; Gao et al., 2020; Cristiano et al., 2021; Jamei et al., 2021; Liu H. Q. et al., 2021). Previous studies have shown that green roofs, especially intensive green roofs (IGR), can reduce air temperature at the pedestrian levels by 0.05°C in Paris, 0.1°C in Tokyo (Morakinyo et al., 2017), and 0.04°C in Berlin (Knaus and Haase, 2020). In addition, the pedestrian-level cooling effect of GR decreased with the increase in roof height (Ng et al., 2012; Herath et al., 2018; Jin et al., 2018; Zhang et al., 2019; Knaus and Haase, 2020), and tended to be zero when roofs were higher than a certain height, for example, 60 m (Ng et al., 2012).
Against global warming and rapid urbanization, extreme heat conditions, such as heatwaves, have become more frequent (IPCC, 2014; Cao et al., 2021). Urban greening can reduce urban temperature, but its cooling effect has been affected by weather conditions, such as air temperature (Wang et al., 2022). For example, the extremely high air temperature may lead to a decrease in the cooling effect of urban vegetation (Wang C. et al., 2019; Wang et al., 2020). The cooling effect of green roofs has been molded and revealed in most previous studies under typical summer conditions (Morakinyo et al., 2017; Susca, 2019). However, little is known about whether such cooling effects of GR can be achieved under extreme heat conditions (Klein and Coffman, 2015; Sun et al., 2016). Can GR work effectively under extreme heat conditions and therefore still provide a significant cooling effect at the pedestrian level? Is the change in cooling effects at the pedestrian level with roof height under extreme heat conditions similar to that under typical summer weather conditions? Addressing such questions can promote the understanding of the cooling effect of GR, mainly because such extreme heat conditions nowadays may be a new normal in the future (Mauree et al., 2019; Qi et al., 2021).
Here, we address these questions by simulating the pedestrian-level cooling effect of green roofs for three typical residential areas in Beijing. These three residential areas have different roof heights, thus representing the typical residential areas in the city. We employed the ENVI-met model to simulate the pedestrian-level air temperature for the selected residential areas in two scenarios: conventional and green roofs. We conducted the simulations under two weather conditions, including typical summer and extreme heat conditions. The objectives of this study were to 1) investigate the pedestrian-level cooling effect of green roofs under extreme heat conditions and 2) explore the change of such cooling effect along with roof height.
2 Materials and Methods
2.1 The Study Area
The three residential areas are located within the fifth ring road of Beijing, the capital of China (39°28′—41°25′N, 115°25′—117°30′E). Beijing has a monsoon-influenced humid continental climate characterized by hot, humid summers and cold, dry winters. The average annual temperature is 12.3°C, and the average annual precipitation, most of which occurs in summer, is 572 mm (Jiao et al., 2021). Beijing has a built-up area of 1,458 km2 and a population of 21.54 million people in 2019.
Three residential areas, namely Guan Yin Jing Yuan (GYJY), Yu Ze Yuan (YZY), and Jin Chan Nan Li (JCNL), have been selected for this study. Their building heights were 18, 27, and 42 m, respectively, representing three typical residential areas in terms of building height in Beijing and most of the cities in China: multi-story, mid-rise, and high-rise(Figure 1). More than 80% of the total number of residential areas in Beijing belong to such types (Zheng et al., 2017). Except for building height, other characteristics of the three residential areas are similar, including the area of residential neighborhoods, the area of roofs, and the percentage of green space on the ground (Supplementary Table A1). They also have the same type of vegetation, including Styphnolobium japonicum (L.) Schott and Platanus orientalis Linn., two typical trees in Beijing (Jiao et al., 2021). The landscape of these three residential areas is common in Beijing.
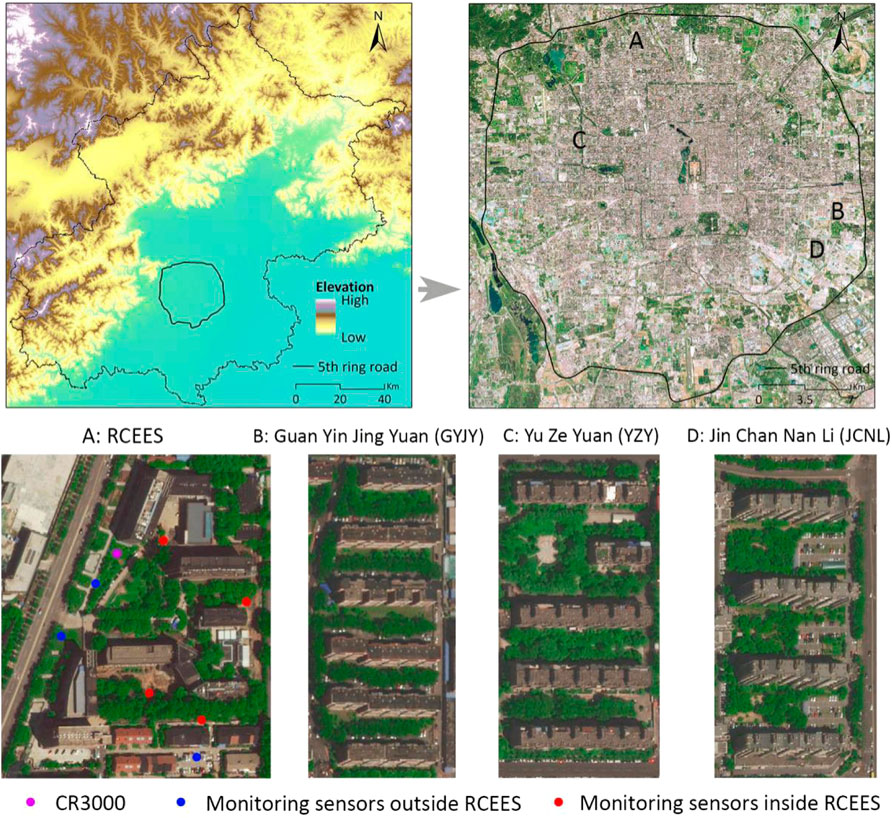
FIGURE 1. Locations and the land cover base map in the true color of these three study residential areas and RCEES where the validation of the ENVI-met model was applied.
2.2 ENVI-met Model Validation
ENVI-met, which is a three-dimensional microclimate model (Huttner and Bruse, 2009; Tsoka et al., 2018), has been adopted in this study to simulate the cooling effect of green roofs. The model can simulate the complex interactions between buildings, vegetation, soil, and atmosphere in the urban environment based on fundamental laws of fluid mechanics, thermodynamics, and atmospheric physics (Crank et al., 2018). It is a grid-based model with a simulation resolution that can be down to 0.5 m in space and 1s in time (Tsoka et al., 2018).
We first evaluate the validation of the ENVI-met (version 4.4.6) model in Beijing. The evaluation has been applied to Research Center for Eco-Environmental Sciences (RCEES) campus (Figure 1). The domain size of the RCEES campus is 212.5 m × 262.5 m × 96 m. The spatial resolution of the ENVI-met model was 2.5 m × 2.5 m × 3.0 m (size of x, y, and z grids). Additional nesting grids were added to reduce the effects of model bounders. Supplementary Table A2 shows the initial input conditions (e.g., wind speed, initial air temperature, etc.) of the ENVI-met model, which used hourly forcing (Salvati and Kolokotroni, 2019).
Air temperature and relative humidity of the three sensors outside the RCEES campus, and wind speed of the auto meteorological station were input as initial conditions (Figure 1) (Salvati and Kolokotroni, 2019). Air temperature and relative humidity were obtained from monitoring sensors, HOBO MX2301, ONSET, with the measurement accuracy of ±0.2°C for air temperature and ±2.5% for relative humidity. The wind speed was collected by CR3000 auto meteorological station with a measurement accuracy of ±0.5 m/s. The measurements and ENVI-met evaluations were carried out starting at 6:00 a.m. on 31 July 2021 and till 11:00 p.m. on 1 August 2021.
The meteorological data (i.e., air temperature and relative humidity at 1.5 m) measured at five sites inside the RCEES campus was used to evaluate the ENVI-met model (Figure 1). The root-mean-square error (RMSE), mean absolute error (MAE), mean bias error (MBE), the index of agreement d, and the coefficient of determination R2 were used to evaluate the accuracy of ENVI-met (Tsoka et al., 2018). The evaluation results were reported in Table 1, showing the high accuracy of the microclimate simulation of the ENVI-met model in Beijing. The simulated and measured results were highly correlated with R2, and d were larger than 0.87 and 0.88 for all cases, respectively. Moreover, RMSE, MAE, and MBE were below 1.7°C for all sensors.
2.3 Scenario Design
We then ran ENVI-met models for the three study residential areas in two roof scenarios and under two kinds of weather conditions. This study designed models using two roof scenarios, including the Base Model (BM) and the Green Roof Model (GRM). BM was built using conventional roofs, and GRM was built based on BM, adding intensive green roofs (IGR). Two typical trees were selected as the three-dimensional (3D) trees, and other plants (e.g., shrubs) were simulated as the simple two-dimensional (2D) plants in ENVI-met models. The parameters of green roofs: the hedge of leaf area index (LAI), the soil substrate layer depth, and the plant height were 2, 70 cm, and 1 m, respectively (Morakinyo et al., 2017). Other parameters, such as materials of buildings and roads, used values presented in (Chen et al., 2020), which was conducted in Beijing.
These simulations were conducted under two weather conditions, including typical summer and extreme heat conditions. We considered the weather conditions on 4 August 2020 as extreme heat conditions, with the highest air temperature of 37.2 °C, ranking the highest of the historical records of the latest 5 years in Beijing. The weather conditions on 1 August 2021 (i.e., the day of the model validation) were identified as the typical summer conditions, with the highest and average air temperatures of 32.7 and 29.3°C, respectively, very common in Beijing. The hourly wind speed, wind direction, air temperature, and relative humidity were input to create boundary conditions for the ENVI-met model, which used the simple forcing option (Supplementary Figure A1, Table 2). The model started at 6:00 a.m. on 3 August 2020 and at 6:00 a.m. on 31 July 2021. Although the models lasted 42 h, only the results of the last 24 h (i.e., 4 August 2020 and 1 August 2021) were used for the analysis because ENVI-met usually required a long initialization period (i.e., spin-up time) to obtain accurate simulation results (Sinsel et al., 2021).
2.4 Data Analysis
We then compared the two scenario models to derive the pedestrian-level air temperature difference between BM and GRM at each grid and every hour in three residential areas. Such temperature reduction due to green roofs was pedestrian-level cooling intensity. We used pedestrian-level cooling intensity to quantify the cooling effect of green roofs. We finally revealed the variations of the pedestrian-level cooling intensity with roof heights (i.e., building height). The polynomial function fitted the relationships between the pedestrian-level cooling intensity and roof height at multiple hours under two weather conditions.
3 Results
3.1 The Cooling Effect of Green Roofs
The cooling intensity has significant spatiotemporal heterogeneity, especially showing large values on downwind sides and in the daytime (e.g., from 10:00 a.m. to 5:00 p.m.), although its average ones were small. Green roofs can provide large cooling on downwind sides, while the small ones were generally found on the upwind sides (Figure 2). For example, the cooling intensity at 4:00 p.m. on the downwind side can be larger than 0.1 °C but be zero on the upwind side (Figure 2). The cooling intensity of green roofs in three residential areas varied significantly diurnally in a similar inverted W-shaped way (Figure 3). The cooling intensity started to increase from 6:00 a.m., when the Sun rose, till it peaked between 10:00 a.m. and 1:00 p.m. It decreased after the first peak to the valley around 2:00 p.m. and then increased to the second peak values between 3:00 p.m. and 5:00 p.m. It finally dropped till 8:00 p.m., the sunset time, and became relatively stable to a small value at night (Figure 3). For example, although the average cooling intensity was small (Figure 3), the largest cooling intensity can exceed 0.2°C at 10:00 a.m. in GYJY (Supplementary Table A3).
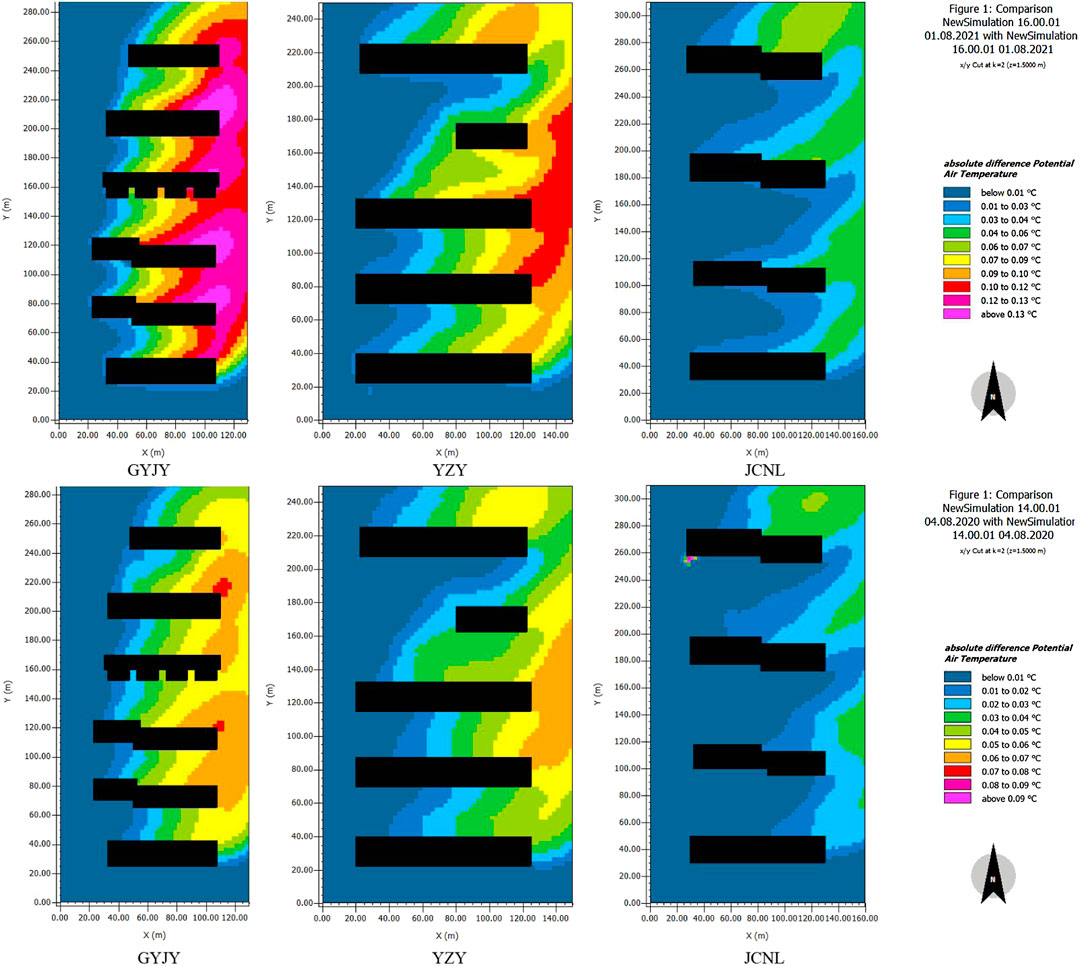
FIGURE 2. The spatial distribution of pedestrian-level cooling intensity of green roofs at the time when the maximum air temperature of the day is reached. They were 4:00 p.m. on 1 August 2021 (Top) and 2:00 p.m. on 4 August 2020 (Bottom).
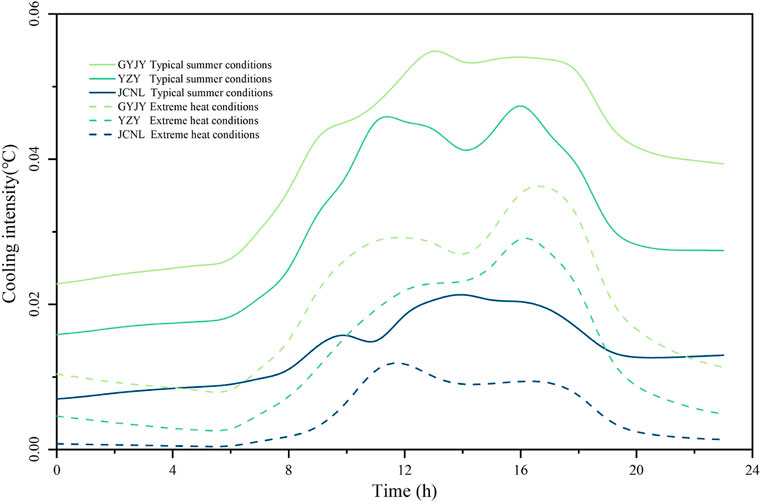
FIGURE 3. Diurnal variation of pedestrian-level cooling intensity of green roofs for three study residential areas under two weather conditions.
Results showed that the cooling effect of green roofs has decreased due to extreme heat conditions. The average pedestrian-level cooling intensity in GYJY, YZY, and JCNL was 0.02 , 0.01, and 0.00°C, respectively, under extreme heat conditions, much smaller than those which were 0.04 , 0.03, and 0.01°C, respectively, under the typical summer conditions (p < 0.01). However, the spatial heterogeneity of the pedestrian-level cooling intensity of green roofs showed similarity under two weather conditions, and so did the diurnal variations of cooling intensity (Figure 2, Figure 3).
In addition, the different levels of pedestrian-level cooling intensity have the same inverted W-shaped diurnal variations. According to each pixel’s averaged pedestrian-level cooling intensities, we divided the cooling effect of GR into different levels. Each level has an interval of 0.02 and 0.01°C of averaged cooling intensities under typical and extreme heat conditions, respectively. The high levels of cooling intensity, for example, the averaged cooling intensity with an interval of 0.12–0.14°C, suggested the large cooling that GR could provide. The diurnal variations of different levels were similar to an inverted W-shape (Supplementary Figure A2). The higher levels of the pedestrian-level cooling intensities reached the first peak earlier than the lower ones, but they reached the valleys and the second peaks almost simultaneously. Thus the higher levels not only reached the large values of cooling intensities earlier but lasted a longer time. This result indicated that their cooling lasted long in locations that achieved high cooling from green roofs. For example, the higher level of cooling intensity with an interval of >0.06 °C and the lower level of cooling intensity with an interval of 0.01–0.02 °C peaked at 10:00 a.m. and 1:00 p.m., respectively, in GYJY. Their second peak occurred at 5:00 p.m. It suggested that such large cooling can keep a long time in locations that achieve cooling greater than 0.06°C under extreme heat conditions (Supplementary Figure A2). Similar results were found in other residential areas and under two kinds of weather conditions.
3.2 Relationship Between Cooling Effect and Roof Height
The pedestrian-level cooling intensity of green roofs decreased with the roof height under two weather conditions, indicating that green roofs built on the high buildings would provide a low cooling effect at the pedestrian levels. For example, the average daytime pedestrian-level cooling intensities in GYJY, YZY, and JCNL with the building height of 18, 27, and 42 m were 0.05°C, 0.04°C, and 0.02°C, respectively, under typical summer conditions (Figure 4). Similarly, the average daytime cooling intensities under extreme heat conditions in these three residential areas were 0.03 , 0.03, and 0.01°C, showing a nonlinear decreasing trend.
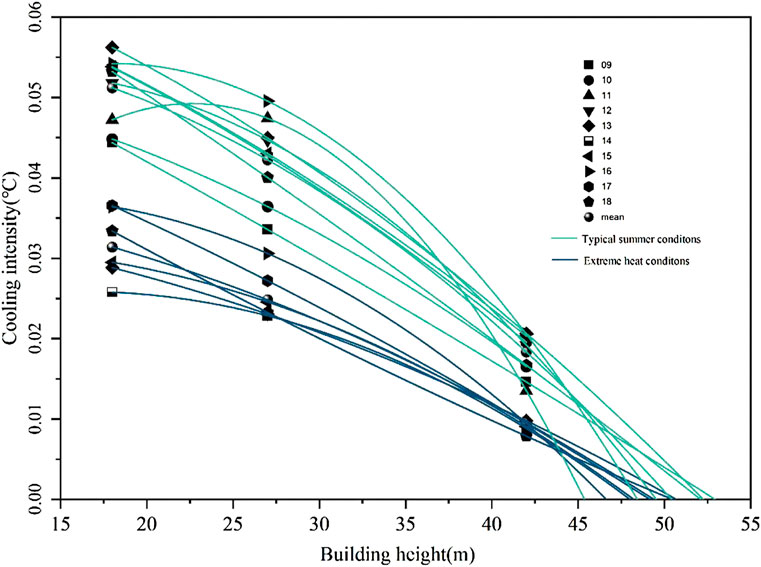
FIGURE 4. Relationship between pedestrian-level cooling intensity and the roof height (or building height). A polynomial function fitted the relationships. Points with different shapes represent the pedestrian-level cooling intensity at different times, and the color of the lines represents two weather conditions.
There was an approximate threshold of roof height of 46–53 m, at which pedestrian-level cooling intensities of green roofs have been predicted to be zero (Figure 4), regardless of weather conditions. Although the cooling effect of green roofs significantly decreased under extreme conditions, its variations with roof height remain the same compared to results under typical summer conditions (Figure 4). For example, under typical summer conditions, GR built on the roofs of 18, 27, and 42 m can provide air temperature reduction of 0.05°C, 0.05°C, and 0.02°C at 4:00 p.m., but lost cooling effect when it was built on the roofs of 49 m. Similar results were found under extreme heat conditions, and the maximum roof height was 47 m. Overall, the pedestrian-level cooling intensity of green roofs on buildings higher than ∼50 m was predicted to be zero. It suggested that GR built on roofs higher than such threshold could not provide cooling at the pedestrian level under two weather conditions.
4 Discussion
4.1 The Effects of Weather Conditions on the Cooling Effect of Green Roofs
Our results underscore the significant reduction of the cooling effect of green roofs under extreme heat conditions. Similar to the results from previous studies conducted in Beijing (∼0.07°C) (Qiu et al., 2021), our results revealed the 0.02–0.05°C of the daytime pedestrian-level air temperature reduction under typical conditions. Although the average cooling intensity was small, we highlight the large air temperature reduction on the downwind sides and in the daytime (e.g., 10:00 a.m. to 5:00 p.m.). However, such air temperature reduction under extreme heat conditions would be much smaller. This study is the first time to reveal that the cooling effect of green roofs at local scales would be reduced under extreme heat conditions, and similar results have been found in previous studies which focused on urban vegetation planted on the ground (Wang et al., 2020).
In addition, the cooling effects of GR varied significantly diurnally, showing an inverted W-shaped way, similar to previous results (Liu et al., 2018; Simon et al., 2018). Such variations were largely related to the response of evapotranspiration to air temperature (McAdam and Brodribb, 2015; Gillner et al., 2017). Increasing air temperature can induce the stomata to open and thus enhance evapotranspiration (Meili et al., 2021). However, the excessive air temperature would lead to the stomata being closed to protect trees from water loss and thus a dramatic reduction in evapotranspiration (Zhou et al., 2017). This water-saving strategy is reflected in the vegetation model in ENVI-met. Trees try to optimize their carbon gain-water loss relations, which are less beneficial under the high air temperature (Liu et al., 2018). Thus vegetation model assumes the high stomatal resistance to save water after midday (Bruse, 2004). Therefore a significant reduction in the cooling effect under extreme heat conditions or at the highest air temperature has been found.
Increasing urban vegetation, including vegetation planted on the ground and the roofs, is still an effective nature-based solution (NBS) to improve the urban thermal environment (Kumar et al., 2021). However, we must be aware that the cooling effect of the two types of aforementioned vegetation would be reduced under extreme heat conditions (Andric et al., 2020; Wang et al., 2020). Such reduction would be a challenge in the future when the extreme heat conditions would be the new normal because urban vegetation cannot provide significant cooling then (Ward et al., 2016; Pascal et al., 2021). Thus ecological management of urban vegetation (e.g., green roofs) is highly desired and warrants future research to achieve significant air temperature reduction under extreme heat conditions. The ecological management, such as the selection of species (Jim, 2015; MacIvor et al., 2016; Eksi et al., 2017), the optimization of the landscape of urban vegetation (Perini and Magliocco, 2014), and the maintenance (e.g., irrigation) of urban vegetation (Costanzo et al., 2016; Chagolla-Aranda et al., 2017), has been expected to help urban vegetation to generate the significant cooling, especially under extreme heat conditions.
4.2 The Effects of Roof Height on the Cooling Effect of Green Roofs
The cooling effect of green roofs at the pedestrian level depends on the evapotranspiration of green roofs and the roof height (Liu Z. et al., 2021). The evapotranspiration of green roofs suggests the cooling capacity of GR, and it is affected by the weather conditions (e.g., air temperature) (Section 4.1) (Rayner et al., 2016; Gilabert et al., 2021). The nonlinear response of evapotranspiration cooling of GR to air temperature leads to a nonlinear relationship between the cooling effect and roof height. Such findings are similar to the results of previous studies (Peng and Jim, 2013; Zhang et al., 2019; Sinsel et al., 2021).
Meanwhile, the roof height would determine how much of such cooling can travel vertically to the pedestrian level (Morakinyo et al., 2017). In this study, especially under typical summer conditions, 46–53 m of roof height was the farthest distance that evaporated cooling can travel vertically. In other words, when the roofs of the building are higher than such threshold, the evaporated cooling of the green roofs cannot travel to the pedestrian level. Similarly, under extreme heat conditions, the evaporated cooling of green roofs was decreased, and could not travel to the pedestrian level unless the roof of the building was low. Thus, a lower building height threshold was found under extreme heat conditions.
However, such a building height threshold, nearly 17-storey of residential buildings, is similar during urban planning and management. Our studies suggested that buildings lower than ∼50 m (i.e., 17 stories) can be applied to plant on the roofs because evaporated cooling of green roofs can travel down to pedestrian levels to improve the urban thermal environment. This urban greening action can significantly promote the cooling effect in urban areas with limited urban green space on the ground (Lin et al., 2021). More than 97% (in number) and 95% (in the area) of the buildings in Beijing were included considering such a threshold (Zheng et al., 2017). Therefore, planting on most buildings in Beijing could reduce air temperature on the pedestrian levels and help improve the thermal environment in Beijing.
In addition, green roof planning requires the socio-ecological thinking of urban ecosystems, in which social and ecological benefits should be integrated (Zhou et al., 2021). Cooling-oriented urban green roof planning needs to consider both outdoor and indoor cooling. Outdoor cooling would improve the urban thermal comfort, especially at the pedestrian level and indoor cooling can reduce the energy resources (e.g., power consumption for air conditioners) used and further anthropogenic heat cities released from the buildings (Besir and Cuce, 2018). Meanwhile, urban planners should consider the cost of green roofs and the population achieving the benefits (Shafique et al., 2018). Although planting green roofs on the low buildings could deliver much cooling to the pedestrian level, they can provide indoor cooling for a small proportion of urban residents who live in low buildings. On the other hand, although green roofs on high buildings can benefit more people, they would cost more (Manso et al., 2021). The optimized roof height at which cooling and social benefits can be balanced to create social and ecological win-wins warrants future research. In addition, the heights of buildings in the urban areas are staggered, showing spatial heterogeneity (Zheng et al., 2017). How to layout the green roof at more coarse scales is also worthy of further research.
This study has some limitations. First, we ran ENVI-met models without model evaluation in study areas (i.e. three residential areas) because there were not enough meteorological data. We evaluated ENVI-met models referencing the observed data on the RCEES campus. The validation suggested that the ENVI-met model had successful applications in Beijing, as much of previous research proved (Wang and Zacharias, 2015; Wu and Chen, 2017; Chen et al., 2020), but evaluating models in all study areas would be desirable. Second, we conducted the analysis only focusing on the air temperature reduction at the pedestrian level. Integrating green roofs’ indoor and outdoor cooling effects would be interesting and warrant future research.
5 Conclusion
This study aimed to reveal whether green roofs can provide a significant cooling effect at pedestrian levels under extreme heat conditions and how such cooling effects varied along with roof height. Using ENVI-met model simulation, we found: 1) Green roofs can provide large cooling which can exceed 0.2°C on the downwind sides and at the daytimes, although the average cooling intensity was small. 2) The pedestrian-level cooling intensity of GR under extreme heat conditions was significantly lower than that under typical summer conditions. 3) These temperature reductions varied significantly diurnally in an inverted W-shaped way under both weather conditions. 4) Results also showed that the pedestrian-level cooling intensity of GR decreased with the increase in roof height in a nonlinear way and has been predicted to be 0 when roofs were higher than ∼50 m (i.e. 17 stories) under both two weather conditions. This study enhances the understanding of the cooling effect of green roofs under extreme heat conditions. It can provide important insight for future cooling-oriented urban green roof planning.
Data Availability Statement
The original contributions presented in the study are included in the article/Supplementary Material; further inquiries can be directed to the corresponding authors.
Author Contributions
Conceptualization: YF, JW, and WZ; formal analysis: YF, JW, and XL; writing—original draft preparation: YF, JW, and WZ; and writing—review and editing: JW and XY.
Funding
This research was funded by the National Natural Science Foundation of China (Grant No. 32001160, No. 32001161), the Education Department of Hunan Province (Grant No. 20B297), and the Science and Technology Bureau, Changsha (kq2202227).
Conflict of Interest
The authors declare that the research was conducted in the absence of any commercial or financial relationships that could be construed as a potential conflict of interest.
Publisher’s Note
All claims expressed in this article are solely those of the authors and do not necessarily represent those of their affiliated organizations, or those of the publisher, the editors, and the reviewers. Any product that may be evaluated in this article, or claim that may be made by its manufacturer, is not guaranteed or endorsed by the publisher.
Supplementary Material
The Supplementary Material for this article can be found online at: https://www.frontiersin.org/articles/10.3389/fenvs.2022.874614/full#supplementary-material
References
Andric, I., Kamal, A., and Al-Ghamdi, S. G. (2020). Efficiency of Green Roofs and Green Walls as Climate Change Mitigation Measures in Extremely Hot and Dry Climate: Case Study of Qatar. Energy Rep. 6, 2476–2489. doi:10.1016/j.egyr.2020.09.006
Berardi, U. (2016). The Outdoor Microclimate Benefits and Energy Saving Resulting from Green Roofs Retrofits. Energy Build. 121, 217–229. doi:10.1016/j.enbuild.2016.03.021
Besir, A. B., and Cuce, E. (2018). Green Roofs and Facades: A Comprehensive Review. Renew. Sustain. Energy Rev. 82, 915–939. doi:10.1016/j.rser.2017.09.106
Bruse, M. (2004). ENVI-met Implementation of the Jacobs A − Gs Model to Calculate the Stomata Conductance. [Online] Available at: http://www.envi-met.net/documents/new_a_gs.pdf (Accessed February 16th, 2004).
Cao, J., Zhou, W., Wang, J., Hu, X., Yu, W., Zheng, Z., et al. (2021). Significant Increase in Extreme Heat Events along an Urban-Rural Gradient. Landsc. Urban Plan. 215, 104210. doi:10.1016/j.landurbplan.2021.104210
Castleton, H. F., Stovin, V., Beck, S. B. M., and Davison, J. B. (2010). Green Roofs; Building Energy Savings and the Potential for Retrofit. Energy Build. 42 (10), 1582–1591. doi:10.1016/j.enbuild.2010.05.004
Chagolla-Aranda, M. A., Simá, E., Xamán, J., Álvarez, G., Hernández-Pérez, I., and Téllez-Velázquez, E. (2017). Effect of Irrigation on the Experimental Thermal Performance of a Green Roof in a Semi-warm Climate in Mexico. Energy Build. 154, 232–243. doi:10.1016/j.enbuild.2017.08.082
Chen, Y., Wu, J., Yu, K., and Wang, D. (2020). Evaluating the Impact of the Building Density and Height on the Block Surface Temperature. Build. Environ. 168, 106493. doi:10.1016/j.buildenv.2019.106493
Costanzo, V., Evola, G., and Marletta, L. (2016). Energy Savings in Buildings or UHI Mitigation? Comparison between Green Roofs and Cool Roofs. Energy Build. 114, 247–255. doi:10.1016/j.enbuild.2015.04.053
Crank, P. J., Sailor, D. J., Ban-Weiss, G., and Taleghani, M. (2018). Evaluating the ENVI-Met Microscale Model for Suitability in Analysis of Targeted Urban Heat Mitigation Strategies. Urban Clim. 26, 188–197. doi:10.1016/j.uclim.2018.09.002
Cristiano, E., Deidda, R., and Viola, F. (2021). The Role of Green Roofs in Urban Water-Energy-Food-Ecosystem Nexus: A Review. Sci. Total Environ. 756, 143876. doi:10.1016/j.scitotenv.2020.143876
Eksi, M., Rowe, D. B., Wichman, I. S., and Andresen, J. A. (2017). Effect of Substrate Depth, Vegetation Type, and Season on Green Roof Thermal Properties. Energy Build. 145, 174–187. doi:10.1016/j.enbuild.2017.04.017
Francis, L. F. M., and Jensen, M. B. (2017). Benefits of Green Roofs: A Systematic Review of the Evidence for Three Ecosystem Services. Urban For. Urban Green. 28, 167–176. doi:10.1016/j.ufug.2017.10.015
Gao, M., Chen, F., Shen, H., Barlage, M., Li, H., Tan, Z., et al. (2019). Efficacy of Possible Strategies to Mitigate the Urban Heat Island Based on Urbanized High-Resolution Land Data Assimilation System (U-HRLDAS). J. Meteorol. Soc. Jpn. 97, 1075–1097. doi:10.2151/jmsj.2019-060
Gao, M., Chen, F., Shen, H., and Li, H. (2020). A Tale of Two Cities: Different Urban Heat Mitigation Efficacy with the Same Strategies. Theor. Appl. Climatol. 142, 1625–1640. doi:10.1007/s00704-020-03390-2
Gilabert, J., Ventura, S., Segura, R., Martilli, A., Badia, A., Llasat, C., et al. (2021). Abating Heat Waves in a Coastal Mediterranean City: What Can Cool Roofs and Vegetation Contribute? Urban Clim. 37, 100863. doi:10.1016/j.uclim.2021.100863
Gillner, S., Korn, S., Hofmann, M., and Roloff, A. (2017). Contrasting Strategies for Tree Species to Cope with Heat and Dry Conditions at Urban Sites. Urban Ecosyst. 20 (4), 853–865. doi:10.1007/s11252-016-0636-z
Herath, H. M. P. I. K., Halwatura, R. U., and Jayasinghe, G. Y. (2018). Evaluation of Green Infrastructure Effects on Tropical Sri Lankan Urban Context as an Urban Heat Island Adaptation Strategy. Urban For. Urban Green. 29, 212–222. doi:10.1016/j.ufug.2017.11.013
Huttner, S., and Bruse, M. (2009). “Numerical Modeling of the Urban Climate- a Preview on ENVI-Met 4.0,” in The seventh International Conference on Urban Climate ICUC-7, Yokohama, Japan, June 29–July 3, 2009.
IPCC (2014). Climate Change 2014: Synthesis Report. Contribution of Working Groups I, II and III to the Fifth Assessment Report of the Intergovernmental Panel on Climate Change. [Online] Available at: https://epic.awi.de/id/eprint/37530/ (Accessed November 1, 2014).
Izquierdo, S., Rodrigues, M., and Fueyo, N. (2008). A Method for Estimating the Geographical Distribution of the Available Roof Surface Area for Large-Scale Photovoltaic Energy-Potential Evaluations. Sol. Energy 82 (10), 929–939. doi:10.1016/j.solener.2008.03.007
Jamei, E., Chau, H. W., Seyedmahmoudian, M., and Stojcevski, A. (2021). Review on the Cooling Potential of Green Roofs in Different Climates. Sci. Total Environ. 791, 148407. doi:10.1016/j.scitotenv.2021.148407
Jiao, M., Xue, H. R., Yan, J. L., Zheng, Z., Wang, J., Zhao, C., et al. (2021). Tree Abundance, Diversity and Their Driving and Indicative Factors in Beijing’s Residential Areas. Ecol. Indic. 125, 107462. doi:10.1016/j.ecolind.2021.107462
Jim, C. Y. (2015). Assessing Climate-Adaptation Effect of Extensive Tropical Green Roofs in Cities. Landsc. Urban Plan. 138, 54–70. doi:10.1016/j.landurbplan.2015.02.014
Jin, C., Bai, X., Luo, T., and Zou, M. (2018). Effects of Green Roofs' Variations on the Regional Thermal Environment Using Measurements and Simulations in Chongqing, China. Urban For. Urban Green. 29, 223–237. doi:10.1016/j.ufug.2017.12.002
Klein, P. M., and Coffman, R. (2015). Establishment and Performance of an Experimental Green Roof under Extreme Climatic Conditions. Sci. Total Environ. 512-513, 82–93. doi:10.1016/j.scitotenv.2015.01.020
Knaus, M., and Haase, D. (2020). Green Roof Effects on Daytime Heat in a Prefabricated Residential Neighbourhood in Berlin, Germany. Urban For. Urban Green. 53, 126738. doi:10.1016/j.ufug.2020.126738
Knowlton, K., Rosenthal, J. E., Hogrefe, C., Lynn, B., Gaffin, S., Goldberg, R., et al. (2004). Assessing Ozone-Related Health Impacts under a Changing Climate. Environ. Health Perspect. 112 (15), 1557–1563. doi:10.1289/ehp.7163
Kong, F., Yin, H., Wang, C., Cavan, G., and James, P. (2014). A Satellite Image-Based Analysis of Factors Contributing to the Green-Space Cool Island Intensity on a City Scale. Urban For. Urban Green. 13 (4), 846–853. doi:10.1016/j.ufug.2014.09.009
Kumar, P., Debele, S. E., Sahani, J., Rawat, N., Marti-Cardona, B., Alfieri, S. M., et al. (2021). Nature-based Solutions Efficiency Evaluation against Natural Hazards: Modelling Methods, Advantages and Limitations. Sci. Total Environ. 784, 147058. doi:10.1016/j.scitotenv.2021.147058
Lin, M., Dong, J., Jones, L., Liu, J., Lin, T., Zuo, J., et al. (2021). Modeling Green Roofs' Cooling Effect in High-Density Urban Areas Based on Law of Diminishing Marginal Utility of the Cooling Efficiency: A Case Study of Xiamen Island, China. J. Clean. Prod. 316, 128277. doi:10.1016/j.jclepro.2021.128277
Liu, H. Q., Kong, F. H., Yin, H. W., Middel, A., Zheng, X. D., Huang, J., et al. (2021a). Impacts of Green Roofs on Water, Temperature, and Air Quality: A Bibliometric Review. Build. Environ. 196, 107794. doi:10.1016/j.buildenv.2021.107794
Liu, Z., Cheng, W., Jim, C. Y., Morakinyo, T. E., Shi, Y., and Ng, E. (2021b). Heat Mitigation Benefits of Urban Green and Blue Infrastructures: A Systematic Review of Modeling Techniques, Validation and Scenario Simulation in ENVI-Met V4. Build. Environ. 200, 107939. doi:10.1016/j.buildenv.2021.107939
Liu, Z. X., Zheng, S. L., and Zhao, L. H. (2018). Evaluation of the ENVI-Met Vegetation Model of Four Common Tree Species in a Subtropical Hot-Humid Area. Atmosphere 9 (5), 198. doi:10.3390/atmos9050198
MacIvor, J. S., Margolis, L., Perotto, M., and Drake, J. A. P. (2016). Air Temperature Cooling by Extensive Green Roofs in Toronto Canada. Ecol. Eng. 95, 36–42. doi:10.1016/j.ecoleng.2016.06.050
Manso, M., Teotónio, I., Silva, C. M., and Cruz, C. O. (2021). Green Roof and Green Wall Benefits and Costs: A Review of the Quantitative Evidence. Renew. Sustain. Energy Rev. 135, 110111. doi:10.1016/j.rser.2020.110111
Mauree, D., Naboni, E., Coccolo, S., Perera, A. T. D., Nik, V. M., and Scartezzini, J.-L. (2019). A Review of Assessment Methods for the Urban Environment and its Energy Sustainability to Guarantee Climate Adaptation of Future Cities. Renew. Sustain. Energy Rev. 112, 733–746. doi:10.1016/j.rser.2019.06.005
McAdam, S. A. M., and Brodribb, T. J. (2015). The Evolution of Mechanisms Driving the Stomatal Response to Vapor Pressure Deficit. Plant Physiol. 167 (3), 833–843. doi:10.1104/pp.114.252940
Meili, N., Manoli, G., Burlando, P., Carmeliet, J., Chow, W. T. L., Coutts, A. M., et al. (2021). Tree Effects on Urban Microclimate: Diurnal, Seasonal, and Climatic Temperature Differences Explained by Separating Radiation, Evapotranspiration, and Roughness Effects. Urban For. Urban Green. 58, 126970. doi:10.1016/j.ufug.2020.126970
Morakinyo, T. E., Dahanayake, K. W. D. K. C., Ng, E., and Chow, C. L. (2017). Temperature and Cooling Demand Reduction by Green-Roof Types in Different Climates and Urban Densities: A Co-Simulation Parametric Study. Energy Build. 145, 226–237. doi:10.1016/j.enbuild.2017.03.066
Ng, E., Chen, L., Wang, Y., and Yuan, C. (2012). A Study on the Cooling Effects of Greening in a High-Density City: An Experience from Hong Kong. Build. Environ. 47, 256–271. doi:10.1016/j.buildenv.2011.07.014
Niemelä, J. (1999). Ecology and Urban Planning. Biodivers. Conservation 8 (1), 119–131. doi:10.1023/A:1008817325994
Pascal, M., Goria, S., Wagner, V., Sabastia, M., Guillet, A., Cordeau, E., et al. (2021). Greening is a Promising but Likely Insufficient Adaptation Strategy to Limit the Health Impacts of Extreme Heat. Environ. Int. 151, 106441. doi:10.1016/j.envint.2021.106441
Peng, L., and Jim, C. (2013). Green-Roof Effects on Neighborhood Microclimate and Human Thermal Sensation. Energies 6 (2), 598–618. doi:10.3390/en6020598
Perini, K., and Magliocco, A. (2014). Effects of Vegetation, Urban Density, Building Height, and Atmospheric Conditions on Local Temperatures and Thermal Comfort. Urban For. Urban Green. 13 (3), 495–506. doi:10.1016/j.ufug.2014.03.003
Qi, J., Ding, L., and Lim, S. (2021). Toward Cool Cities and Communities: A Sensitivity Analysis Method to Identify the Key Planning and Design Variables for Urban Heat Mitigation Techniques. Sustain. Cities Soc. 75, 103377. doi:10.1016/j.scs.2021.103377
Qiu, L., Zhang, H., Zhang, W., Lai, D., and Li, R. (2021). Effect of Existing Residential Renovation Strategies on Building Cooling Load: Cases in Three Chinese Cities. Energy Build. 253, 111548. doi:10.1016/j.enbuild.2021.111548
Rayner, J. P., Farrell, C., Raynor, K. J., Murphy, S. M., and Williams, N. S. G. (2016). Plant Establishment on a Green Roof under Extreme Hot and Dry Conditions: The Importance of Leaf Succulence in Plant Selection. Urban For. Urban Green. 15, 6–14. doi:10.1016/j.ufug.2015.11.004
Sailor, D. J., and Fan, H. (2002). Modeling the Diurnal Variability of Effective Albedo for Cities. Atmos. Environ. 36 (4), 713–725. doi:10.1016/S1352-2310(01)00452-6
Salvati, A., and Kolokotroni, M. (2019). “Microclimate Data for Building Energy Modelling: Study on ENVI-Met Forcing Data,” in Proceedings of the 16th IBPSA Conference, Rome, Italy, September 2–4, 2019. doi:10.26868/25222708.2019.210544
Shafique, M., Kim, R., and Rafiq, M. (2018). Green Roof Benefits, Opportunities and Challenges - A Review. Renew. Sustain. Energy Rev. 90, 757–773. doi:10.1016/j.rser.2018.04.006
Simon, H., Lindén, J., Hoffmann, D., Braun, P., Bruse, M., and Esper, J. (2018). Modeling Transpiration and Leaf Temperature of Urban Trees - A Case Study Evaluating the Microclimate Model ENVI-Met against Measurement Data. Landsc. Urban Plan. 174, 33–40. doi:10.1016/j.landurbplan.2018.03.003
Sinsel, T., Simon, H., Broadbent, A. M., Bruse, M., and Heusinger, J. (2021). Modeling the Outdoor Cooling Impact of Highly Radiative “Super Cool” Materials Applied on Roofs. Urban Clim. 38, 100898. doi:10.1016/j.uclim.2021.100898
Sun, T., Grimmond, C. S. B., and Ni, G.-H. (2016). How Do Green Roofs Mitigate Urban Thermal Stress under Heat Waves? J. Geophys. Res. Atmos. 121, 5320–5335. doi:10.1002/2016JD024873
Susca, T. (2019). Green Roofs to Reduce Building Energy Use? A Review on Key Structural Factors of Green Roofs and Their Effects on Urban Climate. Build. Environ. 162, 106273. doi:10.1016/j.buildenv.2019.106273
Tian, P., Li, J., Cao, L., Pu, R., Wang, Z., Zhang, H., et al. (2021). Assessing Spatiotemporal Characteristics of Urban Heat Islands from the Perspective of an Urban Expansion and Green Infrastructure. Sustain. Cities Soc. 74, 103208. doi:10.1016/j.scs.2021.103208
Tsoka, S., Tsikaloudaki, A., and Theodosiou, T. (2018). Analyzing the ENVI-Met Microclimate Model's Performance and Assessing Cool Materials and Urban Vegetation Applications-A Review. Sustain. Cities Soc. 43, 55–76. doi:10.1016/j.scs.2018.08.009
UN (2018). 2018 Revision of World Urbanization Prospects. Available at: https://www.un.org/development/desa/en/news/population/2018-revision-of-world-urbanization-prospects.html (Accessed May 16, 2018).
Voogt, J. A., and Oke, T. R. (2003). Thermal Remote Sensing of Urban Climates. Remote Sens. Environ. 86 (3), 370–384. doi:10.1016/S0034-4257(03)00079-8
Wang, C., Wang, Z.-H., Wang, C., and Myint, S. W. (2019a). Environmental Cooling provided by Urban Trees under Extreme Heat and Cold Waves in U.S. Cities. Remote Sens. Environ. 227, 28–43. doi:10.1016/j.rse.2019.03.024
Wang, J., Zhou, W., and Jiao, M. (2022). Location Matters: Planting Urban Trees in the Right Places Improves Cooling. Front. Ecol Environ 20, 147–151. doi:10.1002/fee.2455
Wang, J., Zhou, W., Jiao, M., Zheng, Z., Ren, T., and Zhang, Q. (2020). Significant Effects of Ecological Context on Urban Trees' Cooling Efficiency. ISPRS J. Photogramm. Remote Sens. 159, 78–89. doi:10.1016/j.isprsjprs.2019.11.001
Wang, J., Zhou, W., and Wang, J. (2019b). Time-Series Analysis Reveals Intensified Urban Heat Island Effects but without Significant Urban Warming. Remote Sens. 11, 2229. doi:10.3390/rs11192229
Wang, Y., and Zacharias, J. (2015). Landscape Modification for Ambient Environmental Improvement in Central Business Districts – A Case From Beijing. Urban For. Urban Green. 14, 8–18.
Ward, K., Lauf, S., Kleinschmit, B., and Endlicher, W. (2016). Heat Waves and Urban Heat Islands in Europe: A Review of Relevant Drivers. Sci. Total Environ. 569-570, 527–539. doi:10.1016/j.scitotenv.2016.06.119
White, M. A., Nemani, R. R., Thornton, P. E., and Running, S. W. (2002). Satellite Evidence of Phenological Differences between Urbanized and Rural Areas of the Eastern United States Deciduous Broadleaf Forest. Ecosystems 5 (3), 260–273. doi:10.1007/s10021-001-0070-8
Wu, Z., and Chen, L. (2017). Optimizing the Spatial Arrangement of Trees in Residential Neighborhoods for Better Cooling Effects: Integrating Modeling With in-situ Measurements. Landsc. Urban Plan. 167, 463–472.
Zhang, G., He, B.-J., Zhu, Z., and Dewancker, B. J. (2019). Impact of Morphological Characteristics of Green Roofs on Pedestrian Cooling in Subtropical Climates. Int. J. Environ. Res. Public Health 16, 179. doi:10.3390/ijerph16020179
Zheng, Z., Zhou, W., Wang, J., Hu, X., and Qian, Y. (2017). Sixty-Year Changes in Residential Landscapes in Beijing: A Perspective from Both the Horizontal (2D) and Vertical (3D) Dimensions. Remote Sens. 9 (10), 992. doi:10.3390/rs9100992
Zhou, W., Huang, G., and Cadenasso, M. L. (2011). Does Spatial Configuration Matter? Understanding the Effects of Land Cover Pattern on Land Surface Temperature in Urban Landscapes. Landsc. Urban Plan. 102 (1), 54–63. doi:10.1016/j.landurbplan.2011.03.009
Zhou, W., Huang, G., Pickett, S. T. A., Wang, J., Cadenasso, M. L., McPhearson, T., et al. (2021). Urban Tree Canopy Has Greater Cooling Effects in Socially Vulnerable Communities in the US. One Earth 4 (12), 1764–1775. doi:10.1016/j.oneear.2021.11.010
Keywords: urban heat island, green roofs, cooling effects, extreme heat, ENVI-met
Citation: Feng Y, Wang J, Zhou W, Li X and Yu X (2022) Evaluating the Cooling Performance of Green Roofs Under Extreme Heat Conditions. Front. Environ. Sci. 10:874614. doi: 10.3389/fenvs.2022.874614
Received: 12 February 2022; Accepted: 09 June 2022;
Published: 13 July 2022.
Edited by:
Chenghao Wang, Stanford University, United StatesReviewed by:
Jian Peng, Peking University, ChinaJike Chen, the Nanjing University of Information Science and Technology, China
Meiling Gao, Chang’an University, China
Copyright © 2022 Feng, Wang, Zhou, Li and Yu. This is an open-access article distributed under the terms of the Creative Commons Attribution License (CC BY). The use, distribution or reproduction in other forums is permitted, provided the original author(s) and the copyright owner(s) are credited and that the original publication in this journal is cited, in accordance with accepted academic practice. No use, distribution or reproduction is permitted which does not comply with these terms.
*Correspondence: Jia Wang, amlhd2FuZ0ByY2Vlcy5hYy5jbg==; Xiaoying Yu, eXV4aWFveWluZ0BodW5hdS5lZHUuY24=