- 1Faculty of Geography/IGEOG/LABMODEL, Rio de Janeiro State University-Uerj, Rio de Janeiro, Brazil
- 2Faculty of Biology/Department of Biophysics/LARAMG, Rio de Janeiro State University, Rio de Janeiro, Brazil
The Pantanal natural region in South America is the largest wetland worldwide. Due to floods and a diverse lithology, this wetland area exhibits high plant and animal biodiversity levels and encompasses more than 185,000 km2. In 2020, the Pantanal experienced the worst recorded fire episode in history. Hundreds of thousands of hectares burned, threatening habitats and corresponding ecosystem functions. The fire reached regions never burned before, including national conservation units. Although federal authorities have blamed climate change and cultural indigenous practices, environmentalists, scientists, and non-governmental organizations have questioned this foregone conclusion. To better understand this historical event, we examined the burn severity in conservation units most affected by fire and the association with human and climate factors (represented by the fire occurrence distance to roads/waterways/railways and drought severity, respectively) and analyzed fire spatial patterns and durations. Via a comparison of these two factors, we demonstrated that 60% of the fire outbreaks was concentrated at distances less than 5 km from roads, waterways, and railways and that 80% was concentrated at distances less than 10 km from areas with human activities. Since values of the SPEI (drought index) < −2.6 were critical to the spread of fire, a combination of more favorable climate conditions enabled the rapid and irreversible spread of fire. The observed association between fire occurrence and distance to roads makes the ongoing plans for road expansion of great concern, considering the 2020 fire episode, which greatly affected natural vegetation and conservation units across the Pantanal. The determination of trends in fire hot spot regions in the Pantanal can help environmental surveillance and fire control.
Introduction
Wetlands are one of the most important ecosystems in the world. Inland wetlands cover at least 9.5 million km2 (i.e. about 6.5% of the Earth’s land surface), Their ecological services provide an annual monetary value estimated at $4,879 × 109, or ∼981 to 44,597$/ha/year, which includes food production (cattle farming, agriculture, and fishing), recreation (recreative fishing and contemplative ecotourism), climate regulation (storage and sequestration of carbon), water supply and purification, sediment and nutrient retention, and habitat/refugia for biodiversity (Costanza et al., 1997; Russi et al., 2013).
The Pantanal is the largest wetland globally. This wetland is located at the centre of South America in western Brazil’s upper Paraguay River basin (Figure 1). The ecological and economic importance of preserving the Pantanal is unquestionable. Ecological services and economic activities are linked, and both activities depend on ecosystem functioning. A modelling study demonstrated that a notable increase in native vegetation loss in the Pantanal could increase soil erosion and sediment production and affect soil nutrient cycling, resulting in substantial negative impacts on not only biodiversity but also several economic activities, including agriculture and livestock (Guerra et al., 2020a). Hence, preservation policies are necessary.
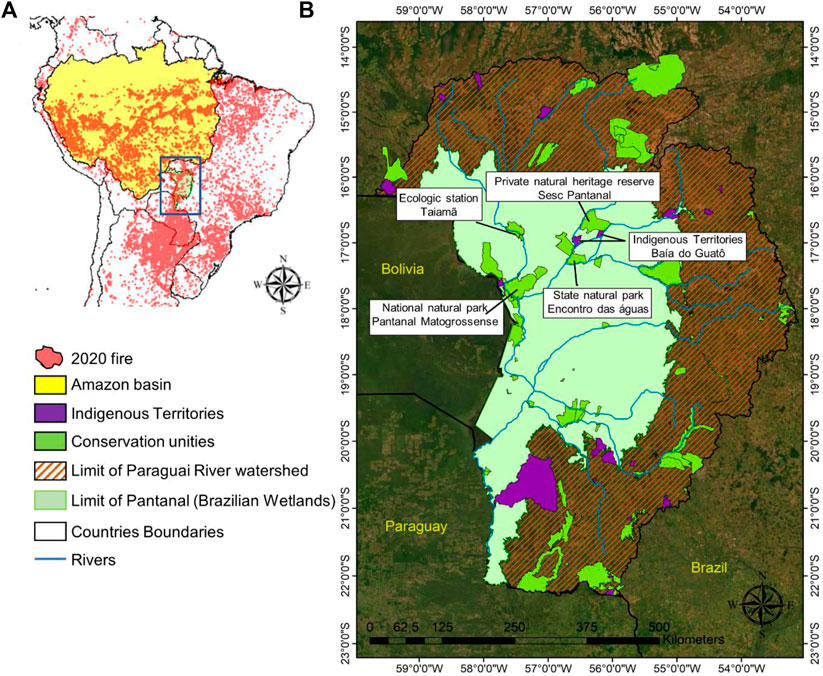
FIGURE 1. Map of the study area. (A) Location of the Brazilian Pantanal (blue rectangle) and 2020 fire distribution in South America. (B) Detailed map of the Brazilian Pantanal indicating the main conservation units affected by the 2020 wildfires.
In 1998, the Brazilian government ratified the Convention on Biological Diversity of the United Nations, which established the goal of protecting 10% of the Pantanal’s territory by 2010 by creating or expanding conservation units (Convention on Biological diversity, 2004). In 2000, the Pantanal was designated a World Heritage Site by the United Nations Education, Scientific, and Cultural Organization (UNESCO). However, currently, only 5.37% of the Pantanal’s territory is protected as conservation units (Chaves and Silva, 2018). Ranching and farming have spread across wetlands, resulting in deforestation and road expansion (Seidl et al., 2001). Furthermore, large infrastructure projects are currently under discussion or in the implementation phase in the Pantanal watershed, including 113 hydroelectric power plants (in the highlands of the upper Paraguay River basin), Paraguay River Waterway (Hydrovia), transoceanic roads, a railway and expansion of the Transpantaneira Highway (MT—060) (Tomas et al., 2019).
In addition to the expansion of human activities, the Pantanal ecosystem is threatened by climate change. Current predictive climate models indicate a progressive increase in the frequency of extreme events (e.g., extreme rainfall and extended droughts) (Marengo et al., 2015). By the end of the 21st century, annual mean warming ranging from 5 to 7 °C and a 30% reduction in precipitation are expected (Marengo et al., 2015). These climate changes can affect Pantanal ecosystem functioning, thereby amplifying and worsening the impact of human activities, including the spread of fire. In general, natural wildfires are part of ecosystem functioning and exert positive impacts on vegetation regeneration and nutrient cycling (Coutinho, 1990). However, human-induced fires occur in regions not adapted to fire and during periods of the year that fires would not naturally occur, leading to rapid fire spread and negative impacts (e.g., high emissions of greenhouse gases, damage to soil fertility, destruction of habitats and reduction in biodiversity).
Considering the timeline of human occupation within the region, data obtained at the Pontes e Lacerda site in the State of Mato Grosso, back to 7.5 kyr BP (Gouveia et al., 2002), reveal that small amounts of microcharcoal records considerably occurred, mostly indicating a near-zero flux, with a maximum value of 9.3–11.3 g of charcoal/10 kg of soil from approximately 2–3 kyr BP. This suggests that few fires occurred in the Pantanal in the past. Modern human activity in the region has altered local fire culture. The few well-dated regional sediment records north of the Pantanal region in the Mato Grosso State at the Alta Floresta site demonstrate that microcharcoal fluxes increased just after modern human colonization, i.e., after the mid-1970s (Cordeiro et al., 2014).
In 2020, the worst wildfire crisis occurred in the history of the Pantanal. This fire burned preserved habitats, including important conservation units. It is estimated that at least ∼17 million vertebrates were killed immediately by the fires in the Pantanal, as a consequence of the impact of such an event in the wetland (Tomas et al., 2021). From January 1 to December 27, the Brazilian National Institute for Space Research (INPE) (INPE - Instituto Nacional de Pesquisas Espaciais, 2021) detected 22,099 fire outbreaks across the Pantanal, 120% more than during the same period in 2019 and 270% more than the historical average from 2000–2019. These observations motivated a large debate in social, political and scientific layers concerning the unusual dry, hot weather conditions that had fueled the event, the role of lightining (Menezes et al., 2022) and the potential impact of human activities. Until now, it is not conclusive the effective role of recent heat waves that broke out during the 2020 climate extreme conditions (Libonati et al., 2022) and the human impact over the sensitivity of the Pantanal ecosystems to fire (Kumar et al., 2022). Although previous studies have well described the climate conditions prevalent during the 2020 severe drought period in the Pantanal (Marengo et al., 2021; Kumar et al., 2022; Libonati et al., 2022; Menezes et al., 2022), the cause of the 2020 fire event is not fully understood. In this work we explore the human activity component as a potential player to initiate/exacerbate the 2020 fire crisis in the Pantanal.
The objectives of this study are 1) to assess the expansion and severity of the 2020 wildfire in conservation units most affected by fire (northern/central-western part of the Pantanal), 2) to determine whether the wildfire crisis was caused by human activity or climate change and 3) to identify high-priority regions for fire management by analysing the spatial-temporal trend pattern.
Materials and Methods
Spatial Distribution of the Fire Outbreaks
We employed the fire database of the Brazilian Institute for Space Research (INPE; source: https://queimadas.dgi.inpe.br/queimadas/bdqueimadas) (INPE - Instituto Nacional de Pesquisas Espaciais, 2021) (which uses the collection six MODIS active fire detection algorithm (Giglio et al., 2016)) to assess the spatial distribution of fire outbreaks during the 2020 fire season (August-September-October) and compared the distribution to historical mean fire outbreaks in the Pantanal (data from 2000–2019). The INPE and NASA fires counts are indicators of the occurrence of fire in vegetation and allow temporal and spatial comparisons. however, they should not be considered as an absolute measure of the occurrence of fire. Some conditions prevent or greatly impact the detection of fires (e.g., Fire fronts with less than 30 m; Fire only on the floor of a dense forest, without affecting the canopy of the trees; Clouds covering the region; and short duration fire, occurring between the time of available images). For MODIS active fire, validation works indicate that the average error is ∼400 m, about 80% of the active fires are within a radius of 0.1 km from the indicated coordinates (Schroeder et al., 2008). The use of active fires has the advantage of detecting the daily location of fire, which can also indicate the local where the fire started. We created a 5x5-kilometre grid for the whole Pantanal and counted the number of fire outbreaks detected by the reference satellite in each grid. Fire outbreak maps were constructed in ArcGIS 10.6 software.
Remote Sensing of the Burn Severity in Conservation Units
We obtained Sentinel-2 Multispectral Instrument (MSI) images, with a spatial resolution of 10–20 m, to measure the extension and severity of fires in Pantanal conservation units the most damaged by fire, located in the northern/central-western part of the Pantanal. Sentinel two MSI images were retrieved from the European Space Agency (ESA) Copernicus Data Hub (https://scihub.copernicus.eu/dhus/#/home). Level 2 surface reflectance was considered, ensuring a correction of the atmospheric effect, terrain, view zenith angle, and solar angle. Pairs of acquisition dates were chosen to provide the best estimate of pre- and postfire conditions, minimizing differences in vegetation phenology and weather conditions. Cloud free images were used. Due to the extension of the area analysed and the need for cloud-free images, different acquisition dates were considered to build a mosaic (totalling four images) representing the pre- and postfire periods. All image processing was performed in Sentinel Application Program (SNAP) software. Table 1 lists the pairs of acquisition dates.
The burn severity was calculated based on the difference normalized burn ratio index (dNBR). The dNBR is a spectral index used for measuring and classifying the burn severity, achieving a high agreement with field measurements (Parks et al., 2014; Saulino et al., 2020). The dNBR is the difference between the normalized burn ratio index (NBR) for pre- and postfire images. The NBR can be calculated with Equation (1).
where NIR is the near-infrared wavelength and SWIR2 is the shortwave infrared wavelength of the MSI, which corresponds to band 8 (0.76–0.90 µm) and band 12 (2.07–2.32 µm), respectively. The dNBR was calculated by subtracting the postfire NBR from the prefire NBR (Equation 2).
The classification of the burn severity level was based on the dNBR values according to the United States Geological Survey (USGS) Fire Effects Monitoring and Inventory Protocol (FireMON) programme (Lutes et al., 2006), as listed in Table 2.
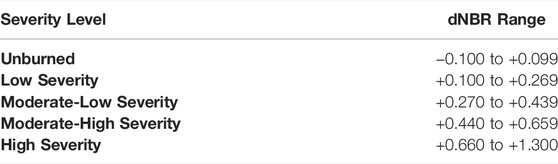
TABLE 2. Burn severity level classification, as proposed by the USGS (Bush et al., 2008).
2020 Climate Data
We used the standardized precipitation and evaporation index (SPEI) as a quantitative index to quantify the intensity of drought episodes in the Pantanal and compared the intensity of drought episodes to the interannual variability in fires. The SPEI is based on precipitation and temperature data, and this index yields the advantage of combining multiscalar characteristics with the capacity to include temperature variability effects in drought assessment. The procedure to calculate the index is detailed in Vincent-Serrano et al. (2010) (Vicente-Serrano et al., 2010) and involves a climatic water balance, the accumulation of a deficit/surplus at different time scales, and an adjustment into a log-logistic probability distribution. The SPEI combines the sensitivity of the well-known Palmer drought severity index (PDSI) to changes in the evapotranspiration demand (caused by temperature variability and trends) with the multitemporal nature of the standardized precipitation index (SPI). It has been pointed as more useful for climate change studies than PDSI and SPI (Lutes et al., 2006). Data were obtained from the SPEI global drought monitoring web page (https://spei.csic.es/map/maps.html#months=1#month=2#year=2021), and the data exhibited a 0.5°spatial resolution and a monthly time resolution. Interannual/monthly time series (spanning the period from January 1970 to December 2020) were extracted for the whole Pantanal Biome using ArcGIS version 10.6. We compared the SPEI time series to local hydrological and climatological parameters. Time series of the annual water discharge from the Ladário station (spanning 1945–2020) and Carcere station (spanning 1970–2018) in the southern and northern Pantanal (Paraguay River), respectively, were obtained from the Brazilian National Water Agency (ANA—Agência Nacional de Águas). Time series of the annual/monthly precipitation (spanning 2002–2020) was obtained as average data over nine precipitation stations in the Paraguay River basin. Data on water discharge and precipitation were obtained from the ANA web platform (http://www.snirh.gov.br/hidroweb/apresentacao).
Spatial Analysis of the Fire Outbreaks
Average nearest neighbour (ANN) analysis (Clark and Evans, 1954), implemented in ArcGIS version 10.6, was performed to assess the spatial distribution of the fire outbreaks. Average nearest neighbour analysis measures the distance between each data location (in our case, the location of the fire outbreaks) and the nearest neighbour location. Then, all these nearest neighbour distances are averaged. The average nearest neighbour ratio is calculated as the observed average distance divided by the expected average distance (with the expected average distance based on a hypothetical random distribution with the same number of features covering the same total area). If the index (average nearest neighbour ratio) is lower than 1, the pattern exhibits clustering. If the index is higher than 1, the trend indicates dispersion. The Z test was applied to indicate whether the observed average nearest neighbour distance is significantly different from the mean random distance.
The Euclidian distance between each fire outbreak and roads/waterways/railways was calculated in ArcGIS version 10.6. We also matched the spatial location of each fire outbreak and the corresponding drought index (SPEI) value. Roads data are from the Brazilian Institute of Geography and Statistics (IBGE) (source: https://www.ibge.gov.br/geociencias/downloads-geociencias.html). Waterways and railways data are from the Brazilian national ministry of infrastructure database (source: https://www.gov.br/infraestrutura/pt-br/assuntos/dados-de-transportes/bit). We used data from official government sources due to its recognize quality.
In addition, we employed the whole annual fire database (from 2000 to 2020) to identify spatial-temporal patterns of fire outbreak locations. We integrated spatial and temporal analysis, creating a space-time cube with fire outbreak location data. Consequently, we applied the Emerging Hot Spot Analysis (EHA) tool implemented in ArcGIS Pro version 2.5. The EHA tool adopts the space-time cube as input and conducts hot spot analysis with the Getis-Ord Gi* statistic in each individual bin. The hot and cold spot trends detected by the Getis-Ord Gi* statistic were evaluated with the Mann-Kendall test to determine whether these trends are persistent, increasing, or decreasing over time. A detailed description of emerging hot spot analysis has been provided by the ESRI (2016) (ESRI, 2016) and Harris et al. (2017) (Harris et al., 2017). In summary, the Getis-Ord test used in the EHA tool first examines the data in each aggregated bin, as well as the surrounding bins, and compares the data to the global average over the time step. If the EHA tool finds that the bin and its neighbours are significantly different from the global mean, then it classifies the bin as a hot or cold spot with an associated p value and Z score. These Z scores are then compared over time using the Mann-Kendall test to examine the temporal trends in the data. The Mann-Kendall test extracts each spatial bin into its own time series of Z scores. This time series is examined for changes between years. A higher value than that in the previous step assigns the time series a value of +1, while a lower value than that in the previous step assigns a value of −1. Then, the Mann-Kendall test determines if the sum is significantly different from the expected sum. The significance values from each test are then considered to classify each bin into one trend category. The ESRI (2016) (ESRI, 2016) provided detailed descriptions of the categories and how they are calculated. Here, in our results, we found three categories of Pantanal fire outbreak trends: 1) new hot spot, i.e., a location that was a statistically significant hot spot in the final time step and had never been a statistically significant hot spot before, namely, a location that was significantly burned for the first time in 2020; 2) consecutive hot spot, i.e., a location that had never been a statistically significant hot spot prior to the final hot spot run and fewer than 90% of all bins were statistically significant hot spots, namely, a location with few fire outbreaks over time but with the two last years, 2019 and 2020, exhibiting a significantly high increases in fire outbreaks; and 3) sporadic hot spot, i.e., a location with a high incidence of fire outbreaks over time, with certain years exhibiting a higher incidence and other years exhibiting a lower incidence of fires.
Land Use and Burned Area Data
Mapping of the land use and fire scars in the Pantanal, as well as in all Brazilian territories, was carried out based on the MapBiomas programme (https://mapbiomas.org/). The programme is a collaborative network formed by NGOs, universities, and technology companies composed of scientists and experts in Brazilian biomes, remote sensing, geographical information systems, and computer science.
Annual land use maps were produced with the random forest algorithm applied to the Landsat archive on the Google Earth Engine (Souza et al., 2020). Fire scar mapping was also based on Landsat image mosaics, with a spatial resolution of 30 m. The classification process was carried out on the Google Earth Engine and the Google Cloud Storage platform using a deep neural network algorithm (Arruda et al., 2021). The algorithm was trained using field data based on burned and unburned training samples. The following spectral bands were employed as remote sensing input data for the classification model: red (0.65 µm), near-infrared (0.86 µm), and shortwave infrared (SWIR 1–1.6 µm and SWIR 2–2.2 µm). In addition, the Landsat normalized difference vegetation index (NDVI) and dNBR were considered (Lutes et al., 2006). Reference maps of burned areas from the MODIS product (MCD64A1 - https://lpdaac.usgs.gov/products/mcd64a1v006/) with a 500-m spatial resolution and fire outbreak data from the INPE (https://queimadas.dgi.inpe.br/queimadas/bdqueimadas) were obtained for validation. The detailed method can be found in the Algorithm Theoretical Basis Document (ATBD) (https://mapbiomas-br-site.s3.amazonaws.com/Metodologia/FOGO/ATBD_-_MapBiomas_Fogo_-_Cole%C3%A7%C3%A3o_1_1.pdf).
An overview of the methodology considering the database used, analysis, results and relevant issues addressed throughout the work are presented in the Supplementary Figure S1.
Results and Discussion
Spatial Distribution of the 2020 Fire Outbreaks
First, we analysed the spatial distribution of the fire outbreaks in the Brazilian Pantanal during the 2020 fire season (August-September-October) and compared this to historical mean data from 2000–2019 to evaluate the actual dimensions. The historical mean (from 2000–2019) of the number of fire outbreaks ranged from 1 to 10 in a 5-km grid, and the outbreaks were concentrated in the southern, eastern, and northeastern parts of the Pantanal (Figure 2A). The spatial distribution of the wildfire outbreaks indicated that although most conservation units were surrounded by fire, they had never burned before 2000–2019 (Figure 2A). This result highlights the importance of establishing conservation units to protect the environment and ensure firefighting effectiveness. In 2020 (Figure 2B,C), the number of fire outbreaks ranged from 1 to 50 in a 5-km grid. In contrast to previous years, wildfires were more concentrated in the untouched northern/central-western part of the Pantanal along the Paraguay River margin. Different types of conservation units were affected, including the National Natural Park Pantanal Matogrossense (NNPPM), State Natural Park Encontro das Águas (SNPEA), Ecologic Station Taiamã (EST), Private Natural Heritage Reserve SESC Pantanal (SESCP), and Guatô indigenous territory (GIT). Fire also occurred in the southern Pantanal, although its spread was less wide-ranging.
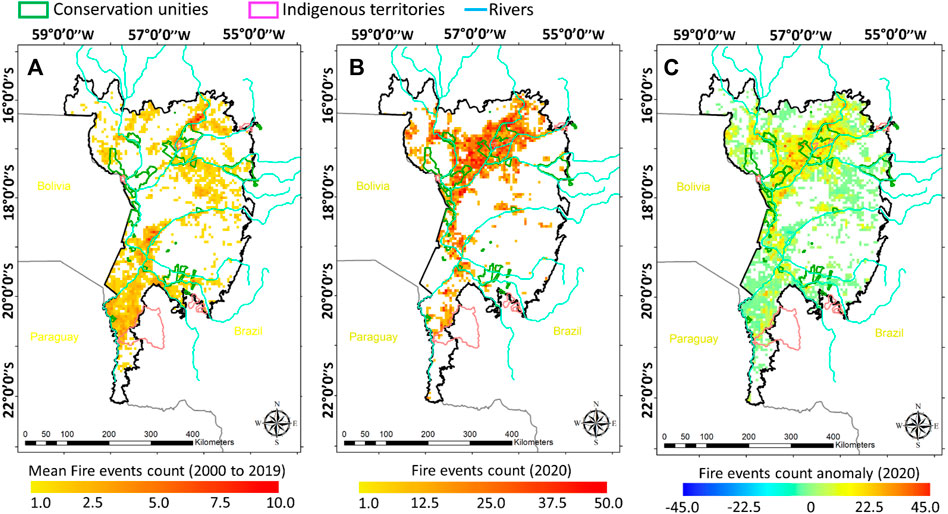
FIGURE 2. Fire outbreak maps. (A) Historical mean fire outbreaks from 2000 to 2019; (B) fire outbreaks in 2020; and (C) 2020 fire outbreak anomaly based on the 2000–2019 mean.
Quantitative Measurements of the Burn Severity in Conservation Units
The quantitative estimations of the burn severity in conservation units are depicted in Figure 3. Among the conservation units, the EST was the least affected by fire. At the EST, most of the western region was preserved. However, the eastern region was greatly affected. According to the burn severity levels defined in Table 1, approximately 56% of the EST area remained unburned, 14% exhibited a low burn severity level, 12% exhibited a moderate-low burn severity level, 16% exhibited a moderate-high burn severity level, and 2% exhibited a high burn severity level. Most of the fires in the NNPPM occurred in the northern/central areas, with 23% of the NNPPM area preserved (unburned), 14% of the area exhibited a low burn severity level, 17% exhibited a moderate-low burn severity level, 42% exhibited a moderate-high burn severity level, and 4% exhibited a high burn severity level. The border region of the GIT was heavily impacted, with few fires in the interior. Fifteen percent of the GIT remained unburned, 28% of the area exhibited a low burn severity level, 26% exhibited a moderate-low burn severity level, 28% exhibited a moderate-high burn severity level, and 3% exhibited a high burn severity level. The southern and western regions of the GIT comprised the most severely burned regions. The SESCP and SNPEA were the conservation units with most of the territory burned, at 93 and 94%, respectively, of the total areas. In the SESCP, only 7.0% of the area remained unburned, 20.7% exhibited a low burn severity level, 33.8% exhibited a moderate-low burn severity level, 36.8% exhibited a moderate-high burn severity level, and 1.7% exhibited a high burn severity level. In the SNPEA, 6% of the area remained unburned, 22.4% of the area exhibited a low burn severity level, 31% exhibited a moderate-low burn severity level, 38% exhibited a moderate-high burn severity level, and 2.6% exhibited a high burn severity level. Although conservation units and indigenous territories impose a known inhibitory effect on deforestation and fires (Nepstad et al., 2006), the 2020 fire demonstrated to be an unprecedented event. For the first time, fire exceeded this barrier and burned most territories. We also determined that until 20–25/08/2020, fire outbreaks occurred along the border of the conservation units and indigenous territories closest to human pathways (roads and waterways) (Supplementary Figure S2). This reflects the human pressure acting on these conservation areas. At the peak of the fire season, from 01–05/09/2020, fire started to spread inside the conservation areas.
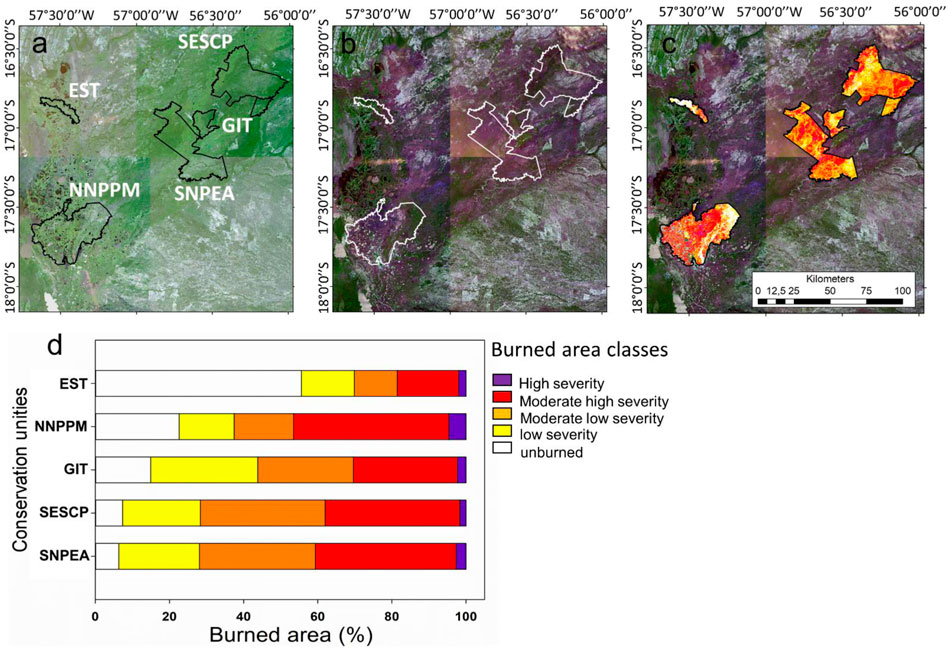
FIGURE 3. Burn severity analysis of the conservation units. (A) Prefire mosaic of Sentinel two MSI true colour images, wherein vegetation appears green; (B) postfire mosaic of Sentinel two MSI true colour images, wherein burned vegetation appears purple; (C) burn severity classification of the conservation units; and (D) quantification of the burn severity in the conservation units.
2020 Drought in the Pantanal and Fire Distribution
Our analysis of the annual mean standard precipitation and evaporation index (SPEI) in the Pantanal from 1970 to 2020 indicated that 2020 was markedly dry (Figure 4A). Water levels were the lowest in the last 45 years (Figure 4B). The northern Pantanal was the most affected by drought (Figure 4C). The SPEI during the 2020 wildfire season ranged from −4.0 in the northern Pantanal area to −1.3 in the southernmost part of the Pantanal, which indicates the different fire sensitivities of these locations.
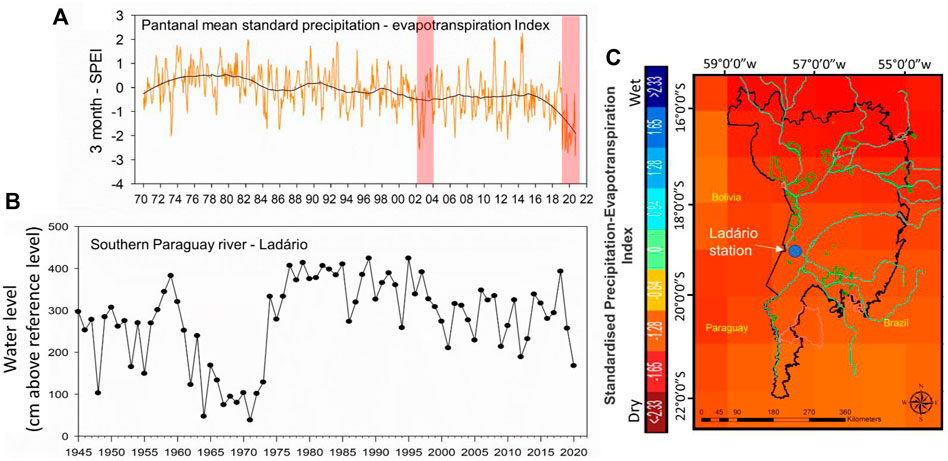
FIGURE 4. Historical droughts in the Pantanal. (A) Integrated 3-months SPEI in the Brazilian Pantanal. Red bands highliths the two most prominent dry periods over the last 50 years, 2002–2004 and 2019–2020. (B) Water level (centimetres above the refence level) at the Ladário station, Paraguay River. (C) Pantanal mean standard precipitation and evaporation index (SPEI) during the 2020 fire season (August-September-October mean).
A comparison of precipitation data pertaining to the Paraguay River basin (Figure 5A) reveals that the two most prominent dry periods over the last 50 years (2002–2004 and 2019–2020), red bands in Figure 4A, exhibited a short wet season (Figure 5B) with a low annual precipitation and, as a consequence, intensification of fire outbreaks occurred (Figure 5C). The main cause of the lack of rainfall during the summers of 2019 and 2020 was a change in the South American monsoon system that reduced seasonal transport of water vapour from the Amazon basin into the Pantanal during the austral summer (Marengo et al., 2021). The reduced accumulated rainfall during the two consecutive summers of 2019 and 2020 caused severe drought conditions, resulting in favourable conditions for fire spread during the fire season in the central-western/northern Pantanal.
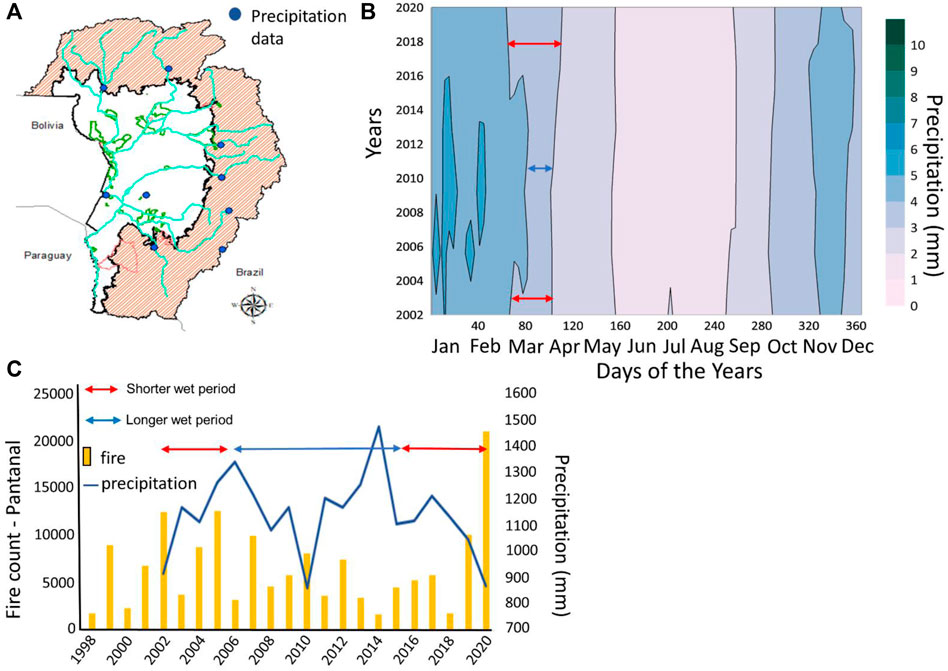
FIGURE 5. Analysis of the precipitation data for the Pantanal. (A) Location of the precipitation monitoring stations. (B) Daily averaged precipitation data from 2002 to 2020. (C) Comparison of fire outbreaks and precipitation in the Brazilian Pantanal.
Data derived from microcharcoal paleoclimatic records in the Amazon basin demonstrate that drier tropical/equatorial climate conditions can lead to an increased risk of wildfires (Bush et al., 2008; Mayle and Power, 2008). Therefore, we would expect that fire outbreaks should be spatially distributed in the Pantanal according to regional drought severity behaviour since vegetation does not differ significantly, with higher frequencies in the central-western/northern Pantanal (the driest region) in 2020. However, we observed a completely different scenario where most fire outbreaks predominantly started close to roads and along waterways (Figure 6A).
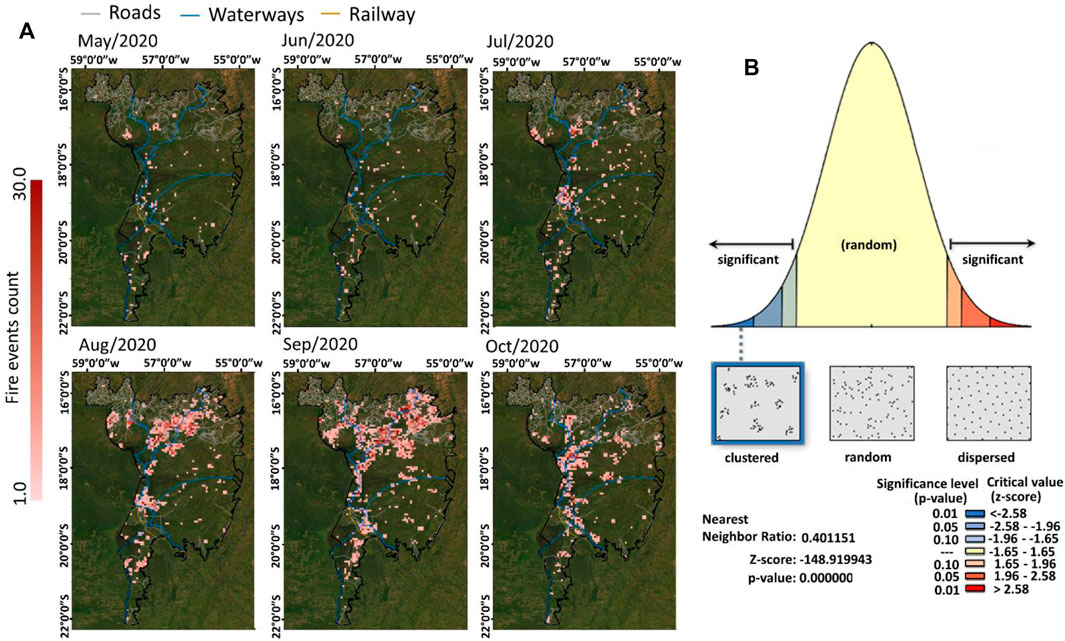
FIGURE 6. Distribution pattern of the fire outbreaks. (A) Monthly fire distribution from May 2020 to October 2020 (data from Brazilian Institute for Space Research-INPE; source: https://queimadas.dgi.inpe.br/queimadas/bdqueimadas) and the locations of roads, waterways, and railways. (B) Result of Average nearest neighbour (ANN) analysis showing a statistically significant clustered pattern in the distribution of fire outbreaks from May 2020 to October 2020.
We analysed the distribution pattern of the fire outbreaks and found a statistically significant clustered distribution (Figure 6B). This result confirms that fire outbreaks were concentrated in hot spot areas, at the termination of roads, and along waterways.
Close Spatial Relationship Between the Fire Outbreaks and Human Accessibility
The finding of a spatial association between the fire outbreaks and roads/waterways/railways in the Pantanal encouraged us to analyse this relationship in greater detail. Thus, we investigated whether the fire outbreaks decayed systematically with the distance from roads, waterways, and railways or whether they occurred randomly. Figure 7 a shows a distance map from roads, waterways, and railways. We also investigated the relationship between the fire outbreaks and SPEI (drought index).
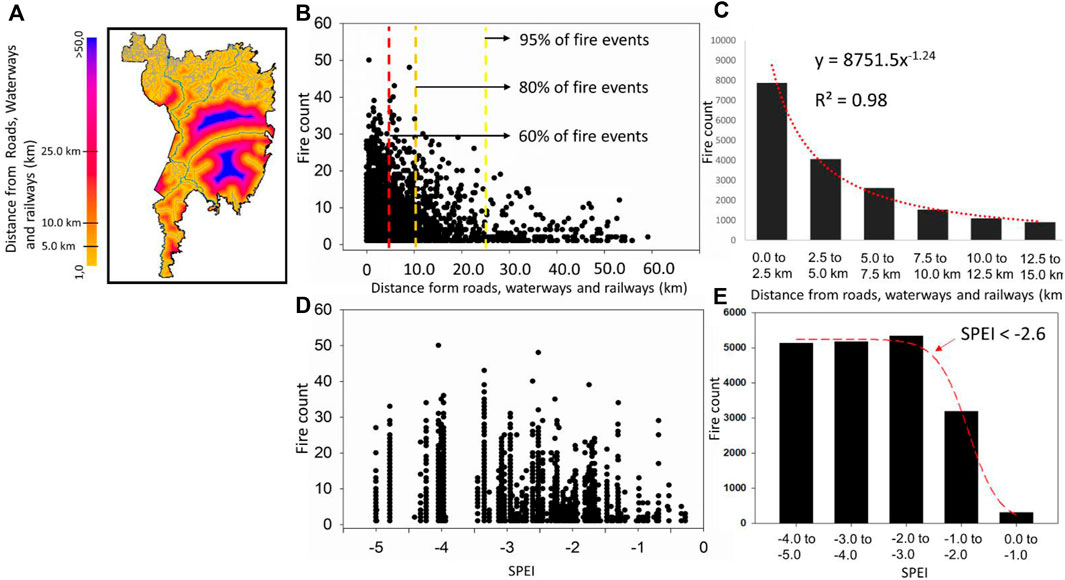
FIGURE 7. Analysis of the relationship between the fire outbreak location, human accessibility, and drought index. (A) Euclidian distance map from roads, waterways, and railways. (B) Scatter plot showing the relationship between each fire outbreak from May 2020 to October 2020 and the distance from roads, waterways, and railways. (C) Exponential model of the fire outbreaks in relation to the distance from roads, waterways, and railways. (D) Scatter plot showing the relationship between each fire outbreak and the monthly SPEI value from May 2020 to October 2020. (E) Histogram showing that most fires occurred at locations with SPEI < -2.6.
From May 2020 to October 2020, 60% of the fire outbreaks occurred less than 5 km from any road/waterway/railway, and 80% occurred at a distance less than 10 km (Figure 7B). The frequency of fire outbreaks and the distance from roads/waterways/railways exhibited a clear association. Notably, the frequency of fire outbreaks increased exponentially with decreasing distance from roads/waterways/railways (r2 = 0.98) (Figure 7C). Regarding the SPEI, we found a rapid increase in fire outbreaks for SPEI values ranging from 0 to −2.6. SPEI < −2.6 was critical to the fire outbreaks during the 2020 wildfire crisis (Figure 7D,E).
Supplementary Figure S3 depicts the relationship between the fire outbreaks, roads/waterways/railways and SPEI for each month from May to October (beginning of the fire in the Pantanal to the end of the fire season). At the beginning of the fire season (May/June/July), most fire outbreaks started close (at a distance less than 5 km) to roads/waterways/railways, the SPEI values were lower than the critical value of < −2.6, and the SPEI value ranged from −2.5 to −1.0 (Supplementary Figure S3A,B,C, respectively). From August to October, the SPEI values became more negative, the number of fire outbreaks increased, and most fire outbreaks remained close to roads/waterways/railways (Supplementary Figure S3D,E,F, respectively). This association was analysed in greater detail by reducing (increasing) the temporal (spatial) scale. We considered periods of 5 days from August 20 to September 15 because that was the critical period for fire spread occurrence (Supplementary Figure S2) and a maximum distance of 10 km (80% of the fire events occurred within this distance). Under this scenario, the association between fire and distance from roads and waterways was also evident (Supplementary Figure 4). The number of fire outbreaks/km2 and the distance from the roads and waterways exhibited statistically significant correlations (statistical significance was based on Student’s t test, for p < 0.001, and n = 20, where we found r = −0.77, r = −0.88, r = −0.80, and r = −0.94 for the periods from 20–25/08/2020, 01–05/09/2020, 5–10/09/2020 and 10–15/09/2020, respectively) (Supplementary Figure S5). Even at the peak of the fire season, from 10–15 September, when the Brazilian Center for Weather Forecasting and Climate Studies/National Institute for Space Research (CPTEC/INPE) determined that the whole Pantanal occurred at a critical risk of fire (Supplementary Figure S6), most fire outbreaks were concentrated close to roads and waterways (Supplementary Figure S4D), and the distance from roads and waterways explained 90% of the fire outbreak variance (R2 = 0.9). This result provides clear evidence that the majority of fires was caused by human activities close to roads and waterways.
Spatial-Temporal Trends of the Fire Outbreaks and Burned Areas
We employed a spatiotemporal implementation of the Getis-Ord Gi* statistic to analyse the spatial and temporal patterns of wildfire outbreak hot spots in the Pantanal from 2000–2020 (Figure 8). We found three statistically significant patterns: 1) new hot spots, i.e., regions with a very low frequency of wildfire outbreaks from 2000 to 2019 and a high increase in fire outbreaks in 2020; 2) consecutive hot spots, i.e., regions with a very low frequency of fire outbreaks from 2000 to 2018 and a high increase in fire outbreaks over the last 2 years (2019 and 2020); and 3) sporadic hot spots, i.e., regions with a high frequency of wildfire outbreaks throughout the whole period from 2000 to 2020 with high variability. The Pantanal regions not classified into any of these three patterns did not exhibit statistically significant trends. Consecutive hot spots and sporadic hot spots occurred in regions close to roads and waterways. This demonstrates that most regions where fire occurs each year occur close to roads and waterways. This result confirms the hypothesis that fires in the Pantanal cannot be attributed to natural causes. Fire outbreak is triggered by human activity and enhanced when favourable dry climate regimes are prevalent. New fire hot spot regions were observed mainly close to roads and waterways. These finds corroborate with recent work, which shows that only 16% of burned area in the Pantanal can be atributed to natural causes (ligthning) (Libonati et al., 2022).
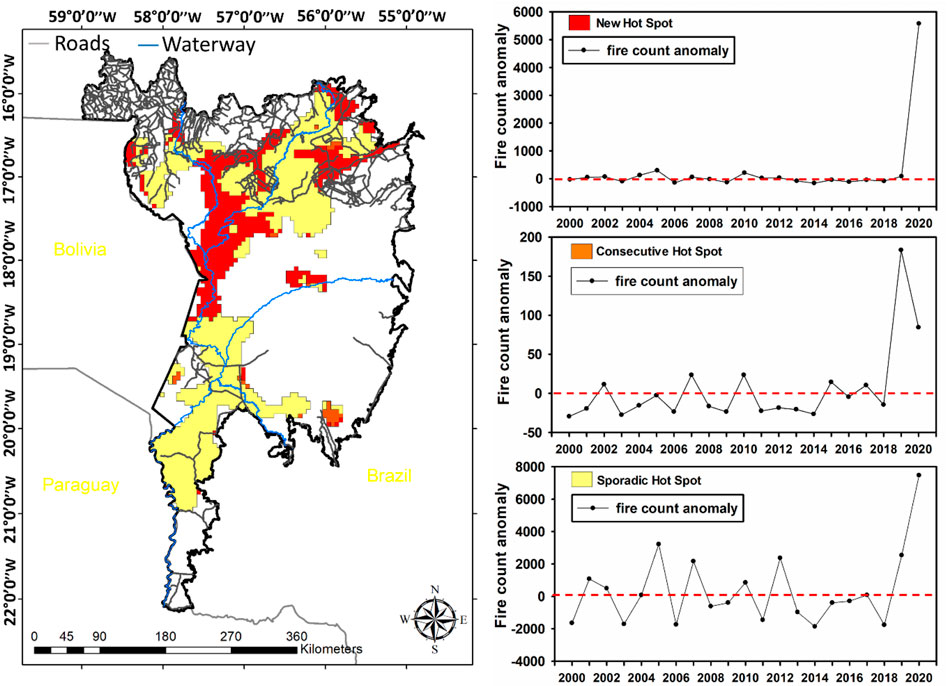
FIGURE 8. Spatial and temporal patterns of fire outbreak hot spots in the Pantanal from 2000–2020. The red areas are classified as new hot spots, the orange areas are classified as consecutive hot spots, and the yellow areas are classified as sporadic hot spots.
As a complement to this fire outbreak study, we also analysed the burned area parameter (a product of the MapBiomas programme (Souza et al., 2020; Arruda et al., 2021)) over the period from 2000 to 2020 at an interannual resolution. We observed that these two parameters exhibited significant covariance (r2 = 0.93, Supplementary Figure S7).
Maps of the fire scar density were generated for the periods from 2000–2004, 2005–2009, 2010–2014, 2015–2019, and 2020 (Supplementary Figure S8). The results show that during the period from 2000–2004, the areas close to roads and waterways and the eastern region/interior of the Pantanal exhibited a high density of fire scars (Supplementary Figure S7A). During the following periods, the density of fire scars decreased in the eastern region/interior of the Pantanal, and fires were concentrated in regions with roads and waterways in the northern and southern parts of the Pantanal (Supplementary Figure S8B,C,D,E). The eastern/interior region of the Pantanal is where deforestation and pasture expansion were concentrated (Guerra et al., 2020b), which indicates that these activities are related to fire occurrence during the period from 2000–2004. From 2005 onwards, the loss of forest cover and pasture expansion decreased (Figure 9C), and the density of fire scars in the eastern region/interior of the Pantanal also decreased (Supplementary Figure S8). The burned area in the Pantanal from 2000 to 2020 attained a significant correlation with the SPEI (r = - 0.59) and forest formation loss (r = - 0.48 for forest and savanna formation) (Supplementary Figure S9). The linear combination of the independent variables of the SPEI and forest loss explained 55% of the burned area variance (t test: for partial coefficients, p < 0.01; for the full model, F21 = 10.99, p < 0.001; r2 = 0.55) (Supplementary Figure S10). This indicates that fire is associated with both land use/cover change and climate conditions, corroborating with recent study that depicts a synergy between climate, land management and fire, in the Pantanal (Kumar et al., 2022).
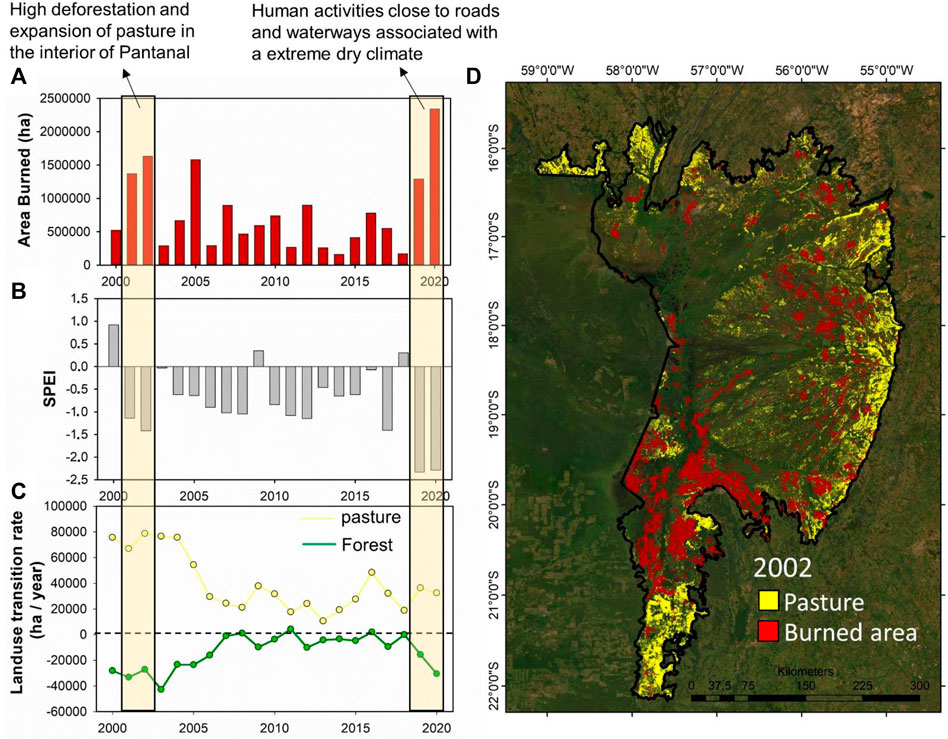
FIGURE 9. Comparison of the burned areas between 2001–2002 and 2019–2020. (A) Interannual variability in the total burned area in the Pantanal. (B) Interannual variability in the SPEI during the fire seasons (August-September-October) in the Pantanal. (C) Interannual variability in the land use transition rates between forestland and pasture areas in the Pantanal (positive values indicate gains, and negative values indicate loss). (D) Pasture and burned areas in 2002.
The years of 2002 and 2020 were compared. Both years indicated an increase in the burned area (Figure 9A), and the climate was dry and preceded by a dry year (Figure 9B). Of these years, 2002 exhibited high deforestation and expansion of pasture areas (Figure 9C), and a high density of fire scars was observed in the eastern region/interior of the Pantanal (Figure 9D). However, in 2020, the expansion of pastures occurred less intensely (Figure 9C), and fires were concentrated close to roads and waterways.
The deforestation rate in the Pantanal, as well as the expansion of pasture areas, decreased with the creation of State Law N° 233/2005 (Supplementary Figure S11), which created rules for deforestation, use of the forest, and sustainable use of fire. This law was formulated 1 year after the creation of the National Plan for the Prevention and Control of Deforestation in the Legal Amazon (PPCDAm) in 2004, which marked the intensification of environmental and economic policies against deforestation (e.g., creation of monitoring programmes, intensification of inspections, law enforcement campaigns, and credit restrictions for landowners participating in illegal deforestation) in the Amazon Forest and other biomes (Moutinho et al., 2016). It was pustuled that reduction in inspectors of the Brazilian environmental protection agency (IBAMA) and cuts in the budget allocated to hiring and training firefighters contributed to the severity of 2020 fires (Libonati et al., 2020; Filho et al., 2021). In this context, our analysis show how intensification of environmental and economic policies against deforestation can be important to avoid fire.
Burned Area, Vegetation Loss, and Pasture Expansion
The 2020 drought was the worst since the 1970s. As a result, river levels dropped, and wetlands dried, resulting in dry organic matter available as fuel for fire. Comparing the 2019 and 2020 land use and cover maps, it can be observed that fires spread in areas that were wetlands in 2019, but in 2020, these areas were classified as grasslands (probably dry aquatic vegetation as macrophytes) (Figure 10). Grassland was the natural vegetation type that burned the most in 2020 (60% of the area burned), followed by natural forest formation (15% of the area burned) and savanna (14% of the area burned) (Supplementary Figure S12). However, the increase in the burned forested area was the greatest over the historical average from 2000–2019 (an increase of ∼677%).
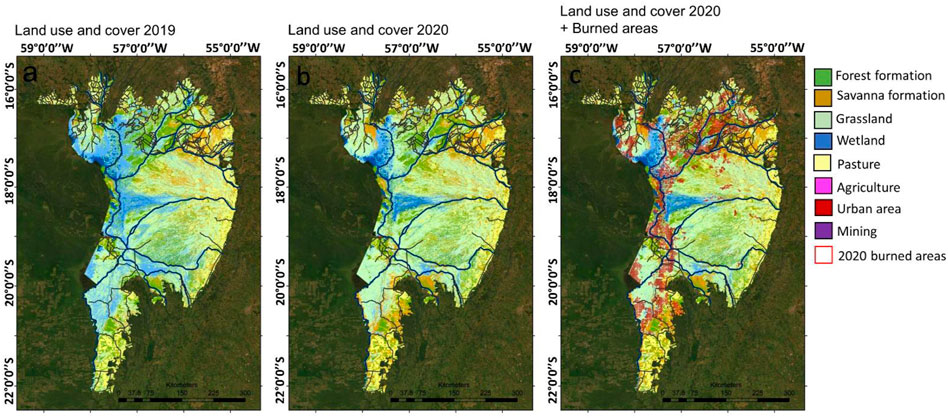
FIGURE 10. Land use and cover changes from 2019 to 2020 and burned areas in 2020. (A) Land use and cover map for 2019. (B) Land use and cover map for 2020. (C) Total burned area in 2020 overlaid on the land use and cover map for 2020.
From 1985 to 2020, the Pantanal lost 21.8% of the forest cover, 35.2% of the savanna cover, and 28.7% of the grassland cover. All the vegetation classes were mostly converted into pasture (82.7% of the forest loss, 62.9% of the savanna loss, and 66.8% of the grassland loss) (Supplementary Figure S13). In 2020, forest loss reached ∼30,623 ha, equalling the forest loss during the period from 2000–2004. As most forest vegetation was historically converted into pasture, it is expected that the burned forest area will be converted into pasture, except in regions where floods usually occur and in protected areas (conservation units and indigenous lands). This makes the expansion or creation of new conservation units even more urgent. The fate of burned vegetation outside conservation units remains uncertain. However, land-use change models point to an increase in agricultural and pasture areas in the north of the Pantanal, where most fires occurred (Guerra et al., 2020b).
Integrated Discussion
Herein we demonstrated that human accessibility played a crucial role in explaining fire spatial pattern during the 2020 fire crisis in the Pantanal. The relation between human accessibility and fire location is a strong evidence that human actions (accidental or criminal) started the 2020 fire in the Pantanal. The fire started close to roads and rivers where human accessibility is more viable, and outside conservation unities showing their protective role. We showed that fire occurrence increased exponentially with decreasing distance from roads and waterways. About 80% of fires were located within 10 km from existing roads and waterways. Similar relation between fire and human transportation networks were found in the Amazon Forest, where ∼90% of fires occur within 10 km from roads and rivers (Adeney et al., 2009). In the Amazon, roads are the major conduits for deforestation and accompanying fires (Nepstad et al., 2001; Carvalho et al., 2002; Kirby et al., 2006; Fearnside, 2007; Adeney et al., 2009). Our results suggest that a similar process is on course in the Pantanal. We found that drought and deforestation explained 55% of the burned area variance. This indicates that fire is associated with human accessibility, land use/cover change and climate conditions, corroborating with recent study that depicts a synergy between climate, land management and fire in the Pantanal (Kumar et al., 2022). The lack of rainfall during the summers of 2019 and 2020, caused by reduced transport of warm and humid summer air from Amazonia (Marengo et al., 2021), and numerous heat waves episodes over the Pantanal in 2020 (Libonati et al., 2022), created favorable conditions for fire to spread. Fires were more intense in the northern forested areas, revealing a synergistic effect between fuel availability and weather-hydrological conditions (Libonati et al., 2022). Changes in land use also contributed to exacerbate the drought effects (Kumar et al., 2022). It is possible that what we observed in the Pantanal was a positive feedback where human induced changes on land use aggravates drought, resulting in more fires that burn natural vegetated areas causing vegetation loss, witch amplify drought and makes the environment more prone to mega fires. Considering the fire ignition process, our work shows clear evidence that the fires were started by humans and exarcebated by the worst drought since the 70s. Future scenarios show that Amazon and Pantanal will become dryer and fire might become the dominant driver of forest degradation (Brando et al., 2020).
Some conservation unities had more than 80% of the total area burned. The recovery of this areas is a matter of concern. Recent assessing shows that about 93% of burned areas in the Pantanal have high or medium potential for natural regeneration (Martins et al., 2022). Considering the history of land use change in the Pantanal, most of deforested areas have been occupied by pasture (Bergier, 2013; Galvanin et al., 2019). Also high concentration of fire outbreaks were observed adjacent to the agricultural Frontier (Marques et al., 2021), which is an indicator that fire has been used for deforestation and agriculture expansion. Thus, in order to recover burned natural habitats, it is important to monitor burned areas and create new conservation unities to avoid human induced land use change (expansion of pasture and other economic activities).
Although Conservation unities and Indigenous territories do not provide absolute protection (Adeney et al., 2009), and were almost totally burned during the 2020 wildfire, they have a recognizable role in forest/habitat preservation and fire inhibition (Nepstad et al., 2006; Chaves and Silva, 2018). Therefore they can guarantee that burned areas will not be occupied, thus allowing forest natural recovery process.
An integrated fire management program taking into account scientific knowledge, and socio-ecological context is urgently needed to avoid new fire crisis (Damasceno-Junior et al., 2021; Garcia et al., 2021). In spite of the fact that the proximity between fire and roads facilitate acidental/criminal fire ignition, it also allows the necessary accessibility for fire combat. It is necessary permanent fire brigades, including indigenous members (Oliveira et al., 2022), and a collaborative network for wildfire prevention (Pivello et al., 2021). Monitoring drought regions close to human pathways using high resolution climate models and remote sensing data are also an important tool for fire prevention, fightning and rescue initiatives (Higa et al., 2022).
Conclusion
Most impacted conservation units had more than 80% of their area burned and exhibited moderate low-high burn severity. The 2020 unprecedented fire crisis in the Pantanal was caused by the combination of human presence, represented by accessibility through roads and waterways, and an extremely dry climate. Human activities near roads and waterways triggered this fire event, while a dryer climate episode provided conditions for the fire to spread.
It is not known whether the 2019–2020 dry events in the Pantanal reflect short-term climate variability or will last for many years similar to the 11-years drought from 1962/1963 to 1972/1973. Pantanal wildfires are directly linked to the Amazon basin. It is expected that changes could occur in the Amazon hydrological cycle as a response to progressive and accelerated deforestation of the rainforest (Nobre et al., 2016), and thereafter, the Pantanal region could be subject to a higher risk of prolonged droughts in the future. Although prolonged drought contributes to fire spreading, recent work has determined that the fire season severity in the Amazon depends more on deforestation than on climate change (Libonati et al., 2021). We demonstrated that in the Pantanal Biome, human activities close to roads and waterways were the determining factor. Therefore, preventing wildfires in terms of the human component through environmental policies (e.g., hiring more inspectors and firefighters, intensifying deforestation inhibition, amplification and creation of conservation units, investment in new agricultural technologies that do not require the use of fire, and environmental education) are vital to Pantanal preservation.
Data Availability Statement
The original contributions presented in the study are included in the article/supplementary material, further inquiries can be directed to the corresponding author.
Author Contributions
NMN, Conceptualization, Methodology, Formal analysis, Investigation, data curation, Writing Original Draft—Review and Editing; HE, Conceptualization, Methodology, formal analysis, Supervision, writing—review and editing. All authors have read and agreed to the published version of the manuscript.
Conflict of Interest
The authors declare that the research was conducted in the absence of any commercial or financial relationships that could be construed as a potential conflict of interest.
Publisher’s Note
All claims expressed in this article are solely those of the authors and do not necessarily represent those of their affiliated organizations, or those of the publisher, the editors and the reviewers. Any product that may be evaluated in this article, or claim that may be made by its manufacturer, is not guaranteed or endorsed by the publisher.
Acknowledgments
We greatly thank Rio de Janeiro State University (UERJ) for support, the Brazilian Space Research Institute (INPE) and the MapBiomas project for the fire database.
Supplementary Material
The Supplementary Material for this article can be found online at: https://www.frontiersin.org/articles/10.3389/fenvs.2022.888578/full#supplementary-material
References
Adeney, J. M., Christensen, N. L., and Pimm, S. L. (2009). Reserves Protect against Deforestation Fires in the Amazon. PLOS ONE 4 (4), e5014. doi:10.1371/journal.pone.0005014
Arruda, V. L. S., Piontekowski, V. J., Alencar, A., Pereira, R. S., and Matricardi, E. A. T. (2021). An Alternative Approach for Mapping Burn Scars Using Landsat Imagery, Google Earth Engine, and Deep Learning in the Brazilian Savanna. Remote Sens. Appl. Soc. Environ. 22, 100472. doi:10.1016/j.rsase.2021.100472
Bergier, I. (2013). Effects of Highland Land-Use over Lowlands of the Brazilian Pantanal. Sci. Total Environ. 463-464, 1060–1066. doi:10.1016/j.scitotenv.2013.06.036
Brando, P. M., Soares-Filho, B., Rodrigues, L., Assunção, A., Morton, D., Tuchschneider, D., et al. (2020). The Gathering Firestorm in Southern Amazonia. Sci. Adv. 6, eaay1632. doi:10.1126/sciadv.aay1632
Bush, M. B., Silman, M. R., McMichael, C., and Saatchi, S. (2008). Fire, Climate Change and Biodiversity in Amazonia: A Late-Holocene Perspective. Phil. Trans. R. Soc. B 363 (1498), 1795–1802. doi:10.1098/rstb.2007.0014
Carvalho, G. O., Nepstad, D., McGrath, D., Diaz, M. D. V., Santilli, M., and Barros, A. C. (2002). Frontier Expansion in the Amazon: Balancing Development and Sustainability. Environ. Sci. Policy Sustain. Dev. 44, 34–44. doi:10.1080/00139150209605606
Chaves, J. V. B., and Silva, J. d. S. V. d. (2018). “Evolução das unidades de conservação no Pantanal no período de 1998 a 2018,” in Anais 7º Simpósio de Geotecnologias no Pantanal, Jardim, MS, 20 a 24 de outubro 2018 Embrapa Informática Agropecuária/INPE, 676
Clark, P. J., and Evans, F. C. (1954). Distance to Nearest Neighbor as a Measure of Spatial Relationships in Populations. Ecology 35, 445–453. doi:10.2307/1931034
Convention on Biological diversity (2004). Convention on Biological Diversity. NY, USA: CBD, Programme of Work for Protected Areas.
Cordeiro, R. C., Turcq, B., Moreira, L. S., Rodrigues, R. d. A. R., Filho, F. F. L. S., Martins, G. S., et al. (2014). Palaeofires in Amazon: Interplay between Land Use Change and Palaeoclimatic Events. Palaeogeogr. Palaeoclimatol. Palaeoecol. 415, 137–151. doi:10.1016/j.palaeo.2014.07.020
Costanza, R., d'Arge, R., de Groot, R., Farber, S., Grasso, M., Hannon, B., et al. (1997). The Value of the World's Ecosystem Services and Natural Capital. Nature 387, 253–260. doi:10.1038/387253a0
Coutinho, L. M. (1990). “Fire in the Ecology of the Brazilian Cerrado,” in Fire in the Tropical Biota: Ecological Processes and Global Challenges. Ecological Studies. Editor J. G. Goldammer (Berlin: Springer-Verlang), 82–105. doi:10.1007/978-3-642-75395-4_6
Damasceno-Junior, G. A., Roque, F. d. O., Garcia, L. C., Ribeiro, D. B., Tomas, W. M., Scremin-Dias, E., et al. (2021). Lessons to Be Learned from the Wildfire Catastrophe of 2020 in the Pantanal Wetland. Wetl. Sci. Pract. 38, 107
ESRI (2016). How Emerging Hot Spot Analysis Works. Available from https://pro.arcgis.com/en/pro-app/latest/tool-reference/space-time-pattern-mining/learnmoreemerging.htm (accessed 10 April 2021).
Fearnside, P. M. (2007). Brazil's Cuiabá- Santarém (BR-163) Highway: The Environmental Cost of Paving a Soybean Corridor through the Amazon. Environ. Manag. 39, 601–614. doi:10.1007/s00267-006-0149-2
Filho, W. L., Azeiteiro, U. M., Salvia, A. L., Fritzen, B., and Libonati, R. (2021). Fire in Paradise: Why the Pantanal Is Burning. Environ. Sci. policy 123, 31–34. doi:10.1016/j.envsci.2021.05.005
Galvanin, E. A. S., Menezes, R., Pereira, M. H. X., and Neves, S. M. A. S. (2019). Mixed-effects Modeling for Analyzing Land Use Change in the Brazilian Pantanal Subregion of Cáceres. Remote Sens. Appl. Soc. Environ. 13, 408–414. doi:10.1016/j.rsase.2018.12.008
Garcia, L. C., Szabo, J. K., Roque, F. d. O., Pereira, A. d. M. M., da Cunha, C. N., Damasceno-Júnior, G. A., et al. (2021). Record-breaking Wildfires in the World's Largest Continuous Tropical Wetland: Integrative Fire Management Is Urgently Needed for Both Biodiversity and Humans. J. Environ. Manag. 293, 112870. doi:10.1016/j.jenvman.2021.112870
Giglio, L., Schroeder, W., and Justice, C. O. (2016). The Collection 6 MODIS Active Fire Detection Algorithm and Fire Products. Remote Sens. Environ. 178, 31–41. doi:10.1016/j.rse.2016.02.054
Gouveia, S. E. M., Pessenda, L. C. R., Aravena, R., Boulet, R., Scheel-Ybert, R., Bendassoli, J. A., et al. (2002). Carbon Isotopes in Charcoal and Soils in Studies of Paleovegetation and Climate Changes during the Late Pleistocene and the Holocene in the Southeast and Centerwest Regions of Brazil. Glob. Planet. Change 33, 95–106. doi:10.1016/s0921-8181(02)00064-4
Guerra, A., Oliveira, P. T. S. d., Roque, F. d. O., Rosa, I. M. D., Ochoa-Quintero, J. M., Guariento, R. D., et al. (2020). The Importance of Legal Reserves for Protecting the Pantanal Biome and Preventing Agricultural Losses. J. Environ. Manag. 260, 110128. doi:10.1016/j.jenvman.2020.110128
Guerra, A., Roque, F. d. O., Garcia, L. C., Ochoa-Quintero, J. M., Oliveira, P. T. S. d., Guariento, R. D., et al. (2020). Drivers and Projections of Vegetation Loss in the Pantanal and Surrounding Ecosystems. Land Use Policy 91, 104388. doi:10.1016/j.landusepol.2019.104388
Harris, N. L., Goldman, E., Gabris, C., Nordling, J., Minnemeyer, S., Ansari, S., et al. (2017). Using Spatial Statistics to Identify Emerging Hot Spots of Forest Loss. Environ. Res. Lett. 12 (2), 024012. doi:10.1088/1748-9326/aa5a2f
Higa, L., Marcato Junior, J., Rodrigues, T., Zamboni, P., Silva, R., Almeida, L., et al. (2022). Active Fire Mapping on Brazilian Pantanal Based on Deep Learning and CBERS 04A Imagery. Remote Sens. 14, 688. doi:10.3390/rs14030688
INPE - Instituto Nacional de Pesquisas Espaciais (2021). Portal Do Monitoramento de Queimadas e Incêndios (Brazilian National Institute for Space Research - Wildfires monitoring program). Available at: http://www.inpe.br/queimadas.
Kirby, K. R., Laurance, W. F., Albernaz, A. K., Schroth, G., Fearnside, P. M., Bergen, S., et al. (2006). The Future of Deforestation in the Brazilian Amazon. Futures 38, 432–453. doi:10.1016/j.futures.2005.07.011
Kumar, S., Getirana, A., Libonati, R., Hain, C., Mahanama, S., and Andela, N. (2022). Changes in Land Use Enhance the Sensitivity of Tropical Ecosystems to Fire-Climate Extremes. Sci. Rep. 12, 964. doi:10.1038/s41598-022-05130-0
Libonati, R., DaCamara, C. C., Peres, L. F., Sander de Carvalho, L. A., and Garcia, L. C. (2020). Rescue Brazil’s Burning Pantanal Wetlands. Nature 588, 217–219. doi:10.1038/d41586-020-03464-1
Libonati, R., Geirinhas, J. R., Silva, P. S., Russo, A., Rodrigues, J. A., Belem, L. B. C., et al. (2022). Assessing the Role of Compound Drought and Heatwave Events on Unprecedented 2020 Wildfires in the Pantanal. Environ. Res. Lett. 17, 015005. doi:10.1088/1748-9326/ac462e
Libonati, R., Pereira, J. M. C., Da Camara, C. C., Peres, L. F., Oom, D., Rodrigues, J. A., et al. (2021). Twenty-first Century Droughts Have Not Increasingly Exacerbated Fire Season Severity in the Brazilian Amazon. Sci. Rep. 11, 4400. doi:10.1038/s41598-021-82158-8
Lutes, D. C., Keane, R. E., Caratti, J. F., Key, C. H., Benson, N. C., Sutherland, S., et al. (2006). FIREMON: Fire Effects Monitoring and Inventory System. Gen. Tech. Rep. RMRS-GTR-164 (Fort Collins, CO: U.S. Department of Agriculture, Forest Service, Rocky Mountain Research Station. 1 CD). doi:10.2737/rmrs-gtr-164
Marengo, J. A., Alves, L. M., and Torres, R. R. (2015). Regional Climate Change Scenarios in the Brazilian Pantanal Watershed. Clim. Res. 68, 201–213. doi:10.3354/cr01324
Marengo, J. A., Cunha, A. P., Cuartas, L. A., Deusdará Leal, K. R., Broedel, E., Seluchi, M. E., et al. (2021). Extreme Drought in the Brazilian Pantanal in 2019-2020: Characterization, Causes, and Impacts. Front. Water 3, 13. doi:10.3389/frwa.2021.639204
Marques, J. F., Alves, M. B., Silveira, C. F., e Silva, A. A., Silva, T. A., dos Santos, V. J., et al. (2021). Fires Dynamics in the Pantanal: Impacts of Anthropogenic Activities and Climate Change. J. Environ. Manag. 299, 113586. doi:10.1016/j.jenvman.2021.113586
Martins, P. I., Belém, L. B. C., Szabo, J. K., Libonati, R., and Garcia, L. C. (2022). Prioritising Areas for Wildfire Prevention and Post-fire Restoration in the Brazilian Pantanal. Ecol. Eng. 176, 1–9. doi:10.1016/j.ecoleng.2021.106517
Mayle, F. E., and Power, M. J. (2008). Impact of a Drier Early-Mid-Holocene Climate upon Amazonian Forests. Phil. Trans. R. Soc. B 363 (1498), 1829–1838. doi:10.1098/rstb.2007.0019
Menezes, L. S., de Oliveira, A. M., Filippe, L., Santos, M., Russo, A., Rodrigo, A., et al. (2022). Lightning Patterns in the Pantanal: Untangling Natural and Anthropogenic-Induced Wildfires. Sci. Total Environ. 820, 153021. doi:10.1016/j.scitotenv.2022.153021
Moutinho, P., Guerra, R., and Azevedo-Ramos, C. (2016). Achieving Zero Deforestation in the Brazilian Amazon: What Is Missing? Elem. Sci. Anthropocene 4:100125. doi:10.12952/journal.elementa.000125
Nepstad, D., Carvalho, G., Barros, A. C., Alencar, A., Capobianco, J. P., Bishop, J., et al. (2001). Road Paving, Fire Regime Feedbacks, and the Future of Amazon Forests. For. Ecol. Manag. 154, 395–407. doi:10.1016/s0378-1127(01)00511-4
Nepstad, D., Schwartzman, S., Bamberger, B., Santilli, M., Ray, D., Schlesinger, P., et al. (2006). Inhibition of Amazon Deforestation and Fire by Parks and Indigenous Lands. Conserv. Biol. 20 (1), 65–73. doi:10.1111/j.1523-1739.2006.00351.x
Nobre, C. A., Sampaio, G., Borma, L. S., Castilla-Rubio, J. C., Silva, J. S., and Cardoso, M. (2016). Land-use and Climate Change Risks in the Amazon and the Need of a Novel Sustainable Development Paradigm. Proc. Natl. Acad. Sci. U.S.A. 113, 10759–10768. doi:10.1073/pnas.1605516113
Oliveira, M. R., Ferreira, B. H. S., Souza, E. B., Lopes, A. A., Bolzan, F. P., Roque, F. O., et al. (2022). Indigenous Brigades Change the Spatial Patterns of Wildfires, and the Influence of Climate on Fire Regimes. J. Appl. Ecol. 59(5):1279–1290. doi:10.1111/1365-2664.14139
Parks, S., Dillon, G., and Miller, C. (2014). A New Metric for Quantifying Burn Severity: The Relativized Burn Ratio. Remote Sens. 6, 1827–1844. doi:10.3390/rs6031827
Pivello, V. R., Vieira, I., Christianini, A. V., Ribeiro, D. B., Menezes, L. d. S., Berlinck, C. N., et al. (2021). Understanding Brazil's Catastrophic Fires: Causes, Consequences and Policy Needed to Prevent Future Tragedies. Perspect. Ecol. conservation 19, 233–255. doi:10.1016/j.pecon.2021.06.005
Russi, D., ten Brink, P., Farmer, A., Badura, T., Coates, D., Förster, J., et al. (2013). The Economics of Ecosystems and Biodiversity for Water and Wetlands. London and Brussels: IEEP. Ramsar Secretariat: Gland.
Saulino, L., Rita, A., Migliozzi, A., Maffei, C., Allevato, E., Garonna, A. P., et al. (2020). Detecting Burn Severity across Mediterranean Forest Types by Coupling Medium-Spatial Resolution Satellite Imagery and Field Data. Remote Sens. 12, 741. doi:10.3390/rs12040741
Schroeder, W., Prins, E., Giglio, L., Csiszar, I., Schmidt, C., Morisette, J., et al. (2008). Validation of GOES and MODIS Active Fire Detection Products Using ASTER and ETM+ Data. Remote Sens. Environ. 112 (5), 2711–2726. doi:10.1016/j.rse.2008.01.005
Seidl, A. F., Silva, J. d. S. V. d., and Moraes, A. S. (2001). Cattle Ranching and Deforestation in the Brazilian Pantanal. Ecol. Econ. 36, 413–425. doi:10.1016/s0921-8009(00)00238-x
Souza, C. M., Shimbo, J. Z., Rosa, M. R., Parente, L. L., Alencar, A. A., Rudorff, B. F. T., et al. (2020). Reconstructing Three Decades of Land Use and Land Cover Changes in Brazilian Biomes with Landsat Archive and Earth Engine. Remote Sens. 12, 2735. doi:10.3390/rs12172735
Tomas, W. M., Berlinck, C. N., Chiaravalloti, R. M., Faggioni, G. P., Strüssmann, C., Libonati, R., et al. (2021). Distance Sampling Surveys Reveal 17 Million Vertebrates Directly Killed by the 2020's Wildfires in the Pantanal, Brazil. Sci. Rep. 11, 23547. doi:10.1038/s41598-021-02844-5
Vicente-Serrano, S. M., Beguería, S., and López-Moreno, J. I. (2010). A Multiscalar Drought Index Sensitive to Global Warming: The Standardized Precipitation Evapotranspiration Index, J. Clim., 23(7), 1696–1718. doi:10.1175/2009JCLI2909.1
Tomas, W. M., Roque, F. d. O., Morato, R. G., Medici, P. E., Chiaravalloti, R. M., Tortato, F. R., et al. (2019). Sustainability Agenda for the Pantanal Wetland: Perspectives on a Collaborative Interface for Science, Policy, and Decision-Making. Trop. Conservation Sci., 12, 194008291987263. doi:10.1177/1940082919872634
Keywords: burn severity, extreme fire event, drought, climate change, land use/cover change
Citation: de Magalhães Neto N and Evangelista H (2022) Human Activity Behind the Unprecedented 2020 Wildfire in Brazilian Wetlands (Pantanal). Front. Environ. Sci. 10:888578. doi: 10.3389/fenvs.2022.888578
Received: 03 March 2022; Accepted: 25 May 2022;
Published: 15 June 2022.
Edited by:
Christoph Rüdiger, Bureau of Meteorology, AustraliaReviewed by:
Pratheesh C. Mammen, Kerala State Disaster Management Authority (SDMA), IndiaFrancis K. Dwomoh, Earth Resources Observation and Science (EROS) Center, United States
Artan Hysa, Epoka University, Albania
Copyright © 2022 de Magalhães Neto and Evangelista. This is an open-access article distributed under the terms of the Creative Commons Attribution License (CC BY). The use, distribution or reproduction in other forums is permitted, provided the original author(s) and the copyright owner(s) are credited and that the original publication in this journal is cited, in accordance with accepted academic practice. No use, distribution or reproduction is permitted which does not comply with these terms.
*Correspondence: Newton de Magalhães Neto, bmV3dG9uLm1hZ2FsaGFlcy5uZXRvQHVlcmouYnI=