- 1Ecology of Plants, Institut für Biologie, Freie Universität Berlin, Berlin, Germany
- 2Berlin-Brandenburg Institute of Advanced Biodiversity Research (BBIB), Berlin, Germany
Symbioses between plants and fungi are important in both promoting plant fitness and maintaining soil structure. The ways in which these relationships change across an urban gradient is subject to debate. Here we measured root colonisation including the presence of arbuscular mycorrhizal fungi, non-mycorrhizal fungi, and root hair presence. We found no evidence of changes in levels of arbuscular mycorrhizal fungal colonisation across an urban gradient, colonisation levels being driven instead by plant community. However, we did observe an increase in non-mycorrhizal fungal colonisation in association with increasing urbanity. Additionally, we observed an urban-related increase in root hair presence. Using principal component analysis we were able to provide strong evidence for these patterns being driven by an “urban syndrome”, rather than soil chemistry. Our findings have important implications for the wider understanding of abiotic stresses on fungal endophyte presence and shed light on the impacts of urbanity upon plant roots.
Introduction
We live in the midst of the anthropocene, an age defined by the profound impact of humanity upon the Earth. Urban centres are the pinnacles of human impact upon the planet and contain a concentration of global-change factors not seen elsewhere (heavy metal pollutants, salinity, nitrogen deposition, heat islands and altered rainfall, to name but a few; Ziska et al., 2003; Carreiro and Tripler, 2005). These landscapes are by no means solely human domains and their ecology has been the target for several decades of research, largely due to the wide array of ecosystem services that are provided by their non-human components (Luederitz et al., 2015). A general rule appears to be that urbanisation results in the loss of animal diversity and can either increase or decrease plant diversity depending on the intensity of urbanisation (McKinney, 2008). However, the basis of all plant, and consequently animal, diversity is the soil. Urban soils have variously been described as compressed (Yang and Zhang, 2011), polluted (Hanfi et al., 2020) and lacking aggregates (Whitehead et al., 2021), expressing increased pH (Asabere et al., 2018) and salinity (Dmuchowski et al., 2021). As such, the ability of symbiotic fungi to buffer the negative impacts of urbanity upon plant hosts makes them a vital component of maintaining urban greenspaces. Urban ecosystems have also been described as hotspots for biological invasions (Gaertner et al., 2017). Fungal symbionts play a variety of roles in plant invasions, with some evidence suggesting that invasive plants are more likely to be facultatively symbiotic than native competitors, or at least not dependent on specific symbionts (Vogelsang and Bever, 2009; Hempel et al., 2013) and plants limited to specific symbionts appear to have their invasive potential constrained (Catford et al., 2009). Additionally, some symbioses are thought to help native plants maintain a competitive advantage over invasive species (Pringle et al., 2009). These interactions appear to be spatially variable, with an apparent split between North America and Europe (Pringle et al., 2009).
One of the most globally significant groups of plant symbionts are the arbuscular mycorrhizal (AM) fungi, which have been demonstrated to not only boost plant fitness (Begum et al., 2019) but also play a key role in the formation and stabilisation of soil aggregates (Piotrowski et al., 2004; Leifheit et al., 2014; Morris et al., 2019). Given the importance of AM fungal symbionts, a substantial amount of effort has been put into understanding the factors that influence AM fungi-plant symbioses. Many of these factors are also known to be impacted by urbanisation. For example; elevated nitrogen (Lovett et al., 2000) and phosphorus (Zhang et al., 2001; Liu et al., 2016) concentrations have been demonstrated to both occur in urban ecosystems and result in a down-regulation of AM fungal symbioses by plants (Bonneau et al., 2013; Nouri et al., 2014). Alterations in soil pH have also been observed to alter AM fungal colonisation, with acidity appearing to limit AM fungal colonisation (Clark, 1997; Coughlan et al., 2000). Despite the influence of acid rain, the overwhelming levels of alkaline compounds provided by concrete dust generally result in a net-alkaline pH for urban soils (Newbound et al., 2010). This elevated pH reduces the availability of heavy metals and thus partially counteracts the high levels of heavy-metal contamination often observed at hotspots of anthropogenic activity (Xian and Shokohifard, 1989). AM fungal colonisation has been reported as being reduced in heavy-metal contaminated soils (Ferrol et al., 2016), although the role of heavy metals in the plant-AM fungi-soil system is likely to be complex, depending on the species involved. Indeed, some AM fungal species confer greater heavy metal tolerance to plants, whilst others can result in plants accumulating heavy metals (Ferrol et al., 2016).
Previous research has sought to explore the ways in which urban systems shape AM fungal diversity. Cousins et al. (2003) investigated AM fungal spore diversity across different land uses in Arizona and observed a shift in community composition between urban sites and agricultural sites. Research carried out in the same study system found that AM fungal colonisation of roots was significantly lower in urban desert preserves than in rural desert (Ontiveros-Valencia, 2009). A very thorough study on the same subject by Bainard et al. (2011) also found a similar pattern in Ontario. They lent significant weight to their conclusions by limiting their study to 26 tree species, of which 11 showed a significant reduction in colonisation in urban sites compared to rural sites. Wiseman and Wells (2005) also found a significantly higher level of AM fungal colonisation in Acer rubrum in forested sites compared to those in developed areas. However, a study in Michigan has reported no change in AM fungal colonisation rate in saplings across a gradient of urbanity (Tonn and Ibáñez, 2017).
AM fungi are, however, not the only fungi which colonise roots. There are a wide range of other fungal symbioses that occur, including ecto- and ericoid-mycorrhiza and an array of non-mycorrhizal symbioses, the most abundant of which are the dark septate endophytes. The position of these non-mycorrhizal fungi on the “parasitism-mutualism continuum” (Mandyam and Jumpponen, 2015) is varied and often hard to discern, with some evidence for it changing according to the species, or even life stage, of the host plant involved (Berthelot et al., 2019). The benefits to plants of these mutualistic non-mycorrhizal symbioses range from increased heavy metal tolerance to accelerated growth rate (Mandyam and Jumpponen, 2005). The position of a particular symbiosis on this continuum may also be impacted by the environment (Berthelot et al., 2017), but this is debated (Kia et al., 2018). Colonisation rates do, however, appear to vary significantly in response to environmental conditions (Rillig et al., 1998) and to be most common in abiotically stressed environments (Mandyam and Jumpponen, 2005).
Here, we carried out an assessment of AM fungal and non-AM fungal colonisation from grassland sites across an urbanity gradient in Berlin, Germany. Through limiting the study to one habitat type and using Principal Component Analysis to extract three major axes of variation from a substantial database of environmental data, we were able to isolate the impacts of an urban ‘syndrome’, here referred to as urbanity, upon root colonisation and morphology. By using a gradient of sites rather than a paired-sample design we were able to use linear statistical models and have a greater degree of control over covarying environmental variables.
Methods
Berlin is a temperate central European city with around 3.5 million inhabitants. It is surrounded by the significantly more rural state of Brandenburg. Berlin’s soil texture ranges from sand to sandy loam with a pH range of 4.1–7.5. Fifty-four grassland sites distributed across Berlin and into Brandenburg were sampled between 30th June and 13 August 2020 for this study. These 16 m2 sites were part of the CityScapeLabs research platform, a network of grassland sites situated along an urban-rural gradient (von der Lippe et al., 2020). Root samples were taken from three locales within each site. Sampling locales were selected which reflected the diversity of plants present in each site. A random selection of roots was taken from each locale. Samples were stored in paper bags before being dried at 60°C overnight. Samples were subsequently stored at 4°C until processing. Only roots that were springy and had an intact cortex and tissue structure were selected for further analysis. Samples were bleached for 30 min in KOH, washed, acidified with 1% HCL for 12 min, and stained using 0.05% Trypan Blue in lactoglycerol for 40 min (Boedijn, 1956). Bleaching, acidification and staining were all performed in a 90°C water bath. Roots were subsequently mounted on slides in lactoglycerol.
AM fungal colonisation was quantified under ×200 magnification in accordance with McGonigle et al. (1990). At ∼100 intersects per slide any AM fungal structure was recorded in a binary fashion (presence/absence), with additional binary counts of arbuscules, AM fungal vesicles and AM fungal coils. Non-AM fungal structures and root hairs were also recorded in the same way; we counted as non-AM fungal structures any fungal presence that was not attributable to AM fungi. Count data were used to calculate the percentage of presence for each variable.
Principal Component Analysis (PCA), performed using the ade4 package (Thioulouse et al., 2018) in R (R Core Team, 2021), was used to extract three main axes of variation from a pre-existing database of environmental data for the sampled sites (von der Lippe et al., 2020). These three axes of data were found to relate to three ecologically relevant syndromes. The PCA axis scores, the age of sites (a binary variable representing pre-vs. post-1945 establishment as a grassland), and the day of the year that sampling took place (to control for seasonal effects) were used to create 10 multiple linear regression models to explain each colonisation/root trait. Akaike model selection, performed using the AICcmodavg package (Mazerolle, 2020) in R, was then used to select the most appropriate model, taking into consideration the percentage of cumulative model weight. For a list of models included please see Supplementary Table S1.
A pre-existing dataset of plant communities was used to explore the impact of plant community composition on the traits measured in this study. This dataset consisted of the percentage-cover of 233 grassland plant species within each study site. Plant communities were plotted using non-metric multidimensional scaling (NMDS) with the root responses and the three PCA environmental axes plotted as contour lines, this was achieved using the vegan package (Oksanen et al., 2020) in R. These data were used to qualitatively assess any possible relationships between plant community, environmental variation and root traits. Responses which demonstrated relationships with plant community were selected for further investigation. A series of multiple linear regression models were created which included the axis scores of the correlating NMDS axis, which functioned as a proxy for plant community. Akaike model selection was then used to select the most appropriate multiple linear regression model.
Results
The PCA of environmental variables created three main axes of variation that could be categorised as 1) urbanity (Figure 1), 2) soil chemistry and water content, and 3) site connectivity and pH. For a full list of variable loadings see Supplementary Table S1. Akaike model selection found that the most appropriate model for each of the response variables varied. For the percentage of AM fungal presence, arbuscules, vesicles (log transformed) and coils (log transformed) a multiple linear regression model containing PCA axes one and two was found to be appropriate. However, for the percentage of non-AM fungal presence and root hairs a single linear regression using only PCA axis 1 as a predictor was a more appropriate model. For the full output of Akaike model selection please see Supplementary Table S3.
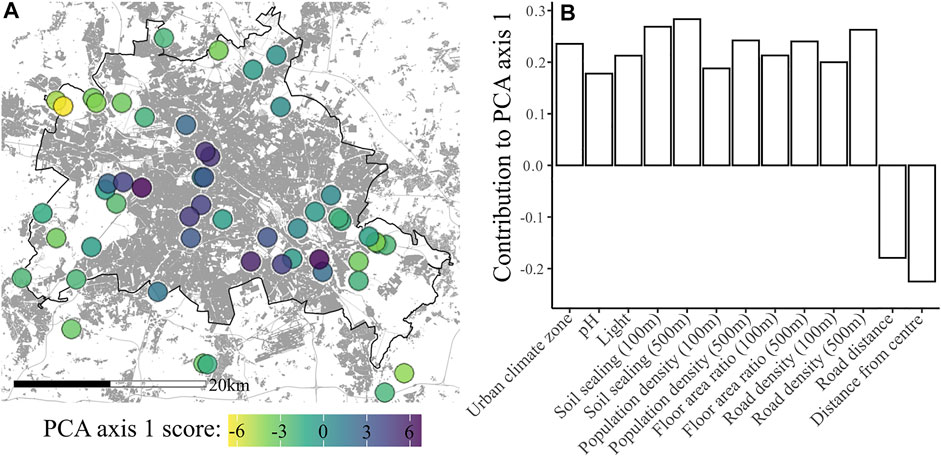
FIGURE 1. (A) Map of site locations. The colour of the point shows PCA axis one score, representing urbanity. The black line shows the border of Berlin and grey areas denote built up areas. (B) Major contributing variables to PCA axis 1, which can be categorised as an urban syndrome. For plots of all three of the main PCA axes please see Supplementary Figure S1, with biplots in Supplementary Figure S2.
We found that only two variables exhibited strong evidence for being driven by the environmental parameters included in their selected linear regression models. These variables were the presence of non-AM fungi and root hairs. Both of these responses showed a positive correlation with PCA axis one scores, meaning values increased at more urban sites (Figure 2). For the results of regression analyses please see Supplementary Table S4.
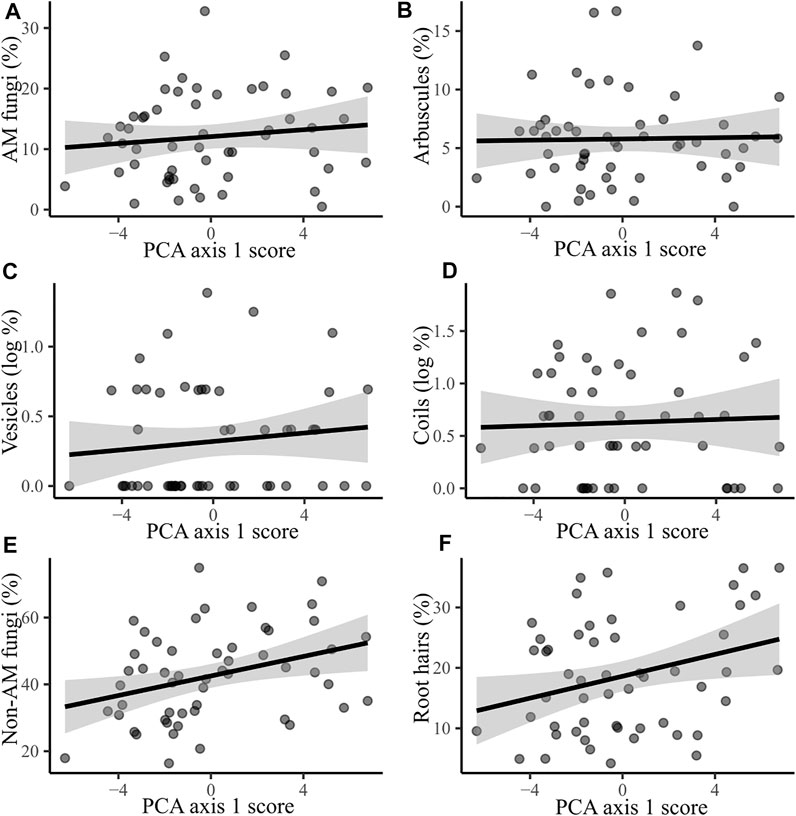
FIGURE 2. The correlation between PCA axis one score (representing urbanity) and the percentage of root locations containing (A) AM fungi; R2 = 0.016, F = 0.821, p = 0.369. (B) Arbuscules; R2 = 0.001, F = 0.026, p = 0.873. (C) Vesicles (log transformed); R2 = 0.015, F = 0.787, p = 0.379. (D) Coils (log transformed); R2 = 0.002, F = 0.088, p = 0.768. (E) Non-AM fungi; R2 = 0.117, F = 6.763, p = 0.012. (F) Root hairs; R2 = 0.094, F = 5.283, p = 0.026. Shaded areas represent 95% confidence intervals. For scatter plots of responses to all three PCA axes, see Supplementary Figure S3.
Through plotting plant communities using NMDS (Supplementary Figure S4), it was clear that plant community composition had a relationship with the levels of AM fungal, arbuscule and root hair presence. Plant communities did not appear to clearly shift with any of the environmental PCA axes although a trend with PCA axis 3, representing site connectivity, could be seen. Multiple linear regressions demonstrated that for AM fungi and arbuscules, presence was driven by plant community, whilst for root hairs the separate impacts of PCA axis one and plant community could not easily be disentangled, although there did not appear to be a significant interaction (Supplementary Table S6).
4 Discussion
We found strong evidence for AM fungi-plant interactions, including the presence of arbuscules, being resilient to the effects of urbanity, being shaped instead by plant community. Contrastingly, our results provide strong evidence for non-AM fungal interactions with plant roots increasing with urbanity. Specifically, our study suggests that this change is due to an intrinsic element of an urban syndrome, rather than just soil chemistry. Functional roles of these non-mycorrhizal fungi within the roots may have been varied. The non-AM fungal structures observed could have belonged to parasitic fungi, non-mycorrhizal mutualists, or AM fungi that were not exhibiting AM characteristics (Orchard et al., 2017). It is unlikely that they resulted from ectomycorrhiza, due to the absence of any distinguishable ectomycorrhizal structures being observed, or ericoid mycorrhiza, given the rarity of ericoid plants within Berlin’s grasslands. If the increase in non-AM fungi was due to a reduction in AM structures being exhibited by AM fungi, we would also expect to see a proportional reduction in observed AM structures, which we did not.
The direction of the relationship between observed non-AM fungal presence and urbanity is rather surprising, because almost all generally-accepted urban-related variables are thought to result in reduced levels of fungal activity. Indeed, many environmental parameters often thought indicative of urban systems were found to be contributors to PCA axes 2 and 3, rather than being collinear with variables such as population density and soil sealing in PCA axis 1. Heavy metals and increased pH (although pH also made a small contribution to PCA axis 1) are two such parameters, both of which are thought to negatively impact fungi in urban systems (Newbound et al., 2010). There are only a small number of urban-related parameters which may be expected to increase fungal activity, of these, the heat island effect is the only one that is represented in our dataset. The presence of an ‘urban climate zone’ parameter within PCA axis one makes temperature a strong candidate for being the cause of increased fungal activity. Additional factors not taken into account in this study such as the presence of animal faeces and remains have previously been demonstrated to increase fungal presence (Sagara, 1995; Watling, 1997; Newbound et al., 2010). Within our dataset graveyards accounted for some of the sites with highest PCA axis one scores, however, the sampling locations within these were not directly associated with graves and in one case was in a section of the graveyard that had been cleared for the creation of the Berlin wall. Site usage, such as dog walking, could also potentially be an explanation for altered fungal presence. However, the unimportance of soil chemistry in our study suggests that soil fertilisation via faecal deposition or decomposition in graveyards is an unlikely causal factor.
Through our use of PCA we were able to separate urbanity from soil chemistry. Our study provides evidence that we cannot solely rely on soil chemistry research to explain plant-fungal interactions in urban systems. Previous research from the same study system has suggested that urban soils have lower levels of aggregate stability (Whitehead et al., 2021), our current study suggests that a lack of AM fungi, previously described as key causal agents in aggregate formation (Lehmann et al., 2020), is likely not the leading cause of the reduction in aggregate stability in this system.
The increase in root hairs with increasing urbanity is a novel result which lacks any obvious explanation. It is surprising that such a trait, so clearly linked to the acquisition of nutrients, correlates with the PCA axis representing urbanity rather than that which represents soil chemistry. The trade-offs involved in root morphology are driven by a complex economy of carbon partitioning, growth rate and mycorrhizal colonisation (Bergmann et al., 2020). Within our study system however, there is no apparent trade-off between root hair presence and AM fungal colonisation, indeed, the only observable trend in fungal activity is collinear with root hair presence. One possible explanation for a change in root hair presence is a change in plant community, evidence for such an explanation can be seen in the NMDS plots in Supplementary Figure S4. However, whilst plant community does indeed appear to have a significant role in determining root hair presence, the lack of any interaction between plant community and PCA axis one suggests that this is likely not the decisive link between root hair presence and urbanity, although it cannot be ruled out.
Our findings suggest that an intrinsic element of urbanity has ecologically significant impacts on fungal presence in plant roots. This increase in non-AM fungal colonisation may be as a result of higher parasite load or an up-regulation of non-mycorrhizal mutualisms. We also found a general lack of significant response in AM fungi colonisation to urbanity, supporting the previous observations of Tonn and Ibáñez (2017). Our results for AM fungal colonisation share the lack of response to urbanity seen in ectomycorrhizal symbioses (Hui et al., 2017). The increase in root hair presence with urbanity is also interesting as there did not appear to be any trade-off with fungal colonisation.
Data Availability Statement
The datasets presented in this study can be found in online repositories. The names of the repository/repositories and accession number(s) can be found below: Figshare: https://doi.org/10.6084/m9.figshare.19248926.v1 and https://doi.org/10.6084/m9.figshare.19276481.v1.
Author Contributions
JW, SH, and MR designed the research. JW conducted fieldwork and lab analyses, and wrote the first draft of the paper. All authors added to and edited the text.
Funding
This work was funded by the German Federal Ministry of Education and Research (BMBF) within the collaborative Project “Bridging in Biodiversity Science (BIBS-phase 2)” (funding number 01LC1501A).
Conflict of Interest
The authors declare that the research was conducted in the absence of any commercial or financial relationships that could be construed as a potential conflict of interest.
Publisher’s Note
All claims expressed in this article are solely those of the authors and do not necessarily represent those of their affiliated organizations, or those of the publisher, the editors and the reviewers. Any product that may be evaluated in this article, or claim that may be made by its manufacturer, is not guaranteed or endorsed by the publisher.
Acknowledgments
We acknowledge support by the Open Access Publication Initiative of Freie Universität Berlin. We would like to thank Lena Fiechter, Moritz von der Lippe and Anne Hiller for providing the environmental database of the CityScapeLab sites. We thank Birgit Seitz for the plant community dataset and fu:stat for their advice on statistical analyses. We also thank our reviewers for their comments.
Supplementary Material
The Supplementary Material for this article can be found online at: https://www.frontiersin.org/articles/10.3389/fenvs.2022.888310/full#supplementary-material
References
Asabere, S. B., Zeppenfeld, T., Nketia, K. A., and Sauer, D. (2018). Urbanization Leads to Increases in pH, Carbonate, and Soil Organic Matter Stocks of Arable Soils of Kumasi, Ghana (West Africa). Front. Environ. Sci. 6, 17. doi:10.3389/fenvs.2018.00119
Bainard, L. D., Klironomos, J. N., and Gordon, A. M. (2011). The Mycorrhizal Status and Colonization of 26 Tree Species Growing in Urban and Rural Environments. Mycorrhiza 21 (2), 91–96. doi:10.1007/s00572-010-0314-6
Begum, N., Qin, C., Ahanger, M. A., Raza, S., Khan, M. I., Ashraf, M., et al. (2019). Role of Arbuscular Mycorrhizal Fungi in Plant Growth Regulation: Implications in Abiotic Stress Tolerance. Front. Plant Sci. 10, 1068. doi:10.3389/fpls.2019.01068
Bergmann, J., Weigelt, A., van Der Plas, F., Laughlin, D. C., Kuyper, T. W., Guerrero-Ramirez, N., et al. (2020). The Fungal Collaboration Gradient Dominates the Root Economics Space in Plants. Sci. Adv. 6 (27), 9. doi:10.1126/sciadv.aba3756
Berthelot, C., Blaudez, D., and Leyval, C. (2017). Differential Growth Promotion of poplar and Birch Inoculated with Three Dark Septate Endophytes in Two Trace Element-Contaminated Soils. Int. J. Phytoremed. 19 (12), 1118–1125. doi:10.1080/15226514.2017.1328392
Berthelot, C., Chalot, M., Leyval, C., and Blaudez, D. (2019). “From Darkness to Light: Emergence of the Mysterious Dark Septate Endophytes in Plant Growth Promotion and Stress Alleviation,” in Endophytes for a Growing World. Editors B. R. Murphy, F. M. Doohan, M. J. Saunders, and T. R. Hodkinson (Cambridge: Cambridge University Press), 143–164. doi:10.1017/9781108607667.008
Boedijn, K. B. (1956). Trypan Blue as a Stain for Fungi. Stain Techn. 31 (3), 115–116. doi:10.3109/10520295609113788
Bonneau, L., Huguet, S., Wipf, D., Pauly, N., and Truong, H. N. (2013). Combined Phosphate and Nitrogen Limitation Generates a Nutrient Stress Transcriptome Favorable for Arbuscular Mycorrhizal Symbiosis in M Edicago Truncatula. New Phytol. 199 (1), 188–202. doi:10.1111/nph.12234
Carreiro, M. M., and Tripler, C. E. (2005). Forest Remnants along Urban-Rural Gradients: Examining Their Potential for Global Change Research. Ecosystems 8 (5), 568–582. doi:10.1007/s10021-003-0172-6
Catford, J. A., Jansson, R., and Nilsson, C. (2009). Reducing Redundancy in Invasion Ecology by Integrating Hypotheses into a Single Theoretical Framework. Divers. Distributions 15 (1), 22–40. doi:10.1111/j.1472-4642.2008.00521.x
Clark, R. B. (1997). Arbuscular Mycorrhizal Adaptation, Spore Germination, Root Colonization, and Host Plant Growth and mineral Acquisition at Low pH. Plant Soil 192 (1), 15–22. doi:10.1023/a:1004218915413
Coughlan, A. P., Dalpé, Y., Lapointe, L., and Piché, Y. (2000). Soil pH-Induced Changes in Root Colonization, Diversity, and Reproduction of Symbiotic Arbuscular Mycorrhizal Fungi from Healthy and Declining maple Forests. Can. J. For. Res. 30 (10), 1543–1554. doi:10.1139/x00-090
Cousins, J. R., Hope, D., Gries, C., and Stutz, J. C. (2003). Preliminary Assessment of Arbuscular Mycorrhizal Fungal Diversity and Community Structure in an Urban Ecosystem. Mycorrhiza 13 (6), 319–326. doi:10.1007/s00572-003-0239-4
Dmuchowski, W., Baczewska-Dabrowska, A., Gozdowski, D., Bragoszewska, P., Gworek, B., Suwara, I., et al. (2021). Effect of Salt Stress in Urban Conditions on Two Acer Species with Different Sensitivity. PeerJ 9, 26. doi:10.7717/peerj.10577
Ferrol, N., Tamayo, E., and Vargas, P. (2016). The Heavy Metal Paradox in Arbuscular Mycorrhizas: from Mechanisms to Biotechnological Applications. J. Exp. Bot. 67 (22), 6253–6265. doi:10.1093/jxb/erw403
Gaertner, M., Wilson, J. R. U., Cadotte, M. W., MacIvor, J. S., Zenni, R. D., and Richardson, D. M. (2017). Non-native Species in Urban Environments: Patterns, Processes, Impacts and Challenges. Biol. Invasions 19 (12), 3461–3469. doi:10.1007/s10530-017-1598-7
Hanfi, M. Y., Mostafa, M. Y. A., and Zhukovsky, M. V. (2020). Heavy Metal Contamination in Urban Surface Sediments: Sources, Distribution, Contamination Control, and Remediation. Environ. Monit. Assess. 192 (1), 32. doi:10.1007/s10661-019-7947-5
Hempel, S., Götzenberger, L., Kühn, I., Michalski, S. G., Rillig, M. C., Zobel, M., et al. (2013). Mycorrhizas in the Central European flora: Relationships with Plant Life History Traits and Ecology. Ecology 94 (6), 1389–1399. doi:10.1890/12-1700.1
Hui, N., Jumpponen, A., Francini, G., Kotze, D. J., Liu, X., Romantschuk, M., et al. (2017). Soil Microbial Communities Are Shaped by Vegetation Type and Park Age in Cities under Cold Climate. Environ. Microbiol. 19 (3), 1281–1295. doi:10.1111/1462-2920.13660
Kia, S. H., Jurkechova, M., Glynou, K., Piepenbring, M., and Maciá-Vicente, J. G. (2018). The Effects of Fungal Root Endophytes on Plant Growth Are Stable along Gradients of Abiotic Habitat Conditions. FEMS Microbiol. Ecol. 94 (2), 10. doi:10.1093/femsec/fix162
Lehmann, A., Zheng, W., Ryo, M., Soutschek, K., Roy, J., Rongstock, R., et al. (2020). Fungal Traits Important for Soil Aggregation. Front. Microbiol. 10, 2904. doi:10.3389/fmicb.2019.02904
Leifheit, E. F., Veresoglou, S. D., Lehmann, A., Morris, E. K., and Rillig, M. C. (2014). Multiple Factors Influence the Role of Arbuscular Mycorrhizal Fungi in Soil Aggregation-A Meta-Analysis. Plant Soil 374 (1-2), 523–537. doi:10.1007/s11104-013-1899-2
Liu, W., Zhang, Y., Jiang, S., Deng, Y., Christie, P., Murray, P. J., et al. (2016). Arbuscular Mycorrhizal Fungi in Soil and Roots Respond Differently to Phosphorus Inputs in an Intensively Managed Calcareous Agricultural Soil. Sci. Rep. 6, 24902. doi:10.1038/srep24902
Lovett, G. M., Traynor, M. M., Pouyat, R. V., Carreiro, M. M., Zhu, W.-X., and Baxter, J. W. (2000). Atmospheric Deposition to Oak Forests along an Urban−Rural Gradient. Environ. Sci. Technol. 34 (20), 4294–4300. doi:10.1021/es001077q
Luederitz, C., Brink, E., Gralla, F., Hermelingmeier, V., Meyer, M., Niven, L., et al. (2015). A Review of Urban Ecosystem Services: Six Key Challenges for Future Research. Ecosystem Serv. 14, 98–112. doi:10.1016/j.ecoser.2015.05.001
Mandyam, K., and Jumpponen, A. (2005). Seeking the Elusive Function of the Root-Colonising Dark Septate Endophytic Fungi. Stud. Mycol. 53, 173–189. doi:10.3114/sim.53.1.173
Mandyam, K. G., and Jumpponen, A. (2015). Mutualism-parasitism Paradigm Synthesized from Results of Root-Endophyte Models. Front. Microbiol. 5, 776. doi:10.3389/fmicb.2014.00776
Mazerolle, M. J. (2020). AICcmodavg: Model Selection and Multimodel Inference Based on (Q)AIC(c). R Package Version 2.3-1. Available at: https://cran.r-project.org/package=AICcmodavg (Accessed January 28, 2022).
McGonigle, T. P., Miller, M. H., Evans, D. G., Fairchild, G. L., and Swan, J. A. (1990). A New Method Which Gives an Objective Measure of Colonization of Roots by Vesicular-Arbuscular Mycorrhizal Fungi. New Phytol. 115 (3), 495–501. doi:10.1111/j.1469-8137.1990.tb00476.x
McKinney, M. L. (2008). Effects of Urbanization on Species Richness: A Review of Plants and Animals. Urban Ecosyst. 11 (2), 161–176. doi:10.1007/s11252-007-0045-4
Morris, E. K., Morris, D. J. P., Vogt, S., Gleber, S.-C., Bigalke, M., Wilcke, W., et al. (2019). Visualizing the Dynamics of Soil Aggregation as Affected by Arbuscular Mycorrhizal Fungi. ISME J. 13 (7), 1639–1646. doi:10.1038/s41396-019-0369-0
Newbound, M., McCarthy, M. A., and Lebel, T. (2010). Fungi and the Urban Environment: A Review. Landscape Urban Plann. 96 (3), 138–145. doi:10.1016/j.landurbplan.2010.04.005
Nouri, E., Breuillin-Sessoms, F., Feller, U., and Reinhardt, D. (2014). Phosphorus and Nitrogen Regulate Arbuscular Mycorrhizal Symbiosis in Petunia Hybrida. Plos One 9 (3), 14. doi:10.1371/journal.pone.0090841
Oksanen, J., Guillaume, B. F., Friendly, M., Kindt, R., Legendre, P., McGlinn, D., et al. (2020). Vegan: Community Ecology Package. R Package Version 2.5-7. Available at: https://CRAN.R-project.org/package=vegan (Accessed August 18, 2021).
Ontiveros-Valencia, A. (2009). Arbuscular Mycorrhizal and Dark Septate Endophytic Fungi and Urban Preserves and Surrounding Sonoran Desert. Master’s Thesis. Tempe (AZ): University of Arizona.
Orchard, S., Standish, R. J., Dickie, I. A., Renton, M., Walker, C., Moot, D., et al. (2017). Fine Root Endophytes under Scrutiny: a Review of the Literature on Arbuscule-Producing Fungi Recently Suggested to Belong to the Mucoromycotina. Mycorrhiza 27 (7), 619–638. doi:10.1007/s00572-017-0782-z
Piotrowski, J. S., Denich, T., Klironomos, J. N., Graham, J. M., and Rillig, M. C. (2004). The Effects of Arbuscular Mycorrhizas on Soil Aggregation Depend on the Interaction between Plant and Fungal Species. New Phytol. 164 (2), 365–373. doi:10.1111/j.1469-8137.2004.01181.x
Pringle, A., Bever, J. D., Gardes, M., Parrent, J. L., Rillig, M. C., and Klironomos, J. N. (2009). Mycorrhizal Symbioses and Plant Invasions. Annu. Rev. Ecol. Evol. Syst. 40, 699–715. doi:10.1146/annurev.ecolsys.39.110707.173454
R Core Team (2021). R: A Language and Environment for Statistical Computing. Vienna, Austria: R Foundation for Statistical Computing. Available at: https://www.R-project.org/.
Rillig, M. C., Allen, M. F., Klironomos, J. N., Chiariello, N. R., and Field, C. B. (1998). Plant Species-specific Changes in Root-Inhabiting Fungi in a California Annual Grassland: Responses to Elevated CO 2 and Nutrients. Oecologia 113 (2), 252–259. doi:10.1007/s004420050376
Sagara, N. (1995). Association of Ectomycorrhizal Fungi with Decomposed Animal Wastes in forest Habitats - a Cleaning Symbiosis. Can. J. Botany 73, S1423–S1433. doi:10.1139/b95-406
Tonn, N., and Ibáñez, I. (2017). Plant-mycorrhizal Fungi Associations along an Urbanization Gradient: Implications for Tree Seedling Survival. Urban Ecosyst. 20 (4), 823–837. doi:10.1007/s11252-016-0630-5
Thioulouse, J., Dray, S., Dufour, A., Siberchicot, A., Jombart, T., and Pavoine, S. (2018). Multivariate Analysis of Ecological Data with ade4. Springer. doi:10.1007/978-1-4939-8850-1
Vogelsang, K. M., and Bever, J. D. (2009). Mycorrhizal Densities Decline in Association with Nonnative Plants and Contribute to Plant Invasion. Ecology 90 (2), 399–407. doi:10.1890/07-2144.1
von der Lippe, M., Buchholz, S., Hiller, A., Seitz, B., and Kowarik, I. (2020). CityScapeLab Berlin: A Research Platform for Untangling Urbanization Effects on Biodiversity. Sustainability 12 (6), 30. doi:10.3390/su12062565
Watling, R. (1997). Pulling the Threads Together: Habitat Diversity. Biodiver. Conserv. 6 (5), 753–763. doi:10.1023/a:1018374404998
Whitehead, J., Hempel, S., Hiller, A., von der Lippe, M., and Rillig, M. C. (2021). Soil Physico-Chemical Properties Change across an Urbanity Gradient in Berlin. Front. Environ. Sci. 9 (516), 765696. doi:10.3389/fenvs.2021.765696
Wiseman, P. E., and Wells, C. (2005). Soil Inoculum Potential and Arbuscular Ycorrhizal Colonization of Acer Rubrum in Forested and Developed Landscapes. J. Arboric. 31 (6), 296–302. doi:10.48044/jauf.2005.038
Xian, X. F., and Shokohifard, G. I. (1989). Effect of pH on Chemical Forms and Plant Availability of Cadmium, Zinc, and lead in Polluted Soils. Water Air Soil Pollut. 45 (3-4), 265–273. doi:10.1007/bf00283457
Yang, J.-L., and Zhang, G.-L. (2011). Water Infiltration in Urban Soils and its Effects on the Quantity and Quality of Runoff. J. Soils Sediments 11 (5), 751–761. doi:10.1007/s11368-011-0356-1
Zhang, G.-L., Burghardt, W., Lu, Y., and Gong, Z.-T. (2001). Phosphorus-enriched Soils of Urban and Suburban Nanjing and Their Effect on Groundwater Phosphorus. J. Plant Nutr. Soil Sci. 164 (3), 295–301. doi:10.1002/1522-2624(200106)164:3<295::aid-jpln295>3.0.co;2-t
Keywords: root endophytes, arbuscular mycorrhiza, urban ecology, root hairs, root colonisation
Citation: Whitehead J, Hempel S and Rillig MC (2022) Non-Mycorrhizal Fungal Presence Within Roots Increases Across an Urban Gradient in Berlin, Germany. Front. Environ. Sci. 10:888310. doi: 10.3389/fenvs.2022.888310
Received: 02 March 2022; Accepted: 15 March 2022;
Published: 14 April 2022.
Edited by:
Rosa Francaviglia, Council for Agricultural and Economics Research (CREA), ItalyReviewed by:
Erica Lumini, National Research Council (CNR), ItalyGiuseppe Badagliacca, Mediterranea University of Reggio Calabria, Italy
Copyright © 2022 Whitehead, Hempel and Rillig. This is an open-access article distributed under the terms of the Creative Commons Attribution License (CC BY). The use, distribution or reproduction in other forums is permitted, provided the original author(s) and the copyright owner(s) are credited and that the original publication in this journal is cited, in accordance with accepted academic practice. No use, distribution or reproduction is permitted which does not comply with these terms.
*Correspondence: Matthias C. Rillig, cmlsbGlnQHplZGF0LmZ1LWJlcmxpbi5kZQ==